- Department of Cell Biology, Albert Einstein College of Medicine, New York, NY, United States
Glycosylation of proteins by N- and O-glycans or glycosaminoglycans (GAGs) mostly begins in the endoplasmic reticulum and is further orchestrated in the Golgi compartment via the action of >100 glycosyltransferases that reside in this complex organelle. The synthesis of glycolipids occurs in the Golgi, also by resident glycosyltransferases. A defect in the glycosylation machinery may impair the functions of glycoproteins and other glycosylated molecules, and lead to a congenital disorder of glycosylation (CDG). Spermatogenesis in the male and oogenesis in the female are tightly regulated differentiation events leading to the production of functional gametes. Insights into roles for glycans in gamete production have been obtained from mutant mice following deletion or inactivation of genes that encode a glycosylation activity. In this review, we will summarize the effects of altering the synthesis of N-glycans, O-glycans, proteoglycans, glycophosphatidylinositol (GPI) anchored proteins, and glycolipids during gametogenesis in the mouse. Glycosylation genes whose deletion causes embryonic lethality have been investigated following conditional deletion using various Cre recombinase transgenes with a cell-type specific promoter. The potential effects of mutations in corresponding glycosylation genes of humans will be discussed in relation to consequences to fertility and potential for use in contraception.
Introduction
The mammalian glycome is defined by the genes that encode activities required for the synthesis of glycosylated proteins and lipids. These include genes that encode glycosyltransferases, nucleotide sugar synthases, nucleotide sugar modifiers, nucleotide sugar transporters and glycosidases required to prune glycans during synthesis. Also required for optimal glycosylation are proteins that maintain the structure and environment of the secretory pathway. A summary of the classes of glycan synthesized by mammals is given in Figure 1. The actual complement of glycan structures expressed in the endoplasmic reticulum (ER), in Golgi compartments, at the cell surface, or secreted by a mammalian cell will depend on the glycosylation-related genes that are active in that cell, and that spectrum will likely vary at different stages of development or differentiation. With few exceptions, all glycoproteins, proteoglycans and glycolipids are synthesized in the secretory pathway, although N-glycans and glycosylphosphatidylinositol (GPI) anchors begin their synthesis on the cytoplasmic side of the ER membrane, before an immature glycan is flipped to the luminal side. Maturation continues in the ER and Golgi compartments. Thus, effects on the structure or biochemical environment of the ER and Golgi compartments may affect glycosylation and alter the nature of the glycans produced. The glycans on mature glycoproteins and glycolipids expressed at the cell surface or secreted from a cell are the functional glycans involved in recognition by glycan binding proteins on other cells, and in the extracellular matrices of a tissue. Glycoprotein exceptions to synthesis in the secretory pathway are proteins modified on Ser or Thr by O-GlcNAc in the cytoplasm or nucleus (Zachara et al., 2015), glycogen that is also synthesized in the cytoplasm, and hyaluronan synthesized at the plasma membrane and extruded to the extracellular matrix (Vasconcelos et al., 2013).
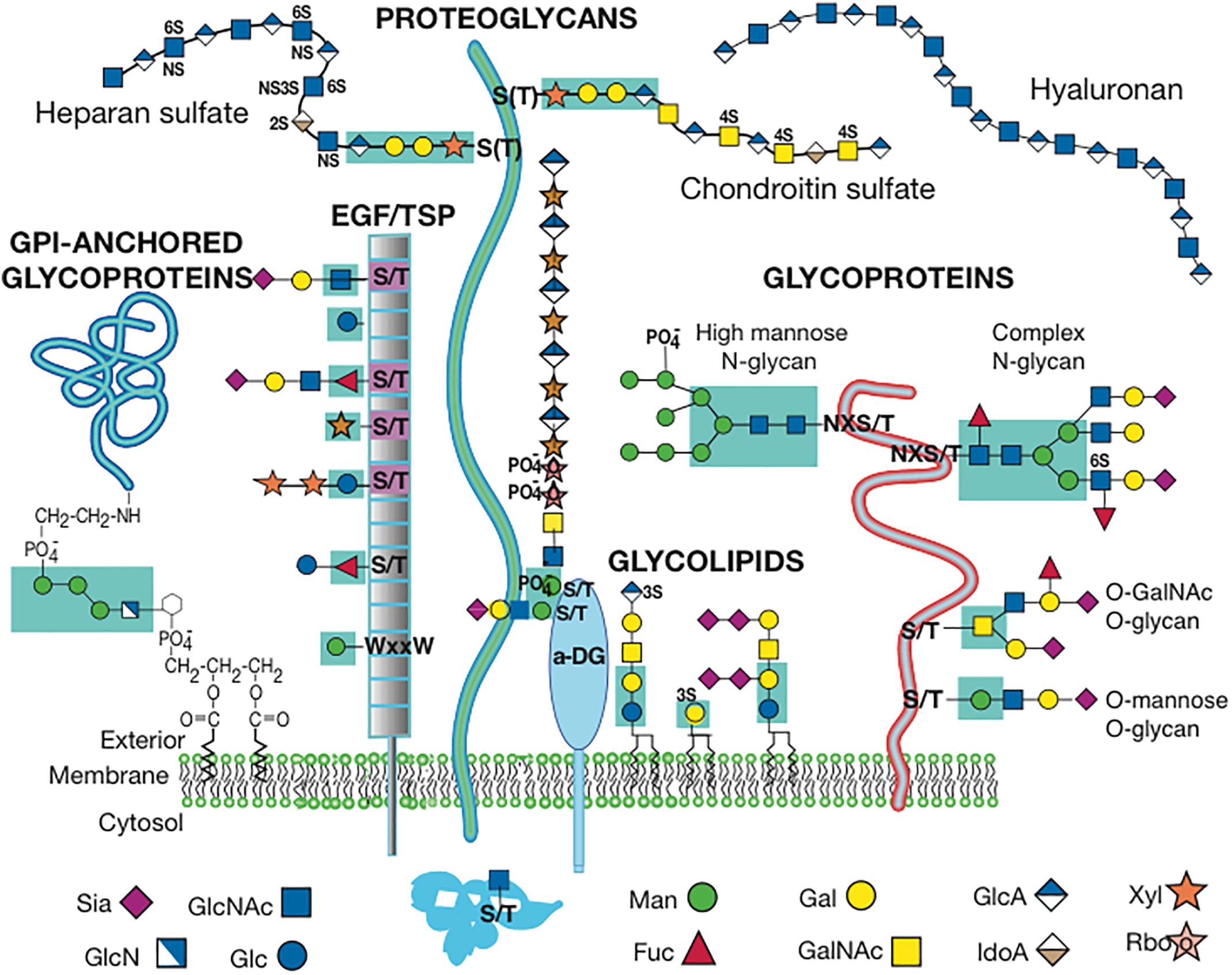
Figure 1. Cell Surface Glycans in Mammals. The diagram depicts one or more glycans from each class of mammalian glycan. The diagram is modified from Figure in Stanley (2016) with permission. Sugar symbols are according to the Symbol Nomenclature for Glycans (Varki et al., 2015).
A commonly used strategy to determine if a particular glycan or sugar residue is necessary for the development or differentiation of a specific cell type is to inactivate or delete a glycosylation gene. However, this has the drawback that many glycosylated molecules of different functions will be altered. To address functions for glycans in individual glycoproteins, one or more known glycosylation sites can be mutated to preclude their modification. However, relatively few glycans are added at a known amino acid, making this approach arduous, although useful once glycosylation sites have been established. Usually it is important not to eliminate glycosylation of a glycoprotein altogether since this often leads to defective folding, aggregation and degradation and/or inability to exit the ER.
This review will describe the consequences for oogenesis (Figure 2) and spermatogenesis (Figure 3) of altering glycan synthesis by targeted inactivation of glycosylation genes responsible for the synthesis of Golgi glycans in the mouse. These glycans are identified in Figures 1, 4. Until recently, glycans in the ovary and testis have been investigated by immunohistochemistry and other histochemical assays and by using glycan binding proteins such as plant lectins (Lee and Damjanov, 1984; Wu et al., 1984; Lohr et al., 2010). However, the application of matrix-assisted laser desorption mass spectrometry imaging (MALDI-MSI) has begun to reveal the molecular nature of glycans along with their location in complex tissues (Drake et al., 2018).
