- 1Department of Biology, University of Florence, Florence, Italy
- 2ASAcampus Joint Laboratory, ASA Res. Div., Deptartment of Experimental and Clinical Biomedical Sciences “Mario Serio”, University of Florence, Florence, Italy
- 3Emergency Surgery Unit-Trauma Team, Emergency Department, Department of Experimental and Clinical Medicine, Careggi University Hospital, University of Florence, Florence, Italy
Wound healing is slowed in Space. Microgravity and possible physical factors associated with Space affect alterations in fibroblast, matrix formation, dysregulation in apoptosis and inflammation. The microbial populations settled on skin, space modules, in space suits, are also playing a pivotal role, as wound healing is also affected by the microbial community. We propose a perspective that includes four domines for the application of human skin microbiota for wound healing in Space: The natural antimicrobial properties of the skin microbiota, the crosstalk of the skin microbiota with the immune system during wound healing, the contribution of the microbiota in precision medicine, and the role of gut-skin and gut-brain axes. A stronger understanding of the connections and metabolic network among bacteria, fungi, the host’s immune system and the host metabolism will support the basis for a better wound healing in Space.
Introduction
Wounding can happen during Space exploration (Gontcharov et al., 2005; Tronnier et al., 2008; Crucian et al., 2016; Farkas and Farkas, 2021). Astronauts are exposed to skin erythema, peeling, dryness, burning, pruritus, sensitivity, thinning, therefore the physiology of the healing process of cutaneous injuries is hence affected (Farahani and DiPietro, 2008; Cubo-Mateo and Gelinsky, 2021). Recent studies have confirmed that wound healing is altered during Space missions (Cubo-Mateo and Gelinsky, 2021). During the normal wound healing process and under normal gravity (on earth), the body can repair wounds, trauma, burns by recreating the skin barrier (Reinke and Sorg, 2012; Singh et al., 2017; Maheswary et al., 2021). The physiology of wound healing under normal conditions is achieved via several defined steps: inflammation, proliferation, epithelialization, maturation, and remodelling phases (Reinke and Sorg, 2012; Singh et al., 2017; Maheswary et al., 2021). Chronic wounds extend the inflammatory phase, with immune cells continually degrading collagen and extracellular matrix (Blaber et al., 2010; Dam and Paller, 2018; Jemison and Olabisi, 2021). The delayed wound healing induced by microgravity differs in aetiology from that of chronic wounds on earth (Jemison and Olabisi, 2021). Microgravity affects matrix formation (Blaber et al., 2010), the alterations in fibroblast and dysregulation in apoptosis (Cialdai and Monici, 2013), inflammation (Blaber et al., 2010), delayed cellular proliferation of the basal skin layer and a thinning of the upper layer of the epidermis (this study is based on a single crew member) (Tronnier et al., 2008). Space radiation including solar particle events, galactic cosmic radiation and intra-vehicular secondary radiation may also compromise the physiology of the skin (Onorato et al., 2020). Space radiations cause damage to the DNA, through direct interaction or production of free radicals (Moreno-Villanueva et al., 2017; Afshinnekoo et al., 2020). Non-ionizing UV radiations also damage the skin as well, but this is not an issue in Space due to extensive protection provided to the astronauts (Tyrrell, 1995; Gasperini et al., 2017; Lipsky and German, 2019). UV exposure is under strict control inside the International Space Station (ISS) also in case of use of UV lamps.
Physical factors are not the only factors that affect the physiology of skin. The microbial populations settled on skin, space modules, in space suits, are also playing a pivotal role. The skin hosts an immense number of microorganisms adapted to utilize the nutrients available. The skin microbiota of healthy adults remains stable over time, despite environmental perturbations—at least on earth (Byrd et al., 2018). It is well known that skin diseases and disorders, are associated with an altered microbial state (Lunjani et al., 2019). Space missions occur in non-sterile, extreme confined environments, where air pressure, temperature, humidity, limited water supply are kept under strict control (Gentry and Cover, 2015). In this environment, astronauts are not able to take proper shower keeping their body clean by using wet tissues, using rinseless shampoo, and they do not change their clothes often (Farkas and Farkas, 2021). It is therefore important to understand the astronauts’ skin microbiomes and their fluctuation over time.
The skin microbiota has been studied by using both cultivable microbes and metagenomic profiles. The application of targeted and untargeted Next Generation Sequencing (NGS) has been used to further differentiate strains and functional variability (Tomida et al., 2013), e.g., the association of microorganisms with sweet and sebaceous glands, hair follicle, sebum and the stratum corneum (Byrd et al., 2018). Bacteria may variate according with the site of the body, in contrast fungal community composition was in general similar across core body sites regardless the physiology (Findley et al., 2013; Byrd et al., 2018).
