- 1Southeastern Cooperative Wildlife Disease Study, Department of Population Health, College of Veterinary Medicine, University of Georgia, Athens, GA, United States
- 2Center for the Ecology of Infectious Diseases, Odum School of Ecology, University of Georgia, Athens, GA, United States
- 3Agriculture and Agri-Food Canada, Lethbridge Research and Development Centre, Lethbridge, AB, Canada
- 4Laboratory of Wildlife Medicine, Nippon Veterinary and Life Science University, Musashino, Japan
- 5New York State Department of Health, Louis Calder Center, Fordham University, Armonk, NY, United States
- 6Vector and Waterborne Pathogens Research Group, College of Science, Health, Engineering and Education, Murdoch University, Murdoch, WA, Australia
- 7Public Health Agency of Canada, National Microbiology Laboratory, Winnipeg, MB, Canada
- 8Key Laboratory of Animal Physiology, Biochemistry and Molecular Biology of Hebei Province, College of Life Sciences, Hebei Normal University, Shijiazhuang, China
- 9Center for Vector-Borne Diseases, University of Rhode Island, Kingston, RI, United States
- 10Campus of Biology and Agricultural Sciences, Department of Veterinary Medicine and Animal Husbandry, National Autonomous University of Yucatan, Merida, Mexico
- 11Warnell School of Forestry and Natural Resources, University of Georgia, Athens, GA, United States
Haemaphysalis longicornis (Acari: Ixodidae), the Asian longhorned tick, is native to East Asia, but has become established in Australia and New Zealand, and more recently in the United States. In North America, there are other native Haemaphysalis species that share similar morphological characteristics and can be difficult to identify if the specimen is damaged. The goal of this study was to develop a cost-effective and rapid molecular diagnostic assay to differentiate between exotic and native Haemaphysalis species to aid in ongoing surveillance of H. longicornis within the United States and help prevent misidentification. We demonstrated that restriction fragment length polymorphisms (RFLPs) targeting the 16S ribosomal RNA and the cytochrome c oxidase subunit I (COI) can be used to differentiate H. longicornis from the other Haemaphysalis species found in North America. Furthermore, we show that this RFLP assay can be applied to Haemaphysalis species endemic to other regions of the world for the rapid identification of damaged specimens. The work presented in this study can serve as the foundation for region specific PCR-RFLP keys for Haemaphysalis and other tick species and can be further applied to other morphometrically challenging taxa.
Introduction
Ticks are important vectors of numerous pathogens for humans and animals throughout the world. The accurate identification of tick species, often through morphological characteristics, is of great importance to public and veterinary health and for the control of associated tick-borne diseases. One of the key morphologic characteristics used to differentiate ixodid ticks are the mouthparts (1, 2), but often these features are damaged during tick collection, making species identification difficult or impossible.
One tick of recent importance in the United States (USA) is Haemaphysalis longicornis, the Asian longhorned tick. Native to East Asia, H. longicornis has become invasive in multiple regions of the world, largely due to its parthenogenetic reproduction, broad habitat use, and high diversity of avian and mammalian hosts (3–5). In the native range of H. longicornis, numerous bacterial, protozoal, and viral pathogens have been detected within this tick, including Anaplasma spp., Borrelia burgdorferi, Theileria spp., Babesia spp., and spotted fever group Rickettsia (6–12). Many of these pathogens are zoonotic in nature, thus this tick is of significant importance to both human and animal health. Recently, H. longicornis has also been confirmed as the vector for an emerging phlebovirus that causes Severe Fever with Thrombocytopenia Syndrome which can have mortality rates up to 40% (13–15). In North America, a pathogen of significant importance is B. burgdorferi, causative agent of Lyme disease. A recent laboratory study showed that H. longicornis is not a competent vector for the B31 strain of B. burgdorferi (16). In addition, field studies in New York, USA have not found H. longicornis on Peromyscus spp., the primary reservoir of B. burgdorferi (17). Collectively, these studies suggest that the transmission of this bacterial pathogen by H. longicornis may be limited. An additional concern with the introduction of an exotic disease vector species is the introduction of exotic pathogens that may be transmitted in its native range. In 2017, the exotic Theileria orientalis Ikeda genotype, historically known to be vectored by H. longicornis, was determined to be the cause of a mortality event in beef cattle in Virginia, USA (18). In addition, tick burdens on infested hosts can become very high leading to decreased production, growth, and in some cases death as a result of exsanguination causing a concern for the agricultural industry and some wildlife species (19–21). While the potential risks associated with the introduction of exotic H. longicornis are great, there is still much work that needs to be done to understand the implications this tick poses to human and animal health within the United States.
Haemaphysalis longicornis was first confirmed in the United States in New Jersey on a sheep in late 2017 (22). However, subsequent investigations of archived specimens revealed that H. longicornis collected as early as 2010 had been previously misidentified as the native rabbit tick, Haemaphysalis leporispalustris (5). With the introduction of H. longicornis, there are now four Haemaphysalis species known in North America: H. leporispalustris, found throughout the Americas and primarily infesting lagomorphs (23, 24); Haemaphysalis juxtakochi ranging throughout the Neotropics with cervids or other larger mammals as primary hosts, though it has been found parasitizing migratory neotropical birds (25–27); and Haemaphysalis chordeilis, sporadically collected from avian species throughout the United States and Canada (28, 29). These ticks all have wide and, in some places, overlapping distributions.
To aid in the understanding and management of the exotic H. longicornis, extensive work has been conducted on the natural history and spread of this tick within the United States (16, 17, 22, 30–32). These studies largely rely on the quick and accurate identification of H. longicornis using key morphological features found on the mouth parts (1). However, identification of Haemaphysalis ticks, both native and exotic, is difficult if the specimen's mouthparts are damaged during removal from a host. In these cases, molecular confirmation is needed to identify the ticks to the species level. This process can be expensive and time-consuming. Previous studies have shown that a restriction fragment length polymorphism (RFLP) assay is a more rapid and cost-effective method of distinguishing between arthropod vector species (33–36). Our aim was to develop a RFLP molecular assay that could accurately distinguish H. longicornis from Haemaphysalis species present in North America, as well as from other known Haemaphysalis spp. distributed globally.
Materials and Methods
Sample Collection
Haemaphysalis longicornis and H. leporispalustris from the United States were collected through a variety of methods as described by Beard et al. (5). Specimens or DNA of H. longicornis (from Australia and China), H. juxtakochi (from Mexico), and H. chordeilis (from Canada) were collected by collaborators as described in previous studies and through a citizen science program (tickspotters.org) (26, 37–39). Some Haemaphysalis spp. endemic to Japan were collected as described by Doi et al. (39), and Haemaphysalis leachi was collected as part of an ongoing canine health survey from the Sarh region of Chad, Africa. All ticks were stored in 70–100% ethanol and morphological identification was done with dissecting and compound light microscopy using dichotomous keys to distinguish between the species when possible (1–3, 28, 40, 41).
