- 1i3S – Institute for Research and Innovation in Health, University of Porto, Porto, Portugal
- 2Institute of Molecular Pathology and Immunology, University of Porto, Porto, Portugal
- 3Faculty of Medicine, University of Porto, Porto, Portugal
- 4Department of Biology, Faculty of Sciences, University of Porto, Porto, Portugal
Gastric and colorectal cancers have a high incidence and mortality worldwide. The presence of cancer stem cells (CSCs) within the tumor mass has been indicated as the main reason for tumor relapse, metastasis and therapy resistance, leading to poor overall survival. Thus, the elimination of CSCs became a crucial goal for cancer treatment. The identification of these cells has been performed by using cell-surface markers, a reliable approach, however it lacks specificity and usually differs among tumor type and in some cases even within the same type. In theory, the ideal CSC markers are those that are required to maintain their stemness features. The knowledge that CSCs exhibit characteristics comparable to normal stem cells that could be associated with the expression of similar transcription factors (TFs) including SOX2, OCT4, NANOG, KLF4 and c-Myc, and signaling pathways such as the Wnt/β-catenin, Hedgehog (Hh), Notch and PI3K/AKT/mTOR directed the attention to the use of these similarities to identify and target CSCs in different tumor types. Several studies have demonstrated that the abnormal expression of some TFs and the dysregulation of signaling pathways are associated with tumorigenesis and CSC phenotype. The disclosure of common and appropriate biomarkers for CSCs will provide an incredible tool for cancer prognosis and treatment. Therefore, this review aims to gather the new insights in gastric and colorectal CSC identification specially by using TFs as biomarkers and divulge promising drugs that have been found and tested for targeting these cells.
Introduction
Gastrointestinal malignancies are listed among the major causes of cancer death worldwide, being associated with environmental and genetic risk factors such as older age, chronic inflammation, family history, smoking, dietary patterns, overweight and physical inactivity, as well as gut microbiota (Lochhead and El-Omar, 2008; Mattiuzzi et al., 2019). Gastric cancer (GC) and colorectal cancer (CRC) are among the top five most incident and deadly cancers worldwide (Bray et al., 2018). As surgical techniques improve, as well as radiotherapy, chemotherapy and neoadjuvant therapies, the 5-year survival rate can reach up to 95% for early GC and 90% for localized CRC (Song et al., 2017; Sonbol et al., 2019). However, most patients have advanced-stage disease at diagnosis and so the best surgical window is missed (Song et al., 2017; Bray et al., 2018). They develop recurrent loco-regional disease or distant metastases with consequent decrease in survival. The heterogeneity of cancer at molecular, histological and phenotypic levels plays an important role in therapy resistance and tumor recurrence, being cancer stem cells (CSCs) among the major causative factors of cancer heterogeneity and treatment failure (Iseghohi, 2016; Gullo et al., 2018). The CSC model of tumor progression hypothesizes that a small subpopulation of cancer cells that display stem-like properties sustains tumor growth, metastasis, relapse and resistance to chemotherapy (Iseghohi, 2016). CSCs can undergo symmetric and asymmetric divisions, having the ability to give rise to all the different types of cancer cells within the tumor (Marjanovic et al., 2013). The origin of CSCs is still unclear and controversial (Dalerba et al., 2007a; Brunner et al., 2012). Various hypotheses suggest that depending on the tumor type, CSCs might be derived from adult stem cells, adult progenitor cells that underwent mutations, or differentiated cells that gained stem-like properties through dedifferentiation (Basu et al., 2016; Phi et al., 2018). A large number of studies demonstrate that CSCs share biomarkers with normal stem cells, thus specific markers for their identification have been explored in recent years. It is known that some transcription factors (TFs) can be re-expressed or reactivated in CSCs, playing a crucial role in the reprogramming of these cells. This review aims to provide a better understanding on how TFs associated with gastric and colorectal CSCs phenotype can be used for CSCs identification, characterization and targeted therapy.
The Challenges of Cancer Stem Cell Identification
The identification of normal stem cells is now an easy process due to their well-recognized set of biomarkers whereas the identification of CSCs is a challenging task resulting from their complex phenotype, that differs from one tumor to another (Pattabiraman and Weinberg, 2014). Additionally, CSCs represent a very small percentage of tumor cells within the total tumor mass making even harder their detection in heterogeneous tumors (Kim Y. et al., 2009). The use of different combinations of cell-surface markers has been the main strategy to identify CSCs in several tumor types (Magee et al., 2012). The cell-surface markers are chosen according to their expression and relevance in the tumor type allowing the separation of CSCs from non-CSCs (Pattabiraman and Weinberg, 2014). In GC and CRC, the list of cell-surface markers capable of identifying CSCs is growing (Table 1). This means that the interest in gastric and colorectal CSCs identification and targeting is rising but also means that the already used markers are not uniformly advantageous for CSCs detection. In particular, inconsistencies remain concerning which cell-surface marker may be the ideal marker to identify gastric and colorectal CSCs from cell lines and primary tumors (Takaishi et al., 2009; Zhang et al., 2011; Jiang Y. et al., 2012; Rocco et al., 2012; Wakamatsu et al., 2012; Brungs et al., 2016).
Gastric Cancer
Several cell-surface markers have emerged for gastric CSC identification. The transmembrane glycoprotein CD44 was the first described cell-surface marker used in gastric CSC identification. Takaishi et al. (2009) and his collaborators analyzed a panel of six GC cell lines and in three of them – NCI-N87, MKN-45 and MKN-74 – a small CD44+ cell subpopulation displayed CSC features such as self-renewal, asymmetric division, spheroid colony formation, and in vivo tumorigenic ability. They also observed that the CD44+ subpopulation had a higher resistance to anticancer drugs when compared to CD44– cells (Takaishi et al., 2009). However, in the other three cell lines – AGS, Kato III and MKN28 – the CD44 cell-surface marker was not able to mark cells with stem cell properties (Takaishi et al., 2009). Clinically, CD44+ cancer cells at the invasive GC front are associated with poor patient survival (Nosrati et al., 2014; Kodama et al., 2017). Later, Zhang et al. (2011) combined CD44 with CD24, a signal transducer, and successfully detected a CD44+CD24+ cellular subpopulation with CSCs characteristics, such as the capability to self-renew and to originate differentiated progeny (Zhang et al., 2011). Additionally, they showed that CD44+CD24+ cells had higher ability to form tumors when injected into immunodeficient mice, compared to the CD44–CD24– cells (Zhang et al., 2011). The CD54 cell-surface marker, also known as ICAM-1 (intercellular adhesion molecule 1), was combined with CD44 to isolate gastric CSCs from tumor tissues and peripheral blood of patients with GC (Chen et al., 2012). The CD44+CD54+ cells exhibited in vitro and in vivo self-renewal ability, formed gastric tumorspheres and originated tumors similar to the original human tumor when injected into immunodeficient mice (Chen et al., 2012). The epithelial cell adhesion molecule (EpCAM) has also been used in combination with CD44 to mark gastric CSCs. The small EpCAM+/CD44+ subpopulation isolated from primary human GC tissues was more resistant to anticancer drugs including 5-fluorouracil (5-FU), doxorubicin, vinblastine and paclitaxel, when compared with EpCAM+/CD44–, EpCAM–/CD44+ and EpCAM–/CD44– cells (Brabletz et al., 2005; Han et al., 2011). It also showed capacity to form sphere-like structures in serum free conditions and greater ability to originate tumors in immunocompromised mice (Han et al., 2011). The tumors formed after inoculation of the EpCAM+/CD44+ cells recapitulated the heterogeneous morphology and phenotype present in the original gastric tumor (Han et al., 2011). Moreover, Fukamachi et al. (2013) identified another potential gastric CSC marker, the CD49f, an integrin α6 (ITGA6) that is a subunit of laminin receptors. Their work showed that CD49f+ cells from GC originated tumors when subcutaneously injected into immunodeficient mice, while CD49f– cells did not (Fukamachi et al., 2013). They also demonstrated that some of the CD49f+ sphere-forming cells were more resistant to doxorubicin, 5-FU and doxifluridine than the other GC cells studied (Fukamachi et al., 2013). Another cell-surface marker identified as a gastric CSC marker is the CD71 transferrin receptor. In this case, it was demonstrated that the CD71– subpopulation from the MKN-1 GC cell line displayed CSC features, contrary to CD71+ cells. The CD71– cells were more resistant to 5-FU than CD71+, had higher tumorigenic ability and were mostly present in the invasive front of the tumor (Ohkuma et al., 2012). The cell-surface glycoprotein CD90 (Thy-1) appeared as a potential gastric CSC marker since it was capable of identifying a small population with in vivo tumorigenic and self-renewal ability (Jiang J. et al., 2012). Additionally, 25% of the gastric primary tumors possessed higher expression of erb-b2 receptor tyrosine kinase 2 (HER2), which was correlated with the higher expression of CD90 (Jiang J. et al., 2012). CD133 (prominin-1), a pentaspan transmembrane glycoprotein, is described as a gastric CSC marker due to the fact that its expression is positively correlated with tumor aggressiveness in GC patients (Fukamachi et al., 2011; Lee et al., 2012; Wakamatsu et al., 2012; Hashimoto et al., 2014; Nosrati et al., 2014). Zhao et al. showed that the frequency of CD133+ in gastric primary tumors samples was higher than CD133– cells and CD133 was associated with poor prognosis in GC (Zhao et al., 2010). Also, spheroid cells from GC cell lines and primary GC tissues presented CD133 expression and displayed several features of CSCs (Zhang X. et al., 2016). New cell-surface markers have emerged in the study of gastric CSCs and demonstrated to be able to mark a small population in GC with stem-like features, specifically Lgr5 (leucine-rich repeat-containing G-protein coupled receptor 5) and CXCR4 (C-X-C chemokine receptor type 4) also known as CD184 (Fujita et al., 2015; Gong et al., 2016). Also, the intracellular enzyme aldehyde dehydrogenase (ALDH) has been used to identify gastric CSCs (Zhi et al., 2011; Wakamatsu et al., 2012). Zhi et al. (2011) were able to divide NCI-N87 and SNU-1 GC cell lines into ALDH+ and ALDH– cells. The ALDH+ cells presented CSC features such as higher levels of SOX2, NANOG and Nestin, formed more sphere-like structures and had higher resistance to 5-FU and cisplatin (Zhi et al., 2011). They also showed that ALDH+ cells were more sensitive to salinomycin, a drug proposed to target CSCs (Zhi et al., 2011).
Colorectal Cancer
Several specific cell-surface markers were used to identify colorectal CSCs (Todaro et al., 2010; Vaiopoulos et al., 2012; Rassouli et al., 2016; Wahab et al., 2017; Zhou et al., 2017; Boesch et al., 2018; Munro et al., 2018; Parizadeh et al., 2019; van der Heijden and Vermeulen, 2019). CD133 was shown to be a robust CSC-surface marker in CRC (O’Brien et al., 2007; Ricci-Vitiani et al., 2007; Akbari et al., 2020). Positive expression of CD133 was, for the first time, associated with a significantly worse survival and poorer clinical response to 5-FU-based chemotherapy in CRC patients (Ong et al., 2010). CD44 is also a valid marker of colorectal CSCs (Du et al., 2008). In combination with EpCAM it has already been considered a better strategy, when compared to CD133 (Dalerba et al., 2007b). Furthermore, CD166 (another transmembrane glycoprotein) could be used to co-purify CSCs (Dalerba et al., 2007b). More recently, colonospheres and chemoresistant CRC cells were found to be enriched with CD133 and CD44 (Huang et al., 2015). A strategy that combines CD133 with CD44, seems to be more reliable to isolate colorectal CSCs from both cell lines and primary tumors (Abbasian et al., 2019). Horst et al. (2009) showed that CD133 may have a better prognostic capacity per se, but the combination of CD133, CD44, and CD166 markers may stratify better the risk of CRC. Lgr5 positivity identifies human colorectal CSCs and is a prognostic factor for CRC (Kemper et al., 2012; Jiang Y. et al., 2016). Lgr5 overexpression has been shown to be important in CRC progression, as a result of its role in potentiating the Wnt signaling pathway (Carmon et al., 2012). de Sousa e Melo et al. (2017) have shown that Lgr5+ CSCs are crucial for the formation of liver metastases, while in the primary tumor Lgr5– cells proliferate and are capable of originating Lgr5+ cells, assuring a rapid tumor growth when treatment ends. Lgr5 combined with CD44 and EpCAM can further improve the identification of CSCs in CRC (Leng et al., 2018). Vermeulen et al. (2008) have shown that spheroid cultures of colon CSCs express CD133, CD166, CD44, CD29, CD24, Lgr5, and nuclear β-catenin, all of which described markers of the CSC population. Also, CD200 showed a role as a CSC marker in colon cancer, and CD49f was associated with tumor cell invasion and metastasis in colon cancer working as an important marker for identifying colorectal CSCs (Robertson et al., 2009; Haraguchi et al., 2013; Zhang S.S. et al., 2016). ALDH1 also appears as a specific marker for colonic CSCs and as an independent prognostic factor for patients with CRC (Huang et al., 2009; Lugli et al., 2010; Kahlert et al., 2012; Goossens-Beumer et al., 2014; Zhou et al., 2017; Munro et al., 2018). In patients with stage II-III rectal cancer that received radio-chemotherapy, ALDH1 expression was correlated with poor prognosis, cancer relapse and metastasis (Huang et al., 2009; Deng et al., 2014).
Transcription Factors as Potential CSC Markers
One of the challenges to target CSCs is to identify specific markers as well as to uncover targetable molecular features associated with their phenotype. It is known that normal stem cells and CSCs share core stemness signaling pathways such as Notch, Hedgehog, WNT/β-catenin and JAK/STAT, that have pivotal roles in maintaining stem cell properties and regulating their transcriptional program (Chen K. et al., 2013). SOX2, OCT4, KLF4, and NANOG are some of the key TFs known to promote stemness by upregulating genes involved in self-renewal and pluripotency, while suppressing genes involved in differentiation (Young, 2011; Tang et al., 2015; Buczek et al., 2018). Key stem cell TFs like SOX2, OCT4, and NANOG have been proven to be overexpressed in CSCs. For that reason, fluorescence reporter systems driven by portions of promoters where these proteins bind were developed to allow CSCs to be labeled and tracked in various types of cancer. These reporter systems seem to be a powerful tool to identify and study CSCs more efficiently than cell-surface markers (Saygin et al., 2016). Tang et al. (2015) developed a flexible lentiviral-based reporter system (SORE6-GFP) that allows direct visualization of CSCs based on SOX2 and OCT4 expression. By using this novel reporter system, our group was able to isolate gastric CSCs from two phenotypically different GC cell lines (AGS and Kato III) (Pádua et al., 2020). Using the same principle, other authors have been developing similar systems to identify and characterize CSCs in a variety of solid tumors. Buczek et al. (2018) established a method using a lentiviral construct carrying the promoter of NANOG to identify prostate CSCs and more recently, Ghanei et al. (2020) introduced a similar approach for the isolation of CSCs in a breast cancer cell line based on the single expression of OCT4. Although the use of cell-surface markers is the most trusted approach to detect these cells, several studies demonstrated that they lack specificity and cannot be used for real time assessment of CSCs behavior, which could give new insights about their properties and possible targets (Tang et al., 2015). Growing evidences support that specific TFs overexpressed in normal gastrointestinal stem cells may contribute to the self-renewal characteristics of CSCs in GC and CRC and are related with patient prognosis (Hadjimichael et al., 2015; Zhao et al., 2017). This makes them a powerful tool in CSCs identification and study.
