- State Key Laboratory of Respiratory Disease, Guangzhou Institute of Respiratory Health, The First Affiliated Hospital, Guangzhou Medical University, Guangzhou, China
The harm of fine particulate matter (PM2.5) to public health is the focus of attention around the world. The Global Burden of Disease (GBD) Study 2015 (GBD 2015 Risk Factors Collaborators, 2016) ranked PM2.5 as the fifth leading risk factor for death, which caused 4.2 million deaths and 103.1 million disability-adjusted life-years (DALYs) loss, representing 7.6% of total global deaths and 4.2% of global DALYs. Epidemiological studies have confirmed that exposure to PM2.5 increases the incidence and mortality of respiratory infections. The host defense dysfunction caused by PM2.5 exposure may be the key to the susceptibility of respiratory system infection. Thus, this review aims to assess the impact of PM2.5 on the host defense of respiratory system. Firstly, we elaborated the epidemiological evidence that exposure to PM2.5 increases the risk of respiratory infections. Secondly, we summarized the experimental evidence that PM2.5 exposure increases the susceptibility of different pathogens (including bacteria and viruses) in respiratory system. Furthermore, here we discussed the underlying host defense mechanisms by which PM2.5 exposure increases the risk of respiratory infections as well as future perspectives.
Introduction
Exposure to air pollution, including gaseous pollution and particulate matter (PM) pollution, is a leading contributor to the Global Burden of Disease (GBD 2015 Risk Factors Collaborators, 2016). In recent years, more and more attention has been paid to the impact of PM pollution on public health. PM is a complex mixture of solids and liquids suspended in the air, which can be classified by its aerodynamic diameter as PM10 (<10 μm, inhalable particulate matter), PM2.5 (<2.5 μm, fine particulate matter) and PM0.1 (<0.1 μm, ultrafine particulate matter). PM originates from natural sources (such as dust, sea salt, and wildfires) and anthropogenic emissions (such as vehicles, household wood and coal burning as well as power plants and industry), and the latter accounts for most of the PM pollution (Cho et al., 2018). The components of PM are extremely complex, including inorganic components (such as heavy and transition metals, elemental carbon, and sulfuric/nitric/ammonia salts), organic components (such as polycyclic aromatic hydrocarbons) and biological components (such as fungi, spores, and viruses) (Zhang et al., 2015; Cho et al., 2018). There are certain differences in the source and composition of different types of particulate matter, and the harm to public health varies as well (Figure 1). Among them, PM2.5 was considered to be the most harmful one. PM2.5 has a large surface area and can adsorb a variety of toxic and harmful substances (Hsu et al., 2016). Because of its small particle size, it can penetrate deep into the lungs and deposit in the terminal bronchioles and alveoli with breath, and even enter the circulatory system through the gas-blood barrier (Pinkerton et al., 2000; Schulze et al., 2017). Exposure to PM2.5 can endanger multiple organs in the body, and even lead to systemic adverse effects (Chauhan and Johnston, 2003). Among them, the most common are the respiratory and cardiovascular systems (Xing et al., 2016). This review focuses on the respiratory system, the primary target organ for PM2.5 exposure.
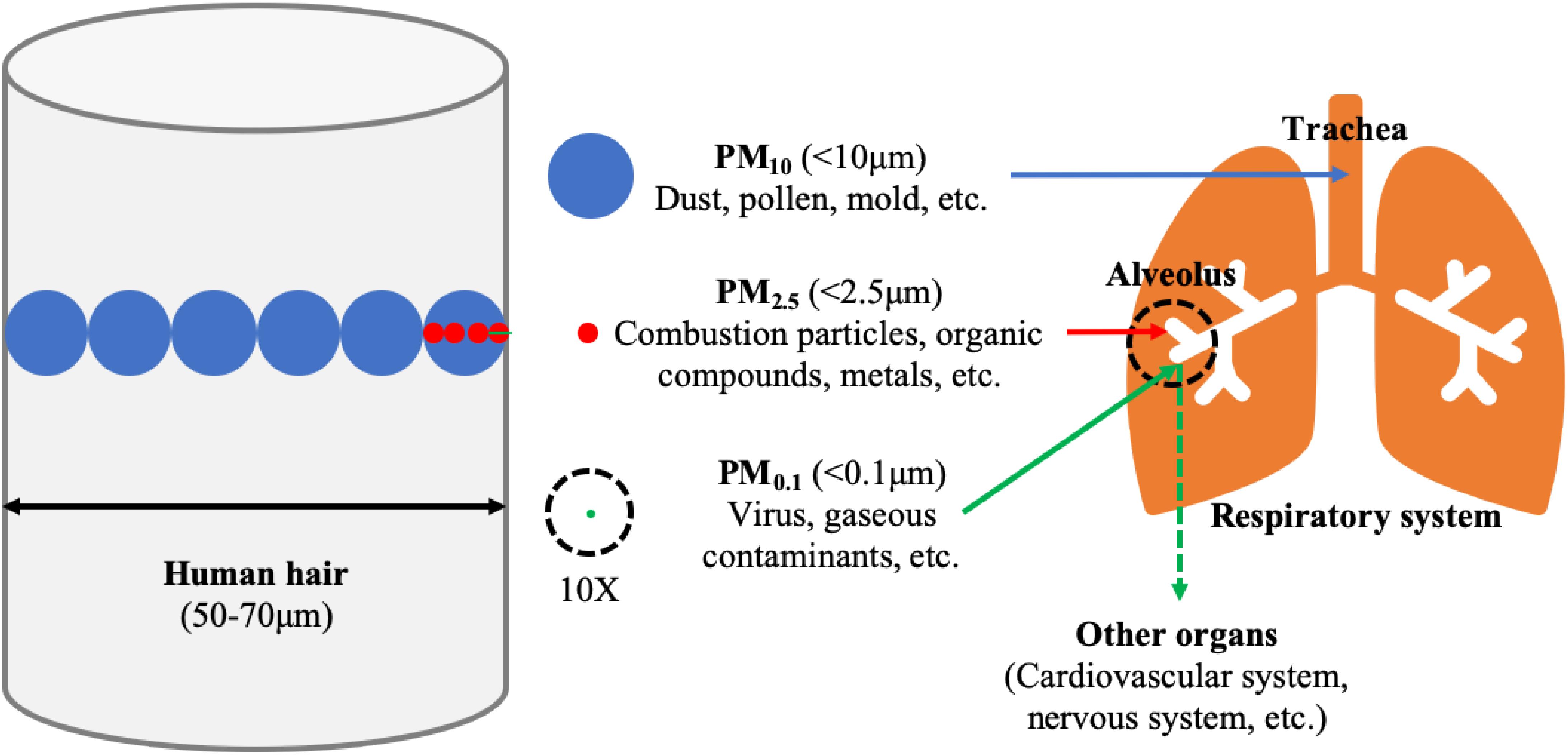
Figure 1. The size, main composition and deposition site in the lung of the particulate matter (PM). The average diameter of human hair is 60 μm, which approximately equivalents to six inhalable particulate matter (PM10, <10 μm in diameter, blue color), or twenty-four fine particulate matter (PM2.5, <2.5 μm in diameter, red color) or six hundred ultrafine particulate matter (PM0.1, <0.1 μm in diameter, green color). Particulate matter, with aerodynamic diameter 2.5–10 μm, is mainly deposited on the trachea. PM less than 2.5 μm in diameter poses the greatest problems, because it can get deep into the terminal bronchioles and alveoli, and some with <0.1 μm in diameter may even get into the bloodstream affecting other organs. 10X: The stuff in the dotted ring is magnified ten times.
