- 1National Center of Stomatology, National Clinical Research Center for Oral Diseases, National Engineering Laboratory for Digital and Material Technology of Stomatology, Department of Orthodontics, Peking University School and Hospital of Stomatology, Beijing, China
- 2CAS Key Laboratory of Bio-Inspired Materials and Interfacial Science, CAS Center for Excellence in Nanoscience, Technical Institute of Physics and Chemistry, Chinese Academy of Sciences, Beijing, China
- 3University of Chinese Academy of Sciences, Beijing, China
Nickel–titanium (NiTi) alloys show broad applicability in biomedical fields. However, the unexpected aggregation of bacteria and the corrosion of body fluid on NiTi-based medical devices often lead to the leakage of nickel ions, resulting in inevitable allergic and cytotoxic activities. Therefore, the capture and detection of nickel ions are important to avoid serious adverse reactions caused by NiTi-based medical devices. Herein, we presented a nickel ion capture strategy by the combination of zwitterionic hydrogels as anti-bacteria layers and carbon disulfide (CS2) components as nickel-catchers (Ni-catchers). On the one hand, the hydration layer of zwitterionic hydrogel can efficiently inhibit bacteria adhesion and reduce nickel ions leakage from NiTi corrosion. On the other hand, Ni-catchers can capture leaked nickel ions from NiTi alloy actively by chelation reaction. Therefore, this strategy shows great capabilities in resisting bacteria adhesion and capturing nickel ions, providing the potential possibility for the detection of nickel ion leakage for implantable biomedical materials and devices.
Introduction
With the rapid development of biomedicine, implantable materials have been widely used in diagnosis, treatment, and prosthesis (Sylvestre et al., 2020). As one of the most popular biomaterials, nickel–titanium (NiTi) alloys have been applied in orthodontic archwires (Liu et al., 2019), bone implants (Raphel et al., 2016), and vascular stents (Robertson and Ritchie, 2007), due to their unique superelasticity and good biocompatibility. However, the leakage of nickel ions (Ni2+ ions) from NiTi alloys often leads to adverse reactions such as allergic signs (e.g., contact mucositis and eczematic and urticarial reactions; Huang et al., 2003; Kolokitha and Chatzistavrou, 2008) and cytotoxic responses (Jia et al., 1999). In addition, the unexpected adhesion of bacteria and acidic oral environment corrode NiTi alloys, aggravating the leakage of Ni2+ ions (Huang et al., 2003). Therefore, a novel strategy for capturing and detecting leaked Ni2+ ions is urgently needed for potential biomedical applications.
In recent years, many passive strategies have been developed to avoid the leakage of Ni2+ ions, including growing oxide films [e.g., TiO2 layer (Gu et al., 2005) and NiTiO3 nanosheet (Hang et al., 2019)], depositing coatings [e.g., diamond-like carbon coating (Hang et al., 2012) and 1H,1H,2H,2H-perfluorodecyltrimethoxysilane (FAS) deposited layer (Liu et al., 2019)], and implanting barrier elements [e.g., carbon (Poon et al., 2005) and oxygen (Wu et al., 2006) plasma immersion ion implantation]. However, most of them can not prohibit long-term leakage of Ni2+ ions because of the low stability of hydrophobic surface in acidic oral environment (Liu et al., 2019) and the corrosion of adhered bacteria (Gu et al., 2005). Moreover, there are many anti-bacteria methods including zwitterionic hydrogels (Li et al., 2008; Shivapooja et al., 2015; Lin et al., 2020), polyethylene glycol (Peng et al., 2017), quaternary ammonium salt groups (Wei et al., 2016, 2017), protein-based surfaces (Liu et al., 2018), and multiwalled carbon nanotubes (Hartono et al., 2018). Thus, combining anti-bacteria and Ni2+ ion capture may be a promising strategy for the new generation of NiTi-based medical devices. As a conventional medical treatment for acute carbonyl nickel poisoning, carbon disulfide (CS2) derivates can serve as Ni-catchers by chelation reaction (Sunderman, 1990). Therefore, we believe that introducing Ni-catchers into anti-bacteria layer can achieve long-term Ni2+ ion capture and detection.
Herein, we present a Ni-catcher-doped zwitterionic hydrogel coating on the surface of NiTi alloy, showing robust anti-bacteria and long-term Ni2+ ion capture (Figure 1). In brief, zwitterionic hydrogel was firstly prepared on the surface of NiTi alloy (H-NiTi) by the copolymerization of 2-hydroxyethyl methacrylate (HEMA), 3-dimethyl(methacryloyloxyethyl)ammonium propane-sulfonate (DMAPS), and methyl methacrylate (MMA; Su et al., 2020). Then, the CS2 as a Ni-catcher was grafted into zwitterionic hydrogel by immersing H-NiTi in the mixture of CS2 and triethylamine (TEA) to obtain CS-NiTi (Figure 1A and Supplementary Figure 1). The zwitterionic hydrogel can prevent the adhesion of bacteria by the hydration layer on the surface of CS-NiTi. Moreover, the Ni-catcher endows this coating with Ni2+ ion capture through chelation reaction. Therefore, this work provides a promising avenue to capture and detect Ni2+ ions, which could be used for implantable biomedical materials and devices.