Sample Preparation
Ticks collected during this study were bisected and genomic DNA was extracted using a commercial extraction kit (DNeasy® Blood and Tissue Kit, Qiagen, Hilden Germany) following the manufacturer's protocol. The 16S rRNA and cytochrome c oxidase subunit 1 (COI) genes were targeted for PCR amplification (Table 1). PCR products were visualized on 2% agarose gels stained with GelRed (Biotium, Hayward, California). Amplicons were purified using the QIAquick gel extraction kit (Qiagen) and submitted for bi-directional sequencing at the Genewiz Corporation (South Plainfield, NJ). Chromatograms were analyzed using Geneious R11 (Auckland, New Zealand, https://www.geneious.com). Sequences of unique PCR-RFLPs obtained in this study were deposited in GenBank (accession numbers MN661147-MN661151, MN663150-MN663156, MN991269, and MN994495).
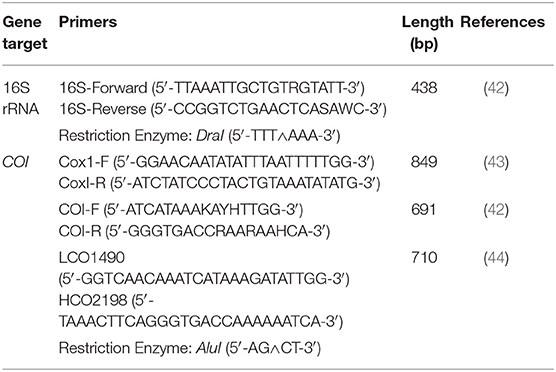
Table 1. PCR protocols, gene targets, and restriction enzymes used to obtain 16S rRNA and cytochrome c oxidase subunit 1 (COI) gene sequences and RFLPs for Haemaphysalis spp.
For COI, three primer pairs were used (Table 1). Primers COI-F/COI-R (691 bp amplicon) and LCO1490/HCO2198 (709 bp amplicon) amplify the same region, with LCO1490/HCO2198 having a 10bp overhang on either side of the COI-F/COI-R primer binding region. As a result, subsequent PCR-RFLP patterns are nearly identical with either set. Additionally, a larger segment of the COI gene (820 bp amplicon) was examined using the primer pair Cox1-F/cox1-R. These primers amplify an additional 163bp segment of the COI gene that was not already obtained by the previous two primer pairs. This was done to determine if more distinguishable PCR-RFLP patterns may exist between certain Haemaphysalis spp. Unfortunately, not all Haemaphysalis spp. collected during this study amplified with any of the three COI primers tested; however, this inconsistent amplification of the COI gene has been documented previously with other genera of ticks (42).
Restriction Length Polymorphism Assay
All DNA sequences of H. longicornis, H. leporispalustris, H. juxtakochi, and H. chordeilis collected during this study were aligned and screened with commercially available restriction enzymes to determine candidates to use for the PCR-RFLP assay. DraI and AluI restriction enzymes (ThermoFisher Scientific, Waltham, MA) were deemed appropriate and used for digestion of the 16S and COI gene regions, respectively (Table 1). The manufacturer's protocols were followed for digestion of PCR products. Digested DNA fragments were visualized with gel electrophoresis using 4% agarose gels stained with GelRed to allow for better separation of fragments and to effectively differentiate between the species of ticks from the Haemaphysalis genus.
Bioinformatic Analysis of 16S and COI Haemaphysalis spp. Cut Patterns and Phylogenetic Analysis
For the 16S analysis, the query “Haemaphysalis 16S” in GenBank returned a total of 1,217 sequences. After filtering for overlapping tick gene sequences and excluding pathogens and endosymbionts isolated from Haemaphysalis spp., excess regions were trimmed and 184 Haemaphysalis tick 16S gene sequences that overlapped with our amplified region (~438 bp) remained for in silico RFLP cut pattern analysis (Table 2). Remaining sequences were aligned, and after artificial digestion with DraI through Geneious R11, 16S PCR-RFLP cut patterns were compared with other Haemaphysalis spp. Similarly, for the COI analysis, two search queries, “Haemaphysalis cytochrome c oxidase subunit I” and “Haemaphysalis COI”, were used to obtain 594 sequences for this gene target. After removing endosymbiont and pathogen sequences, duplicate identical sequences, and trimming excess regions, 124 sequences were available for analysis (Table 3). Because multiple primer sets were used with one amplifying a longer region, sequences included in the study were split into two groups based on amplicon length (~680 and ~820 bp). Amplicons of the two lengths were digested with AluI in Geneious R11 for comparison of the PCR-RFLP cut pattern comparisons.
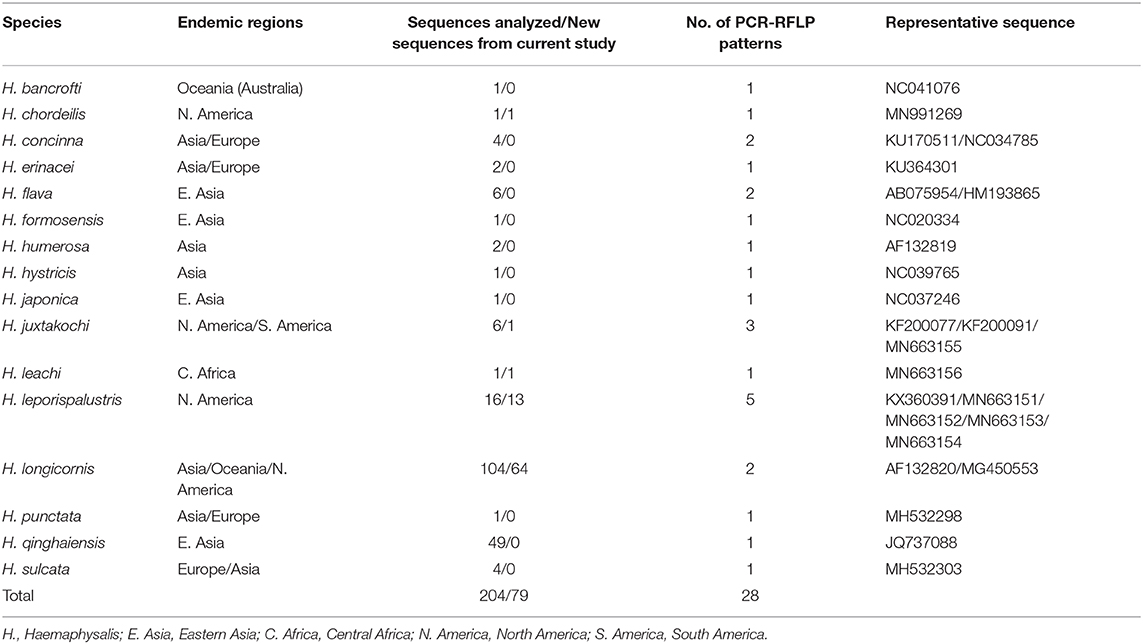
Table 3. The cytochrome c oxidase subunit I (COI) (~680 bp) gene sequences from Haemaphysalis spp. analyzed for PCR-RFLP patterns.
Two phylogenetic trees using either the 16S or the COI sequences included in this study were generated by aligning sequences using ClustalW and the maximum-likelihood algorithm in MEGA X with the two 16S rRNA and COI gene segments of Rhipicephalus sanguineus (NC00274 and JX1325) used as the outgroup (45, 46).