PM2.5 may be the environmental risk factor that poses the greatest public health hazard. The GBD 2015 ranks PM2.5 as the fifth leading risk factor for death, with exposure to PM2.5 causing 4.2 million deaths (7.6% of global deaths) and loss of 10.31 million disability-adjusted life-years (DALYs) (4.2% of global DALYs) (Cohen et al., 2017). As claimed by the World Health Organization (WHO), 9 out of every 10 people in urban areas are exposed to high levels of PM2.5 (annual average concentration >10 μg/m3) from outdoor air pollution, and about 3 billion people using non-renewable fuels are exposed to serious indoor air pollution (World Health Organization [WHO], 2019). According to the Air Quality Life Index (AQLI), sustained exposure to an additional 10 μg/m3 of PM2.5 reduces life expectancy by 0.98 years1. In addition, an increase of 10 μg/m3 per day of PM2.5 concentration increases 0.29% of overall non-accidental mortality and 0.22% of respiratory disease mortalities (Chen et al., 2017).
A large number of epidemiological studies have shown that PM2.5 exposure is closely related to a variety of respiratory diseases (Jo et al., 2017; Wang C. et al., 2019). It is noteworthy that exposure to PM2.5 increases the susceptibility to respiratory infections. For instance, numerous clinical studies have found that PM2.5 exposure is positively correlated with the number of outpatient visits, emergency visits, and hospitalizations for acute upper or lower respiratory infections (Li et al., 2017; Xia et al., 2017; Strosnider et al., 2019). Related animal models also support the notion that PM2.5 exposure increases susceptibility to the lung infection (Yang et al., 2001; Sigaud et al., 2007; Zhao et al., 2014). However, the underlying mechanisms remain elusive. We speculate that PM2.5 exposure may impair the host defense of the respiratory system, making the body more susceptible to infection. The purpose of this article is to review the existing epidemiological and experimental evidence to support the above hypothesis and to summarize the possible underlying mechanisms.
The Epidemiological Evidence
The respiratory system is the primary route for inhaled PM2.5. Exposure to PM2.5 can cause the development and progression of acute and chronic lung diseases, such as tracheal and pulmonary inflammation (Kampa and Castanas, 2008; Jedrychowski et al., 2013; Ge et al., 2018), asthma and its acute exacerbations (Habre et al., 2014; Zheng et al., 2015), chronic obstructive pulmonary disease (COPD) and its acute exacerbations (Faustini et al., 2013; Tsai et al., 2013). We are concerned with the fact that PM2.5 exposure increases the susceptibility to respiratory infections.
Outpatient, emergency, and hospitalization-related data on respiratory infections confirmed that PM2.5 exposure was positively associated with the increased respiratory infections. Li et al. (2017) studied the effects of air pollution on outpatients’ acute respiratory outcomes and their study indicated that PM2.5 exposure was positively correlated with outpatient visits for upper respiratory tract infection (URTI). A study in the U.S. state of Georgia also showed that pediatric emergency visits for URTI were associated with PM2.5 concentrations (Strickland et al., 2016). Another study reported that PM2.5 was significantly associated with emergency room visits for respiratory diseases, particularly for URTI and lower respiratory tract infection (LRTI) (Xu et al., 2016). Recently, Strosnider et al. (2019) confirmed that PM2.5 exposure was positively correlated with emergency visits for multiple respiratory diseases, including respiratory infections. In addition, Xia et al. (2017) studied the association between different types of air pollution and respiratory hospitalization and found that short-term exposure of PM2.5 was positively correlated with the number of hospitalizations for acute respiratory infections. Similarly, another four studies also supported a significant positive correlation between PM2.5 and the number of hospitalizations for URTI and LRTI (Belleudi et al., 2010; Li et al., 2018; Liu et al., 2019b; Zhang D. et al., 2019).
The effect of PM2.5 exposure on respiratory infections is not immediate, but there is a certain lag effect. For example, PM2.5 exposure was positively associated with an increase in hospitalization for acute respiratory infections, but this correlation was delayed by 7–13 days (Xia et al., 2017). Liang et al. (2014) analyzed the time curve of PM2.5 concentration and human influenza in Beijing urban area from 2008 to 2011, and proved that there was a significant correlation between PM2.5 exposure and influenza, but this correlation showed a 1–2 months delay. In a study of 150,000 cases, Horne et al. (2018) investigated the relationship between PM2.5 and the health status of patients with acute LRTI and discovered that the association of PM2.5 exposure with respiratory syncytial virus (RSV) infection occurred in 2–4 weeks. The hysteresis effects varied in different studies. Therefore, when investigating the association between PM2.5 exposure and respiratory infections, researchers may need to choose the appropriate follow-up time.
Exposure to PM2.5 increases the susceptibility to respiratory infections, especially in children and the elderly, as well as the vulnerable groups with hereditary or underlying diseases. A study on the relationship between PM2.5 and the health status of patients with acute LRTI revealed that about 77% of the subjects were infants aged 0–2 years. RSV and influenza virus were the main pathogens (Horne et al., 2018). Another study on risks of respiratory infections associated with air pollution in China showed that children under the age of 14 were the predominantly susceptible population of acute respiratory infections caused by air pollution, accounting for 80% of hospitalized cases of respiratory system infections in the study (Xia et al., 2017). Similarly, Liu et al. (2019b) found that students aged 6–17 years were more vulnerable to PM2.5 exposure. The maternal exposure to air pollution before birth may result in an impaired lung development and increase the risk of respiratory system infections (Pinkerton and Joad, 2006; Jedrychowski et al., 2013). In addition, by analyzing the association between PM2.5 pollution and hospital emergency room visits for total and cause-specific respiratory diseases in urban areas in Beijing, Xu et al. (2016) found that people over 60 years of age demonstrated a higher risk of respiratory disease (including URTI and LRTI) after PM2.5 exposure. Exposure to PM2.5 is more likely to cause respiratory infections in people with congenital immune deficiencies due to hereditary diseases. For instance, exposure to PM2.5 in patients with cystic fibrosis has been reported to be associated with the acquisition of Pseudomonas aeruginosa (P. aeruginosa). During the follow-up period, each additional PM2.5 exposure of 10 μg/m3 increased the risk of 24% P. aeruginosa acquisition (Psoter et al., 2015). Another study has found that each additional PM2.5 exposure of 10 μg/m3 increased the risk of methicillin-resistant Staphylococcus aureus (MRSA) by 68% (Psoter et al., 2017).