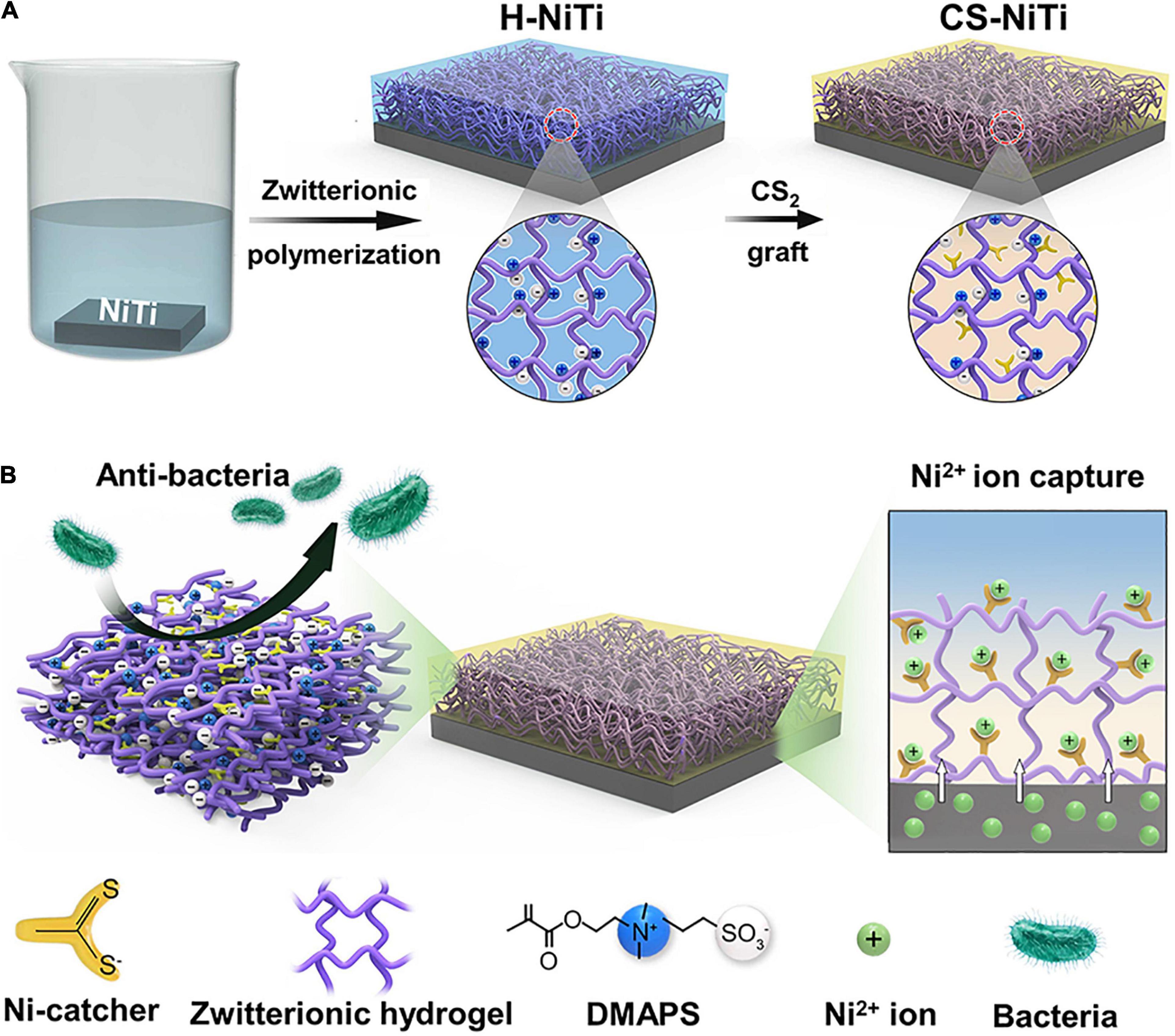
Figure 1. Design principle of CS-NiTi for long-term Ni2+ ion capture. (A) Schematic of the fabrication process of CS-NiTi. (B) The as-prepared CS-NiTi shows dual functions of anti-bacteria and Ni2+ ion capture.
Materials and Methods
Chemicals and Materials
Nickel–titanium alloy was purchased from Sunway Technology Co. Ltd. (Guangdong, China). Brain heart infusion (BHI) was obtained from Oxoid Co. (Hampshire, United Kingdom). Dulbecco’s modified Eagle medium (DMEM) was bought from Thermo Fisher Biochemical Products Co. Ltd. (Beijing, China). Tris–HCl buffer was purchased from Coolaber Science Technology (Beijing, China). Phosphate-buffered saline (PBS) was bought from HyClone Laboratories Inc. (Logan, UT, United States). Acetone (>99.5%, AR) and alcohol (≥99.8%, GR) were obtained from the Beijing Chemical Co. (Beijing, China). Bis(2-methacryloxyethyl)phosphate (bis-HEMAP) and 2,2-diethoxyacetophenone (DEAP) were bought from J&K Scientific (Beijing, China). HEMA and DMAPS were purchased from Aladdin Ltd. (Shanghai, China). MMA and poly(ethylene glycol)dimethacrylate (PEGDMA) were purchased from Alfa Aesar (Haverhill, MA, United States). CS2 and TEA were obtained from Macklin Inc. (Shanghai, China). Deionized water (>1.82 MΩ cm, Milli-Q system, Merck & Co., Kenilworth, NJ, United States) was used. All reagents were used as received.
Preparation of CS-NiTi
The CS-NiTi was fabricated according to previous literature, with some modifications (Su et al., 2020). Firstly, pristine NiTi alloy was immersed in bis-HEMAP (5% ethanol solution) for 24 h and washed with deionized water for three times. Then, the 0.5 wt% of the photoinitiator DEAP was coated on the surface of NiTi alloy. The hydrogel precursor solution was obtained by mixing the crosslinker of PEGDMA (2 wt%) with the hydrogel monomers solution, including DMAPS (5 wt%), HEMA (40 wt%), and MMA (5 wt%). Then, it was degassed for 20 min by KQ5200DE ultrasonic cleaner (Kun Shan Ultrasonic Instruments Co. Ltd., Shanghai, China). The degassed precursor solution was poured on the surface of NiTi alloy and exposed to UV irradiation (wavelength ≈ 365 nm) in a dark chamber for 600 s by a UV LED curing system (Beijing NBET Technology Co. Ltd., Beijing, China), to obtain H-NiTi. To graft Ni-catchers on H-NiTi, H-NiTi was immersed into a mixture for 24 h containing CS2, TEA, and deionized water. LCS-NiTi, MCS-NiTi, and HCS-NiTi were obtained by regulating the concentration of CS2 (0.2, 0.6, and 1.0 mol/L), respectively.