Results
16S Haemaphysalis spp. Cut Patterns
The query of the 16S gene of Haemaphysalis ticks from GenBank and those obtained during this study, yielded a total of 309 sequences from 35 species of proper length (~438 bp) for analysis (Table 2). Of the Haemaphysalis ticks with multiple available sequences for comparison, some intraspecies variation was detected for PCR-RFLP cut patterns. Haemaphysalis 16S PCR-RFLP patterns were compared with the Haemaphysalis spp. endemic to the same regions (Figure 3). Only one other Haemaphysalis species, H. asiatica (KC170734), shared the same PCR-RFLP cut pattern as H. longicornis (Figure 3A, blue box).
For Haemaphysalis spp. endemic to Asia, H. campanulata (AB819170), H. inermis (U95872), H. kitaokai (MH208539), H. sulcata (KR870979), and H. yeni (AB819223) all share a similar cut pattern (Figure 3A, red box). Furthermore, H. kitaokai (AB819202), H. mageshimaensis (AB819213), and an unidentified Haemaphysalis sp. from the Yunnan province of China (KU664520) also share a similar RFLP cut pattern (Figure 3A, purple box). Haemaphysalis spp. endemic to Africa, Europe, and Oceania all had unique PCR-RFLP cut patterns (Figures 3B–D).
COI Haemaphysalis spp. Cut Patterns
The query of the COI gene of Haemaphysalis ticks from GenBank and those obtained in this study yielded a total of 204 sequences from 15 species (Table 3). More intraspecies COI sequence variation was detected compared to the 16S gene target. PCR-RFLP patterns were compared with other Haemaphysalis spp. endemic to the same regions (Figure 4). Multiple PCR-RFLP cut patterns were detected for H. longicornis. Of these, a H. longicornis (AF132820) cut pattern was shared with H. flava (AB075954; Figure 4A, red box), which is endemic throughout Asia.
Additionally, H. hystricis (NC039765) and H. japonica (NC037246) share the same PCR-RFLP cut pattern (Figure 4A, green box). PCR-RFLP cut patterns for Haemaphysalis spp. endemic to Europe were all unique (Figure 2B). For Haemaphysalis spp. endemic to Oceania, specifically Australia, H. longicornis (AF132820) shared a cut pattern with an unidentified Haemaphysalis sp. collected from a koala (Phascolarctos cinereus) from Victoria, Australia (KM821503; Figure 4C, red box). Additionally, H. bancrofti (NC041076) shared a cut pattern with another unidentified Haemaphysalis sp. (KM821502; Figure 4C, green box) also collected from a koala from the same region of Australia as previously mentioned. Due to the small number of available COI sequences at 820 bp of length (n = 17, 11 different Haemaphysalis spp.), the larger segment of the gene was excluded from analysis.
Phylogenetic Analysis
Utilizing the 41 sequences of the unique 16S PCR-RFLPs and the 28 unique from the COI PCR-RFLPs (Tables 2, 3), two maximum-likelihood trees were generated for the respective gene targets (Figures 1, 2). There appears to be no clear clustering of tick sequences based on geographic location as Haemaphysalis spp. endemic to Asia are dispersed throughout in both the trees. Sequences from both gene targets confirm that H. longicornis was genetically distinct from the other Haemaphysalis spp. endemic to North America (Figures 1, 2). For the 16S rRNA gene analysis, H. leporispalustis and H. juxtakochi cluster together but are in two distinct clades in the COI analysis due to low bootstrap support for the placement of the H. juxtakochi clade (Figures 1, 2). Interestingly, H. chordeilis does not group with either North America species with either gene target but instead groups with H. punctata, a species native to Eurasia (Figures 1, 2).
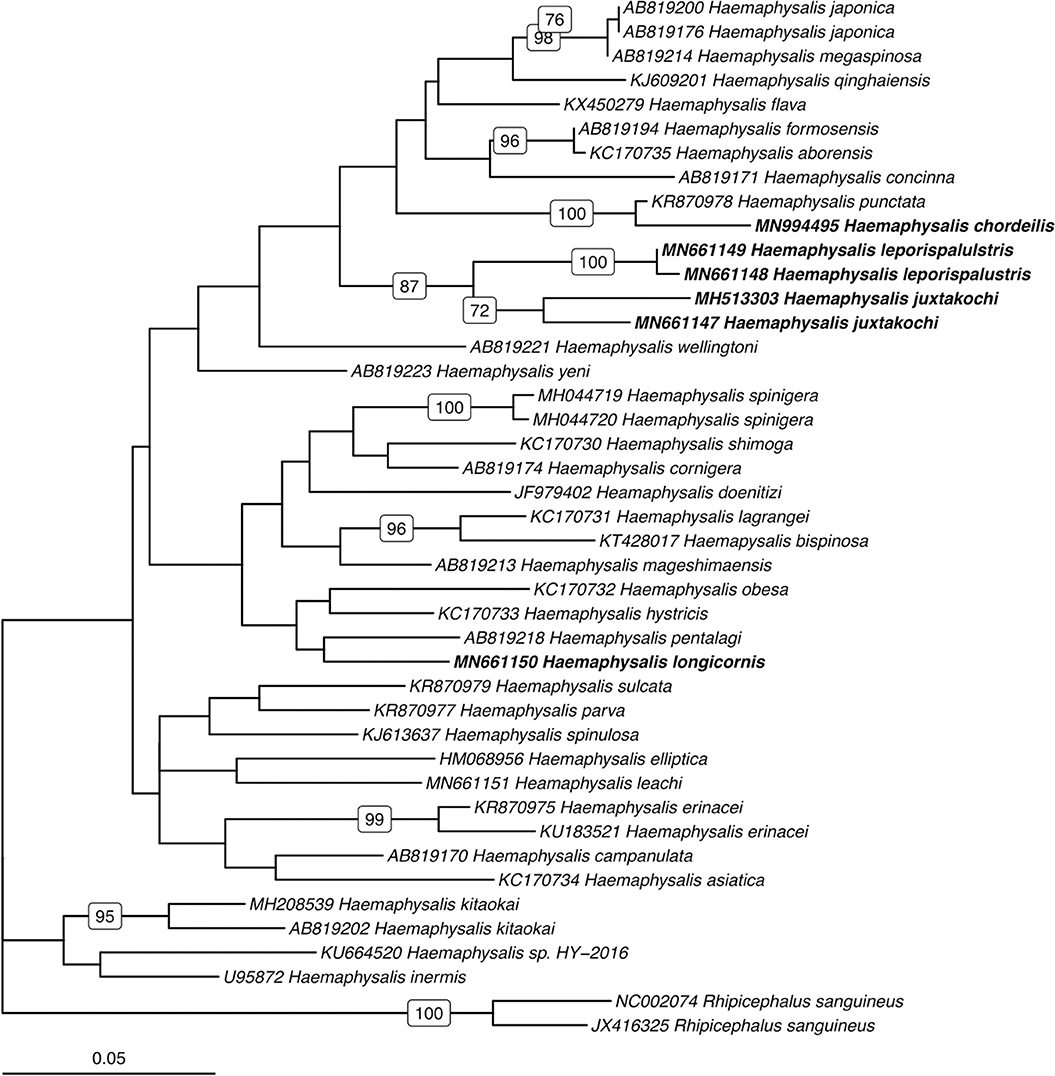
Figure 1. Phylogenetic analysis of the Haemaphysalis spp. 16S rRNA gene sequence analyzed for PCR-RFLP. Bolded sequences represent species present in North America. Numbers on branches indicate bootstrap values after 500 iterations, values below 70% were omitted from the tree.
