- 1School of Engineering and Materials Science, Queen Mary University of London, London, United Kingdom
- 2UCD School of Medicine, UCD Conway Institute of Biomolecular and Biomedical Research, University College Dublin, Dublin, Ireland
- 3Trinity Centre for Biomedical Engineering, Trinity College Dublin, Dublin, Ireland
- 4Barts and the London School of Medicine and Dentistry, Queen Mary University of London, London, United Kingdom
- 5Department of Diabetes, School of Life Course Sciences, King’s College London, London, United Kingdom
This study examined the role of sirtuins in the regenerative potential of articular chondrocytes. Sirtuins (SIRT1-7) play a key role in regulating cartilage homeostasis. By inhibiting pro-inflammatory pathways responsible for cartilage degradation and promoting the expression of key matrix components, sirtuins have the potential to drive a favourable balance between anabolic and catabolic processes critical to regenerative medicine. When subjected to osmolarity and glucose concentrations representative of the in vivo niche, freshly isolated bovine chondrocytes exhibited increases in SIRT1 but not SIRT3 gene expression. Replicating methods adopted for the in vitro monolayer expansion of chondrocytes for cartilage regenerative therapies, we found that SIRT1 gene expression declined during expansion. Manipulation of sirtuin activity during in vitro expansion by supplementation with the SIRT1-specific activator SRT1720, nicotinamide mononucleotide, or the pan-sirtuin inhibitor nicotinamide, significantly influenced cartilage regeneration in subsequent 3D culture. Tissue mass, cellularity and extracellular matrix content were reduced in response to sirtuin inhibition during expansion, whilst sirtuin activation enhanced these measures of cartilage tissue regeneration. Modulation of sirtuin activity during monolayer expansion influenced H3K27me3, a heterochromatin mark with an important role in development and differentiation. Unexpectedly, treatment of primary chondrocytes with sirtuin activators in 3D culture reduced their matrix synthesis. Thus, modulating sirtuin activity during the in vitro monolayer expansion phase may represent a distinct opportunity to enhance the outcome of cartilage regenerative medicine techniques.
1 Introduction
It is estimated that around 10,000 people in the United Kingdom each year experience cartilage damage that warrants treatment (NICE, 2017). If untreated, such cartilage injuries impair quality of life and increase the risk of osteoarthritis (OA). This has motivated the development of cell-based cartilage repair techniques such as autologous chondrocyte implantation (ACI) and evolving tissue engineering/regenerative medicine approaches (Brittberg et al., 1994; Huang et al., 2016). In many cell-based repair techniques chondrocytes are isolated from a cartilage biopsy and increased in number by maintaining the cells in monolayer culture, such that a much larger cell population can then be re-implanted into the defect to repair the cartilage. However, the capacity of cells to regenerate cartilage upon re-implantation progressively declines during in vitro culture due to the process of de-differentiation, with associated loss of expression of cartilage extracellular matrix (ECM) macromolecules such as type II collagen and aggrecan (Dell’Accio et al., 2001; Giovannini et al., 2010; Niemeyer et al., 2012). The development of methods that retain chondrocyte regenerative potential during monolayer culture would provide a major advance for cartilage repair techniques, potentially enhancing clinical outcomes (Saris et al., 2009; Niemeyer et al., 2012).
Sirtuins are a family of seven enzymes (SIRT1-7) which have been established to play a key role in regulating cartilage homeostasis. To date, most studies have focussed on SIRT1 and have revealed that SIRT1 protects against cartilage degradation through multiple mechanisms to delay the onset and progression of both age-associated and instability-linked OA (Matsuzaki et al., 2014). However, transcription and protein levels of SIRT1 decline during ageing and reduced SIRT1 levels correlate with increased severity of OA (Fujita et al., 2011; Li et al., 2016). Importantly, the genetic manipulation of SIRT1 expression has revealed a causal link between SIRT1 deficiency and OA disease progression in animal models (Gabay et al., 2013a; Gabay et al., 2013b). Further studies have demonstrated that SIRT3 and SIRT6 also promote cartilage health (Nagai et al., 2015; Wu et al., 2015; Fu et al., 2016), while a contrasting role was recently revealed for SIRT7 as a negative regulator of cartilage homeostasis, through suppression of the transcriptional activity of pro-chondrogenic SOX9 (Korogi et al., 2018).
The sirtuins are nicotinamide adenine dinucleotide (NAD)-dependent de-acetylases which target histones, transcription factors and enzymes to regulate cell behaviour. SIRT1 de-acetylates the pro-inflammatory nuclear factor-kappa B (NF-κB) transcription factor (Yeung et al., 2004; Lei et al., 2012), inactivating it and thereby inhibiting the pro-inflammatory pathways responsible for cartilage degradation mediated by matrix metalloproteinases (MMPs) and a disintegrin-like and metalloproteinases with thrombospondin motifs (ADAMTS) (Kiernan et al., 2003; Yeung et al., 2004; Matsushita et al., 2013; Moon et al., 2013; Elayyan et al., 2017). SIRT1 also transcriptionally suppresses the senescence marker p16 through epigenetic modification at the p16 promoter (Li and Tollefsbol, 2011) and downregulates pro-apoptotic proteins such as p53 to promote cell survival under stress (Luo et al., 2001; Vaziri et al., 2001; Takayama et al., 2009). Conversely, SIRT1-mediated de-acetylation activates the pro-chondrogenic transcription factor SOX9, promoting expression of key matrix components aggrecan and type II collagen (Dvir-Ginzberg et al., 2008; Fujita et al., 2011; Bar Oz et al., 2016). Thus, sirtuins have the potential to drive a favourable balance between anabolic and catabolic processes that is critical to regenerative medicine. However, the importance of sirtuin activity during monolayer expansion for cell-based repair techniques has not been examined previously.
In vivo, chondrocytes experience high osmolarity conditions and low glucose levels compared to other tissues. In articular cartilage the Donnan osmotic effect of negatively charged proteoglycan in the ECM increases osmolarity of the cartilage niche from ∼350 mOsm in the superficial zone to 450 mOsm in the deeper regions, and this is increased further by joint loading (Maroudas and Evans, 1972; Oswald et al., 2008). Culture media has been optimised to replicate the osmotic environment of other tissues with the result that media typically used for in vitro chondrocyte culture has a lower osmolarity than these specialised cells experience in vivo, at approximately 250–280 mOsm. Conversely, typical culture media contains 10 mM glucose which far exceeds in vivo concentrations which can be as low as 1 mM in the deep zone of articular cartilage (Windhaber et al., 2003). The transition from cartilage tissue to monolayer culture is therefore accompanied by a shift from high osmolarity and low glucose to low osmolarity and high glucose. It is well established that glucose restriction promotes sirtuin activity (Lin et al., 2002; Li and Tollefsbol, 2011), and we demonstrated previously that chondrocytes expanded in physiological glucose levels of 1 mM subsequently generated greater tissue mass in pellet culture than 10 mM glucose typical of in vitro expansion conditions (Heywood et al., 2014). However, the mechanism remains to be established and the potential role of sirtuins in this phenomenon is not known.
A number of small molecule activators of sirtuins have been reported to ameliorate the decline in sirtuin function in aging and disease. Resveratrol is known to augment sirtuin activity in cartilage and other tissues, although the exact mechanism of action has been debated and it affects many other targets. The activity of sirtuins is regulated by the availability of their common substrate, NAD (Imai and Guarente, 2014). Several NAD precursors have emerged, including nicotinamide riboside (NR) and nicotinamide mononucleotide (NMN) which have been demonstrated to successfully enhance sirtuin activity when supplemented in the diet, or in culture medium of cells in vitro (Imai, 2010). In addition, novel potent synthetic activators have been developed, such as the SIRT1-specific activator, SRT1720 (Milne et al., 2007). Therefore, this study tests the hypothesis that activation of sirtuins by supplementation with SRT1720 or NMN during chondrocyte maintenance in vitro augments subsequent cartilage regeneration potential. This study demonstrates, for the first time, that treatment with small molecule activators of sirtuin activity during the in vitro cell expansion phase may augment the regenerative potential of the expanded chondrocytes. Ultimately, this study aims to enhance the clinical success of cartilage repair procedures such as ACI.
2 Materials and methods
2.1 Ethics statement
Bovine metacarpophalangeal joints were procured as surplus to other food/agricultural use from an abattoir in the United Kingdom, operated under the relevant regulations on animal welfare. This study does not use tissue from animals maintained for research purposes.
2.2 Chondrocyte isolation and seeding
Cartilage was dissected under sterile conditions and chondrocytes were isolated from the tissue by sequential incubation at 37°C in complete Dulbecco’s modified Eagle medium (DMEM) supplemented with 20% (v/v) foetal bovine serum (DMEM + 20% FBS) and 5.7 mg/ml pronase for 1 h followed by incubation in DMEM + 20% FBS with 100 U/ml collagenase overnight, as described previously (Heywood and Lee, 2017). All reagents were from Sigma-Aldrich (Poole, United Kingdom). Cells obtained from different donors were isolated and maintained separately, reflecting cell therapy practices, and cultures from each donor allocated to all treatment groups. Freshly isolated chondrocytes were maintained in suspension culture for short term experiments or seeded either into culture dishes or onto serum coated coverslips, at a density of 2 × 104 cells/cm2 and cultured under standard 5% (v/v) CO2 conditions in culture media as outlined below.
2.3 Chondrocyte expansion and sirtuin modulation
Control culture media consisted of DMEM+10% (v/v) FBS containing 10 mM glucose and supplemented with 2 mM L-glutamine, 18 mM HEPES buffer, 88 U/ml penicillin, and 88 mg/ml streptomycin (all from Sigma-Aldrich). Media containing 1 mM glucose was supplemented with 9 mM galactose to maintain osmotic balance, as described previously (Heywood et al., 2014). Osmolarity of 10 mM glucose base media was increased through the addition of D-mannitol (Sigma-Aldrich). Osmolarity was confirmed using a freezing-point depression osmometer (model 3250, Advanced Instruments, Norwood, MA). For short term studies on the role of osmolarity or glucose, samples of 1 × 106 freshly isolated chondrocytes were incubated in suspension with appropriate culture media for 5 h or 6 h respectively. Cells were passaged at 85%–90% confluence using trypsin-EDTA (Sigma-Aldrich) to recover the cells, with cell number determined using a haemocytometer, before re-seeding. Cell proliferation at intervening time points was monitored by manually counting cells from representative fields of view from each flask, with phase contrast images captured daily using a microscope-mounted camera. Once the cells had reached a total of 2, 4, 10, and 25 population doublings (PDs), cell extracts for gene expression analysis were collected and cells plated onto coverslips were prepared for immunofluorescent staining. 0 PD cells for gene expression analysis were maintained in culture dishes for 24 h following isolation with treatments as indicated. At 4 PDs cells were detached with trypsin-EDTA, washed and resuspended in fresh culture media before preparation of cell pellets as described below.