Characterizations
The morphology of pristine NiTi, H-NiTi, and CS-NiTi were observed with S-4800 scanning electron microscope (SEM; Hitachi, Tokyo, Japan) and Single Lens Reflex camera (SLR camera; Nikon D80, Tokyo, Japan). Water contact angles (WCAs) were measured by dropping 2 μl of water on the surface of pristine NiTi, H-NiTi, and CS-NiTi by the Dataphysics OCA 20 Contact-Angle System (Filderstadt, Germany). The S element analysis was performed on ESCALAB 250Xi X-ray photoelectron spectroscopy analysis (XPS; Thermo Fisher Scientific, Waltham, MA, United States). The transmittance was measured by UV-2600 UV-VIS spectrophotometer (SHIMADZU, Kyoto, Japan) with wavelength from 450 to 700 nm.
Cell Culture and Cellular Toxicity Test
Cell Counting Kit-8 (CCK-8) assay was performed to investigate the cell viability (Xiao et al., 2020). And the cytotoxicity of materials including pristine NiTi, H-NiTi, and CS-NiTi was evaluated with HepG2 cells. HepG2 cells with the density of 1 × 105 cells per well were seeded into the 24-well plates in DMEM containing 10% fetal bovine serum (FBS) and 1% antibiotic solution (penicillin and streptomycin) and then cultured at 37 C in a humidified atmosphere with 5% CO2 for 36 h. Pristine NiTi, H-NiTi, and CS-NiTi were added to each well, and the cells were incubated for 12 h. Subsequently, CCK-8 solutions were added to the wells followed by 2-h incubation at 37°C. And then the supernatant was obtained and added to 96-well plates. Finally, microplate reader was used to measure the absorbance values per well at a test wavelength of 450 nm and a reference wavelength of 690 nm to analyze the cell viability. Control experiments were done under the same condition with PBS. Eq. (1) was used to calculate the cell viability rate:
where As represents the absorbance of the treatments of different groups and Ac represents the absorbance of the control treatments with PBS. All the experiments were performed in triplicate.
Bacteria Cultivation
Streptococcus mutans (UA159) was used as the model bacteria. S. mutans was revived by incubating its freezing solution with 1 ml of BHI at 37°C for 48 h. Then the suspension of S. mutans was centrifugated at 2,000 rpm for 10 min at room temperature to discard the supernatant. Then, the S. mutans aggregation was resuspended in another 1 ml of BHI and incubation at 37°C for another 48 h. After the logarithmic phase was reached, the bacteria were harvested by centrifugation at 2,000 rpm for 10 min at room temperature and washed with Tris–HCl buffer three times. Subsequently, the bacteria were suspended in 50 ml of Tris–HCl buffer at a final concentration of 1 × 108 CFU/ml (Liu et al., 2018).
Anti-bacteria Property
To test anti-bacteria ability, the pristine NiTi, H-NiTi, and CS-NiTi were incubated in the as-prepared bacteria suspensions for 1, 3, 6, 12, 18, and 24 h at 37°C. After the incubation, they were taken out and fixed in 2.5% glutaraldehyde for 1 h at room temperature, followed by immersing in a graded series of alcohol concentrations (30, 50, 70, 80, 90, and 100%) for 10 min to dehydrate the adhered bacteria. SEM was used to observe bacteria adhesion performance. The quantitative evaluation of the adhered bacteria was obtained by Cell Profiler 4.0.7. The anti-bacteria efficiency (Eanti–bacteria) could be calculated by Eq. (2):
where DCS–NiTi and DNiTi refer to the bacteria density on the surface of CS-NiTi and pristine NiTi, respectively.
Performance of Ni2+ Ion Capture
The performance of Ni2+ ion capture was investigated on 710-OES inductively coupled plasma emission spectrometer (ICP; Varian, Palo Alto, CA, United States) by detecting the concentration of nickel ions in the supernatant of the remaining bacteria suspension incubated with pristine NiTi, H-NiTi, LCS-NiTi, MCS-NiTi, and HCS-NiTi for different times at 37°C. The capture efficiency of Ni2+ ions (Ecapture) could be calculated by Eq. (3):
where CCS–NiTi and CH–NiTi refer to the concentration of Ni2+ ions in the supernatant of remaining bacteria suspension incubated with CS-NiTi and H-NiTi, respectively.