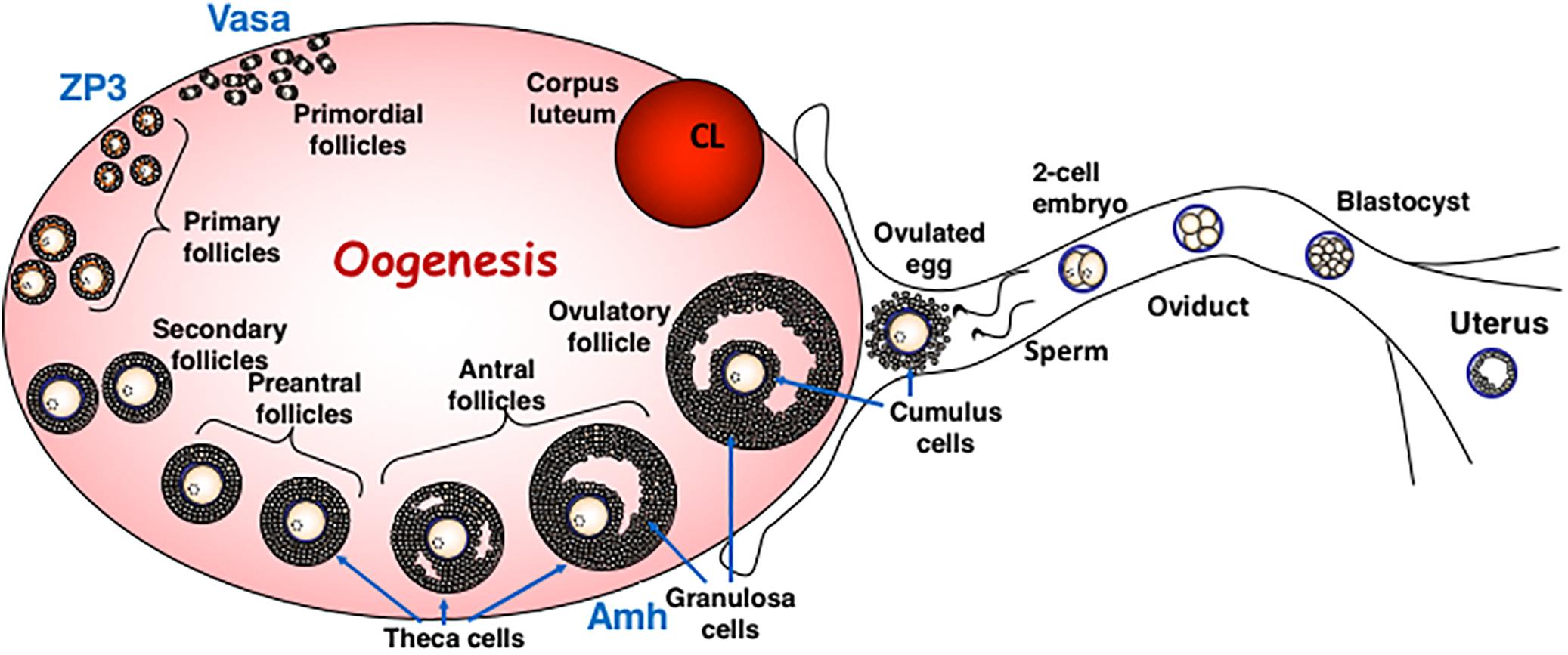
Figure 2. Oogenesis in Mammals. The diagram describes the different stages of oogenesis and identifies the follicular stage in which the ZP3 and Vasa promoters are active in oocytes. Both these promoters are used for oocyte-specific deletion by Cre recombinase. The diagram is modified from (Zhao and Dean, 2002) with permission.
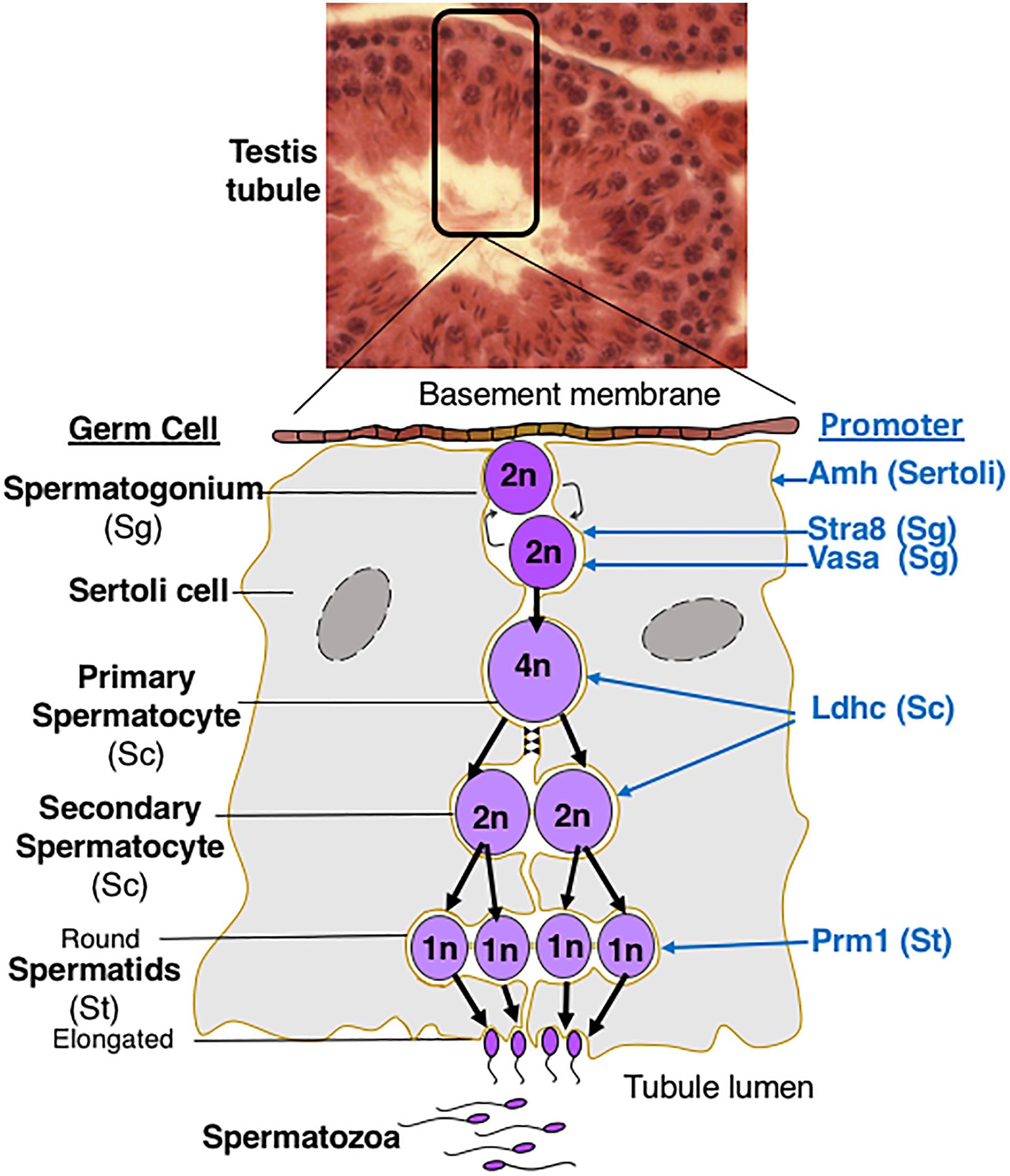
Figure 3. Spermatogenesis in Mammals. The diagram describes germ cell differentiation during spermatogenesis and identifies the germ cell type in which different promoters are active. 2n diploid; 4n, tetraploid; 1n haploid.