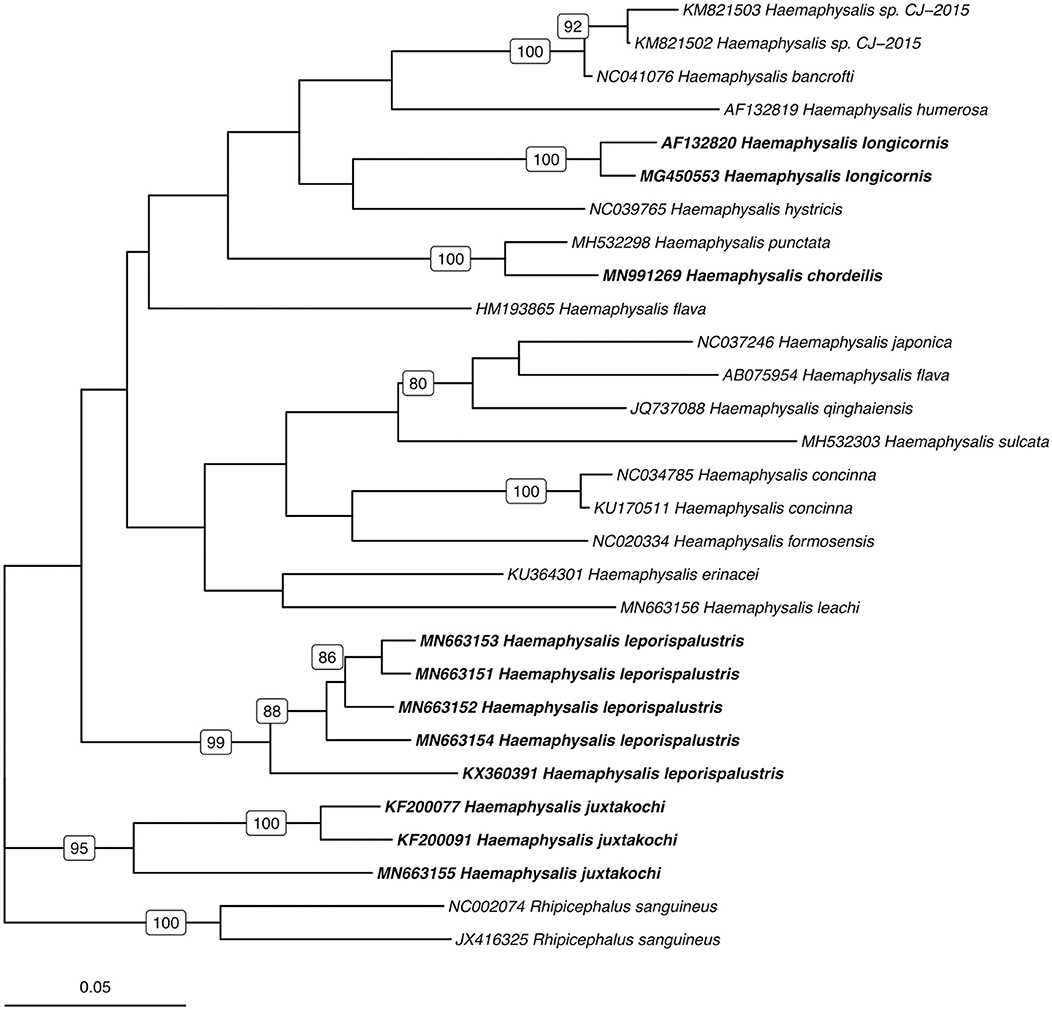
Figure 2. Phylogenetic analysis of the Haemaphysalis spp. COI gene sequence analyzed for PCR-RFLP. Bolded sequences represent species present in North America. Numbers on branches indicate bootstrap values after 500 iterations, values below 70% were omitted from the tree.
Molecular Key for North American Haemaphysalis spp. RFLP Cut Patterns
For Haemaphysalis spp. endemic to North America, H. longicornis had one or more unique PCR-RFLP cut patterns for both gene targets, all of which allow for effective differentiation between the other tick species endemic to this region (Figure 5). The inclusion of our sequence data for Haemaphysalis spp. present in North America revealed greater diversity than what is currently represented by sequences analyzed from GenBank. For the 16S PCR-RFLP, H. longicornis and H. chordeilis had a single cut pattern and H. juxtakochi and H. leporispalustris both had two unique and descriptive PCR-RFLP cut patterns (Figure 5A). Unlike the 16S PCR-RFLP, all Haemaphysalis ticks of North America had multiple cut patterns for the COI PCR-RFLP with the exception of the single H. chordeilis sequence included in the study. H. longicornis and H. juxtakochi both had 2 and 3 unique COI PCR-RFLP cut patterns, respectively, and H. leporispalustris had 5 cut patterns (Figure 5B). A key to species of Haemaphysalis ticks of North America was constructed using the 16S PCR-RFLP patterns (Figure 3, See Key to Haemaphysalis ticks found in North America); a key for the COI PCR-RFLP patterns was not included due to the higher intraspecific variation.
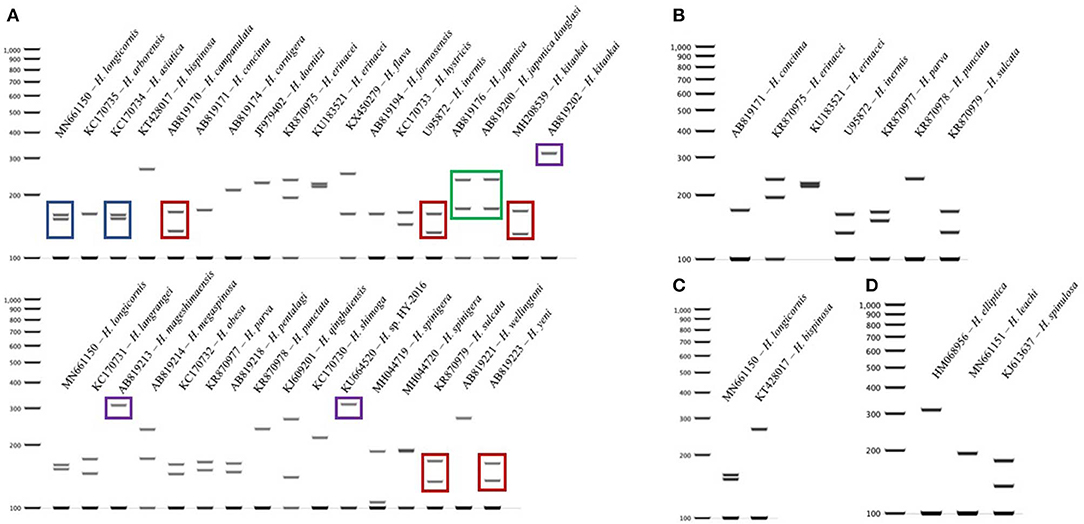
Figure 3. 16S PCR-RFLP cut patterns for Haemaphysalis spp. endemic to different regions of the world. (A) 16S PCR-RFLP cut patterns of Haemaphysalis spp. endemic to Asia. Different colored boxes indicate that the PCR-RFLP cut patterns is shared with at least one other Haemaphysalis spp.; (B) 16S PCR-RFLP cut patterns of Haemaphysalis spp. endemic to Europe; (C) 16S PCR-RFLP cut patterns of Haemaphysalis spp. endemic to Oceania; (D) 16S PCR-RFLP cut patterns of Haemaphysalis spp. endemic to Africa.