Modulators of sirtuin activity were 10 mM nicotinamide (NAM), 100 µM nicotinamide mononucleotide (NMN; both Sigma Aldrich) or 100 nM SRT1720 (Merck, Watford, United Kingdom). Solutions of NAM and NMN were prepared in culture media and SRT1720 was prepared as a stock solution at 25 mM in DMSO before dilution in culture media, such that DMSO represented less than 0.001% v/v at the final concentration of 100 nM. The efficacy of NMN to increase chondrocyte NAD levels was confirmed using a commercial fluorometric NAD assay kit (ab176723, Abcam, Cambridge, United Kingdom) after 4 h exposure using the manufacturer’s methods. Chondrocytes supplemented with the nicotinamide phosphoribosyltransferase (NAMPT) inhibitor FK866 (Sigma Aldrich) at 10 nM, which decreases cellular NAD, acted as a negative control (Supplementary Figures S1A,B), whereas the SRT1720 dose was selected from viability dose-response curves (Supplementary Figure S1C) and published efficacy (Milne et al., 2007).
2.4 3D chondrogenic pellet culture
Freshly isolated chondrocytes (0 PD) or chondrocytes cultured to 4 PDs were centrifuged at 380 × g such that a cell mass formed at the base of the centrifuge tube. Cell pellets consisting of 0.5 × 106 or 1 × 106 chondrocytes were cultured in 1 ml or 2 ml of control media respectively, with the addition of 50 µM ascorbate (Sigma-Aldrich), such that the treatments applied during the preceding monolayer phase were discontinued. Cell pellets were maintained with loose caps at 37°C and 5% CO2, with medium replacement every 2–3 days. After culture for up to 28 days, the wet weight of the cell pellets was recorded and the pellets frozen prior to biochemical analysis of ECM constituents. In addition, NMN (100 µM) or SRT1720 (100 nM) were included in the culture media of pellets formed from freshly isolated cells, to examine the effect of treatments on differentiated cells during the 3D culture phase.
2.5 Biochemical analysis
Samples for biochemical analysis were digested with papain as described previously (Heywood et al., 2004), prior to quantification of sulphated glycosaminoglycan (sGAG) content using the dimethylmethylene blue assay (Farndale et al., 1986), and estimation of collagen content using the hydroxyproline assay (Kafienah and Sims, 2004). DNA content was assessed with a fluorometric assay using Hoechst 33258 (Sigma-Aldrich) as established by Kim et al. (1988).
2.6 qPCR
Gene expression following expansion for up to 25 PDs was determined by quantitative polymerase chain reaction (qPCR). Total RNA of monolayer cells was extracted using TRIzol reagent (Invitrogen) according to the manufacturer’s instructions. RNA yield and quality was assessed using a NanoDrop spectrophotometer (Thermo Fisher Scientific). 500 ng of RNA from each sample was reverse transcribed to cDNA using the Thermoscript reverse transcription kit (Invitrogen) with oligo dT primers according to the manufacturer’s instructions. Quantitative PCR was performed on samples in parallel using a MX3000P real time PCR machine (Stratagene, La Jolla, CA) with 1 μl of cDNA (or water control) in a 10 μl final reaction volume using hot-start DNA polymerase (Qiagen, Crawley, United Kingdom) in the presence of SYBR green (Sigma-Aldrich), ROX dye (Invitrogen), and 0.25 μM of specific primers (Table 1), with melt curve analysis of the final product. Data were normalised to endogenous control genes, β-2-microglobulin (B2M) and/or β-actin (ACTB).
2.7 Immunofluorescent staining
Parallel cell cultures seeded onto glass coverslips were fixed at indicated time points in 4% paraformaldehyde for 10 min. After washing in sterile phosphate buffered saline, fixed specimens were stored at 4°C until all time points were collected for a given experimental replicate. Coverslips from all time points were processed in the same batch. Cells were permeabilised with 0.5% Triton X–100 in phosphate buffered saline (PBS) for 5 min and blocked with 5% goat serum in 0.1% BSA-PBS (all Sigma–Aldrich) for 1 h. Cells were incubated with primary antibodies in 0.1% BSA-PBS at 4°C overnight as follows: rabbit polyclonal anti-Sirt1 (Abcam ab13749, RRID: AB_300612) at 1:100, mouse monoclonal anti-H3K27me3 (Abcam ab6147, RRID: AB_449502) at 1:500. Following repeated washing in 0.1% BSA-PBS, cells were incubated with goat anti-rabbit Alexa Fluor 555 (Invitrogen A-21430, RRID: AB_2535851) and goat anti-mouse Alexa Fluor 488 (Invitrogen A-11017, RRID: AB_2534084) conjugated secondary antibodies at 1:1000 for 1 h at room temperature. Nuclei were detected with 1 μg/ml 4′,6-diamidino-2-phenylindole (DAPI; Sigma–Aldrich) in PBS. Following washes in PBS, coverslips were mounted with Fluoromount-G (Cambridge Bioscience, Cambridge, United Kingdom).
Immunofluorescently labelled coverslips were imaged on a Leica DMI4000B fluorescent microscope with a 63x/1.30 oil objective and captured as 16-bit images. A custom script implemented using MATLAB (MathWorks, Cambridge, United Kingdom) was used to identify nuclei and analyse nuclear intensity of SIRT1 and H3K27me3. DAPI labelled nuclei were identified using a Canny threshold. The mean intensity within a region of interest specified by each nucleus was evaluated in each fluorescent channel and output for statistical analysis.
2.8 Statistical analysis
Statistical analyses were performed using Minitab 18 software (Minitab, Coventry, United Kingdom) or Microsoft Excel. When data sets adhered to a normal distribution, two-way t-test, ANOVA, or a general linear model for analysis of variance were used with Bonferroni correction or Tukey tests for multiple comparisons. For non-parametric data sets, Mann–Whitney tests were used to compare conditions. Data quoted in the text are presented as mean ± s.e.m. Details of specific statistical tests and n values can be found in the figure legends.
3 Results
3.1 Physiological glucose and osmolarity enhance SIRT1 but not SIRT3 expression
The chondrocyte transition from cartilage tissue to monolayer culture is typically accompanied by a shift from a microenvironment with high osmolarity and low glucose to one with low osmolarity and high glucose. Accordingly we assessed the expression of sirtuins 1 and 3 (SIRT1 and SIRT3) in primary chondrocytes subjected to levels of glucose and osmolarity representative of the in vivo niche. Exposure of freshly isolated chondrocytes to media with osmolarity ranging from 250 to 500 mOsm for 5 h induced an osmolarity associated increase in SIRT1 gene expression (Figure 1A). However, SIRT3 gene expression was not significantly altered (Figure 1B). Culture of chondrocytes in 1 mM glucose for 6 h increased SIRT1 expression (Figure 1C), but had little effect on SIRT3 expression (Figure 1D). Accordingly, SIRT1 is highly responsive to departures from in vivo osmolarity and glucose concentrations typically associated with the switch to monolayer culture.
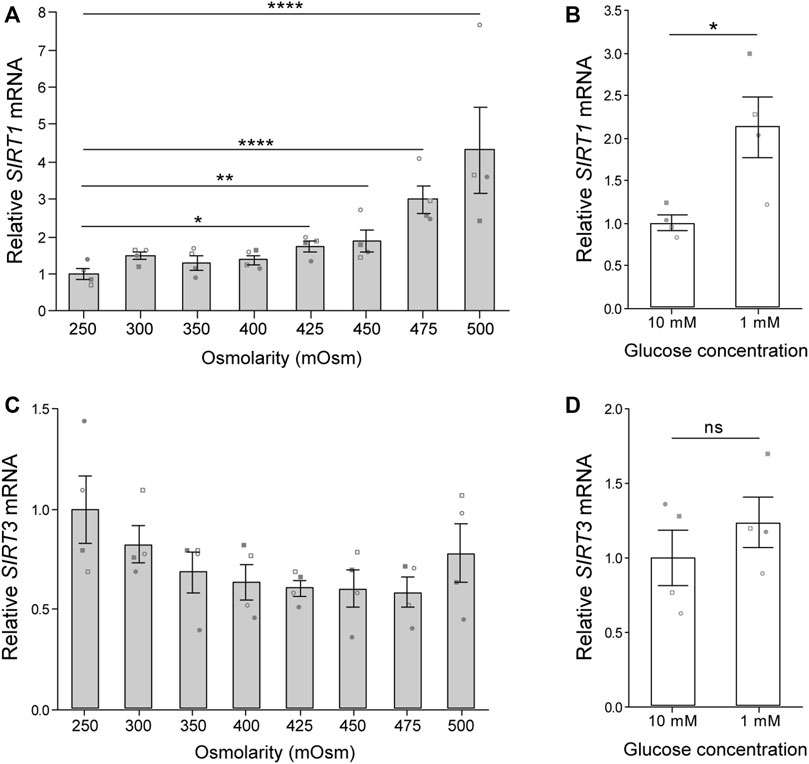
FIGURE 1. Osmolarity and glucose concentration regulate SIRT1 but not SIRT3 gene expression. (A,B) Gene expression of (A) sirtuin-1 (SIRT1) and (B) sirtuin-3 (SIRT3) normalised to β-2-microglobulin (B2M) endogenous control and presented relative to 250 mOsm. Mean ± s.e.m., n = 4 donors represented by unique symbols, General linear model with Dunnet multiple comparisons against 250 mOsm: *p = 0.011, **p = 0.005, ****p < 0.0001. (C,D) Gene expression of (C) SIRT1 and (D) SIRT3 normalised to β-actin (ACTB) endogenous control and presented relative to 10 mM glucose. Mean ± s.e.m., n = 4 donors represented by unique symbols, paired t-test: *p = 0.030, n. s. P > 0.05. Donors in A,B are distinct from donors in C,D.