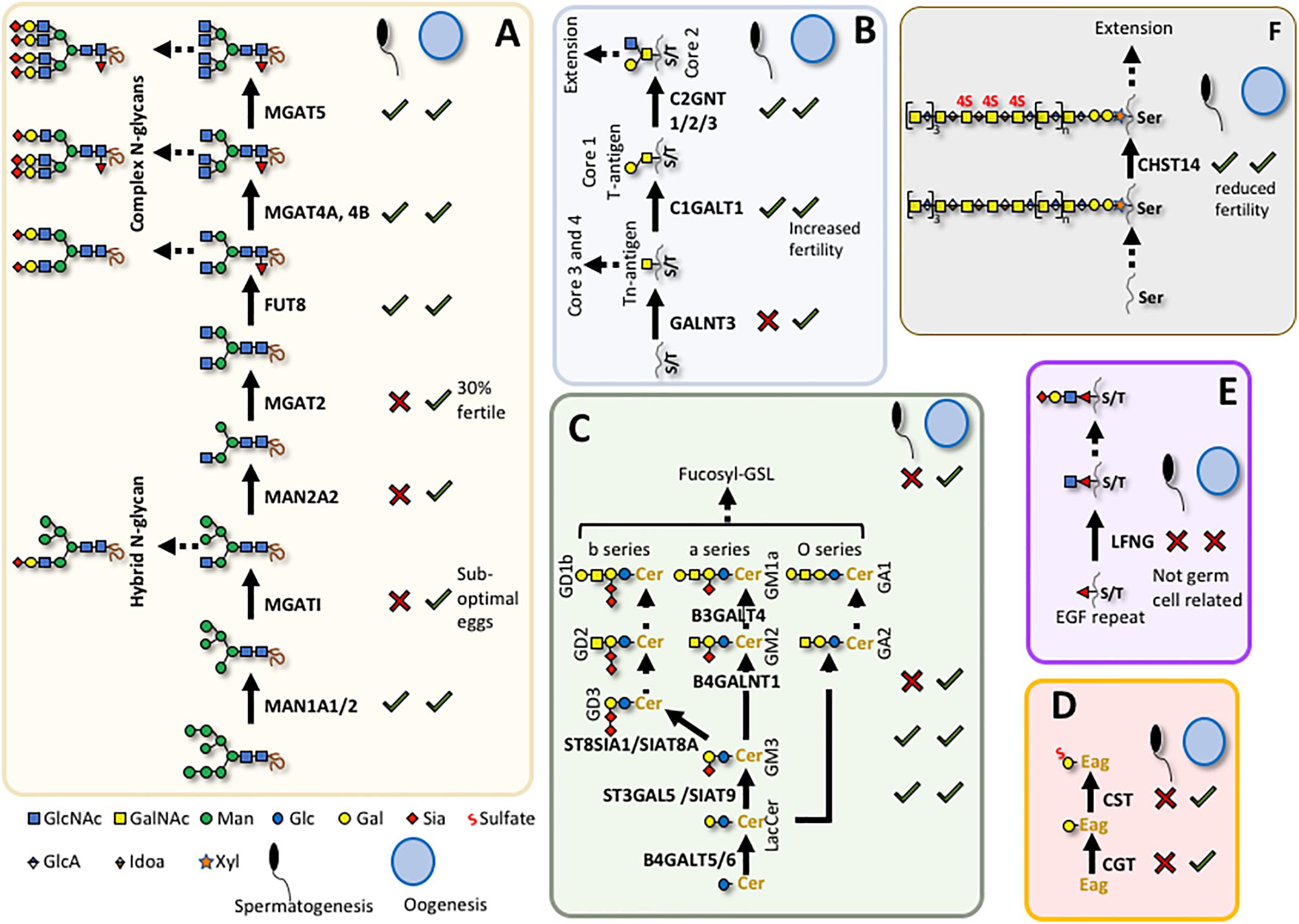
Figure 4. Glycans required for spermatogenesis or oogenesis. Summary of known roles for individual glycans in mouse gametogenesis (see main text). (A) N-glycan maturation; (B) O-GalNAc core 1 and core 2 glycan synthesis; (C) Glycolipid synthesis; (D) Seminolipid synthesis; (E) O-Fucose glycan synthesis; (F) Chondroitin sulfate synthesis. X indicates a defect in male or female gametogenesis and √ indicates no known defect when related gene is deleted; Cer, ceramide; Eag, alkylacylglycerol. Sugar symbols are according to the Symbol Nomenclature for Glycans (Varki et al., 2015).
Golgi Glycans Important for Oogenesis in Mammals
Deletion or inactivation of glycosyltransferases that function in the Golgi compartment may have profound or relatively mild effects (Stanley, 2016). For example, inactivation of the Mgat1 gene which blocks synthesis of all complex and hybrid N-glycans, leads to embryonic lethality (Ioffe and Stanley, 1994; Metzler et al., 1994). By contrast, inactivation of Mgat5, which prevents the addition of a single β1,6 branch of complex N-glycans, has much milder consequences (Dennis et al., 2002; Partridge et al., 2004). Conditional deletion of Mgat1 in oocytes using the ZP3-Cre transgene (Lewandoski et al., 1997) was used to identify roles for complex and hybrid N-glycans in differentiation of oocytes from the primordial follicle stage (Figure 2). Loss of MGAT1 and hybrid and complex N-glycans allowed progression of mutant oocytes to ovulation and fertilization (Shi et al., 2004). Heterozygous embryos from mutant egg and wild type sperm give rise to embryos that develop normally. Mgat1 null oocytes fertilized by Mgat1 null sperm also give Mgat1[-/-] embryos that develop to ∼E9.5 as observed in a global knockout (Ioffe and Stanley, 1994; Metzler et al., 1994). However, closer examination revealed that females with Mgat1 null oocytes have ∼25% more empty deciduae than control females, and the zona pellucida of mutant oocytes is very thin. Zona pellucida (ZP) glycoproteins lacked complex N-glycans showing that deletion of Mgat1 was efficient. While all embryos from Mgat1 null oocytes implant, not all continue to develop, suggesting that a proportion of oocytes do not give rise to fully functional embryos. Defective oogenesis became more apparent when females were stimulated to ovulate by hormonal treatment. Females producing Mgat1 null oocytes gave ∼50% fewer ovulated eggs, even though they generated equivalent numbers of antral follicles to controls. In addition, embryos arising from Mgat1 null eggs were slow in developing through the early stages of embryogenesis prior to implantation. Thus, MGAT1 and complex N-glycans (Figure 4A) are required for the development of fully competent oocytes and ovulated eggs. In addition, these experiments clearly demonstrated that complex or hybrid N-glycans are not required for sperm binding or fertilization. Further investigations revealed that oocytes lacking MGAT1 have aberrant development of preovulatory follicles (retarded folliculogenesis), and structural alterations of the cumulus in cumulus-oocyte complexes (COC; Williams and Stanley, 2009). The reason for sub-optimal development of oocytes that lack MGAT1 may be related to a reduction in growth factor signaling since complex N-glycans regulate the retention of growth factor receptors at the cell surface, and thereby regulate signaling duration (Boscher et al., 2011).
Whole body deletion of Man2a1 which encodes an alpha-mannosidase II that acts after MGAT1 to prepare the substrate of MGAT2 (Figure 4A), does not result in obvious defects in female fertility (Chui et al., 1997). Global deletion of both Man2a1 and Man2a2 (previously called alpha-mannosidase IIX) causes embryonic or perinatal lethality (Akama et al., 2006) and oogenesis in conditional mutants has not been examined. Mgat2 null mice also die perinatally (Ye and Marth, 2004) in an inbred mouse model, and only 30% of the surviving females in an outbred mouse background were fertile. However, no further description of the basis for the fertility defect, and no targeted deletion in oocytes have been reported. The sugar added after GlcNAc to complex N-glycans in the trans Golgi compartment is Gal (Figure 4A). Deletion of B4galt1 also causes perinatal lethality in one mouse strain (Lu et al., 1997) but not in another (Asano et al., 1997). Females lacking B4GALT1 were fertile but had a slight reduction in litter size when compared to wild type (Asano et al., 1997).
The deletion of another Golgi glycosyltransferase by ZP3-Cre that affects oogenesis gives rise to a phenotype distinct from the loss of Mgat1. The glycosyltransferase C1GALT1 (also known as T synthase) transfers Gal to GalNAc attached at Ser/Thr residues, and initiates the synthesis of core 1 and 2 O-GalNAc glycans in the Golgi (Figures 1, 4B). Deletion of C1galt1 is embryonic lethal at ∼E13.5 (Xia et al., 2004). Conditional deletion by ZP3-Cre in primary follicles (Figure 2) is efficient and strikingly gives rise to an increase in the number of eggs and pups produced by females that lack C1GALT1 in oocytes (Williams and Stanley, 2008). The increase of ∼30–50% in ovulated eggs leads to sustained increases in litter size. While follicular development is enhanced, there is no increase in apoptosis of mutant oocytes (Williams and Stanley, 2008). In addition, oocytes lacking C1GALT1 generate more multiple oocyte follicles (MOF) at late stages of folliculogenesis. The formation of MOFs may be due to the compromised follicular basal lamina apparent in follicles containing C1galt1 null oocytes (Christensen et al., 2015; Grasa et al., 2016). In fact, robust fusion of mutant follicles is observed in in vitro cultures containing follicles with C1galt1 mutant oocytes, whereas no MOF arose in cultured control oocytes (Christensen et al., 2017). The enhanced folliculogenesis and fertility in females with oocytes lacking C1GALT1 reflects the enhanced sensitivity of follicles containing mutant oocytes to stimulation by follicle stimulating hormone (FSH), reduced apoptosis and altered BMP15-GDF9 signaling (Grasa et al., 2015).