Discussion
Here we analyze new sequences of several Haemaphysalis spp., including the first genetic data for H. chordeilis, and describe a molecular assay that can aid in the rapid and accurate identification of any life stage of the four Haemaphysalis spp. currently in North America. Ticks collected from hosts are often submitted to diagnostic laboratories damaged or without mouthparts, which are required for specific identification of Haemaphysalis spp., so this technique is especially useful. Given the range expansion, or recognized range, of H. longicornis in the United States, the need for a rapid response is key and this technique provides an alternative method to accurately identify ticks when morphology becomes unreliable. Furthermore, inclusion of Haemaphysalis spp. from other regions of the world suggests that this method can potentially be useful for distinguishing a wide range of species.
The restriction enzymes for the PCR-RFLP were selected to optimize the differentiation of H. longicornis from other Haemaphysalis spp. in North America. The 16S PCR-RFLP was more reliable than the COI PCR-RFLP, as there were many more sequences available for comparison and the RFLP patterns were unique between species (Table 2, Figure 5). However, regardless of gene target, H. longicornis was effectively differentiated from other Haemaphysalis spp. native to North America. Unfortunately, sequence data for only one specimen of H. chordeilis was made available for analysis, so there is a possibility that there may be more than one PCR-RFLP cut pattern for this species for both the 16S and COI gene targets (Figure 5). Although this is a limitation for this molecular key, H. chordeilis is an avian specialist and is only rarely reported in the United States (29). The sequence of H. chordeilis was excluded in both gene analyses from clades including the other two native North American species (H. juxtakochi and H. leporispalustris) which may be explained based on previous morphologic work (Figures 1, 2) (47, 48). Although there is taxonomic debate regarding the validity of all Haemaphysalis subgenera H. chordeilis and H. punctata are both in the subgenus Aboimisalis, whereas H. leporispalustris and H. juxtakochi are both in the subgenus Gonixodes (47–49). Also, based on morphologic characteristics, H. chordeilis and H. punctata are very similar and may be difficult to differentiate. The addition of the 16S and COI H. chordeilis sequence data from this study provides valuable insight into the taxonomic position of this species. Furthermore, analysis of the PCR-RFLPs indicates that these closely related and morphologically similar Haemaphysalis spp. can be effectively differentiated with the COI PCR-RFLP (Figures 4A, 5B).
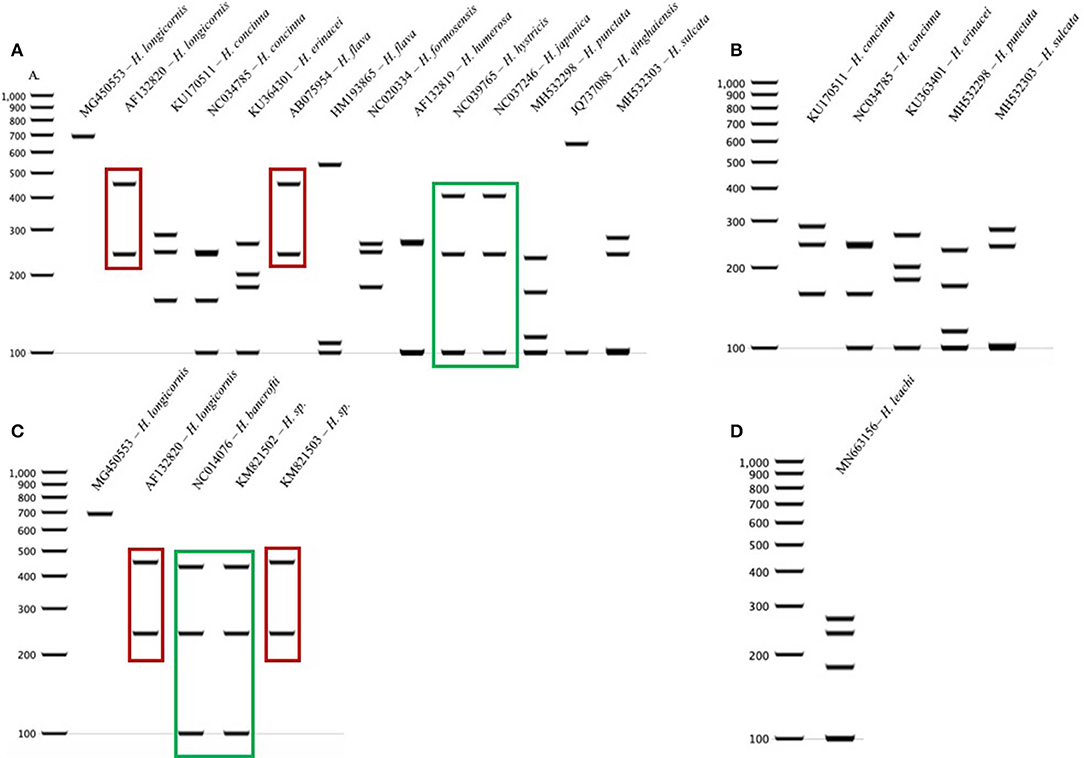
Figure 4. COI PCR-RFLP cut patterns for Haemaphysalis spp. endemic to different regions of the world. (A) COI rRNA PCR-RFLP cut patterns of Haemaphysalis spp. endemic to Asia. Different colored boxes indicate that the PCR-RFLP cut patterns is shared with at least one other Haemaphysalis spp.; (B) COI PCR-RFLP cut patterns of Haemaphysalis spp. endemic to Europe; (C) COI PCR-RFLP cut patterns of Haemaphysalis spp. endemic to Oceania; (D) COI PCR-RFLP cut patterns of Haemaphysalis spp. endemic to Africa.
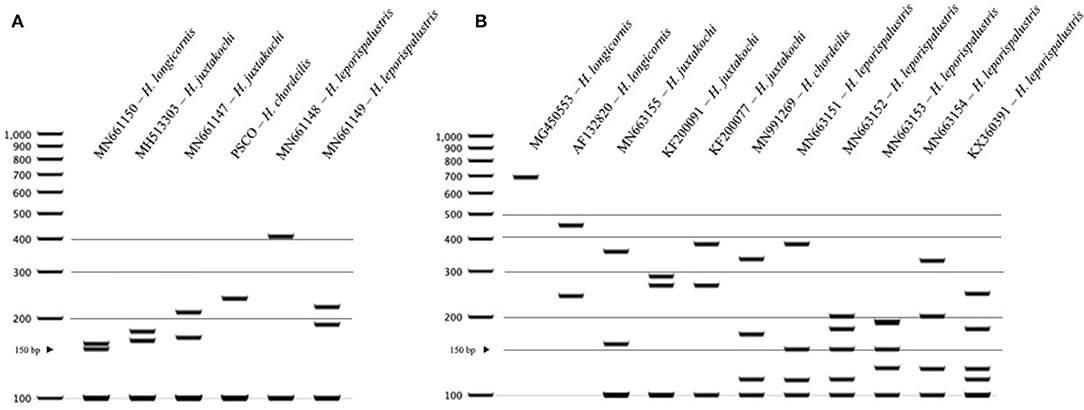
Figure 5. PCR-RFLP cut patterns for Haemaphysalis spp. of North America. (A) 16S PCR-RFLP cut patterns of Haemaphysalis spp. (B) COI PCR-RFLP cut patterns of Haemaphysalis spp.