Results
Characterizations of CS-NiTi
To verify the introduction of Ni-catchers, we compared the pristine NiTi, H-NiTi, and CS-NiTi from surface morphology, WCAs, XPS spectra, transmittance, optical images, and biocompatibility. As shown in Figure 2A, the introduction of Ni-catchers resulted in the porous morphology of CS-NiTi, compared with the flat morphology of H-NiTi, probably because of the phase separation (Su et al., 2020). Furthermore, the WCAs decreased from 83.5 ± 3.7° (NiTi) to 59.0 ± 3.4° (CS-NiTi), indicating the hydrophilicity of CS-NiTi (Figure 2B). The change of wettability might be attributed to the formation of hydrophilic xanthates in the presence of TEA (Weißl et al., 2018) and the porous morphology of CS-NiTi. More hydrophilic surface was beneficial to the formation of the hydration layer, enhancing the anti-bacteria adhesion ability of NiTi alloys (Lin et al., 2020). XPS was further employed to verify the presence of Ni-catchers in CS-NiTi. As shown in Figure 2C and Supplementary Figure 2, two S 2p peaks at 163 and 168 eV were observed in the XPS spectrum of CS-NiTi, compared with the single S 2p peak at 168 eV for H-NiTi and no S 2p peak for NiTi. The S 2p peak at 168 eV shown in H-NiTi represented the S element in zwitterionic hydrogel network. The new S 2p peak at 163 eV indicated the successful introduction of Ni-catchers in CS-NiTi (Deng et al., 2013). Moreover, the appearance of zwitterionic hydrogels changed from colorless to yellow with lower transmittance after Ni-catcher (CS2) graft (Figure 2D), for xanthates always appear as a series of yellow compounds. The transmittance of Ni-catcher-doped zwitterionic hydrogel coating was higher than 60%, which has an insignificant impact on the appearance of NiTi alloys and would not affect the application of NiTi alloys in the implantable biomedical field. Furthermore, we tested the biocompatibility of CS-NiTi using CCK-8 test (Xiao et al., 2020). Higher HepG2 cell viability in Figure 2E indicated the excellent biocompatibility of CS-NiTi, showing promising applicability in biomedical field. Therefore, we successfully fabricated CS-NiTi with good biocompatibility.
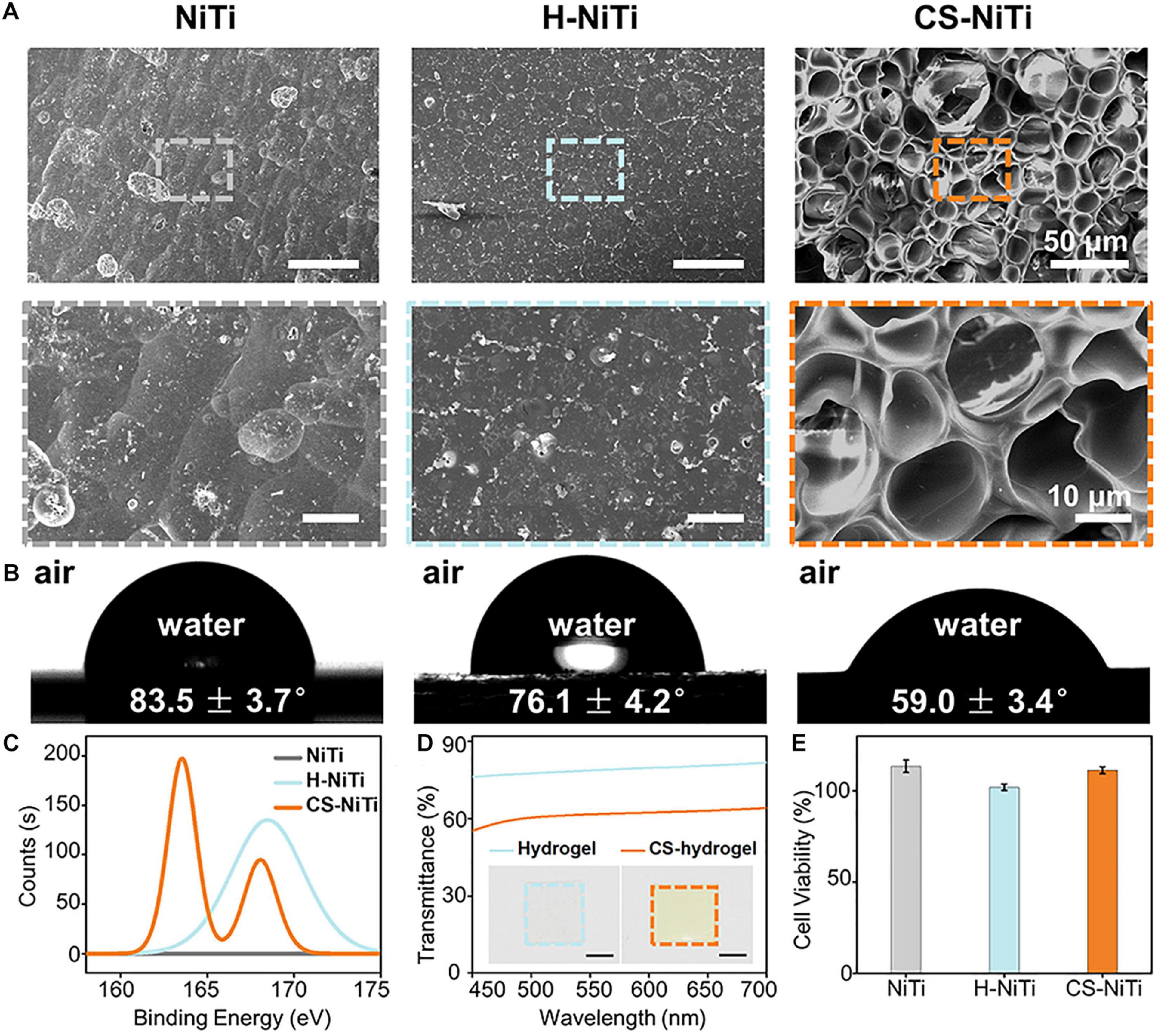
Figure 2. Characterizations of CS-NiTi. SEM images (A), water contact angles (WCAs; B), X-ray photoelectron spectroscopy (XPS) spectra (C), transmittance, (D) and biocompatibility (E) of pristine NiTi, H-NiTi, and CS-NiTi. The insets in (D) show the optical images of zwitterionic hydrogel and CS-hydrogel. Scale bar, 5 mm for (D).
