- 1Department of Anatomy and Neurosciences, VU Medical Centre, Vrije Universiteit Amsterdam, Amsterdam, Netherlands
- 2Berlin Institute of Health, Charité – Universitätsmedizin Berlin, Berlin, Germany
- 3The School of Life Sciences, Immanuel Kant Baltic Federal University, Kaliningrad, Russia
- 4Max Delbrück Center for Molecular Medicine, Berlin, Germany
Serotonin (5-HT) is a crucial signal in the neurogenic niche microenvironment. Dysregulation of the 5-HT system leads to mood disorders but also to changes in appetite and metabolic rate. Tryptophan hydroxylase 2-deficient (Tph2-/-) mice depleted of brain 5-HT display alterations in these parameters, e.g., increased food consumption, modest impairment of sleep and respiration accompanied by a less anxious phenotype. The newly discovered neural stem cell niche of the adult hypothalamus has potential implications of mediating stress responses and homeostatic functions. Using Tph2-/- mice, we explore stem cell behavior and cell genesis in the adult hypothalamus. Specifically, we examine precursor cell proliferation and survival in Tph2-/- mice at baseline and following Western-type diet (WD). Our results show a decline in BrdU numbers with aging in the absence of 5-HT. Furthermore, wild type mice under dietary challenge decrease cell proliferation and survival in the hypothalamic niche. In contrast, increased high-calorie food intake by Tph2-/- mice does not come along with alterations in cell numbers. However, lack of brain 5-HT results in a shift of cell phenotypes that was abolished under WD. We conclude that precursor cells in the hypothalamus retain fate plasticity and respond to environmental challenges. A novel link between 5-HT signaling and cell genesis in the hypothalamus could be exploited as therapeutic target in metabolic disease.
Introduction
Besides the well-known neurogenic niches, the subventricular zone and the subgranular zone of the dentate gyrus, the hypothalamus has emerged as third region of postnatal neurogenesis and gliogenesis (Kokoeva et al., 2005; Lee et al., 2012; Robins et al., 2013a; Chaker et al., 2016). Positioned between the third ventricle and the median eminence, specialized radial glial tanycytes are thought to regulate the hypothalamic in- and output of hormones and nutrients to maintain body homeostasis (reviewed in Bolborea and Dale, 2013). Cell genesis within the adult hypothalamus may have an important role in feeding and reproduction control, in mediating stress responses, and in energy metabolism. Recent studies indicate the stem cell niche is responsive to mitogens (Haan et al., 2013; Robins et al., 2013a), leptin (Kokoeva et al., 2005) and dietary challenges (Lee et al., 2012, 2014), and thereby regulates appetite and energy expenditure (Pierce and Xu, 2010). The widespread monoamine serotonin (5-HT) impacts the variety of these functions (reviewed in Donovan and Tecott, 2013). Dysregulation of the 5-HT system leads to age-related memory loss and depression (reviewed in Alenina and Klempin, 2015), but also to changes in appetite and energy metabolism (Nigro et al., 2013; D’Agostino et al., 2018). At the same time, the precise role of 5-HT in the regulation of hypothalamic functions remains unknown. Here, we address this using tryptophan hydroxylase 2-deficient (Tph2-/-) mice selectively depleted of brain 5-HT. Tph2-/- mice exhibit transient early postnatal growth retardation, modest impairment of sleep and respiration, accompanied by a less anxious and highly aggressive phenotype (Alenina et al., 2009; Mosienko et al., 2012). Given the role of 5-HT in stress response and energy balance, we studied cell proliferation and fate plasticity in the hypothalamic nuclei of Tph2-/- mice, and the effect of high fat high cholesterol diet Western-type diet (WD) on cell survival. Our data reveal an age-dependent decline in BrdU numbers and alterations in food intake and cell phenotypes in the lack of brain 5-HT.