SOX2 (SRY-Box Transcription Factor 2)
SOX2 is a master regulator that belongs to the family of high-mobility group TFs. It plays many roles throughout development and cell differentiation in normal tissues, namely in mammalian embryogenesis, morphogenesis and homeostasis of the foregut-derived epithelia of the esophagus, lung and trachea (Avilion et al., 2003; Sarkar and Hochedlinger, 2013). SOX2 role in stemness was strengthen with Takahashi and Yamanaka’s findings when reprogramming mouse embryonic fibroblasts into induced pluripotent stem (iPS) cells, by introducing SOX2 along with OCT3/4, c-Myc and KLF4 (Takahashi and Yamanaka, 2006). In cancer, SOX2 has increasingly been associated with a CSC phenotype in several tumors (Wuebben and Rizzino, 2017; Takeda et al., 2018). In GC, SOX2 role is still controversial: some authors associate its high expression with a more aggressive phenotype, poor prognosis and worse response to therapy whereas others have shown the opposite (Hütz et al., 2013; Camilo et al., 2014; Carrasco-Garcia et al., 2016; Zhang X. et al., 2016; Wuebben and Rizzino, 2017; Basati et al., 2020; Pádua et al., 2020). Our group identified subpopulations of gastric CSCs in two human cell lines based on the expression of SOX2 and showed that SORE6+ cells presented CSCs properties, including higher proliferation and ability to form gastrospheres, enhanced in vivo tumorigenesis and increased resistance to 5-FU (Pádua et al., 2020). Additionally, Hütz et al. (2013) observed that inhibition of SOX2 resulted in reduced cell proliferation and migration, increased apoptosis, changes in cell cycle and reduced tumorigenic potential of cells in vivo. Similar results were observed in vivo, where suppression of SOX2 resulted in reduced tumor growth and decreased tumorigenicity (Tian et al., 2012; Hütz et al., 2013). In CRC, SOX2 overexpression has been associated with tumor progression and disease recurrence and SOX2 de novo expression was associated with poorly differentiated and more invasive tumors and poor patient overall survival (Lundberg et al., 2014; Lundberg et al., 2016). Takeda et al. (2018) have shown that SOX2+ cells developed chemoresistance to oxaliplatin and 5-FU, exhibiting typical asymmetric cell division and higher CSC markers expression. They concluded that colon cancer cells expressing SOX2 behave like CSCs and are therefore associated with poor prognosis (Lundberg et al., 2016; Takeda et al., 2018). Taken together, these findings indicate that SOX2 has a critical role in several aspects of CSCs biology.
OCT4 (POU Class 5 Homeobox 1)
OCT4, a homeodomain TF of the Pit-Oct-Unc family, is expressed in embryonic stem cells, where it maintains stem cell-like properties, and in adult stem cells, being involved in their proliferation and differentiation (Loh et al., 2006; Han et al., 2014). Abnormal expression of OCT4 has been observed in different tumor types, including GC and CRC (Dai et al., 2013; Basati et al., 2020). Studies report that its expression is positively correlated with more aggressive and metastatic tumors, as well as with poorer overall prognosis (Zhang X. et al., 2016; Basati et al., 2020). Chen and collaborators demonstrated that overexpression of OCT4 in GC cells led differentiated cancer cells to become undifferentiated and acquiring self-renewal capacity (Chen et al., 2009) and another study reported that downregulation of OCT4 induced differentiation in GC cells (Tai et al., 2005). Additionally, Zhang X. et al. (2016) demonstrated a p-ERK mediated positive feedback loop between the cell surface marker CD44 and OCT4, responsible for sustaining gastric CSCs properties. OCT4 has also been reported in colon CSCs and its knockdown inhibits cell migration and invasion, suggesting it may act as a novel prognostic marker in CRC (Miyoshi et al., 2010, 2018; Dai et al., 2013; Amini et al., 2014).
KLF4 (Kruppel Like Factor 4)
KLF4, also known as the gut-enriched Kruppel like factor (GKLF), is strongly expressed in post-mitotic and terminally differentiated epithelial tissues, along with those of the gastrointestinal tract (Cho et al., 2007; Cui et al., 2013). It is suggested that it may have an anticancer role in GC, being downregulated due to hypermethylation and loss of heterozygosity in gastric CSCs (Cho et al., 2007). Several studies have shown that KLF4 low expression is negatively associated with patient overall survival and may be a useful prognostic marker in GC patients (Li et al., 2012; Zhang et al., 2012; Hsu et al., 2013; Hashimoto et al., 2017; Zhao et al., 2020). Regarding CRC, KLF4 role is still not clear. Some studies reveal KLF4 is overexpressed in colon CSCs and its knockdown affects the stemness phenotype and decreases the cells malignant profile, while others demonstrate that loss of expression is associated with stem-like features namely formation of colonospheres, cell growth arrest, uncontrolled cell proliferation, pluripotency and self-renewal (Shie et al., 2000; Wei et al., 2006; Leng et al., 2013; Hadjimichael et al., 2015).
NANOG (Nanog Homeobox)
NANOG was first discovered in embryonic stem cells (ESCs) and is a key TF involved in self-renewal and multipotency (Chambers et al., 2003). It is typically silenced in normal somatic cells, though abnormal expression has been reported in malignant tumors, such as GC and CRC (Lin et al., 2012; Hadjimichael et al., 2015). Previous studies demonstrate that NANOG is highly expressed in GC and significantly associated with tumor size and grade, along with decreased overall survival (Iv Santaliz-Ruiz et al., 2014; Basati et al., 2020). Although studies indicate NANOG is associated with a CSC phenotype, it remains unclear its role in CSC maintenance in GC (Iv Santaliz-Ruiz et al., 2014). With respect to CRC, NANOG overexpression has been associated with colony formation and stem cell properties, as well as worse prognosis (Ibrahim et al., 2012; Zhang et al., 2013; Hadjimichael et al., 2015).
c-Myc (MYC Proto-Oncogene, bHLH Transcription Factor)
c-Myc is an essential TF that regulates genes that take part in biological processes such as self-renewal, differentiation, growth and metabolism (Dang, 2013; Bretones et al., 2015). Although it is one of the most commonly activated oncogenes involved in the pathogenesis of cancer, its overexpression alone is unable to induce the transformation of normal cells into tumor cells (Yang et al., 2020). The role of c-Myc in GC is less studied than in other tumor types. It has been suggested as a CSC marker in some tumors such as small-cell lung cancer, prostate cancer, neuroblastoma, glioblastoma and hematopoietic malignancies, but the expression and relevance in GC has not yet been clarified (Yang et al., 2020). Some authors associate c-Myc deregulation with poor prognostic features (Han et al., 1999; de Souza et al., 2013; Wang et al., 2016). Upregulation of c-Myc is common in 70% of CRC cases and it has been shown to have a crucial role in maintaining chemoresistance and self-renewal, being overexpressed in colon CSCs (Muzny et al., 2012; Zhang et al., 2019). Despite some controversial results, it has been shown that high expression of c-Myc is an independent poor prognostic factor in CRC (Lee et al., 2015; Wang et al., 2017).
SOX9 (SRY-Box Transcription Factor 9)
SOX9 regulates developmental processes such as male sex determination, chondrogenesis, neurogenesis, and neural crest development (Jo et al., 2014). Also, it plays a vital role in cell fate decisions and stem cell maintenance during embryonic development and adulthood in several organs, including the gastrointestinal tract (Bastide et al., 2007; Huch and Clevers, 2011). Its role in GC is still conflicting, while some studies defend an association between lower survival and SOX9 high expression, others demonstrate poor prognosis with a decreased level of SOX9 expression (Sun et al., 2012; Santos et al., 2016; Mesquita et al., 2019). Also, both oncogenic and tumor suppressor activity of SOX9 have been implicated in CRC (Darido et al., 2008; Lu et al., 2008; Matheu et al., 2012; Prévostel and Blache, 2017). Previous studies suggest that this TF can influence tumor proliferation and progression, mainly through the regulation of the CSC pool, and could correlate with poor prognosis (Lu et al., 2008; Espersen et al., 2015; Javier et al., 2016).
GLI1 (GLI Family Zinc Finger 1)
GLI1 is part of the Sonic Hedgehog (SHH) pathway and seems to be essential for the maintenance of cancer cells with stem like properties in both GC and CRC (Zhang X. et al., 2016; Yang et al., 2018). In GC, its expression is significantly higher in metastatic cancer tissues and is positively correlated with a more aggressive tumor phenotype (Zhang X. et al., 2016). Furthermore, it has been observed that GLI1 overexpression promotes a CSC phenotype enhancing cell proliferation, migration and therapy resistance (Dong et al., 2019; Yao et al., 2019). GLI1 also plays an important role in CSC characteristics related with aggressiveness and metastatic spread of CRC cells leading to decreased survival. Furthermore, GLI1 knockdown downregulates CD133/SOX9 expression and clonogenic ability of CRC cells, indicating this TF could be a potential marker for CSCs in CRC (Yang et al., 2018).
STAT3 (Signal Transducer and Activator of Transcription 3)
STAT3 plays an important role in the regulation of various physiological functions, such as inflammation, proliferation and invasion, being highly expressed in gastric and colorectal CSCs (Yu H. et al., 2009; Lin et al., 2011; Hajimoradi et al., 2016; Sonbol et al., 2019). In gastric CSCs, high expression of STAT3 has been reported to be involved in stemness properties and invasive ability (Hajimoradi et al., 2016; Jiang et al., 2017; Sonbol et al., 2019). Regarding its prognostic value several studies report STAT3 activation as a marker of unfavorable outcome (Kim D.Y. et al., 2009; Deng et al., 2010, 2013; Ji et al., 2016). In CRC, STAT3 is one of the major oncogenic proteins associated with proliferation, angiogenesis, invasion and chemo-radiotherapy resistance (Lin et al., 2005, 2011; Munro et al., 2018). Also, its inhibition prevents tumor initiation, being an attractive therapeutic target for CRC (Lin et al., 2011).
SALL4 (Spalt Like Transcription Factor 4)
As a TF, SALL4 plays essential roles in maintaining pluripotency and self-renewal of ESCs, being downregulated or silenced in differentiated cells (Zhang et al., 2006, 2015; Yang et al., 2008). SALL4 acts as an oncogene and it is associated with cancer initiation, development, and progression (Ma et al., 2006). Zhang et al. (2014) showed that the overexpression of SALL4 is associated with gastric CSC features and could be involved in the generation and maintenance of these cells. Later, Yuan et al. (2016) suggested a novel mechanism for SALL4 role in GC, showing that this TF binds to the promoter region of CD44 and activates it expression, enhancing cell proliferation, migration and invasion. Increasing evidence indicates that upregulation of SALL4 is associated with lymph node metastasis and poorer overall prognosis (Zhang et al., 2014, 2018b). In CRC, SALL4 overexpression is detected in 87% of tumor tissues and it is correlated with tumor cell metastasis to lymph nodes being associated with poor prognosis and showing its essential role in maintaining the properties of CSCs (Forghanifard et al., 2013; Zhang et al., 2015).
β-Catenin (Catenin Beta 1)
The Wnt/β-catenin pathway is implicated in the regulation of the epithelial stem cell self-renewal (Behrens et al., 1996). Alone, β-catenin signaling has been shown as necessary for the maintenance of CSC features (Huang et al., 2007; Kanwar et al., 2010; Jiang R. et al., 2016). The dysregulation of the Wnt/β-catenin signaling pathway has been implicated in colon carcinogenesis and plays a critical role in regulating the growth and maintenance of colonospheres (Kolligs et al., 2002; Kanwar et al., 2010). The activation of this pathway can lead to the conversion of intestinal stem cells into CSCs, where expression levels of β-catenin are higher (Kanwar et al., 2010). Some studies revealed that high levels of nuclear β-catenin, in CRC patients, were associated with a poor prognosis and could be used as a biomarker for late phase CRC (Chen Z. et al., 2013). Gastric CSCs self-renewal and proliferation ability, both in vitro and in vivo, are also improved by the Wnt/β-catenin signaling (Mao et al., 2014; Chiurillo, 2015).
CSCs and Tumor Microenvironment
The close interaction between CSCs and their niche is fundamental for maintaining the stemness of CSCs and tumor progression. The CSC niche, a specific tumor microenvironment, which consists of stroma, micro-vessels, hypoxic regions, cancer-associated fibroblasts (CAFs), cancer-associated mesenchymal stem cells (MSCs), tumor-associated macrophages (TAMs) and extracellular matrix, secretes soluble factors (e.g., cytokines and growth factors) that are necessary for cancer cell survival (Quante et al., 2013; Lau et al., 2017; Yang et al., 2020). Growth factors and cytokines regulate Wnt, Notch, JAK-STAT3 and other signaling pathways thereby stimulating growth, epithelial-to-mesenchymal transition (EMT), invasion, angiogenesis, metastasis and inhibiting apoptosis (Yang et al., 2020). These pathways are required for the self-renewal and maintenance of CSCs. For instance, growth factors like hepatocyte growth factor (HGF), secreted by the stromal myofibroblasts, activate the Wnt-signaling in a subset of colon cancer cells that maintain the CSC phenotype (Vermeulen et al., 2010). Another example is hypoxia, which also maintains a stem-like phenotype in CRC and GC through the increased expression of hypoxia-inducible factors (HIFs), the transcription factors HIF-1α and HIF-2α, that maintain the Wnt/β-catenin signaling pathway and activate stemness-related TFs such as OCT4 (Gidekel et al., 2003; Liu et al., 2008; Mazumdar et al., 2010; Yeung et al., 2011; Vadde et al., 2017). On the other hand, cancer cells also secrete growth factors and proteases to promote changes in their microenvironment (Ishimoto et al., 2014). One significant example of this crosstalk between cancer cells and the microenvironment is the secretion of cytokines like interleukin-6 (IL-6) by the cancer-associated mesenchymal stem cells that enhance the progression of CRC through the IL-6/JAK2/STAT3 signaling (Zhang et al., 2018a). The same is observed in GC through the secretion of interleukin-8 (Li W. et al., 2015). On the other hand, cancer cells mediate the production of inflammatory cytokines with pro-tumorigenic roles or the inhibition of cytokines involved in immune surveillance, altering the composition of the immune cells in the tumor microenvironment (Quante et al., 2013; West et al., 2015). Specifically, Rezalotfi et al. (2019) suggested that CSCs could alter the cytokines in the tumor microenvironment by demonstrating that the balance between suppressive regulatory T cells (Treg) and T helper cells producing IL17 (Th17) could be affected. Chaudhry et al. (2009) disclosed further that STAT3 is fundamental for the inhibition of Treg cells development and Th17 differentiation. In fact, the STAT3 transcription factor, in collaboration with NFκB, regulates the expression of these genes encoding critical cancer-promoting inflammatory mediators, establishing a crosstalk between cancer and immune cells of the microenvironment and perpetuating the effects of STAT3 activation in cancer cells (Yu et al., 2007; Grivennikov and Karin, 2010; Yang et al., 2019). However, despite the growing evidences on the interaction of gastric and colorectal CSCs with the tumor microenvironment, the specific molecules involved and their signaling pathways still need further investigation in order to design safe therapies.
Therapeutic Approaches to Target CSCs
The therapeutic approach in GC and CRC is determined by the stage of the disease at the time of diagnosis. Patients are treated with surgery, chemotherapy and/or radiation. In GC, surgery remains the main treatment for stage I. It can also be performed at stages II and III but chemotherapy (perioperative, neoadjuvant or adjuvant) is necessary to improve overall survival of the patients. For stage IV, chemotherapy with doublet or triplet platinum/fluoro-pyrimidine combinations or capecitabine is the main treatment (Neri et al., 2007; Smyth et al., 2016). Resective surgery is the main curative treatment used in non-metastasized CRC, although neo-adjuvant treatments are also administered in rectal carcinoma (Kuipers et al., 2015). After surgery, 5-FU-based chemotherapy is used to reduce the risk of tumor recurrence and improve overall survival of patients (Dienstmann et al., 2017). Currently, the decision of giving adjuvant treatment to early-stage CRC patients is recommended to high risk patients with one or more risk factors (Kannarkatt et al., 2017). Patients with very high risk – microsatellite stable (MSS) and T4 may be considered for the addition of oxaliplatin (Labianca et al., 2013). However, in some cases of GC and CRC, after a believed efficacious treatment, the cancer reappears locally or in distant metastasis. This results from the presence of CSCs that were able to resist the therapy applied, revealing that the existence of CSCs is one of the biggest difficulties in cancer treatment (Dean et al., 2005). Thus, direct targeting of CSCs seems to be the key for tumor complete elimination. The therapy against CSCs using specific molecules should eradicate these cells while the conventional therapy eliminates the non-CSCs present in the tumor bulk. Nevertheless, this type of treatment should be administrated in combination due to the possibility of cell plasticity that facilitates the appearance of de novo CSCs from non-CSCs. Moreover, targeting specific TFs or signaling pathways responsible for maintaining the CSC phenotype could become novel therapies against GC and CRC. For that reason, several clinical trials are being undertaken to explore the efficacy of diverse compounds, that are capable of modulating or inactivating proteins that gastric and colorectal CSCs use to grow and survive, allowing their elimination. Additionally, it is worth to mention that several studies have been conducted to evaluate the efficacy of small molecules in targeting CSCs in vitro and in vivo, compounds capable of eliminating or reducing the CSC population that could also be part of the path in cancer therapy targeting CSCs (Gupta et al., 2009; Abetov et al., 2015; Shapiro et al., 2016; Müller et al., 2017; Park et al., 2017; Pádua et al., 2020). Table 2 lists the drugs that have been or are being investigated in clinical trials, alone or in combination with other compounds, to treat GC and CRC.