Anti-bacteria Property of CS-NiTi
As a kind of widely used orthodontic material, NiTi alloys are often exposed to the oral environment. However, cleaning teeth is difficult during orthodontic treatment, leading to the adhesion of bacteria and causing caries and periodontitis. To investigate the anti-bacteria property in the oral environment, CS-NiTi was incubated in 1 × 108 CFU/ml bacteria suspension (S. mutans, the largest proportion of bacteria in oral environment) for different times, taking pristine NiTi and H-NiTi as controls. As shown in Figure 3A and Supplementary Figure 3, many bacteria adhered on the surface of pristine NiTi after being incubated for 1 h. In contrast, there were a few bacteria on the surfaces of H-NiTi and CS-NiTi, indicating their good anti-bacteria properties. By prolonging incubation time, a growing number of bacteria adhered on the surface of pristine NiTi and formed a biofilm at 12 h. In contrast, the bacteria density was still very low on the surfaces of H-NiTi and CS-NiTi. The quantitative analysis in Figure 3B revealed a good anti-bacteria efficiency in H-NiTi and CS-NiTi at 6 h, showing the highest efficiency of ca. 84% for H-NiTi and ca. 81% for CS-NiTi. By prolonging the incubation time to 24 h, the anti-bacteria efficiency maintained higher than 72% for H-NiTi and 66% for CS-NiTi. These results indicated that CS-NiTi had excellent long-term anti-bacteria property. Moreover, ICP was employed for exploring the performance of Ni2+ ion capture by monitoring the concentration of Ni2+ ions in bacteria suspension (1 × 108 CFU/ml). As shown in Figure 3C, the concentration of Ni2+ ions in CS-NiTi was significantly lower than that of NiTi and H-NiTi. Even though it was incubated for 24 h, the concentration of Ni2+ ions for CS-NiTi was still as low as 0.38 ± 0.07 mg/L, indicating the excellent ability of Ni2+ ion capture of CS-NiTi. Therefore, CS-NiTi showed dual properties of anti-bacteria and Ni2+ ion capture at the same time.
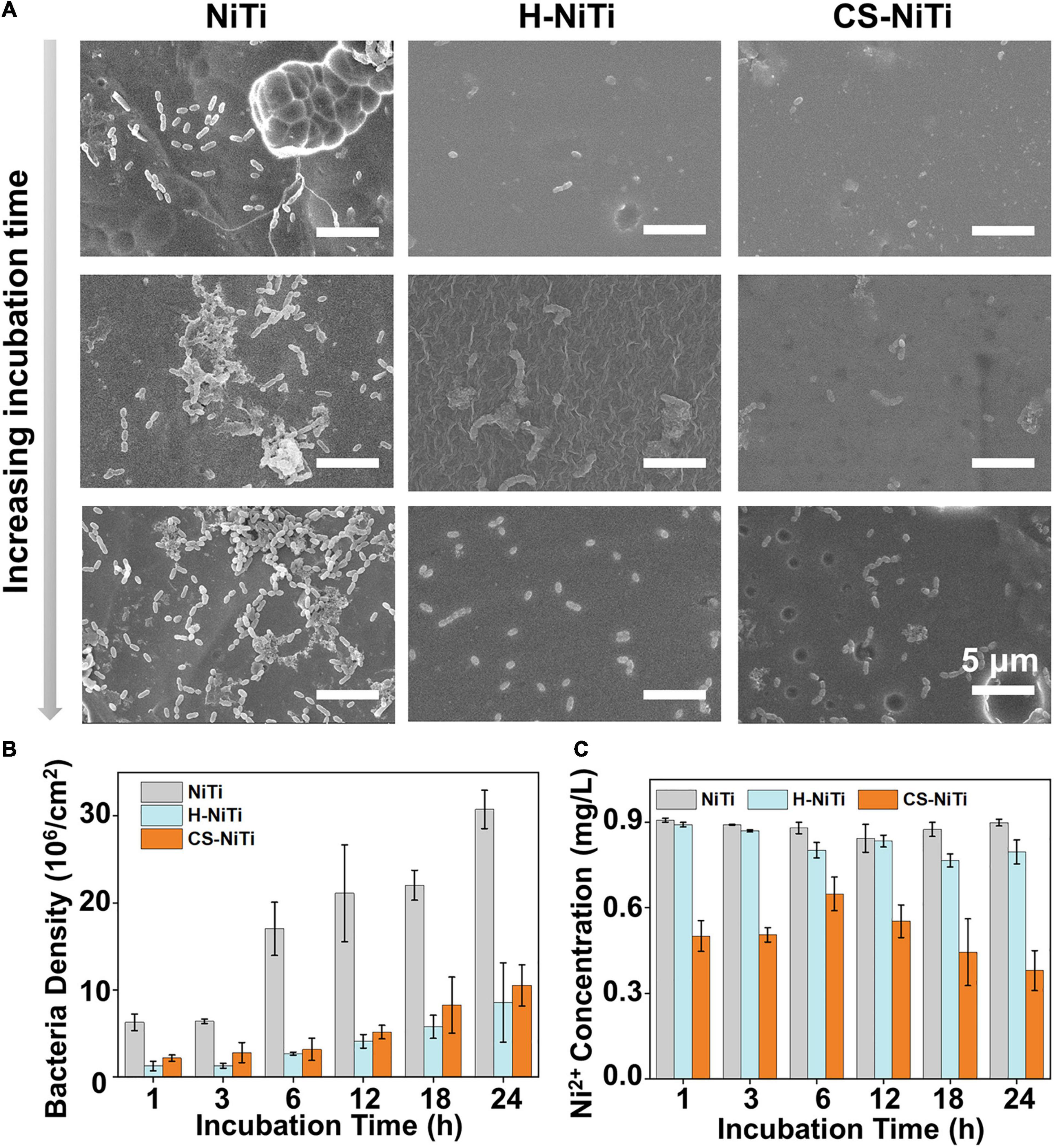
Figure 3. The anti-bacteria property of CS-NiTi. (A) SEM images of pristine NiTi, H-NiTi, and CS-NiTi after being incubated with bacteria suspension for different times (1, 12, and 24 h). The quantitative analysis of bacteria adhesion (B) and Ni2+ ion capture performance (C) on the surface of pristine NiTi, H-NiTi, and CS-NiTi at different incubation times.