Materials and Methods
Animals and Treatment
Tph2-/- mice (Alenina et al., 2009) were bred onto the C57BL/6N background for more than 10 generations. Mice were housed under standard laboratory conditions with a light/dark cycle of 12 h each. Brain slices of young-adult (6 weeks of age, postnatal day (P) 42; n = 10), adult (3 months of age, P80; n = 8), and 1-year-old (P1y; n = 8) female mice and their littermates (CTR) (Klempin et al., 2013) were analyzed for baseline cell proliferation in the hypothalamus. Animals received three intraperitoneal injections (i.p.) of BrdU (5-bromo–2′-deoxyuridine, 50 mg/kg; Sigma-Aldrich) at 8:00 (2 h after lights went on), 14:00, and 20:00, and were killed 24 h after the first injection. Estrous cycle was not determined. Another cohort of three-month-old female Tph2-/- mice and their littermates (n = 20) were randomly assigned to two groups for standard diet (SD, 5.0% fat, 17.8% protein, 11.0% sugar) or WD (21.2% fat, 17.5% protein, 33.2% sugar, 2.071 mg/kg Cholesterol; TD-88137 ssniff, Germany). At day 6, 7, and 8 of the diet, BrdU (50 mg/kg) was injected twice per day. Animals were sacrificed after 7 weeks (49 days) of either diet to determine proliferation and survival of newly generated cells in the hypothalamus.
Tissue Preparation and Immunohistochemistry
Mice were deeply anesthetized and perfused transcardially with 0.9% sodium chloride followed by 4% paraformaldehyde (PFA). Brains were removed and placed into PFA overnight, and transferred into 30% sucrose the following day. BrdU immunohistochemistry followed the peroxidase method in accordance with an established protocol (Klempin et al., 2013). Briefly, one in-six series of sequential 40 μm coronal sections were stained free floating, and all immunoreactive cells detected throughout the hypothalamus were counted. The mean of both hemispheres was taken, and the total number of BrdU-positive (BrdU+) cells was estimated by multiplying cell counts by six. For the first experiment, BrdU numbers were determined per hypothalamic nuclei (arcuate nucleus, median eminence, third ventricle, and ventromedial nucleus). For Ki67 and phenotypic analysis, one-in-twelve series of sections were labeled for multiple immunofluorescence staining. BrdU+ cells were evaluated using Keyence (BioRevo BZ-9000) or Leica TCS SP5 confocal microscope (Leica, Germany) for three-dimensional colocalization. Primary antibodies were applied in the following concentrations: anti-BrdU (rat, 1:500; Biozol/AbD serotec), anti-Glial Fibrillary Acidic Protein (GFAP; rabbit, 1:1000; Abcam), anti-Iba1 (rabbit, 1:500; Wako), anti-Ki67 (rat/mouse, 1:500; eBios), anti-NG2 (rabbit, 1:200; Abcam), anti-NeuN (rabbit, 1:500; Millipore), anti-RIP (rabbit, 1:1000; Merck), anti-S100β (mouse, 1:1000; SIGMA) and anti-Sox2 (goat, 1:1000; Santa Cruz Biotechnology); Alexa488-, Cy3-, or Cy5-conjugated secondary antibodies were used (1:250; Invitrogen, United States).
Statistical Analysis
Statistical tests to detect differences between group means were performed by two-way ANOVA (experiment 1: genotype × age; experiment 2: genotype × diet), followed by Tukey’s post hoc tests, in cases where a significant F statistic was obtained (GraphPad PRISM 6.01 software). Student’s t-test was used for pair-wise comparison of BrdU and Ki67 analysis, and of hypothalamic subnuclei. All values are expressed as mean ± SEM. P-values of 0.05 were considered statistically significant.
Results
Cell Proliferation in the Adult Hypothalamus of Tph2-/- Mice Declines With Aging
We first quantified baseline cell proliferation (Figure 1A) in the hypothalamus of Tph2-/- and CTR mice at different ages. In Tph2-/- mice, a dramatic decline in BrdU+ cells was observed with aging [F(2,20) = 9.786, p genotype ×age = 0.0011; Figure 1B]. At P42, the number of BrdU+ cells was significantly higher relative to CTR (p = 0.0027; Figure 1B) while it was lower at P1y (p = 0.0431; Figure 1B). At P80, the amount of BrdU+ cells in the hypothalamus of CTR and Tph2-/- mice was similar (p = 0.5353; Figure 1B). When we looked at the distribution of BrdU+ cells within the various hypothalamic areas and nuclei (Figure 1C), the increase at P42 in Tph2-/- mice was reflected by increased cell numbers in the median eminence (Student’s t-test p = 0.0339) and arcuate nucleus (p = 0.0166), a tendency was observed for the ventromedial nucleus (p = 0.0668), while at P1y, Tph2-/- mice revealed significant fewer cells lining the third ventricle relative to CTR (p = 0.0057).