The ISS is not a sterile environment and its microbial community is routinely monitored on various surfaces, as part of standard operations and maintenance requirement procedures (NASA, 2005). Influx of new microbes from travels at the ISS may quickly resemble astronaut skin microbiomes: it is transient (following crew exchanges), and some can settle permanently (Voorhies et al., 2019), showing that there is shift of the microbial communities, but also a small proportion of ubiquitous bacteria are long-term residents (in dust, Actinobacteria, Proteobacteria, and Firmicutes) (Checinska et al., 2015). A survey of cultivable bacterial and fungal populations from surface wipes over 14 months from the ISS surfaces, showed a range of 104–109 CFU/m2 changing over time but remained similar between locations. With reference to the phyla, the bacteria Actinobacteria, Firmicutes, and Proteobacteria were the most represented, while Ascomycota and Basidiomycota phyla represented the fungal domine. The dominant organisms are associated with the human microbiome and may include opportunistic pathogens. Methylobacteriaceae were also dominant across the ISS (as well as in some hospitals) (Checinska Sielaff et al., 2019), and Staphylococcaceae and Enterobacteriaceae were the most predominant organisms on the US module, very similar to fitness centres and, again, hospitals (Mukherjee et al., 2014; Lax et al., 2017). Interestingly, 46% of viable bacteria and 40% of viable fungi from the overall meta-taxonomical 16S were culturable, reflecting that a high percentage of possible opportunistic pathogens are present and alive, suggesting that ISS is like other built environments (Checinska Sielaff et al., 2019). Recent findings suggest that possible thinning of the upper layer of the epidermis and a significant loss of elasticity of the skin (Tronnier et al., 2008) could increase the exposure of the microbial communities that reside in the deeper layers of the skin, including the stratum corneum (Zeeuwen et al., 2012; Lipsky et al., 2020). In this conditions the skin of astronauts is continuously exposed to different microbial communities with relatively low biodiversity (Checinska Sielaff et al., 2019; Voorhies et al., 2019).
A crosstalk in Space: application of the knowledge on skin microbiota during wound healing.
The skin microbiome is protective against pathogens, nevertheless in certain conditions the microorganisms that are ordinarily beneficial to their host can become pathogenic (dysbiosis) (Byrd et al., 2018). Skin microorganisms are important in educating the innate and adaptive arms of the cutaneous immune system (Byrd et al., 2018), and the commensal skin microbiome during healing is essential for the regulation of the cutaneous immune system (Tomic-Canic et al., 2020). Skin microorganisms can provide protection from pathogens through modulation of antimicrobials (Tomic-Canic et al., 2020). It is urgent to better understand the crosstalk of the skin microbiota and the immune system in Space, as it is difficult to disentangle the components of microgravity, space radiations, stress, and the effect of the microbiota itself (Siddiqui et al., 2021). Exposure to microgravity during Space flights produces immunosuppression, and this can lead to dysbiosis or ill-states (Spatz et al., 2021).
The Skin Microbiota has Natural Antimicrobial Properties
The role of microorganisms in chronic wounds provides important insight on how to handle would healing in Space (Figure 1). The role of the microbiome in chronic wound is not fully understood and the importance of topical antimicrobial agents in their treatment is continuously debated (Tomic-Canic et al., 2020). Pathogens or pathobionts are suspected to delay the healing process (Eming et al., 2014; Misic et al., 2014). Alterations in the skin microbiome might contribute to the high frequency of skin rashes/hypersensitivity episodes experienced by astronauts in Space (Voorhies et al., 2019). Microorganisms can act competitively to exclude one another or synergistically for mutual benefits depending by the interaction between the skin surface and other microorganisms that live on it (Cleary et al., 2018).
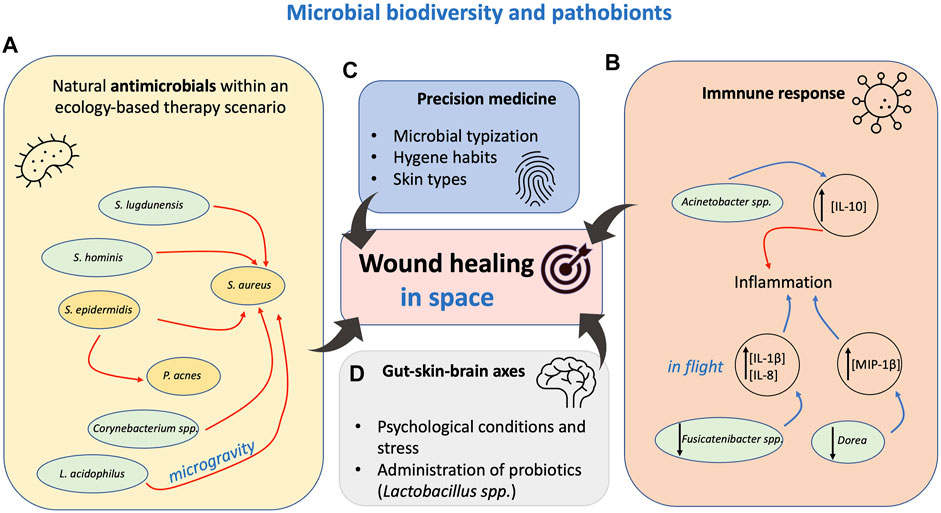
FIGURE 1. Perspectives for the application of human skin microbiota for wound healing in Space. Four possible domines for intervention developed from studies aimed to improve medical applications on Earth. These solutions can also find application in Space. (A) The ecological network among microorganisms is also regulated by natural antimicrobial. Few examples are shown as reported in the main narrative. S, Staphylococcus spp. L, Lactobacillus. P, Propionibacterium. Red arrows represent inhibition. Gold boxes are common pathobionts. Green boxes are other organisms. (B) Crosstalk of the skin microbiota with the immune system during wound healing. Microorganisms can support cytokine interleukin regulation. Up and down black arrows mean activation and reduction, respectively. Blue arrow represents IL expression. (C,D) The typization of the skin microbiota can lead to a better treatment of wound and prevention of complications. Gut-skin, gut-brain axes can be crucial for healing: in mice the administration of probiotics has shown better regulation of healing. Please refer to the text for all the details.