3.2 SIRT1 expression declines during in vitro monolayer expansion
Cell therapies typically involve chondrocyte population expansion by monolayer culture in vitro before re-implantation into the damaged site at a high cell density. Population expansion is typically limited to 3–4 population doublings (PDs) due to the concomitant loss of phenotypic expression during monolayer culture. In agreement with previous studies (Dell’Accio et al., 2001; Giovannini et al., 2010), a rapid loss of chondrocyte phenotypic marker gene expression for aggrecan (ACAN) and collagen type 2 (COL2A1) was observed with monolayer expansion (Figures 2A,B). In a new finding, SIRT1 expression also decreased with increasing PD number, such that a 51.1 ± 6.1% reduction in SIRT1 mRNA expression occurred by 4 PDs (Figure 2C), with a similar reduction observed for SIRT1 protein expression assessed using immunofluorescent staining (p < 0.001; Figures 2D,E). A positive correlation was observed between the loss of SIRT1 expression and the phenotypic markers ACAN (r = 0.71, p < 0.001) and COL2A1 (r = 0.93, p < 0.001); Figures 2F,G). This supports previous findings relating SIRT1 activity and COL2A1 expression (Oppenheimer et al., 2014).
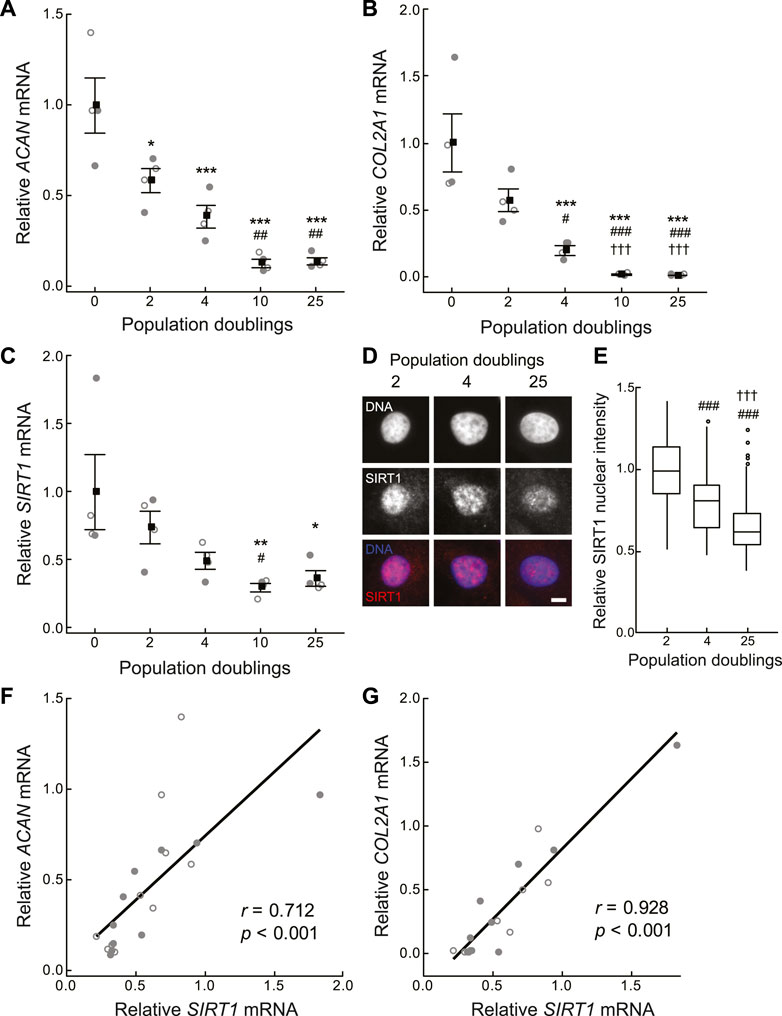
FIGURE 2. Cartilage matrix and sirtuin 1 gene expression declines during in vitro monolayer expansion of primary chondrocytes. (A–C) Gene expression of (A) aggrecan (ACAN), (B) collagen type II α-1 chain (COL2A1) and (C) sirtuin 1 (SIRT1) is normalised to β-2-microglobulin (B2M) and β-actin (ACTB) endogenous control genes and presented relative to 0 population doublings (PDs). Mean ± s.e.m., n = 4 from two experiments with individual data points represented by open and closed circles respectively, General linear model with Tukey pairwise comparisons: *p < 0.05, **p < 0.01 and ***p < 0.001 vs. 0 PDs, #p < 0.05, ##p < 0.01 and ###p < 0.001 vs. 2 PDs, †††p < 0.001 vs. 4 PDs. (D) Representative nuclear SIRT1 immunofluorescent staining. Scale bar 5 µm. (E) Nuclear SIRT1 protein levels relative to 2 PDs. Boxes represent median and interquartile range, with whiskers extending to 1.5 × interquartile range or the max/min data points, n2PD = 140, n4PD = 113, n25PD = 354 cells, General linear model with Tukey pairwise comparisons: ###p < 0.001 vs. 2 PDs, †††p < 0.001 vs. 4 PDs. (F,G) Scatter plots of (F) ACAN, and (G) COL2A1 against SIRT1 expression. n = 20 from two experiments with individual data points represented by open and closed circles respectively, Pearson correlation coefficient (r) and p-value < 0.001.
3.3 Chondrocytes have similar morphology and proliferation kinetics in the presence or absence of NAM
To assess whether sirtuin activity during population expansion is required to maintain the regenerative capacity of chondrocytes, medium was supplemented with the sirtuin reaction product and physiological pan-sirtuin inhibitor, nicotinamide (NAM), at 10 mM. NAM binds the regulatory C-pocket of sirtuins to inhibit NAD hydrolysis (Bitterman et al., 2002) and is demonstrated to be capable of abolishing sirtuin enzymatic activity at 10 mM (Guan et al., 2014). In addition to enhancing SIRT1 gene expression (Figure 1B), glucose deprivation is reported to enhance sirtuin activity by a mechanism linked to the induction of mitochondrial respiration upon glucose scarcity that is hypothesised to increase the NAD/NADH ratio (Lin et al., 2002; Heywood et al., 2006; Heywood et al., 2010). Therefore, chondrocytes expanded under 1 mM glucose were also assessed.
Chondrocytes expanded in monolayer exhibited similar morphology regardless of glucose concentration or sirtuin inhibition with NAM (Figure 3A). Population doubling time (PDT) was calculated from the linear proliferation phase up to 4 PDs. NAM did not markedly alter PDT at early timepoints, which was 1.33 and 1.37 days in media containing 10 mM glucose in the presence and absence of NAM, respectively (Figure 3B). Culture under 1 mM glucose conditions slightly increased these respective PDT to 1.65 and 1.57 days in the presence and absence of NAM, respectively (Figure 3C). Thus, expansion in 1 mM glucose resulted in a delay of 1–2 days in reaching four population doublings when compared to 10 mM glucose. Longer term, cells cultured in the presence of NAM treatment initiated proliferative arrest earlier than in the absence of NAM, illustrated by the premature deviation from the linear initial proliferation rate (Figures 3D,E) and consistent with the known role of SIRT1 antagonising senescence (Bitterman et al., 2002; Li and Tollefsbol, 2011).
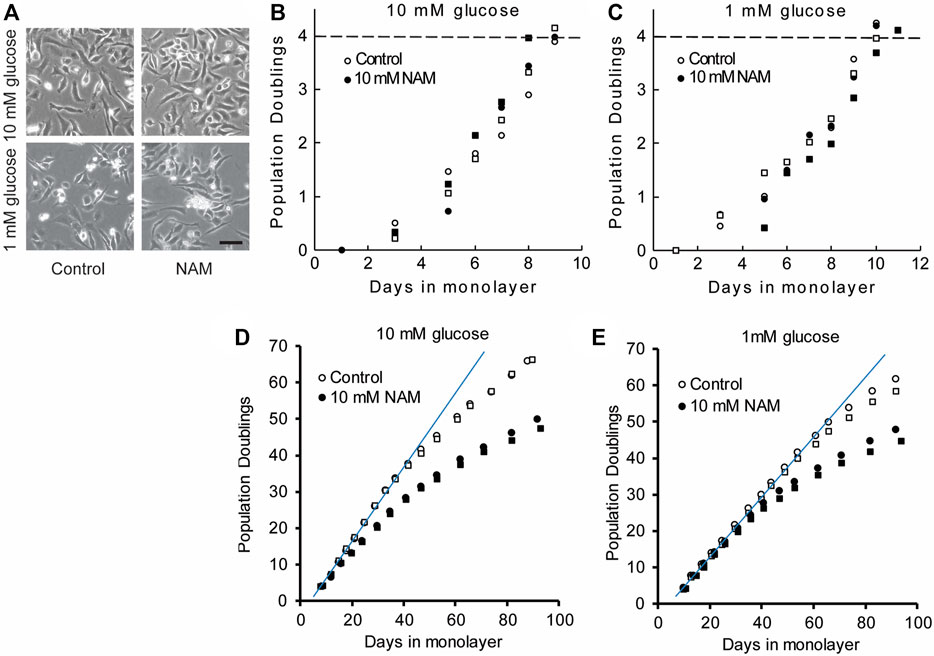
FIGURE 3. Chondrocytes have similar morphology and early proliferation kinetics in the presence or absence of the sirtuin inhibitor, NAM. (A) Chondrocytes expanded in monolayer exhibited similar morphology regardless of glucose concentration or sirtuin inhibition with NAM, illustrated by representative images at day 6. Scale bar 100 µm. (B,C) Proliferation kinetics data for chondrocytes expanded for 4PD in (B) 10 mM glucose and (C) 1 mM glucose with and without NAM. Data from two experiments indicated by circles and squares. The population doubling times calculated from the linear region of the population doubling/days in monolayer plots (B,C) were 1.49 ± 0.11 and 1.47 ± 0.12 days (mean ± s.e.m.) for control and NAM treated cells respectively. The final population doubling number at which cells were harvested to prepare cell pellets, indicated by the dashed line in (B) and (C) was 4.06 ± 0.08 and 4.05 ± 0.06 (mean ± s.e.m.) for control and NAM treated cells respectively. (D,E) Long-term proliferation kinetics in which a linear fit indicates the trajectory of the initial linear growth phase. During long-term culture, premature proliferative arrest was observed in the presence of NAM treatment for both 10 mM and 1 mM glucose media (D,E), illustrated by earlier deviation from linear growth compared to cells without NAM.