Mechanism of Ni2+ Ion Capture
To further investigate the performance of Ni2+ ion capture, a series of CS-NiTi were fabricated by regulating the CS2 concentration in the mixed solution, that is, 0.2 mol/L of CS2 for low concentration (denoted as LCS-NiTi), 0.6 mol/L of CS2 for medium concentration (MCS-NiTi), and 1.0 mol/L of CS2 for high concentration (HCS-NiTi). With the increasing concentration of Ni-catchers, the surface became more hydrophilic, revealing by the WCAs changing from 73.0 ± 2.8° to 59.0 ± 3.4°, along with the increased pore size (Supplementary Figures 4A,B). Furthermore, the appearance of CS-hydrogel changed from colorless with high transparency to yellow with reduced transparency (Supplementary Figure 4C). To verify the long-term ability of Ni2+ ion capture, we incubated H-NiTi, LCS-NiTi, MCS-NiTi, and HCS-NiTi with S. mutans suspensions for different days. After the incubation, the concentration of leaked Ni2+ ions from NiTi alloy was reduced from 0.91 ± 0.01 mg/L (H-NiTi) to 0.65 ± 0.02 mg/L (HCS-NiTi) with the incubation time of 1 day (Figures 4A–D). By prolonging the incubation time to 5 days, the concentration of leaked Ni2+ ions increased to 1.12 ± 0.03 mg/L for H-NiTi gradually. On the contrary, the concentration of leaked Ni2+ ions in HCS-NiTi reduced from 0.65 ± 0.02 to 0.48 ± 0.07 mg/L dramatically, indicating its excellent long-term property of Ni2+ ion capture.
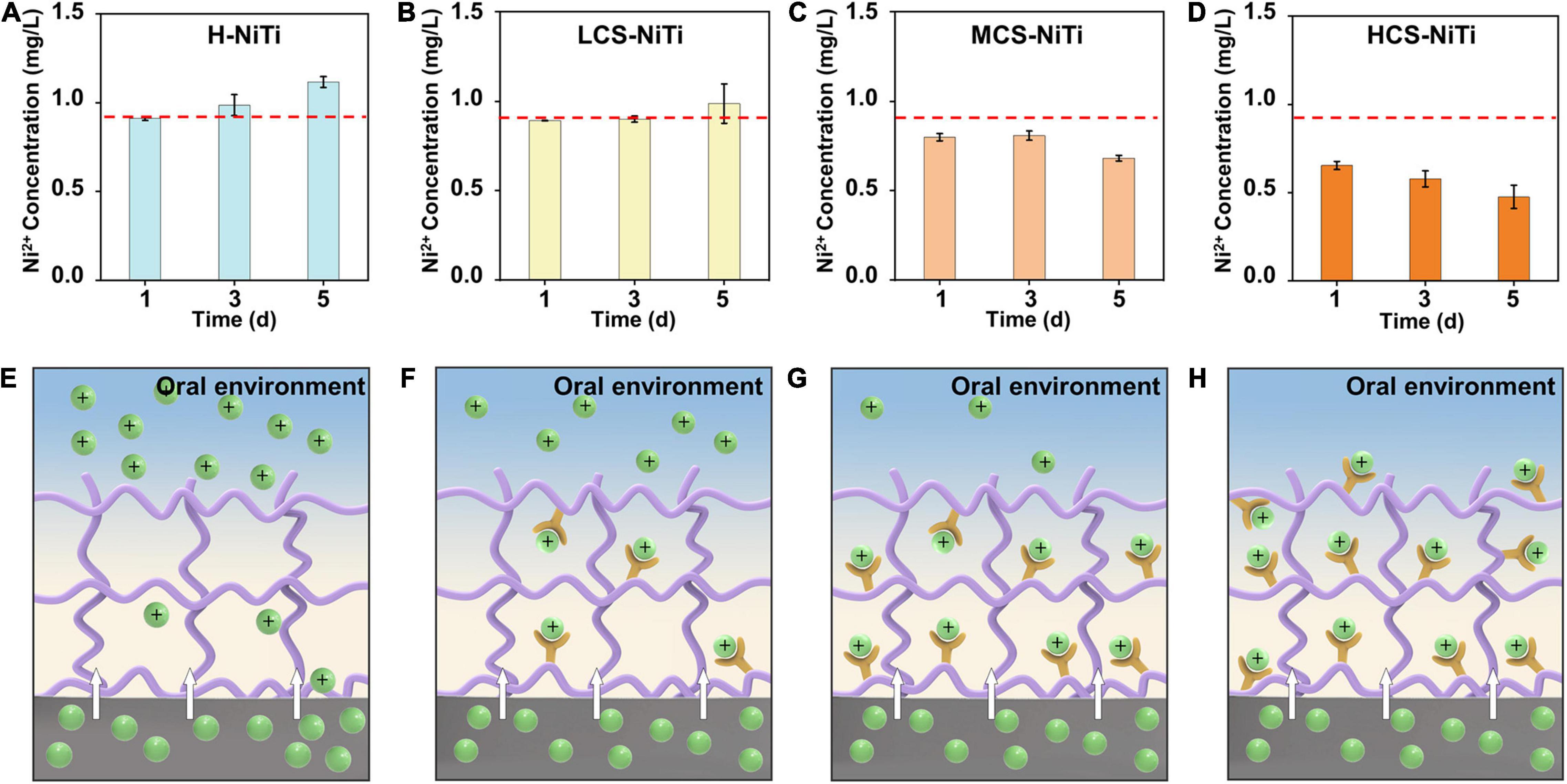
Figure 4. The mechanism of Ni2+ ion capture of CS-NiTi. (A–D) The quantitative analysis of Ni2+ ion capture performance of H-NiTi, LCS-NiTi, MCS-NiTi, and HCS-NiTi. (E–H) Schematic of Ni2+ ion capture property of CS-NiTi with different concentrations of Ni-catchers.