The Experimental Evidence
In vivo studies have shown that as a risk factor for respiratory infection, PM2.5 exposure, can prime the lung for greater susceptibility to pathogens by impairing the respiratory host defense. Yang et al. (2001) found that PM exposure suppressed macrophage function and slowed the pulmonary clearance of Listeria monocytogenes (L. monocytogenes) in rats. Another research discovered that the colony-forming units (CFUs) of P. aeruginosa detected in the lung were significantly greater in the PM-exposed mice compared to the control mice (Liu et al., 2019a). Zhao et al. (2014) found that prior PM2.5 exposure markedly increased the susceptibility of rats to subsequent Staphylococcus aureus (S. aureus) infection. Similarly, Duan et al. (2013) found that PM2.5 exposure increased the susceptibility of rats to Klebsiella pneumoniae (K. pneumoniae) infection. Sigaud et al. (2007) and Migliaccio et al. (2013) established an exposure model in mice and subsequently infected with Streptococcus pneumoniae (S. pneumoniae). They found that PM exposure reduced bacterial clearance in the lungs of mice. In addition, Ma et al. (2017) discovered that exposure to PM2.5 lowers influenza virus resistance. We summarized the in vivo experimental studies of PM2.5 on respiratory host defense (Table 1).
In vitro experiments have also confirmed that PM2.5 exposure increased the susceptibility of respiratory infection. For example, PM2.5-pretreated A549 cells have a significantly increased risk of infection with Mycobacterium tuberculosis (M. tuberculosis) (Rivas-Santiago et al., 2015), and PM can disrupt the airway epithelium through oxidative burst to promote P. aeruginosa infection (Liu et al., 2019a). Similarly, Chen et al. (2018) found that PM suppressed airway antibacterial defense, causing an increased susceptibility to P. aeruginosa. In addition, adhesion is the key to microbial invasion of the respiratory tract. PM increased the binding of S. pneumoniae to both primary alveolar macrophages (AMs) and the murine macrophage cell line J774 A.1 but decreased internalization of bacteria (Zhou and Kobzik, 2007). Mushtaq et al. (2011) have discovered that urban PM increased the adhesion of S. pneumoniae to human tracheal epithelial cells. We also summarized the in vitro experimental studies of PM2.5 on respiratory host defense (Table 2).
Possible Mechanisms
PM2.5 exposure impairs the host defense of respiratory system causing the body more susceptible to infection. We dissect the underlying mechanisms from the following three aspects: defective airway epithelial host defense functions, alterations in respiratory microecology, insufficiency and dysfunction of immune cells (Figure 2).
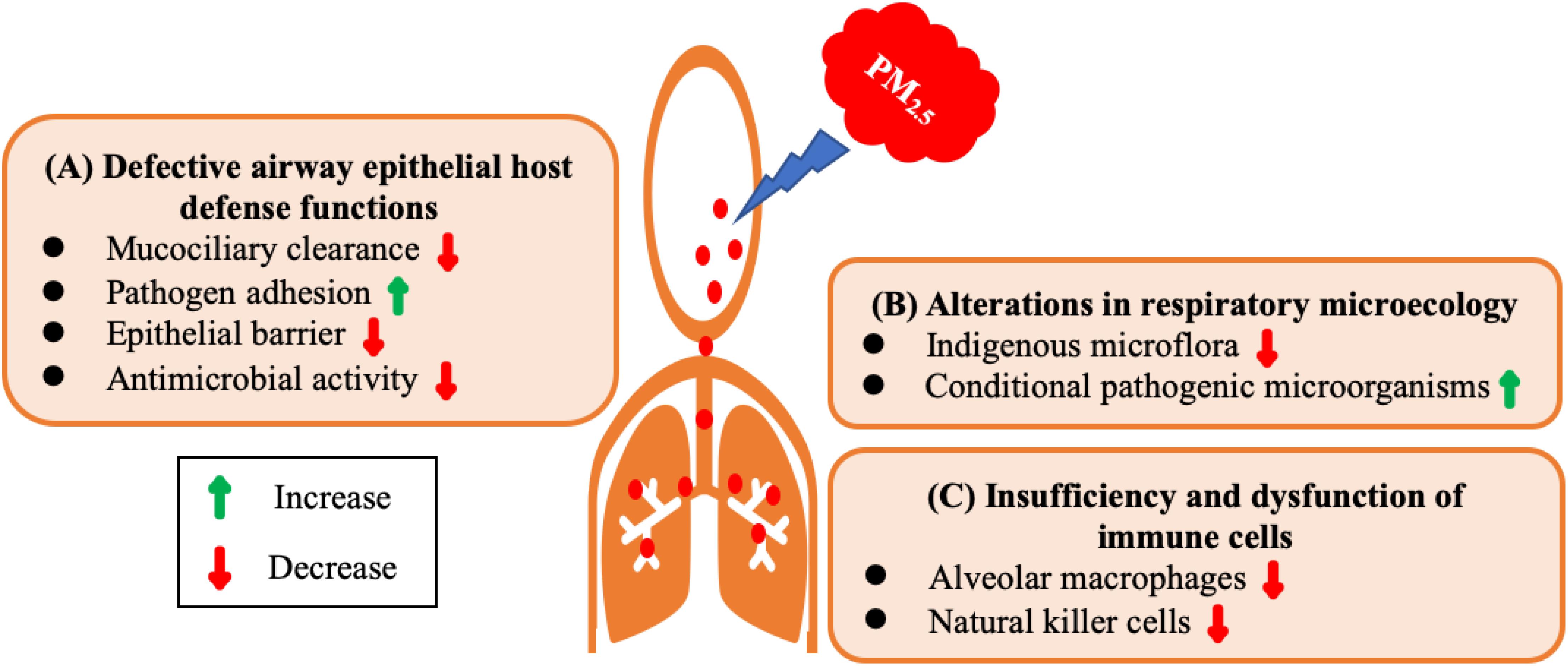
Figure 2. Possible mechanisms underlying defective host defense of respiratory system by PM2.5 exposure. (A) Defective airway epithelial host defense functions. PM2.5 exposure increases adhesion of pathogens to epithelial cells, impairs epithelial barrier function, compromises mucociliary clearance, and reduces antimicrobial activity. (B) Alterations in respiratory microecology. PM2.5 exposure decreases indigenous microflora and increases the content of conditional pathogenic microorganisms. (C) Insufficiency and dysfunction of immune cells. PM2.5 exposure decreases the number of natural killer cells and impairs the phagocytic capacity of alveolar macrophages.
Defective Airway Epithelial Host Defense Functions
At the interface between the external environment and the host, the airway epithelium serves as the first line of host defense against pathogens. The airway epithelial host defense functions mainly include mucociliary clearance, the barrier functions of the epithelium, and the secretion of a number of proteins and peptides with antimicrobial activities (Figure 3A). Mucociliary clearance in the airway epithelium is a critical protective function and is essential for the clearance of respiratory pathogens. In general, most foreign bodies inhaled into the lungs can be removed in time by the mucociliary clearance system. However, it has been reported that PM2.5 exposure decreased bacterial clearance by impairing the bronchial mucociliary system (Duan et al., 2013). Mucin hyperproduction or hypersecretion is a common reason for decreased mucociliary clearance. Val et al. (2012) found that the expression of MUC5AC, one of the predominant mucins produced by the airway epithelium, was upregulated via the epidermal growth factor receptor (EGFR) pathway after PM2.5 exposure in mice (Figure 3B).