3.4 Treatment with the sirtuin inhibitor, NAM, during monolayer expansion diminished chondrocyte regenerative capacity
3D pellet cultures recapitulate the re-implantation phase of cell therapy techniques such as ACI, where the cells must be able to regenerate a cartilaginous matrix for clinical success. Cells were recovered from monolayer following 4 PDs and 3D pellets were prepared (Giovannini et al., 2010). The pellets were cultured for a further 28 days, using control media for all groups. The equivalence in population doubling prior to cell pellet formation, illustrated in Figures 3B,C, is important because this is linked to regenerative capacity (Dell’Accio et al., 2001; Giovannini et al., 2010). Cells cultured in monolayer for 4 PDs in the presence of the sirtuin inhibitor, NAM, generated smaller tissue mass after 28 days pellet culture compared with both the untreated control cells and freshly isolated cells that had not been expanded in monolayer prior to pellet formation (0 PD; Figures 4A,B). Wet weight was significantly reduced with expansion in NAM to 44.17 ± 0.02% (p < 0.001) of that of untreated controls for 10 mM glucose cultures (Figure 4B). Tissue composition mirrored wet weight, with significantly reduced collagen (41.51 ± 0.08%, p < 0.05) and sGAG (45.78 ± 0.03%, p < 0.001) content in pellets from cells exposed to NAM treatment during the preceding expansion phase (Figures 4C,D). Glucose deprivation is reported to increase sirtuin activity (Kaeberlein et al., 2002; Lin et al., 2002) and expression (Heywood et al., 2016). Expansion in media containing 1 mM glucose resulted in cells that produced significantly greater tissue mass upon 3D pellet culture compared to cells expanded in media containing 10 mM glucose (p < 0.05; Figure 4B). This effect was abolished by the presence of NAM during expansion, providing a significant interaction between glucose concentration and NAM supplementation (p = 0.002; Figure 4B). Trends in pellet DNA content at day 28 mirrored ECM, with freshly isolated (0 PD) and control cells proliferating during the 3D pellet phase, with a 58%–102% increase in DNA (Figure 4E). By contrast, NAM treated chondrocytes which proliferated as normal in 2D (Figures 3B,C) failed to demonstrate an increase in DNA on incorporation into 3D pellet culture (Figure 4E). Accordingly, inhibition of sirtuin activity during the monolayer expansion phase is linked to a marked reduction in cartilage tissue regenerated upon transfer to 3D pellet culture.
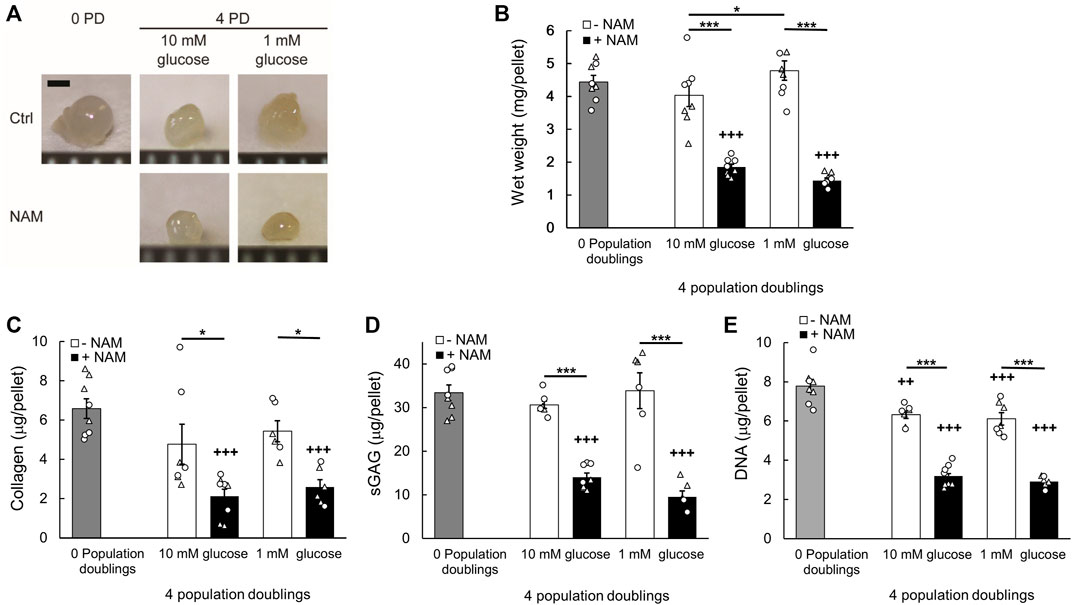
FIGURE 4. Inhibition of sirtuin activity during monolayer expansion abrogates chondrocyte regenerative capacity. (A) Representative cell pellets at day 28 of 3D culture created from chondrocytes which were either freshly isolated, 0 population doublings (0 PD), or monolayer expanded for 4 PDs in media containing either 10 mM or 1 mM glucose in the presence or absence of the sirtuin inhibitor nicotinamide (NAM). Scale bar 1 mm. (B) Pellet wet weight, (C) collagen content, (D) sulphated glycosaminoglycan (sGAG) content, and (E) DNA content at day 28. Mean ± s.e.m, n0PD = 8 (B–E), n4PD 10mM Ctrl = 9 (B) 7 (C–E), n4PD 10mM NAM = 10 (B) 8 (C–E), n4PD 1mM Ctrl = 10 (B) 6(C–E), n4PD 1mM NAM = 12 (B) 6 (C–D) 7 (E) from two experiments indicated by symbol shape, General linear model with Tukey pairwise comparisons: *p < 0.05, ***p < 0.001; +++p < 0.001 vs. 0 PD.
3.5 SIRT1 expression correlates with epigenetic chromatin modification in monolayer
Treatment with the sirtuin inhibitor NAM, restricted to the monolayer expansion phase, markedly altered regeneration in the subsequent 3D pellet (Figure 4). However, there was no evidence that the presence of NAM significantly affected the time course of ACAN and COL2A1 gene expression over the preceding 4 PDs in monolayer (Supplementary Figures S2A,B). Therefore, we examined evidence of epigenetic changes, consistent with an enduring cell phenotype. The chromatin mark H3K27me3 is associated with heterochromatin formation and gene silencing and has an important role in development and differentiation (Kuzmichev et al., 2002; Bosch-Presegue and Vaquero, 2015; Lui et al., 2016). Furthermore, SIRT1 is reported to participate in the polycomb repressive complex 4 (PRC4), a complex with H3K27me3 histone methyltransferase activity (Bosch-Presegue and Vaquero, 2015). In this study, H3K27me3 immunofluorescent intensity correlated positively with SIRT1 expression in the nuclei of chondrocytes cultured in both 10 mM and 1 mM glucose to 4 PDs (Figure 5). Treatment with the sirtuin inhibitor, NAM, during monolayer expansion decreased nuclear levels of both SIRT1 and H3K27me3 (Figures 5A–D) and weakened the correlation between these (Figures 5E,F). These data support the proposal that sirtuins are involved in the maintenance of this chromatin mark during monolayer expansion.
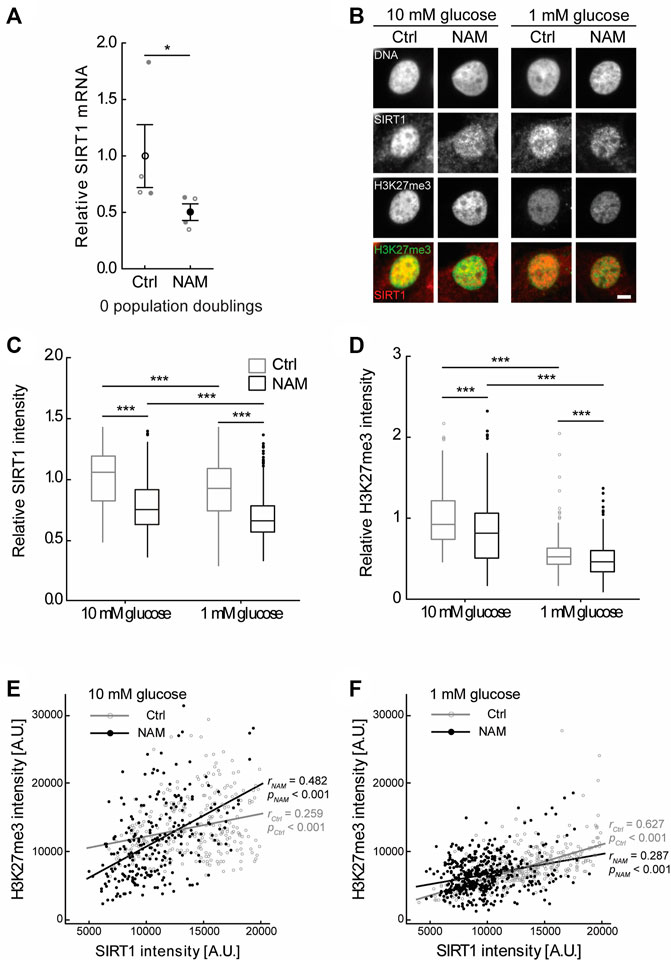
FIGURE 5. Treatment with the sirtuin inhibitor, NAM, decreased expression of SIRT1 and abundance of the epigenetic mark H3K27me3. (A) Treatment of primary (0 PD) chondrocytes for 24 h with 10 mM NAM significantly inhibited SIRT1 gene expression. Mean ± s.e.m., n = 4 from two experiments with individual data points represented by open and closed circles respectively, Mann-Whitney test: *p < 0.05. (B) Representative SIRT1 and H3K27me3 immunofluorescent nuclear staining in chondrocytes expanded for four population doublings (PDs) in media containing either 10 mM or 1 mM glucose in the presence or absence of the sirtuin inhibitor nicotinamide (NAM). Scale bar 5 µm. (C) Nuclear SIRT1 and (D) H3K27me3 levels in chondrocytes at 4 PDs relative to 10 mM glucose control cells. Boxes represent median and interquartile range, with whiskers extending to 1.5 × interquartile range or the max/min data points. (E,F) Scatter plots of nuclear H3K27me3 against nuclear SIRT1 intensity in 4 PD chondrocytes cultured in (E) 10 mM glucose or (F) 1 mM glucose in the presence or absence of NAM. n10mM Ctrl = 270 cells, n10mM NAM = 246 cells, n1mM Ctrl = 416 cells, n1mM NAM = 468 cells from two experiments. (C,D) Mann-Whitney test: ***p < 0.001. (E,F) Pearson correlation coefficient (r) and p-value presented.