Interestingly, when both Mgat1 and C1galt1 were deleted by ZP3-Cre, the results were significantly worse for folliculogenesis, ovulation and fertility than deletion of either one alone (Williams and Stanley, 2011). Removing N-glycans and core 1 and 2 O-GalNAc glycans together abolishes the high fertility phenotype observed in C1galt1 mutant females and worsens the folliculogenesis defects observed in Mgat1 null mice, resulting in an overall reduction in egg production and fertility. Nevertheless, eggs lacking both complex and hybrid N-glycans as well as core 1 and 2 O-glycans (and therefore all Gal residues on N- and O-GalNAc glycans) were fertilized, showing that all these glycans are dispensable for fertilization. However, follicle production was greatly reduced, as were the number of ovulated double mutant eggs, and females had, at most, one litter (Williams et al., 2007; Grasa et al., 2012). The extracellular matrix of the cumulus in the cumulus-oocyte complexes (COCs) with double mutant oocytes was also altered (Lo et al., 2018). Ultimately, the combined inactivation of Mgat1 and C1galt1 in oocytes led to premature ovarian failure (POF), a novel model for investigation of this syndrome in human premature ovarian insufficiency (POI; Williams and Stanley, 2011; Grasa et al., 2016). In vitro culture of double mutant follicles has shown that a subset retains the potential to develop to antral follicles in vitro if obtained prior to the development of POF (Kaune et al., 2017). In summary, the generation of complex or hybrid N-glycans as well as core 1 and 2 O-GalNAc glycans in the Golgi is essential for fully functional oogenesis and the production of a complete complement of developmentally-competent eggs and embryos.
Another class of O-glycans is found only on Ser/Thr of epidermal growth factor-like (EGF) repeats at specific consensus sequences (Figure 1; Haltiwanger et al., 2017). Notch receptors and canonical Notch ligands are highly modified by these O-glycans because of the large number of EGF repeats in their extracellular domains. O-fucose, O-glucose and O-GlcNAc residues are transferred to proteins with EGF repeats in the ER by POFUT1, POGLUT1 and EOGT respectively, and further elongated in Golgi compartments (Takeuchi and Haltiwanger, 2014). Deletion of the initiating glycosyltransferase genes is embryonic lethal for O-fucose and O-glucose glycans but not for O-GlcNAc glycans (Varshney and Stanley, 2018). Female mice lacking secretory pathway O-GlcNAc glycans are fertile. Surprisingly, females with ZP3-Cre-mediated deletion of Pofut1 leading to the loss of all O-fucose glycans in oocytes, also have normal fertility, and thus apparently normal oogenesis (Shi et al., 2005), despite proposed roles for Notch signaling in oogenesis (Xu and Gridley, 2013; Feng et al., 2014). By contrast, the global deletion of Lfng, a Golgi GlcNAc-transferase which adds GlcNAc to O-fucose-modified EGF repeats, leads to infertility in female mice due to defective follicular development and meiotic maturation of oocytes (Hahn et al., 2005). Because the loss of O-fucose on EGF repeats has no effect on female fertility, the Lfng null phenotype must not be due to defect(s) in oocytes but in some other cell(s) required for normal oogenesis (Figure 4E). No effects on female fertility were reported in mice lacking the related GlcNAc-transferases Mfng or Rfng (Moran et al., 2009).
Only in a few cases have specific roles in oogenesis and/or female fertility been reported for mice with disrupted synthesis of glycosaminoglycans (GAG), glycosylphosphatidylinositol (GPI) anchors or glycolipids. While female mice lacking dermatan-4-O-sulfotransferase 1 (CHST14) are fertile when crossed with wild type males and Chst14 null males are also fertile when crossed to wild type females, homozygous null females crossed with homozygous null males give no progeny (Akyuz et al., 2013; Figure 4F). GAGs as well as glycolipids and other glycans of glycoproteins are main components of the extracellular matrix and, it is known that the composition and structure of the extracellular matrix is critical for the development of follicles and eggs in the ovary (Nagyova, 2018). The extracellular matrix and COC are significantly compromised in mice with oocytes lacking MGAT1 (Williams and Stanley, 2009) or both MGAT1 and C1GALT1 (Lo et al., 2018). ZP3 promoter-directed conditional deletion of Piga, the enzyme that initiates GPI-anchor synthesis in the endoplasmic reticulum (ER), gives rise to females in which folliculogenesis and egg production appear normal but mutant eggs lacking PIGA and thus GPI-anchored proteins at the cell surface are unable to fuse with sperm (Alfieri et al., 2003). One reason for this may be the absence of the GPI-anchored protein JUNO at the egg membrane. JUNO is essential for sperm binding to the egg via its ligand Izumo1 (Bianchi et al., 2014).
Despite the indications from mouse mutants that fertility would be expected to be affected in women with altered glycosylation, a literature search did not reveal known genetic bases associated with glycosylation genes that correlate with defective oogenesis, fertility or POI in humans. Nor do the results in mice to date suggest that disruption of glycosylation would be an effective female contraceptive.
Golgi Glycans Important for Spermatogenesis in Mammals
Spermatogenesis in mammals is a multi-step differentiation process in which spermatogonia (Sg), spermatocytes (Sc), spermatids (St) and ultimately spermatozoa are generated in close contact with Sertoli cells (Figure 3). The first Golgi glycosylation gene knockout in the mouse to reveal a role for N-glycans in spermatogenesis was global deletion of Man2a2 (Akama et al., 2002; Fukuda and Akama, 2003), an alpha-mannosidase II which removes two mannose residues from the N-glycan product of MGAT1 and generates the substrate for MGAT2 (Figure 4A). The protein is enriched in germ cells except spermatogonia and condensing spermatids, and is not prominent in somatic cells. The N-glycan product of alpha-mannosidase IIx, detected in testis sections using the lectin Griffonia simplicifolia (GSA), was detected on spermatogenic cells (except spermatogonia) but not somatic cells (Sertoli, Leydig), suggesting that MAN2A2 activity is mainly restricted to germ cells in the seminiferous tubule (Akama et al., 2002). Germ cells of the Man2a2 whole body knockout form multi-nuclear cells (MNC or syncytia) and the mice are infertile. Complex N-glycans are absent based on the lack of GSA binding to germ cells. Hybrid N-glycans are expected to be present after deletion of Man2a2 (Figure 4A). Thus, neither hybrid nor oligomannose N-glycans on glycoproteins were able to support spermatogenesis or the production of normal numbers of mature sperm. Testes from the Man2a2 global knockout were smaller, contained fewer spermatids and produced immature sperm. Adhesion of Man2a2 mutant germ cells to Sertoli cells was reduced and a N-glycan terminating in GlcNAc was proposed to be necessary for germ-Sertoli cell adhesion. Global deletion of the other alpha mannosidase II encoded by the Man2a1 gene does not affect viability or male fertility, but whole body knockout of both Man2a1 and Man2a2 is neonatal lethal (Akama et al., 2006; Hato et al., 2006). Unusual hybrid N-glycans observed in tissues from E15.5 Man2a1/Man2a2 double knockout embryos (Hato et al., 2006) were not reported in germ cells lacking MAN2A2 (Akama et al., 2002). Disruption of the Man1a2 gene that encodes an alpha mannosidase involved in the pruning of oligomannose N-glycans, is perinatal lethal (Tremblay et al., 2007), and conditional deletion in germ cells has not been reported.