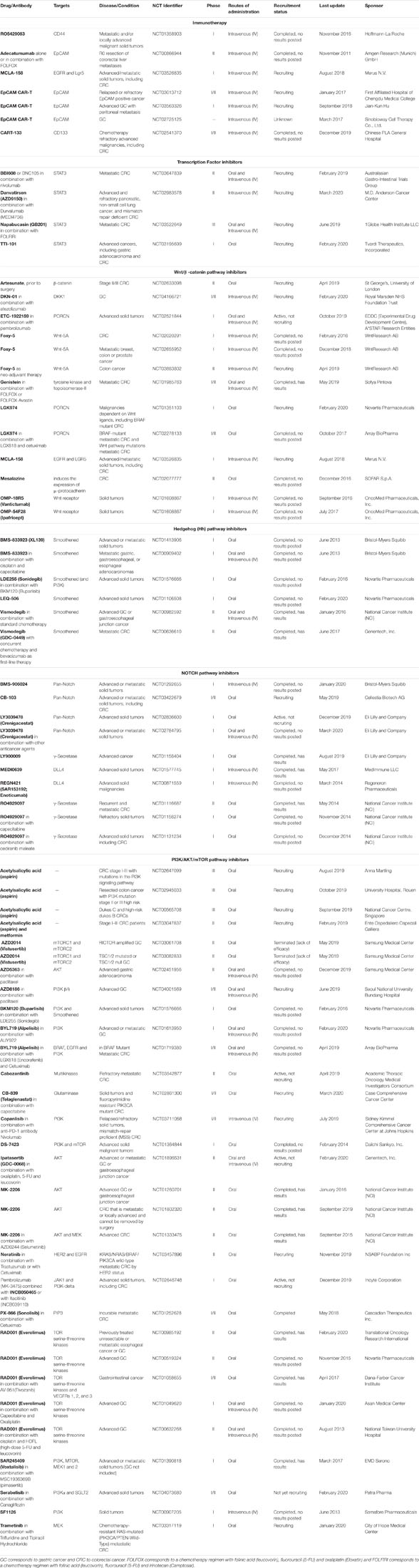
Table 2. Complete and ongoing clinical trials of therapeutic agents targeting gastric and/or colorectal CSCs, correlated signaling pathways and the transcription factor STAT3.
Immunotherapy
Cancer immunotherapy has emerged as a potential tool for cancer treatment (Farkona et al., 2016). Several immunotherapeutic strategies have been developed including cancer vaccines, oncolytic viruses, monoclonal antibodies or recombinant proteins, chimeric antigen receptor T cell (CAR-T) cells and other cellular therapies, lymphocyte-activating cytokines and checkpoint inhibitors (Riley et al., 2019). Immunotherapy aims to improve the immune system response against cancer cells through natural mechanisms (Riley et al., 2019). Therefore, it can be used to target cancer cells and also CSCs in the tumor microenvironment (Badrinath and Yoo, 2019). Many immunotherapeutic agents targeting CSCs have been tested in clinical trials (Menon et al., 2016). Monoclonal antibodies that specifically target CSC surface biomarkers have been used in gastric and colorectal cancer. Ongoing there is a Phase I study of RO5429083, a monoclonal antibody against CD44 in patients with metastatic and/or locally advanced malignant solid tumors (NCT01358903). Also used were EpCAM antibodies, such as edrecolomab, that was tested in patients with resected stage II adenocarcinoma of the colon (Niedzwiecki et al., 2011) and adecatumumab, that is being tested in a Phase II study to evaluate efficacy and safety, alone or with FOLFOX, in metastasized CRC (NCT00866944). A Phase I dose finding study is evaluating the bispecific antibody targeting EGFR and Lgr5 (MCLA-158) in metastatic CRC and other advanced solid tumors (NCT03526835). In addition, CAR-T cell therapies, have been developed to target CSCs in GC and CRC. There are three Phase I or II clinical trials in GC using CAR-T cells targeting EpCAM (NCT03013712, NCT03563326, and NCT02725125), one of them consisting in the intraperitoneal infusion in advanced GC with peritoneal metastasis. NCT03013712 includes patients with colon cancer. Moreover, there is a Phase I/II clinical study of CAR-T cells targeting CD133 in relapsed and/or chemotherapy refractory malignancies including CRC (NCT02541370).
Targeting the Transcription Factor STAT3
From the list of TFs strongly associated with CSC phenotype in GC and CRC, STAT3 became crucial as a molecular target for cancer therapy because napabucasin (BBI608), the first-in-class cancer stemness (CSCs) inhibitor that works by targeting STAT3, effectively blocks cancer relapse and metastasis in xenografted human cancers (Li Y. et al., 2015; Zhang Y. et al., 2016). Napabucasin is a naturally occurring drug orally administered. It inhibits CSC self-renewal and induces apoptosis in CSCs by targeting CSC signaling pathways (STAT3, NANOG and β-catenin) in GC (Bekaii-Saab and El-Rayes, 2017). In CRC, napabucasin has been investigated in several studies. A phase I/II clinical trial investigated napabucasin with other standard therapeutic treatments for advanced gastrointestinal malignancies (Bendell et al., 2017). Another study – CanStem303C – a randomized phase III clinical trial done in adult patients with previously treated metastatic CRC evaluated napabucasin in combination with FOLFIRI (Grothey et al., 2017). Napabucasin monotherapy has been reported in a published phase III trial. The CO.23 trial evaluated the efficacy of napabucasin monotherapy versus placebo in metastasized CRC, which failed to demonstrate a significant difference in the napabucasin group survival. However, in a pre-specified biomarker analysis, phosphorylated STAT3 (pSTAT3)-positive patients experienced a significant survival benefit from napabucasin over placebo (Jonker et al., 2018; Sonbol et al., 2019). Another ongoing trial involving STAT3 inhibition is the phase II trial MODULATE (NCT03647839) which specifically aims to study the modulation of the tumor microenvironment using either vascular disrupting agents (BNC105) or STAT3 inhibition (BBI608), in synergy with an immune checkpoint protein (PD1) inhibitor (nivolumab). This trial is recruiting microsatellite stable, refractory CRC cases.
Targeting the Wnt/β-Catenin Signaling Pathway
Wnt/β-catenin signaling pathway is a major regulator of normal intestinal development and its over-activation behaves as a hallmark of CRC, being particularly significant in drug resistance and stemness maintenance of colorectal CSCs (Takebe et al., 2011; Basu et al., 2016). The activation of the Wnt/β-catenin signaling pathway is associated with poor prognosis (Janssen et al., 2006). It results mostly from the accumulation of mutations in the APC tumor suppressor gene, oncogenic KRAS-signaling pathway, β-catenin and p53. Mutations that do not allow the formation of the APC/Axin/GSK3β destruction complex result in the accumulation and nuclear translocation of β-catenin that binds to transcription factors of T cell factor family (TCF4) and activates target genes like c-Myc, cyclinD1 and survivin, some involved in maintaining stemness (Myant et al., 2013; Lee et al., 2015; Zhou et al., 2017). It has been shown that loss of APC in CRC triggered the expression of a Rac1 GTPase via the induced expression of c-Myc, necessary to intestinal stem cell proliferation and CRC initiation (Myant et al., 2013). On the other hand, p53 may affect the outcome of Wnt signaling in CRC development (Voorneveld et al., 2015), having a role in the acquisition of pluripotency during reprogramming (Takahashi and Yamanaka, 2006; Krizhanovsky and Lowe, 2009). The Wnt signaling pathway is also dysregulated in GC (Chiurillo, 2015). However, the involvement and mechanisms are not yet as fully understood as in CRC. A number of studies suggest β-catenin and APC as driver genes, revealing somatic mutations in both genes that might have relevance in GC (Horii et al., 1992; Nakatsuru et al., 1993; Woo et al., 2001; Clements et al., 2002; Zhang and Xue, 2008). Genomic analysis of several gastric primary tumors disclosed that Wnt/β-catenin, together with NF-κB and proliferation/stem cell pathways, were deregulated in more than 70% of the primary tumors. Patient stratification by combinations of these oncogenic pathways revealed to be a great tool for GC clinical behavior assessment (Ooi et al., 2009). Many molecules have been used to target the Wnt/β-catenin in gastrointestinal CSCs (Chiurillo, 2015; Parizadeh et al., 2019; Patel et al., 2019; Yang et al., 2020). Genistein, a soy-derived compound, was tested in a Phase I/II research trial, in combination with FOLFOX or FOLFOX-Bevacizumab, where it was demonstrated to be well tolerated by patients and may have improved efficacy in the treatment of metastatic CRC (Pintova et al., 2019). In vitro and in vivo studies have shown that Genistein affects mainly Wnt/β-catenin and PI3K/AKT pathways (Su et al., 2003; Kim et al., 2005; Zhang and Chen, 2011; Wang et al., 2012).
Targeting the Hedgehog Signaling Pathway
The dysregulation of Hedgehog (Hh) signaling pathway has been reported as another main cause of CSCs self-renewal and chemoresistance, being associated with poor clinical outcome in patients with GC or CRC (Ma et al., 2005; Varnat et al., 2009; Takebe et al., 2011; Usui et al., 2018). In this pathway, target gene expression is predominantly regulated by the Smoothened (SMO) protein but GLI inhibitors are also used (Rimkus et al., 2016; Didiasova et al., 2018). In GC cells, SMO regulates nuclear translocation of GLI-1 that in turn promotes transcription of target genes, such as CD44 (Yoon et al., 2014). Yoon et al. (2014) showed that in AGS, MKN-45, and NCI-N87 GC cell lines, the Hh pathway inhibition using SMO shRNA or small-molecule inhibitors significantly decreased spheroid formation ability and tumor growth. Vismodegib (GDC-0449), the first Hh pathway inhibitor used in cancer research, is currently undergoing Phase II trials in advanced GC and in metastatic CRC (Gupta et al., 2010; Berlin et al., 2013). Examination of tumor samples revealed that Vismodegib has not increased progression-free or overall survival as a whole, but only in a limited subset of patients with high CD44 expression (Cohen et al., 2013; Yoon et al., 2014). Disappointingly, these treatments with Vismodegib, did not increase progression-free survival in CRC patients (Low and de Sauvage, 2010; McMillan and Matsui, 2012). When the SMO inhibitor AY9944 was used in combination with the GLI-1 inhibitor GANT61, there was an increased response to anti-cancer drugs in tumor organoids and a decreased capacity to form colonies in SW480 and HCT116 CRC cells (Usui et al., 2018). These results indicate that this strategy might be worthwhile in CRC.
Targeting the Notch Signaling Pathway
The Notch signaling pathway is one of the most activated signaling pathways in cancer, namely in GC and CRC, and promotes metastization (Du et al., 2014; Hayakawa et al., 2019). It has a key role in the maintenance and differentiation of CSCs (Quail et al., 2012; Lu et al., 2013; Yang et al., 2020). In GC and CRC, the expression of Notch1 or Jagged1 is associated with poor prognosis (Yeh et al., 2009; Kang et al., 2012; Jackstadt et al., 2019; Kim et al., 2019; Mohamed et al., 2019). In CRC, Notch1 is associated with more aggressive subtypes, recruiting neutrophils to drive metastasis (Jackstadt et al., 2019). Due to the fact that the Notch pathway is related to CSC self-renewal and angiogenesis, targeting this pathway became a potential anti-CSC therapeutic approach (Venkatesh et al., 2018). Strategies used to inhibit Notch signaling include γ-secretase inhibition (GSI), Notch receptor (e.g., Notch1, Notch2, and Notch3) or ligand (e.g., Jagged1 and Jagged2) antibodies and combination therapy with inhibitors of other pathways. The inhibition of Notch receptors using two GSIs has allowed to disclose the importance of Notch pathway in the growth and survival of GC cells (Brzozowa et al., 2013). Furthermore, GSIs lead to the induction of apoptosis and inhibition of tumor-sphere formation of CD44+ gastric CSCs (Barat et al., 2017). However, it is important to mention that GSIs do not only target Notch-related proteins but also proteases involved in numerous cellular processes, which could originate adverse effects in vivo (Shih Ie and Wang, 2007; Wang et al., 2010; Brzozowa et al., 2013). Nevertheless, various clinical trials have been performed to evaluate the efficacy of targeting the Notch signaling pathway in GC and CRC (Table 2). RO4929097, a selective GSI, showed good anti-tumor activity in preclinical and early trials but it was not good enough for metastatic CRC (Luistro et al., 2009; Strosberg et al., 2012; Tolcher et al., 2012). Combinations of RO4929097 with other drugs in advanced solid tumors, including CRC, were well tolerated and presented some clinical benefit (Sahebjam et al., 2013; LoConte et al., 2015). LY900009 is another GSI tested in advanced cancers, including those of gastrointestinal tract. It is currently in a phase I trial and revealed a safety profile, with the majority of patients experiencing low-grade gastrointestinal adverse effects (Pant et al., 2016). Furthermore, it was demonstrated to have an inhibitory effect on Notch signaling pathway, inducing goblet cell differentiation and increased mucin production, similarly to that observed in rats (Pant et al., 2016). Moreover, MEDI0639 is a monoclonal antibody that specifically binds to DLL4 and prevents its interaction with Notch receptors, thereby inhibiting Notch-mediated signaling and target gene transcription, which may block tumor angiogenesis and eventually tumor cell growth (Ishigami et al., 2013). A phase I study in advanced solid tumors demonstrated that MEDI0639 is well tolerated and preliminary results show evidence of antitumor activity (Falchook et al., 2015).
Targeting the PI3K/AKT/mTOR Signaling Pathway
The PI3K/AKT/mammalian target of rapamycin (mTOR) signaling pathway is typically abnormally activated in many carcinomas (Michl and Downward, 2005; Johnson et al., 2010; Narayanankutty, 2019). It is thought to be crucial in angiogenesis, cell proliferation, metabolism, survival, metastasis and drug resistance (Cantley, 2002; Edinger and Thompson, 2002; Fingar et al., 2004; Al-Batran et al., 2012; Tapia et al., 2014). AKT is commonly overexpressed in tumors and plays an important role in the metabolic reprogramming of cancer (Yap et al., 2011; Iida et al., 2013). Although the PI3K/AKT/mTOR pathway has been extensively studied, there are few studies in CSCs. In GC and CRC, activation of mTOR appears to cause tumor progression and poor patient survival (Lang et al., 2007; Murayama et al., 2009; Xiao et al., 2009; Yu G. et al., 2009; An et al., 2010). Thus, its inhibition seems to be fundamental for GC and CRC therapy. A phase II study using RAD001 (everolimus) in previously treated unresectable or metastatic esophageal cancer or GC was performed over the rational that everolimus may stop tumor growth by blocking some of the fundamental enzymes for cell growth and by blocking angiogenesis. Everolimus was well tolerated by the patients however this study displayed a strong weakness by being single-arm and non-comparative. However, mTOR suppression decreased ALDH1 activity, which is a marker of CSCs in CRC (Xia and Xu, 2015). Growth inhibition, using a dual PI3K/mTOR inhibitor, PF-04691502, was observed in vitro and in xenografted CRC tumors (Fang et al., 2013). Another mTOR inhibitor decreased survival and invasion of colorectal CSCs in vitro, and suppressed tumor growth in vivo (Francipane and Lagasse, 2013). The allosteric AKT inhibitor (MK-2206) led to a decrease in CSCs proliferation, and reduction of the capacity to form colonospheres in vitro and to initiate tumor formation in vivo. Mice with xenografted tumors showed a significant decrease in tumor progression. Also, MK-2206 significantly inhibited the growth of patient-derived tumorspheres (Malkomes et al., 2016). A phase II study in advanced gastric or gastroesophageal junction cancer has revealed that MK-2206, as second-line therapy, at a dose of 60 mg was well tolerated by patients and showed some modest evidence of activity, however, the overall survival (5.1 months) was lower than the study efficacy endpoint (6.5 months) (Ramanathan et al., 2015). However, there is a Phase II clinical trial with MK-2206, to study patients with previously treated colon or rectal cancer that has spread and cannot be removed by surgery, concluding that in contrast to robust preclinical data, it does not have effect in these tumors (Dasari et al., 2016). MK-2206 was also used in combination with AZD6244 (Selumetinib), a mitogen-activated protein extracellular signal-regulated kinase (MEK)1/2 inhibitor, in a Phase II trial but the level of target inhibition obtained with the maximum non-toxic dose was not the expected (Do et al., 2015). SAR245409 (Voxtalisib) was tested in a Phase I research trial in combination with another MEK inhibitor, MSC1936369B (Pimasertib), in advanced or metastatic solid tumors (Schram et al., 2018). The primary purpose of the study was to determine the maximum tolerated dose of the drug combination. The drug RAD001 (Everolimus) downregulates mTOR. A combination of RAD001, mFOLFOX-6 and Bevacizumab has been shown to be efficacious and safe in metastatic CRC (Weldon Gilcrease et al., 2019). A multicenter phase II study for patients with refractory, metastatic CRC concluded good tolerability and efficacy of Everolimus combined with Tivozanib (an oral VEGF receptor-1, -2, -3 inhibitor) with 50% of the patients having stable disease at 2 months (Wolpin et al., 2013).