Microgravity can affect the biology of microorganisms. Under simulated microgravity, the liquid phase of a culture of Lactobacillus acidophilus exhibited higher antibacterial activity against Staphylococcus aureus in a time-dependent manner (Shao et al., 2017). Targeting S. aureus and Staphylococcus epidermidis in wounds is important. S. epidermidis can either help or hurt the skin barrier being strain-dependent in disease (Brown and Horswill, 2020). Staphylococcus lugdunensis inhibited S. aureus growth through the production of the antibiotic lugdunin, a thiazolidine-containing cyclic peptide (Zipperer et al., 2016). In another study, S. epidermidis strains were capable of inhibiting Propionibacterium acnes grown in-vitro (Christensen et al., 2016). P. acnes is involved in developing cutaneous inflammation. The peptidoglycan of P. acnes can activate monocytes to produce cytokines such as IL-1β, IL-8, and TNF-α, which cause granulomatous responses in inflammatory skin disorder (Kistowska et al., 2014; Lee et al., 2019).
In a different study, multiple coagulase-negative Staphylococcus spp, S. epidermidis and Staphylococcus hominis were shown to produce lantibiotics that were able to synergize with the human antimicrobial peptide cathelicidin and to inhibit the growth of S. aureus (Nakatsuji et al., 2017; Byrd et al., 2018). Strains producing lantibiotics were depleted in individuals with atopic dermatitis, who are frequently colonized with S. aureus (Byrd et al., 2018). Other studies have shown that S. aureus shifts toward commensalism in response to Corynebacterium species, affecting S. aureus behaviour and fitness, leading to a reduced virulence of S. aureus (Ramsey et al., 2016). In vitro mono-vs. co-culture of commensal Corynebacterium striatum increased transcription of genes related to human nasal colonization and decreased transcription of virulence genes of S. aureus (Ramsey et al., 2016). Biotechnological application of Corynebacterium’s metabolic products could lead to develop anti-virulence therapies against S. aureus (Ramsey et al., 2016).
It must be mentioned that the direct influences of changes of the microbial community should be carefully considered, since changes in abundance may not be solely beneficial but leading to opposite results, For example, Propionibacterium species induced S. aureus aggregation and biofilm formation in a manner dependent on dose, growth phase and pH (Wollenberg et al., 2014).
The discovery, characterization and production of the antimicrobials or elicitors involved in quorum sensing or signalling could find important biotechnological applications to control pathobionts. The interactions among microorganisms in wounds are common but poorly characterized.
Bacterial community instability was associated with faster healing and more positive clinical outcomes (Loesche et al., 2017). One hundred subjects diagnosed with diabetic foot ulcers were enrolled into a prospective, longitudinal cohort study to analyse the temporal dynamics of the bacterial community of the ulcers. In this study the bacterial community stability reflected a delayed healing phenotype (Loesche et al., 2017). This observation may apparently be counterintuitive, as in many other pathologies bacterial community instability was associated with disease (Martinez et al., 2008). Nevertheless, as proposed by Loesche and collaborators “instability in the microbiome is a reflection of effective control of wound bacteria, which prevents any community structure from stabilizing” (Loesche et al., 2017). Stabilization could proceed on the trajectory of the progressive ulceration. Temporal stability of the microbiota of wound should be further studied in Space.
In another similar study, the mycobiome (the fungal microbial community) in chronic wounds is predictive of healing time, associated with poor outcomes when forming mixed fungal-bacterial biofilms (Kalan et al., 2016). Cladosporium herbarum and Candida albicans have been identified as the most abundant species in chronic wounds (Kalan et al., 2016). More information must be acquired from the microbiome of chronic wounds, which is believed to play an important role in impaired healing and the development of infection-related complications (Loesche et al., 2017). It is not known to what extent the mycobiome composition in Space provides little predictive value of wound outcomes, and culture-independent studies have been limited by cross-sectional design and small cohort size. An interesting approach adopted by Loesche and collaborators was to define the outcome of the diabetic foot ulcers by classifying the microbiota in different community types (Loesche et al., 2017). In this disease the transition patterns and frequencies of microbial populations were associated with healing time, and this can be extended to the prognosis. Similar experimental approaches could be done in microgravity scenarios.
Crosstalk of the Skin Microbiota With the Immune System During wound Healing
The immune system is interconnected with the skin microbiota, especially by targeting pathogen-associated molecular patterns (PAMPs), through pattern recognition receptors (PRRs). For example, Propionibacterium acnes and the lipopolysaccharides induce the expression of antimicrobial peptides and proinflammatory cytokines/chemokines in human sebocytes contributing to the host defence and skin inflammation (Nagy et al., 2006; Naik et al., 2012). Microorganisms could be beneficial if able to regulate the immune response toward a normal healing process. For example, the lack of interleukin-10 (IL-10) (a key anti-inflammatory cytokine in immunologic tolerance) results in a strong inflammatory response. IL-10 is a key mediator of the pro-to anti-inflammatory transition that counters collagen deposition (Singampalli et al., 2020). In healthy individuals, IL-10 expression was positively correlated with the abundance of the gammaproteobacterial genus Acinetobacter on the skin (Hanski et al., 2012) (Figure 1). Abundance of Acinetobacter could be monitored in astronauts, and further biotechnological elicitors released by Acinetobacter could be used as food additive to support wound healing in microgravity, as well as on earth.