3.6 Pharmacological augmentation of sirtuin activity in monolayer enhances pellet culture mass
Next, we tested whether augmentation of sirtuin activity in chondrocytes during monolayer culture enhances subsequent cartilage tissue generation upon transfer to a cell pellet culture model of re-implantation. 100 μM NMN or 100 nM SRT1720 (Milne et al., 2007), were added during chondrocyte expansion to 4 PDs, at which point 3D pellets were prepared. NMN is anticipated to activate the whole sirtuin family (SIRT1-7) by acting as the precursor of their common sirtuin substrate, NAD (Imai, 2010; Imai and Guarente, 2014), whereas the synthetic sirtuin activator SRT1720 has specificity for SIRT1 (Milne et al., 2007). During expansion, chondrocytes treated with NMN and SRT1720 had similar proliferation rates compared to untreated controls (data not shown). Day 21 pellets are illustrated in Figure 6A and compared to cell pellets which were prepared from the freshly isolated cells (0 PD) to indicate the performance of fully differentiated cells from each donor. The wet weights were significantly greater for cells expanded in the presence of sirtuin activators, being 60% and 34% greater compared to untreated controls, for NMN and SRT1720 respectively (p < 0.001; Figure 6B). Analysis of the tissue composition (Figures 6C–E) indicated that the key effect was improved maintenance of cell number during the pellet phase. In untreated cells, measured cell numbers were lower after pellet culture than when seeded within the pellet, suggesting loss of viability (Figure 6E). Additionally, the tissue regenerated by the NMN treatment group had 38% greater collagen content (p < 0.05) while sGAG levels were not significantly enhanced.
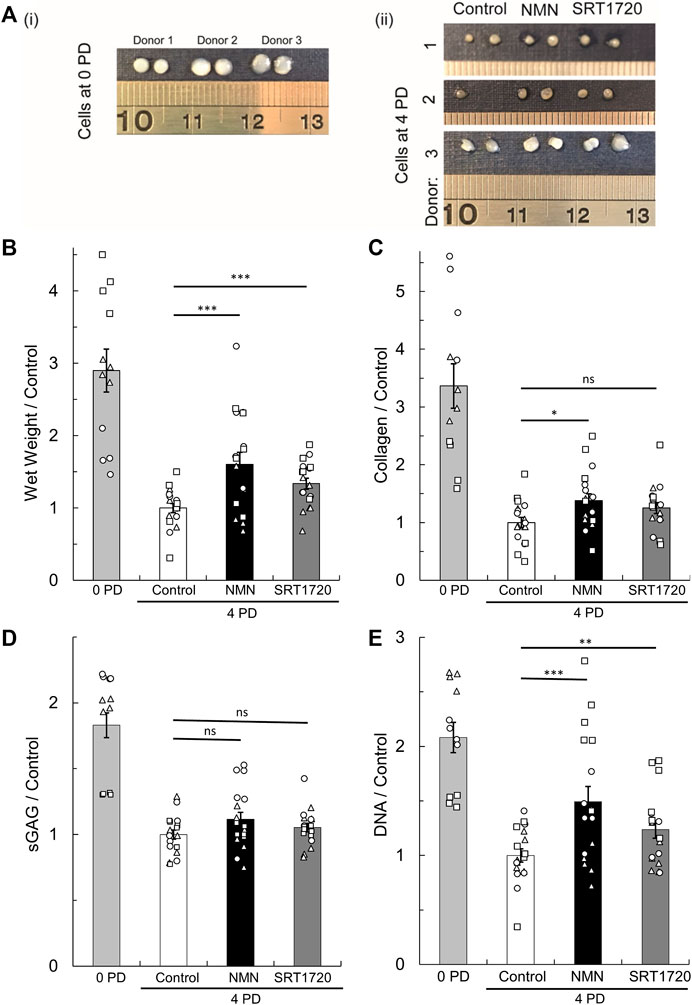
FIGURE 6. Regeneration of cartilage tissue following transfer of monolayer cells to 3D pellet culture. (A) Representative tissue masses formed after 21 days culture of cell pellets created from (i) freshly isolated cells (0 PD) or (ii) monolayer expanded for four population doublings (4 PDs) in media containing 10 mM glucose supplemented with the pan-sirtuin activator, 100 µM NMN, or the SIRT1 specific activator, 100 nM SRT1720, or untreated controls. Scale units cm. (B) Pellet wet weight, (C) collagen content, (D) sulphated glycosaminoglycan (sGAG) content, and (E) DNA content at day 21 relative to the 4 PD control. Mean ± s.e.m, n0PD = 12, n4PD = 18 from three donors indicated by symbol shape. 2-factor ANOVA with replication and bonferroni correction: *p < 0.05, **p < 0.01 ***p < 0.001, nsp > 0.05. The cell number seeded to pellets for each treatment group is shown in Supplementary Figure S3.
3.7 The beneficial effects of sirtuin activation are culture context dependent
Freshly isolated chondrocytes (0 PD) were prepared as cell pellets without prior monolayer culture, to examine the effect of sirtuin activation during the 3D culture phase (Figure 7). Unexpectedly, the presence of NMN during 3D pellet culture resulted in a 55% reduction in the tissue mass compared to untreated controls (p < 0.0001; Figure 7A). Both NMN and SRT1720 reduced collagen content, by 59% (p < 0.0001) and 25% (p < 0.01) respectively, while sGAG and DNA content were unaffected. Therefore, treatment of differentiated cells with sirtuin activators in a 3D tissue formation context may be detrimental to tissue regeneration, with implications for approaches that aim to use delivery of activators to enhance repair in situ.
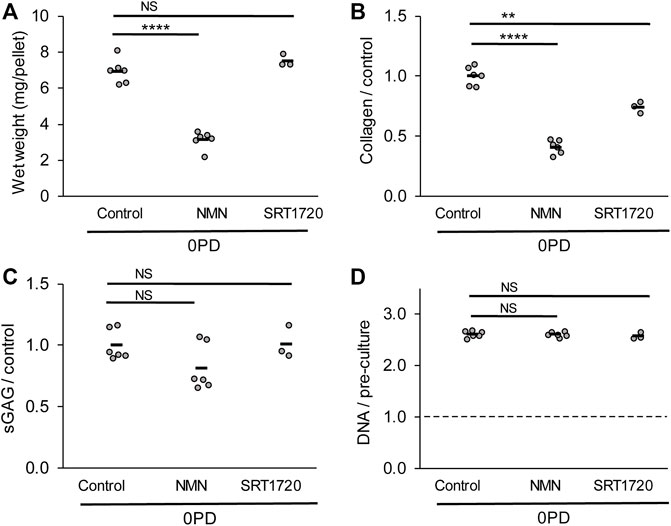
FIGURE 7. Regeneration of cartilage tissue by freshly isolated (0 PD) chondrocytes is impaired by the presence of sirtuin activators during 3D pellet culture. (A) Cell pellet wet weights, (B) collagen, (C) sulphated glycosaminoglycan and (D) DNA content after 21 days pellet culture in the presence or absence of sirtuin activators 100 µM NMN or 100 nM SRT1720. DNA content is normalised to pre-culture pellet values at day 0. n = 6 for all groups except SRT1720 where n = 3. The freshly isolated (0 PD) cells were pooled from four donors before pellet formation. Two-way t-test with Bonferroni correction: ***p < 0.001 ****p < 0.0001, NSp > 0.05.
4 Discussion
In this study, we examined the potential role of small molecule modulators of sirtuins to augment the regenerative potential of articular chondrocytes, using methods that replicate those adopted for the expansion of cells in regenerative therapies for cartilage repair. This study has demonstrated that SIRT1 gene expression progressively declines with culture duration during in vitro monolayer expansion, and that pharmacological modulators of sirtuin activity during in vitro monolayer culture influence the ability of expanded chondrocytes to regenerate a cartilaginous matrix upon transfer to a 3D culture environment. Importantly the regenerative capacity of monolayer-expanded chondrocytes can be manipulated via activation or inhibition of sirtuin activity during the expansion phase alone. We observed that SIRT1 gene expression diminishes during monolayer culture (Figure 2). Moreover, inhibition of sirtuin activity during the expansion phase, using NAM, further reduced regenerative capacity during subsequent 3D culture. Accordingly, we tested whether media supplementation during monolayer expansion with known activators of sirtuins would enhance the ability of the cells to synthesise a cartilaginous ECM in 3D, as a novel means of enhancing regenerative medicine approaches to cartilage repair.
Nicotinamide mononucleotide (NMN) is a precursor of the common sirtuin substrate, nicotinamide adenine dinucleotide (NAD), and has been demonstrated by others to enhance sirtuin activity when supplemented in the diet, or in culture medium of cells in vitro (Imai, 2010). Its efficacy in enhancing chondrocyte NAD levels in vitro was confirmed in our hands (Supplementary Figure S1). In addition, we examined the effect of the synthetic SIRT1-specific activator, SRT1720 (Milne et al., 2007). Cells expanded in the presence of either sirtuin activator generated significantly greater cartilage tissue mass on transfer to 3D culture (Figure 6). This was attributed both to an increase in cell number in the mature 3D tissue (NMN and SRT1720 treatments) and an increased accumulation of collagen ECM (NMN treatment) compared to controls. The positive regulation of cartilage-specific matrix gene expression, such as type II collagen, is an established role of SIRT1 in cartilage (Dvir-Ginzberg et al., 2008). However, comparison of the regenerated tissue composition, particularly cellularity (Figure 6E), suggests that the predominant effect of sirtuin activation in this system is to support the survival and proliferation of culture expanded chondrocytes following transfer from monolayer to a 3D environment. Consistent with this, expansion in the presence of the sirtuin inhibitor, NAM, produced the opposite effect, with significantly reduced cell numbers in the regenerated tissues (Figure 4E). Based on 7.7 pg of DNA per chondrocyte (Kim et al., 1988), we observe an approximate two-fold increase in cellularity in the 0 PD and control 4 PD conditions while overall cell number remains unchanged with NAM treatment (Figure 4E). The relative contribution of proliferation and apoptosis to these observations could be explored in the future.
These data are consistent with the pro-survival actions of sirtuins, which are reported to block apoptotic cell death under stress conditions, through a variety of mechanisms including inhibition of the pro-apoptotic protein, p53 (Luo et al., 2001; Vaziri et al., 2001; Takayama et al., 2009; Gagarina et al., 2010), Ku70 (Cohen et al., 2004), forkhead transcription factors (Motta et al., 2004), and p65 (Yeung et al., 2004). Alternatively, sirtuins may promote cell survival through crosstalk with hypoxia-inducible factor 1-α (HIF1α). It is reported that SIRT1 depletion or inactivation hyperacetylates HIF1α and reduces HIF1α accumulation under hypoxic conditions. Chondrocytes become increasingly dependent on oxidative phosphorylation within the aerobic environment in monolayer culture, which contrasts with the highly glycolytic metabolism of primary cells (Heywood and Lee, 2010, Heywood and Lee, 2017). The activation of sirtuins may therefore promote cell survival on transfer to 3D pellet culture via HIF1α activation, which adapts cell metabolism to the limited oxygen within the pellet culture. SIRT1 is established to modulate HIF1α activity and expression via direct interaction (Laemmle et al., 2012; Joo et al., 2015) or epigenetic modification of the HIF1α promoter (Dong et al., 2016; Luo and Wang, 2018). Further work is required to determine which mechanisms mediate the enhanced cellularity of tissues following cell expansion with sirtuin activators observed in this study.
