- 1Division of Cell Biology, Histology and Embryology, Gottfried Schatz Research Center, Medical University of Graz, Graz, Austria
- 2Institute of Cell Biology, Faculty of Medicine, University of Ljubljana, Ljubljana, Slovenia
- 3Group for Hematology and Stem Cells, Institute for Medical Research, University of Belgrade, Belgrade, Serbia
- 4LAQV, REQUIMTE, Department of Chemical Sciences‐Applied Chemistry Lab, Faculty of Pharmacy, University of Porto, Porto, Portugal
- 5iBB–Institute for Bioengineering and Biosciences, Department of Bioengineering, Instituto Superior Técnico, Universidade de Lisboa, Lisboa, Portugal
- 6Associate Laboratory i4HB–Institute for Health and Bioeconomy at Instituto Superior Técnico, Universidade de Lisboa, Lisboa, Portugal
- 7Center for Marine Sciences (CCMar), Faculty of Sciences and Technology, University of Algarve, Faro, Portugal
- 8Department of Chemistry and Pharmacy, Faculty of Sciences and Technology, University of Algarve, Faro, Portugal
- 9Department of Obstetrics and Feto-maternal Medicine, Inselspital, Bern University Hospital, University of Bern, Bern, Switzerland
- 10Department for BioMedical Research (DBMR), University of Bern, Bern, Switzerland
Knowledge of the beneficial effects of perinatal derivatives (PnD) in wound healing goes back to the early 1900s when the human fetal amniotic membrane served as a biological dressing to treat burns and skin ulcerations. Since the twenty-first century, isolated cells from perinatal tissues and their secretomes have gained increasing scientific interest, as they can be obtained non-invasively, have anti-inflammatory, anti-cancer, and anti-fibrotic characteristics, and are immunologically tolerated in vivo. Many studies that apply PnD in pre-clinical cutaneous wound healing models show large variations in the choice of the animal species (e.g., large animals, rodents), the choice of diabetic or non-diabetic animals, the type of injury (full-thickness wounds, burns, radiation-induced wounds, skin flaps), the source and type of PnD (placenta, umbilical cord, fetal membranes, cells, secretomes, tissue extracts), the method of administration (topical application, intradermal/subcutaneous injection, intravenous or intraperitoneal injection, subcutaneous implantation), and the type of delivery systems (e.g., hydrogels, synthetic or natural biomaterials as carriers for transplanted cells, extracts or secretomes). This review provides a comprehensive and integrative overview of the application of PnD in wound healing to assess its efficacy in preclinical animal models. We highlight the advantages and limitations of the most commonly used animal models and evaluate the impact of the type of PnD, the route of administration, and the dose of cells/secretome application in correlation with the wound healing outcome. This review is a collaborative effort from the COST SPRINT Action (CA17116), which broadly aims at approaching consensus for different aspects of PnD research, such as providing inputs for future standards for the preclinical application of PnD in wound healing.
Introduction
Human skin is the largest organ of the body that provides complex functions. It protects the body against mechanical, chemical, and physical impact, as well as dehydration. In addition, it modulates the body temperature and serves as a sensory organ. The compact part of the skin, the cutis, consists of the epidermis, a keratinized stratified squamous epithelium, and the underlying dermis, which is built from connective tissue. Below the cutis is the loosely-layered subcutis, which is enriched by adipose tissue.
Severe skin injury is the result of wounds caused by incision, excision, abrasion, burn, radiation or pressure. The degree of wounding describes the depth of damage to the respective skin layers. In first-degree wounds, only the epidermis is affected, in second-degree wounds, the epidermis and dermis are affected, and in third-degree wounds, all three skin layers including the subcutis are affected.
Skin damage is repaired by wound healing, a multistep, finely orchestrated process that includes hemostasis, inflammation, tissue growth (proliferation), and tissue remodeling (maturation) (Xue et al., 2018). This process is highly efficient in healthy individuals. However, the type, extent, and depth of injury, as well as any deviations in the fragile wound repair response, affect the healing outcome. Parameters such as aging, comorbidities (e.g., diabetes, obesity, arterial or venous insufficiencies, autoimmune diseases), and severe burn injuries constitute some of the causes that delay wound healing, often due to insufficient blood supply based on impaired wound revascularization (Demidova-Rice et al., 2012). Non-healing wounds that persist for more than 3 months are called chronic wounds. These types of wounds have failed to proceed through an orderly and timely reparative process to produce anatomic and functional integrity of the injured site (Lazarus et al., 1994) and are often detained in a self-perpetuating inflammatory stage that hinders progression to proliferation (Stojadinovic et al., 2008). Approximately 40 million patients worldwide suffer from chronic wounds, which are still a challenge to treat and constitute a significant financial burden on the health care system (Sen et al., 2009). Thus, new therapeutic approaches for wound healing are highly warranted.
A promising strategy for wound treatment is the application of human perinatal derivatives (PnD). PnD are birth-associated tissues such as the placenta and its annexes (human amniotic membrane (hAM), chorionic membrane, decidua, umbilical cord) and the amniotic fluid. PnD are an abundant source of extracellular matrix proteins, growth factors, and cells that have already been used in a wide variety of applications in tissue engineering and regenerative medicine (Deus et al., 2020). The application of the hAM has a long tradition in the treatment of wounds. Early studies from 1909 to 1913 reported successful use of the hAM in skin transplantation (Davis, 1909) and for treating burns and skin ulcerations as a biological dressing. Its use significantly reduced pain and increased the epithelialization rate of the traumatized skin without signs of infection (Sabella, 1913; Stern, 1913). Several clinical trials in the subsequent decades confirmed the successful use of the hAM for skin injuries (Silini et al., 2015). The twenty-first century brought the breakthrough for cell isolations from different placental regions and the progressive investigation of their therapeutic potential. Minimal criteria for the definition of PnD derived cells were described in 2008 (Parolini et al., 2008), and ongoing extensive characterization of PnD was performed (Caruso et al., 2012; Silini et al., 2020).
PnD derived cells have anti-inflammatory, immunomodulatory, anti-cancer, anti-fibrotic, anti-apoptotic, and anti-oxidant effects. They are immunologically tolerated in vivo and have been transplanted without signs of immunological rejection, meaning that the application does not require immunosuppressive treatment (Ueta et al., 2002; Bailo et al., 2004; Jirsova and Jones, 2017).
With the expanding knowledge that cells act by paracrine mechanisms, the released secretomes (cell-derived conditioned media, cell-derived extracellular vesicles) are gaining increasing interest. Placental cells secrete factors that are crucial for wound healing such as EGF, IL-8, and IGF-1, which modulate migration and proliferation of keratinocytes, fibroblasts, and endothelial cells (Kim et al., 2012). hAM mesenchymal stromal cells (hAMSC) and their secretomes exhibit beneficial, survival-enhancing effects on endothelial cells in vitro and were shown to stabilize endothelial networks in the Matrigel assay (König et al., 2012; König et al., 2015). Further, co-transplantation of hAMSC and placental endothelial cells enabled the formation of human capillaries connecting to the murine blood circulation in a mouse model in vivo (Kinzer et al., 2014).
As the formation of new blood vessels is critical for normal wound healing, innovations to improve wound revascularization could also lead to significant advances in wound healing therapy and patient care (Demidova-Rice et al., 2012). Improved techniques for tissue preservation, and recent advances in isolation and culture procedures for PnD derived cells paved the way for established clinical uses and investigative pre-clinical and clinical trials such as the application of placenta derived mesenchymal stromal-like cells (PDA-002) for the treatment of patients with diabetic foot ulcer wounds and peripheral arterial disease (Silini et al., 2015)1.
This review provides a thorough overview of the application of PnD in wound healing to assess its efficacy in preclinical animal models based on different wound types. We highlight the advantages and limitations of the most commonly used animal models and evaluate the impact of the type of PnD, the delivery method and the dose of cells/secretome application in correlation with the wound healing outcome.
Methods and Search Strategy to Collect the Data
We performed a systematic literature search of the PubMed® database covering a period from 2004 to 2020 and used the Boolean search string (Supplements) in accordance with the consensus of the scientific network of the COST SPRINT Action (CA17116) to identify articles exploring therapeutic options of PnD in in vivo experimental models of wound healing. Titles and abstracts were screened to select publications including in vivo models evaluating the efficacy of PnD. The search was limited to original research publications available as full text in English. The publications were cross-checked for meeting the inclusion criteria by an independent study. The selection workflow was compliant with the PRISMA guidelines (Page et al., 2021a; Page et al., 2021b).
We obtained 141 manuscripts investigating the application of PnD to animal wound- or angiogenic models. We further focused on studies of cutaneous wound healing, which used human PnD. Articles with insufficient data (insufficient group sizes, no statistics, no controls, no adequate figures) were excluded. After the screening process, we identified 79 relevant articles. We observed a continuous increase in publications on the application of PnD in animal models of cutaneous wound healing during the last decade. The major part of these studies was performed in Asia (China 41%, Iran 15%, rest of Asia 22%), followed by the United States and Canada (11%), Europe (6%), South America (4%) and Africa (Egypt 1%). From the relevant articles, we extracted data concerning animal species (rodents, large animals), diabetic status (diabetic or non-diabetic animals), the type of wounds (full-thickness wounds, burns, radiation-induced wounds, skin flaps, subcutaneous pockets), the source and type of PnD (placenta, umbilical cord, fetal membranes, cells, secretomes, tissue extracts), the method and dose of administration (topical application, intradermal/subcutaneous injection, intravenous or intraperitoneal injection, subcutaneous implantation) and the use of delivery systems (e.g., hydrogels, synthetic or natural biomaterials as carriers for transplanted cells, extracts or secretomes). Data extraction was performed to meet the populations, interventions, comparators, outcomes and study designs (PICOS) criteria (McKenzie et al., 2021).
PnD Used for Cutaneous Wound Healing in Preclinical Studies
Types, Dosage and Application Mode of PnD
In the papers included in this review, PnD were applied in the form of cells, cell secretomes (cell-derived conditioned media (CM), cell-derived small extracellular vesicles (sEV), tissue membranes and tissue extracts (Figure 1). Naming and abbreviations of the PnD types in the reviewed studies varied due to the authors’ discretion. To improve the comparability of data, we harmonized terms according to the recently published consensus nomenclature for perinatal tissues and cells (Silini et al., 2020). For example, as there is no consensus on the zones of Wharton’s jelly and on the experimental protocols for isolation of the cells thereof, we have used the single term human umbilical cord mesenchymal stromal cells (hUC-MSC), which comprises all potential cell subpopulations. The types and combinations of the PnD applied in these studies as well as the delivery systems are outlined in detail in Tables 1–5. Overall, in 14 of 79 studies, two different PnD types were addressed. The authors either compared the effect of different PnD (e.g., cells vs. cell-derived CM, cells vs. cell-derived sEV, cells vs. tissue), or two PnD types were applied in combination (different cell types, cells and cell-derived CM, cells and tissue).
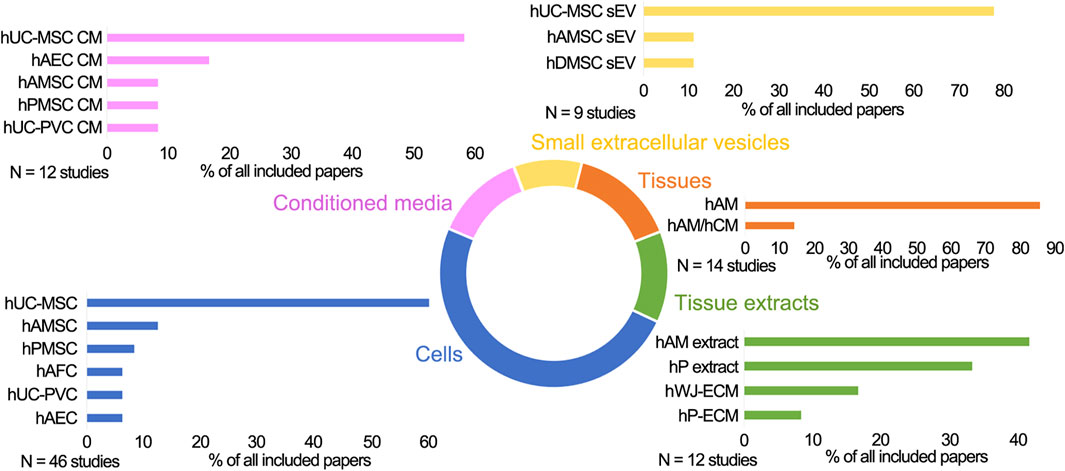
FIGURE 1. The schematic presentation of the perinatal derivatives used in skin wound healing in preclinical studies. Cells: hUC-MSC, human umbilical cord mesenchymal stromal cells; hAMSC, human amniotic membrane mesenchymal stromal cells; hPMSC, human placenta mesenchymal stromal cells; hUC-PVC, human umbilical cord perivascular cells; hAEC, human amniotic membrane epithelial cells; hAFSC, human amniotic fluid stem cells; hAFC, human amniotic fluid cells; Conditioned media (CM) derived from hUC-MSC, hAEC, hAMSC, hPMSC, hUC-PVC; Small extracellular vesicles (sEV) derived from hUC-MSC, hAMSC, hDMSC; Tissues: hAM, human amniotic membrane; hAM/hCM, human amniotic/chorionic membrane; Tissue extracts derived from hAM, hP (human placenta), hP-ECM (human placenta-derived extracellular matrix), hWJ-ECM (human Wharton’s jelly derived extracellular matrix).
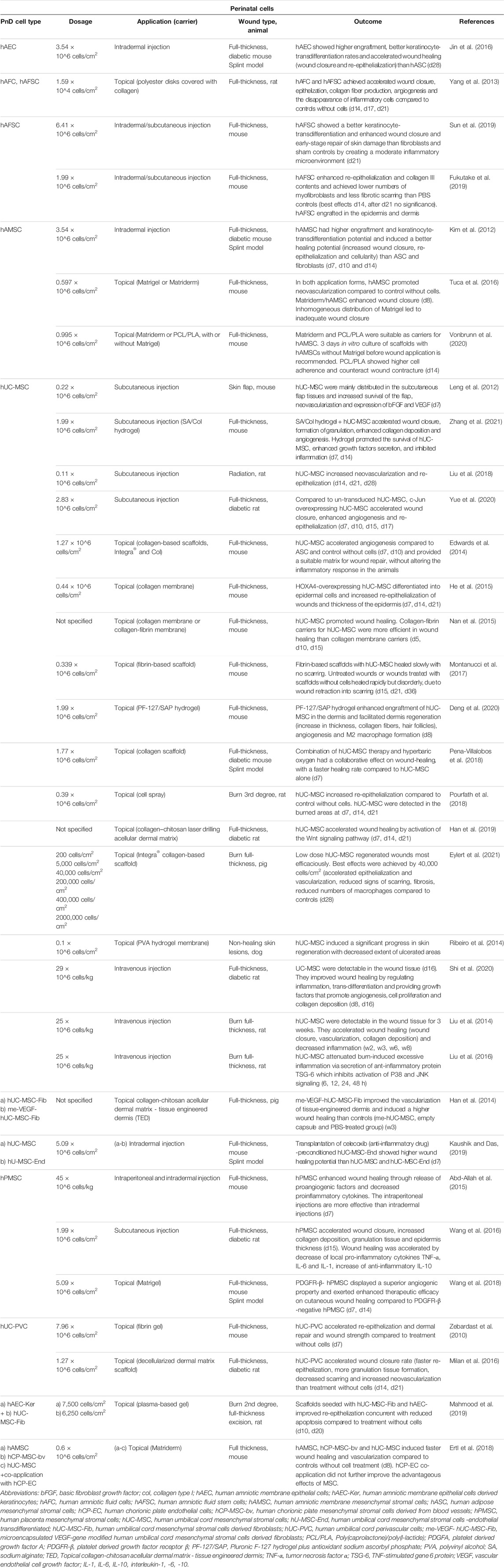
TABLE 1. Application of perinatal cells in in vivo animal models of skin wound healing. Time points indicated in the “Outcome” column mean days (d), weeks (w) or hours (h) after treatment.