The RFLP patterns of H. longicornis were indistinguishable from a few Haemaphysalis species outside of North America (Figures 3A, 4A). However, H. longicornis can be distinguished from all of the species with available sequence data if both gene targets are included in the analysis. For the 16S PCR-RFLP, H. longicornis shared a cut pattern with H. asiatica which is endemic to Thailand and the surrounding countries and, thus far has, only been described to be infesting felids and canids native to that region (Figure 3A) (50). It is likely that the range of H. longicornis and H. asiatica overlap so further optimization of this assay within this region is warranted before use. We also detected more interspecies overlap in the 16S PCR-RFLPs, likely due to the larger amount of available sequence data. For the COI PCR-RFLP, there was less available sequence data for PCR-RFLP comparisons. One of the two H. longicornis cut patterns was shared with H. flava which is endemic to eastern Asia and infests a wide range of mammalian hosts including those utilized by H. longicornis, most notably sheep, cattle, and horses (Figure 4A) (41, 51).
Haemaphysalis is a highly speciose genus, being the second largest after Ixodes (52). Our study provided new sequence data from several Haemaphysalis species including H. chordeilis and the invasive H. longicornis. Specifically, for H. leporispalustris (rabbit tick) that is native to North America, we noted considerable sequence variation (92–99%) and multiple RFLPs for the COI gene target, which was unexpected because the intraspecific variation noted for other Haemaphysalis spp. with numerous sequences available rarely resulted in several unique RFLP patterns [e.g., H. longicornis and H. qinghaiensis; Tables 2, 3; (53, 54)]. This highlights the need for additional sequencing of individual ticks from different populations and host species to further evaluate the intraspecific genetic variability of different gene targets and the utility of the PCR-RFLPs described in this study. In summary, the 16S PCR-RFLP performed better than the COI PCR-RFLP as Haemaphysalis spp. sequences from North America and other countries had lower intraspecific variation, and there were a larger number of 16S sequences available on GenBank for comparison, for this reason only a key to species was generated for the 16S PCR-RFLP assay. While further optimization is warranted in other regions of the world where a higher diversity of Haemaphysalis spp. ticks of human and veterinary importance are present, the methods detailed here provide a faster and more cost-effective alternative to sequencing damaged tick specimens, especially those from North America. Finally, this study can serve as the foundation for similar, more region-specific PCR-RFLP molecular keys for the Haemaphysalis spp. and other vector species of one health importance.
Key to Haemaphysalis Ticks Found in North America, Based on DraI Restriction Digestion of the 16S rRNA Gene Amplified by PCR (Figure 5A)
Data Availability Statement
The datasets generated for this study can be found in the NCBI GenBank: MN661147-MN661151, MN663150-MN663156, MN991269, and MN994495.
Author Contributions
AT, KD, and MY designed and developed the assays and wrote the paper. AT developed the molecular key, analyzed final datasets, and created the figures and tables. CC, SD, KD, RF, TG, PI, LL, JL, TM, CO, RR-V, MR, DS, SV, and SW were integral in the collection of specimens for analysis. All authors contributed to manuscript revision, read and approved the submitted version.
Funding
This work was supported by the National Institute of General Medical Sciences of the National Institutes of Health under award number R25GM109435 and the National Science Foundation under Grant No. DGE-1545433. The content is solely the responsibility of the authors and does not necessarily represent the official views of the National Institutes of Health nor the National Science Foundation. Partial support was provided through Cooperative Agreement 17-9100-1407-CA, Veterinary Services, Animal and Plant Health Inspection Service, U.S. Department of Agriculture. Additional support was provided by the member states and wildlife agencies of the Southeastern Cooperative Wildlife Disease Study through the Federal Aid to Wildlife Restoration Act (50 Stat. 917).
Conflict of Interest
The authors declare that the research was conducted in the absence of any commercial or financial relationships that could be construed as a potential conflict of interest.
Acknowledgments
The authors would like to thank the many field and laboratory technicians that aided in the collection and morphologic identification of the ticks collected during this study as well as Dr. Carter A. Mitchell, Kayla Garrett, and Anna Willoughby for their critical review of the manuscript and molecular key.
References
1. Egizi AM, Robbins RG, Beati L, Nava S, Evans CR, Occi JL, et al. A pictorial key to differentiate the recently detected exotic Haemaphysalis longicornis Neumann, 1901 (Acari, Ixodidae) from native congeners in North America. Zookeys. (2019) 818:117–28. doi: 10.3897/zookeys.818.30448
2. Keirans JE, Litwak TR. Pictorial key to the adults of hard ticks, family Ixodidae (Ixodida: Ixodoidea), east of the Mississippi River. J Med Entomol. (1989) 26:435–48. doi: 10.1093/jmedent/26.5.435
3. Hoogstraal H, Roberts FHS, Kohls TGM, Tipton VJ. Review of Haemaphysalis (Kaiseriana) longicornis Neumann (Resurrected) of Australia, New Zealand, New Caledonia, Fiji, Japan, Korea, and Northeastern China and USSR. and Its Parthenogenetic and Bisexual Populations (Ixodoidea, Ixodidae). J Parasitol. (1968) 54:1197–213.
4. Heath ACG. Biology, ecology and distribution of the tick, Haemaphysalis longicornis Neumann (Acari: Ixodidae) in New Zealand. N Z Vet J. (2016) 64:10–20. doi: 10.1080/00480169.2015.1035769
5. Beard C Ben, Occi J, Bonilla DL, Egizi AM, Fonseca DM, Mertins JW, et al. Multistate infestation with the exotic disease – vector tick Haemaphysalis longicornis — United States, August 2017 – September 2018. Morbitity Mortal Wkly Rep. (2018) 67:1310–3. doi: 10.15585/mmwr.mm6747a3
6. Lee MJ, Chae JS. Molecular detection of Ehrlichia chaffeensis and Anaplasma bovis in the salivary glands from Haemaphysalis longicornis ticks. Vector Borne Zoonot Dis. (2010) 10:411–3. doi: 10.1089/vbz.2008.0215
7. Lu X, Lin XD, Wang JB, Qin XC, Tian JH, Guo WP, et al. Molecular survey of hard ticks in endemic areas of tick-borne diseases in China. Ticks Tick Borne Dis. (2013) 4:288–96. doi: 10.1016/j.ttbdis.2013.01.003
8. Sivakumar T, Tattiyapong M, Okubo K, Suganuma K, Hayashida K, Igarashi I, et al. PCR detection of Babesia ovata from questing ticks in Japan. Ticks Tick Borne Dis. (2014) 5:305–10. doi: 10.1016/j.ttbdis.2013.12.006
9. Kang JG, Ko S, Barney Smith W, Kim HC, Lee IY, Chae JS. Prevalence of Anaplasma, Bartonella and Borrelia Species in Haemaphysalis longicornis collected from goats in North Korea. J Vet Sci. (2016) 17:207–16.