Discussion
The identification of CSCs remains a challenging task, particularly in solid tumors like GC and CRC. The use of cell surface markers as a primary tool to identify gastric and colorectal CSCs has disclosed some weaknesses and for that reason the uncovering of more reliable biomarkers must become a priority. These biomarkers should include the TFs that are required for the maintenance of gastric and colorectal CSCs phenotype, as well as components of the signaling pathways that have key roles in CSC features. This explains why TFs such as STAT3 and signaling pathways like Wnt/β-catenin, Hedgehog, NOTCH and PI3K/AKT/mTOR emerged as powerful targets, whose inactivation or modulation could eliminate gastric and colorectal CSCs. This fact is corroborated by several completed and ongoing clinical trials targeting these potential biomarkers in both tumor types, where some of the molecules have shown promising results. The incapacity to achieve the wanted levels of target inhibition was the major shortcoming of the clinical trials. Yet, the use of higher doses is not possible due to toxicity problems, which led to the development of combinations of drugs targeting different pathways. Furthermore, it is more advisable to measure the outcome of the treatments in terms of CSCs behavior, by assessing capacity to metastasize and re-growth after removing the drug.
Future Perspectives
The validation of potential gastric and colorectal CSCs biomarkers and their association with GC and CRC stage is imperative to understand patient prognosis and apply a more suitable therapy. The development of a robust therapy combining CSC targets with conventional chemotherapy could be the solution to overcome resistance to anti-cancer drugs and completely eliminate cancer. Considering these issues, it is crucial that future studies further explore the role of TFs and components of signaling pathways on cancer stemness in order to develop therapies that could eradicate CSCs.
Author Contributions
All authors listed have made a substantial, direct and intellectual contribution to the work, and approved it for publication.
Funding
This work was supported by the FEDER – Fundo Europeu de Desenvolvimento Regional funds through the COMPETE 2020 – Operacional Programme for Competitiveness and Internationalisation (POCI), Portugal 2020, and by Portuguese funds through the FCT – Fundação para a Ciência e a Tecnologia/Ministério da Ciência, Tecnologia e Inovação in the framework of the project “Institute for Research and Innovation in Health Sciences” (POCI-01-0145-FEDER-007274); projects POCI-01-0145-FEDER-029017 and POCI-01-0145-FEDER-016390 funded by FCT. DP acknowledges FCT for financial support through a Ph.D. fellowship (SFRH/BD/146186/2019).
Conflict of Interest
The authors declare that the research was conducted in the absence of any commercial or financial relationships that could be construed as a potential conflict of interest.
References
Abbasian, M., Mousavi, E., Arab-Bafrani, Z., and Sahebkar, A. (2019). The most reliable surface marker for the identification of colorectal cancer stem-like cells: a systematic review and meta-analysis. J. Cell. Physiol. 234, 8192–8202. doi: 10.1002/jcp.27619
Abetov, D., Mustapova, Z., Saliev, T., Bulanin, D., Batyrbekov, K., and Gilman, C. P. (2015). Novel small molecule inhibitors of cancer stem cell signaling pathways. Stem Cell Rev. Rep. 11, 909–918. doi: 10.1007/s12015-015-9612-x
Akbari, M., Shomali, N., Faraji, A., Shanehbandi, D., Asadi, M., Mokhtarzadeh, A., et al. (2020). CD133: an emerging prognostic factor and therapeutic target in colorectal cancer. Cell Biol. Int. 44, 368–380. doi: 10.1002/cbin.11243
Al-Batran, S. E., Ducreux, M., and Ohtsu, A. (2012). mTOR as a therapeutic target in patients with gastric cancer. Int. J. Cancer 130, 491–496. doi: 10.1002/ijc.26396
Amini, S., Fathi, F., Mobalegi, J., Sofimajidpour, H., and Ghadimi, T. (2014). The expressions of stem cell markers: Oct4, Nanog, Sox2, nucleostemin, Bmi, Zfx, Tcl1, Tbx3, Dppa4, and Esrrb in bladder, colon, and prostate cancer, and certain cancer cell lines. Anat. Cell Biol. 47, 1–11. doi: 10.5115/acb.2014.47.1.1
An, J. Y., Kim, K. M., Choi, M. G., Noh, J. H., Sohn, T. S., Bae, J. M., et al. (2010). Prognostic role of p-mTOR expression in cancer tissues and metastatic lymph nodes in pT2b gastric cancer. Int. J. Cancer 126, 2904–2913. doi: 10.1002/ijc.24872
Avilion, A. A., Nicolis, S. K., Pevny, L. H., Perez, L., Vivian, N., and Lovell-Badge, R. (2003). Multipotent cell lineages in early mouse development depend on SOX2 function. Genes Dev. 17, 126–140. doi: 10.1101/gad.224503
Badrinath, N., and Yoo, S. Y. (2019). Recent advances in cancer stem cell-targeted immunotherapy. Cancers 11:310. doi: 10.3390/cancers11030310
Barat, S., Chen, X., Bui, C. K., Bozko, P., Götze, J., Christgen, M., et al. (2017). Gamma-secretase inhibitor IX (GSI) impairs concomitant activation of notch and wnt-beta-catenin pathways in CD44+ gastric cancer stem cells. Stem Cells Transl. Med. 6, 819–829. doi: 10.1002/sctm.16-0335
Basati, G., Mohammadpour, H., and Emami Razavi, A. (2020). Association of high expression levels of SOX2, NANOG, and OCT4 in gastric cancer tumor tissues with progression and poor prognosis. J. Gastrointest. Cancer 51, 41–47. doi: 10.1007/s12029-018-00200-x
Bastide, P., Darido, C., Pannequin, J., Kist, R., Robine, S., Marty-Double, C., et al. (2007). Sox9 regulates cell proliferation and is required for Paneth cell differentiation in the intestinal epithelium. J. Cell Biol. 178, 635–648. doi: 10.1083/jcb.200704152
Basu, S., Haase, G., and Ben-Ze’ev, A. (2016). Wnt signaling in cancer stem cells and colon cancer metastasis. F1000Res. 5:F1000 Faculty Rev-699. doi: 10.12688/f1000research.7579.1
Behrens, J., von Kries, J. P., Kuhl, M., Bruhn, L., Wedlich, D., Grosschedl, R., et al. (1996). Functional interaction of beta-catenin with the transcription factor LEF-1. Nature 382, 638–642. doi: 10.1038/382638a0
Bekaii-Saab, T., and El-Rayes, B. (2017). Identifying and targeting cancer stem cells in the treatment of gastric cancer. Cancer 123, 1303–1312. doi: 10.1002/cncr.30538
Bendell, J. C., Hubbard, J. M., O’Neil, B. H., Jonker, D. J., Starodub, A., Peyton, J. D., et al. (2017). Phase 1b/II study of cancer stemness inhibitor napabucasin (BBI-608) in combination with FOLFIRI +/- bevacizumab (bev) in metastatic colorectal cancer (mCRC) patients (pts). J. Clin. Oncol. 35, 3529–3529. doi: 10.1200/JCO.2017.35.15_suppl.3529
Berlin, J., Bendell, J. C., Hart, L. L., Firdaus, I., Gore, I., Hermann, R. C., et al. (2013). A randomized phase II trial of vismodegib versus placebo with FOLFOX or FOLFIRI and bevacizumab in patients with previously untreated metastatic colorectal cancer. Clin. Cancer Res. 19, 258–267. doi: 10.1158/1078-0432.CCR-12-1800
Boesch, M., Spizzo, G., and Seeber, A. (2018). Concise review: aggressive colorectal cancer: role of epithelial cell adhesion molecule in cancer stem cells and epithelial-to-mesenchymal transition. Stem Cells Transl. Med. 7, 495–501. doi: 10.1002/sctm.17-0289
Brabletz, T., Jung, A., Spaderna, S., Hlubek, F., and Kirchner, T. (2005). Opinion: migrating cancer stem cells - an integrated concept of malignant tumour progression. Nat. Rev. Cancer 5, 744–749. doi: 10.1038/nrc1694
Bray, F., Ferlay, J., Soerjomataram, I., Siegel, R. L., Torre, L. A., and Jemal, A. (2018). Global cancer statistics 2018: GLOBOCAN estimates of incidence and mortality worldwide for 36 cancers in 185 countries. CA Cancer J. Clin. 68, 394–424. doi: 10.3322/caac.21492
Bretones, G., Delgado, M. D., and León, J. (2015). Myc and cell cycle control. Biochim. Biophys. Acta 1849, 506–516. doi: 10.1016/j.bbagrm.2014.03.013
Brungs, D., Aghmesheh, M., Vine, K. L., Becker, T. M., Carolan, M. G., and Ranson, M. (2016). Gastric cancer stem cells: evidence, potential markers, and clinical implications. J. Gastroenterol. 51, 313–326. doi: 10.1007/s00535-015-1125-5
Brunner, T. B., Kunz-Schughart, L. A., Grosse-Gehling, P., and Baumann, M. (2012). Cancer stem cells as a predictive factor in radiotherapy. Semin. Radiat. Oncol. 22, 151–174. doi: 10.1016/j.semradonc.2011.12.003
Brzozowa, M., Mielańczyk, L., Michalski, M., Malinowski, L., Kowalczyk-Ziomek, G., Helewski, K., et al. (2013). Role of Notch signaling pathway in gastric cancer pathogenesis. Contemp. Oncol. 17, 1–5. doi: 10.5114/wo.2013.33765
Buczek, M. E., Reeder, S. P., and Regad, T. (2018). “Identification and isolation of cancer stem cells using NANOG-EGFP reporter system,” in Cancer Stem Cells: Methods and Protocols, eds G. Papaccio and V. Desiderio (New York, NY: Springer), 139–148.
Camilo, V., Barros, R., Celestino, R., Castro, P., Vieira, J., Teixeira, M. R., et al. (2014). Immunohistochemical molecular phenotypes of gastric cancer based on SOX2 and CDX2 predict patient outcome. BMC Cancer 14:753. doi: 10.1186/1471-2407-14-753
Cantley, L. C. (2002). The phosphoinositide 3-kinase pathway. Science 296, 1655–1657. doi: 10.1126/science.296.5573.1655
Carmon, K. S., Lin, Q., Gong, X., Thomas, A., and Liu, Q. (2012). LGR5 interacts and cointernalizes with Wnt receptors to modulate Wnt/β-catenin signaling. Mol. Cell. Biol. 32, 2054–2064.
Carrasco-Garcia, E., Santos, J. C., Garcia, I., Brianti, M., García-Puga, M., Pedrazzoli, J., et al. (2016). Paradoxical role of SOX2 in gastric cancer. Am. J. Cancer Res. 6, 701–713.
Chambers, I., Colby, D., Robertson, M., Nichols, J., Lee, S., Tweedie, S., et al. (2003). Functional expression cloning of nanog, a pluripotency sustaining factor in embryonic stem cells. Cell 113, 643–655.
Chaudhry, A., Rudra, D., Treuting, P., Samstein, R. M., Liang, Y., Kas, A., et al. (2009). CD4+ regulatory T cells control TH17 responses in a Stat3-dependent manner. Science 326, 986–991. doi: 10.1126/science.1172702
Chen, K., Huang, Y.-H., and Chen, J.-L. (2013). Understanding and targeting cancer stem cells: therapeutic implications and challenges. Acta Pharmacol. Sin. 34, 732–740. doi: 10.1038/aps.2013.27
Chen, T., Yang, K., Yu, J., Meng, W., Yuan, D., Bi, F., et al. (2012). Identification and expansion of cancer stem cells in tumor tissues and peripheral blood derived from gastric adenocarcinoma patients. Cell Res. 22, 248–258. doi: 10.1038/cr.2011.109
Chen, Z., He, X., Jia, M., Liu, Y., Qu, D., Wu, D., et al. (2013). β-catenin overexpression in the nucleus predicts progress disease and unfavourable survival in colorectal cancer: a meta-analysis. PLoS One 8:e63854. doi: 10.1371/journal.pone.0063854
Chen, Z., Xu, W. R., Qian, H., Zhu, W., Bu, X. F., Wang, S., et al. (2009). Oct4, a novel marker for human gastric cancer. J. Surg. Oncol. 99, 414–419. doi: 10.1002/jso.21270
Chiurillo, M. A. (2015). Role of the Wnt/β-catenin pathway in gastric cancer: an in-depth literature review. World J. Exp. Med. 5, 84–102. doi: 10.5493/wjem.v5.i2.84
Cho, Y. G., Song, J. H., Kim, C. J., Nam, S. W., Yoo, N. J., Lee, J. Y., et al. (2007). Genetic and epigenetic analysis of the KLF4 gene in gastric cancer. APMIS 115, 802–808. doi: 10.1111/j.1600-0463.2007.apm_643.x
Clements, W. M., Wang, J., Sarnaik, A., Kim, O. J., MacDonald, J., Fenoglio-Preiser, C., et al. (2002). beta-Catenin mutation is a frequent cause of Wnt pathway activation in gastric cancer. Cancer Res. 62, 3503–3506.
Cohen, D. J., Christos, P. J., Kindler, H. L., Catenacci, D. V. T., Bekaii-Saab, T. B., Tahiri, S., et al. (2013). Vismodegib (V), a hedgehog (HH) pathway inhibitor, combined with FOLFOX for first-line therapy of patients (pts) with advanced gastric and gastroesophageal junction (GEJ) carcinoma: a New York Cancer Consortium led phase II randomized study. J. Clin. Oncol. 31, 4011–4011. doi: 10.1200/jco.2013.31.15_suppl.4011
Cui, J., Shi, M., Quan, M., and Xie, K. (2013). Regulation of EMT by KLF4 in gastrointestinal cancer. Curr. Cancer Drug Targets 13, 986–995. doi: 10.2174/15680096113136660104
Dai, X., Ge, J., Wang, X., Qian, X., Zhang, C., and Li, X. (2013). OCT4 regulates epithelial-mesenchymal transition and its knockdown inhibits colorectal cancer cell migration and invasion. Oncol. Rep. 29, 155–160. doi: 10.3892/or.2012.2086
Dalerba, P., Cho, R. W., and Clarke, M. F. (2007a). Cancer stem cells: models and concepts. Annu. Rev. Med. 58, 267–284. doi: 10.1146/annurev.med.58.062105.204854
Dalerba, P., Dylla, S. J., Park, I.-K., Liu, R., Wang, X., Cho, R. W., et al. (2007b). Phenotypic characterization of human colorectal cancer stem cells. Proc. Natl. Acad. Sci. U.S.A. 104, 10158–10163. doi: 10.1073/pnas.0703478104
Dang, C. V. (2013). MYC, metabolism, cell growth, and tumorigenesis. Cold Spring Harb. Perspect. Med. 3:a014217. doi: 10.1101/cshperspect.a014217
Darido, C., Buchert, M., Pannequin, J., Bastide, P., Zalzali, H., Mantamadiotis, T., et al. (2008). Defective claudin-7 regulation by Tcf-4 and Sox-9 disrupts the polarity and increases the tumorigenicity of colorectal cancer cells. Cancer Res. 68, 4258–4268.