It is well known that environmental biodiversity, human microbiota, and allergy are correlated (Hanski et al., 2012). Pro-inflammatory cytokines MCP-1, IL-8, IL-1b and MIP-1β showed a significant increase in their concentration during flight (flight day, FD 180), while TNFa had a near significant rise. Conversely, a decrease in OTU of Fusicatenibacter spp. and Dorea spp. was measured when IL-8, IL-1 β and MIP-1β increased during flight (Figure 1). Cytokine concentrations reverted to pre-flight levels within 2 months of returning to earth (Voorhies et al., 2019). It would be interesting to further understand the association of the astronauts’ immune dysregulation with the skin microbiome. The main picture is still far to be resolved. The analysis of skin microbiota forehead and forearm (Voorhies et al., 2019) (Voorhies et al., 2019) (Voorhies et al., 2019) (Voorhies et al., 2019) during inflight showed a significant reduction of Proteobacteria, mainly, Gamma and Betaproteobacteria, with an associated increase in Firmicutes, including Staphylococcal, and Streptococcal species (Voorhies et al., 2019) (Figure 1). A better regulation of Proteobacteria on skin could also provide further details on how the dynamics of the microbiota can regulate pro-inflammatory cytokines and wound healing in Space. Voorhies and co-authors proposed that it is possible that the constant filtration of environmental air in the ISS contributes to the overall reduction of skin Proteobacteria (Voorhies et al., 2019). Recent studies conducted in children showed the importance of “green” areas around the homes. Children living nearby green areas showed reduced atopic sensitization, the same absence of “green” resources (possibly “green” areas) could reduce Proteobacteria on the skin to a pathological level (Ruokolainen et al., 2015). Absence of “green” resources (or better, reservoir of similar microbial communities) could control the incidence of skin clinical symptoms during long-duration orbital spaceflight (Crucian et al., 2016; Voorhies et al., 2019). It is reasonable to assume that skin clinical symptoms and changes in skin structure may facilitate the establishment of skin infections, inflammation, leading to reduced wound healing during spaceflight. Further research needs to be addressed.
Precision Medicine, Personal Skin Features in Wound Healing
The microbial typing for each individual is ideal for precision medicine approaches, leading treatment of wounds (Hülpüsch et al., 2021) (Figure 1). This important field of research needs to be further explored in Space: it would be important to understand to what extent the skin microbiota changes in astronauts according with each personal skin moisture, pH, personal hygiene habits. It is not clear how these factors affect wound healing and it would need further investigations (Voorhies et al., 2019). In this context it would be interesting to stimulate ad hoc skin probiotic communities in an ecology-based therapy scenario, by limiting dysbiosis that leads to cutaneous disorders (Zhou et al., 2020).
Gut-Skin, Gut-Brain Axes can be Crucial for Wound Healing in Space
The crosstalk between the immune system and the skin microbiota can lead to normal healing (Byrd et al., 2018). In this context the gut-brain/skin axis can play a role in wound healing (Figure 1). The gut microbiome influences many domains of the human body: e.g., the central nervous system (Ma et al., 2019), endocrine control (Régnier et al., 2021), immune fitness (Balikji et al., 2021). New biotechnological approaches should identify microbial elicitors that could be used to control inflammatory cytokines and be administered as probiotics or as additive in food. Probiotics actively can crosstalk between the immune system and the skin microbiota. From the biotechnological potential a few examples have been proposed: in mice, the administration of Lactobacillus reuteri enhanced wound-healing properties through the up-regulation of the neuropeptide hormone oxytocin (Poutahidis et al., 2013). In mice, diet control has been proved to alter the formation of chronic wounds, as a diet with kefir products or the administration of Lactobacillus johnsonii showed to support some benefits (Guéniche et al., 2006; Huseini et al., 2012). In another murine model, preparations of orally administered Lactobacillus acidophilus, showed enhanced wound contraction and fast epithelization when compared with the not treated. The treatment with L. acidophilus also increased breaking strength in sutured incision wound, increased granuloma dry weight and marked increase in collagen content indicating wound healing (Gudadappanavar et al., 2017). The use of Lactobacillus spp. in food biotechnology is well known, it has essentially negligible biological risk, and it is known for balancing gut microbial population (Bernardeau et al., 2006; Widyastuti et al., 2021).
It is reasonable to assume that the gut-skin and gut-brain axes biochemical signalling could also affect wound healing in Space, having a profound influence on astronaut health (Siddiqui et al., 2021). Further studies must be completed. For example, depression and chronic wounds were demonstrated to share common pathologic features, which included dysregulated inflammation and altered microbiome (Hadian et al., 2020). For this reason, it should not be excluded that isolation and stress associated with the fluctuation of the microbiome can originate delay in healing. Recently, in a questionary survey about the irritable bowel syndrome symptoms, the perceived immune fitness (subjective judgment), and impaired wound healing were studied in a cohort of 1942 Dutch students (Balikji et al., 2021). The assessment showed that impaired wound healing (self-reported) was significantly associated with irritable bowel syndrome, showing that when both conditions occurred, complaints were significantly more severe (Balikji et al., 2021).
Conclusion
The last frontiers on the association of the skin microbiome and wound healing during short and long mission in Space show that further understanding is needed. The perspectives for further research range from studies on microbial dynamics, natural antimicrobial properties, understating how personal skin features affect healing, the crosstalk of the microbiome with the immune system, to the skin-gut-brain axes.
Further biotechnological applications will help to understand better the skin microbiome and the healing process: for example, the three-dimensional bioprinting of skin utilizing different cell types (fibroblasts and keratinocytes) as bio-inks for tissue engineering (Jemison and Olabisi, 2021). This technology can help in studying the healing process associated with specific probiotic microbial communities (mediated by elicitors), or the deposition of different cell types along with specific biomaterials (Cubo-Mateo and Gelinsky, 2021). This is particularly important for travels to Mars, where also surgery can be a possible scenario (Kirkpatrick et al., 1997; Farahani and DiPietro, 2008; Cubo-Mateo and Gelinsky, 2021).