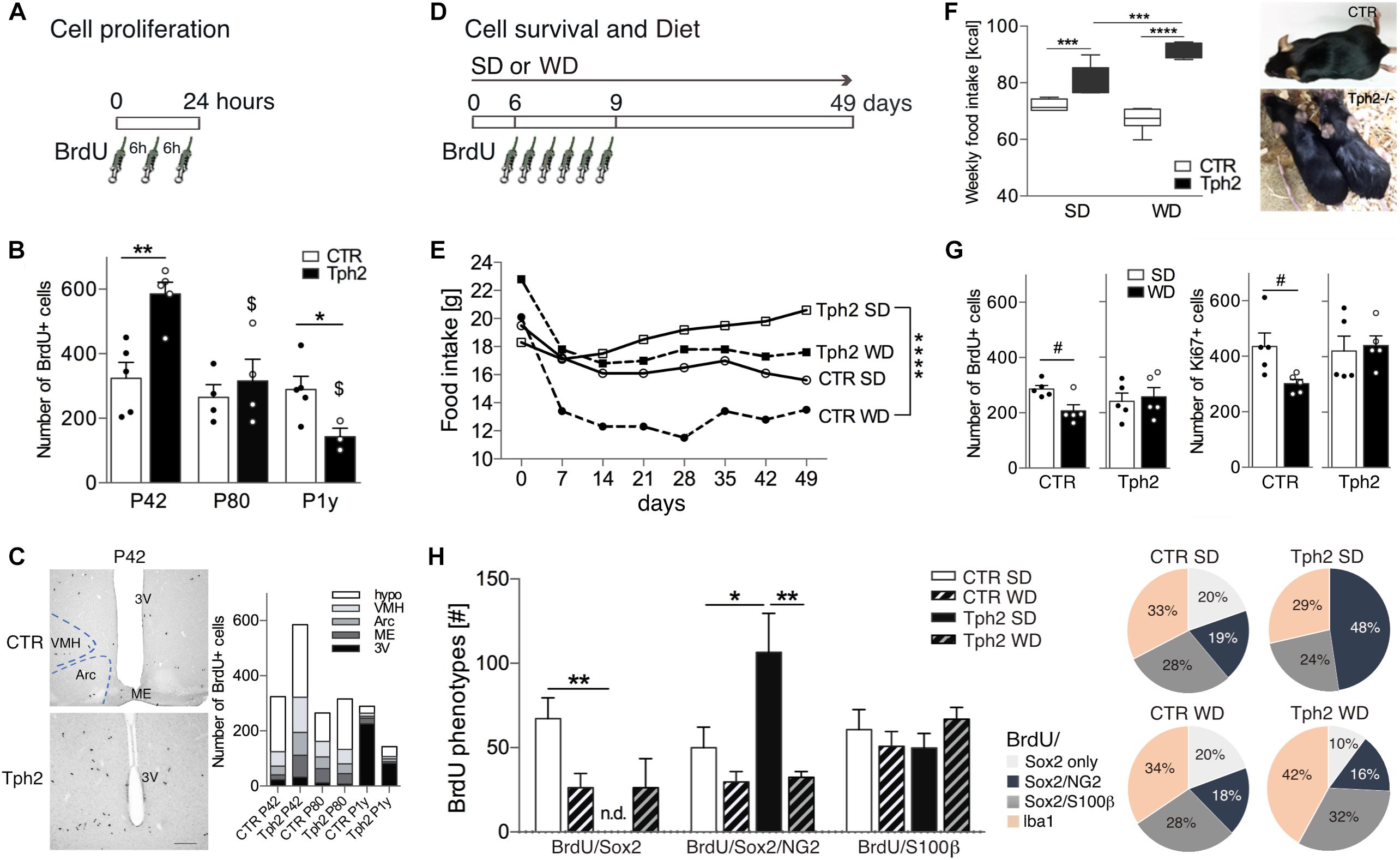
Figure 1. Cell proliferation, food intake and cell survival at baseline and after Western-type diet (WD). (A) The number of BrdU-positive (BrdU+) cells in the hypothalamic nuclei was quantified at 24 h after 3 injections of BrdU 6 h apart. (B) Cell proliferation (entire hypothalamus) was significantly higher in young-adult (P42) Tph2-/- mice (Tph2), declined with aging and was significantly lower at 1 year of age (P1y) relative to littermates (CTR). (C) Representative images of BrdU peroxidase staining at P42, and cell numbers per hypothalamus (hypo; outside subnuclei) and per Arc, arcuate nucleus, VMH, ventromedial nucleus, ME, median eminence and 3V, third ventricle; Scale bar 150 μm. (D) Three-month-old Tph2 and littermates (CTR) were subjected to either standard diet (SD) or WD and BrdU was injected on days 6, 7, 8, twice per day, to determine cell proliferation and cell survival 6 weeks later. (E,F) Average daily food intake was least in CTR and largest in Tph2 (E, in g per cage of n = 5; F, in Kcal). Tph2 displayed oily and sticky fur upon WD. (G,H) At 49 days, WD resulted in a significant decrease in BrdU+ and Ki67+ cells in the hypothalamus of CTR, while it had no effect in Tph2 (G, Student’s t-test #p < 0.05). Decreased cell survival in CTR was reflected by a reduction in BrdU/Sox2+ cells, however, phenotype distribution was unaffected. In Tph2 under SD, half of cells co-express BrdU/Sox2/NG2, abolishing the number (graph) and percentage (pie diagram) of BrdU/Sox2-only cells (H); notably, this was not observed after WD. Two-way ANOVA followed by Tukey’s post hoc test ∗p < 0.5, ∗∗p < 0.01, ∗∗∗p < 0.001, ∗∗∗∗p < 0.0001 between genotypes, $p < 0.05 to P42 of the same genotype.
Cell Survival Is Not Affected by Increased High-Calorie Food Intake in Tph2-/- Mice
Given the role in energy metabolism, we elucidated the effect of WD for 7 weeks on the number of newly generated cells in the hypothalamus of three-month-old Tph2-/- mice and littermates (Figure 1D). During the first week of WD, food consumption [g] declined but then remained constant for each group over time (Figure 1E). Average food intake [g] was least in CTR fed WD and largest