NAM was used in this study as it is reported to bind to the regulatory C-pocket of sirtuins and inhibit their enzymatic activity (Bitterman et al., 2002; Guan et al., 2014). The supplementation or depletion of NAM is reported to inhibit or activate sirtuins, respectively (Luo et al., 2001; Anderson et al., 2003; Gallo et al., 2004). However, some NAM may be converted into NAD via the NAD salvage pathway, the rate limiting step of which is the conversion of NAM to NMN by nicotinamide phosphoribosyl transferase (NAMPT). Therefore, NAM treatment has the potential to indirectly activate sirtuins. However, the present study observed that chondrocytes treated with the putative sirtuin inhibitor NAM had the opposite response to treatment with the NAD precursor, NMN (compare Figures 4, 6). Therefore, the inhibitory effects of NAM treatment appear to outweigh any effects from de-novo NAD synthesis in this study. Differences in the efficacy of NAM as a sirtuin inhibitor may occur due to differential expression of NAMPT. For example, normal chondrocytes such as those used in this study are reported to express low levels of NAMPT compared to cells derived from OA tissue used in other studies (Dvir-Ginzberg et al., 2008). Indeed, NAMPT has also been shown to be upregulated in interleukin-1β mediated chondrocyte dedifferentiation, leading to SIRT1 activation (Hong et al., 2011). Further support for NAM as an inhibitor of sirtuins is shown in the long-term proliferation data (Figures 3D,E). As discussed above, sirtuins are established to antagonise cell apoptosis and growth arrest and we observed that the presence of NAM during long-term monolayer culture resulted in premature onset of proliferative growth arrest.
Sirtuins are involved in epigenetic regulation of numerous genes. For example, established SIRT1 targets include histones such as histone H4 lysine 16 (H4K16), histone H3K9, and H3K14 which regulate chromatin structure and biological function (Imai et al., 2000; Bosch-Presegue and Vaquero, 2015). Sirtuins are also involved in histone methylation, by modulating the activity of several methyltransferases (Roth and Chen, 2014; Bosch-Presegue and Vaquero, 2015). For example, SIRT1 associates with enhancer of zeste homolog 2 (EZH2) within polycomb repressive complexes (Kuzmichev et al., 2005), an enzyme with H3K27me3 histone methyltransferase activity (Bosch-Presegue and Vaquero, 2015). H3K27me3 is associated with heterochromatin formation and gene silencing and has an important role in development, differentiation and cell memory (Margueron and Reinberg, 2011; Bosch-Presegue and Vaquero, 2015; Heo et al., 2015; Marasca et al., 2018). H3K27 methylation plays a critical role in chondrocyte proliferation and hypertrophy in the growth plate (Lui et al., 2016), while inhibition of EZH2 histone-lysine N-methyltransferase activity, that leads to reduced H3K27me3, ameliorates OA development (Chen et al., 2016). Consistent with other studies, this study identified a correlation between SIRT1 expression and H3K27me3 (Vazquez et al., 2018) and found that the sirtuin inhibitor NAM reduced H3K27me3 (Figures 5B,D). This is consistent with a role of sirtuins in the maintenance of this chromatin mark during monolayer expansion of chondrocytes. Accordingly, the pharmacological modulation of sirtuin activity during monolayer expansion may leave an epigenetic fingerprint on the cells which could convey persistent effects on cell function following re-implantation.
Such persistence post-expansion may be advantageous as effects gained by in vitro treatment with sirtuin activators can be maintained following wash-out of the drug prior to transplantation, thereby avoiding direct exposure of the patient to the sirtuin modulating drugs. Previous reports have suggested that overexpression of sirtuins in vivo may have detrimental effects, for example SIRT1 and SIRT7 overexpression is reported to be an indicator of poor prognosis in some cancers (Deng et al., 2018; Ma et al., 2018). Moreover, in this study we observed that primary chondrocytes treated directly in pellet culture with sirtuin activators behaved differently from monolayer-expanded cells with markedly decreased matrix synthesis (Figure 7). Together these finding suggest that restricting modulation of sirtuin activation to the in vitro expansion phase of cellular therapies may deliver a number of benefits.
NMN is a common activator for all sirtuins by acting as a precursor for their substrate NAD (Imai, 2010; Imai and Guarente, 2014). By contrast, the synthetic sirtuin activator, SRT1720, has many hundred-fold greater potency for SIRT1 than the closest homologues (Milne et al., 2007). The similar response to each activator illustrated in Figure 6 supports the hypothesis that the effect of sirtuin activation on cartilage regeneration may be attributable, at least in part, to SIRT1. Unexpectedly, this study found that the application of the pan-sirtuin activator, NMN, to freshly isolated chondrocytes in a 3D pellet inhibited tissue regeneration (Figure 7), particularly decreasing wet weight and collagen content. The mechanism is unknown and could be the subject of future studies. One potential cause may be due to the co-activation of SIRT7. In direct contrast to SIRT1 and SIRT6 which are required for cartilage homeostasis (Nagai et al., 2015; Wu et al., 2015; Fu et al., 2016), the selective knockdown of SIRT7 was reported to proportionally increase matrix synthesis in chondrocytes (Korogi et al., 2018). This highlights the importance of specificity of selected activators to sirtuin family members. Smith et al. (2022) recently used pellet culture to induce chondrogenic differentiation in human embryonic stem cells and found that activation of SIRT1 using 5 μM SRT1720 during pellet culture leads to increased collagen type II and aggrecan gene and protein expression, but reduced sGAG accumulation. This contrasts with our findings with mature freshly isolated bovine chondrocytes where we observed a decrease in total collagen with a 50 × lower dose of SIRT1720 (Figure 7B), indicating that the response to SIRT1 activation may depend on dose and the differentiation state of the chondroprogenitor cell or chondrocyte. These studies highlight the importance of the context in which sirtuin activators are applied. For example, although sirtuin activators may be beneficial if applied in monolayer or during differentiation, further research is required to determine whether they may be detrimental to healthy cartilage if given systemically to a patient or injected into the joint.
The use of sirtuin activators during the cell processing stage has potential to enhance the outcome of cell-based cartilage repair techniques such as ACI. However, the regenerative capacity of expanded chondrocytes was not restored to that of freshly isolated (0 PD) cells. Further dose-optimisation may be achieved, but the efficacy of small molecule sirtuin activators will be dependent on the retained expression of the target sirtuins, which we have demonstrated to decline in vitro (Figures 2C–D). Similarly, the expression of SIRT1 is reported to be reduced in chondrocytes derived from OA tissue (Dvir-Ginzberg et al., 2008). Thus, variations in sirtuin expression may explain the variable response of cells isolated from different donors to sirtuin activators following equivalent population expansion (Figure 6), in addition to other biological variation. Therefore, research effort should be directed toward identifying the physiological conditions that support the retention of sirtuin expression in vitro.
The present study demonstrates for the first time that modulation of sirtuin activity during in vitro monolayer expansion influences subsequent chondrocyte regenerative potential. Given that in vitro monolayer expansion is a requirement of cell therapy-based cartilage repair strategies, these findings have relevance to the clinical success of cartilage repair procedures. Significant increases in regenerated tissue mass, cell survival and collagen content were achieved by supplementing expansion media with selected small molecule sirtuin activators. Importantly, however, the use of these sirtuin activators on differentiated cells in situ may be contraindicated.
Data availability statement
The original contributions presented in the study are included in the article/Supplementary Materials, further inquiries can be directed to the corresponding author.
Author contributions
HH designed the study, performed experiments, analysed and interpreted the data and wrote the manuscript. ST designed the study, performed experiments, analysed and interpreted the data, performed statistical tests and critically revised the manuscript. RJ conducted cell culture and performed biochemistry assays with data analysis and reviewed the manuscript. PC devised the study, contributed to scientific discussions and to the manuscript and provided funding. DL devised and supervised the study, contributed to scientific discussions, data interpretation, critically revised the manuscript and provided funding.
Funding
This work was supported by The Dunhill Medical Trust (R339/0214) and the Biotechnology and Biological Sciences Research Council (BB/N018532/1).
Acknowledgments
The authors thank Miss Sabha Asghar and Miss Leila R. Hosseini for assistance with cell proliferation analysis and image analysis.
Conflict of interest
The authors declare that the research was conducted in the absence of any commercial or financial relationships that could be construed as a potential conflict of interest.
Publisher’s note
All claims expressed in this article are solely those of the authors and do not necessarily represent those of their affiliated organizations, or those of the publisher, the editors and the reviewers. Any product that may be evaluated in this article, or claim that may be made by its manufacturer, is not guaranteed or endorsed by the publisher.