In this context, human skin-associated microorganisms provide a pivotal role to the skin-microbe ecosystems that still need to be fully understood. A stronger understanding of the connections and metabolic network among bacteria, fungi, the host’s immune system and the host metabolism will support the basis for a better wound healing in Space. Improving our knowledge in this field of the biomedicine not only can be important in the perspective of ensuring adequate medical assistance in future space exploration missions beyond LEO, but it can have important repercussions on earth. In fact, chronic ulcers, which are continually at risk of infection, are a huge health problem, with high social costs and poor quality of patients’s life.
Data Availability Statement
The original contributions presented in the study are included in the article/Supplementary Material, further inquiries can be directed to the corresponding author.
Author Contributions
MMar and DC prepared the manuscript. DP and MMon revised the manuscript.
Funding
WHISPER—“Wound Healing In Space: problems and Perspectives for tissue Regeneration and engineering,” Sci Space Microgravity Application Promotion Program, ESA Contract Number 4000130928/20/NL/PG/pt.
Conflict of Interest
The authors declare that the research was conducted in the absence of any commercial or financial relationships that could be construed as a potential conflict of interest.
Publisher’s Note
All claims expressed in this article are solely those of the authors and do not necessarily represent those of their affiliated organizations, or those of the publisher, the editors and the reviewers. Any product that may be evaluated in this article, or claim that may be made by its manufacturer, is not guaranteed or endorsed by the publisher.
Acknowledgments
This perspective was written within the activities of ESA Topical Team on “Tissue Healing in Space: Techniques for Promoting and Monitoring Tissue Repair and Regeneration”.
References
Afshinnekoo, E., Scott, R. T., MacKay, M. J., Pariset, E., Cekanaviciute, E., Barker, R., et al. (2020). Fundamental Biological Features of Spaceflight: Advancing the Field to Enable Deep-Space Exploration. Cell 183, 1162–1184. doi:10.1016/j.cell.2020.10.050
Balikji, J., Hoogbergen, M. M., Garssen, J., and Verster, J. C. (2021). The Association of Irritable Bowel Complaints and Perceived Immune Fitness Among Individuals that Report Impaired Wound Healing: Supportive Evidence for the Gut-Brain-Skin axis. Gastroenterol. Insights 12, 423–432. doi:10.3390/gastroent12040040
Bernardeau, M., Guguen, M., and Vernoux, J. P. (2006). Beneficial Lactobacilli in Food and Feed: Long-Term Use, Biodiversity and Proposals for Specific and Realistic Safety Assessments. FEMS Microbiol. Rev. 30, 487–513. doi:10.1111/j.1574-6976.2006.00020.x
Blaber, E., Marçal, H., and Burns, B. P. (2010). Bioastronautics: The Influence of Microgravity on Astronaut Health. Astrobiology 10, 463–473. doi:10.1089/ast.2009.0415
Brown, M. M., and Horswill, A. R. (2020). Staphylococcus Epidermidis-Skin Friend or Foe? PLOS Pathog. 16, e1009026. doi:10.1371/journal.ppat.1009026
Byrd, A. L., Belkaid, Y., and Segre, J. A. (2018). The Human Skin Microbiome. Nat. Rev. Microbiol. 16, 143–155. doi:10.1038/nrmicro.2017.157
Checinska, A., Probst, A. J., Vaishampayan, P., White, J. R., Kumar, D., Stepanov, V. G., et al. (2015). Microbiomes of the Dust Particles Collected from the International Space Station and Spacecraft Assembly Facilities. Microbiome 3, 50. doi:10.1186/s40168-015-0116-3
Checinska Sielaff, A., Urbaniak, C., Mohan, G. B. M., Stepanov, V. G., Tran, Q., Wood, J. M., et al. (2019). Characterization of the Total and Viable Bacterial and Fungal Communities Associated with the International Space Station Surfaces. Microbiome 7, 50. doi:10.1186/s40168-019-0666-x
Christensen, G. J. M., Scholz, C. F. P., Enghild, J., Rohde, H., Kilian, M., Thürmer, A., et al. (2016). Antagonism between Staphylococcus Epidermidis and Propionibacterium Acnes and its Genomic Basis. BMC Genomics 17, 152. doi:10.1186/s12864-016-2489-5
Cialdai, F., and Monici, M. (2013). Wound Healing: What Happens in Microgravity? Cbiot 2, 250–256. doi:10.2174/22115501113026660025
Cleary, J. M., Lipsky, Z. W., Kim, M., Marques, C. N. H., and German, G. K. (2018). Heterogeneous Ceramide Distributions Alter Spatially Resolved Growth of Staphylococcus aureus on Human Stratum Corneum. J. R. Soc. Interf. 15, 20170848. doi:10.1098/rsif.2017.0848
Crucian, B., Babiak-Vazquez, A., Johnston, S., Pierson, D., Ott, C. M., and Sams, C. (2016). Incidence of Clinical Symptoms during Long-Duration Orbital Spaceflight. Ijgm 9, 383–391. doi:10.2147/IJGM.S114188
Cubo-Mateo, N., and Gelinsky, M. (2021). Wound and Skin Healing in Space: The 3D Bioprinting Perspective. Front. Bioeng. Biotechnol. 15, e00051. doi:10.3389/fbioe.2021.720217
Dam, D. H. M., and Paller, A. S. (2018). Gangliosides in Diabetic Wound Healing. Prog. Mol. Biol. Translational Sci. 156, 229–239. doi:10.1016/bs.pmbts.2017.12.006
Eming, S. A., Martin, P., and Tomic-Canic, M. (2014). Wound Repair and Regeneration: Mechanisms, Signaling, and Translation. Sci. Transl. Med. 6, 265sr6. doi:10.1126/scitranslmed.3009337
Farahani, R. M., and DiPietro, L. A. (2008). Microgravity and the Implications for Wound Healing. Int. Wound J. 5, 552–561. doi:10.1111/j.1742-481X.2008.00438.x
Farkas, Á., and Farkas, G. (2021). Effects of Spaceflight on Human Skin. Skin Pharmacol. Physiol. 34, 239–245. doi:10.1159/000515963
Findley, K., Oh, J., Oh, J., Yang, J., Conlan, S., Deming, C., et al. (2013). Topographic Diversity of Fungal and Bacterial Communities in Human Skin. Nature 498, 367–370. doi:10.1038/nature12171
Gasperini, A. E., Sanchez, S., Doiron, A. L., Lyles, M., and German, G. K. (2017). Non-ionising UV Light Increases the Optical Density of Hygroscopic Self Assembled DNA crystal Films. Sci. Rep. 7, 6631. doi:10.1038/s41598-017-06884-8
Gentry, G. J., and Cover, J. (2015). International Space Station (ISS) Environmental Control and Life Support (ECLS) System Overview of Events: 2010 - 2014. 45th Int. Conf. Environ. Syst.