10. Watts JG, Playford MC, Hickey KL. Theileria orientalis: a review. N Z Vet J. (2016) 64:3–9. doi: 10.1080/00480169.2015.1064792
11. Hong SH, Kim SY, Song BG, Rho JR, Cho CR, Kim CN, et al. Detection and characterization of an emerging type of Babesia sp. similar to Babesia motasi for the first case of human babesiosis and ticks in Korea. Emerg Microbes Infect. (2019) 8:869–78. doi: 10.1080/22221751.2019.1622997
12. Lee J-H, Park H-S, Jung K-D, Jang W-J, Koh S-E, Kang S-S, et al. Identification of the spotted fever group rickettsiae detected from Haemaphysalis longicornis in Korea. Microbiol Immunol. (2003) 47:301–4. doi: 10.1111/j.1348-0421.2003.tb03399.x
13. Zhuang L, Sun Y, Cui XM, Tang F, Hu JG, Wang LY, et al. Transmission of severe fever with thrombocytopenia syndrome virus by Haemaphysalis longicornis ticks, China. Emerg Infect Dis. (2018) 24:868–71. doi: 10.3201/eid2405.151435
14. Zhang Y-Z, He Y-W, Dai Y-A, Xiong Y, Zheng H, Zhou D-J, et al. Hemorrhagic fever caused by a novel Bunyavirus in China: pathogenesis and correlates of fatal outcome. Clin Infect Dis. (2012) 54:527–33. doi: 10.1093/cid/cir804
15. Li Z, Bao C, Hu J, Liu W, Wang X, Zhang L, et al. Ecology of the tick-borne phlebovirus causing severe fever with thrombocytopenia syndrome in an endemic area of China. PLoS Negl Trop Dis. (2016) 10:e0004574. doi: 10.1371/journal.pntd.0004574
16. Breuner NE, Ford SL, Hojgaard A, Osikowicz LM, Parise CM, Rosales Rizzo MF, et al. Failure of the Asian longhorned tick, Haemaphysalis longicornis, to serve as an experimental vector of the Lyme disease spirochete, Borrelia burgdorferi sensu stricto. Ticks Tick Borne Dis. (2019) 2019:101311. doi: 10.1016/j.ttbdis.2019.101311
17. Tufts DM, VanAcker MC, Fernandez MP, DeNicola A, Egizi A, Diuk-Wasser MA. Distribution, host-seeking phenology, and host and habitat associations of Haemaphysalis longicornis Ticks, Staten Island, New York, USA. Emerg Infect Dis. (2019) 25:792–6. doi: 10.3201/eid2504.181541
18. Oakes VJ, Yabsley MJ, Schwartz D, Leroith T, Bissett C, Broaddus C, et al. Theileria orientalis Ikeda Genotype in Cattle, Virginia, USA. Emerg Infect Dis. (2019) 25:1653–9. doi: 10.3201/eid2509.190088
19. Neilson FJAA, Mossman DH. Anaemia and deaths in red deer (Cervus elaphus) fawns associated with heavy infestations of cattle tick (Haemaphysalis longicornis). N Z Vet J. (1982) 30:125–6. doi: 10.1080/00480169.1982.34908
20. Heath ACG, Tenquist JD, Bishop DM. Goats, hares, and rabbits as hosts for the New Zealand cattle tick, Haemaphysalis longicornis. N Z J Zool. (1987) 14:549–55. doi: 10.1080/03014223.1988.10422638
21. Heath ACG, Pearce DM, Tenquist JD, Cole DJW. Some effects of a tick infestation (Haemaphysalis longicornis) on sheep. N Z J Agric Res. (1977) 20:19–22. doi: 10.1080/00288233.1977.10427296
22. Rainey T, Occi JL, Robbins RG, Egizi A. Discovery of Haemaphysalis longicornis (Ixodida: Ixodidae) Parasitizing a Sheep in New Jersey, United States. J Med Entomol. (2018) 55:757–9. doi: 10.1093/jme/tjy006
23. Eremeeva ME, Weiner LM, Zambrano ML, Dasch GA, Hu R, Vilcins I, et al. Detection and characterization of a novel spotted fever group Rickettsia genotype in Haemaphysalis leporispalustris from California, USA. Ticks Tick Borne Dis. (2018) 9:814–8. doi: 10.1016/j.ttbdis.2018.02.023
24. Labruna MB, Leite RC, Faccini JLH, Ferreira F. Life cycle of the tick Haemaphysalis leporis-palustris (Acari: Ixodidae) under laboratory conditions. Exp Appl Acarol. (2000) 24:683–94. doi: 10.1023/A:1010768511790
25. García GG, Castro AD, Bermúdez SC, Nava S. Some ecological aspects of free-living Haemaphysalis juxtakochi Cooley, 1946 (Acari: Ixodidae) in Panama. Rev MVZ Córdoba. (2017) 19:3984. doi: 10.21897/rmvz.118
26. Ojeda-Chi MM, Rodriguez-Vivas RI, Esteve-Gasent MD, Pérez de León A, Modarelli JJ, Villegas-Perez S. Molecular detection of rickettsial tick-borne agents in white-tailed deer (Odocoileus virginianus yucatanensis), mazama deer (Mazama temama), and the ticks they host in Yucatan, Mexico. Ticks Tick Borne Dis. (2019) 10:365–70. doi: 10.1016/j.ttbdis.2018.11.018
27. Budachetri K, Williams J, Mukherjee N, Sellers M, Moore F, Karim S. The microbiome of neotropical ticks parasitizing on passerine migratory birds. Ticks Tick Borne Dis. (2017) 8:170–3. doi: 10.1016/j.ttbdis.2016.10.014
28. Lindquist EE, Galloway TD, Artsob H, Lindsay LR, Drebot M, Wood H, et al. A Handbook to the Ticks of Canada (Ixodida: Ixodidae, Argasidae). Biological Survey of Canada (2016). doi: 10.3752/9780968932186
29. Bishopp FC, Trembley HL. Distribution and hosts of certain North American Ticks. J Parasitol. (1945) 31:1–54.
30. Rochlin I. Modeling the Asian longhorned tick (Acari: Ixodidae) suitable habitat in North America. J Med Entomol. (2018) 20:1–8. doi: 10.1093/jme/tjy210
31. Hutcheson HJ, Robbin Lindsay L, Dergousoff SJ. Haemaphysalis longicornis: a tick of considerable importance, now established in North America. Can J Public Heal. (2018) 110:118–9. doi: 10.17269/s41997-018-0152-4
32. Magori K. Preliminary prediction of the potential distribution and consequences of Haemaphysalis longicornis using a simple rule-based climate envelope model. bioRxiv. (2018) 2018:389940. doi: 10.1101/389940
33. Severson DW. RFLP analysis of insect genomes. In: Crampton JM, Ben Beard C, Louis C, editors. The Molecular Biology of Insect Disease Vectors: A Methods Manual. Dordrecht: Springer (1997). p. 309–20. doi: 10.1007/978-94-009-1535-0_26
34. Poucher KL, Hutcheson HJ, Keirans JE, Durden LA, Iv WCB, Poucher KL, et al. Molecular genetic key for the identification of 17 Ixodes species of the United States (Acari: Ixodidae): a methods model source. J Parasitol. (1999) 85:623–9.