in Tph2-/- mice fed SD [F(3,21) = 21.06, p < 0.0001; Figure 1E]. Translated to calorie intake (Kcal), Tph2-/- mice consumed more of both diets, most of the WD [F(1,23) = 27.99, p genotype × diet < 0.0001; Figure 1F]. Tph2-/- mice displayed oily and sticky fur starting at day 3 of WD that was absent in CTR (Figure 1F). Seven weeks of WD resulted in significantly decreased survival of BrdU+ cells in the hypothalamus of CTR (SD vs. WD, Student’s t-test p = 0.0154; Figure 1G). In contrast, increased high-calorie consumption by Tph2-/- mice did not affect cell numbers (SD vs. WD, Student’s t-test p = 0.7351; Figure 1G). Cell proliferation at 49 days was assessed by Ki67-labeling; here too, WD significantly decreased the number of newly generated cells in CTR (Student’s t-test p = 0.0284) while no effect was observed in Tph2-/- mice (Student’s t-test p = 0.7665; Figure 1G).
Lack of Brain 5-HT Affects Phenotype Distribution
We next assessed phenotypes of BrdU+ cells (Figure 1H) to determine the cell type affected in the absence of 5-HT and upon WD. BrdU+ cells were co-stained for the neural stem/progenitor markers GFAP and Sox2 (SRY-related HMG-box gene 2), the proteoglycan NG2, the oligodendrocyte lineage marker RIP, the astrocytes marker S100β, and the microglia marker Iba1. Sox2+ cells were highly proliferative; lining the third ventricle, they were considered as tanycytes (Figures 2A,B). Our results show a remarkably sparse number of BrdU-expressing tanycytes in CTR independent of the diet (<2.0% of total BrdU). Notably, no BrdU/Sox2-tanycyte was observed in Tph2-/- mice. GFAP+ cells located along the dorsal part of the ventricle (α2 tanycytes, (Robins et al., 2013a); Figure 2C) often co-expressed S100β (Figure 2C1), and were rarely dividing (Figure 2C2). BrdU/GFAP+ cells were only seen at SD for both genotypes accounting to 4% in CTR, and 5.8% of total BrdU in Tph2-/- mice (Figure 2C2). Analysis of glial phenotype distribution revealed two-thirds of BrdU+ cells expressed Sox2 (Figure 1H). In CTR, cells were predominantly triple labeled for BrdU/Sox2/S100β (∼30%) and BrdU/Sox2/NG2 (∼20%; Figures 1H, 2D). Notably, all BrdU/S100β+ cells co-expressed Sox2. In Tph2-/- mice at SD conditions, half