Dasari, A., Overman, M. J., Fogelman, D. R., Kee, B. K., Menter, D., Raghav, K. P. S., et al. (2016). A phase II and co-clinical study of an AKT inhibitor in patients (pts) with biomarker-enriched, previously treated metastatic colorectal cancer (mCRC). J. Clin. Oncol. 34, 3563–3563. doi: 10.1200/JCO.2016.34.15_suppl.3563
de Sousa e Melo, F., Kurtova, A. V., Harnoss, J. M., Kljavin, N., Hoeck, J. D., Hung, J., et al. (2017). A distinct role for Lgr5+ stem cells in primary and metastatic colon cancer. Nature 543, 676–680. doi: 10.1038/nature21713
de Souza, C. R. T., Leal, M. F., Calcagno, D. Q., Costa Sozinho, E. K., Borges, B. D. N., Montenegro, R. C., et al. (2013). MYC deregulation in gastric cancer and its clinicopathological implications. PLoS One 8:e64420. doi: 10.1371/journal.pone.0064420
Dean, M., Fojo, T., and Bates, S. (2005). Tumour stem cells and drug resistance. Nat. Rev. Cancer 5, 275–284. doi: 10.1038/nrc1590
Deng, J., Liang, H., Zhang, R., Sun, D., Pan, Y., Liu, Y., et al. (2013). STAT3 is associated with lymph node metastasis in gastric cancer. Tumour Biol. 34, 2791–2800. doi: 10.1007/s13277-013-0837-5
Deng, J.-Y., Sun, D., Liu, X.-Y., Pan, Y., and Liang, H. (2010). STAT-3 correlates with lymph node metastasis and cell survival in gastric cancer. World J. Gastroenterol. 16, 5380–5387. doi: 10.3748/wjg.v16.i42.5380
Deng, Y., Zhou, J., Fang, L., Cai, Y., Ke, J., Xie, X., et al. (2014). ALDH1 is an independent prognostic factor for patients with stages II-III rectal cancer after receiving radiochemotherapy. Br. J. Cancer 110, 430–434. doi: 10.1038/bjc.2013.767
Didiasova, M., Schaefer, L., and Wygrecka, M. (2018). Targeting GLI transcription factors in cancer. Molecules 23:1003. doi: 10.3390/molecules23051003
Dienstmann, R., Vermeulen, L., Guinney, J., Kopetz, S., Tejpar, S., and Tabernero, J. (2017). Consensus molecular subtypes and the evolution of precision medicine in colorectal cancer. Nat. Rev. Cancer 17, 79–92. doi: 10.1038/nrc.2016.126
Do, K., Speranza, G., Bishop, R., Khin, S., Rubinstein, L., Kinders, R. J., et al. (2015). Biomarker-driven phase 2 study of MK-2206 and selumetinib (AZD6244, ARRY-142886) in patients with colorectal cancer. Invest. New Drugs 33, 720–728. doi: 10.1007/s10637-015-0212-z
Dong, H., Liu, H., Zhou, W., Zhang, F., Li, C., Chen, J., et al. (2019). GLI1 activation by non-classical pathway integrin α(v)β(3)/ERK1/2 maintains stem cell-like phenotype of multicellular aggregates in gastric cancer peritoneal metastasis. Cell Death Dis. 10, 574–574. doi: 10.1038/s41419-019-1776-x
Du, L., Wang, H., He, L., Zhang, J., Ni, B., Wang, X., et al. (2008). CD44 is of functional importance for colorectal cancer stem cells. Clin. Cancer Res. 14, 6751–6760. doi: 10.1158/1078-0432.CCR-08-1034
Du, X., Cheng, Z., Wang, Y.-H., Guo, Z.-H., Zhang, S.-Q., Hu, J.-K., et al. (2014). Role of Notch signaling pathway in gastric cancer: a meta-analysis of the literature. World J. Gastroenterol. 20, 9191–9199. doi: 10.3748/wjg.v20.i27.9191
Edinger, A. L., and Thompson, C. B. (2002). Akt maintains cell size and survival by increasing mTOR-dependent nutrient uptake. Mol. Biol. Cell 13, 2276–2288.
Espersen, M. L., Olsen, J., Linnemann, D., Hogdall, E., and Troelsen, J. T. (2015). Clinical implications of intestinal stem cell markers in colorectal cancer. Clin. Colorectal Cancer 14, 63–71. doi: 10.1016/j.clcc.2014.12.004
Falchook, G. S., Dowlati, A., Naing, A., Gribbin, M. J., Jenkins, D. W., Chang, L. L., et al. (2015). Phase I study of MEDI0639 in patients with advanced solid tumors. J. Clin. Oncol. 33, 3024–3024. doi: 10.1200/jco.2015.33.15_suppl.3024
Fang, D. D., Zhang, C. C., Gu, Y., Jani, J. P., Cao, J., Tsaparikos, K., et al. (2013). Antitumor efficacy of the dual PI3K/mTOR inhibitor PF-04691502 in a Human Xenograft tumor model derived from colorectal cancer stem cells harboring a PIK3CA mutation. PLoS One 8:e67258. doi: 10.1371/journal.pone.0067258
Farkona, S., Diamandis, E. P., and Blasutig, I. M. (2016). Cancer immunotherapy: the beginning of the end of cancer? BMC Med. 14:73. doi: 10.1186/s12916-016-0623-5
Fingar, D. C., Richardson, C. J., Tee, A. R., Cheatham, L., Tsou, C., and Blenis, J. (2004). mTOR controls cell cycle progression through its cell growth effectors S6K1 and 4E-BP1/eukaryotic translation initiation factor 4E. Mol. Cell. Biol. 24, 200–216. doi: 10.1128/mcb.24.1.200-216.2004
Forghanifard, M. M., Moghbeli, M., Raeisossadati, R., Tavassoli, A., Mallak, A. J., Boroumand-Noughabi, S., et al. (2013). Role of SALL4 in the progression and metastasis of colorectal cancer. J. Biomed. Sci. 20:6. doi: 10.1186/1423-0127-20-6
Francipane, M. G., and Lagasse, E. (2013). Selective targeting of human colon cancer stem-like cells by the mTOR inhibitor Torin-1. Oncotarget 4, 1948–1962. doi: 10.18632/oncotarget.1310
Fujita, T., Chiwaki, F., Takahashi, R. U., Aoyagi, K., Yanagihara, K., Nishimura, T., et al. (2015). Identification and characterization of CXCR4-positive gastric cancer stem cells. PLoS One 10:e0130808. doi: 10.1371/journal.pone.0130808
Fukamachi, H., Seol, H. S., Shimada, S., Funasaka, C., Baba, K., Kim, J. H., et al. (2013). CD49f(high) cells retain sphere-forming and tumor-initiating activities in human gastric tumors. PLoS One 8:e72438. doi: 10.1371/journal.pone.0072438
Fukamachi, H., Shimada, S., Ito, K., Ito, Y., and Yuasa, Y. (2011). CD133 is a marker of gland-forming cells in gastric tumors and Sox17 is involved in its regulation. Cancer Sci. 102, 1313–1321. doi: 10.1111/j.1349-7006.2011.01947.x
Ghanei, Z., Jamshidizad, A., Joupari, M. D., and Shamsara, M. (2020). Isolation and characterization of breast cancer stem cell-like phenotype by Oct4 promoter-mediated activity. J. Cell. Physiol. doi: 10.1002/jcp.29437 [Epub ahead of print].
Gidekel, S., Pizov, G., Bergman, Y., and Pikarsky, E. (2003). Oct-3/4 is a dose-dependent oncogenic fate determinant. Cancer Cell 4, 361–370.
Gong, X., Azhdarinia, A., Ghosh, S. C., Xiong, W., An, Z., Liu, Q., et al. (2016). LGR5-targeted antibody–drug conjugate eradicates gastrointestinal tumors and prevents recurrence. Mol. Cancer Ther. 15, 1580–1590. doi: 10.1158/1535-7163.MCT-16-0114
Goossens-Beumer, I. J., Zeestraten, E. C., Benard, A., Christen, T., Reimers, M. S., Keijzer, R., et al. (2014). Clinical prognostic value of combined analysis of Aldh1, Survivin, and EpCAM expression in colorectal cancer. Br. J. Cancer 110, 2935–2944. doi: 10.1038/bjc.2014.226
Grivennikov, S. I., and Karin, M. (2010). Dangerous liaisons: STAT3 and NF-kappaB collaboration and crosstalk in cancer. Cytokine Growth Factor Rev. 21, 11–19. doi: 10.1016/j.cytogfr.2009.11.005
Grothey, A., Shah, M. A., Yoshino, T., Cutsem, E. V., Taieb, J., Xu, R., et al. (2017). CanStem303C trial: a phase III study of napabucasin (BBI-608) in combination with 5-fluorouracil (5-FU), leucovorin, irinotecan (FOLFIRI) in adult patients with previously treated metastatic colorectal cancer (mCRC). J. Clin. Oncol. 35, TS3619–TS3619. doi: 10.1200/JCO.2017.35.15_suppl.TPS3619
Gullo, I., Carneiro, F., Oliveira, C., and Almeida, G. M. (2018). Heterogeneity in gastric cancer: from pure morphology to molecular classifications. Pathobiology 85, 50–63. doi: 10.1159/000473881
Gupta, P. B., Onder, T. T., Jiang, G., Tao, K., Kuperwasser, C., Weinberg, R. A., et al. (2009). Identification of selective inhibitors of cancer stem cells by high-throughput screening. Cell 138, 645–659. doi: 10.1016/j.cell.2009.06.034
Gupta, S., Takebe, N., and Lorusso, P. (2010). Targeting the Hedgehog pathway in cancer. Ther. Adv. Med. Oncol. 2, 237–250. doi: 10.1177/1758834010366430
Hadjimichael, C., Chanoumidou, K., Papadopoulou, N., Arampatzi, P., Papamatheakis, J., and Kretsovali, A. (2015). Common stemness regulators of embryonic and cancer stem cells. World J. Stem Cells 7, 1150–1184. doi: 10.4252/wjsc.v7.i9.1150
Hajimoradi, M., Mohammad Hassan, Z., Ebrahimi, M., Soleimani, M., Bakhshi, M., Firouzi, J., et al. (2016). STAT3 is overactivated in gastric cancer stem-like cells. Cell J. 17, 617–628. doi: 10.22074/cellj.2016.3834
Han, M.-E., Jeon, T.-Y., Hwang, S.-H., Lee, Y.-S., Kim, H.-J., Shim, H.-E., et al. (2011). Cancer spheres from gastric cancer patients provide an ideal model system for cancer stem cell research. Cell. Mol. Life Sci. 68:3589. doi: 10.1007/s00018-011-0672-z
Han, S., Kim, H. Y., Park, K., Cho, H. J., Lee, M. S., Kim, H. J., et al. (1999). c-Myc expression is related with cell proliferation and associated with poor clinical outcome in human gastric cancer. J. Korean Med. Sci. 14, 526–530. doi: 10.3346/jkms.1999.14.5.526
Han, S.-M., Han, S.-H., Coh, Y.-R., Jang, G., Chan Ra, J., Kang, S.-K., et al. (2014). Enhanced proliferation and differentiation of Oct4- and Sox2-overexpressing human adipose tissue mesenchymal stem cells. Exp. Mol. Med. 46:e101. doi: 10.1038/emm.2014.28
Haraguchi, N., Ishii, H., Mimori, K., Ohta, K., Uemura, M., Nishimura, J., et al. (2013). CD49f-positive cell population efficiently enriches colon cancer-initiating cells. Int. J. Oncol. 43, 425–430. doi: 10.3892/ijo.2013.1955
Hashimoto, I., Nagata, T., Sekine, S., Moriyama, M., Shibuya, K., Hojo, S., et al. (2017). Prognostic significance of KLF4 expression in gastric cancer. Oncol. Lett. 13, 819–826. doi: 10.3892/ol.2016.5499
Hashimoto, K., Aoyagi, K., Isobe, T., Kouhuji, K., and Shirouzu, K. (2014). Expression of CD133 in the cytoplasm is associated with cancer progression and poor prognosis in gastric cancer. Gastric Cancer 17, 97–106. doi: 10.1007/s10120-013-0255-9
Hayakawa, Y., Tsuboi, M., Asfaha, S., Kinoshita, H., Niikura, R., Konishi, M., et al. (2019). BHLHA15-positive secretory precursor cells can give rise to tumors in intestine and colon in mice. Gastroenterology 156, 1066.e–1081.e. doi: 10.1053/j.gastro.2018.11.024
Horii, A., Nakatsuru, S., Miyoshi, Y., Ichii, S., Nagase, H., Kato, Y., et al. (1992). The APC gene, responsible for familial adenomatous polyposis, is mutated in human gastric cancer. Cancer Res. 52, 3231–3233.