A marked spermatogenic phenotype is obtained when Mgat1 is specifically deleted in spermatogonia using Stra8-iCre (Batista et al., 2012). In males with germ cells lacking both complex and hybrid N-glycans, testes are small, MNCs are very prominent in testis tubules, apoptosis is increased, and there are no mature sperm in epididymis. Investigation into potential mechanisms of the block in spermatogenesis identified ERK1/2 signaling as markedly reduced in the absence of MGAT1 (Biswas et al., 2018). A physiological inhibitor of MGAT1 termed GnT1IP or MGAT4D, and expressed most highly in male germ cells (Huang and Stanley, 2010; Huang et al., 2015), is being investigated as a potential regulator of complex N-glycan production during spermatogenesis. Whole body disruption of Mgat2 gene expression is lethal in the early days postpartum in inbred mice. On an “outbred” background, the few survivors have spermatogonia and spermatocytes but no round spermatids or mature spermatozoa in the lumen of the seminiferous tubules (Wang et al., 2002). Inactivation of Fut8 encoding the fucosyltransferase responsible for N-glycan core fucosylation, induces early postnatal death (Wang et al., 2005) and no targeted deletion in germ cells has been reported.
The absence of core 1 and 2 O-glycans due to deletion of C1galt1 by Stra8-iCre, has no effect on spermatogenesis (Batista et al., 2012). Interestingly, neither does deletion of Pofut1, and thus the absence of O-fucose glycans does not disrupt spermatogenesis (Batista et al., 2012). Deletion of Pofut1 in Sertoli cells using Amh-Cre also has no deleterious effect on spermatogenesis (Hasegawa et al., 2012). Nor does the absence of NOTCH1 in germ cells (Batista et al., 2012). However, global deletion of the Golgi GlcNAc-transferase encoded by Lfng, which acts after POFUT1, gives rise to a defective rete testis (Hahn et al., 2009). This results in few sperm in the epididymis, although spermatogenesis progresses normally. Since this was not observed in Pofut1 conditional germ cell knockout males, the Lfng knockout rete testis phenotype is probably due to defective development of non-germ cells of the testis. Global deletion of B4galt1 leads to delayed spermatogenesis in the few males that do not die perinatally (Lu et al., 1997). Seminiferous tubules are smaller and spermatids are fewer in B4galt1 null testes. However, fertility and viability are not affected in B4galt1 null males on a different genetic background (Nishie et al., 2007).
In summary, hybrid and complex N-glycans (Figure 4A) are required in germ cells for spermatogenesis to progress to mature sperm but core 1 and core 2 O-GalNAc glycans (Figure 4B) or O-fucose glycans (Figure 4E) are dispensable. Interestingly, however, some GalNAc residues on Ser/Thr, potentially unmodified further or extended to make core 3 or core 4 O-GalNAc glycans (Brockhausen and Stanley, 2017), are required for spermatogenesis. Deletion of a GalNAc transferase termed Galnt3, which is one of approximately 20 polypeptide GalNAc transferases that initiate the synthesis of O-GalNAc glycans in the Golgi, leads to disrupted spermatogenesis and male infertility (Ichikawa et al., 2009; Miyazaki et al., 2013). GALNT3 localizes to early and medial Golgi compartments of spermatids and spermatocytes (Miyazaki et al., 2013). GALNT3 seems to be important for O-GalNAc addition to proteins in testis because its deletion can be easily detected by lectins that bind to GalNAc-O-Ser/Thr. Deletion leads to defects in acrosome formation, increased apoptosis in the seminiferous tubule, reduced production of sperm, and oligoasthenoteratozoospermia (rare and immotile sperm, deformed round head spermatozoa). A related member of the GALNT family termed GALNTL5, which is lacking a portion of the usual GALNT C-terminal domain and has no known GalNAc-transferase activity, is exclusively expressed in testis germ cells. The protein is detected in the cytoplasm of round spermatids, around the acrosome of elongated spermatids, and in the neck region of spermatozoa. Haploinsufficiency causes spermatogenic defects in the mouse due to immotile spermatozoa (Takasaki et al., 2014). Heterozygosity of GALNTL5 also leads to immotile sperm in men (Hagiuda et al., 2019). It will be interesting to know the function of this novel glycosyltransferase-like protein.
Glycolipids synthesized in the Golgi (Figure 4C) are also important in spermatogenesis. This was initially noted in mice lacking GM2/GD2 synthase (B4GALNT1) which synthesize only Glc-ceramide (Glc-Cer), Lactosyl-Cer, GM3 and GD3 Golgi glycolipids (Takamiya et al., 1998). Male mice lacking B4GALNT1 are sterile and do not produce sperm. Spermatogenesis proceeds to the stage of round spermatids but maturing spermatids fuse into MNCs and sperm are not produced. Investigations of Glycosphingolipids (GSL) in wild type testes compared to Galnt1[-/-] testes identified novel fucosylated neutral and monosialylated GSL with very long polyenoic ceramides termed FGSL (Sandhoff et al., 2005). However, the absence of sialylated GSL following deletion of GM3 synthase (ST3GAL5/SIAT9) or GD3 synthase (ST8SIA1) and therefore the loss of sialylated FGSL has no effect on male fertility (Kawai et al., 2001; Yamashita et al., 2003, 2005). Thus, the neutral Golgi FGSL found in germ cells but not Leydig or Sertoli cells (Sandhoff et al., 2005) appear to be essential for spermatogenesis and male fertility. This result suggests that O-series gangliosides are sufficient for male spermatogenesis and sialic acid residues are not necessary on these glycolipids during germ cells maturation (Figure 4C). The loss of seminolipid (HSO3-3-monogalactosylalkylacylglycerol or SO4-Gal-Eag; Figure 4D) by deletion of the galactosyltransferase CGT also disrupts spermatogenesis (Fujimoto et al., 2000). Cgt is expressed in later-stage spermatocytes and deletion does not disrupt the formation of spermatogonia or early spermatocytes. However, mutant males have no spermatids or sperm. Deletion of Cgt precludes the addition of Gal to the precursor of seminolipid (Eag). However, subsequent deletion of the sulfotransferase Cst gives a similar phenotype to deletion of Cgt (Honke et al., 2002) showing that Gal-Eag is not able to fulfill the functions of SO4-Gal-Eag in testis. Cst is expressed in germ cells and germ cells of Cst null males do not proceed through the first meiotic division (Honke et al., 2002; Zhang et al., 2005).
The GPI-anchor on the subset of GPI-anchored proteins in testis is initiated on the ER membrane and additional sugars are added in the Golgi compartment (Ferguson et al., 2017; Figure 1). While numerous GPI-anchored proteins are important in the formation of sperm, effects specific to GPI anchor Golgi glycosyltransferases have not been reported. The ER resident protein PGAP1 (post GPI attachment to proteins 1) is a GPI inositol-deacylase involved in the maturation of the GPI-anchor by removing palmitate from inositol. A whole body deletion of the gene does not affect spermatogenesis but induces a severe reduction in male fertility. The ability of sperm to ascend into the oviduct and to attach to the zona pellucida of the oocyte is severely impaired (Ueda et al., 2007). Inactivation of the Piga gene which blocks the formation of GPI anchors altogether is embryonic lethal, but chimeric mice generated by injecting Piga null ES cells into wild type blastocysts allowed mice with Piga null and wild type cells to be born and spermatogenesis to be investigated (Lin et al., 2000). Chimeric males were sterile. While spermatogenesis was markedly reduced, sperm were made and sperm lacking PIGA were detected in the epididymis. However, Piga null sperm were not transmitted.
In searches for connections between reduced fertility in men and defective glycosylation, only one mutation in a putative glycosyltransferase gene has been identified - GALNTL5 (Hagiuda et al., 2019). However, many more glycosyltransferase defects may ultimately be tied to altered spermatogenesis and fertility in men if results from gene deletions in mouse are a guide. In addition, the mechanisms of defective spermatogenesis in mice vary depending on the glycosylation pathway affected. Glycosyltransferases whose loss appears to spare spermatogonia and spermatocytes but result in fused spermatids and no mature sperm are good candidates for reversible contraception in men. Thus, MGAT1 (Figure 4A) and B4GALNT1 (Figure 4C) make good inhibitor targets for development as male contraceptives.