of the cells co-expressed BrdU/Sox2/NG2 [F(2,46) = 7.764, p genotype = 0.0012; Figures 1H, 2E], lowering the amount of BrdU/Sox2-only cells. After WD, the decrease in BrdU+ cell numbers in CTR was reflected by decreased double and triple labeling of glial markers with a significant reduction in BrdU/Sox2+ cells (SD vs. WD, p = 0.024; Figure 1H). However, phenotype distribution was unaffected by WD in CTR. In Tph2-/- mice upon dietary challenge, the large population of BrdU/Sox2/NG2+ precursor cells was diminished to 16%, and fate distribution among glial cells was similar to CTR (Figure 1H, pie diagram). Approximately 30% of cells in all groups adopted an oligodendrocyte fate co-expressing BrdU/RIP (Figure 2F). Another third of BrdU+ cells (Sox2-) adopted a microglia fate characterized by co-expression of Iba1 (Figure 2G). We did not observe BrdU/NeuN+ cells in any group (Figure 2H). Congruent to NeuN staining, no BrdU+ cell expressing the neuronal marker β-III-Tubulin was found (Tuj1; not shown).
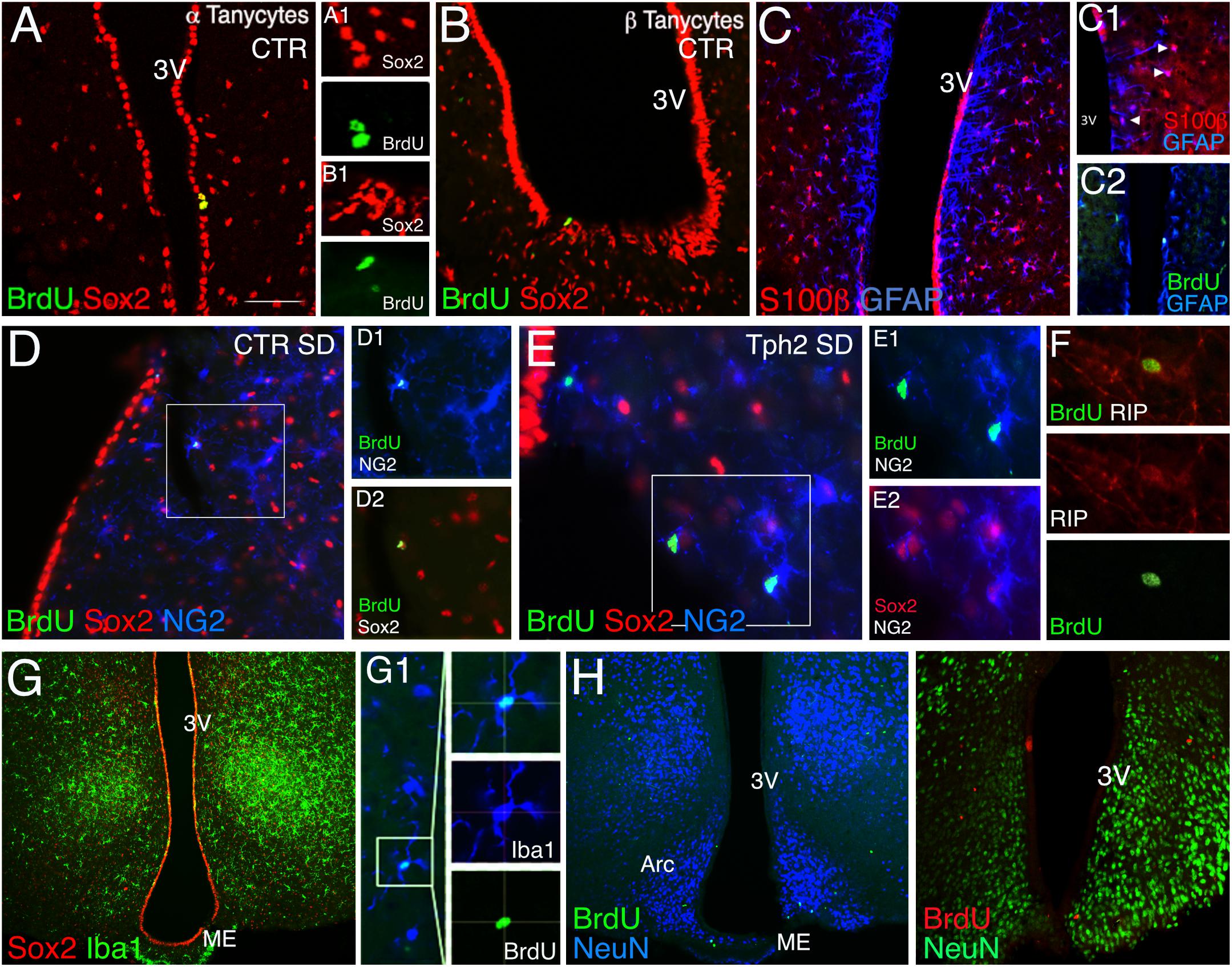
Figure 2. Immunohistochemistry of cell phenotypes. (A,B) In CTR mice, a sparse number of BrdU/Sox2-tanycytes was found lining the third ventricle and was absent in Tph2-/- mice (Tph2; α tanycyte, A1, β tanycyte, B1) Scale bar 150 μm. (C) GFAP+ cells lining the dorsal part of the ventricle often co-express S100β, arrowheads (C1), and were rarely dividing (BrdU/GFAP+, C2). (D–F) BrdU/Sox2/NG2+ cells account to ∼20% in CTR (D) and ∼50% in Tph2 (E) at standard diet, SD. Approx. 30% of these cells followed the oligodendrocyte fate expressing the lineage marker RIP (F). (G) One third of BrdU+ cells adopted a microglia fate in all groups (Iba1+/Sox2-). (H) No BrdU/NeuN co-expressing cell was found in all groups at 49 days of the experiment. Arc, arcuate nucleus, ME, median eminence, and 3V, third ventricle.
Discussion
Our data demonstrate an age-dependent decline in cell proliferation in the hypothalamus of Tph2-/- mice, but not in CTR, and a large population of proliferating Sox2/NG2 cells. In the lack of brain 5-HT, no proliferating Sox2-tanycytes were detected and the high-calorie-induced decrease in cell proliferation and survival was absent. Furthermore, we did not find newly generated neurons at 6 weeks after BrdU but a diet-induced altered phenotype distribution in Tph2-/- mice.