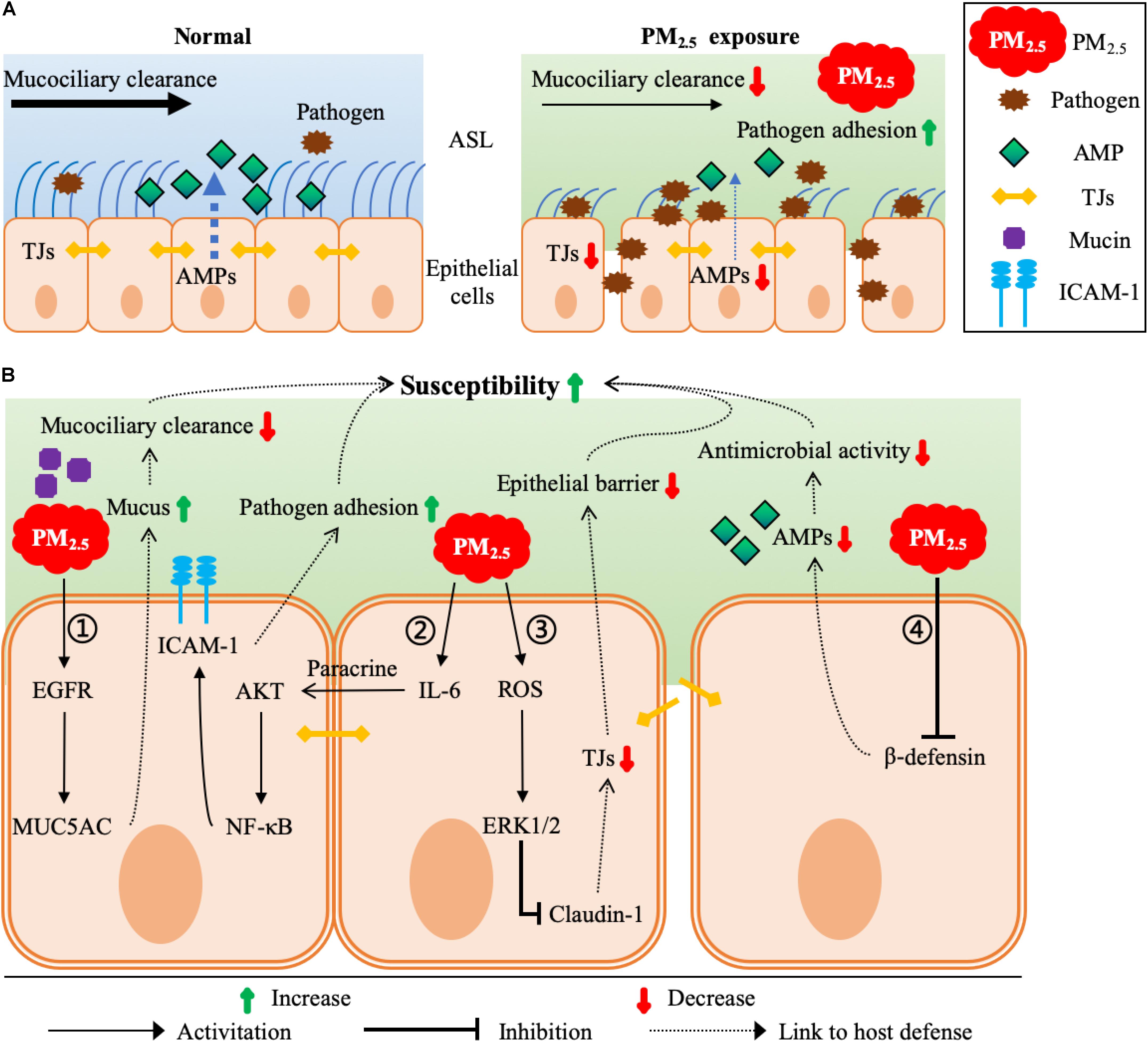
Figure 3. Molecular mechanisms underlying PM2.5-induced defective airway epithelial host defense functions. (A) Major host defense functions of normal and PM2.5-exposured airway epithelia. Normal airway epithelia are protected from pathogens mainly by mucociliary clearance, the barrier functions of the epithelium, and the secretion of a number of AMPs with antimicrobial activities. However, PM2.5 exposure disrupts these host defense functions, resulting in more pathogens in the airways. (B) Underlying molecular mechanisms: ① PM2.5 → EGFR ↑→ MUC5AC ↑→ Mucus ↑→ Mucociliary clearance ↓: PM2.5 up-regulates the expression of MUC5AC (one of the predominant mucins produced by the airway epithelium) by activating EGFR pathway, resulting in decreased mucociliary clearance. ② PM2.5 → IL-6 ↑→ AKT ↑→ NF-κB ↑→ ICAM-1 ↑→ Pathogen adhesion ↑: PM2.5 activates AKT/NF-κB pathway through IL-6 paracrine signaling, which then up-regulates the expression of ICAM-1 (an important glycoprotein on the cell surface) in the lung to increase the adhesion of pathogens to the airway epithelium. ③ PM2.5 → ROS ↑→ ERK-1/2 ↑→ Claudin-1 ↓→ TJs ↓→ Epithelial barrier ↓: PM2.5 down-regulates the expression of claudin-1 (a major structural protein of TJs) via generating ROS and activating ERK1/2 pathway, resulting in more pathogens to translocate across the disrupted epithelial barrier. ④ PM2.5 →β-defensin ↓→ AMPs ↓→ Antimicrobial activity ↓: PM2.5 inhibits the expression and secretion of β-defensin (one of the major AMPs in ASL), which allows more pathogens to survive and exacerbates respiratory infections.
The adhesion of pathogens to host cells is a prerequisite for infection. A study has reported that exposure to urban PM increased the adhesion of S. pneumoniae to human airway epithelial cells, and the addition of N-acetylcysteine (NAC, an antioxidant) reversed this process, possibly be related to reactive oxygen species (ROS) produced by oxidative stress (Mushtaq et al., 2011). In addition, Liu et al. (2018) reported that ROS induced by PM2.5 activated the AKT/STAT3/NF-κB pathway through IL-6 paracrine signaling, which then upregulated the expression of intercellular adhesion molecule-1 (ICAM-1, an important glycoprotein on the cell surface) in the lung to increase the adhesion of pathogens to the airway epithelium (Figure 3B). Woo et al. (2018) also found that PM2.5 could enhance the adhesion of P. aeruginosa to epithelial cells, the mechanism of which depended on the increased bacterial surface hydrophobicity and damaged human cell plasma membrane by PM2.5.
Tight junctions (TJs) are the significant protein complexes at cell-cell interfaces that connect adjacent cells with each other to form lung epithelial barrier against pathogens (Schlingmann et al., 2015). Lack of an intact TJs structure, the airway epithelial barrier cannot keep tight. It will allow pathogens to translocate across the barrier, making the lungs more susceptible to infection. A recent study reported that PM impaired TJs of airway epithelial barrier via oxidative stress to promote P. aeruginosa infection (Liu et al., 2019a). Claudin-1 is a major structural protein of TJs. Similarly, another study also discovered that exposure to PM downregulated claudin-1 expression in human airway cells via the ERK1/2 signaling pathway (Kim et al., 2017) (Figure 3B).