Conclusion and Future Directions
Although relatively few mice with germ-cell specific deletion or inactivation of a glycosylation gene have been investigated, it is clear from these experiments that different classes of glycans are required for different aspects of oogenesis and spermatogenesis in mammals. Thus, complex N-glycans are necessary for optimal oogenesis and the production of fully functional ovulated eggs, and they are essential for the production of sperm. This is not due to general defects in protein folding or degradation since MGAT1 acts in the medial Golgi, long after glycoproteins have exited the ER. By contrast, core 1 and core 2 O-GalNAc glycans are not required for spermatogenesis but are important for the regulation of oogenesis and female fertility and for protection from POF. O-fucose glycans are dispensable for both oogenesis and spermatogenesis when deleted in germ cells. The requirement for LFNG in both female and male fertility is predicted to be due to its functions in non-germ cells. Complex glycolipids are necessary for spermatogenesis but appear to be dispensable for oogenesis. Glycosaminoglycans have not been sufficiently investigated to know if they may be important in fertility. Inhibiting synthesis of all GPI anchors does not prevent sperm from being produced but mutant sperm are not transmitted. In females, the absence of GPI anchors impairs the ability of oocytes to fuse with sperm. There is only one human mutation in a putative O-GalNAc-transferase, GALNTL5, which is associated with infertility. However, other genetic bases of defective oogenesis, spermatogenesis or fertility are sure to be detected in humans based on results to date in mutant mice. The sensitivity of spermatogenesis to defects in all these pathways clearly indicates the potential to give rise to idiopathic cases of infertility.
Author Contributions
PS and AA wrote the manuscript. PS modified the figures. AA designed Figures 3, 4.
Funding
The writing of this review was supported by funds from grant RO1 105399 to PS from the National Institute of General Medical Sciences. Funding was also provided by grant RO1 36434 to PS from the National Cancer Institute.
Conflict of Interest Statement
The authors declare that the research was conducted in the absence of any commercial or financial relationships that could be construed as a potential conflict of interest.
References
Akama, T. O., Nakagawa, H., Sugihara, K., Narisawa, S., Ohyama, C., Nishimura, S., et al. (2002). Germ cell survival through carbohydrate-mediated interaction with Sertoli cells. Science 295, 124–127. doi: 10.1126/science.1065570
Akama, T. O., Nakagawa, H., Wong, N. K., Sutton-Smith, M., Dell, A., Morris, H. R., et al. (2006). Essential and mutually compensatory roles of {alpha}-mannosidase II and {alpha}-mannosidase IIx in N-glycan processing in vivo in mice. Proc. Natl. Acad. Sci. U.S.A. 103, 8983–8988. doi: 10.1073/pnas.0603248103
Akyuz, N., Rost, S., Mehanna, A., Bian, S., Loers, G., Oezen, I., et al. (2013). Dermatan 4-O-sulfotransferase1 ablation accelerates peripheral nerve regeneration. Exp. Neurol. 247, 517–530. doi: 10.1016/j.expneurol.2013.01.025
Alfieri, J. A., Martin, A. D., Takeda, J., Kondoh, G., Myles, D. G., and Primakoff, P. (2003). Infertility in female mice with an oocyte-specific knockout of GPI-anchored proteins. J. Cell Sci. 116, 2149–2155. doi: 10.1242/jcs.00430
Asano, M., Furukawa, K., Kido, M., Matsumoto, S., Umesaki, Y., Kochibe, N., et al. (1997). Growth retardation and early death of beta-1,4-galactosyltransferase knockout mice with augmented proliferation and abnormal differentiation of epithelial cells. EMBO J. 16, 1850–1857. doi: 10.1093/emboj/16.8.1850
Batista, F., Lu, L., Williams, S. A., and Stanley, P. (2012). Complex N-glycans are essential, but core 1 and 2 mucin O-glycans, O-fucose glycans, and NOTCH1 are dispensable, for mammalian spermatogenesis. Biol. Reprod. 86:179. doi: 10.1095/biolreprod.111.098103
Bianchi, E., Doe, B., Goulding, D., and Wright, G. J. (2014). Juno is the egg Izumo receptor and is essential for mammalian fertilization. Nature 508, 483–487. doi: 10.1038/nature13203
Biswas, B., Batista, F., Sundaram, S., and Stanley, P. (2018). MGAT1 and complex N-Glycans regulate ERK signaling during spermatogenesis. Sci. Rep. 8:2022. doi: 10.1038/s41598-018-20465-3
Boscher, C., Dennis, J. W., and Nabi, I. R. (2011). Glycosylation, galectins and cellular signaling. Curr. Opin. Cell Biol. 23, 383–392. doi: 10.1016/j.ceb.2011.05.001
Brockhausen, I., and Stanley, P. (2017). “O-GalNAc Glycans,” in Essentials of Glycobiology, eds A. Rd, R. D. Varki, J. D. Cummings, P. Esko, G. W. Stanley, M. Hart, et al. (Cold Spring Harbor, NY: Cold Spring Harbor Laboratory Press), 113–123.
Christensen, A. P., Patel, S. H., Grasa, P., Christian, H. C., and Williams, S. A. (2015). Oocyte glycoproteins regulate the form and function of the follicle basal lamina and theca cells. Dev. Biol. 401, 287–298. doi: 10.1016/j.ydbio.2014.12.024
Christensen, A. P., Peyrache, E., Kaune, H., and Williams, S. A. (2017). Formation of multiple-oocyte follicles in culture. In Vitro Cell Dev. Biol. Anim. 53, 791–797. doi: 10.1007/s11626-017-0175-9
Chui, D., Oh-Eda, M., Liao, Y. F., Panneerselvam, K., Lal, A., Marek, K. W., et al. (1997). Alpha-mannosidase-II deficiency results in dyserythropoiesis and unveils an alternate pathway in oligosaccharide biosynthesis. Cell 90, 157–167. doi: 10.1016/s0092-8674(00)80322-0
Dennis, J. W., Pawling, J., Cheung, P., Partridge, E., and Demetriou, M. (2002). UDP-N-acetylglucosamine:alpha-6-D-mannoside beta1,6 N-acetylglucosaminyltransferase V (Mgat5) deficient mice. Biochim. Biophys. Acta 1573, 414–422. doi: 10.1016/s0304-4165(02)00411-7
Drake, R. R., Powers, T. W., Norris-Caneda, K., Mehta, A. S., and Angel, P. M. (2018). In situ imaging of N-Glycans by MALDI imaging mass spectrometry of fresh or formalin-fixed paraffin-embedded tissue. Curr. Protoc. Protein Sci. 94:e68. doi: 10.1002/cpps.68
Feng, Y. M., Liang, G. J., Pan, B., Qin, X. S., Zhang, X. F., Chen, C. L., et al. (2014). Notch pathway regulates female germ cell meiosis progression and early oogenesis events in fetal mouse. Cell Cycle 13, 782–791. doi: 10.4161/cc.27708
Ferguson, M. A. J., Hart, G. W., and Kinoshita, T. (2017). “Glycosylphosphatidylinositol Anchors,” in Essentials of Glycobiology, eds A. Rd, R. D. Varki, J. D. Cummings, P. Esko, G. W. Stanley, M. Hart, et al. (Cold Spring Harbor, NY: Cold Spring Harbor Laboratory Press), 137–150.
Fujimoto, H., Tadano-Aritomi, K., Tokumasu, A., Ito, K., Hikita, T., Suzuki, K., et al. (2000). Requirement of seminolipid in spermatogenesis revealed by UDP-galactose: ceramide galactosyltransferase-deficient mice. J. Biol. Chem. 275, 22623–22626. doi: 10.1074/jbc.c000200200
Fukuda, M. N., and Akama, T. O. (2003). The in vivo role of alpha-mannosidase IIx and its role in processing of N-glycans in spermatogenesis. Cell Mol. Life Sci. 60, 1351–1355. doi: 10.1007/s00018-003-2339-x
Grasa, P., Kaune, H., and Williams, S. A. (2012). Embryos generated from oocytes lacking complex N- and O-glycans have compromised development and implantation. Reproduction 144, 455–465. doi: 10.1530/REP-12-0084
Grasa, P., Ploutarchou, P., and Williams, S. A. (2015). Oocytes lacking O-glycans alter follicle development and increase fertility by increasing follicle FSH sensitivity, decreasing apoptosis, and modifying GDF9:BMP15 expression. FASEB J. 29, 525–539. doi: 10.1096/fj.14-253757
Grasa, P., Sheikh, S., Krzys, N., Millar, K., Janjua, S., Nawaggi, P., et al. (2016). Dysregulation of follicle development in a mouse model of premature ovarian insufficiency. Reproduction 152, 591–601. doi: 10.1530/rep-16-0091
Hagiuda, J., Takasaki, N., Oya, M., Ishikawa, H., and Narimatsu, H. (2019). Mutation of GALNTL5 gene identified in patients diagnosed with asthenozoospermia. Hum. Fertil. doi: 10.1080/14647273.2018.1562239 [Epub ahead of print].