Neural stem/progenitor cell populations of α and β tanycytes in the adult hypothalamus, and postnatal born neurons, contribute to brain plasticity and potentially respond to hormones and nutrition to maintain metabolic rate (Lee et al., 2012; Bolborea and Dale, 2013; Haan et al., 2013; Robins et al., 2013a). Serotonin is involved in the regulation of feeding and metabolism, e.g., food intake enhances hypothalamic 5-HT indicating satiety (Leibowitz and Alexander, 1998; Schwartz et al., 2015; Nectow et al., 2017). On cellular level, 5-HT2C receptors on POMC neurons might particularly mediate the 5-HT effects (Lam et al., 2008; D’Agostino et al., 2018). Our data show that in the absence of brain 5-HT, Tph2-/- mice consumed significantly more suggesting they might lack satiety. This might correspond to no detectable proliferating Sox2-tanycytes; in a next step, POMC neurons in Tph2-/- mice should be studied (Klein et al., 2019). Furthermore, in the lack of brain 5-HT, a different subpopulation of precursor cells, Sox2/NG2, is favored and affected by WD; indicating 5-HT plays an important role at the stem/progenitor cell stage. Our earlier findings on neurogenesis in the hippocampus of Tph2-/- mice also revealed changes at Sox2 cell stage, suggesting physiological adaptations to changes in 5-HT supply to maintain homeostasis (Klempin et al., 2013). Hypothalamic Sox2/NG2 or NG2-only cells are highly proliferative with glial and some neuronal potential (Robins et al., 2013b). Fate plasticity makes them favorably affected to changes in the environment and in turn might act as compensatory mechanism. Increased proliferation of Sox2/NG2+ cells in Tph2-/- mice might compensate for the lack of BrdU/Sox2-tanycytes. Although a small number of newborn neurons was reported in few lineage tracing studies (Lee et al., 2012; Robins et al., 2013a) we have not detected any using our treatment protocol–based on BrdU and available markers of lineage progression–and it remains speculative whether the high number of NG2 cells eventually become neurons (Robins et al., 2013b).