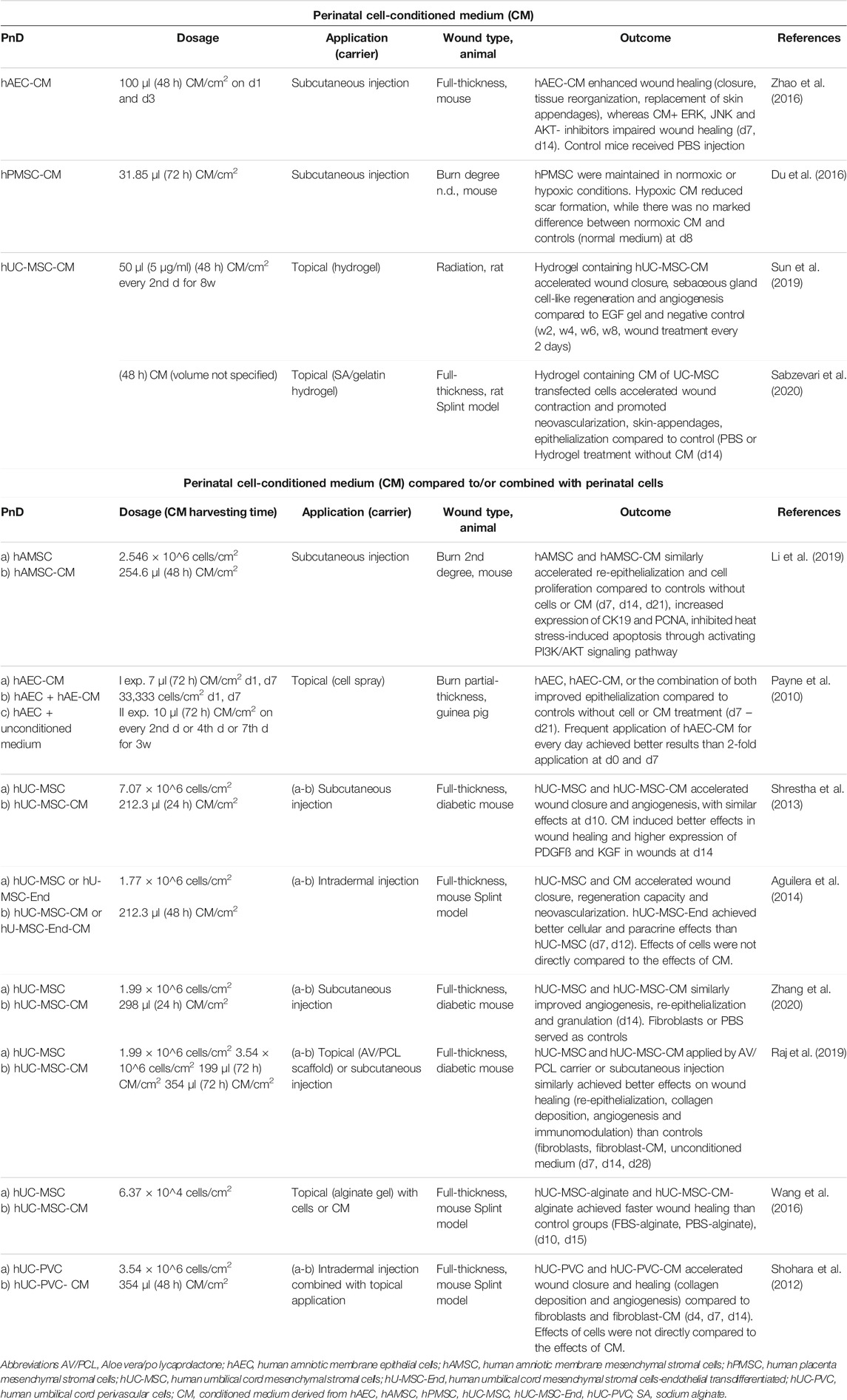
TABLE 2. Application of perinatal cell-conditioned medium (CM) alone or compared to/combined with perinatal cells in in vivo animal models of skin wound healing. Time points indicated in the “Dosage” and Outcome” columns mean days (d), weeks (w) or hours (h) of/after treatment.
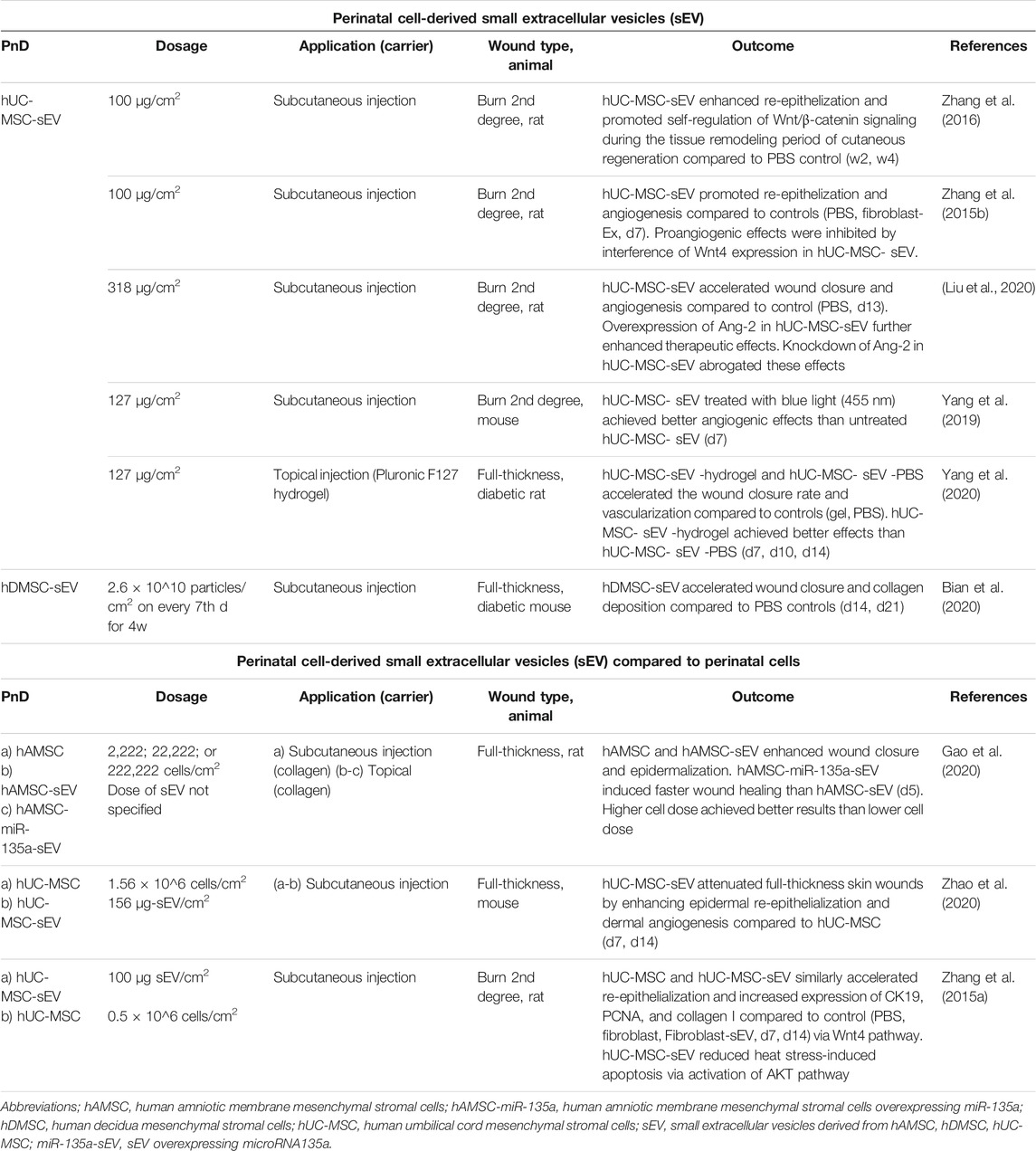
TABLE 3. Application of perinatal cell-derived small extracellular vesicles (sEV) alone or compared to perinatal cells in in vivo animal models of skin wound healing. Time points indicated in the “Dosage” and “Outcome” columns mean days (d) or weeks (w) of/after treatment.
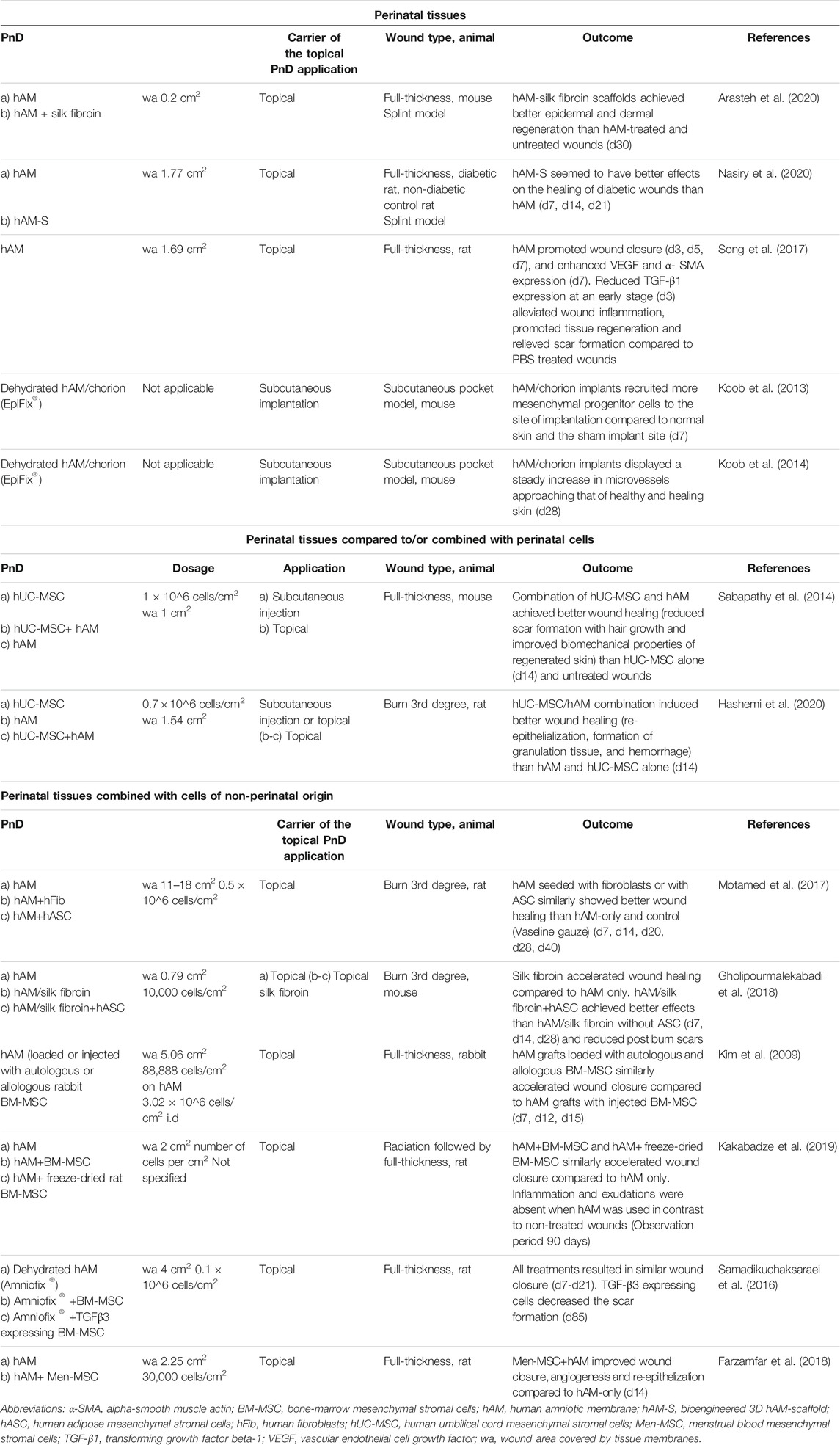
TABLE 4. Application of perinatal tissues alone or compared to/combined with perinatal cells or cells of non-perinatal origin in in vivo animal models of skin wound healing. Stated time points in the “Outcome” column mean days (d) or weeks (w) after treatment.
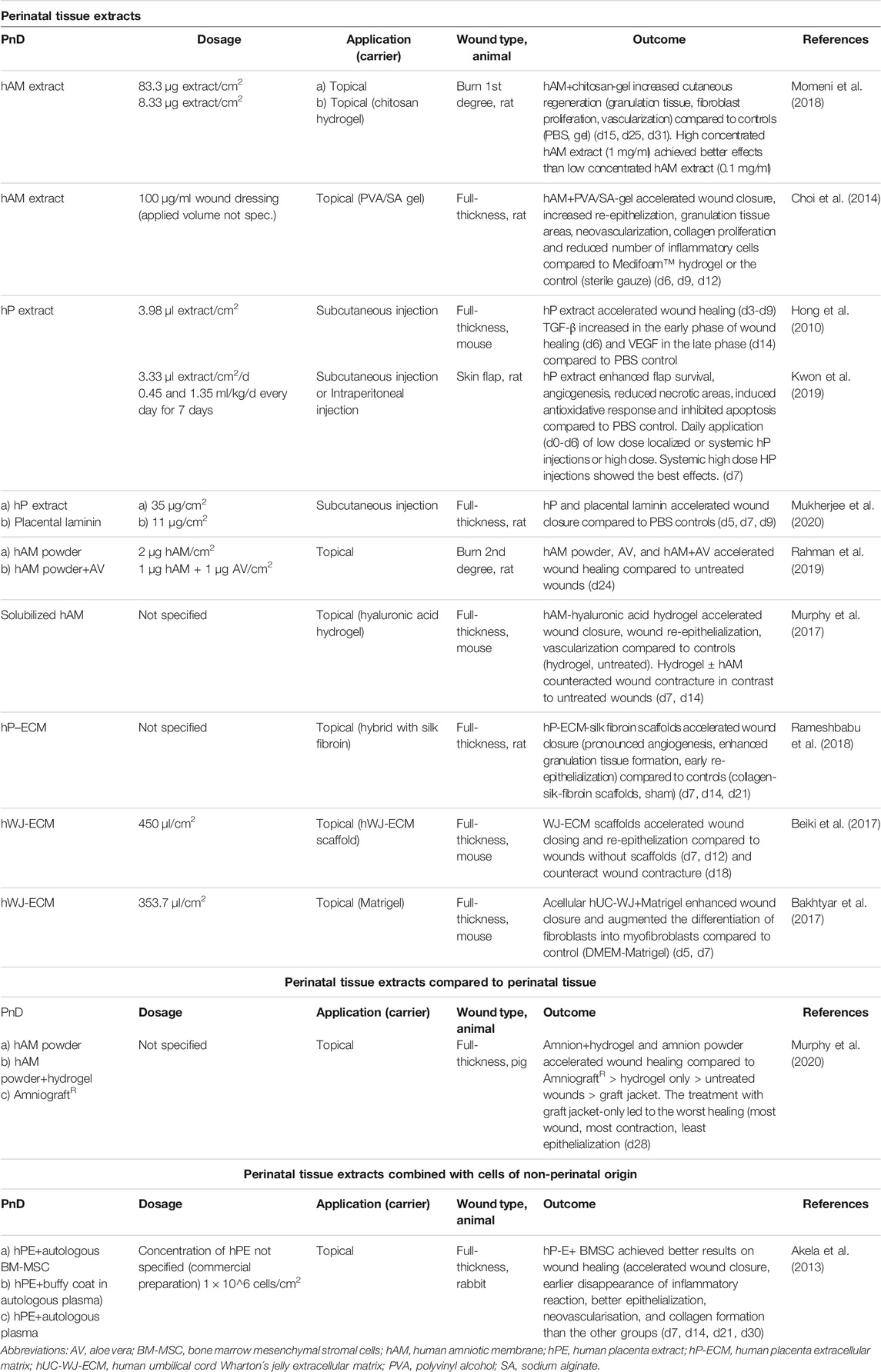
TABLE 5. Application of perinatal tissue extract alone or compared to/combined with with perinatal tissue or cells of non-perinatal origin in in vivo animal models of skin wound healing. Time points indicated in the “Dosage” and “Outcome” column mean days (d) or weeks (w) of/after treatment.