Horst, D., Kriegl, L., Engel, J., Kirchner, T., and Jung, A. (2009). Prognostic significance of the cancer stem cell markers CD133, CD44, and CD166 in colorectal cancer. Cancer Invest. 27, 844–850. doi: 10.1080/07357900902744502
Hsu, L.-S., Chan, C.-P., Chen, C.-J., Lin, S.-H., Lai, M.-T., Hsu, J.-D., et al. (2013). Decreased Kruppel-like factor 4 (KLF4) expression may correlate with poor survival in gastric adenocarcinoma. Med. Oncol. 30:632. doi: 10.1007/s12032-013-0632-6
Huang, E. H., Hynes, M. J., Zhang, T., Ginestier, C., Dontu, G., Appelman, H., et al. (2009). Aldehyde dehydrogenase 1 is a marker for normal and malignant human colonic stem cells (SC) and tracks SC overpopulation during colon tumorigenesis. Cancer Res. 69, 3382–3389. doi: 10.1158/0008-5472.CAN-08-4418
Huang, R., Wang, G., Song, Y., Tang, Q., You, Q., Liu, Z., et al. (2015). Colorectal cancer stem cell and chemoresistant colorectal cancer cell phenotypes and increased sensitivity to Notch pathway inhibitor. Mol. Med. Rep. 12, 2417–2424. doi: 10.3892/mmr.2015.3694
Huang, W. S., Wang, J. P., Wang, T., Fang, J. Y., Lan, P., and Ma, J. P. (2007). ShRNA-mediated gene silencing of beta-catenin inhibits growth of human colon cancer cells. World J. Gastroenterol. 13, 6581–6587. doi: 10.3748/wjg.v13.i48.6581
Huch, M., and Clevers, H. (2011). Sox9 marks adult organ progenitors. Nat. Genet. 43, 9–10. doi: 10.1038/ng0111-9
Hütz, K., Mejías-Luque, R., Farsakova, K., Ogris, M., Krebs, S., Anton, M., et al. (2013). The stem cell factor SOX2 regulates the tumorigenic potential in human gastric cancer cells. Carcinogenesis 35, 942–950. doi: 10.1093/carcin/bgt410
Ibrahim, E. E., Babaei-Jadidi, R., Saadeddin, A., Spencer-Dene, B., Hossaini, S., Abuzinadah, M., et al. (2012). Embryonic NANOG activity defines colorectal cancer stem cells and modulates through AP1- and TCF-dependent mechanisms. Stem Cells 30, 2076–2087. doi: 10.1002/stem.1182
Iida, M., Brand, T. M., Campbell, D. A., Starr, M. M., Luthar, N., Traynor, A. M., et al. (2013). Targeting AKT with the allosteric AKT inhibitor MK-2206 in non-small cell lung cancer cells with acquired resistance to cetuximab. Cancer Biol. Ther. 14, 481–491. doi: 10.4161/cbt.24342
Iseghohi, S. O. (2016). Cancer stem cells may contribute to the difficulty in treating cancer. Genes Dis. 3, 7–10. doi: 10.1016/j.gendis.2016.01.001
Ishigami, S., Arigami, T., Uenosono, Y., Okumura, H., Kurahara, H., Uchikado, Y., et al. (2013). Clinical implications of DLL4 expression in gastric cancer. J. Exp. Clin. Cancer Res. 32:46. doi: 10.1186/1756-9966-32-46
Ishimoto, T., Sawayama, H., Sugihara, H., and Baba, H. (2014). Interaction between gastric cancer stem cells and the tumor microenvironment. J. Gastroenterol. 49, 1111–1120. doi: 10.1007/s00535-014-0952-0
Iv Santaliz-Ruiz, L. E., Xie, X., Old, M., Teknos, T. N., and Pan, Q. (2014). Emerging role of nanog in tumorigenesis and cancer stem cells. Int. J. Cancer 135, 2741–2748. doi: 10.1002/ijc.28690
Jackstadt, R., van Hooff, S. R., Leach, J. D., Cortes-Lavaud, X., Lohuis, J. O., Ridgway, R. A., et al. (2019). Epithelial NOTCH signaling rewires the tumor microenvironment of colorectal cancer to drive poor-prognosis subtypes and metastasis. Cancer Cell 36, 319.e–336.e. doi: 10.1016/j.ccell.2019.08.003
Janssen, K. P., Alberici, P., Fsihi, H., Gaspar, C., Breukel, C., Franken, P., et al. (2006). APC and oncogenic KRAS are synergistic in enhancing Wnt signaling in intestinal tumor formation and progression. Gastroenterology 131, 1096–1109. doi: 10.1053/j.gastro.2006.08.011
Javier, B. M., Yaeger, R., Wang, L., Sanchez-Vega, F., Zehir, A., Middha, S., et al. (2016). Recurrent, truncating SOX9 mutations are associated with SOX9 overexpression, KRAS mutation, and TP53 wild type status in colorectal carcinoma. Oncotarget 7, 50875–50882. doi: 10.18632/oncotarget.9682
Ji, K., Zhang, M., Chu, Q., Gan, Y., Ren, H., Zhang, L., et al. (2016). The role of p-STAT3 as a prognostic and clinicopathological marker in colorectal cancer: a systematic review and meta-analysis. PLoS One 11:e0160125. doi: 10.1371/journal.pone.0160125
Jiang, J., Zhang, Y., Chuai, S., Wang, Z., Zheng, D., Xu, F., et al. (2012). Trastuzumab (herceptin) targets gastric cancer stem cells characterized by CD90 phenotype. Oncogene 31, 671–682. doi: 10.1038/onc.2011.282
Jiang, R., Niu, X., Huang, Y., and Wang, X. (2016). β-Catenin is important for cancer stem cell generation and tumorigenic activity in nasopharyngeal carcinoma. Acta Biochim. Biophys. Sin. 48, 229–237. doi: 10.1093/abbs/gmv134
Jiang, Y., He, Y., Li, H., Li, H. N., Zhang, L., Hu, W., et al. (2012). Expressions of putative cancer stem cell markers ABCB1, ABCG2, and CD133 are correlated with the degree of differentiation of gastric cancer. Gastric Cancer 15, 440–450. doi: 10.1007/s10120-012-0140-y
Jiang, Y., Li, W., He, X., Zhang, H., Jiang, F., and Chen, Z. (2016). Lgr5 expression is a valuable prognostic factor for colorectal cancer: evidence from a meta-analysis. BMC Cancer 16:12. doi: 10.1186/s12885-015-1985-3
Jiang, Y. X., Yang, S. W., Li, P. A., Luo, X., Li, Z. Y., Hao, Y. X., et al. (2017). The promotion of the transformation of quiescent gastric cancer stem cells by IL-17 and the underlying mechanisms. Oncogene 36, 1256–1264. doi: 10.1038/onc.2016.291
Jo, A., Denduluri, S., Zhang, B., Wang, Z., Yin, L., Yan, Z., et al. (2014). The versatile functions of Sox9 in development, stem cells, and human diseases. Genes Dis. 1, 149–161. doi: 10.1016/j.gendis.2014.09.004
Johnson, S. M., Gulhati, P., Rampy, B. A., Han, Y., Rychahou, P. G., Doan, H. Q., et al. (2010). Novel expression patterns of PI3K/Akt/mTOR signaling pathway components in colorectal cancer. J. Am. Coll. Surg. 210, 767–776, 776–778. doi: 10.1016/j.jamcollsurg.2009.12.008
Jonker, D. J., Nott, L., Yoshino, T., Gill, S., Shapiro, J., Ohtsu, A., et al. (2018). Napabucasin versus placebo in refractory advanced colorectal cancer: a randomised phase 3 trial. Lancet Gastroenterol. Hepatol. 3, 263–270. doi: 10.1016/S2468-1253(18)30009-8
Kahlert, C., Gaitzsch, E., Steinert, G., Mogler, C., Herpel, E., Hoffmeister, M., et al. (2012). Expression analysis of aldehyde dehydrogenase 1A1 (ALDH1A1) in colon and rectal cancer in association with prognosis and response to chemotherapy. Ann. Surg. Oncol. 19, 4193–4201. doi: 10.1245/s10434-012-2518-9
Kang, H., An, H. J., Song, J. Y., Kim, T. H., Heo, J. H., Ahn, D. H., et al. (2012). Notch3 and Jagged2 contribute to gastric cancer development and to glandular differentiation associated with MUC2 and MUC5AC expression. Histopathology 61, 576–586. doi: 10.1111/j.1365-2559.2012.04274.x
Kannarkatt, J., Joseph, J., Kurniali, P. C., Al-Janadi, A., and Hrinczenko, B. (2017). Adjuvant chemotherapy for stage II colon cancer: a clinical dilemma. J. Oncol. Pract. 13, 233–241. doi: 10.1200/jop.2016.017210
Kanwar, S. S., Yu, Y., Nautiyal, J., Patel, B. B., and Majumdar, A. P. (2010). The Wnt/beta-catenin pathway regulates growth and maintenance of colonospheres. Mol. Cancer 9:212. doi: 10.1186/1476-4598-9-212
Kemper, K., Prasetyanti, P. R., De Lau, W., Rodermond, H., Clevers, H., and Medema, J. P. (2012). Monoclonal antibodies against Lgr5 identify human colorectal cancer stem cells. Stem Cells 30, 2378–2386. doi: 10.1002/stem.1233
Kim, D.-Y., Cha, S.-T., Ahn, D.-H., Kang, H.-Y., Kwon, C.-I., Ko, K.-H., et al. (2009). STAT3 expression in gastric cancer indicates a poor prognosis. J. Gastroenterol. Hepatol. 24, 646–651. doi: 10.1111/j.1440-1746.2008.05671.x
Kim, E. J., Shin, H.-K., and Park, J. H. Y. (2005). Genistein inhibits insulin-like growth factor-I receptor signaling in HT-29 human colon cancer cells: a possible mechanism of the growth inhibitory effect of Genistein. J. Med. Food 8, 431–438. doi: 10.1089/jmf.2005.8.431
Kim, H. B., Lim, H. J., Lee, H. J., Park, J. H., and Park, S. G. (2019). Evaluation and clinical significance of Jagged-1-activated notch signaling by APEX1 in colorectal cancer. Anticancer Res. 39, 6097–6105. doi: 10.21873/anticanres.13817
Kim, Y., Joo, K. M., Jin, J., and Nam, D.-H. (2009). Cancer stem cells and their mechanism of chemo-radiation resistance. Int. J. Stem Cells 2, 109–114. doi: 10.15283/ijsc.2009.2.2.109
Kodama, H., Murata, S., Ishida, M., Yamamoto, H., Yamaguchi, T., Kaida, S., et al. (2017). Prognostic impact of CD44-positive cancer stem-like cells at the invasive front of gastric cancer. Br. J. Cancer 116, 186–194. doi: 10.1038/bjc.2016.401
Kolligs, F. T., Bommer, G., and Goke, B. (2002). Wnt/beta-catenin/tcf signaling: a critical pathway in gastrointestinal tumorigenesis. Digestion 66, 131–144. doi: 10.1159/000066755
Krizhanovsky, V., and Lowe, S. W. (2009). Stem cells: the promises and perils of p53. Nature 460, 1085–1086. doi: 10.1038/4601085a
Kuipers, E. J., Grady, W. M., Lieberman, D., Seufferlein, T., Sung, J. J., Boelens, P. G., et al. (2015). Colorectal cancer. Nat. Rev. Dis. Primers 1:15065. doi: 10.1038/nrdp.2015.65
Labianca, R., Nordlinger, B., Beretta, G. D., Mosconi, S., Mandalà, M., Cervantes, A., et al. (2013). Early colon cancer: ESMO clinical practice guidelines for diagnosis, treatment and follow-up. Ann. Oncol. 24, (Suppl. 6), vi64–vi72. doi: 10.1093/annonc/mdt354
Lang, S. A., Gaumann, A., Koehl, G. E., Seidel, U., Bataille, F., Klein, D., et al. (2007). Mammalian target of rapamycin is activated in human gastric cancer and serves as a target for therapy in an experimental model. Int. J. Cancer 120, 1803–1810. doi: 10.1002/ijc.22442
Lau, E. Y.-T., Ho, N. P.-Y., and Lee, T. K.-W. (2017). Cancer stem cells and their microenvironment: biology and therapeutic implications. Stem Cells Int. 2017:3714190. doi: 10.1155/2017/3714190
Lee, H. H., Seo, K. J., An, C. H., Kim, J. S., and Jeon, H. M. (2012). CD133 expression is correlated with chemoresistance and early recurrence of gastric cancer. J. Surg. Oncol. 106, 999–1004. doi: 10.1002/jso.23178
Lee, K. S., Kwak, Y., Nam, K. H., Kim, D. W., Kang, S. B., Choe, G., et al. (2015). c-MYC copy-number gain is an independent prognostic factor in patients with colorectal cancer. PLoS One 10:e0139727. doi: 10.1371/journal.pone.0139727
Leng, Z., Tao, K., Xia, Q., Tan, J., Yue, Z., Chen, J., et al. (2013). Kruppel-like factor 4 acts as an oncogene in colon cancer stem cell-enriched spheroid cells. PLoS One 8:e56082. doi: 10.1371/journal.pone.0056082
Leng, Z., Xia, Q., Chen, J., Li, Y., Xu, J., Zhao, E., et al. (2018). Lgr5+CD44+EpCAM+ strictly defines cancer stem cells in human colorectal cancer. Cell. Physiol. Biochem. 46, 860–872. doi: 10.1159/000488743
Li, M. X., Wang, Q., Wang, B., Yan, D. W., Tang, H. M., Peng, Z. H., et al. (2012). [Association between gut-enriched Kruppel-like factor and prognosis of patients with gastric cancer]. Zhonghua Wei Chang Wai Ke Za Zhi 15, 732–735.
Li, W., Zhou, Y., Yang, J., Zhang, X., Zhang, H., Zhang, T., et al. (2015). Gastric cancer-derived mesenchymal stem cells prompt gastric cancer progression through secretion of interleukin-8. J. Exp. Clin. Cancer Res. 34:52. doi: 10.1186/s13046-015-0172-3
Li, Y., Rogoff, H. A., Keates, S., Gao, Y., Murikipudi, S., Mikule, K., et al. (2015). Suppression of cancer relapse and metastasis by inhibiting cancer stemness. Proc. Natl. Acad. Sci. U.S.A. 112, 1839–1844. doi: 10.1073/pnas.1424171112
Lin, L., Liu, A., Peng, Z., Lin, H. J., Li, P. K., Li, C., et al. (2011). STAT3 is necessary for proliferation and survival in colon cancer-initiating cells. Cancer Res. 71, 7226–7237. doi: 10.1158/0008-5472.CAN-10-4660
Lin, Q., Lai, R., Chirieac, L. R., Li, C., Thomazy, V. A., Grammatikakis, I., et al. (2005). Constitutive activation of JAK3/STAT3 in colon carcinoma tumors and cell lines: inhibition of JAK3/STAT3 signaling induces apoptosis and cell cycle arrest of colon carcinoma cells. Am. J. Pathol. 167, 969–980. doi: 10.1016/s0002-9440(10)61187-x
Lin, T., Ding, Y.-Q., and Li, J.-M. (2012). Overexpression of Nanog protein is associated with poor prognosis in gastric adenocarcinoma. Med. Oncol. 29, 878–885. doi: 10.1007/s12032-011-9860-9
Liu, L., Ning, X., Sun, L., Zhang, H., Shi, Y., Guo, C., et al. (2008). Hypoxia-inducible factor-1 alpha contributes to hypoxia-induced chemoresistance in gastric cancer. Cancer Sci. 99, 121–128. doi: 10.1111/j.1349-7006.2007.00643.x
Lochhead, P., and El-Omar, E. M. (2008). Gastric cancer. Br. Med. Bull. 85, 87–100. doi: 10.1093/bmb/ldn007
LoConte, N. K., Razak, A. R. A., Ivy, P., Tevaarwerk, A., Leverence, R., Kolesar, J., et al. (2015). A multicenter phase 1 study of γ -secretase inhibitor RO4929097 in combination with capecitabine in refractory solid tumors. Invest. New Drugs 33, 169–176. doi: 10.1007/s10637-014-0166-6
Loh, Y.-H., Wu, Q., Chew, J.-L., Vega, V. B., Zhang, W., Chen, X., et al. (2006). The Oct4 and Nanog transcription network regulates pluripotency in mouse embryonic stem cells. Nat. Genet. 38, 431–440. doi: 10.1038/ng1760
Low, J. A., and de Sauvage, F. J. (2010). Clinical experience with Hedgehog pathway inhibitors. J. Clin. Oncol. 28, 5321–5326. doi: 10.1200/jco.2010.27.9943
Lu, B., Fang, Y., Xu, J., Wang, L., Xu, F., Xu, E., et al. (2008). Analysis of SOX9 expression in colorectal cancer. Am. J. Clin. Pathol. 130, 897–904. doi: 10.1309/ajcpw1w8gjbqgcni
Lu, J., Ye, X., Fan, F., Xia, L., Bhattacharya, R., Bellister, S., et al. (2013). Endothelial cells promote the colorectal cancer stem cell phenotype through a soluble form of Jagged-1. Cancer Cell 23, 171–185. doi: 10.1016/j.ccr.2012.12.021
Lugli, A., Iezzi, G., Hostettler, I., Muraro, M. G., Mele, V., Tornillo, L., et al. (2010). Prognostic impact of the expression of putative cancer stem cell markers CD133, CD166, CD44s, EpCAM, and ALDH1 in colorectal cancer. Br. J. Cancer 103, 382–390. doi: 10.1038/sj.bjc.6605762
Luistro, L., He, W., Smith, M., Packman, K., Vilenchik, M., Carvajal, D., et al. (2009). Preclinical profile of a potent γ-secretase inhibitor targeting notch signaling with in vivo efficacy and pharmacodynamic properties. Cancer Res. 69, 7672–7680. doi: 10.1158/0008-5472.Can-09-1843
Lundberg, I. V., Edin, S., Eklof, V., Oberg, A., Palmqvist, R., and Wikberg, M. L. (2016). SOX2 expression is associated with a cancer stem cell state and down-regulation of CDX2 in colorectal cancer. BMC Cancer 16:471. doi: 10.1186/s12885-016-2509-5
Lundberg, I. V., Lofgren Burstrom, A., Edin, S., Eklof, V., Oberg, A., Stenling, R., et al. (2014). SOX2 expression is regulated by BRAF and contributes to poor patient prognosis in colorectal cancer. PLoS One 9:e101957. doi: 10.1371/journal.pone.0101957
Ma, X., Chen, K., Huang, S., Zhang, X., Adegboyega, P. A., Evers, B. M., et al. (2005). Frequent activation of the hedgehog pathway in advanced gastric adenocarcinomas. Carcinogenesis 26, 1698–1705. doi: 10.1093/carcin/bgi130
Ma, Y., Cui, W., Yang, J., Qu, J., Di, C., Amin, H. M., et al. (2006). SALL4, a novel oncogene, is constitutively expressed in human acute myeloid leukemia (AML) and induces AML in transgenic mice. Blood 108, 2726–2735.