The airway epithelial cells are covered with a very thin fluid layer (airway surface liquid, ASL), which is an important component of the respiratory innate immunity. Antimicrobial peptides (AMPs) content is a significant and indispensable factor affecting the antibacterial effect of ASL. AMPs include salivary agglutinin (SAG), beta-defensins, lactoferrin, secretory IgA, and surfactant protein D (SPD) (Fabian et al., 2012; Kendall et al., 2013; Vargas Buonfiglio et al., 2018). Zhang S. et al. (2019) found that PM2.5 exposure attenuated the antibacterial activity of airways by down-regulating the expression of SAG. In addition, several studies indicated that PM2.5 exposure down-regulated airway β-defensin expression levels through oxidative stress (Rivas-Santiago et al., 2015; Vargas Buonfiglio et al., 2017; Chen et al., 2018). Collectively, these studies suggested that PM2.5 could compromise the host defense function of airway epithelial cells by downregulating the expression of AMPs (Figure 3B).
Alterations in Respiratory Microecology
In healthy humans, the low respiratory tract is usually sterile, and without permanent bacterial colonization, while the upper respiratory tract (especially oropharynx) has a normal bacterial flora, which is also an important component of respiratory tract’s natural immune defense, providing a biological barrier against foreign matter or pathogenic microorganisms througha space-occupying effect, nutritional competition, and secretion of bacteriostatic or bactericidal substances (Akata et al., 2016; Marsh et al., 2016; Wang L. et al., 2019). The oropharyngeal microecosystems of rats changed following PM exposure, including a decline of indigenous microflora and an increase of the content of conditional pathogenic microorganisms (Xiao et al., 2013). By analyzing 16S rRNA sequencing of respiratory tract lavage fluid, a recent study confirmed that there is a link between PM2.5 exposure and alterations of the respiratory tract microecology (Yang et al., 2019).
Insufficiency and Dysfunction of Immune Cells
A variety of immune cells are resident in the respiratory tract, including AMs, polymorphonuclear granulocytes (PMNs), lymphocytes, etc. Their numbers and functions are essential to protect against pathogen invasion. Zhao et al. (2014) found that the reduction of phagocytic phagocytosis caused by PM2.5 exposure was related to a decrease of NKs in a PM2.5 exposure rat model and subsequently infected with S. aureus. Another research found that PM2.5 exposure can trigger a Th2-type immune response and reduce the phagocytic capacity of AMs, which may be related to Toll-like receptor 2 (TLR2) and Toll-like receptor 4 (TLR4) (Zhao et al., 2012). In addition, Ma et al. (2017) found that long-term exposure to PM2.5 lowered influenza virus resistance via down-regulating pulmonary macrophage Kdm6a and mediated histones modification in IL-6 and IFN-β promoter regions. Another two studies indicated that PM2.5 can be used as an immunity inhibitor to reduce the phagocytic capacity of macrophages, thereby increasing the susceptibility to S. pneumoniae infection (Migliaccio et al., 2013; Zhao et al., 2014). PM exposure has also been indicated to decrease the ability of macrophages to produce antimicrobial oxidants in response to L. monocytogenes, which may play a role in the increased susceptibility of rats to respiratory infection (Yang et al., 2001).
Conclusion and Future Perspectives
In summary, there are sufficient epidemiological evidences from outpatient, emergency and hospitalization-related data that exposure to PM2.5 increases susceptibility to respiratory infections. However, studies on the association between PM2.5 exposure and age, gender, and specific pathogens remain controversial. In the future, a meta-analysis of existing research can be attempted to further confirm the susceptible population of PM2.5 exposure to respiratory infections. We notice that there is a lag effect in the association between PM2.5 exposure and respiratory infections. This may be due to the variations of the average incubation period of different pathogens. We also note that only a few epidemiological studies have reported the relationship between PM2.5 exposure and the infection rates of specific pathogens associated with respiratory infections. Is there a specific pathogen preference for respiratory infections caused by PM2.5 exposure? This still requires the unremitting efforts of the researchers.
In vivo and in vitro studies have shown that PM2.5 exposure is beneficial to the adhesion, colonization and growth of microorganisms, but it is not conducive to the removal from the body. However, most of the current in vivo experiments establish a PM2.5 exposure animal model by intratracheal instillation. This model usually affects the lower respiratory tract and is prone to uneven distribution in the lung lobes. Concentration and enrichment of PM2.5 aerosol combined with the oral-inhalation or whole-body exposure system may be the best model for simulating human exposure. In recent years, an emerging technology has appeared, combing versatile aerosol concentration enrichment system (VACES) with oral-inhalation or whole-body exposure system. The advantages and disadvantages of these two models are shown in Table 3. Furthermore, current in vitro experiments are limited to epithelial cells- and alveolar macrophage-mediated innate immune responses, lacking attention to adaptive immune responses. Either in vivo or in vitro, the exposure doses currently used in the study are usually based on the corresponding toxicity experiments. How can such exposure levels represent real-world or clinical scenarios?
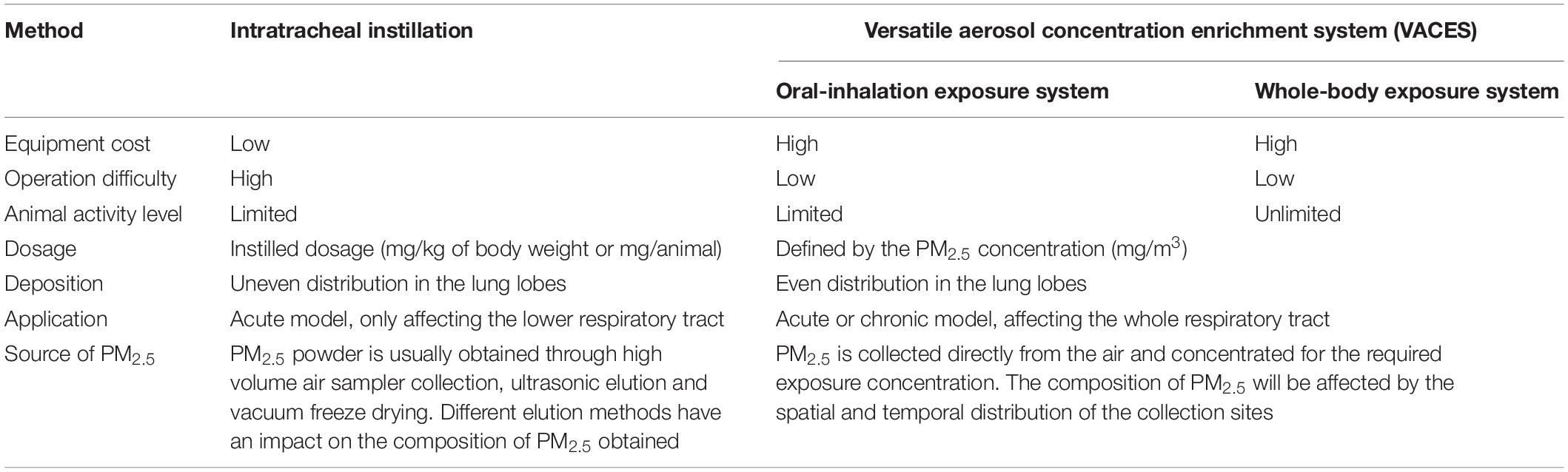
Table 3. Strengths and weaknesses of intratracheal instillation and versatile aerosol concentration enrichment system (VACES) on PM2.5 exposure to experimental rodents.
PM2.5 exposure may impair the host defense system of respiratory system, making the organism susceptible to infection. In brief, the possible mechanisms include defective airway epithelial host defense functions, alterations of the respiratory tract microecology, and insufficient number or dysfunction of immune cells (Figure 2). However, there is still a lack of robust research on molecular mechanisms. Further efforts are desperately needed to elucidate the underlying mechanisms at the molecular level.