To standardize the different dosages of PnD used in the reviewed studies, the doses are presented as the number, weight (μg), or volume (μl) of the specific PnD per wound area (cm2), when the PnD were administered locally (topically, intradermally/subcutaneously), or per body weight (kg) of an animal, when PnD were applied systemically (intravenously or intraperitoneally). To determine the dose of locally administered PnD per cm2, we divided the quantity of applied PnD by the wound area. In most of the studies, both the wound area and the exact quantity of PnD were indicated.
Perinatal Cells
Perinatal cells were the most commonly used PnD type in preclinical studies of cutaneous wound healing (49.5%) (Table 1). Mostly, hUC-MSC were used (60.4%), followed by hAMSC (12.5%) and MSC isolated from the whole placenta (hPMSC, 8.3%). Cells were applied as suspensions injected intradermally/subcutaneously or intravenously, topically by cell spraying, or seeded on electrospun or biological scaffolds, including the amniotic membrane. Cell therapy implemented by administering cells to the bloodstream may lead to the accumulation of injected cells in other organs such as the lungs (Ankrum and Karp, 2010).
Perinatal cells were applied in doses ranging from 200 cells per cm2 up to 8 million cells per cm2 (Figure 2A). The most frequently applied dose was around 2 million cells per cm2 (mode = 1.99 × 106/cm2, median = 1 × 106/cm2). Regarding mode of application, lower doses of cells were used for topical application (median = 0.6 × 106/cm2) than for subcutaneous (median = 1.78 × 106/cm2) and intradermal (median = 3.54 × 106/cm2) applications (Figure 2B). Mostly used dose for full-thickness wound model was 1.99 × 106/cm2 (median = 1.88 × 106/cm2), while for the other wound models the doses were usually less than 1 × 106/cm2 (median = 0.12 × 106/cm2 for burn wounds, 0.11 × 106/cm2 for radiation wounds, 0.22 × 106/cm2 for skin flaps, and 0.1 × 106/cm2 for non-healing skin lesions). The median cell doses for mice were higher (1.99 × 106/cm2) than those for the rats (0.16 × 106/cm2). The systemic doses were 25 × 106/kg and 29 × 106/kg when applied intravenously, and 45 × 106/kg, when applied intraperitoneally (Liu et al., 2014; Abd-Allah et al., 2015; Liu et al., 2016; Shi et al., 2020). Moreover, few studies compared the effect of applying different cell concentrations. Namely, in a full-thickness skin wound model on rats, increasing the number of hAMSC injected into the wound bed from 2 × 103/cm2 to 2 × 105 cells/cm2, enhanced the wound healing results (Gao et al., 2020). On the other hand, in a full-thickness burn wound model on pigs, low doses, ranging from 200 to 40,000 cells per cm2, topically applied in combination with a collagen-based scaffold, demonstrated superior wound healing of full-thickness burn wounds, compared to higher doses (2 × 105/cm2 to 2 × 106/cm2) and controls (Eylert et al., 2021). In a study that compared systemic (intraperitoneal) and local (intradermal) application, the intraperitoneal injections of hPMSC showed better results than the local one, in terms of histological scores, expression of the healing promoting factors, as well as the engraftment into the wounded skin (Abd-Allah et al., 2015).
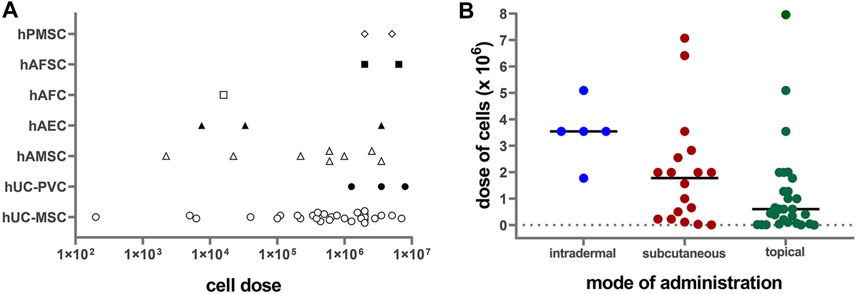
FIGURE 2. (A) Correlation of applied PnD cell type versus cell number/cm2 on a logarithmic scale (Log10). hUC-MSC, human umbilical cord mesenchymal stromal cells; hAMSC, human amniotic membrane mesenchymal stromal cells; hPMSC, human placenta mesenchymal stromal cells; hAFC, human amniotic fluid cells; hAFSC, human amniotic fluid cells; hUC-PVC, human umbilical cord perivascular cells; hAEC, human amniotic membrane epithelial cells. (B) Correlation of cell doses with the mode of administration. Lines represent median values.
Perinatal Cell-Derived Conditioned Medium (CM)
Of the studies included in this review, 12.9% used perinatal cell-derived CM (Table 2). The CM derived from hUC-MSC was the most commonly used CM (58.3%). The CM is a medium collected from cell cultures and contains various cell products released by cells. These products may be proteins, non-coding RNAs, growth factors, antioxidants, proteasomes, and extracellular vesicles, which vary depending on the cell type and cell culture conditions (Maguire, 2013). The advantage of using CM collected from MSC to study regeneration lies in the ease of medium availability, storing, freezing, drying, and transporting, as well as in its lack of immunogenicity (Gunawardena et al., 2019).
CM was usually collected after 24, 48, and 72 h of cell cultivation. It was mainly applied intradermally/subcutaneously or topically and in combination with hydrogels or as a cell spray. Doses of the CM used ranged from 7 to 354 μl/cm2. No studies examined the effects of different CM dosages on the efficacy of wound healing.
Several wound healing studies have investigated the combined treatment of cell-derived CM and corresponding cells, or have compared the efficacy of cell-derived CM versus cells alone. While both CM and its corresponding perinatal cells, alone or in combination, showed an increased rate of re-epithelization and accelerated wound closure compared to control, no significant difference in efficacy between the CM and the cells has been demonstrated (Payne et al., 2010; Shohara et al., 2012; Shrestha et al., 2013; Aguilera et al., 2014; Raj et al., 2019; Zhang et al., 2020). Only rare studies used multiple doses of CM (Payne et al., 2010; Sun et al., 2019). It was observed that frequent application of CM achieved better results on wound healing than 2-fold application (Payne et al., 2010).
Perinatal Cell-Derived Small Extracellular Vesicles (sEV)
The least used PnD type (9.6%) were perinatal cell-derived EV (Table 3). The cell secretome generally contains a mixture of different EV subtypes, including exosomes, apoptotic bodies and microparticles (Gangoda et al., 2015). The exosomes are the most characterized and the smallest EV. They are of endosomal origin with the size of 30–150 nm in diameter and carry many different components, such as lipids, proteins, mRNAs, non-coding RNAs, and even DNA derived from cells (reviewed in Rashed et al. (2017)). It was first thought that exosomes only play a role in removing unnecessary molecules from cells, but nowadays their involvement in a plethora of different cell responses is documented (Keller et al., 2006). According to their shape, size, and marker expression (HSP70, CD9, CD63, CD81), the EV described in the processed literature were characterized as exosomes. However, the International Society for Extracellular Vesicles in their position statement from 2018 (van Deun et al., 2017; Théry et al., 2018) urge authors to use an operational term for EV subtypes unless they can unequivocally prove their endosomal origin (by live imaging techniques, for example). Therefore, according to these recommendations, in this review we will refer to these EV as small EV, or sEV. The sEV derived from perinatal cells are a relatively new PnD type used for skin wound healing since they first appeared in publications only a few years ago. All included studies used sEV derived from MSC, most commonly from hUC-MSC (77.8%).
The sEV were applied mostly by subcutaneous injection in animals with burn or full-thickness wounds. The doses of sEV were predominantly expressed as μg/cm2, except for one study where the authors used the number of particles as a measure for the sEV quantity (Bian et al., 2020). The doses were comparatively uniform throughout the analyzed studies, mainly using 100 to 156 μg/cm2 (Zhang et al., 2015a; Zhang et al., 2015b; Zhang et al., 2016; Yang et al., 2019; Yang et al., 2020; Zhao et al., 2020) and 318 μg/cm2 in one study (Liu et al., 2021). There were no studies that compared different dosages of sEV.
Perinatal cell-derived sEV showed similar (Zhang et al., 2015a) or even better (Zhao et al., 2020) beneficial effects on wound healing compared to perinatal cells. Gao et al. (2020) observed that sEV overexpressing micro RNA (miR)-135a significantly accelerated fibroblast cell migration by downregulating LATS2 levels to promote wound healing in rats making it the first evidence of positive miRNA effect on this process. This was an interesting aspect, because so far, different miRNAs (including miR-135a) were mainly shown to impair wound healing (Bibby et al., 2021).
Perinatal Tissues
Perinatal tissues (of which hAM predominate with 85.7%) were applied in 15.1% of the included preclinical studies (Table 4). The hAM serves as a potential wound dressing due to its high biocompatibility, antimicrobial and anti-scarring properties. Its anti-inflammatory properties have been attributed to the decrease of pro-inflammatory cytokine expression (transforming growth factor ß and interleukin 10). Furthermore, it produces B defensins, elastase inhibitors, elastin, and lactoferrin with antimicrobial effects, making it highly attractive in healing leg ulcers (ElHeneidy et al., 2016). Decellularized or dehydrated perinatal tissues were used as grafts or as support for perinatal cells or cells of another origin and were applied topically. In cases where the entire full-thickness or burn wound area was covered with the hAM (Kim et al., 2009; Samadikuchaksaraei et al., 2016; Motamed et al., 2017; Song et al., 2017; Farzamfar et al., 2018; Gholipourmalekabadi et al., 2018; Kakabadze et al., 2019; Arasteh et al., 2020; Nasiry et al., 2020), the wound areas are given instead of dosages (Table 4). Also, in two studies where a proprietary human amnion/chorion graft (EpiFix®) was implanted subcutaneously, calculating the dosages was inapplicable (Koob et al., 2013; Koob et al., 2014).
It was shown that combinations of cells and decellularized hAM achieved better results on wound healing than either cells or hAM alone (Kim et al., 2009; Sabapathy et al., 2014; Samadikuchaksaraei et al., 2016; Motamed et al., 2017; Farzamfar et al., 2018; Gholipourmalekabadi et al., 2018; Kakabadze et al., 2019; Hashemi et al., 2020).
Perinatal Tissue Extracts
Of the included studies, 12.9% used perinatal tissue extracts (Table 5). The most frequently used tissue extract was hAM extract (41.7%). Tissue extracts are usually obtained by tissue lysis and centrifugation, hence they do not contain cells, but are rich in an array of proteins, minerals, amino acids, and steroid hormones (Datta and Bhattacharyy, 2012). Tissue extracts possess anti-inflammatory, antioxidant, and cytoprotective properties and stimulate proliferation and reparative processes similar to their tissues of origin (reviewed in Pogozhykh et al. (2018)).
Perinatal tissue extracts (some of which were prepared as a powder) were applied topically or by subcutaneous injection, mostly on full-thickness skin wounds, and once on either a burn wound or a skin flap model, respectively. The amount of the extracts used was usually expressed in μg of lyophilized powder or, in some cases, in μl of proprietary human placental extracts. The doses ranged from 1 μg to 83.3 μg/cm2. In two studies that compared the application of lower and higher doses of perinatal tissue extracts, higher doses performed better in terms of wound healing rate, reduced inflammation (Momeni et al., 2018), and skin flap survival (Kwon et al., 2019). Acellular umbilical cord-derived Wharton’s jelly extract (hWJ-ECM) was applied with Matrigel or in the form of a spongy scaffold in doses of 353.7 and 450 μl/cm2, respectively, providing enhanced wound closure and re-epithelization (Bakhtyar et al., 2017; Beiki et al., 2017). In one study, hybrid extracellular matrix sponges containing human placenta-derived extracellular matrix were applied within the wound area, but no volume or other quantitative value of the applied matrix was specified (Rameshbabu et al., 2018).
It was shown that amnion-derived hydrogel and amnion powder achieved better results on wound healing than AmnioGraft® (Murphy et al., 2020). The combination of the human placental extract with autologous bone marrow MSC achieved better results on wound healing than placental extract applied without cells (Akela et al., 2013).
In vitro Characterization and Functional Testing of PnD Before Their Application in Preclinical Studies
The evaluation of the in vitro characterization (Figure 3A) displayed the sEV as the most in vitro characterized PnD, as they were properly characterized in all included studies. The methods used for the characterization were: transmission electron microscopy to observe their morphology (100%), Western blot to determine the expression of exosome markers (89%), and nanoparticle tracking analysis to measure the size of sEV (89%). The reputable characterization of sEV is most probably due to the exact guidelines for isolation and characterization (van Deun et al., 2017; Théry et al., 2018). Encouraging the scientific community to properly perform exosome characterization has proven to be a good practice that provides transparency to the studies, and should also be considered in other fields. Cells were also fairly well characterized in vitro (84.8%). The predominant methods were: flow cytometry to identify the presence of the cell surface markers (87.2%), assessment of the differentiation potential into multiple lineages to confirm their stemness (50%), and bright field microscopy to verify morphological characteristics (35.9%). Of perinatal cell-derived CM, 83.3% were characterized in vitro. CM was biochemically analyzed in just one of the studies (Sabzevari et al., 2020), however, we further defined CM as “in vitro characterized” also when the corresponding cells were identified and characterized at the molecular and morphological levels. Perinatal tissues were in vitro characterized with a proportion of 50%; this group also includes commercially available perinatal tissues (71.4%).