In CTR mice, BrdU/Sox2+ cells were affected upon high-calorie consumption, reducing this precursor subset. In aged CTR mice, no difference in cell proliferation was observed; and a decline in only Sox2-tanycytes, e.g., starting at 11 months of age, was reported earlier (Zhang et al., 2017). In Tph2-/- mice, lack of brain 5-HT per se might not be responsible for the age-dependent decline in cell proliferation but overall changes in tanycyte and precursor numbers which in turn lead to reduced BrdU detected at P1y.
In this study, we explored the novel neural stem cell niche of the adult hypothalamus and analyzed the role of brain 5-HT in food intake and cell genesis. We show that precursor cells in the hypothalamus retain fate plasticity and respond to both intrinsic changes and environmental stimuli.
Ethics Statement
All procedures were approved by LAGeSo (Berlin, Germany) and carried out in accordance with the European Communities Council Directive 2010-63 UE.
Author Contributions
MvL and FK designed the research, analyzed data and wrote the manuscript. MvL, MS, and FK performed experiments. NA bred and provided Tph2-/- animals.
Funding
This work was supported by Rahel Hirsch Fellowship (Charité Berlin) to FK, Berlin Institute of Health (BIH) Gender Equality Fund to MvL and FK, 5-100 Russian Academic Excellence Project funding to MS and FK, and funding by the German Federal Ministry of Education and Research under the framework of the EU-ERA-NET NEURON project RESPOND to NA.
Conflict of Interest Statement
The authors declare that the research was conducted in the absence of any commercial or financial relationships that could be construed as a potential conflict of interest.
References
Alenina, N., Kikic, D., Todiras, M., Mosienko, V., Qadri, F., Plehm, R., et al. (2009). Growth retardation and altered autonomic control in mice lacking brain serotonin. Proc. Natl. Acad. Sci. U.S.A. 106, 10332–10337. doi: 10.1073/pnas.0810793106
Alenina, N., and Klempin, F. (2015). The role of serotonin in adult hippocampal neurogenesis. Behav. Brain Res. 277, 49–57. doi: 10.1016/j.bbr.2014.07.038
Bolborea, M., and Dale, N. (2013). Hypothalamic tanycytes: potential roles in the control of feeding and energy balance. Trends Neurosci. 36, 91–100. doi: 10.1016/j.tins.2012.12.008
Chaker, Z., George, C., Petrovska, M., Caron, J. B., Lacube, P., Caille, I., et al. (2016). Hypothalamic neurogenesis persists in the aging brain and is controlled by energy-sensing IGF-I pathway. Neurobiol. Aging 41, 64–72. doi: 10.1016/j.neurobiolaging.2016.02.008
D’Agostino, G., Lyons, D., Cristiano, C., Lettieri, M., Olarte-Sanchez, C., Burke, L. K., et al. (2018). Nucleus of the Solitary Tract Serotonin 5-HT2C Receptors Modulate Food Intake. Cell Metabol. 28:619-630.e615. doi: 10.1016/j.cmet.2018.07.017
Donovan, M. H., and Tecott, L. H. (2013). Serotonin and the regulation of mammalian energy balance. Front. Neurosci. 7:36. doi: 10.3389/fnins.2013.00036
Haan, N., Goodman, T., Najdi-Samiei, A., Stratford, C. M., Rice, R., El Agha, E., et al. (2013). Fgf10-expressing tanycytes add new neurons to the appetite/energy-balance regulating centers of the postnatal and adult hypothalamus. J. Neurosci. 33, 6170–6180. doi: 10.1523/JNEUROSCI.2437-12.2013
Klein, C., Jonas, W., Wiedmer, P., Schreyer, S., Akyuz, L., Spranger, J., et al. (2019). High-fat diet and physical exercise differentially modulate adult neurogenesis in the mouse hypothalamus. Neuroscience 400, 146–156. doi: 10.1016/j.neuroscience.2018.12.037
Klempin, F., Beis, D., Mosienko, V., Kempermann, G., Bader, M., and Alenina, N. (2013). Serotonin is required for exercise-induced adult hippocampal neurogenesis. J. Neurosci. 33, 8270–8275. doi: 10.1523/JNEUROSCI.5855-12.2013
Kokoeva, M. V., Yin, H., and Flier, J. S. (2005). Neurogenesis in the hypothalamus of adult mice: potential role in energy balance. Science 310, 679–683.