Author Contributions
XT conceived and designed the manuscript. LY, CL, and XT wrote the manuscript and critically revised it. CL and XT generated the figures. XT provided guidance and edited the manuscript.
Funding
The authors were supported by the National Thousand Young Talents Program (XT), the National Natural Science Foundation of China (81770015, XT), Local Innovative and Research Teams Project of Guangdong Pearl River Talents Program (2017BT01S155), and Open Project of State Key Laboratory of Respiratory Disease (SKLRD-OP-201906).
Conflict of Interest
The authors declare that the research was conducted in the absence of any commercial or financial relationships that could be construed as a potential conflict of interest.
Acknowledgments
This is a short text to acknowledge the contributions of specific colleagues, institutions, or agencies that aided the efforts of the authors.
Abbreviations
AMPs, antimicrobial peptides; AMs, alveolar macrophages; AQLI, Air Quality Life Index; ASL, airway surface liquid; CFUs, colony-forming units; COPD, chronic obstructive pulmonary disease; DALYs, disability-adjusted life-years; GBD, global burden of disease; ICAM-1, intercellular adhesion molecule-1; K. pneumoniae, klebsiella pneumoniae; L. monocytogenes, Listeria monocytogenes; LRTI, lower respiratory tract infection; M. tuberculosis, Mycobacterium tuberculosis; MRSA, Methicillin-resistant Staphylococcus aureus; NAC, N-acetylcysteine; NKs, natural killer cells; P. aeruginosa, Pseudomonas aeruginosa; PM, particulate matter; PM10, inhalable particulate matter; PM2.5, fine particulate matter; PM0.1, ultrafine particulate matter; PMNs, polymorphonuclear granulocytes; ROS, reactive oxygen species; RSV, respiratory syncytial virus; S. aureus, Staphylococcus aureus; S. pneumoniae, Streptococcus pneumoniae; SAG, salivary agglutinin; SPD, surfactant protein D; TJs, tight junctions; TLR2, Toll-like receptor 2; TLR4, Toll-like receptor 4; URTI, upper respiratory tract infection; VACES, versatile aerosol concentration enrichment system; WHO, World Health Organization.
Footnotes
References
Akata, K., Yatera, K., Yamasaki, K., Kawanami, T., Naito, K., Noguchi, S., et al. (2016). The significance of oral streptococci in patients with pneumonia with risk factors for aspiration: the bacterial floral analysis of 16S ribosomal RNA gene using bronchoalveolar lavage fluid. BMC Pulm. Med. 16:79. doi: 10.1186/s12890-016-0235-z
Belleudi, V., Faustini, A., Stafoggia, M., Cattani, G., Marconi, A., Perucci, C. A., et al. (2010). Impact of fine and ultrafine particles on emergency hospital admissions for cardiac and respiratory diseases. Epidemiology 21, 414–423. doi: 10.1097/EDE.0b013e3181d5c021
Chauhan, A. J., and Johnston, S. L. (2003). Air pollution and infection in respiratory illness. Br. Med. Bull. 68, 95–112. doi: 10.1093/bmb/ldg022
Chen, R., Yin, P., Meng, X., Liu, C., Wang, L., Xu, X., et al. (2017). Fine particulate air pollution and daily mortality. A nationwide analysis in 272 Chinese cities. Am. J. Respir. Crit. Care Med. 196, 73–81. doi: 10.1164/rccm.201609-1862OC
Chen, X., Liu, J., Zhou, J., Wang, J., Chen, C., Song, Y., et al. (2018). Urban particulate matter (PM) suppresses airway antibacterial defence. Respir. Res. 19:5. doi: 10.1186/s12931-017-0700-0
Cho, C. C., Hsieh, W. Y., Tsai, C. H., Chen, C. Y., Chang, H. F., and Lin, C. S. (2018). In vitro and in vivo experimental studies of PM2.5 on disease progression. Int. J. Environ. Res. Public Health 15:1380. doi: 10.3390/ijerph15071380
Cohen, A. J., Brauer, M., Burnett, R., Anderson, H. R., Frostad, J., Estep, K., et al. (2017). Estimates and 25-year trends of the global burden of disease attributable to ambient air pollution: an analysis of data from the global burden of diseases study 2015. Lancet 389, 1907–1918. doi: 10.1016/S0140-6736(17)30505-6
Duan, Z., Du, F. Y., Yuan, Y. D., Zhang, Y. P., Yang, H. S., and Pan, W. S. (2013). [Effects of PM2.5 exposure on Klebsiella pneumoniae clearance in the lungs of rats]. Zhonghua Jie He He Hu Xi Za Zhi 36, 836–840.
Fabian, T. K., Hermann, P., Beck, A., Fejerdy, P., and Fabian, G. (2012). Salivary defense proteins: their network and role in innate and acquired oral immunity. Int. J. Mol. Sci. 13, 4295–4320. doi: 10.3390/ijms13044295
Faustini, A., Stafoggia, M., Colais, P., Berti, G., Bisanti, L., Cadum, E., et al. (2013). Air pollution and multiple acute respiratory outcomes. Eur. Respir. J. 42, 304–313. doi: 10.1183/09031936.00128712
GBD 2015 Risk Factors Collaborators (2016). Global, regional, and national comparative risk assessment of 79 behavioural, environmental and occupational, and metabolic risks or clusters of risks, 1990-2015: a systematic analysis for the Global Burden of Disease Study 2015. Lancet 388, 1659–1724. doi: 10.1016/S0140-6736(16)31679-8
Ge, E., Lai, K., Xiao, X., Luo, M., Fang, Z., Zeng, Y., et al. (2018). Differential effects of size-specific particulate matter on emergency department visits for respiratory and cardiovascular diseases in Guangzhou, China. Environ. Pollut. 243(Pt A), 336–345. doi: 10.1016/j.envpol.2018.08.068
Habre, R., Moshier, E., Castro, W., Nath, A., Grunin, A., Rohr, A., et al. (2014). The effects of PM2.5 and its components from indoor and outdoor sources on cough and wheeze symptoms in asthmatic children. J. Expo. Sci. Environ. Epidemiol. 24, 380–387. doi: 10.1038/jes.2014.21
Horne, B. D., Joy, E. A., Hofmann, M. G., Gesteland, P. H., Cannon, J. B., Lefler, J. S., et al. (2018). Short-term elevation of fine particulate matter air pollution and acute lower respiratory infection. Am. J. Respir. Crit. Care Med. 198, 759–766. doi: 10.1164/rccm.201709-1883OC
Hsu, C. Y., Chiang, H. C., Lin, S. L., Chen, M. J., Lin, T. Y., and Chen, Y. C. (2016). Elemental characterization and source apportionment of PM10 and PM2.5 in the western coastal area of Central Taiwan. Sci. Total Environ. 541, 1139–1150. doi: 10.1016/j.scitotenv.2015.09.122