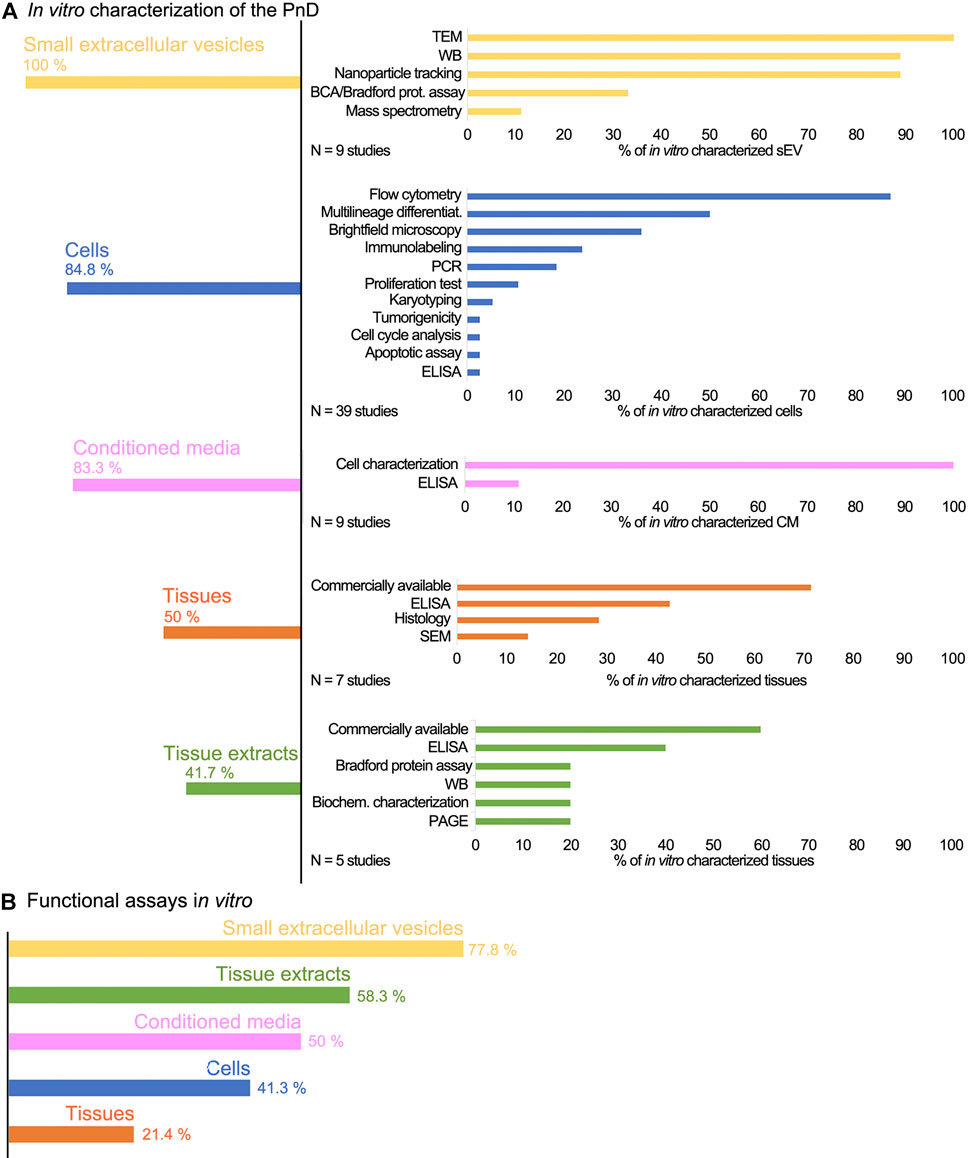
FIGURE 3. (A) The schematic presentation of the PnD characterization and (B) verification in vitro. The sEV derived from perinatal MSC are overall the most characterized and in vitro tested PnD used in skin wound healing in preclinical studies. Conversely, the least characterized and in vitro tested PnD are the perinatal tissues. The commercially available PnD or the PnD that have been described in the previous studies by the same authors, were defined as characterized in vitro, although the exact data were not provided in the actual study.
We considered commercially obtained perinatal tissues as “in vitro characterized,” since the vendors clearly describe them. As the most commonly used perinatal tissue is the hAM, we assume that the poor percentage of the in vitro characterization is due to the fact that the hAM has been characterized and tested many times before. Further, identification and preparation of the hAM are unambiguous and straightforward (Jerman et al., 2014; Poženel et al., 2019; Jerman et al., 2020; Ramuta et al., 2020; Weidinger et al., 2020), whereas cells and cell-derived secretomes or sEV require precise characterization. Since the vast majority of the researchers isolated cells from the perinatal tissues by themselves, adequate characterization is mandatory to assure that a pure cell population has been isolated. The least in vitro characterized PnD were perinatal tissue extracts (41.7%).
In addition to in vitro characterization, in vitro functional tests were performed (Figure 3B). The differentiation and proliferation assays, scratch wound assay, and cytotoxicity assay were the most prevalently used to evaluate the effect of different PnD on cell cultures in vitro. The in vitro functional tests are usually quick and performed under controlled conditions. Compared to the animal models, they certainly lack the complexity of the native tissue. Nevertheless, monitoring the specific mechanisms in vitro is a good indicator of how the tested materials affect the biological processes in vivo. Among the PnD used in the preclinical studies of skin wound healing, 77.8% of the perinatal cell-derived sEV were functionally tested in vitro. Moreover, 58.3% of included studies performed functional tests in vitro on perinatal tissue extracts, and half of the studies functionally tested the perinatal cell-derived CM. On the other hand, despite being the most frequently used PnD in skin wound healing, only 41.3% of the perinatal cells were subjected to functional in vitro assays before their application in preclinical studies. The least in vitro tested PnD were perinatal tissues (21.3%).
Animal Models of Cutaneous Wound Healing
Animal models span various wound types, multiple animal species, and strategic scientific approaches for wound treatment. According to most common problems in wound healing in humans, such as full-thickness wounds, chronic ulcers caused by diabetes, poorly healing wounds after radiation, or severe burn injuries, the animal models are adapted to these conditions as well as possible.
The herein described animal models dealt with full-thickness wounds (53 studies), burn wounds (18 studies), radiation wounds (three studies), skin flaps (two studies), and subcutaneous pockets (two studies). One case report described the application of PnD on non-healing skin lesions (Figure 4; Tables 1–5).
Wound Models
Full-Thickness Wound Models
In full-thickness wounds, all skin layers including the subcutaneous tissue, are removed. Full-thickness wounds were inflicted mainly by biopsy punches or by scalpels and surgical scissors. In addition to healthy animals, this wound type has also been applied to diabetic animals to mimic impaired healing conditions and chronic wounds.
Diabetic Wound Models
Diabetes is a major health care problem. A diabetes-related foot ulcer is one of the most challenging complications in the treatment of diabetic patients. More than half of diabetic ulcers become infected and lead to amputation in 20% of cases. (Armstrong et al., 2017). Thus, several studies use diabetic mouse and rat models to develop new strategies for treatment.
Experimental type 1 diabetes can be studied on a genetic non-obese diabetic mouse line or on mice/rats treated with streptozotocin, which destroys pancreatic beta cells. The advantage of diabetes induced by streptozotocin injection is that there is no limitation to a particular mouse or rat strain. Two studies used the non-obese diabetic/severe combined immunodeficiency (NOD/SCID) mice, which have a metabolic state similar to type 1 diabetes ((Jin et al., 2016) and (Kim et al., 2012)). One study used a streptozotocin-induced C57BL/6J mouse (Zhang et al., 2020). Four studies used diabetic rat models on streptozotocin-induced SD rats (Han et al., 2019; Shi et al., 2020; Yang et al., 2020; Yue et al., 2020). One further study used streptozotocin-induced Wistar rats (Milan et al., 2016).
Type 2 diabetes can be induced by a high-fat diet or genetically in the leptin-deficient mouse line (Boyko et al., 2017). Four studies (Shrestha et al., 2013; Peña-Villalobos et al., 2018; Raj et al., 2019; Bian et al., 2020) used leptin-deficient db/db mice as a diabetes type 2 model. Affected mice show morbid obesity, chronic hyperglycemia, pancreatic beta-cell atrophy, and hypoinsulinemia. They are polyphagic, polydipsic, and polyuric, similar to diabetic type 2 patients. This mouse line is recommended for wound healing models2.
Wang et al. (2016) used the Goto-Kakizaki rats as a non-obese model of type 2 diabetes with metabolic, hormonal, and vascular disorders similar to human diabetes.
Burn Injury Wound Models
Burn wounds can be created by scald, contact, chemical or electrical combustion. The severity of the burn wound (Supplementary Table S1) depends on the temperature and exposure time of the heat source to the skin, as well as on the skin thickness.
Except for the study on pigs (Eylert et al., 2021), all burn injury wound models of the reviewed studies were performed on rodents (primarily mice and rats, with one study was performed on guinea pigs). Burn wounds were created by scalding with hot water (70°C–94°C) for 6–100 s (Liu et al., 2014; Zhang et al., 2015a; Zhang et al., 2015b; Du et al., 2016; Liu et al., 2016; Zhang et al., 2016; Momeni et al., 2018; Li et al., 2019; Yang et al., 2019; Liu et al., 2021) or by a heated brass rod or aluminum devices (100–105°C, for 5–30 s), where wound size and shape depend on the size and shape of the instrument used (Motamed et al., 2017; Gholipourmalekabadi et al., 2018; Pourfath et al., 2018; Mahmood et al., 2019; Rahman et al., 2019; Hashemi et al., 2020). As wounding by the brass bar technique leads to scab formation, the developed necrosis was usually excised 12–48 h before application of PnD.
In most studies, severe burn injuries of 2nd or 3rd degree were performed. Some authors described the injury as “full-thickness burn injuries” which would correspond to at least category IIa according to the severity score. In cases with extensive wounds, an injection of balanced salt solution was given to prevent shock.
Uniform partial-thickness burns cannot be reproducibly created in mice and rats due to their estrous hair cycle (Robson et al., 1980), and superficial burns in pigs heal too quickly, because the porcine epidermis is 2-fold thicker than the human equivalent (Hammond, 2000). Thus, a higher temperature is needed to create severe burn wounds in pigs. (Eylert et al., 2021) used a heated aluminum device (200°C) for 20s and digital force gauge and histologically confirmed full-thickness burn wounds 48 h post-burn via punch-biopsy. Payne et al. (2010) studied partial-thickness burn wounds on guinea pigs, which do not have hair cycles and are therefore the more satisfactory model to evaluate this wound type. Only one study investigated superficial first-degree burn wounds on rats (Momeni et al., 2018).
Some authors described the percentage of the total body surface area (%TBSA) affected by a burn. 10% TBSA wounds were performed on guinea pigs (Payne et al., 2010), 30% TBSA and 50% TBSA on rats (Liu et al., 2014; Liu et al., 2016).
Due to a large number of different parameters such as wound size, heat exposure time, application of brass bar or water, inconsistent use of the severity score, and different treatments and application modes with PnD, a comparison of investigated experiments is not possible.
Radiation-Induced Wound Models
Radiation dermatitis is a common side effect of radiotherapy. Many of the radiation-induced skin changes are minor and reversible. Nevertheless, when acute changes do not resolve, skin ulcers, fibrosis, or necrosis of underlying structures may occur. Disruption of the epithelial basement membrane and breakdown of the barrier function substantially increase the risk for these injuries (Hymes et al., 2006). The severity grade is shown in Supplementary Table S2 according to the Classification of radiation dermatitis from the National Cancer Institute (Hymes et al., 2006).
In the studies reviewed, 3rd or 4th-grade radiation wounds were created in rats (Liu et al., 2014; Kakabadze et al., 2019; Sun et al., 2019), respectively. Radiation dose and exposure time varied between the different studies. Liu et al. (2014) administered a dose of 45 Gy for 7.5 min per animal, Sun et al. (2019) administered 40 Gy for 25 min and Kakabadze et al. (2019) used a dose of 60 Gy without specifying of the radiation duration.
Sun et al. (2019) topically applied Hydrogel containing hUC-MSC-CM every second day after radiation for 8 weeks. PnD treatment accelerated wound closure, sebaceous gland regeneration, and angiogenesis compared to EGF application and negative control (weeks 2, 4, 6, 8).
Liu et al. (2014) created ulcers and Kakabadze et al. (2019) excised the radiation areas 3 weeks post-radiation to simulate a chronic radiation injury before starting the treatment with PnD. Subcutaneous injection of hUC-MSC increased neovascularization and re-epithelization, days 14, 21, 28 after treatment (Liu et al., 2018). Topical application of decellularized hAM seeded with BM-MSC (Kakabadze et al., 2019) accelerated wound closure compared to decellularized hAM. Inflammation and exudations were absent when the decellularized hAM was used (observation period 90 days).
Due to different intervals of evaluation, only days 7 and 14 could be used for a comparison, whereby the comparison is limited by different doses of radiation, different surgical interventions post-radiation, and different time points of application of the various PnD. In all investigated works, wounds treated with PnD showed a significantly accelerated wound healing on day 14 compared to control groups.
Due to the limited data and varying methods, a clear recommendation for the optimal treatment method concerning the application of PnD for the healing of radiation-induced wounds is not possible.
Skin Flap Models
A skin flap is a full-thickness mass of skin containing superficial fascia, transplanted from a donor site to a recipient site with an intact blood supply. Skin flap surgery is a common procedure in reconstructive surgery. In this field, surgeons often struggle with ischemia-associated complications such as tissue necrosis or wound breakdown (Schmauss et al., 2018). Flap models in rodents are versatile and have a long tradition in experimental surgery. In studies included in this review, two types of skin flaps were performed: the epigastric ischemic skin flap (EIF) and the McFarlane flap.
The EIF is an axial skin flap, designed at the pedicled superficial inferior epigastric vessels. The skin flap is lifted from its remote end, and the vascular pedicle gets clamped for a specific time to induce flap ischemia. Leng et al. (Leng et al., 2012) performed the EIF on mice at a size of 3 × 6 cm and clamped the respective blood vessels for 6 h. Then, the flap was opened to remove the clamps and to enable flap reperfusion. The flap was sutured in situ and hUC-MSC were subcutaneously injected into the flap at 10 distributed points. After 7 days, the survival area of the flap was evaluated concerning the gross appearance, necrosis, and vascularization. hUC-MSC were detectable in the flap tissues and increased the survival of the flap, neovascularization, and expression of bFGF and VEGF compared to controls without cell treatment.
The McFarlane flap model is characterized by a cranially based and randomly perfused dorsal skin flap, which is elevated beneath the musculus panniculus carnosus. It has a defined width-to-length ratio to ensure a predictable rate of necrosis. Perforating blood vessels were electrically cauterized to ensure a completely random vascular pattern. Kwon et al. (2019) performed this model on rats with a flap size of 3 × 10 cm to investigate therapeutical effects of human placenta extract (hP-E) applied by subcutaneous or intraperitoneal injections. hP-E enhanced flap survival, angiogenesis, reduced necrotic areas, induced antioxidative response, and inhibited apoptosis compared to phosphate-buffered solution (PBS) control. Daily application (d0-d6) of low dose (10 mg/kg/d) localized or systemic hP-E injections or high dose (40 mg/kg/d) systemic hP-E injections showed the best effects of high dose administration (d7).
Although data on using PnD in skin flap models are rare, both approaches showed promising results.