The possible mechanism of Ni2+ ion capture is proposed in Figures 4E–H. Due to the corrosion of the adhered bacteria and acidic oral microenvironment, Ni2+ ions are released slowly and persistently from NiTi alloy (Huang et al., 2003). Briefly, the leaked Ni2+ ions can pass through the zwitterionic hydrogel coating on the surface of NiTi alloy and be released to oral environment easily (Figure 4E), as the diffusion of Ni2+ ions is not efficiently restricted in single zwitterionic hydrogel. In contrast, the concentration of Ni2+ ions is dramatically decreased after introducing Ni-catchers because grafted CS2 can capture free Ni2+ ions and restrain their diffusion to oral environment (Figures 4F–H). Moreover, the long-term capture efficiency of Ni2+ ions is improved with the increasing concentration of Ni-catchers. Therefore, the introduction of Ni-catchers endows CS-NiTi with robust Ni2+ ion capture property.
Discussion
In summary, we demonstrate a long-term Ni2+ ion capture coating on the surface of NiTi alloy. The zwitterionic hydrogels and Ni-catchers endow CS-NiTi with excellent anti-bacteria and Ni2+ ion capture properties. The CS-NiTi exhibited long-term anti-bacteria efficiency of ca. 66% at 24 h and Ni2+ ion capture efficiency of ca. 57% at 5 days. Furthermore, by integrating chelation reaction and introducing color change, this coating may apply to Ni2+ ion capture and detection. Therefore, this coating provides a new clue to design the next generation of biomaterials and devices with capture and detection of Ni2+ ions.
Data Availability Statement
The raw data supporting the conclusions of this article will be made available by the authors, without undue reservation.
Author Contributions
QN and JM conceived the idea. DH and XF contributed the fabrication process. XF and WX performed the experiments. XF and XL analyzed the results. XF, XL, QN, and JM wrote the manuscript. All authors contributed to the article and approved the submitted version.
Funding
This research is supported by the National Natural Science Foundation of China (Nos. 51973003, 21875269, and 21988102), National Key R&D Program of China (Nos. 2019YFA0709300 and 2018YFC1105301), and Youth Innovation Promotion Association, CAS (No. 2017036).
Conflict of Interest
The authors declare that the research was conducted in the absence of any commercial or financial relationships that could be construed as a potential conflict of interest.
Supplementary Material
The Supplementary Material for this article can be found online at: https://www.frontiersin.org/articles/10.3389/fbioe.2021.698745/full#supplementary-material
References
Deng, M., Karpuzov, D., Liu, Q., and Xu, Z. (2013). Cryo-XPS study of xanthate adsorption on pyrite. Surf. Interface Anal. 45, 805–810. doi: 10.1002/sia.5165
Gu, Y. W., Tay, B. Y., Lim, C. S., and Yong, M. S. (2005). Characterization of bioactive surface oxidation layer on NiTi alloy. Appl. Surf. Sci. 252, 2038–2049. doi: 10.1016/j.apsusc.2005.03.207
Hang, R., Liu, S., Liu, Y., Zhao, Y., Bai, L., Jin, M., et al. (2019). Preparation, characterization, corrosion behavior and cytocompatibility of NiTiO3 nanosheets hydrothermally synthesized on biomedical NiTi alloy. Mater. Sci. Eng. C 97, 715–722. doi: 10.1016/j.msec.2018.12.124
Hang, R., Ma, S., and Chu, P. K. (2012). Effects of diamond-like carbon coatings with different thickness on mechanical properties and corrosion behavior of biomedical NiTi alloy. Rare Metal Mater. Eng. 41, 1505–1510. doi: 10.1016/s1875-5372(13)60001-6
Hartono, M. R., Kushmaro, A., Chen, X., and Marks, R. S. (2018). Probing the toxicity mechanism of multiwalled carbon nanotubes on bacteria. Environ. Sci. Pollut. Res. 25, 5003–5012. doi: 10.1007/s11356-017-0782-8
Huang, H. H., Chiu, Y. H., Lee, T. H., Wu, S. C., Yang, H. W., Su, K. H., et al. (2003). Ion release from NiTi orthodontic wires in artificial saliva with various acidities. Biomaterials 24, 3585–3592. doi: 10.1016/s0142-9612(03)00188-1
Jia, W., Beatty, M. W., Reinhardt, R. A., Petro, T. M., Cohen, D. M., Maze, C. R., et al. (1999). Nickel release from orthodontic arch wires and cellular immune response to various nickel concentrations. J. Biomed. Mater. Res. 48, 488–495. doi: 10.1002/(sici)1097-4636(1999)48:4<488::aid-jbm14<3.0.co;2-d
Kolokitha, O. E. G., and Chatzistavrou, E. (2008). Allergic reactions to nickel-containing orthodontic appliances: clinical signs and treatment alternatives. World J. Orthod. 9, 399–406.