Gontcharov, I. B., Kovachevich, I. V., Pool, S. L., Navinkov, O. L., Barratt, M. R., Bogomolov, V. V., et al. (2005). In-flight Medical Incidents in the NASA-Mir Program. Aviat. Space Environ. Med. 76, 692–696. Available at: http://www.ncbi.nlm.nih.gov/pubmed/16018356.
Gudadappanavar, A., Hombal, P., Timashetti, S., and Javali, S. (2017). Influence of Lactobacillus Acidophilus and Lactobacillus Plantarum on Wound Healing in Male Wistar Rats - an Experimental Study. Int. J. App Basic Med. Res. 7, 233. doi:10.4103/ijabmr.IJABMR_329_16
Guéniche, A., Benyacoub, J., Buetler, T. M., Smola, H., and Blum, S. (2006). Supplementation with Oral Probiotic Bacteria Maintains Cutaneous Immune Homeostasis after UV Exposure. Eur. J. Dermatol 16(5), 511–517. doi:10.1684/ejd.2006.0023
Hadian, Y., Fregoso, D., Nguyen, C., Bagood, M. D., Dahle, S. E., Gareau, M. G., et al. (2020). Microbiome‐skin‐brain axis: A Novel Paradigm for Cutaneous Wounds. Wound Rep. Reg. 28, 282–292. doi:10.1111/wrr.12800
Hanski, I., von Hertzen, L., Fyhrquist, N., Koskinen, K., Torppa, K., Laatikainen, T., et al. (2012). Environmental Biodiversity, Human Microbiota, and Allergy Are Interrelated. Proc. Natl. Acad. Sci. U.S.A. 109, 8334–8339. doi:10.1073/pnas.1205624109
Hülpüsch, C., Weins, A. B., Traidl‐Hoffmann, C., and Reiger, M. (2021). A new era of Atopic Eczema Research: Advances and Highlights. Allergy 76, 3408–3421. doi:10.1111/all.15058
Huseini, H. F., Rahimzadeh, G., Fazeli, M. R., Mehrazma, M., and Salehi, M. (2012). Evaluation of Wound Healing Activities of Kefir Products. Burns 38, 719–723. doi:10.1016/j.burns.2011.12.005
Jemison, M., and Olabisi, R. (2021). Biomaterials for Human Space Exploration: A Review of Their Untapped Potential. Acta Biomater. 128, 77–99. doi:10.1016/j.actbio.2021.04.033
Kalan, L., Loesche, M., Hodkinson, B. P., Heilmann, K., Ruthel, G., Gardner, S. E., et al. (2016). Redefining the Chronic-Wound Microbiome: Fungal Communities Are Prevalent, Dynamic, and Associated with Delayed Healing. MBio 6;7(5), e01058-16. doi:10.1128/mBio.01058-16
Kirkpatrick, A. W., Campbell, M. R., Novinkov, O. L., Goncharov, I. B., and Kovachevich, I. V. (1997). Blunt Trauma and Operative Care in Microgravity: a Review of Microgravity Physiology and Surgical Investigations with Implications for Critical Care and Operative Treatment in Space. J. Am. Coll. Surg. 184, 441–453. Available at: http://www.ncbi.nlm.nih.gov/pubmed/9145063.