Hahn, K. L., Beres, B., Rowton, M. J., Skinner, M. K., Chang, Y., Rawls, A., et al. (2009). A deficiency of lunatic fringe is associated with cystic dilation of the rete testis. Reproduction 137, 79–93. doi: 10.1530/REP-08-0207
Hahn, K. L., Johnson, J., Beres, B. J., Howard, S., and Wilson-Rawls, J. (2005). Lunatic fringe null female mice are infertile due to defects in meiotic maturation. Development 132, 817–828. doi: 10.1242/dev.01601
Haltiwanger, R. S., Wells, L., Freeze, H. H., and Stanley, P. (2017). “Other Classes of Eukaryotic Glycans,” in Essentials of Glycobiology, eds A. Rd, R. D. Varki, J. D. Cummings, P. Esko, G. W. Stanley, M. Hart, et al. (Cold Spring Harbor, NY: Cold Spring Harbor Laboratory Press), 151–160.
Hasegawa, K., Okamura, Y., and Saga, Y. (2012). Notch signaling in Sertoli cells regulates cyclical gene expression of Hes1 but is dispensable for mouse spermatogenesis. Mol. Cell Biol. 32, 206–215. doi: 10.1128/MCB.06063-11
Hato, M., Nakagawa, H., Kurogochi, M., Akama, T. O., Marth, J. D., Fukuda, M. N., et al. (2006). Unusual N-glycan structures in alpha-mannosidase II/IIx double null embryos identified by a systematic glycomics approach based on two-dimensional LC mapping and matrix-dependent selective fragmentation method in MALDI-TOF/TOF mass spectrometry. Mol. Cell Proteomics 5, 2146–2157. doi: 10.1074/mcp.m600213-mcp200
Honke, K., Hirahara, Y., Dupree, J., Suzuki, K., Popko, B., Fukushima, K., et al. (2002). Paranodal junction formation and spermatogenesis require sulfoglycolipids. Proc. Natl. Acad. Sci. U.S.A. 99, 4227–4232. doi: 10.1073/pnas.032068299
Huang, H. H., Hassinen, A., Sundaram, S., Spiess, A. N., Kellokumpu, S., and Stanley, P. (2015). GnT1IP-L specifically inhibits MGAT1 in the Golgi via its luminal domain. eLife 4. doi: 10.7554/eLife.08916
Huang, H. H., and Stanley, P. (2010). A testis-specific regulator of complex and hybrid N-glycan synthesis. J. Cell Biol. 190, 893–910. doi: 10.1083/jcb.201004102
Ichikawa, S., Sorenson, A. H., Austin, A. M., Mackenzie, D. S., Fritz, T. A., Moh, A., et al. (2009). Ablation of the Galnt3 gene leads to low-circulating intact fibroblast growth factor 23 (Fgf23) concentrations and hyperphosphatemia despite increased Fgf23 expression. Endocrinology 150, 2543–2550. doi: 10.1210/en.2008-0877
Ioffe, E., and Stanley, P. (1994). Mice lacking N-acetylglucosaminyltransferase I activity die at mid-gestation, revealing an essential role for complex or hybrid N-linked carbohydrates. Proc. Natl. Acad. Sci. U.S.A. 91, 728–732. doi: 10.1073/pnas.91.2.728
Kaune, H., Sheikh, S., and Williams, S. A. (2017). Analysis of in vitro follicle development during the onset of premature ovarian insufficiency in a mouse model. Reprod. Fertil. Dev. 29, 1538–1544. doi: 10.1071/RD15524
Kawai, H., Allende, M. L., Wada, R., Kono, M., Sango, K., Deng, C., et al. (2001). Mice expressing only monosialoganglioside GM3 exhibit lethal audiogenic seizures. J. Biol. Chem. 276, 6885–6888. doi: 10.1074/jbc.c000847200
Lee, M. C., and Damjanov, I. (1984). Anatomic distribution of lectin-binding sites in mouse testis and epididymis. Differentiation 27, 74–81. doi: 10.1111/j.1432-0436.1984.tb01410.x
Lewandoski, M., Wassarman, K. M., and Martin, G. R. (1997). Zp3-cre, a transgenic mouse line for the activation or inactivation of loxP-flanked target genes specifically in the female germ line. Curr. Biol. 7, 148–151. doi: 10.1016/s0960-9822(06)00059-5
Lin, S. R., Yu, I. S., Huang, P. H., Tsai, C. W., and Lin, S. W. (2000). Chimaeric mice with disruption of the gene coding for phosphatidylinositol glycan class A (Pig-a) were defective in embryogenesis and spermatogenesis. Br. J. Haematol. 110, 682–693. doi: 10.1046/j.1365-2141.2000.02209.x
Lo, B. K. M., Archibong-Omon, A., Ploutarchou, P., Day, A. J., Milner, C. M., and Williams, S. A. (2018). Oocyte-specific ablation of N- and O-glycans alters cumulus cell signalling and extracellular matrix composition. Reprod. Fertil. Dev. 31, 529–537. doi: 10.1071/RD18209
Lohr, M., Kaltner, H., Schwartz-Albiez, R., Sinowatz, F., and Gabius, H. J. (2010). Towards functional glycomics by lectin histochemistry: strategic probe selection to monitor core and branch-end substitutions and detection of cell-type and regional selectivity in adult mouse testis and epididymis. Anat. Histol. Embryol. 39, 481–493. doi: 10.1111/j.1439-0264.2010.01019.x
Lu, Q., Hasty, P., and Shur, B. D. (1997). Targeted mutation in beta1,4-galactosyltransferase leads to pituitary insufficiency and neonatal lethality. Dev. Biol. 181, 257–267. doi: 10.1006/dbio.1996.8444
Metzler, M., Gertz, A., Sarkar, M., Schachter, H., Schrader, J. W., and Marth, J. D. (1994). Complex asparagine-linked oligosaccharides are required for morphogenic events during post-implantation development. EMBO J. 13, 2056–2065. doi: 10.1002/j.1460-2075.1994.tb06480.x
Miyazaki, T., Mori, M., Yoshida, C. A., Ito, C., Yamatoya, K., Moriishi, T., et al. (2013). Galnt3 deficiency disrupts acrosome formation and leads to oligoasthenoteratozoospermia. Histochem. Cell Biol. 139, 339–354. doi: 10.1007/s00418-012-1031-3
Moran, J. L., Shifley, E. T., Levorse, J. M., Mani, S., Ostmann, K., Perez-Balaguer, A., et al. (2009). Manic fringe is not required for embryonic development, and fringe family members do not exhibit redundant functions in the axial skeleton, limb, or hindbrain. Dev. Dyn. 238, 1803–1812. doi: 10.1002/dvdy.21982
Nagyova, E. (2018). The biological role of hyaluronan-rich oocyte-cumulus extracellular matrix in female reproduction. Int. J. Mol. Sci. 19:283. doi: 10.3390/ijms19010283
Nishie, T., Miyaishi, O., Azuma, H., Kameyama, A., Naruse, C., Hashimoto, N., et al. (2007). Development of immunoglobulin A nephropathy- like disease in beta-1,4-galactosyltransferase-I-deficient mice. Am. J. Pathol. 170, 447–456. doi: 10.2353/ajpath.2007.060559
Partridge, E. A., Le Roy, C., Di Guglielmo, G. M., Pawling, J., Cheung, P., Granovsky, M., et al. (2004). Regulation of cytokine receptors by Golgi N-glycan processing and endocytosis. Science 306, 120–124. doi: 10.1126/science.1102109
Sandhoff, R., Geyer, R., Jennemann, R., Paret, C., Kiss, E., Yamashita, T., et al. (2005). Novel class of glycosphingolipids involved in male fertility. J. Biol. Chem. 280, 27310–27318. doi: 10.1074/jbc.m502775200
Shi, S., Stahl, M., Lu, L., and Stanley, P. (2005). Canonical Notch signaling is dispensable for early cell fate specifications in mammals. Mol. Cell. Biol. 25, 9503–9508. doi: 10.1128/mcb.25.21.9503-9508.2005