Supplementary material
The Supplementary Material for this article can be found online at: https://www.frontiersin.org/articles/10.3389/fbioe.2022.971932/full#supplementary-material
References
Anderson, R. M., Bitterman, K. J., Wood, J. G., Medvedik, O., and Sinclair, D. A. (2003). Nicotinamide and PNC1 govern lifespan extension by calorie restriction in Saccharomyces cerevisiae. Nature 423 (6936), 181–185. doi:10.1038/nature01578
Bar Oz, M., Kumar, A., Elayyan, J., Reich, E., Binyamin, M., Kandel, L., et al. (2016). Acetylation reduces SOX 9 nuclear entry and ACAN gene transactivation in human chondrocytes. Aging Cell 15 (3), 499–508. doi:10.1111/acel.12456
Bitterman, K. J., Anderson, R. M., Cohen, H. Y., Latorre-Esteves, M., and Sinclair, D. A. (2002). Inhibition of silencing and accelerated aging by nicotinamide, a putative negative regulator of yeast sir2 and human SIRT1. J. Biol. Chem. 277 (47), 45099–45107. doi:10.1074/jbc.M205670200
Bosch-Presegue, L., and Vaquero, A. (2015). Sirtuin-dependent epigenetic regulation in the maintenance of genome integrity. FEBS J. 282 (9), 1745–1767. doi:10.1111/febs.13053
Brittberg, M., Lindahl, A., Nilsson, A., Ohlsson, C., Isaksson, O., and Peterson, L. (1994). Treatment of deep cartilage defects in the knee with autologous chondrocyte transplantation. N. Engl. J. Med. Overseas. Ed. 331 (14), 889–895. doi:10.1056/NEJM199410063311401
Chen, L., Wu, Y., Wu, Y., Wang, Y., Sun, L., and Li, F. (2016). The inhibition of EZH2 ameliorates osteoarthritis development through the Wnt/β-catenin pathway. Sci. Rep. 6, 29176. doi:10.1038/srep29176
Cohen, H. Y., Miller, C., Bitterman, K. J., Wall, N. R., Hekking, B., Kessler, B., et al. (2004). Calorie restriction promotes mammalian cell survival by inducing the SIRT1 deacetylase. Science 305 (5682), 390–392. doi:10.1126/science.1099196
Dell'Accio, F., De Bari, C., and Luyten, F. P. (2001). Molecular markers predictive of the capacity of expanded human articular chondrocytes to form stable cartilage in vivo. Arthritis Rheum. 44 (7), 1608–1619. doi:10.1002/1529-0131(200107)44:7<1608:aid-art284>3.0.co;2-t
Deng, Z., Wang, X., Long, X., Liu, W., Xiang, C., Bao, F., et al. (2018). Sirtuin 7 promotes colorectal carcinoma proliferation and invasion through the inhibition of E-cadherin. Exp. Ther. Med. 15 (3), 2333–2342. doi:10.3892/etm.2017.5673
Dong, S. Y., Guo, Y. J., Feng, Y., Cui, X. X., Kuo, S. H., Liu, T., et al. (2016). The epigenetic regulation of HIF-1α by SIRT1 in MPP + treated SH-SY5Y cells. Biochem. Biophys. Res. Commun. 470 (2), 453–459. doi:10.1016/j.bbrc.2016.01.013
Dvir-Ginzberg, M., Gagarina, V., Lee, E. J., and Hall, D. J. (2008). Regulation of cartilage-specific gene expression in human chondrocytes by SirT1 and nicotinamide phosphoribosyltransferase. J. Biol. Chem. 283 (52), 36300–36310. doi:10.1074/jbc.M803196200
Elayyan, J., Lee, E. J., Gabay, O., Smith, C. A., Qiq, O., Reich, E., et al. (2017). LEF1-mediated MMP13 gene expression is repressed by SIRT1 in human chondrocytes. FASEB J. 31 (7), 3116–3125. doi:10.1096/fj.201601253R
Farndale, R. W., Buttle, D. J., and Barrett, A. J. (1986). Improved quantitation and discrimination of sulphated glycosaminoglycans by use of dimethylmethylene blue. Biochimica Biophysica Acta - General Subj. 883 (2), 173–177. doi:10.1016/0304-4165(86)90306-5
Fu, Y., Kinter, M., Hudson, J., Humphries, K. M., Lane, R. S., White, J. R., et al. (2016). Aging promotes sirtuin 3-dependent cartilage superoxide dismutase 2 acetylation and osteoarthritis. Arthritis Rheumatol. 68 (8), 1887–1898. doi:10.1002/art.39618
Fujita, N., Matsushita, T., Ishida, K., Kubo, S., Matsumoto, T., Takayama, K., et al. (2011). Potential involvement of SIRT1 in the pathogenesis of osteoarthritis through the modulation of chondrocyte gene expressions. J. Orthop. Res. 29 (4), 511–515. doi:10.1002/jor.21284
Gabay, O., Sanchez, C., Dvir-Ginzberg, M., Gagarina, V., Zaal, K. J., Song, Y., et al. (2013a). Sirtuin 1 enzymatic activity is required for cartilage homeostasis in vivo in a mouse model. Arthritis Rheum. 65 (1), 159–166. doi:10.1002/art.37750
Gabay, O., Zaal, K. J., Sanchez, C., Dvir-Ginzberg, M., Gagarina, V., Song, Y., et al. (2013b). Sirt1-deficient mice exhibit an altered cartilage phenotype. Jt. Bone Spine 80 (6), 613–620. doi:10.1016/j.jbspin.2013.01.001
Gagarina, V., Gabay, O., Dvir-Ginzberg, M., Lee, E. J., Brady, J. K., Quon, M. J., et al. (2010). SirT1 enhances survival of human osteoarthritic chondrocytes by repressing protein tyrosine phosphatase 1B and activating the insulin-like growth factor receptor pathway. Arthritis Rheum. 62 (5), 1383–1392. doi:10.1002/art.27369
Gallo, C. M., Smith, D. L., and Smith, J. S. (2004). Nicotinamide clearance by Pnc1 directly regulates Sir2-mediated silencing and longevity. Mol. Cell. Biol. 24 (3), 1301–1312. doi:10.1128/MCB.24.3.1301-1312.2004
Giovannini, S., Diaz-Romero, J., Aigner, T., Mainil-Varlet, P., and Nesic, D. (2010). Population doublings and percentage of S100-positive cells as predictors of in vitro chondrogenicity of expanded human articular chondrocytes. J. Cell. Physiol. 222 (2), 411–420. doi:10.1002/jcp.21965
Guan, X., Lin, P., Knoll, E., and Chakrabarti, R. (2014). Mechanism of inhibition of the human sirtuin enzyme SIRT3 by nicotinamide: Computational and experimental studies. PLoS One 9 (9), e107729. doi:10.1371/journal.pone.0107729
Heo, S. J., Thorpe, S. D., Driscoll, T. P., Duncan, R. L., Lee, D. A., and Mauck, R. L. (2015). Biophysical regulation of chromatin architecture instills a mechanical memory in mesenchymal stem cells. Sci. Rep. 5, 16895. doi:10.1038/srep16895
Heywood, H. K., Bader, D. L., and Lee, D. A. (2006). Rate of oxygen consumption by isolated articular chondrocytes is sensitive to medium glucose concentration. J. Cell. Physiol. 206 (2), 402–410. doi:10.1002/jcp.20491
Heywood, H. K., Caton, P. W., and Lee, D. A. (2016). SIRT1, an enzyme vital to cartilage homeostasis, is regulated by osmolarity and glucose levels. Int. J. Exp. Pathol. 97 (6). A2. doi:10.1111/iep.12211
Heywood, H. K., Knight, M. M., and Lee, D. A. (2010). Both superficial and deep zone articular chondrocyte subpopulations exhibit the Crabtree effect but have different basal oxygen consumption rates. J. Cell. Physiol. 223 (3), 630–639. doi:10.1002/jcp.22061
Heywood, H. K., and Lee, D. A. (2017). Bioenergetic reprogramming of articular chondrocytes by exposure to exogenous and endogenous reactive oxygen species and its role in the anabolic response to low oxygen. J. Tissue Eng. Regen. Med. 11 (8), 2286–2294. doi:10.1002/term.2126
Heywood, H. K., and Lee, D. A. (2010). Low oxygen reduces the modulation to an oxidative phenotype in monolayer-expanded chondrocytes. J. Cell. Physiol. 222 (1), 248–253. doi:10.1002/jcp.21946
Heywood, H. K., Nalesso, G., Lee, D. A., and Dell'accio, F. (2014). Culture expansion in low-glucose conditions preserves chondrocyte differentiation and enhances their subsequent capacity to form cartilage tissue in three-dimensional culture. Biores. Open Access 3 (1), 9–18. doi:10.1089/biores.2013.0051
Heywood, H. K., Sembi, P. K., Lee, D. A., and Bader, D. L. (2004). Cellular utilization determines viability and matrix distribution profiles in chondrocyte-seeded alginate constructs. Tissue Eng. 10 (9-10), 1467–1479. doi:10.1089/ten.2004.10.1467
Hong, E. H., Yun, H. S., Kim, J., Um, H. D., Lee, K. H., Kang, C. M., et al. (2011). Nicotinamide phosphoribosyltransferase is essential for interleukin-1β-mediated dedifferentiation of articular chondrocytes via SIRT1 and extracellular signal-regulated kinase (ERK) complex signaling. J. Biol. Chem. 286 (32), 28619–28631. doi:10.1074/jbc.M111.219832
Huang, B. J., Hu, J. C., and Athanasiou, K. A. (2016). Cell-based tissue engineering strategies used in the clinical repair of articular cartilage. Biomaterials 98, 1–22. doi:10.1016/j.biomaterials.2016.04.018
Imai, S. (2010). A possibility of nutriceuticals as an anti-aging intervention: Activation of sirtuins by promoting mammalian NAD biosynthesis. Pharmacol. Res. 62 (1), 42–47. doi:10.1016/j.phrs.2010.01.006
Imai, S., Armstrong, C. M., Kaeberlein, M., and Guarente, L. (2000). Transcriptional silencing and longevity protein Sir2 is an NAD-dependent histone deacetylase. Nature 403 (6771), 795–800. doi:10.1038/35001622
Imai, S., and Guarente, L. (2014). NAD+ and sirtuins in aging and disease. Trends Cell Biol. 24 (8), 464–471. doi:10.1016/j.tcb.2014.04.002
Joo, H. Y., Yun, M., Jeong, J., Park, E. R., Shin, H. J., Woo, S. R., et al. (2015). SIRT1 deacetylates and stabilizes hypoxia-inducible factor-1α (HIF-1α) via direct interactions during hypoxia. Biochem. Biophys. Res. Commun. 462 (4), 294–300. doi:10.1016/j.bbrc.2015.04.119
Kaeberlein, M., Andalis, A. A., Fink, G. R., and Guarente, L. (2002). High osmolarity extends life span in Saccharomyces cerevisiae by a mechanism related to calorie restriction. Mol. Cell. Biol. 22 (22), 8056–8066. doi:10.1128/MCB.22.22.8056-8066.2002
Kafienah, W., and Sims, T. J. (2004). Biochemical methods for the analysis of tissue-engineered cartilage. Methods Mol. Biol. 238, 217–230. doi:10.1385/1-59259-428-x:217
Kiernan, R., Bres, V., Ng, R. W., Coudart, M. P., El Messaoudi, S., Sardet, C., et al. (2003). Post-activation turn-off of NF-kappa B-dependent transcription is regulated by acetylation of p65. J. Biol. Chem. 278 (4), 2758–2766. doi:10.1074/jbc.M209572200