Kistowska, M., Gehrke, S., Jankovic, D., Kerl, K., Fettelschoss, A., Feldmeyer, L., et al. (2014). IL-1β Drives Inflammatory Responses to Propionibacterium Acnes In Vitro and In Vivo. J. Invest. Dermatol. 134, 677–685. doi:10.1038/jid.2013.438
Lax, S., Sangwan, N., Smith, D., Larsen, P., Handley, K. M., Richardson, M., et al. (2017). Bacterial Colonization and Succession in a Newly Opened Hospital. Sci. Transl. Med. 9(391), eaah6. doi:10.1126/scitranslmed.aah6500
Lee, E. H., Shin, J. H., Kim, S. S., Joo, J.-H., Choi, E., and Seo, S. R. (2019). Suppression of Propionibacterium Acnes-Induced Skin Inflammation by Laurus Nobilis Extract and its Major Constituent Eucalyptol. Ijms 20, 3510. doi:10.3390/ijms20143510
Lipsky, Z. W., and German, G. K. (2019). Ultraviolet Light Degrades the Mechanical and Structural Properties of Human Stratum Corneum. J. Mech. Behav. Biomed. Mater. 100, 103391. doi:10.1016/j.jmbbm.2019.103391
Lipsky, Z. W., Marques, C. N. H., and German, G. K. (2020). Lipid Depletion Enables Permeation of Staphylococcus aureus Bacteria through Human Stratum Corneum. Tissue Barriers 8, 1754706. doi:10.1080/21688370.2020.1754706
Loesche, M., Gardner, S. E., Kalan, L., Horwinski, J., Zheng, Q., Hodkinson, B. P., et al. (2017). Temporal Stability in Chronic Wound Microbiota Is Associated with Poor Healing. J. Invest. Dermatol. 137, 237–244. doi:10.1016/j.jid.2016.08.009
Lunjani, N., Hlela, C., and O’Mahony, L. (2019). Microbiome and Skin Biology. Curr. Opin. Allergy Clin. Immunol. 19, 328–333. doi:10.1097/ACI.0000000000000542
Ma, Q., Xing, C., Long, W., Wang, H. Y., Liu, Q., and Wang, R.-F. (2019). Impact of Microbiota on central Nervous System and Neurological Diseases: the Gut-Brain axis. J. Neuroinflammation 16, 53. doi:10.1186/s12974-019-1434-3
Maheswary, T., Nurul, A. A., and Fauzi, M. B. (2021). The Insights of Microbes' Roles in Wound Healing: A Comprehensive Review. Pharmaceutics 13, 981. doi:10.3390/pharmaceutics13070981
Martinez, C., Antolin, M., Santos, J., Torrejon, A., Casellas, F., Borruel, N., et al. (2008). Unstable Composition of the Fecal Microbiota in Ulcerative Colitis during Clinical Remission. Am. J. Gastroenterol. 103, 643–648. doi:10.1111/j.1572-0241.2007.01592.x
Misic, A. M., Gardner, S. E., and Grice, E. A. (2014). The Wound Microbiome: Modern Approaches to Examining the Role of Microorganisms in Impaired Chronic Wound Healing. Adv. Wound Care 3, 502–510. doi:10.1089/wound.2012.0397
Moreno-Villanueva, M., Wong, M., Lu, T., Zhang, Y., and Wu, H. (2017). Interplay of Space Radiation and Microgravity in DNA Damage and DNA Damage Response. npj Microgravity 3, 14. doi:10.1038/s41526-017-0019-7
Mukherjee, N., Dowd, S., Wise, A., Kedia, S., Vohra, V., and Banerjee, P. (2014). Diversity of Bacterial Communities of Fitness center Surfaces in a U.S. Metropolitan Area. Ijerph 11, 12544–12561. doi:10.3390/ijerph111212544
Nagy, I., Pivarcsi, A., Kis, K., Koreck, A., Bodai, L., McDowell, A., et al. (2006). Propionibacterium Acnes and Lipopolysaccharide Induce the Expression of Antimicrobial Peptides and Proinflammatory Cytokines/chemokines in Human Sebocytes. Microbes Infect. 8, 2195–2205. doi:10.1016/j.micinf.2006.04.001
Naik, S., Bouladoux, N., Wilhelm, C., Molloy, M. J., Salcedo, R., Kastenmuller, W., et al. (2012). Compartmentalized Control of Skin Immunity by Resident Commensals. Science 337, 1115–1119. doi:10.1126/science.1225152
Nakatsuji, T., Chen, T. H., Narala, S., Chun, K. A., Two, A. M., Yun, T., et al. (2017). Antimicrobials from Human Skin Commensal Bacteria Protect against Staphylococcus aureus and Are Deficient in Atopic Dermatitis. Sci. Transl. Med. 9. doi:10.1126/scitranslmed.aah4680
Onorato, G., Di Schiavi, E., and Di Cunto, F. (2020). Understanding the Effects of Deep Space Radiation on Nervous System: The Role of Genetically Tractable Experimental Models. Front. Phys. 8, 362. doi:10.3389/fphy.2020.00362
Poutahidis, T., Kearney, S. M., Levkovich, T., Qi, P., Varian, B. J., Lakritz, J. R., et al. (2013). Microbial Symbionts Accelerate Wound Healing via the Neuropeptide Hormone Oxytocin. PLoS One 8, e78898. doi:10.1371/journal.pone.0078898
Ramsey, M. M., Freire, M. O., Gabrilska, R. A., Rumbaugh, K. P., and Lemon, K. P. (2016). Staphylococcus aureus Shifts toward Commensalism in Response to Corynebacterium Species. Front. Microbiol. 7, 1230. doi:10.3389/fmicb.2016.01230
Régnier, M., Van Hul, M., Knauf, C., and Cani, P. D. (2021). Gut Microbiome, Endocrine Control of Gut Barrier Function and Metabolic Diseases. J. Endocrinol. 248, R67–R82. doi:10.1530/JOE-20-0473