Jedrychowski, W. A., Perera, F. P., Spengler, J. D., Mroz, E., Stigter, L., Flak, E., et al. (2013). Intrauterine exposure to fine particulate matter as a risk factor for increased susceptibility to acute broncho-pulmonary infections in early childhood. Int. J. Hyg. Environ. Health 216, 395–401. doi: 10.1016/j.ijheh.2012.12.014
Jo, E. J., Lee, W. S., Jo, H. Y., Kim, C. H., Eom, J. S., Mok, J. H., et al. (2017). Effects of particulate matter on respiratory disease and the impact of meteorological factors in Busan, Korea. Respir. Med. 124, 79–87. doi: 10.1016/j.rmed.2017.02.010
Kampa, M., and Castanas, E. (2008). Human health effects of air pollution. Environ. Pollut. 151, 362–367. doi: 10.1016/j.envpol.2007.06.012
Kendall, M., Ding, P., Mackay, R. M., Deb, R., McKenzie, Z., Kendall, K., et al. (2013). Surfactant protein D (SP-D) alters cellular uptake of particles and nanoparticles. Nanotoxicology 7, 963–973. doi: 10.3109/17435390.2012.689880
Kim, S. S., Kim, C. H., Kim, J. W., Kung, H. C., Park, T. W., Shin, Y. S., et al. (2017). Airborne particulate matter increases MUC5AC expression by downregulating Claudin-1 expression in human airway cells. BMB Rep. 50, 516–521. doi: 10.5483/bmbrep.2017.50.10.100
Li, R., Jiang, N., Liu, Q., Huang, J., Guo, X., Liu, F., et al. (2017). Impact of air pollutants on outpatient visits for acute respiratory outcomes. Int. J. Environ. Res. Public Health 14:47. doi: 10.3390/ijerph14010047
Li, Y. R., Xiao, C. C., Li, J., Tang, J., Geng, X. Y., Cui, L. J., et al. (2018). Association between air pollution and upper respiratory tract infection in hospital outpatients aged 0-14 years in Hefei, China: a time series study. Public Health 156, 92–100. doi: 10.1016/j.puhe.2017.12.006
Liang, Y., Fang, L., Pan, H., Zhang, K., Kan, H., Brook, J. R., et al. (2014). PM2.5 in Beijing – temporal pattern and its association with influenza. Environ. Health 13:102. doi: 10.1186/1476-069X-13-102
Liu, C. W., Lee, T. L., Chen, Y. C., Liang, C. J., Wang, S. H., Lue, J. H., et al. (2018). PM2.5-induced oxidative stress increases intercellular adhesion molecule-1 expression in lung epithelial cells through the IL-6/AKT/STAT3/NF-kappaB-dependent pathway. Part Fibre Toxicol. 15:4. doi: 10.1186/s12989-018-0240-x
Liu, J., Chen, X., Dou, M., He, H., Ju, M., Ji, S., et al. (2019a). Particulate matter disrupts airway epithelial barrier via oxidative stress to promote Pseudomonas aeruginosa infection. J. Thorac. Dis. 11, 2617–2627. doi: 10.21037/jtd.2019.05.77
Liu, J., Li, Y., Li, J., Liu, Y., Tao, N., Song, W., et al. (2019b). Association between ambient PM2.5 and children’s hospital admissions for respiratory diseases in Jinan, China. Environ. Sci. Pollut. Res. Int. 26, 24112–24120. doi: 10.1007/s11356-019-05644-7
Ma, J. H., Song, S. H., Guo, M., Zhou, J., Liu, F., Peng, L., et al. (2017). Long-term exposure to PM2.5 lowers influenza virus resistance via down-regulating pulmonary macrophage Kdm6a and mediates histones modification in IL-6 and IFN-beta promoter regions. Biochem. Biophys. Res. Commun. 493, 1122–1128. doi: 10.1016/j.bbrc.2017.09.013
Marsh, R. L., Kaestli, M., Chang, A. B., Binks, M. J., Pope, C. E., Hoffman, L. R., et al. (2016). The microbiota in bronchoalveolar lavage from young children with chronic lung disease includes taxa present in both the oropharynx and nasopharynx. Microbiome 4:37. doi: 10.1186/s40168-016-0182-1
Migliaccio, C. T., Kobos, E., King, Q. O., Porter, V., Jessop, F., and Ward, T. (2013). Adverse effects of wood smoke PM(2.5) exposure on macrophage functions. Inhal. Toxicol. 25, 67–76. doi: 10.3109/08958378.2012.756086
Mushtaq, N., Ezzati, M., Hall, L., Dickson, I., Kirwan, M., Png, K. M., et al. (2011). Adhesion of Streptococcus pneumoniae to human airway epithelial cells exposed to urban particulate matter. J. Allergy Clin. Immunol. 127, 1236.e2–1242.e2. doi: 10.1016/j.jaci.2010.11.039
Pinkerton, K. E., Green, F. H., Saiki, C., Vallyathan, V., Plopper, C. G., Gopal, V., et al. (2000). Distribution of particulate matter and tissue remodeling in the human lung. Environ. Health Perspect. 108, 1063–1069. doi: 10.1289/ehp.001081063
Pinkerton, K. E., and Joad, J. P. (2006). Influence of air pollution on respiratory health during perinatal development. Clin. Exp. Pharmacol. Physiol. 33, 269–272. doi: 10.1111/j.1440-1681.2006.04357.x
Psoter, K. J., De Roos, A. J., Mayer, J. D., Kaufman, J. D., Wakefield, J., and Rosenfeld, M. (2015). Fine particulate matter exposure and initial Pseudomonas aeruginosa acquisition in cystic fibrosis. Ann. Am. Thorac. Soc. 12, 385–391. doi: 10.1513/AnnalsATS.201408-400OC
Psoter, K. J., De Roos, A. J., Wakefield, J., Mayer, J. D., and Rosenfeld, M. (2017). Air pollution exposure is associated with MRSA acquisition in young U.S. children with cystic fibrosis. BMC Pulm. Med. 17:106. doi: 10.1186/s12890-017-0449-8
Rivas-Santiago, C. E., Sarkar, S., Cantarella, P. IV, Osornio-Vargas, A., Quintana-Belmares, R., Meng, Q., et al. (2015). Air pollution particulate matter alters antimycobacterial respiratory epithelium innate immunity. Infect. Immun. 83, 2507–2517. doi: 10.1128/IAI.03018-14
Schlingmann, B., Molina, S. A., and Koval, M. (2015). Claudins: gatekeepers of lung epithelial function. Semin. Cell Dev. Biol. 42, 47–57. doi: 10.1016/j.semcdb.2015.04.009
Schulze, F., Gao, X., Virzonis, D., Damiati, S., Schneider, M. R., and Kodzius, R. (2017). Air quality effects on human health and approaches for its assessment through microfluidic chips. Genes (Basel) 8:244. doi: 10.3390/genes8100244
Sigaud, S., Goldsmith, C. A., Zhou, H., Yang, Z., Fedulov, A., Imrich, A., et al. (2007). Air pollution particles diminish bacterial clearance in the primed lungs of mice. Toxicol. Appl. Pharmacol. 223, 1–9. doi: 10.1016/j.taap.2007.04.014
Strickland, M. J., Hao, H., Hu, X., Chang, H. H., Darrow, L. A., and Liu, Y. (2016). Pediatric emergency visits and short-term changes in PM2.5 concentrations in the U.S. State of Georgia. Environ. Health Perspect. 124, 690–696. doi: 10.1289/ehp.1509856
Strosnider, H. M., Chang, H. H., Darrow, L. A., Liu, Y., Vaidyanathan, A., and Strickland, M. J. (2019). Age-specific associations of ozone and fine particulate matter with respiratory emergency department visits in the United States. Am. J. Respir. Crit. Care Med. 199, 882–890. doi: 10.1164/rccm.201806-1147OC