Kim, Y. J., Sah, R. L., Doong, J. Y., and Grodzinsky, A. J. (1988). Fluorometric assay of DNA in cartilage explants using Hoechst 33258. Anal. Biochem. 174 (1), 168–176. doi:10.1016/0003-2697(88)90532-5
Korogi, W., Yoshizawa, T., Karim, M. F., Tanoue, H., Yugami, M., Sobuz, S. U., et al. (2018). SIRT7 is an important regulator of cartilage homeostasis and osteoarthritis development. Biochem. Biophys. Res. Commun. 496, 891–897. doi:10.1016/j.bbrc.2018.01.129
Kuzmichev, A., Margueron, R., Vaquero, A., Preissner, T. S., Scher, M., Kirmizis, A., et al. (2005). Composition and histone substrates of polycomb repressive group complexes change during cellular differentiation. Proc. Natl. Acad. Sci. U. S. A. 102 (6), 1859–1864. doi:10.1073/pnas.0409875102
Kuzmichev, A., Nishioka, K., Erdjument-Bromage, H., Tempst, P., and Reinberg, D. (2002). Histone methyltransferase activity associated with a human multiprotein complex containing the Enhancer of Zeste protein. Genes Dev. 16 (22), 2893–2905. doi:10.1101/gad.1035902
Laemmle, A., Lechleiter, A., Roh, V., Schwarz, C., Portmann, S., Furer, C., et al. (2012). Inhibition of SIRT1 impairs the accumulation and transcriptional activity of HIF-1α protein under hypoxic conditions. PLoS One 7 (3), e33433. doi:10.1371/journal.pone.0033433
Lei, M., Wang, J. G., Xiao, D. M., Fan, M., Wang, D. P., Xiong, J. Y., et al. (2012). Resveratrol inhibits interleukin 1β-mediated inducible nitric oxide synthase expression in articular chondrocytes by activating SIRT1 and thereby suppressing nuclear factor-κB activity. Eur. J. Pharmacol. 674 (2-3), 73–79. doi:10.1016/j.ejphar.2011.10.015
Li, Y., and Tollefsbol, T. O. (2011). p16(INK4a) suppression by glucose restriction contributes to human cellular lifespan extension through SIRT1-mediated epigenetic and genetic mechanisms. PLoS One 6 (2), e17421. doi:10.1371/journal.pone.0017421
Li, Y., Xiao, W., Wu, P., Deng, Z., Zeng, C., Li, H., et al. (2016). The expression of SIRT1 in articular cartilage of patients with knee osteoarthritis and its correlation with disease severity. J. Orthop. Surg. Res. 11 (1), 144. doi:10.1186/s13018-016-0477-8
Lin, S. J., Kaeberlein, M., Andalis, A. A., Sturtz, L. A., Defossez, P. A., Culotta, V. C., et al. (2002). Calorie restriction extends Saccharomyces cerevisiae lifespan by increasing respiration. Nature 418 (6895), 344–348. doi:10.1038/nature00829
Lui, J. C., Garrison, P., Nguyen, Q., Ad, M., Keembiyehetty, C., Chen, W., et al. (2016). EZH1 and EZH2 promote skeletal growth by repressing inhibitors of chondrocyte proliferation and hypertrophy. Nat. Commun. 7, 13685. doi:10.1038/ncomms13685
Luo, J., Nikolaev, A. Y., Imai, S., Chen, D., Su, F., Shiloh, A., et al. (2001). Negative control of p53 by Sir2α promotes cell survival under stress. Cell 107 (2), 137–148. doi:10.1016/s0092-8674(01)00524-4
Luo, W., and Wang, Y. (2018). Epigenetic regulators: Multifunctional proteins modulating hypoxia-inducible factor-alpha protein stability and activity. Cell. Mol. Life Sci. 75 (6), 1043–1056. doi:10.1007/s00018-017-2684-9
Ma, M. C., Chiu, T. J., Lu, H. I., Huang, W. T., Lo, C. M., Tien, W. Y., et al. (2018). SIRT1 overexpression is an independent prognosticator for patients with esophageal squamous cell carcinoma. J. Cardiothorac. Surg. 13 (1), 25. doi:10.1186/s13019-018-0718-5
Marasca, F., Bodega, B., and Orlando, V. (2018). How Polycomb-Mediated Cell Memory Deals with a Changing Environment: Variations in PcG complexes and proteins assortment convey plasticity to epigenetic regulation as a response to environment. Bioessays 40 (4), e1700137. doi:10.1002/bies.201700137
Margueron, R., and Reinberg, D. (2011). The Polycomb complex PRC2 and its mark in life. Nature 469 (7330), 343–349. doi:10.1038/nature09784
Maroudas, A., and Evans, H. (1972). A study of ionic equilibria in cartilage. Connect. Tissue Res. 1 (1), 69–77. doi:10.3109/03008207209152058
Matsushita, T., Sasaki, H., Takayama, K., Ishida, K., Matsumoto, T., Kubo, S., et al. (2013). The overexpression of SIRT1 inhibited osteoarthritic gene expression changes induced by interleukin-1β in human chondrocytes. J. Orthop. Res. 31 (4), 531–537. doi:10.1002/jor.22268
Matsuzaki, T., Matsushita, T., Takayama, K., Matsumoto, T., Nishida, K., Kuroda, R., et al. (2014). Disruption of Sirt1 in chondrocytes causes accelerated progression of osteoarthritis under mechanical stress and during ageing in mice. Ann. Rheum. Dis. 73 (7), 1397–1404. doi:10.1136/annrheumdis-2012-202620
Milne, J. C., Lambert, P. D., Schenk, S., Carney, D. P., Smith, J. J., Gagne, D. J., et al. (2007). Small molecule activators of SIRT1 as therapeutics for the treatment of type 2 diabetes. Nature 450 (7170), 712–716. doi:10.1038/nature06261
Moon, M. H., Jeong, J. K., Lee, Y. J., Seol, J. W., Jackson, C. J., and Park, S. Y. (2013). SIRT1, a class III histone deacetylase, regulates TNF-alpha-induced inflammation in human chondrocytes. Osteoarthr. Cartil. 21 (3), 470–480. doi:10.1016/j.joca.2012.11.017
Motta, M. C., Divecha, N., Lemieux, M., Kamel, C., Chen, D., Gu, W., et al. (2004). Mammalian SIRT1 represses forkhead transcription factors. Cell 116 (4), 551–563. doi:10.1016/s0092-8674(04)00126-6
Nagai, K., Matsushita, T., Matsuzaki, T., Takayama, K., Matsumoto, T., Kuroda, R., et al. (2015). Depletion of SIRT6 causes cellular senescence, DNA damage, and telomere dysfunction in human chondrocytes. Osteoarthr. Cartil. 23 (8), 1412–1420. doi:10.1016/j.joca.2015.03.024
NICE (2017). Autologous chondrocyte implantation for treating symptomatic articular cartilage defects of the knee (TA477). London, United Kingdom: National Institute for Health Care Excellence, Resource impact report, 1–9.
Niemeyer, P., Pestka, J. M., Salzmann, G. M., Sudkamp, N. P., and Schmal, H. (2012). Influence of cell quality on clinical outcome after autologous chondrocyte implantation. Am. J. Sports Med. 40 (3), 556–561. doi:10.1177/0363546511428879
Oppenheimer, H., Kumar, A., Meir, H., Schwartz, I., Zini, A., Haze, A., et al. (2014). Set7/9 impacts COL2A1 expression through binding and repression of SirT1 histone deacetylation. J. Bone Min. Res. 29 (2), 348–360. doi:10.1002/jbmr.2052
Oswald, E. S., Chao, P. H., Bulinski, J. C., Ateshian, G. A., and Hung, C. T. (2008). Dependence of zonal chondrocyte water transport properties on osmotic environment. Cell. Mol. Bioeng. 1 (4), 339–348. doi:10.1007/s12195-008-0026-6
Roth, M., and Chen, W. Y. (2014). Sorting out functions of sirtuins in cancer. Oncogene 33 (13), 1609–1620. doi:10.1038/onc.2013.120
Saris, D. B., Vanlauwe, J., Victor, J., Almqvist, K. F., Verdonk, R., Bellemans, J., et al. (2009). Treatment of symptomatic cartilage defects of the knee: Characterized chondrocyte implantation results in better clinical outcome at 36 months in a randomized trial compared to microfracture. Am. J. Sports Med. 37 (1), 10S–19S. doi:10.1177/0363546509350694
Smith, C. A., Humphreys, P. A., Bates, N., Naven, M. A., Cain, S. A., Dvir-Ginzberg, M., et al. (2022). SIRT1 activity orchestrates ECM expression during hESC-chondrogenic differentiation. FASEB J. 36 (5), e22314. doi:10.1096/fj.202200169R
Takayama, K., Ishida, K., Matsushita, T., Fujita, N., Hayashi, S., Sasaki, K., et al. (2009). SIRT1 regulation of apoptosis of human chondrocytes. Arthritis Rheum. 60 (9), 2731–2740. doi:10.1002/art.24864
Vaziri, H., Dessain, S. K., Ng Eaton, E., Imai, S. I., Frye, R. A., Pandita, T. K., et al. (2001). hSIR2(SIRT1) functions as an NAD-dependent p53 deacetylase. Cell 107 (2), 149–159. doi:10.1016/s0092-8674(01)00527-x
Vazquez, M. J., Toro, C. A., Castellano, J. M., Ruiz-Pino, F., Roa, J., Beiroa, D., et al. (2018). SIRT1 mediates obesity- and nutrient-dependent perturbation of pubertal timing by epigenetically controlling Kiss1 expression. Nat. Commun. 9 (1), 4194. doi:10.1038/s41467-018-06459-9
Windhaber, R. A., Wilkins, R. J., and Meredith, D. (2003). Functional characterisation of glucose transport in bovine articular chondrocytes. Pflugers Arch. - Eur. J. Physiol. 446 (5), 572–577. doi:10.1007/s00424-003-1080-5
Wu, Y., Chen, L., Wang, Y., Li, W., Lin, Y., Yu, D., et al. (2015). Overexpression of Sirtuin 6 suppresses cellular senescence and NF-κB mediated inflammatory responses in osteoarthritis development. Sci. Rep. 5, 17602. doi:10.1038/srep17602
Keywords: chondrocyte, cartilage tissue engineering, sirtuin, glucose restriction, pellet culture, nicotinamide adenine dinucleotide
Citation: Heywood HK, Thorpe SD, Jeropoulos RM, Caton PW and Lee DA (2022) Modulation of sirtuins during monolayer chondrocyte culture influences cartilage regeneration upon transfer to a 3D culture environment. Front. Bioeng. Biotechnol. 10:971932. doi: 10.3389/fbioe.2022.971932
Received: 17 June 2022; Accepted: 18 November 2022;
Published: 06 December 2022.
Edited by:
Anna Lange-Consiglio, University of Milan, ItalyReviewed by:
Ryan Michael Porter, University of Arkansas for Medical Sciences, United StatesAnnunziata Mauro, University of Teramo, Italy
Copyright © 2022 Heywood, Thorpe, Jeropoulos, Caton and Lee. This is an open-access article distributed under the terms of the Creative Commons Attribution License (CC BY). The use, distribution or reproduction in other forums is permitted, provided the original author(s) and the copyright owner(s) are credited and that the original publication in this journal is cited, in accordance with accepted academic practice. No use, distribution or reproduction is permitted which does not comply with these terms.
*Correspondence: David A. Lee, ZC5hLmxlZUBxbXVsLmFjLnVr