Reinke, J. M., and Sorg, H. (2012). Wound Repair and Regeneration. Eur. Surg. Res. 49, 35–43. doi:10.1159/000339613
Ruokolainen, L., Hertzen, L., Fyhrquist, N., Laatikainen, T., Lehtomäki, J., Auvinen, P., et al. (2015). Green Areas Around Homes Reduce Atopic Sensitization in Children. Allergy 70, 195–202. doi:10.1111/all.12545
Shao, D., Yao, L., Riaz, M. s., Zhu, J., Shi, J., Jin, M., et al. (2017). Simulated Microgravity Affects Some Biological Characteristics of Lactobacillus Acidophilus. Appl. Microbiol. Biotechnol. 101, 3439–3449. doi:10.1007/s00253-016-8059-6
Siddiqui, R., Qaisar, R., Goswami, N., Khan, N. A., and Elmoselhi, A. (2021). Effect of Microgravity Environment on Gut Microbiome and Angiogenesis. Life 11, 1008. doi:10.3390/life11101008
Singampalli, K. L., Balaji, S., Wang, X., Parikh, U. M., Kaul, A., Gilley, J., et al. (2020). The Role of an IL-10/hyaluronan axis in Dermal Wound Healing. Front. Cel Dev. Biol. 8, 636. doi:10.3389/fcell.2020.00636
Singh, S., Young, A., and McNaught, C.-E. (2017). The Physiology of Wound Healing. Surgery (Oxford) 35, 473–477. doi:10.1016/j.mpsur.2017.06.004
Spatz, J. M., Fulford, M. H., Tsai, A., Gaudilliere, D., Hedou, J., Ganio, E., et al. (2021). Human Immune System Adaptations to Simulated Microgravity Revealed by Single-Cell Mass Cytometry. Sci. Rep. 11, 11872. doi:10.1038/s41598-021-90458-2
Tomic-Canic, M., Burgess, J. L., O’Neill, K. E., Strbo, N., and Pastar, I. (2020). Skin Microbiota and its Interplay with Wound Healing. Am. J. Clin. Dermatol. 21, 36–43. doi:10.1007/s40257-020-00536-w
Tomida, S., Nguyen, L., Chiu, B.-H., Liu, J., Sodergren, E., Weinstock, G. M., et al. (2013). Pan-genome and Comparative Genome Analyses of Propionibacterium Acnes Reveal its Genomic Diversity in the Healthy and Diseased Human Skin Microbiome. MBio 4(3), e00003-13. doi:10.1128/mBio.00003-13
Tronnier, H., Wiebusch, M., and Heinrich, U. (2008). Change in Skin Physiological Parameters in Space – Report on and Results of the First Study on Man. Skin Pharmacol. Physiol. 21, 283–292. doi:10.1159/000148045
Tyrrell, R. M. (1995). Ultraviolet Radiation and Free Radical Damage to Skin. Biochem. Soc. Symp. 61, 47–53. doi:10.1042/bss0610047
Voorhies, A. A., Mark Ott, C., Mehta, S., Pierson, D. L., Crucian, B. E., Feiveson, A., et al. (2019). Study of the Impact of Long-Duration Space Missions at the International Space Station on the Astronaut Microbiome. Sci. Rep. 9, 9911. doi:10.1038/s41598-019-46303-8
Widyastuti, Y., Febrisiantosa, A., and Tidona, F. (2021). Health-Promoting Properties of Lactobacilli in Fermented Dairy Products. Front. Microbiol. 12, 673890. doi:10.3389/fmicb.2021.673890
Wollenberg, M. S., Claesen, J., Escapa, I. F., Aldridge, K. L., Fischbach, M. A., and Lemon, K. P. (2014). Propionibacterium -produced Coproporphyrin III Induces Staphylococcus aureus Aggregation and Biofilm Formation. MBio 5(4), e01286-14. doi:10.1128/mBio.01286-14
Zeeuwen, P. L., Boekhorst, J., van den Bogaard, E. H., de Koning, H. D., van de Kerkhof, P. M., Saulnier, D. M., et al. (2012). Microbiome Dynamics of Human Epidermis Following Skin Barrier Disruption. Genome Biol. 13, R101. doi:10.1186/gb-2012-13-11-r101
Zhou, H., Shi, L., Ren, Y., Tan, X., Liu, W., and Liu, Z. (2020). Applications of Human Skin Microbiota in the Cutaneous Disorders for Ecology-Based Therapy. Front. Cel. Infect. Microbiol. 10, 570261. doi:10.3389/fcimb.2020.570261
Keywords: microbiome and dysbiosis, wound healing, space mission, skin, wound
Citation: Marvasi M, Monici M, Pantalone D and Cavalieri D (2022) Exploitation of Skin Microbiota in Wound Healing: Perspectives During Space Missions. Front. Bioeng. Biotechnol. 10:873384. doi: 10.3389/fbioe.2022.873384
Received: 10 February 2022; Accepted: 11 April 2022;
Published: 29 April 2022.
Edited by:
Mona Kamal Marei, Alexandria University, EgyptReviewed by:
Guy K. German, Binghamton University, United StatesMaria A. Mariggiò, University of Studies G. d'Annunzio Chieti and Pescara, Italy
Copyright © 2022 Marvasi, Monici, Pantalone and Cavalieri. This is an open-access article distributed under the terms of the Creative Commons Attribution License (CC BY). The use, distribution or reproduction in other forums is permitted, provided the original author(s) and the copyright owner(s) are credited and that the original publication in this journal is cited, in accordance with accepted academic practice. No use, distribution or reproduction is permitted which does not comply with these terms.
*Correspondence: Duccio Cavalieri, ZHVjY2lvLmNhdmFsaWVyaUB1bmlmaS5pdCYjeDAyMDBhOw==