Shi, S., Williams, S. A., Seppo, A., Kurniawan, H., Chen, W., Ye, Z., et al. (2004). Inactivation of the Mgat1 gene in oocytes impairs oogenesis, but embryos lacking complex and hybrid N-glycans develop and implant. Mol. Cell. Biol. 24, 9920–9929. doi: 10.1128/mcb.24.22.9920-9929.2004
Stanley, P. (2016). What have we learned from glycosyltransferase knockouts in mice? J. Mol. Biol. 428, 3166–3182. doi: 10.1016/j.jmb.2016.03.025
Takamiya, K., Yamamoto, A., Furukawa, K., Zhao, J., Fukumoto, S., Yamashiro, S., et al. (1998). Complex gangliosides are essential in spermatogenesis of mice: possible roles in the transport of testosterone. Proc. Natl. Acad. Sci. U.S.A. 95, 12147–12152. doi: 10.1073/pnas.95.21.12147
Takasaki, N., Tachibana, K., Ogasawara, S., Matsuzaki, H., Hagiuda, J., Ishikawa, H., et al. (2014). A heterozygous mutation of GALNTL5 affects male infertility with impairment of sperm motility. Proc. Natl. Acad. Sci. U.S.A. 111, 1120–1125. doi: 10.1073/pnas.1310777111
Takeuchi, H., and Haltiwanger, R. S. (2014). Significance of glycosylation in Notch signaling. Biochem. Biophys. Res. Commun. 453, 235–242. doi: 10.1016/j.bbrc.2014.05.115
Tremblay, L. O., Nagy Kovacs, E., Daniels, E., Wong, N. K., Sutton-Smith, M., Morris, H. R., et al. (2007). Respiratory distress and neonatal lethality in mice lacking Golgi alpha1,2-mannosidase IB involved in N-glycan maturation. J. Biol. Chem. 282, 2558–2566. doi: 10.1074/jbc.m608661200
Ueda, Y., Yamaguchi, R., Ikawa, M., Okabe, M., Morii, E., Maeda, Y., et al. (2007). PGAP1 knock-out mice show otocephaly and male infertility. J. Biol. Chem. 282, 30373–30380. doi: 10.1074/jbc.m705601200
Varki, A., Cummings, R. D., Aebi, M., Packer, N. H., Seeberger, P. H., Esko, J. D., et al. (2015). Symbol nomenclature for graphical representations of glycans. Glycobiology 25, 1323–1324.
Varshney, S., and Stanley, P. (2018). Multiple roles for O-glycans in Notch signalling. FEBS Lett. 592, 3819–3834. doi: 10.1002/1873-3468.13251
Vasconcelos, G. L., Saraiva, M. V., Costa, J. J., Passos, M. J., Silva, A. W., Rossi, R. O., et al. (2013). Effects of growth differentiation factor-9 and FSH on in vitro development, viability and mRNA expression in bovine preantral follicles. Reprod. Fertil. Dev. 25, 1194–1203. doi: 10.1071/RD12173
Wang, X., Inoue, S., Gu, J., Miyoshi, E., Noda, K., Li, W., et al. (2005). Dysregulation of TGF-β1 receptor activation leads to abnormal lung development and emphysema-like phenotype in core fucose-deficient mice. Proc. Natl. Acad. Sci. U.S.A. 102, 15791–15796. doi: 10.1073/pnas.0507375102
Wang, Y., Schachter, H., and Marth, J. D. (2002). Mice with a homozygous deletion of the Mgat2 gene encoding UDP-N-acetylglucosamine:ααα-6-d-mannoside β1,2-N-acetylglucosaminyltransferase II: a model for congenital disorder of glycosylation type IIa. Biochim. Biophys. Acta 1573, 301–311. doi: 10.1016/s0304-4165(02)00397-5
Williams, S. A., and Stanley, P. (2008). Mouse fertility is enhanced by oocyte-specific loss of core 1-derived O-glycans. FASEB J. 22, 2273–2284. doi: 10.1096/fj.07-101709
Williams, S. A., and Stanley, P. (2009). Oocyte-specific deletion of complex and hybrid N-glycans leads to defects in preovulatory follicle and cumulus mass development. Reproduction 137, 321–331. doi: 10.1530/REP-07-0469
Williams, S. A., and Stanley, P. (2011). Premature ovarian failure in mice with oocytes lacking core 1-derived O-glycans and complex N-glycans. Endocrinology 152, 1057–1066. doi: 10.1210/en.2010-0917
Williams, S. A., Xia, L., Cummings, R. D., Mcever, R. P., and Stanley, P. (2007). Fertilization in mouse does not require terminal galactose or N-acetylglucosamine on the zona pellucida glycans. J. Cell Sci. 120, 1341–1349. doi: 10.1242/jcs.004291
Wu, T. C., Lee, M. C., Wan, Y. J., and Damjanov, I. (1984). Lectin binding sites of the mouse ovary, intraovarian and ovulated ova. Histochemistry 80, 527–533. doi: 10.1007/bf02400967
Xia, L., Ju, T., Westmuckett, A., An, G., Ivanciu, L., Mcdaniel, J. M., et al. (2004). Defective angiogenesis and fatal embryonic hemorrhage in mice lacking core 1-derived O-glycans. J. Cell Biol. 164, 451–459. doi: 10.1083/jcb.200311112
Xu, J., and Gridley, T. (2013). Notch2 is required in somatic cells for breakdown of ovarian germ-cell nests and formation of primordial follicles. BMC Biol. 11:13. doi: 10.1186/1741-7007-11-13
Yamashita, T., Hashiramoto, A., Haluzik, M., Mizukami, H., Beck, S., Norton, A., et al. (2003). Enhanced insulin sensitivity in mice lacking ganglioside GM3. Proc. Natl. Acad. Sci. U.S.A. 100, 3445–3449. doi: 10.1073/pnas.0635898100
Yamashita, T., Wu, Y.-P., Sandhoff, R., Werth, N., Mizukami, H., Ellis, J. M., et al. (2005). Interruption of ganglioside synthesis produces central nervous system degeneration and altered axon-glial interactions. Proc. Natl. Acad. Sci. U.S.A. 102, 2725–2730. doi: 10.1073/pnas.0407785102
Ye, Z., and Marth, J. D. (2004). N-glycan branching requirement in neuronal and postnatal viability. Glycobiology 14, 547–558. doi: 10.1093/glycob/cwh069
Zachara, N., Akimoto, Y., and Hart, G. W. (2015). “The O-GlcNAc Modification,” in Essentials of Glycobiology, eds A. Rd, R. D. Varki, J. D. Cummings, P. Esko, G. W. Stanley, M. Hart, et al. (Cold Spring Harbor, NY: Cold Spring Harbor Laboratory Press), 239–251.
Zhang, Y., Hayashi, Y., Cheng, X., Watanabe, T., Wang, X., Taniguchi, N., et al. (2005). Testis-specific sulfoglycolipid, seminolipid, is essential for germ cell function in spermatogenesis. Glycobiology 15, 649–654. doi: 10.1093/glycob/cwi043
Keywords: glycosylation, glycans, Golgi, spermatogenesis, oogenesis, fertility
Citation: Akintayo A and Stanley P (2019) Roles for Golgi Glycans in Oogenesis and Spermatogenesis. Front. Cell Dev. Biol. 7:98. doi: 10.3389/fcell.2019.00098
Received: 17 March 2019; Accepted: 21 May 2019;
Published: 07 June 2019.
Edited by:
Vladimir Lupashin, University of Arkansas for Medical Sciences, United StatesReviewed by:
Taroh Kinoshita, Osaka University, JapanMichael Tiemeyer, University of Georgia, United States
Copyright © 2019 Akintayo and Stanley. This is an open-access article distributed under the terms of the Creative Commons Attribution License (CC BY). The use, distribution or reproduction in other forums is permitted, provided the original author(s) and the copyright owner(s) are credited and that the original publication in this journal is cited, in accordance with accepted academic practice. No use, distribution or reproduction is permitted which does not comply with these terms.
*Correspondence: Pamela Stanley, cGFtZWxhLnN0YW5sZXlAZWluc3RlaW4ueXUuZWR1