Subcutaneous Pocket Model
Koob et al. (2013), Koob et al. (2014) created subcutaneous pockets in mice and implanted commercially available dehydrated human amnion/chorion-tissue allografts (PurionR) at a size of 5 × 5 mm to investigate their biological and angiogenic properties. hAM/chorion implants recruited more mesenchymal progenitor cells to the site of implantation compared to uninjured skin and the sham implant site (day 7). In addition, implants displayed a steady increase in microvessels approaching that of healthy and healing skin after 28 days.
Animal Species Used for Cutaneous Wound Healing Models
Rodents
The vast majority of animal wound models (92%) were performed on rodents, with 40 and 32 studies performed on mice and rats, respectively. Only one study was performed on guinea pigs. Rodents are easy to handle and inexpensive with respect to costs for food, medication, and wound dressings. They are simple to house and have a short life cycle. Generally, smaller wounds were created on mice and rats than on larger animals, which helps to reduce the quantity of applied PnD material. However, rodents’ wounds close due to the contraction of the musculus panniculus carnosus, a thin layer of skeletal muscle located in subcutaneous tissue, which is virtually non-existent in most regions of the human body (Zomer and Trentin, 2018). This physiological difference creates difficulties in replicating the wound closure processes of human skin, which should be considered. For long-time experiments on full-thickness wounds, anti-contractive tools should be chosen (described in the chapter Wound Healing in Rodents).
Mouse Strains Used in Wound Healing Studies
The C57BL/6 mouse was the most commonly used strain in the analyzed studies (Figure 5A). It is a general-purpose strain. Some of its characteristics are a high susceptibility to diet-induced obesity, diabetes II, and atherosclerosis. It has a nearly black coat, is easy to breed, and is robust. However, it tends to bite, and male mice remove hair from their cage mates (Sarna, 2000). Its barbering behavior may be counterproductive for an undisturbed wound healing process, as these animals might nibble on wound dressings. Most importantly, these animals are unusually sensitive to pain and cold, and analgesic medications are less effective (Mogil et al., 1999). Thus, the selection of C57BL/6 mice for wound healing experiments needs to be carefully evaluated.
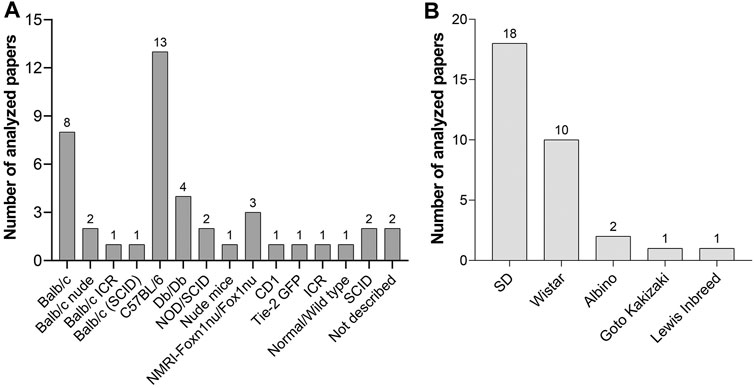
FIGURE 5. (A) Mouse strains and (B) rat strains used for wound healing experiments with PnD treatment in scientific papers published between 2004 and 2020.
Another commonly used mouse type was the albino Balb/c strain with its different inbreeds (Balb/c ICR, Balb/c (SCID), and Balb/c nude). BALB/c mice are more docile than C57BL/6 mice and are particularly well known for the production of monoclonal antibodies. BALB/c mice are used in many research fields (cardiovascular research, cancer, infectious diseases, neurobiology immunology, inflammation, autoimmunity). As most substrains display high levels of anxiety, (Griebel et al., 1993; Belzung and Berton, 1997) a familiar, calm environment is recommended.
The ICR inbred strain serves as a general-purpose strain and does not develop insulitis or diabetes. These mice were recommended amongst others for drug testing and as a control for non-obese diabetic mice3.
CD1 are albino mice and are suggested as a general multipurpose model, for safety and efficacy testing, as an aging, pseudopregnancy, and surgical model4.
SCID Mice are homozygous for the severe combined immune deficiency spontaneous mutation (Prkdcscid). They are characterized by an absence of functional T cells and B cells, and a normal hematopoietic microenvironment. As SCID mice accept allogeneic and xenogeneic grafts, they are an ideal model for cell transfer experiments. Immunodeficient mice should be housed in a specific-pathogen-free environment to avoid infections5.
Nude mice such as the Balb/c nude or the NMRI-Foxn1nu/Foxn1nu inbred are characterized by thymic aplasia, which results in immunodeficiency due to the lack of T cells. They have no rejection responses. The mutation leads to a keratinization defect of the hair follicles and the epidermis6. The mostly hairless phenotype helps to avoid a shaving procedure before the wound healing experiments. They are sensitive to cold and need to be kept at warm temperature conditions.
TIE-2 GFP (B6.Cg-Tg287Sato/1) strain expresses Green Fluorescent Protein under the direction of the endothelial-specific receptor tyrosine kinase (Tek, formerly, Tie2) promoter. This mouse type is especially appropriate to study neovascularisation during the wound healing process as GFP-expressing endothelial cells can be visualized via fluorescent microscopy (Wang et al., 2018).
Rat Strains Used in Wound Healing Studies
Compared to mouse strains, fewer rat strains were used in the investigated wound healing experiments (Figure 5B). Sprague Dawley (SD) and Wistar rats are the most popular albino rats used for laboratory research. SD rats are calm and less active than Wistar rats and are therefore easy to handle. Lewis rats are highly sensitive to the induction of autoimmune diseases as well as to diet-induced obesity and diabetes and streptozotocin-induced diabetes7. The Goto-Kakizaki inbred line was used as a non-obese model of type 2 diabetes.
Wound Healing in Rodents
In general, larger wounds were created on rats than on mice (one to two full-thickness wounds/animal with an average size of 1.52 vs. 2.11 cm2 and one to two burn wounds/animal with an average size of 1.37 vs. 3.8 cm2 in mice and rats, respectively. Larger wounds were radiation wounds created on rats (8 cm2) and skin flaps (18 cm2 on mice, 30 cm2 on rats). Several approaches were developed to get similar conditions to human wound healing through the generation of granulation tissue and re-epithelialization rather than contraction of the musculus panniculus carnosus. One possibility is to investigate early stages of wound healing in short-term studies lasting up to 8 days post wounding, where no apparent wound contraction occurs (as performed by Tuca et al. (2016), Ertl et al. (2018)).
For longer-term experiments on full-thickness wounds, anti-contractive tools should be chosen: Splinting wound models use silicone or rigid plastic rings strapped around the wound area to the underlying muscles to prevent wound contraction. In the reviewed papers, the silicone rings were mostly sutured or fixed with glue (Kim et al., 2012; Shohara et al., 2012; Aguilera et al., 2014; Jin et al., 2016; Wang et al., 2016; Peña-Villalobos et al., 2018; Wang et al., 2018; Kaushik and Das, 2019; Arasteh et al., 2020; Nasiry et al., 2020; Sabzevari et al., 2020). Others directly sutured scaffolds loaded with PnD to the wound site (Yang et al., 2013; Edwards et al., 2014; Milan et al., 2016; Montanucci et al., 2017). Edwards et al. (2014) additionally placed a titanized mesh between the wound bed and a collagen-based scaffold (loaded with hUC-MSC) to avoid tissue contraction. However, these methods carry risks of inflammation and surgical site infection (Mulholland, 2020). Some studies did not use suturing of the scaffolds (or at least did not mention suturing) (Nan et al., 2015; Du et al., 2016; Han et al., 2019; Raj et al., 2019).
Beiki et al. (2017) observed that scaffolds produced from liquid WJ-ECM accelerated wound closing and counteract wound contracture during the observation period of 18 days. Also, a hyaluronic acid hydrogel with or without solubilized hAM counteracted wound contracture (Murphy et al., 2017). We could see from Vonbrunn et al. (2020) that the application of stiff carrier materials for PnD such as electrospun Poly(ε-caprolactone)/poly(l-lactide) (PCL/PLA) could enable uncomplicated experiments on rodents without suturing and could open new therapeutic approaches due to the anti-contractive properties of the material. Still, numerous long term-studies were performed on rodents, where no anti-contractive strategies were applied. This hinders the reproducibility of data, and the studies’ outcomes seem questionable. Anti-contractive properties are strongly needed, especially if PnD were just subcutaneously injected around the wound area or are topically applied in fluid or gel-like solutions or as soft membranes.
Another problem for the reproducibility of data is that there is significant variability in the application of wound dressings. Wound dressings are used to keep the wound area free of contamination. In the majority of the analyzed studies on rodents, it is not described whether a wound dressing was applied or not (Figure 6). Thus, it is difficult to evaluate the wound healing progress under unspecified experimental settings. In two studies, wounds were left open (Liu et al., 2014; Liu et al., 2016).
A popular wound dressing material for rodents is a dressing with Tegaderm™ plaster. It was applied without suturing (Zebardast et al., 2010; Tuca et al., 2016; Ertl et al., 2018; Bian et al., 2020; Vonbrunn et al., 2020), sutured onto the skin (Eylert et al., 2021), or was combined with a cohesive bandage (Arasteh et al., 2020). Other possibilities for wound dressings are fat gauze or oil gauze – with and without suturing to the skin (Montanucci et al., 2017; Motamed et al., 2017; Song et al., 2017; Gao et al., 2020; Hashemi et al., 2020; Zhao et al., 2020).
As unsutured plasters were partly detached from the wounds after a few days, we recommend removing the plasters 3 days post wounding, and thereafter leaving the wounds open to get standardized conditions for the wound healing process.
An accurate description of the advantages or disadvantages of used wound dressings in future publications would be helpful to standardize experiments and to enable comparability of the experiments concerning the healing outcome.
Wound Healing in Large Animals
On large animals, experiments were mainly performed on pigs. Pigs have the advantage that the porcine skin more closely correlates to human skin in thickness and structure than rodent skin. Wound closure in pigs is similar to wound healing in humans because the musculus panniculus carnosus is vestigial or absent in most body regions. Therefore, wounds heal by re-epithelialization rather than by contraction, and no anti-contractive strategies or devices are needed. However, experiments on pigs are cost-intensive and far more complex with respect to medication, anesthesia, and wound dressing, making them mainly useful for preclinical trials of therapies (Boyko et al., 2017).
As the number of animals in experiments is limited due to costs and husbandry, multiple wounds were performed on each subject.
Three studies included in the review utilized pigs as experimental animals (Han et al., 2014; Murphy et al., 2020; Eylert et al., 2021). Multiple wounds were created on the back of the animals (minimum 4, maximum not specified) and topically treated with PnD for 3–4 weeks. (Eylert et al., 2021) performed multiple full-thickness burn wounds with a size of 5 × 5 cm in diameter. It was shown that wounds treated with Integra® seeded with hUC-MSC at a low dose (40,000 cells/cm2) regenerated wounds most efficaciously. Wounds were dressed with a layer of topical antibiotics, fat gauze, multiple layers of gauze, Tegaderm®, and a compression jacket. The initial wound margins were marked by a skin stapler. Wound dressing changes were performed two to three times per week.
Murphy et al. (2020) created eight 4 × 4 cm square-shaped full-thickness wounds on the central back and marked initial wound margins by tattooing before excision. Amnion-hydrogel and amnion powder treatments achieved the most rapid wound healing compared to commercially available Amniograft®, followed by hydrogel only, untreated wounds, or graft jacket, respectively. The treatment with the graft jacket alone led to the worst healing outcome (largest wound area, most contraction, least epithelialization). The wound dressing consisted of a topical antibiotic cream, Tegaderm® and a cast padding, cohesive bandaging, a protective saddle, and a jacket.
Han et al., 2014 (Han et al., 2014) created circular full-thickness wounds (diameter 3 cm). Collagen-chitosan-based scaffolds loaded with microencapsulated VEGF gene-modified hUC-MSC improved the vascularization of the tissue-engineered dermis and induced a better wound healing than controls (microencapsulated hUC-MSC, empty capsule, and PBS-treated group). The wound dressing was not described.
One case report described the wound treatment on dogs (Ribeiro et al., 2014). Two dogs suffering from non-healing skin lesions were treated with poly(vinyl alcohol) hydrogel (PVA) membrane supplied with low-dose UC-MSC 0.1 × 10^6 cells/cm2. hUC-MSC induced significant progress in skin regeneration with the decreased extent of ulcerated areas.
Two studies were performed on rabbits, where full-thickness wounds were treated with rabbit BM-MSC either in combination with hAM (Kim et al., 2009) or with human placenta extract (Akela et al., 2013).
Summary and Conclusion
The pre-clinical evidence of our review indicates that PnD-based therapy is in general effective to promote cutaneous wound healing. The significant amount of variability between types, dosage, and application mode of PnD, as well as between the animal models, hinders the comparability of data. Perinatal cells were the most commonly used PnD type, but only a tiny minority of studies compared the effect of applying different cell concentrations or modes of administration. Perinatal cell treatment worked at both low and high dosing, and further studies are required to evaluate the most optimal cell therapy. Treatment with perinatal cell-derived CM achieved similar beneficial effects as with cells, and frequent multiple applications of CM showed better outcomes than single- or two-fold treatments. Perinatal cell-derived sEV showed similar or even better beneficial effects on wound healing than perinatal cells. No studies compared different dosages of perinatal cell-sEV. The combination of cells and perinatal tissue membranes achieved better results on wound healing than either cells or tissue alone, and the combination of perinatal tissue extracts with cells achieved better results than tissue extracts without cells.
As an inadequate characterization of PnD may lead to a false identification of the PnD that hinders reproducibility and comparability of various studies, we propose the following guidelines for the PnD characterization before their application in pre-clinical studies:
1) A detailed and traceable cell/tissue isolation procedure – if PnD are commercially available, the company should be indicated (catalog and lot numbers, etc.).
2) Each PnD should be characterized by at least two in vitro methods: For cells, this should include the phenotype (morphology, immunolabeling for mesenchymal/epithelial markers, stem cell markers) and the ability to differentiate into all three germ layers (if stem cells are used). For the tissues, histology and immunolabeling to prove the identity/characteristics of the PnD, should be provided. In the case of purchased cells/tissues, the authors should indicate the main characteristics of PnD, as provided by the company.
3) Functional tests on cytotoxicity, promotion of proliferation, migration, and differentiation should be performed to ensure the quality of the PnD.
4) Dosage of PnD, characteristics of the wound, and the entire operating procedure, with mode and timing of application, should be described in detail so that the methodology is fully reproducible.
In addition, well-defined and standardized animal models are required for a comparison of outcome measures between studies. They may help to minimize redundancy in animal experimentation in line with the Replacement, Reduction, and Refinement (3R) principles for more ethical use of animals in research (Russell and Burch, 1960). The needs for standardization range from the choice of animal species and strains (with a preference for docile strains without barbering behavior and low anxiety levels) to precise experimental settings with regard to the extent and deepness of wounding and the adequate wound dressing. On rodents, anti-contractive strategies, ideally without suturing of devices, should be considered for long-term experiments (longer than 1 week) on full-thickness wounds.
An accurate description of the advantages or disadvantages of methods used in future publications would be helpful to standardize experiments and to enable comparability of the experiments concerning the healing outcome.
In conclusion, PnD have a promising potential to be widely used as a source of biological material to assist wound healing, now and in the future. Further concerted actions will be needed to bridge the gap between PnD basic research, pre-clinical studies, and their translation into the clinic. The COST SPRINT Action (CA17116) aims to provide comprehensive and evidence-based guidelines on all levels (Silini et al., 2020) to promote the safety and efficacy of the therapeutic use of PnD. The present review will contribute to the establishment of standards of care in wound healing.