Li, G., Cheng, G., Xue, H., Chen, S., Zhang, F., and Jiang, S. (2008). Ultra low fouling zwitterionic polymers with a biomimetic adhesive group. Biomaterials 29, 4592–4597. doi: 10.1016/j.biomaterials.2008.08.021
Lin, X., Wu, K., Zhou, Q., Jain, P., Boit, M. O., Li, B., et al. (2020). Photoreactive carboxybetaine copolymers impart biocompatibility and inhibit plasticizer leaching on polyvinyl chloride. ACS Appl. Mater. Interfaces 12, 41026–41037. doi: 10.1021/acsami.0c09457
Liu, R., Liu, X., Zhou, J., Nie, Q., Meng, J., Lin, J., et al. (2019). Bioinspired superhydrophobic Ni-Ti archwires with resistance to bacterial adhesion and nickel ion release. Adv. Mater. Interfaces 6:1801569. doi: 10.1002/admi.201801569
Liu, X., Peng, L., Meng, J., Zhu, Z., Han, B., and Wang, S. (2018). Protein-mediated anti-adhesion surface against oral bacteria. Nanoscale 10, 2711–2714. doi: 10.1039/c7nr08844b
Peng, L., Chang, L., Liu, X., Lin, J., Liu, H., Han, B., et al. (2017). Antibacterial property of a polyethylene glycol-grafted dental material. ACS Appl. Mater. Interfaces 9, 17688–17692. doi: 10.1021/acsami.7b05284
Poon, R. W. Y., Yeung, K. W. K., Liu, X. Y., Chu, P. K., Chung, C. Y., Lu, W. W., et al. (2005). Carbon plasma immersion ion implantation of nickel-titanium shape memory alloys. Biomaterials 26, 2265–2272. doi: 10.1016/j.biomaterials.2004.07.056
Raphel, J., Holodniy, M., Goodman, S. B., and Heilshorn, S. C. (2016). Multifunctional coatings to simultaneously promote osseointegration and prevent infection of orthopaedic implants. Biomaterials 84, 301–314. doi: 10.1016/j.biomaterials.2016.01.016
Robertson, S. W., and Ritchie, R. O. (2007). In vitro fatigue-crack growth and fracture toughness behavior of thin-walled superelastic Nitinol tube for endovascular stents: a basis for defining the effect of crack-like defects. Biomaterials 28, 700–709. doi: 10.1016/j.biomaterials.2006.09.034
Shivapooja, P., Yu, Q., Orihuela, B., Mays, R., Rittschof, D., Genzer, J., et al. (2015). Modification of silicone elastomer surfaces with zwitterionic polymers: short-term fouling resistance and triggered biofouling release. ACS Appl. Mater. Interfaces 7, 25586–25591. doi: 10.1021/acsami.5b09199
Su, X., Hao, D., Xu, X., Guo, X., Li, Z., and Jiang, L. (2020). Hydrophilic/hydrophobic heterogeneity anti-biofouling hydrogels with well-regulated rehydration. ACS Appl. Mater. Interfaces 12, 25316–25323. doi: 10.1021/acsami.0c05406
Sunderman, F. W. (1990). Use of sodium diethyldithiocarbamate in the treatment of nickel carbonyl poisoning. Ann. Clin. Lab. Sci. 20, 12–21.
Sylvestre, M., Crane, C. A., and Pun, S. H. (2020). Progress on modulating tumor-associated macrophages with biomaterials. Adv. Mater. 32:1902007. doi: 10.1002/adma.201902007
Wei, T., Zhan, W., Cao, L., Hu, C., Qu, Y., Yu, Q., et al. (2016). Multifunctional and regenerable antibacterial surfaces fabricated by a universal strategy. ACS Appl. Mater. Interfaces 8, 30048–30057. doi: 10.1021/acsami.6b11187
Wei, T., Zhan, W., Yu, Q., and Chen, H. (2017). Smart biointerface with photoswitched functions between bactericidal activity and bacteria-releasing ability. ACS Appl. Mater. Interfaces 9, 25767–25774. doi: 10.1021/acsami.7b06483
Weißl, M., Niegelhell, K., Reishofer, D., Zankel, A., Innerlohinger, J., and Spirk, S. (2018). Homogeneous cellulose thin films by regeneration of cellulose xanthate: properties and characterization. Cellulose 25, 711–721. doi: 10.1007/s10570-017-1576-3
Wu, S. L., Chu, P. K., Liu, X. M., Chung, C. Y., Ho, J. P. Y., Chu, C. L., et al. (2006). Surface characteristics, mechanical properties, and cytocompatibility of oxygen plasma-implanted porous nickel titanium shape memory alloy. J. Biomed. Mater. Res. A 79A, 139–146. doi: 10.1002/jbm.a.30705
Keywords: nickel ion capture, anti-bacteria adhesion, zwitterionic hydrogel, surface modification, nickel–titanium alloy
Citation: Fu X, Liu X, Hao D, Xiao W, Nie Q and Meng J (2021) Nickel-Catcher-Doped Zwitterionic Hydrogel Coating on Nickel–Titanium Alloy Toward Capture and Detection of Nickel Ions. Front. Bioeng. Biotechnol. 9:698745. doi: 10.3389/fbioe.2021.698745
Received: 22 April 2021; Accepted: 25 May 2021;
Published: 24 June 2021.
Edited by:
Tailin Xu, Shenzhen University, ChinaReviewed by:
Feilong Zhang, Nanyang Technological University, SingaporeZhen Gu, University of Science and Technology Beijing, China
Li Huizeng, Institute of Chemistry (CAS), China
Copyright © 2021 Fu, Liu, Hao, Xiao, Nie and Meng. This is an open-access article distributed under the terms of the Creative Commons Attribution License (CC BY). The use, distribution or reproduction in other forums is permitted, provided the original author(s) and the copyright owner(s) are credited and that the original publication in this journal is cited, in accordance with accepted academic practice. No use, distribution or reproduction is permitted which does not comply with these terms.
*Correspondence: Qiong Nie, bmllcWlvbmdAYmptdS5lZHUuY24=; Jingxin Meng, bWVuZ2p4NjI4QG1haWwuaXBjLmFjLmNu
†These authors have contributed equally to this work and share first authorship