Tsai, S. S., Chang, C. C., and Yang, C. Y. (2013). Fine particulate air pollution and hospital admissions for chronic obstructive pulmonary disease: a case-crossover study in Taipei. Int. J. Environ. Res. Public Health 10, 6015–6026. doi: 10.3390/ijerph10116015
Val, S., Belade, E., George, I., Boczkowski, J., and Baeza-Squiban, A. (2012). Fine PM induce airway MUC5AC expression through the autocrine effect of amphiregulin. Arch. Toxicol. 86, 1851–1859. doi: 10.1007/s00204-012-0903-6
Vargas Buonfiglio, L. G., Borcherding, J. A., Frommelt, M., Parker, G. J., Duchman, B., Vanegas Calderon, O. G., et al. (2018). Airway surface liquid from smokers promotes bacterial growth and biofilm formation via iron-lactoferrin imbalance. Respir. Res. 19:42. doi: 10.1186/s12931-018-0743-x
Vargas Buonfiglio, L. G., Mudunkotuwa, I. A., Abou Alaiwa, M. H., Vanegas Calderon, O. G., Borcherding, J. A., Gerke, A. K., et al. (2017). Effects of coal fly ash particulate matter on the antimicrobial activity of airway surface liquid. Environ. Health Perspect. 125:077003. doi: 10.1289/ehp876
Wang, C., Feng, L., and Chen, K. (2019). The impact of ambient particulate matter on hospital outpatient visits for respiratory and circulatory system disease in an urban Chinese population. Sci. Total Environ. 666, 672–679. doi: 10.1016/j.scitotenv.2019.02.256
Wang, L., Cheng, H., Wang, D., Zhao, B., Zhang, J., Cheng, L., et al. (2019). Airway microbiome is associated with respiratory functions and responses to ambient particulate matter exposure. Ecotoxicol. Environ. Saf. 167, 269–277. doi: 10.1016/j.ecoenv.2018.09.079
Woo, S. H., Lee, S. M., Park, K. C., Park, G. N., Cho, B., Kim, I., et al. (2018). Effects of fine particulate matter on Pseudomonas aeruginosa adhesion and biofilm formation in vitro. Biomed. Res. Int. 2018:6287932. doi: 10.1155/2018/6287932
World Health Organization [WHO] (2019). World Health Statistics 2019 Monitoring Health for the SDGs. Geneva: World Health Organization.
Xia, X., Zhang, A., Liang, S., Qi, Q., Jiang, L., and Ye, Y. (2017). The association between air pollution and population health risk for respiratory infection: a case study of Shenzhen, China. Int. J. Environ. Res. Public Health 14:950. doi: 10.3390/ijerph14090950
Xiao, C., Li, S., Zhou, W., Shang, D., Zhao, S., Zhu, X., et al. (2013). The effect of air pollutants on the microecology of the respiratory tract of rats. Environ. Toxicol. Pharmacol. 36, 588–594. doi: 10.1016/j.etap.2013.04.012
Xing, Y. F., Xu, Y. H., Shi, M. H., and Lian, Y. X. (2016). The impact of PM2.5 on the human respiratory system. J. Thorac. Dis. 8, E69–E74. doi: 10.3978/j.issn.2072-1439.2016.01.19
Xu, Q., Li, X., Wang, S., Wang, C., Huang, F., Gao, Q., et al. (2016). Fine particulate air pollution and hospital emergency room visits for respiratory disease in urban areas in Beijing. China, in 2013. PLoS One 11:e0153099. doi: 10.1371/journal.pone.0153099
Yang, B., Zhang, Y., Li, B., Zou, Y., and Xiao, C. (2019). Fine particulate matter alters the microecology of the murine respiratory tract. Environ. Sci. Pollut. Res. Int. 26, 8623–8632. doi: 10.1007/s11356-019-04372-2
Yang, H. M., Antonini, J. M., Barger, M. W., Butterworth, L., Roberts, B. R., Ma, J. K., et al. (2001). Diesel exhaust particles suppress macrophage function and slow the pulmonary clearance of Listeria monocytogenes in rats. Environ. Health Perspect. 109, 515–521. doi: 10.1289/ehp.01109515
Zhang, D., Tian, Y., Zhang, Y., Cao, Y., Wang, Q., and Hu, Y. (2019). Fine particulate air pollution and hospital utilization for upper respiratory tract infections in Beijing, China. Int. J. Environ. Res. Public Health 16:533. doi: 10.3390/ijerph16040533
Zhang, R., Wang, G., Guo, S., Zamora, M. L., Ying, Q., Lin, Y., et al. (2015). Formation of urban fine particulate matter. Chem. Rev. 115, 3803–3855. doi: 10.1021/acs.chemrev.5b00067
Zhang, S., Huo, X., Zhang, Y., Huang, Y., Zheng, X., and Xu, X. (2019). Ambient fine particulate matter inhibits innate airway antimicrobial activity in preschool children in e-waste areas. Environ. Int. 123, 535–542. doi: 10.1016/j.envint.2018.12.061
Zhao, C., Liao, J., Chu, W., Wang, S., Yang, T., Tao, Y., et al. (2012). Involvement of TLR2 and TLR4 and Th1/Th2 shift in inflammatory responses induced by fine ambient particulate matter in mice. Inhal. Toxicol. 24, 918–927. doi: 10.3109/08958378.2012.731093
Zhao, H., Li, W., Gao, Y., Li, J., and Wang, H. (2014). Exposure to particular matter increases susceptibility to respiratory Staphylococcus aureus infection in rats via reducing pulmonary natural killer cells. Toxicology 325, 180–188. doi: 10.1016/j.tox.2014.09.006
Zheng, X. Y., Ding, H., Jiang, L. N., Chen, S. W., Zheng, J. P., Qiu, M., et al. (2015). Association between air pollutants and asthma emergency room visits and hospital admissions in time series studies: a systematic review and meta- analysis. PLoS One 10:e0138146. doi: 10.1371/journal.pone.0138146
Keywords: PM2.5, respiratory system, infection, susceptibility, host defense
Citation: Yang L, Li C and Tang X (2020) The Impact of PM2.5 on the Host Defense of Respiratory System. Front. Cell Dev. Biol. 8:91. doi: 10.3389/fcell.2020.00091
Received: 12 November 2019; Accepted: 04 February 2020;
Published: 04 March 2020.
Edited by:
De Yun Wang, National University of Singapore, SingaporeReviewed by:
Xinming Wang, Chinese Academy of Sciences, ChinaMariateresa Giuliano, University of Campania Luigi Vanvitelli, Italy
Copyright © 2020 Yang, Li and Tang. This is an open-access article distributed under the terms of the Creative Commons Attribution License (CC BY). The use, distribution or reproduction in other forums is permitted, provided the original author(s) and the copyright owner(s) are credited and that the original publication in this journal is cited, in accordance with accepted academic practice. No use, distribution or reproduction is permitted which does not comply with these terms.
*Correspondence: Xiaoxiao Tang, dGFuZ3hpYW94aWFvQGdpcmQuY24=