Data Availability Statement
The original contributions presented in the study are included in the article/Supplementary Material, further inquiries can be directed to the corresponding author.
Author Contributions
ILO, MEK, SM, and PF conceptualized the review. All authors analyzed the data. MP, UJ, HO, and LT prepared the Figures and Tables. ILO wrote the Introduction. MEK, UJ, LT, HO, and SM wrote the section “PnD used for cutaneous wound healing in preclinical studies.” MP and ILO wrote the section “Animal Models of cutaneous wound healing.” ILO coordinated the work and compiled the manuscript. All authors contributed to revising and editing the manuscript and approved the submitted version.
Funding
This work was funded by: the COST Action 17116 – Sprint – International Network for Translating Research on Perinatal Derivatives into Therapeutic Approaches – CUP J56C18001930006, by the Ministry of Education, Science, and Technological Development of Republic of Serbia (Contract No. 451-03-9/2021-14/200015), by the Slovenian Research Agency (project J7-2594, and research core funding no. P3-0108), by FEDER - Fundo Europeu de Desenvolvimento Regional funds through the COMPETE 2020 - Operational Programme for Competitiveness and Internationalization (POCI), and by Portuguese funds through Fundação para a Ciência e a Tecnologia (FCT) in the framework of the project POCI-01-0145-FEDER-032610 - PTDC/MEC-DER/32610/2017. It was also supported by FCT under the project UIDB/50006/2020, UIDB/04326/2020 and UIDB/04565/2020.
Conflict of Interest
The authors declare that the research was conducted in the absence of any commercial or financial relationships that could be construed as a potential conflict of interest.
Publisher’s Note
All claims expressed in this article are solely those of the authors and do not necessarily represent those of their affiliated organizations, or those of the publisher, the editors and the reviewers. Any product that may be evaluated in this article, or claim that may be made by its manufacturer, is not guaranteed or endorsed by the publisher.
Supplementary Material
The Supplementary Material for this article can be found online at: https://www.frontiersin.org/articles/10.3389/fbioe.2021.742858/full#supplementary-material
Footnotes
2https://www.criver.com/products-services/find-model/jax-dbdb-mice?region=3616/
3https://www.jax.org/strain/009122
4https://www.criver.com/products-services/find-model/cd-1r-igs-mouse?region=3616
5https://www.criver.com/products-services/research-models-services/animal-models/mice/immunodeficient-mice/scid-mice?region=3616
7https://www.janvier-labs.com/en/fiche_produit/lewis_rat/
References
Abd-Allah, S. H., El-Shal, A. S., Shalaby, S. M., Abd-Elbary, E., Mazen, N. F., and Abdel Kader, R. R. (2015). The Role of Placenta-Derived Mesenchymal Stem Cells in Healing of Induced Full-Thickness Skin Wound in a Mouse Model. IUBMB Life 67, 701–709. doi:10.1002/iub.1427
Aguilera, V., Briceño, L., Contreras, H., Lamperti, L., Sepúlveda, E., Díaz-Perez, F., et al. (2014). Endothelium Trans Differentiated from Wharton's Jelly Mesenchymal Cells Promote Tissue Regeneration: Potential Role of Soluble Pro-angiogenic Factors. PLoS One 9, e111025. doi:10.1371/journal.pone.0111025
Akela, A., Nandi, S. K., Das, P., Banerjee, D., Roy, S., and Datta, U. (2013). Autologous Bone Marrow-Derived Cells with Placental Extract for Healing Excisional Cutaneous Wounds in Animal Model. Int. Wound J. 10, 167–176. doi:10.1111/j.1742-481X.2012.00964.x
Ankrum, J., and Karp, J. M. (2010). Mesenchymal Stem Cell Therapy: Two Steps Forward, One Step Back. Trends Mol. Med. 16, 203–209. doi:10.1016/j.molmed.2010.02.005
Arasteh, S., Khanjani, S., Golshahi, H., Mobini, S., Jahed, M. T., Heidari-Vala, H., et al. (2020). Efficient Wound Healing Using a Synthetic Nanofibrous Bilayer Skin Substitute in Murine Model. J. Surg. Res. 245, 31–44. doi:10.1016/j.jss.2019.07.017
Armstrong, D. G., Boulton, A. J. M., and Bus, S. A. (2017). Diabetic Foot Ulcers and Their Recurrence. N. Engl. J. Med. 376, 2367–2375. doi:10.1056/NEJMra1615439
Bailo, M., Soncini, M., Vertua, E., Signoroni, P. B., Sanzone, S., Lombardi, G., et al. (2004). Engraftment Potential of Human Amnion and Chorion Cells Derived from Term Placenta. Transplantation 78, 1439–1448. doi:10.1097/01.TP.0000144606.84234.49
Bakhtyar, N., Jeschke, M. G., Mainville, L., Herer, E., and Amini-Nik, S. (2017). Acellular Gelatinous Material of Human Umbilical Cord Enhances Wound Healing: A Candidate Remedy for Deficient Wound Healing. Front. Physiol. 8, 200. doi:10.3389/fphys.2017.00200
Beiki, B., Zeynali, B., and Seyedjafari, E. (2017). Fabrication of a Three Dimensional Spongy Scaffold Using Human Wharton's Jelly Derived Extra Cellular Matrix for Wound Healing. Mater. Sci. Eng. C 78, 627–638. doi:10.1016/j.msec.2017.04.074
Belzung, C., and Berton, F. (1997). Further Pharmacological Validation of the BALB/c Neophobia in the Free Exploratory Paradigm as an Animal Model of Trait Anxiety. Behav. Pharmacol. 8, 541–548. doi:10.1097/00008877-199711000-00012
Bian, X., Li, B., Yang, J., Ma, K., Sun, M., Zhang, C., et al. (2020). Regenerative and Protective Effects of dMSC-sEVs on High-Glucose-Induced Senescent Fibroblasts by Suppressing RAGE Pathway and Activating Smad Pathway. Stem Cel Res. Ther. 11, 166. doi:10.1186/s13287-020-01681-z
Bibby, G., Krasniqi, B., Reddy, I., Sekar, D., and Ross, K. (2021). Capturing the RNA Castle: Exploiting MicroRNA Inhibition for Wound Healing. FEBS J. doi:10.1111/febs.16160
Boyko, T. V., Longaker, M. T., and Yang, G. P. (2017). Laboratory Models for the Study of Normal and Pathologic Wound Healing. Plast. Reconstr. Surg. 139, 654–662. doi:10.1097/PRS.0000000000003077
Caruso, M., Evangelista, M., and Parolini, O. (2012). Human Term Placental Cells: Phenotype, Properties and New Avenues in Regenerative Medicine. Int. J. Mol. Cel Med. 1, 64–74. PMCID: PMC3920494.
Choi, J. K., Din, F., Kim, D. W., Kim, Y. I., Kim, J. O., and Ku, J. K. (2014). Amniotic Membrane extract-loaded Double-Layered Wound Dressing: Evaluation of Gel Properties and Wound healing. Drug. Dev. Ind. Pharm. 40, 852–859. doi:10.3109/03639045.2013.788015
Datta, P., and Bhattacharyy, D. (2012). “Aqueous Extract of Human Placenta,” in Recent Advances in Research on the Human Placenta. Editor J. Zheng (Rijeka, Croatia: IntechOpen Europe). doi:10.5772/31669
Davis, J. S. (1909). Skin Grafting at the Johns Hopkins Hospital. Ann. Surg. 50, 542–549. doi:10.1097/00000658-190909000-00002
Demidova-Rice, T. N., Durham, J. T., and Herman, I. M. (2012). Wound Healing Angiogenesis: Innovations and Challenges in Acute and Chronic Wound Healing. Adv. Wound Care 1, 17–22. doi:10.1089/wound.2011.0308
Deng, Q., Huang, S., Wen, J., Jiao, Y., Su, X., and Shi, G. (2020). PF-127 Hydrogel Plus Sodium Ascorbyl Phosphate Improves Wharton's jelly Mesenchymal Stem Cell-Mediated Skin Wound Healing in Mice. Stem Cell Res. 11, 143–158. doi:10.1186/s13287-020-01638-2
Deus, I. A., Mano, J. F., and Custódio, C. A. (2020). Perinatal Tissues and Cells in Tissue Engineering and Regenerative Medicine. Acta Biomater. 110, 1–14. doi:10.1016/j.actbio.2020.04.035
Du, L., Lv, R., Yang, X., Cheng, S., Ma, T., and Xu, J. (2016). Hypoxic Conditioned Medium of Placenta-Derived Mesenchymal Stem Cells Protects against Scar Formation. Life Sci. 149, 51–57. doi:10.1016/j.lfs.2016.02.050
Edwards, S. S., Zavala, G., Prieto, C. P., Elliott, M., Martínez, S., Egaña, J. T., et al. (2014). Functional Analysis Reveals Angiogenic Potential of Human Mesenchymal Stem Cells from Wharton's Jelly in Dermal Regeneration. Angiogenesis 17, 851–866. doi:10.1007/s10456-014-9432-7
Ertl, J., Pichlsberger, M., Tuca, A.-C., Wurzer, P., Fuchs, J., Geyer, S. H., et al. (2018). Comparative Study of Regenerative Effects of Mesenchymal Stem Cells Derived from Placental Amnion, Chorion and Umbilical Cord on Dermal Wounds. Placenta 65, 37–46. doi:10.1016/j.placenta.2018.04.004
Eylert, G., Dolp, R., Parousis, A., Cheng, R., Auger, C., Holter, M., et al. (2021). Skin Regeneration Is Accelerated by a Lower Dose of Multipotent Mesenchymal Stromal/stem Cells-A Paradigm Change. Stem Cel Res. Ther. 12, 82. doi:10.1186/s13287-020-02131-6
Farzamfar, S., Salehi, M., Ehterami, A., Naseri-Nosar, M., Vaez, A., Zarnani, A. H., et al. (2018). Promotion of Excisional Wound Repair by a Menstrual Blood-Derived Stem Cell-Seeded Decellularized Human Amniotic Membrane. Biomed. Eng. Lett. 8, 393–398. doi:10.1007/s13534-018-0084-1
Fukutake, M., Ochiai, D., Masuda, H., Abe, Y., Sato, Y., Otani, T., et al. (2019). Human Amniotic Fluid Stem Cells Have a Unique Potential to Accelerate Cutaneous Wound Healing With Reduced Fibrotic Scarring Like a Fetus. Human Cell 32, 51–63. doi:10.1007/s13577-018-0222-1
Gangoda, L., Boukouris, S., Liem, M., Kalra, H., and Mathivanan, S. (2015). Extracellular Vesicles Including Exosomes Are Mediators of Signal Transduction: Are They Protective or Pathogenic? Proteomics 15, 260–271. doi:10.1002/pmic.201400234
Gao, S., Chen, T., Hao, Y., Zhang, F., Tang, X., Wang, D., et al. (2020). Exosomal miR-135a Derived from Human Amnion Mesenchymal Stem Cells Promotes Cutaneous Wound Healing in Rats and Fibroblast Migration by Directly Inhibiting LATS2 Expression. Stem Cel Res. Ther. 11, 56. doi:10.1186/s13287-020-1570-9
Gholipourmalekabadi, M., Seifalian, A. M., Urbanska, A. M., Omrani, M. D., Hardy, J. G., Madjd, Z., et al. (2018). 3D Protein-Based Bilayer Artificial Skin for the Guided Scarless Healing of Third-Degree Burn Wounds In Vivo. Biomacromolecules 19, 2409–2422. doi:10.1021/acs.biomac.7b01807
Griebel, G., Belzung, C., Misslin, R., and Vogel, E. (1993). The Free-Exploratory Paradigm. Behav. Pharmacol. 4, 637–644. doi:10.1097/00008877-199312000-00009
Gunawardena, T. N. A., Rahman, M. T., Abdullah, B. J. J., and Abu Kasim, N. H. (2019). Conditioned media Derived from Mesenchymal Stem Cell Cultures: The Next Generation for Regenerative Medicine. J. Tissue Eng. Regen. Med. 13, 569–586. doi:10.1002/term.2806
Hammond, S. (2000). Transcutaneous Immunization of Domestic Animals: Opportunities and Challenges. Adv. Drug Deliv. Rev. 43, 45–55. doi:10.1016/s0169-409x(00)00076-4
Han, Y., Tao, R., Han, Y., Sun, T., Chai, J., Xu, G., et al. (2014). Microencapsulated VEGF Gene-Modified Umbilical Cord Mesenchymal Stromal Cells Promote the Vascularization of Tissue-Engineered Dermis: an Experimental Study. Cytotherapy 16, 160–169. doi:10.1016/j.jcyt.2013.10.014