Magee, J. A., Piskounova, E., and Morrison, S. J. (2012). Cancer stem cells: impact, heterogeneity, and uncertainty. Cancer Cell 21, 283–296. doi: 10.1016/j.ccr.2012.03.003
Malkomes, P., Lunger, I., Luetticke, A., Oppermann, E., Haetscher, N., Serve, H., et al. (2016). Selective AKT inhibition by MK-2206 represses colorectal cancer-initiating stem cells. Ann. Surg. Oncol. 23, 2849–2857. doi: 10.1245/s10434-016-5218-z
Mao, J., Fan, S., Ma, W., Fan, P., Wang, B., Zhang, J., et al. (2014). Roles of Wnt/β-catenin signaling in the gastric cancer stem cells proliferation and salinomycin treatment. Cell Death Dis. 5:e1039. doi: 10.1038/cddis.2013.515
Marjanovic, N. D., Weinberg, R. A., and Chaffer, C. L. (2013). Cell plasticity and heterogeneity in cancer. Clin. Chem. 59, 168–179. doi: 10.1373/clinchem.2012.184655
Matheu, A., Collado, M., Wise, C., Manterola, L., Cekaite, L., Tye, A. J., et al. (2012). Oncogenicity of the developmental transcription factor Sox9. Cancer Res. 72, 1301–1315. doi: 10.1158/0008-5472.CAN-11-3660
Mattiuzzi, C., Sanchis-Gomar, F., and Lippi, G. (2019). Concise update on colorectal cancer epidemiology. Ann. Transl. Med. 7:609. doi: 10.21037/atm.2019.07.91
Mazumdar, J., O’Brien, W. T., Johnson, R. S., LaManna, J. C., Chavez, J. C., Klein, P. S., et al. (2010). O2 regulates stem cells through Wnt/β-catenin signalling. Nat. Cell Biol. 12, 1007–1013. doi: 10.1038/ncb2102
McMillan, R., and Matsui, W. (2012). Molecular pathways: the hedgehog signaling pathway in cancer. Clin. Cancer Res. 18, 4883–4888.
Menon, S., Shin, S., and Dy, G. (2016). Advances in cancer immunotherapy in solid tumors. Cancers 8:E106. doi: 10.3390/cancers8120106
Mesquita, P., Freire, A. F., Lopes, N., Gomes, R., Azevedo, D., Barros, R., et al. (2019). Expression and clinical relevance of SOX9 in gastric cancer. Dis. Markers 2019:8267021. doi: 10.1155/2019/8267021
Michl, P., and Downward, J. (2005). Mechanisms of disease: PI3K/AKT signaling in gastrointestinal cancers. Z. Gastroenterol. 43, 1133–1139.
Miyoshi, N., Fujino, S., Ohue, M., Yasui, M., Takahashi, Y., Sugimura, K., et al. (2018). The POU5F1 gene expression in colorectal cancer: a novel prognostic marker. Surg. Today 48, 709–715. doi: 10.1007/s00595-018-1644-9
Miyoshi, N., Ishii, H., Nagai, K., Hoshino, H., Mimori, K., Tanaka, F., et al. (2010). Defined factors induce reprogramming of gastrointestinal cancer cells. Proc. Natl. Acad. Sci. U.S.A. 107, 40–45. doi: 10.1073/pnas.0912407107
Mohamed, S. Y., Kaf, R. M., Ahmed, M. M., Elwan, A., Ashour, H. R., and Ibrahim, A. (2019). The prognostic value of cancer stem cell markers (Notch1, ALDH1, and CD44) in primary colorectal carcinoma. J. Gastrointest. Cancer 50, 824–837. doi: 10.1007/s12029-018-0156-6
Müller, S., Cañeque, T., Acevedo, V., and Rodriguez, R. (2017). Targeting cancer stem cells with small molecules. Isr. J. Chem. 57, 239–250. doi: 10.1002/ijch.201600109
Munro, M. J., Wickremesekera, S. K., Peng, L., Tan, S. T., and Itinteang, T. (2018). Cancer stem cells in colorectal cancer: a review. J. Clin. Pathol. 71, 110–116. doi: 10.1136/jclinpath-2017-204739
Murayama, T., Inokuchi, M., Takagi, Y., Yamada, H., Kojima, K., Kumagai, J., et al. (2009). Relation between outcomes and localisation of p-mTOR expression in gastric cancer. Br. J. Cancer 100, 782–788. doi: 10.1038/sj.bjc.6604915
Muzny, D. M., Bainbridge, M. N., Chang, K., Dinh, H. H., Drummond, J. A., Fowler, G., et al. (2012). Comprehensive molecular characterization of human colon and rectal cancer. Nature 487, 330–337. doi: 10.1038/nature11252
Myant, K. B., Cammareri, P., McGhee, E. J., Ridgway, R. A., Huels, D. J., Cordero, J. B., et al. (2013). ROS production and NF-κB activation triggered by RAC1 facilitate WNT-driven intestinal stem cell proliferation and colorectal cancer initiation. Cell Stem Cell 12, 761–773. doi: 10.1016/j.stem.2013.04.006
Nakatsuru, S., Yanagisawa, A., Furukawa, Y., Ichii, S., Kato, Y., Nakamura, Y., et al. (1993). Somatic mutations of the APC gene in precancerous lesion of the stomach. Hum. Mol. Genet. 2, 1463–1465. doi: 10.1093/hmg/2.9.1463
Narayanankutty, A. (2019). PI3K/ Akt/ mTOR pathway as a therapeutic target for colorectal cancer: a review of preclinical and clinical evidence. Curr. Drug Targets 20, 1217–1226. doi: 10.2174/1389450120666190618123846
Neri, B., Pantaleo, P., Giommoni, E., Grifoni, R., Paoletti, C., Rotella, V., et al. (2007). Oxaliplatin, 5-fluorouracil/leucovorin and epirubicin as first-line treatment in advanced gastric carcinoma: a phase II study. Br. J. Cancer 96, 1043–1046. doi: 10.1038/sj.bjc.6603644
Niedzwiecki, D., Bertagnolli, M. M., Warren, R. S., Compton, C. C., Kemeny, N. E., Benson, A. B., et al. (2011). Documenting the natural history of patients with resected stage II adenocarcinoma of the colon after random assignment to adjuvant treatment with edrecolomab or observation: results from CALGB 9581. J. Clin. Oncol. 29, 3146–3152. doi: 10.1200/jco.2010.32.5357
Nosrati, A., Naghshvar, F., and Khanari, S. (2014). Cancer stem cell markers CD44, CD133 in primary gastric adenocarcinoma. Int. J. Mol. Cell. Med. 3, 279–286.
O’Brien, C. A., Pollett, A., Gallinger, S., and Dick, J. E. (2007). A human colon cancer cell capable of initiating tumour growth in immunodeficient mice. Nature 445, 106–110. doi: 10.1038/nature05372
Ohkuma, M., Haraguchi, N., Ishii, H., Mimori, K., Tanaka, F., Kim, H. M., et al. (2012). Absence of CD71 transferrin receptor characterizes human gastric adenosquamous carcinoma stem cells. Ann. Surg. Oncol. 19, 1357–1364. doi: 10.1245/s10434-011-1739-7
Ong, C. W., Kim, L. G., Kong, H. H., Low, L. Y., Iacopetta, B., Soong, R., et al. (2010). CD133 expression predicts for non-response to chemotherapy in colorectal cancer. Mod. Pathol. 23, 450–457. doi: 10.1038/modpathol.2009.181
Ooi, C. H., Ivanova, T., Wu, J., Lee, M., Tan, I. B., Tao, J., et al. (2009). Oncogenic pathway combinations predict clinical prognosis in gastric cancer. PLoS Genet. 5:e1000676. doi: 10.1371/journal.pgen.1000676
Pádua, D., Barros, R., Amaral, A. L., Mesquita, P., Freire, A. F., Sousa, M., et al. (2020). A SOX2 reporter system identifies gastric cancer stem-like cells sensitive to monensin. Cancers 12:495. doi: 10.3390/cancers12020495
Pant, S., Jones, S. F., Kurkjian, C. D., Infante, J. R., Moore, K. N., Burris, H. A., et al. (2016). A first-in-human phase I study of the oral Notch inhibitor, LY900009, in patients with advanced cancer. Eur. J. Cancer 56, 1–9. doi: 10.1016/j.ejca.2015.11.021
Parizadeh, S. M., Jafarzadeh-Esfehani, R., Hassanian, S. M., Parizadeh, S. M. R., Vojdani, S., Ghandehari, M., et al. (2019). Targeting cancer stem cells as therapeutic approach in the treatment of colorectal cancer. Int. J. Biochem. Cell Biol. 110, 75–83. doi: 10.1016/j.biocel.2019.02.010
Park, J. H., Chung, S., Matsuo, Y., and Nakamura, Y. (2017). Development of small molecular compounds targeting cancer stem cells. Medchemcomm 8, 73–80. doi: 10.1039/c6md00385k
Patel, S., Alam, A., Pant, R., and Chattopadhyay, S. (2019). Wnt signaling and its significance within the tumor microenvironment: novel therapeutic insights. Front. Immunol. 10:2872. doi: 10.3389/fimmu.2019.02872
Pattabiraman, D. R., and Weinberg, R. A. (2014). Tackling the cancer stem cells - what challenges do they pose? Nat. Rev. Drug Discov. 13, 497–512. doi: 10.1038/nrd4253
Phi, L. T. H., Sari, I. N., Yang, Y.-G., Lee, S.-H., Jun, N., Kim, K. S., et al. (2018). Cancer Stem Cells (CSCs) in drug resistance and their therapeutic implications in cancer treatment. Stem Cells Int. 2018:5416923. doi: 10.1155/2018/5416923
Pintova, S., Dharmupari, S., Moshier, E., Zubizarreta, N., Ang, C., and Holcombe, R. F. (2019). Genistein combined with FOLFOX or FOLFOX–Bevacizumab for the treatment of metastatic colorectal cancer: phase I/II pilot study. Cancer Chemother. Pharmacol. 84, 591–598. doi: 10.1007/s00280-019-03886-3
Prévostel, C., and Blache, P. (2017). The dose-dependent effect of SOX9 and its incidence in colorectal cancer. Eur. J. Cancer 86, 150–157. doi: 10.1016/j.ejca.2017.08.037
Quail, D. F., Taylor, M. J., and Postovit, L. M. (2012). Microenvironmental regulation of cancer stem cell phenotypes. Curr. Stem Cell Res. Ther. 7, 197–216. doi: 10.2174/157488812799859838
Quante, M., Varga, J., Wang, T. C., and Greten, F. R. (2013). The gastrointestinal tumor microenvironment. Gastroenterology 145, 63–78. doi: 10.1053/j.gastro.2013.03.052
Ramanathan, R. K., McDonough, S. L., Kennecke, H. F., Iqbal, S., Baranda, J. C., Seery, T. E., et al. (2015). Phase 2 study of MK-2206, an allosteric inhibitor of AKT, as second-line therapy for advanced gastric and gastroesophageal junction cancer: a SWOG cooperative group trial (S1005). Cancer 121, 2193–2197. doi: 10.1002/cncr.29363
Rassouli, F. B., Matin, M. M., and Saeinasab, M. (2016). Cancer stem cells in human digestive tract malignancies. Tumor Biol. 37, 7–21. doi: 10.1007/s13277-015-4155-y
Rezalotfi, A., Ahmadian, E., Aazami, H., Solgi, G., and Ebrahimi, M. (2019). Gastric cancer stem cells effect on Th17/Treg Balance; a bench to beside perspective. Front. Oncol. 9:226. doi: 10.3389/fonc.2019.00226
Ricci-Vitiani, L., Lombardi, D. G., Pilozzi, E., Biffoni, M., Todaro, M., Peschle, C., et al. (2007). Identification and expansion of human colon-cancer-initiating cells. Nature 445, 111–115. doi: 10.1038/nature05384
Riley, R. S., June, C. H., Langer, R., and Mitchell, M. J. (2019). Delivery technologies for cancer immunotherapy. Nat. Rev. Drug Discov. 18, 175–196. doi: 10.1038/s41573-018-0006-z
Rimkus, T. K., Carpenter, R. L., Qasem, S., Chan, M., and Lo, H. W. (2016). Targeting the sonic hedgehog signaling pathway: review of smoothened and GLI inhibitors. Cancers 8:22. doi: 10.3390/cancers8020022
Robertson, J. H., Yang, S. Y., Winslet, M. C., and Seifalian, A. M. (2009). Functional blocking of specific integrins inhibit colonic cancer migration. Clin. Exp. Metastasis 26, 769–780. doi: 10.1007/s10585-009-9276-5
Rocco, A., Liguori, E., Pirozzi, G., Tirino, V., Compare, D., Franco, R., et al. (2012). CD133 and CD44 cell surface markers do not identify cancer stem cells in primary human gastric tumors. J. Cell. Physiol. 227, 2686–2693. doi: 10.1002/jcp.23013
Sahebjam, S., Bedard, P. L., Castonguay, V., Chen, Z., Reedijk, M., Liu, G., et al. (2013). A phase I study of the combination of ro4929097 and cediranib in patients with advanced solid tumours (PJC-004/NCI 8503). Br. J. Cancer 109, 943–949. doi: 10.1038/bjc.2013.380
Santos, J. C., Carrasco-Garcia, E., Garcia-Puga, M., Aldaz, P., Montes, M., Fernandez-Reyes, M., et al. (2016). SOX9 elevation acts with canonical WNT signaling to drive gastric cancer progression. Cancer Res. 76, 6735–6746. doi: 10.1158/0008-5472.CAN-16-1120
Sarkar, A., and Hochedlinger, K. (2013). The sox family of transcription factors: versatile regulators of stem and progenitor cell fate. Cell Stem Cell 12, 15–30. doi: 10.1016/j.stem.2012.12.007
Saygin, C., Samour, M., Chumakova, A., Jarrar, A., Lathia, J. D., and Reizes, O. (2016). “Reporter systems to study cancer stem cells,” in Stem Cell Heterogeneity: Methods and Protocols, ed. K. Turksen (New York, NY: Springer), 319–333.
Schram, A. M., Gandhi, L., Mita, M. M., Damstrup, L., Campana, F., Hidalgo, M., et al. (2018). A phase Ib dose-escalation and expansion study of the oral MEK inhibitor pimasertib and PI3K/MTOR inhibitor voxtalisib in patients with advanced solid tumours. Br. J. Cancer 119, 1471–1476. doi: 10.1038/s41416-018-0322-4
Shapiro, G., Bedard, P., Infante, J., Bauer, T., Prawira, A., Laksin, O., et al. (2016). Phase 1 results of PTC596, a novel small molecule targeting cancer stem cells (CSCs) by reducing levels of BMI1 protein. Eur. J. Cancer 69, S148.
Shie, J. L., Chen, Z. Y., O’Brien, M. J., Pestell, R. G., Lee, M. E., and Tseng, C. C. (2000). Role of gut-enriched Kruppel-like factor in colonic cell growth and differentiation. Am. J. Physiol. Gastrointest. Liver Physiol. 279, G806–G814. doi: 10.1152/ajpgi.2000.279.4.G806
Shih Ie, M., and Wang, T. L. (2007). Notch signaling, gamma-secretase inhibitors, and cancer therapy. Cancer Res. 67, 1879–1882.
Smyth, E. C., Verheij, M., Allum, W., Cunningham, D., Cervantes, A., Arnold, D., et al. (2016). Gastric cancer: ESMO Clinical Practice Guidelines for diagnosis, treatment and follow-up†. Ann. Oncol. 27(Suppl._5), v38–v49. doi: 10.1093/annonc/mdw350
Sonbol, M. B., Ahn, D. H., and Bekaii-Saab, T. (2019). Therapeutic targeting strategies of cancer stem cells in gastrointestinal malignancies. Biomedicines 7:17. doi: 10.3390/biomedicines7010017
Song, Z., Wu, Y., Yang, J., Yang, D., and Fang, X. (2017). Progress in the treatment of advanced gastric cancer. Tumor Biol. 39:1010428317714626. doi: 10.1177/1010428317714626
Strosberg, J. R., Yeatman, T., Weber, J., Coppola, D., Schell, M. J., Han, G., et al. (2012). A phase II study of RO4929097 in metastatic colorectal cancer. Eur. J. Cancer 48, 997–1003. doi: 10.1016/j.ejca.2012.02.056
Su, S.-J., Chow, N.-H., Kung, M.-L., Hung, T.-C., and Chang, K.-L. (2003). Effects of soy isoflavones on apoptosis induction and G2-M arrest in human hepatoma cells involvement of caspase-3 activation, Bcl-2 and Bcl-XL downregulation, and Cdc2 kinase activity. Nutr. Cancer 45, 113–123. doi: 10.1207/S15327914NC4501_13
Sun, M., Uozaki, H., Hino, R., Kunita, A., Shinozaki, A., Ushiku, T., et al. (2012). SOX9 expression and its methylation status in gastric cancer. Virchows Arch. 460, 271–279. doi: 10.1007/s00428-012-1201-7
Tai, M.-H., Chang, C.-C., Olson, L. K., and Trosko, J. E. (2005). Oct4 expression in adult human stem cells: evidence in support of the stem cell theory of carcinogenesis. Carcinogenesis 26, 495–502. doi: 10.1093/carcin/bgh321
Takahashi, K., and Yamanaka, S. (2006). Induction of pluripotent stem cells from mouse embryonic and adult fibroblast cultures by defined factors. Cell 126, 663–676. doi: 10.1016/j.cell.2006.07.024
Takaishi, S., Okumura, T., Tu, S., Wang, S. S. W., Shibata, W., Vigneshwaran, R., et al. (2009). Identification of gastric cancer stem cells using the cell surface marker CD44. Stem Cells 27, 1006–1020. doi: 10.1002/stem.30
Takebe, N., Harris, P. J., Warren, R. Q., and Ivy, S. P. (2011). Targeting cancer stem cells by inhibiting Wnt, Notch, and Hedgehog pathways. Nat. Rev. Clin. Oncol. 8, 97–106. doi: 10.1038/nrclinonc.2010.196
Takeda, K., Mizushima, T., Yokoyama, Y., Hirose, H., Wu, X., Qian, Y., et al. (2018). Sox2 is associated with cancer stem-like properties in colorectal cancer. Sci. Rep. 8:17639.