Lam, D. D., Przydzial, M. J., Ridley, S. H., Yeo, G. S., Rochford, J. J., O’Rahilly, S., et al. (2008). Serotonin 5-HT2C receptor agonist promotes hypophagia via downstream activation of melanocortin 4 receptors. Endocrinology 149, 1323–1328.
Lee, D. A., Bedont, J. L., Pak, T., Wang, H., Song, J., Miranda-Angulo, A., et al. (2012). Tanycytes of the hypothalamic median eminence form a diet-responsive neurogenic niche. Nat. Neurosci. 15, 700–702. doi: 10.1038/nn.3079
Lee, D. A., Yoo, S., Pak, T., Salvatierra, J., Velarde, E., Aja, S., et al. (2014). Dietary and sex-specific factors regulate hypothalamic neurogenesis in young adult mice. Front. Neurosci. 8:157. doi: 10.3389/fnins.2014.00157
Leibowitz, S. F., and Alexander, J. T. (1998). Hypothalamic serotonin in control of eating behavior, meal size, and body weight. Biol. Psychiatry 44, 851–864.
Mosienko, V., Bert, B., Beis, D., Matthes, S., Fink, H., Bader, M., et al. (2012). Exaggerated aggression and decreased anxiety in mice deficient in brain serotonin. Transl. Psychiatry 2:e122. doi: 10.1038/tp.2012.44
Nectow, A. R., Schneeberger, M., Zhang, H., Field, B. C., Renier, N., Azevedo, E., et al. (2017). Identification of a Brainstem Circuit Controlling Feeding. Cell 170:429-442.e411. doi: 10.1016/j.cell.2017.06.045
Nigro, S. C., Luon, D., and Baker, W. L. (2013). Lorcaserin: a novel serotonin 2C agonist for the treatment of obesity. Curr. Med. Res. Opin. 29, 839–848. doi: 10.1185/03007995.2013.794776
Pierce, A. A., and Xu, A. W. (2010). De novo neurogenesis in adult hypothalamus as a compensatory mechanism to regulate energy balance. J. Neurosci. 30, 723–730. doi: 10.1523/JNEUROSCI.2479-09.2010
Robins, S. C., Stewart, I., McNay, D. E., Taylor, V., Giachino, C., Goetz, M., et al. (2013a). alpha-Tanycytes of the adult hypothalamic third ventricle include distinct populations of FGF-responsive neural progenitors. Nat. Commun. 4:2049. doi: 10.1038/ncomms3049
Robins, S. C., Trudel, E., Rotondi, O., Liu, X., Djogo, T., Kryzskaya, D., et al. (2013b). Evidence for NG2-glia derived, adult-born functional neurons in the hypothalamus. PLoS One 8:e78236. doi: 10.1371/journal.pone.0078236
Schwartz, C., Hampton, M., and Andrews, M. T. (2015). Hypothalamic gene expression underlying pre-hibernation satiety. Genes Brain Behav. 14, 310–318. doi: 10.1111/gbb.12199
Keywords: hypothalamus, BrdU, 5-HT, Tph2, NG2, Western-type diet
Citation: van Lingen M, Sidorova M, Alenina N and Klempin F (2019) Lack of Brain Serotonin Affects Feeding and Differentiation of Newborn Cells in the Adult Hypothalamus. Front. Cell Dev. Biol. 7:65. doi: 10.3389/fcell.2019.00065
Received: 11 December 2018; Accepted: 09 April 2019;
Published: 26 April 2019.
Edited by:
Aixa Victoria Morales, Cajal Institute (CSIC), SpainReviewed by:
Erica R. Glasper, University of Maryland, United StatesSilvina Laura Diaz, National Scientific and Technical Research Council (CONICET), Argentina
Copyright © 2019 van Lingen, Sidorova, Alenina and Klempin. This is an open-access article distributed under the terms of the Creative Commons Attribution License (CC BY). The use, distribution or reproduction in other forums is permitted, provided the original author(s) and the copyright owner(s) are credited and that the original publication in this journal is cited, in accordance with accepted academic practice. No use, distribution or reproduction is permitted which does not comply with these terms.
*Correspondence: Friederike Klempin, ZnJpZWRlcmlrZS5rbGVtcGluQG1kYy1iZXJsaW4uZGU=