Han, Y., Sun, T., Han, Y., Lin, L., Liu, C., Liu, J., et al. (2019). Human Umbilical Cord Mesenchymal Stem Cells Implantation Accelerates Cutaneous Wound Healing in Diabetic Rats via the Wnt Signaling Pathway. Eur. J. Med. Res. 24, 10. doi:10.1186/s40001-019-0366-9
Hashemi, S. S., Pourfath, M. R., Derakhshanfar, A., Behzad-Behbahani, A., and Moayedi, J. (2020). The Role of Labeled Cell Therapy with and without Scaffold in Early Excision Burn Wounds in a Rat Animal Model. Iran J. Basic Med. Sci. 23, 673–679. doi:10.22038/ijbms.2020.34324.8156
He, L., Tu, H., He, W., Guo, L. L., and Yu, S. (2015). Lentiviral-Mediated Overexpression of Homeobox A4 by Human Umbilical Cord Mesenchymal Stem Cells Repairs Full-Thickness Skin Defects. Mol. Med. Rep. 11, 3517–3522. doi:10.3892/mmr.2015.3208
Hong, J. W., Lee, W. J., Hahn, S. B., Kim, B. J., and Lew, D. H. (2010). The Effect of Human Placenta Extract in a Wound Healing Model. Ann. Plast. Surg. 35, 96–100. doi:10.1097/SAP.0b013e3181b0bb67
Hymes, S. R., Strom, E. A., and Fife, C. (2006). Radiation Dermatitis: Clinical Presentation, Pathophysiology, and Treatment 2006. J. Am. Acad. Dermatol. 54, 28–46. doi:10.1016/j.jaad.2005.08.054
Jerman, U. D., Veranič, P., and Kreft, M. E. (2014). Amniotic Membrane Scaffolds Enable the Development of Tissue-Engineered Urothelium with Molecular and Ultrastructural Properties Comparable to that of Native Urothelium. Tissue Eng. C Methods 20, 317–327. doi:10.1089/ten.TEC.2013.0298
Jerman, U. D., Veranič, P., Cirman, T., and Kreft, M. E. (2020). Human Amniotic Membrane Enriched with Urinary Bladder Fibroblasts Promote the Re-Epithelization of Urothelial Injury. Cel Transpl. 29, 096368972094666. doi:10.1177/0963689720946668
Jin, E., Kim, T.-H., Han, S., and Kim, S.-W. (2016). Amniotic Epithelial Cells Promote Wound Healing in Mice through High Epithelialization and Engraftment. J. Tissue Eng. Regen. Med. 10, 613–622. doi:10.1002/term.2069
Jirsova, K., and Jones, G. L. A. (2017). Amniotic Membrane in Ophthalmology: Properties, Preparation, Storage and Indications for Grafting-A Review. Cell Tissue Bank 18, 193–204. doi:10.1007/s10561-017-9618-5
Kakabadze, Z., Chakhunashvili, D., Chakhunashvili, D., Gogilashvili, K., Ediberidze, K., Chakhunashvili, K., et al. (2019). Bone Marrow Stem Cell and Decellularized Human Amniotic Membrane for the Treatment of Nonhealing Wound after Radiation Therapy. Exp. Clin. Transpl. 17, 92–98. doi:10.6002/ect.MESOT2018.O29
Kaushik, K., and Das, A. (2019). Cycloxygenase-2 Inhibition Potentiates Trans-differentiation of Wharton's Jelly-Mesenchymal Stromal Cells into Endothelial Cells: Transplantation Enhances Neovascularization-Mediated Wound Repair. Cytotherapy 21, 260–273. doi:10.1016/j.jcyt.2019.01.004
Keller, S., Sanderson, M. P., Stoeck, A., and Altevogt, P. (2006). Exosomes: from Biogenesis and Secretion to Biological Function. Immunol. Lett. 107, 102–108. doi:10.1016/j.imlet.2006.09.005
Kim, S. S., Song, C. K., Shon, S. K., Lee, K. Y., Kim, C. H., Lee, M. J., et al. (2009). Effects of Human Amniotic Membrane Grafts Combined with Marrow Mesenchymal Stem Cells on Healing of Full-Thickness Skin Defects in Rabbits. Cell Tissue Res. 336, 59–66. doi:10.1007/s00441-009-0766-1
Kim, S.-W., Zhang, H.-Z., Guo, L., Kim, J.-M., and Kim, M. H. (2012). Correction: Amniotic Mesenchymal Stem Cells Enhance Wound Healing in Diabetic NOD/SCID Mice through High Angiogenic and Engraftment Capabilities. PLoS One 7. doi:10.1371/annotation/f6ebe3d3-ef7c-42ce-86fe-d5a661d7f67f
Kinzer, M., Hingerl, K., König, J., Reinisch, A., Strunk, D., Huppertz, B., et al. (2014). Mesenchymal Stromal Cells from the Human Placenta Promote Neovascularization in a Mouse Model In Vivo. Placenta 35, 517–519. doi:10.1016/j.placenta.2014.04.004
König, J., Huppertz, B., Desoye, G., Parolini, O., Fröhlich, J. D., Weiss, G., et al. (2012). Amnion-derived Mesenchymal Stromal Cells Show Angiogenic Properties but Resist Differentiation into Mature Endothelial Cells. Stem Cell Develop. 21, 1309–1320. doi:10.1089/scd.2011.0223
König, J., Weiss, G., Rossi, D., Wankhammer, K., Reinisch, A., Kinzer, M., et al. (2015). Placental Mesenchymal Stromal Cells Derived from Blood Vessels or Avascular Tissues: what Is the Better Choice to Support Endothelial Cell Function? Stem Cell Develop. 24, 115–131. doi:10.1089/scd.2014.0115
Koob, T. J., Rennert, R., Zabek, N., Massee, M., Lim, J. J., Temenoff, J. S., et al. (2013). Biological Properties of Dehydrated Human Amnion/chorion Composite Graft: Implications for Chronic Wound Healing. Int. Wound J. 10, 493–500. doi:10.1111/iwj.12140
Koob, T. J., Lim, J. J., Massee, M., Zabek, N., Rennert, R., Gurtner, G., et al. (2014). Angiogenic Properties of Dehydrated Human Amnion/chorion Allografts: Therapeutic Potential for Soft Tissue Repair and Regeneration. Vasc. Cel 6, 10. doi:10.1186/2045-824X-6-10
Kwon, J. W., Hong, S. E., Kang, S. R., and Park, B. Y. (2019). Effect of Human Placental Extract Treatment on Random-Pattern Skin Flap Survival in Rats. J. Invest. Surg. 32, 304–313. doi:10.1080/08941939.2017.1417518
Lazarus, G. S., Cooper, D. M., Knighton, D. R., Margolis, D. J., Percoraro, R. E., Rodeheaver, G., et al. (1994). Definitions and Guidelines for Assessment of Wounds and Evaluation of Healing. Wound Repair Regen. 2, 165–170. doi:10.1046/j.1524-475X.1994.20305.x
Leng, X., Zhang, Q., Zhai, X., and Chen, Z. (2012). Local Transplant of Human Umbilical Cord Matrix Stem Cells Improves Skin Flap Survival in a Mouse Model. Tohoku J. Exp. Med. 227, 191–197. doi:10.1620/tjem.227.191
Li, J.-Y., Ren, K.-K., Zhang, W.-J., Xiao, L., Wu, H.-Y., Liu, Q.-Y., et al. (2019). Human Amniotic Mesenchymal Stem Cells and Their Paracrine Factors Promote Wound Healing by Inhibiting Heat Stress-Induced Skin Cell Apoptosis and Enhancing Their Proliferation through Activating PI3K/AKT Signaling Pathway. Stem Cel Res. Ther. 10, 247. doi:10.1186/s13287-019-1366-y
Liu, L., Yu, Y., Hou, Y., Chai, J., Duan, H., Chu, W., et al. (2014). Human Umbilical Cord Mesenchymal Stem Cells Transplantation Promotes Cutaneous Wound Healing of Severe Burned Rats. PLoS One 9, e88348. doi:10.1371/journal.pone.0088348
Liu, L., Song, H., Duan, H., Chai, J., Yang, J., Li, X., et al. (2016). TSG-6 Secreted by Human Umbilical Cord-MSCs Attenuates Severe Burn-Induced Excessive Inflammation via Inhibiting Activations of P38 and JNK Signaling. Sci. Rep. 6, 30121. doi:10.1038/srep30121
Liu, Z., Yu, D., Xu, J., Li, X., Wang, X., He, Z., et al. (2018). Human Umbilical Cord Mesenchymal Stem Cells Improve Irradiation-Induced Skin Ulcers Healing of Rat Models. Biomed. Pharmacother. 101, 729–736. doi:10.1016/j.biopha.2018.02.093
Liu, J., Yan, Z., Yang, F., Huang, Y., Yu, Y., Zhou, L., et al. (2021). Exosomes Derived from Human Umbilical Cord Mesenchymal Stem Cells Accelerate Cutaneous Wound Healing by Enhancing Angiogenesis through Delivering Angiopoietin-2. Stem Cel Rev. Rep. 17, 305–317. doi:10.1007/s12015-020-09992-7
Maguire, G. (2013). Stem Cell Therapy without the Cells. Commun. Integr. Biol. 6, e26631. doi:10.4161/cib.26631
Mahmood, R., Mehmood, A., Choudhery, M. S., Awan, S. J., Khan, S. N., and Riazuddin, S. (2019). Human Neonatal Stem Cell-Derived Skin Substitute Improves Healing of Severe Burn Wounds in a Rat Model. Cell Biol. Int. 43, 147–157. doi:10.1002/cbin.11072
McKenzie, J., Brennan, S., Ryan, R., Thomson, H., Johnston, R., and Thomas, J. (2021). “Chapter 3: Defining the Criteria for Including Studies and How They Will Be Grouped for the Synthesis,” in Cochrane Handbook for Systematic Reviews of Interventions Version 6.2. Editors J. Higgins, J. Thomas, J. Chandler, M. Cumpston, T. Li, M. Pageet al. (Wiley Blackwell: London, UK). Available at: https://training.cochrane.org/handbook/current/chapter-03 (Accessed March 30, 2021).
Milan, P. B., Lotfibakhshaiesh, N., Joghataie, M. T., Ai, J., Pazouki, A., Kaplan, D. L., et al. (2016). Accelerated Wound Healing in a Diabetic Rat Model Using Decellularized Dermal Matrix and Human Umbilical Cord Perivascular Cells. Acta Biomater. 45, 234–246. doi:10.1016/j.actbio.2016.08.053
Mogil, J. S., Wilson, S. G., Bon, K., Eun Lee, S., Chung, K., Raber, P., et al. (1999). Heritability of Nociception I: Responses of 11 Inbred Mouse Strains on 12 Measures of Nociception. Pain 80, 67–82. doi:10.1016/S0304-3959(98)00197-3
Momeni, M., Zarehaghighi, M., Hajimiri, M., Khorasani, G., Dinarvand, R., Nekookar, A., et al. (2018). In Vitro and In Vivo Investigation of a Novel Amniotic‐based Chitosan Dressing for Wound Healing. Wound Rep. Reg. 26, 87–101. doi:10.1111/wrr.12618
Montanucci, P., Di Pasquali, C., Ferri, I., Pescara, T., Pennoni, I., Siccu, P., et al. (2017). Human Umbilical Cord Wharton Jelly-Derived Adult Mesenchymal Stem Cells, in Biohybrid Scaffolds, for Experimental Skin Regeneration. Stem Cell Int. 2017, 1–13. doi:10.1155/2017/1472642
Motamed, S., Taghiabadi, E., Molaei, H., Sodeifi, N., Hassanpour, S. E., Shafieyan, S., et al. (2017). Cell-based Skin Substitutes Accelerate Regeneration of Extensive Burn Wounds in Rats. Am. J. Surg. 214, 762–769. doi:10.1016/j.amjsurg.2017.04.010
Mulholland, E. J. (2020). Electrospun Biomaterials in the Treatment and Prevention of Scars in Skin Wound Healing. Front. Bioeng. Biotechnol. 8, 481. doi:10.3389/fbioe.2020.00481
Mukherjee, C., Saleem, S., Das, S., Biswas, S. C., and Bhattacharyya, D. (2020). Human Placental Laminin: Role in Neuronal Differentiation, Cell Adhesion and Proliferation. J. Biosci. 45, 93–115. doi:10.1007/s12038-020-00043-4
Murphy, S. V., Skardal, A., Song, L., Sutton, K., Haug, R., Mack, D. L., et al. (2017). Solubilized Amnion Membrane Hyaluronic Acid Hydrogel Accelerates Full-Thickness Wound Healing. Stem Cell Transl. Med. 6, 2020–2032. doi:10.1002/sctm.17-0053
Murphy, S. V., Skardal, A., Nelson, R. A., Sunnon, K., Reid, T., Clouse, C., et al. (2020). Amnion Membrane Hydrogel and Amnion Membrane Powder Accelerate Wound Healing in a Full Thickness Porcine Skin Wound Model. Stem Cell Transl. Med. 9, 80–92. doi:10.1002/sctm.19-0101
Nan, W., Liu, R., Chen, H., Xu, Z., Chen, J., Wang, M., et al. (2015). Umbilical Cord Mesenchymal Stem Cells Combined with a Collagenfibrin Double-Layered Membrane Accelerates Wound Healing. Wounds 27, 134–140. PMID: 25965183.