35. West DF, Payette T, Mundy T, Black IV WC. Regional molecular genetic key of thirteen snow pool Aedes species (Diptera: Culicidae) in Northern Colorado. J Med Entomol. (1997) 34:404–10. doi: 10.1093/jmedent/34.4.404
36. Alam MS, Kato H, Fukushige M, Wagatsuma Y, Itoh M. Application of RFLP-PCR-based identification for sand fly surveillance in an area endemic for Kala-Azar in Mymensingh, Bangladesh. J Parasitol Res. (2012) 2012:1–4. doi: 10.1155/2012/467821
37. Greay TL, Oskam CL, Gofton AW, Rees RL, Ryan UM, Irwin PJ. A survey of ticks (Acari: Ixodidae) of companion animals in Australia. Parasit Vec. (2016) 9:1–10. doi: 10.1186/s13071-016-1480-y
38. Chen Z, Yang X, Bu F, Yang X, Liu J. Morphological, biological and molecular characteristics of bisexual and parthenogenetic Haemaphysalis longicornis. Vet Parasitol. (2012) 189:344–52. doi: 10.1016/j.vetpar.2012.04.021
39. Doi K, Kato T, Hayama S. Infestation of introduced raccoons (Procyon lotor) with indigenous ixodid ticks on the Miura Peninsula, Kanagawa Prefecture, Japan. Int J Parasitol Parasites Wildl. (2018) 7:355–359. doi: 10.1016/j.ijppaw.2018.09.002
40. Hoogstraal H. African Ixodoidea. VoI. I. Ticks of the Sudan (With Special Reference to Equatoria Province and with Preliminary Reviews of the Genera Boophilus, Margaropus, and Hyalomma) Washington, DC: Department of the Navy, Bureau of Medicine and Surgery (1956).
41. Yamaguti N, Tipton VJ, Keegan HL, Toshioka S. Ixodid ticks of Japan, Korea and the Ryukyu Islands. Brigham Young Univ Sci Bull Biol Ser. (1971) 15:1–226.
42. Lv J, Wu S, Zhang Y, Chen Y, Feng C, Yuan X, et al. Assessment of four DNA fragments (COI, 16S rDNA, ITS2, 12S rDNA) for species identification of the Ixodida (Acari: Ixodida). Parasit Vec. (2014) 7:1–11. doi: 10.1186/1756-3305-7-93
43. Chitimia L, Lin RQ, Cosoroaba I, Wu XY, Song HQ, Yuan ZG, et al. Genetic characterization of ticks from southwestern Romania by sequences of mitochondrial cox1 and nad5 genes. Exp Appl Acarol. (2010) 52:305–11. doi: 10.1007/s10493-010-9365-9
44. Folmer O, Black M, Hoeh W, Lutz R, Vrijenhoek R. DNA primers for amplification of mitochondrial cytochrome c oxidase subunit I from diverse metazoan invertebrates. Mol Mar Biol Biotechnol. (1994) 3:294–9.
45. Thompson JD, Gibson T, Higgins DG. Multiple sequence alignment using ClustalW and ClustalX. Curr Protoc Bioinforma. (2002) Chapter 2:Unit 2.3. doi: 10.1002/0471250953.bi0203s00
46. Stecher G, Tamura K, Kumar S. Molecular Evolutionary Genetics Analysis (MEGA) for macOS. Mol Biol Evol. (2020). doi: 10.1093/molbev/msz312. [Epub ahead of print].
47. Hoogstraal H. Redescription of the type material of Haemaphysalis (Aboimisalis) cinnabarina (Revalidated) and Its Junior Synonym H. (A.) sanguinolenta Described by Koch in 1844 from Brazil (Ixodoidea: Ixodidae). J Parasitol. (1973) 59:379–83. doi: 10.2307/3278839
48. Barros-Battesti DM, Onofrio VC, Arzua M, Labruna MB. Comments on the validity of Haemaphysalis cinnabarina KOCH, 1844 (Acari: Ixodidae), A taxon known solely by the type specimens from Northern Brazil. Rev Bras Parasitol Vet. (2008) 17:53–5. doi: 10.1590/s1984-29612008000100012
49. Kohls GM. Records and new synonymy of new world Haemaphysalis ticks, with descriptions of the nymph and larva of H. juxtakochi Cooley. J Parasitol. (1960) 46:355–61.
50. Grassman LI, Sarataphan N, Tewes ME, Silvy NJ, Nakanakrat T. Ticks (Acari: Ixodidae) Parasitizing Wild Carnivores in Phu Khieo Wildlife Sanctuary, Thailand. J Parasitol. (2004) 90:657–9. doi: 10.1645/GE-3327RN
51. Inokuma H, Beppu T, Okuda M, Shimada Y, Sakata Y. Detection of ehrlichial DNA in Haemaphysalis ticks recovered from dogs in Japan that is closely related to a novel Ehrlichia sp. found in cattle ticks from Tibet, Thailand, and Africa. J Clin Microbiol. (2004) 42:1353–5. doi: 10.1128/JCM.42.3.1353-1355.2004
52. Kolonin GV, Andreev VL. Species diversity of Ixodid ticks of world fauna (Acari, Ixodidae). In: Khakhin GV, Kolonin GV, Bamkirova IA, editors. Disease Parasites of Wild Animals. Moscow: Zoological Insitute Akademii Nauk (2014). p. 130–49.
53. Liu X, Chen Z, Ren Q, Luo J, Xu X, Wu F, et al. Genetic diversity of Haemaphysalis qinghaiensis (Acari: Ixodidae) in western China. Exp Appl Acarol. (2018) 74:427–41. doi: 10.1007/s10493-018-0242-2
Keywords: Haemaphysalis, Asian longhorned tick, PCR-RFLP, molecular key, invasive, phylogenetic
Citation: Thompson AT, Dominguez K, Cleveland CA, Dergousoff SJ, Doi K, Falco RC, Greay T, Irwin P, Lindsay LR, Liu J, Mather TN, Oskam CL, Rodriguez-Vivas RI, Ruder MG, Shaw D, Vigil SL, White S and Yabsley MJ (2020) Molecular Characterization of Haemaphysalis Species and a Molecular Genetic Key for the Identification of Haemaphysalis of North America. Front. Vet. Sci. 7:141. doi: 10.3389/fvets.2020.00141
Received: 22 November 2019; Accepted: 25 February 2020;
Published: 13 March 2020.
Edited by:
Guiquan Guan, Chinese Academy of Agricultural Sciences, ChinaReviewed by:
Quan Liu, Foshan University, ChinaAbdul Jabbar, The University of Melbourne, Australia
Copyright © 2020 Thompson, Dominguez, Cleveland, Doi, Falco, Greay, Irwin, Liu, Mather, Oskam, Rodriguez-Vivas, Ruder, Shaw, Vigil, White, Yabsley, and Her Majesty the Queen in Right of Canada (for Dergousoff and Lindsay). This is an open-access article distributed under the terms of the Creative Commons Attribution License (CC BY). The use, distribution or reproduction in other forums is permitted, provided the original author(s) and the copyright owner(s) are credited and that the original publication in this journal is cited, in accordance with accepted academic practice. No use, distribution or reproduction is permitted which does not comply with these terms.
*Correspondence: Alec T. Thompson, YWxlYy50aG9tcHNvbkB1Z2EuZWR1; Michael J. Yabsley, bXlhYnNsZXlAdWdhLmVkdQ==