Nasiry, D., Khalatbary, A. R., Abdollahifar, M.-A., Amini, A., Bayat, M., Noori, A., et al. (2020). Engraftment of Bioengineered Three-Dimensional Scaffold from Human Amniotic Membrane-Derived Extracellular Matrix Accelerates Ischemic Diabetic Wound Healing. Arch. Dermatol. Res. 313, 567. doi:10.1007/s00403-020-02137-3
Omran, E., Hieneedy, H., Halwagy, A., Al-Inany, H., Al-Ansary, M., and Gad, A. (2016). Amniotic Membrane Can Be a Valid Source for Wound Healing. Int. J. Womens Health 8, 225–231. doi:10.2147/IJWH.S96636
Page, M. J., McKenzie, J. E., Bossuyt, P. M., Boutron, I., Hoffmann, T. C., Mulrow, C. D., et al. (2021a). The PRISMA 2020 Statement: an Updated Guideline for Reporting Systematic Reviews. BMJ 372, n71. doi:10.1136/bmj.n71
Page, M. J., Moher, D., Bossuyt, P. M., Boutron, I., Hoffmann, T. C., Mulrow, C. D., et al. (2021b). PRISMA 2020 Explanation and Elaboration: Updated Guidance and Exemplars for Reporting Systematic Reviews. BMJ 372, n160. doi:10.1136/bmj.n160
Parolini, O., Alviano, F., Bagnara, G. P., Bilic, G., Bühring, H.-J., Evangelista, M., et al. (2008). Concise Review: Isolation and Characterization of Cells from Human Term Placenta: Outcome of the First International Workshop on Placenta Derived Stem Cells. Stem Cells 26, 300–311. doi:10.1634/stemcells.2007-0594
Payne, W. G., Wachtel, T. L., Smith, C. A., Uberti, M. G., Ko, F., and Robson, M. C. (2010). Effect of Amnion-Derived Cellular Cytokine Solution on Healing of Experimental Partial-Thickness burns. World J. Surg. 34, 1663–1668. doi:10.1007/s00268-010-0420-9
Peña-Villalobos, I., Casanova-Maldonado, I., Lois, P., Prieto, C., Pizarro, C., Lattus, J., et al. (2018). Hyperbaric Oxygen Increases Stem Cell Proliferation, Angiogenesis and Wound-Healing Ability of WJ-MSCs in Diabetic Mice. Front. Physiol. 9, 995. doi:10.3389/fphys.2018.00995
Pogozhykh, O., Prokopyuk, V., Figueiredo, C., and Pogozhykh, D. (2018). Placenta and Placental Derivatives in Regenerative Therapies: Experimental Studies, History, and Prospects. Stem Cell Int. 2018, 1–14. doi:10.1155/2018/4837930
Pourfath, M. R., Behzad-Behbahani, A., Hashemi, S. S., Derakhsahnfar, A., Taheri, M. N., and Salehi, S. (2018). Monitoring Wound Healing of Burn in Rat Model Using Human Wharton's Jelly Mesenchymal Stem Cells Containing cGFP Integrated by Lentiviral Vectors. Iran J. Basic Med. Sci. 21, 70–76. doi:10.22038/IJBMS.2017.19783.5212
Poženel, L., Lindenmair, A., Schmidt, K., Kozlov, A. V., Grillari, J., Wolbank, S., et al. (2019). Critical Impact of Human Amniotic Membrane Tension on Mitochondrial Function and Cell Viability In Vitro. Cells 8, 1641. doi:10.3390/cells8121641
Rahman, M. S., Islam, R., Rana, M. M., Spitzhorn, L.-S., Rahman, M. S., Adjaye, J., et al. (2019). Characterization of Burn Wound Healing Gel Prepared from Human Amniotic Membrane and Aloe Vera Extract. BMC Complement. Altern. Med. 19, 115. doi:10.1186/s12906-019-2525-5
Raj, V., Claudine, S., Subramanian, A., Tam, K., Biswas, A., Bongso, A., et al. (2019). Histological, Immunohistochemical, and Genomic Evaluation of Excisional and Diabetic Wounds Treated with Human Wharton's Jelly Stem Cells with and without a Nanocarrier. J. Cel Biochem. 120, 11222–11240. doi:10.1002/jcb.28398
Rameshbabu, A. P., Datta, S., Bankoti, K., Subramani, E., Chaudhury, K., Lalzawmliana, V., et al. (2018). Polycaprolactone Nanofibers Functionalized with Placental Derived Extracellular Matrix for Stimulating Wound Healing Activity. J. Mater. Chem. B 6, 6767–6780. doi:10.1039/C8TB01373J
Ramuta, T. Ž., Jerman, U. D., Tratnjek, L., Janev, A., Magatti, M., Vertua, E., et al. (2020). The Cells and Extracellular Matrix of Human Amniotic Membrane Hinder the Growth and Invasive Potential of Bladder Urothelial Cancer Cells. Front. Bioeng. Biotechnol. 8, 554530. doi:10.3389/fbioe.2020.554530
Rashed, M. H., Bayraktar, E., Helal, G. K., Abd-Ellah, M., Amero, P., Chavez-Reyes, A., et al. (2017). Exosomes: From Garbage Bins to Promising Therapeutic Targets. Int. J. Mol. Sci. 18, 538. doi:10.3390/ijms18030538
Ribeiro, J., Pereira, T., Amorim, I., Caseiro, A. R., Lopes, M. A., Lima, J., et al. (2014). Cell Therapy with Human MSCs Isolated from the Umbilical Cord Wharton Jelly Associated to a PVA Membrane in the Treatment of Chronic Skin Wounds. Int. J. Med. Sci. 11, 979–987. doi:10.7150/ijms.9139
Robson, M. C., DelBeccaro, E. J., Heggers, J. P., and Loy, G. L. (1980). Increasing Dermal Perfusion after Burning by Decreasing Thromboxane Production. J. Trauma Inj. Infect. Crit. Care 20, 722–725. doi:10.1097/00005373-198009000-00002
Russell, M. S., and Burch, R. L. (1960). The Principles of Humane Experimental Technique. Med. J. Aust. 1, 500. doi:10.5694/j.1326-5377.1960.tb73127.x
Sabapathy, V., Sundaram, B., Sreelakshmi, V. M., Mankuzhy, P., and Kumar, S. (2014). Human Wharton's Jelly Mesenchymal Stem Cells Plasticity Augments Scar-free Skin Wound Healing with Hair Growth. PLoS One 9, e93726. doi:10.1371/journal.pone.0093726
Sabella, N. (1913). Use of Fetal Membranes in Skin Grafting. Medication Reconciliation 83, 478–480. doi:10.1177/001452461302401011
Sabzevari, R., Roushandeh, A. M., Mehdipour, A., Alini, M., and Roudkenar, M. H. (2020). SA/G Hydrogel Containing hCAP-18/LL-37-Engineered WJ-MSCs-Derived Conditioned Medium Promoted Wound Healing in Rat Model of Excision Injury. Life Sci. 261, 118381. doi:10.1016/j.lfs.2020.118381
Samadikuchaksaraei, A., Mehdipour, A., Habibi Roudkenar, M., Verdi, J., Joghataei, M. T., As'adi, K., et al. (2016). A Dermal Equivalent Engineered with TGF-β3 Expressing Bone Marrow Stromal Cells and Amniotic Membrane: Cosmetic Healing of Full-Thickness Skin Wounds in Rats. Artif. Organs 40, E266–E279. doi:10.1111/aor.12807
Sarna, J. (2000). The Dalila Effect: C57BL6 Mice Barber Whiskers by Plucking. Behav. Brain Res. 108, 39–45. doi:10.1016/S0166-4328(99)00137-0
Schmauss, D., Weinzierl, A., Schmauss, V., and Harder, Y. (2018). Common Rodent Flap Models in Experimental Surgery. Eur. Surg. Res. 59, 255–264. doi:10.1159/000492414
Sen, C. K., Gordillo, G. M., Roy, S., Kirsner, R., Lambert, L., Hunt, T. K., et al. (2009). Human Skin Wounds: a Major and Snowballing Threat to Public Health and the Economy. Wound Repair Regen. 17, 763–771. doi:10.1111/j.1524-475X.2009.00543.x
Shi, R., Lian, W., Jin, Y., Cao, C., Han, S., Yang, X., et al. (2020). Role and Effect of Vein-Transplanted Human Umbilical Cord Mesenchymal Stem Cells in the Repair of Diabetic Foot Ulcers in Rats. Acta Biochim. Biophys. Sin. (Shanghai) 52, 620–630. doi:10.1093/abbs/gmaa039
Shohara, R., Yamamoto, A., Takikawa, S., Iwase, A., Hibi, H., Kikkawa, F., et al. (2012). Mesenchymal Stromal Cells of Human Umbilical Cord Wharton's Jelly Accelerate Wound Healing by Paracrine Mechanisms. Cytotherapy 14, 1171–1181. doi:10.3109/14653249.2012.706705
Shrestha, C., Zhao, L., Chen, K., He, H., and Mo, Z. (2013). Enhanced Healing of Diabetic Wounds by Subcutaneous Administration of Human Umbilical Cord Derived Stem Cells and Their Conditioned media. Int. J. Endocrinol. 2013, 1–10. doi:10.1155/2013/592454
Silini, A. R., Cargnoni, A., Magatti, M., Pianta, S., and Parolini, O. (2015). The Long Path of Human Placenta, and its Derivatives, in Regenerative Medicine. Front. Bioeng. Biotechnol. 3, 162. doi:10.3389/fbioe.2015.00162
Silini, A. R., Di Pietro, R., Lang-Olip, I., Alviano, F., Banerjee, A., Basile, M., et al. (2020). Perinatal Derivatives: Where Do We Stand? A Roadmap of the Human Placenta and Consensus for Tissue and Cell Nomenclature. Front. Bioeng. Biotechnol. 8, 610544. doi:10.3389/fbioe.2020.610544
Song, M., Wang, W., Ye, Q., Bu, S., Shen, Z., and Zhu, Y. (2017). The Repairing of Full-Thickness Skin Deficiency and its Biological Mechanism Using Decellularized Human Amniotic Membrane as the Wound Dressing. Mater. Sci. Eng. C 77, 739–747. doi:10.1016/j.msec.2017.03.232
Stern, M. (1913). The Grafting of Preserved Amniotic Membrane to Burned and Ulcerated Surfaces, Substituing Skin Grafts. JAMA 60, 973. doi:10.1001/jama.1913.04340130021008
Stojadinovic, A., Carlson, J. W., Schultz, G. S., Davis, T. A., and Elster, E. A. (2008). Topical Advances in Wound Care. Gynecol. Oncol. 111, S70–S80. doi:10.1016/j.ygyno.2008.07.042
Sun, J., Zhang, Y., Song, X., Zhu, J., and Zhu, Q. (2019). The Healing Effects of Conditioned Medium Derived from Mesenchymal Stem Cells on Radiation-Induced Skin Wounds in Rats. Cel Transpl. 28, 105–115. doi:10.1177/0963689718807410
Théry, C., Witwer, K. W., Aikawa, E., Alcaraz, M. J., Anderson, J. D., Andriantsitohaina, R., et al. (2018). Minimal Information for Studies of Extracellular Vesicles 2018 (MISEV2018): a Position Statement of the International Society for Extracellular Vesicles and Update of the MISEV2014 Guidelines. J. Extracell Vesicles 7, 1535750. doi:10.1080/20013078.2018.1535750
Tuca, A.-C., Ertl, J., Hingerl, K., Pichlsberger, M., Fuchs, J., Wurzer, P., et al. (2016). Comparison of Matrigel and Matriderm as a Carrier for Human Amnion-Derived Mesenchymal Stem Cells in Wound Healing. Placenta 48, 99–103. doi:10.1016/j.placenta.2016.10.015
Ueta, M., Kweon, M.-N., Sano, Y., Sotozono, C., Yamada, J., Koizumi, N., et al. (2002). Immunosuppressive Properties of Human Amniotic Membrane for Mixed Lymphocyte Reaction. Clin. Exp. Immunol. 129, 464–470. doi:10.1046/j.1365-2249.2002.01945.x
van Deun, J., Van Deun, J., Mestdagh, P., Agostinis, P., Akay, Ö., Anand, S., et al. (2017). EV-TRACK: Transparent Reporting and Centralizing Knowledge in Extracellular Vesicle Research. Nat. Methods 14, 228–232. doi:10.1038/nmeth.4185
Vonbrunn, E., Mueller, M., Pichlsberger, M., Sundl, M., Helmer, A., Wallner, S. A., et al. (2020). Electrospun PCL/PLA Scaffolds Are More Suitable Carriers of Placental Mesenchymal Stromal Cells Than Collagen/Elastin Scaffolds and Prevent Wound Contraction in a Mouse Model of Wound Healing. Front. Bioeng. Biotechnol. 8, 604123. doi:10.3389/fbioe.2020.604123
Wang, S., Yang, H., Tang, Z., Long, G., and Huang, W. (2016). Wound Dressing Model of Human Umbilical Cord Mesenchymal Stem Cells-Alginates Complex Promotes Skin Wound Healing by Paracrine Signaling. Stem Cell Int. 2016, 1–8. doi:10.1155/2016/3269267
Wang, S., Mo, M., Wang, J., Sadia, S., Shi, B., Fu, X., et al. (2018). Platelet-derived Growth Factor Receptor Beta Identifies Mesenchymal Stem Cells with Enhanced Engraftment to Tissue Injury and Pro-angiogenic Property. Cell. Mol. Life Sci. 75, 547–561. doi:10.1007/s00018-017-2641-7
Weidinger, A., Poženel, L., Wolbank, S., and Banerjee, A. (2020). Sub-Regional Differences of the Human Amniotic Membrane and Their Potential Impact on Tissue Regeneration Application. Front. Bioeng. Biotechnol. 8, 613804. doi:10.3389/fbioe.2020.613804
Xue, M., Zhao, R., Lin, H., and Jackson, C. (2018). Delivery Systems of Current Biologicals for the Treatment of Chronic Cutaneous Wounds and Severe burns. Adv. Drug Deliv. Rev. 129, 219–241. doi:10.1016/j.addr.2018.03.002
Yang, J. D., Choi, D. S., Cho, Y. K., Kim, T. K., Lee, J. W., Choi, K. Y., et al. (2013). Effect of Amniotic Fluid Stem Cells and Amniotic Fluid Cells on the Wound Healing Process in a white Rat Model. Arch. Plast. Surg. 40, 496–504. doi:10.5999/aps.2013.40.5.496
Yang, K., Li, D., Wang, M., Xu, Z., Chen, X., Liu, Q., et al. (2019). Exposure to Blue Light Stimulates the Proangiogenic Capability of Exosomes Derived from Human Umbilical Cord Mesenchymal Stem Cells. Stem Cel Res. Ther. 10, 358. doi:10.1186/s13287-019-1472-x
Yang, J., Chen, Z., Pan, D., Li, H., and Shen, J. (2020). Umbilical Cord-Derived Mesenchymal Stem Cell-Derived Exosomes Combined Pluronic F127 Hydrogel Promote Chronic Diabetic Wound Healing and Complete Skin Regeneration. Int. J. Nanomedicine 15, 5911–5926. doi:10.2147/IJN.S249129
Yue, C., Guo, Z., Luo, Y., Yuan, J., Wan, X., and Mo, Z. (2020). c-Jun Overexpression Accelerates Wound Healing in Diabetic Rats by Human Umbilical Cord-Derived Mesenchymal Stem Cells. Stem Cell Int. 2020, 1–10. doi:10.1155/2020/7430968
Zebardast, N., Lickorish, D., and Davies, J. E. (2010). Human Umbilical Cord Perivascular Cells (HUCPVC). Organogenesis 6, 197–203. doi:10.4161/org.6.4.12393
Zhang, B., Wang, M., Gong, A., Zhang, X., Wu, X., Zhu, Y., et al. (2015a). HucMSC-Exosome Mediated-Wnt4 Signaling Is Required for Cutaneous Wound Healing. Stem Cells 33, 2158–2168. doi:10.1002/stem.1771
Zhang, B., Wu, X., Zhang, X., Sun, Y., Yan, Y., Shi, H., et al. (2015b). Human Umbilical Cord Mesenchymal Stem Cell Exosomes Enhance Angiogenesis through the Wnt4/β-Catenin Pathway. Stem Cell Transl. Med. 4, 513–522. doi:10.5966/sctm.2014-0267
Zhang, B., Shi, Y., Gong, A., Pan, Z., Shi, H., Yang, H., et al. (2016). HucMSC Exosome-Delivered 14-3-3ζ Orchestrates Self-Control of the Wnt Response via Modulation of YAP during Cutaneous Regeneration. Stem Cells 34, 2485–2500. doi:10.1002/stem.2432
Zhang, S., Chen, L., Zhang, G., and Zhang, B. (2020). Umbilical Cord-Matrix Stem Cells Induce the Functional Restoration of Vascular Endothelial Cells and Enhance Skin Wound Healing in Diabetic Mice via the Polarized Macrophages. Stem Cel Res. Ther. 11, 39. doi:10.1186/s13287-020-1561-x
Zhang, Z., Li, Z., Li, Y., Wang, Y., Yao, M., Zhang, K., et al. (2021). Sodium Alginate/Collagen Hydrogel Loaded With Human Umbilical Cord Mesenchymal Stem Cells Promotes Wound Healing and Skin Remodeling. Cell Tissue Res. 383, 809–821. doi:10.1007/s00441-020-03321-7
Zhao, G., Liu, F., Liu, Z., Zuo, K., Wang, B., Zhang, Y., et al. (2020). MSC-derived Exosomes Attenuate Cell Death through Suppressing AIF Nucleus Translocation and Enhance Cutaneous Wound Healing. Stem Cel Res. Ther. 11, 174. doi:10.1186/s13287-020-01616-8
Zhao, B., Liu, J. Q., Zhang, Z., Zhang, J., Wang, S. Y., and Han, S. C. (2018). Human Amniotic Epithelial Stem Cells Promote Wound Healing by Facilitating Migration and Proliferation of Keratinocytes via ERK, JNK and AKT Signaling Pathways. Cell Tissue Res. 365, 85–99. doi:10.1007/s00441-016-2366-1
Keywords: perinatal derivatives, placenta, cells, preclinical studies, animal models, wound healing, skin, cutaneous
Citation: Pichlsberger M, Jerman UD, Obradović H, Tratnjek L, Macedo AS, Mendes F, Fonte P, Hoegler A, Sundl M, Fuchs J, Schoeberlein A, Kreft ME, Mojsilović S and Lang-Olip I (2021) Systematic Review of the Application of Perinatal Derivatives in Animal Models on Cutaneous Wound Healing. Front. Bioeng. Biotechnol. 9:742858. doi: 10.3389/fbioe.2021.742858
Received: 16 July 2021; Accepted: 06 September 2021;
Published: 24 September 2021.
Edited by:
Peter Ponsaerts, University of Antwerp, BelgiumReviewed by:
Chia-Ching (Josh) Wu, National Cheng Kung University, TaiwanMenekse Ermis Sen, Terasaki Institute for Biomedical Innovation, United States
Copyright © 2021 Pichlsberger, Jerman, Obradović, Tratnjek, Macedo, Mendes, Fonte, Hoegler, Sundl, Fuchs, Schoeberlein, Kreft, Mojsilović and Lang-Olip. This is an open-access article distributed under the terms of the Creative Commons Attribution License (CC BY). The use, distribution or reproduction in other forums is permitted, provided the original author(s) and the copyright owner(s) are credited and that the original publication in this journal is cited, in accordance with accepted academic practice. No use, distribution or reproduction is permitted which does not comply with these terms.
*Correspondence: Ingrid Lang-Olip, SW5ncmlkLmxhbmdAbWVkdW5pZ3Jhei5hdA==
†These authors have contributed equally to this work