Tang, B., Raviv, A., Esposito, D., Flanders, K. C., Daniel, C., Nghiem, B. T., et al. (2015). A flexible reporter system for direct observation and isolation of cancer stem cells. Stem Cell Rep. 4, 155–169. doi: 10.1016/j.stemcr.2014.11.002
Tapia, O., Riquelme, I., Leal, P., Sandoval, A., Aedo, S., Weber, H., et al. (2014). The PI3K/AKT/mTOR pathway is activated in gastric cancer with potential prognostic and predictive significance. Virchows Arch. 465, 25–33. doi: 10.1007/s00428-014-1588-4
Tian, T., Zhang, Y., Wang, S., Zhou, J., and Xu, S. (2012). Sox2 enhances the tumorigenicity and chemoresistance of cancer stem-like cells derived from gastric cancer. J. Biomed. Res. 26, 336–345. doi: 10.7555/JBR.26.20120045
Todaro, M., Francipane, M. G., Medema, J. P., and Stassi, G. (2010). Colon cancer stem cells: promise of targeted therapy. Gastroenterology 138, 2151–2162. doi: 10.1053/j.gastro.2009.12.063
Tolcher, A. W., Messersmith, W. A., Mikulski, S. M., Papadopoulos, K. P., Kwak, E. L., Gibbon, D. G., et al. (2012). Phase I Study of RO4929097, a gamma secretase inhibitor of notch signaling, in patients with refractory metastatic or locally advanced solid tumors. J. Clin. Oncol. 30, 2348–2353. doi: 10.1200/jco.2011.36.8282
Usui, T., Sakurai, M., Umata, K., Elbadawy, M., Ohama, T., Yamawaki, H., et al. (2018). Hedgehog signals mediate anti-cancer drug resistance in three-dimensional primary colorectal cancer organoid culture. Int. J. Mol. Sci. 19:1098. doi: 10.3390/ijms19041098
Vadde, R., Vemula, S., Jinka, R., Merchant, N., Bramhachari, P. V., and Nagaraju, G. P. (2017). Role of hypoxia-inducible factors (HIF) in the maintenance of stemness and malignancy of colorectal cancer. Crit. Rev. Oncol. Hematol. 113, 22–27. doi: 10.1016/j.critrevonc.2017.02.025
Vaiopoulos, A. G., Kostakis, I. D., Koutsilieris, M., and Papavassiliou, A. G. (2012). Colorectal cancer stem cells. Stem Cells 30, 363–371. doi: 10.1002/stem.1031
van der Heijden, M., and Vermeulen, L. (2019). Stem cells in homeostasis and cancer of the gut. Mol. Cancer 18:66. doi: 10.1186/s12943-019-0962-x
Varnat, F., Duquet, A., Malerba, M., Zbinden, M., Mas, C., Gervaz, P., et al. (2009). Human colon cancer epithelial cells harbour active HEDGEHOG-GLI signalling that is essential for tumour growth, recurrence, metastasis and stem cell survival and expansion. EMBO Mol. Med. 1, 338–351. doi: 10.1002/emmm.200900039
Venkatesh, V., Nataraj, R., Thangaraj, G. S., Karthikeyan, M., Gnanasekaran, A., Kaginelli, S. B., et al. (2018). Targeting Notch signalling pathway of cancer stem cells. Stem Cell Investig. 5:5. doi: 10.21037/sci.2018.02.02
Vermeulen, L., De Sousa, E., Melo, F., van der Heijden, M., Cameron, K., de Jong, J. H., et al. (2010). Wnt activity defines colon cancer stem cells and is regulated by the microenvironment. Nat. Cell Biol. 12, 468–476. doi: 10.1038/ncb2048
Vermeulen, L., Todaro, M., de Sousa Mello, F., Sprick, M. R., Kemper, K., Perez Alea, M., et al. (2008). Single-cell cloning of colon cancer stem cells reveals a multi-lineage differentiation capacity. Proc. Natl. Acad. Sci. U.S.A. 105, 13427–13432. doi: 10.1073/pnas.0805706105
Voorneveld, P. W., Kodach, L. L., Jacobs, R. J., van Noesel, C. J., Peppelenbosch, M. P., Korkmaz, K. S., et al. (2015). The BMP pathway either enhances or inhibits the Wnt pathway depending on the SMAD4 and p53 status in CRC. Br. J. Cancer 112, 122–130. doi: 10.1038/bjc.2014.560
Wahab, S. M. R., Islam, F., Gopalan, V., and Lam, A. K. (2017). The identifications and clinical implications of cancer stem cells in colorectal cancer. Clin. Colorectal Cancer 16, 93–102. doi: 10.1016/j.clcc.2017.01.011
Wakamatsu, Y., Sakamoto, N., Oo, H. Z., Naito, Y., Uraoka, N., Anami, K., et al. (2012). Expression of cancer stem cell markers ALDH1, CD44 and CD133 in primary tumor and lymph node metastasis of gastric cancer. Pathol. Int. 62, 112–119. doi: 10.1111/j.1440-1827.2011.02760.x
Wang, H., Li, Q., and Chen, H. (2012). Genistein affects histone modifications on Dickkopf-Related Protein 1 (DKK1) gene in SW480 human colon cancer cell line. PLoS One 7:e40955. doi: 10.1371/journal.pone.0040955
Wang, W., Deng, J., Wang, Q., Yao, Q., Chen, W., Tan, Y., et al. (2017). Synergistic role of Cul1 and c-Myc: prognostic and predictive biomarkers in colorectal cancer. Oncol. Rep. 38, 245–252. doi: 10.3892/or.2017.5671
Wang, X., Liu, Y., Shao, D., Qian, Z., Dong, Z., Sun, Y., et al. (2016). Recurrent amplification of MYC and TNFRSF11B in 8q24 is associated with poor survival in patients with gastric cancer. Gastric Cancer 19, 116–127. doi: 10.1007/s10120-015-0467-2
Wang, Z., Li, Y., Ahmad, A., Azmi, A. S., Banerjee, S., Kong, D., et al. (2010). Targeting Notch signaling pathway to overcome drug resistance for cancer therapy. Biochim. Biophys. Acta 1806, 258–267. doi: 10.1016/j.bbcan.2010.06.001
Wei, D., Kanai, M., Huang, S., and Xie, K. (2006). Emerging role of KLF4 in human gastrointestinal cancer. Carcinogenesis 27, 23–31. doi: 10.1093/carcin/bgi243
Weldon Gilcrease, G., Stenehjem, D. D., Wade, M. L., Weis, J., McGregor, K., Whisenant, J., et al. (2019). Phase I/II study of everolimus combined with mFOLFOX-6 and bevacizumab for first–line treatment of metastatic colorectal cancer. Invest. New Drugs 37, 482–489. doi: 10.1007/s10637-018-0645-2
West, N. R., McCuaig, S., Franchini, F., and Powrie, F. (2015). Emerging cytokine networks in colorectal cancer. Nat. Rev. Immunol. 15, 615–629. doi: 10.1038/nri3896
Wolpin, B. M., Ng, K., Zhu, A. X., Abrams, T., Enzinger, P. C., McCleary, N. J., et al. (2013). Multicenter phase II study of tivozanib (AV-951) and everolimus (RAD001) for patients with refractory, metastatic colorectal cancer. Oncologist 18, 377–378. doi: 10.1634/theoncologist.2012-0378
Woo, D. K., Kim, H. S., Lee, H. S., Kang, Y. H., Yang, H. K., and Kim, W. H. (2001). Altered expression and mutation of beta-catenin gene in gastric carcinomas and cell lines. Int. J. Cancer 95, 108–113.
Wuebben, E. L., and Rizzino, A. (2017). The dark side of SOX2: cancer - a comprehensive overview. Oncotarget 8, 44917–44943. doi: 10.18632/oncotarget.16570
Xia, P., and Xu, X. Y. (2015). PI3K/Akt/mTOR signaling pathway in cancer stem cells: from basic research to clinical application. Am. J. Cancer Res. 5, 1602–1609.
Xiao, L., Wang, Y. C., Li, W. S., and Du, Y. (2009). The role of mTOR and phospho-p70S6K in pathogenesis and progression of gastric carcinomas: an immunohistochemical study on tissue microarray. J. Exp. Clin. Cancer Res. 28:152. doi: 10.1186/1756-9966-28-152
Yang, J., Chai, L., Fowles, T. C., Alipio, Z., Xu, D., Fink, L. M., et al. (2008). Genome-wide analysis reveals Sall4 to be a major regulator of pluripotency in murine-embryonic stem cells. Proc. Natl. Acad. Sci. U.S.A. 105, 19756–19761. doi: 10.1073/pnas.0809321105
Yang, L., Shi, P., Zhao, G., Xu, J., Peng, W., Zhang, J., et al. (2020). Targeting cancer stem cell pathways for cancer therapy. Signal Transduct. Target. Ther. 5:8. doi: 10.1038/s41392-020-0110-5
Yang, Z., Zhang, C., Qi, W., Cui, Y., and Xuan, Y. (2018). GLI1 promotes cancer stemness through intracellular signaling pathway PI3K/Akt/NFkappaB in colorectal adenocarcinoma. Exp. Cell Res. 373, 145–154. doi: 10.1016/j.yexcr.2018.10.006
Yang, Z. H., Dang, Y. Q., and Ji, G. (2019). Role of epigenetics in transformation of inflammation into colorectal cancer. World J. Gastroenterol. 25, 2863–2877. doi: 10.3748/wjg.v25.i23.2863
Yao, Y., Zhou, D., Shi, D., Zhang, H., Zhan, S., Shao, X., et al. (2019). GLI1 overexpression promotes gastric cancer cell proliferation and migration and induces drug resistance by combining with the AKT-mTOR pathway. Biomed. Pharmacother. 111, 993–1004. doi: 10.1016/j.biopha.2019.01.018
Yap, T. A., Yan, L., Patnaik, A., Fearen, I., Olmos, D., Papadopoulos, K., et al. (2011). First-in-man clinical trial of the oral pan-AKT inhibitor MK-2206 in patients with advanced solid tumors. J. Clin. Oncol. 29, 4688–4695. doi: 10.1200/jco.2011.35.5263
Yeh, T. S., Wu, C. W., Hsu, K. W., Liao, W. J., Yang, M. C., Li, A. F., et al. (2009). The activated Notch1 signal pathway is associated with gastric cancer progression through cyclooxygenase-2. Cancer Res. 69, 5039–5048. doi: 10.1158/0008-5472.CAN-08-4021
Yeung, T. M., Gandhi, S. C., and Bodmer, W. F. (2011). Hypoxia and lineage specification of cell line-derived colorectal cancer stem cells. Proc. Natl. Acad. Sci. U.S.A. 108, 4382–4387. doi: 10.1073/pnas.1014519107
Yoon, C., Park, D. J., Schmidt, B., Thomas, N. J., Lee, H.-J., Kim, T. S., et al. (2014). CD44 expression denotes a subpopulation of gastric cancer cells in which hedgehog signaling promotes chemotherapy resistance. Clin. Cancer Res. 20, 3974–3988. doi: 10.1158/1078-0432.CCR-14-0011
Young, R. A. (2011). Control of the embryonic stem cell state. Cell 144, 940–954. doi: 10.1016/j.cell.2011.01.032
Yu, G., Wang, J., Chen, Y., Wang, X., Pan, J., Li, G., et al. (2009). Overexpression of phosphorylated mammalian target of rapamycin predicts lymph node metastasis and prognosis of chinese patients with gastric cancer. Clin. Cancer Res. 15, 1821–1829. doi: 10.1158/1078-0432.CCR-08-2138
Yu, H., Kortylewski, M., and Pardoll, D. (2007). Crosstalk between cancer and immune cells: role of STAT3 in the tumour microenvironment. Nat. Rev. Immunol. 7, 41–51. doi: 10.1038/nri1995
Yu, H., Pardoll, D., and Jove, R. (2009). STATs in cancer inflammation and immunity: a leading role for STAT3. Nat. Rev. Cancer 9, 798–809. doi: 10.1038/nrc2734
Yuan, X., Zhang, X., Zhang, W., Liang, W., Zhang, P., Shi, H., et al. (2016). SALL4 promotes gastric cancer progression through activating CD44 expression. Oncogenesis 5:e268. doi: 10.1038/oncsis.2016.69
Zhang, C., Li, C., He, F., Cai, Y., and Yang, H. (2011). Identification of CD44+CD24+ gastric cancer stem cells. J. Cancer Res. Clin. Oncol. 137, 1679–1686. doi: 10.1007/s00432-011-1038-5
Zhang, H., and Xue, Y. (2008). Wnt pathway is involved in advanced gastric carcinoma. Hepatogastroenterology 55, 1126–1130.
Zhang, H. L., Wang, P., Lu, M. Z., Zhang, S. D., and Zheng, L. (2019). c-Myc maintains the self-renewal and chemoresistance properties of colon cancer stem cells. Oncol. Lett. 17, 4487–4493. doi: 10.3892/ol.2019.10081
Zhang, J., Espinoza, L. A., Kinders, R. J., Lawrence, S. M., Pfister, T. D., Zhou, M., et al. (2013). NANOG modulates stemness in human colorectal cancer. Oncogene 32, 4397–4405. doi: 10.1038/onc.2012.461
Zhang, J., Tam, W. L., Tong, G. Q., Wu, Q., Chan, H. Y., Soh, B. S., et al. (2006). Sall4 modulates embryonic stem cell pluripotency and early embryonic development by the transcriptional regulation of Pou5f1. Nat. Cell Biol. 8, 1114–1123. doi: 10.1038/ncb1481
Zhang, L., Xu, Z., Xu, X., Zhang, B., Wu, H., Wang, M., et al. (2014). SALL4, a novel marker for human gastric carcinogenesis and metastasis. Oncogene 33, 5491–5500. doi: 10.1038/onc.2013.495
Zhang, N., Zhang, J., Wang, Z. W., Zha, L., and Huang, Z. (2012). Altered expression of Krüppel-like factor 4 and β-catenin in human gastric cancer. Oncol. Lett. 3, 1017–1022. doi: 10.3892/ol.2012.619
Zhang, S. S., Huang, Z. W., Li, L. X., Fu, J. J., and Xiao, B. (2016). Identification of CD200+ colorectal cancer stem cells and their gene expression profile. Oncol. Rep. 36, 2252–2260. doi: 10.3892/or.2016.5039
Zhang, X., Hua, R., Wang, X., Huang, M., Gan, L., Wu, Z., et al. (2016). Identification of stem-like cells and clinical significance of candidate stem cell markers in gastric cancer. Oncotarget 7, 9815–9831. doi: 10.18632/oncotarget.6890
Zhang, Y., Jin, Z., Zhou, H., Ou, X., Xu, Y., Li, H., et al. (2016). Suppression of prostate cancer progression by cancer cell stemness inhibitor napabucasin. Cancer Med. 5, 1251–1258. doi: 10.1002/cam4.675
Zhang, X., Hu, F., Li, G., Li, G., Yang, X., Liu, L., et al. (2018a). Human colorectal cancer-derived mesenchymal stem cells promote colorectal cancer progression through IL-6/JAK2/STAT3 signaling. Cell Death Dis. 9:25. doi: 10.1038/s41419-017-0176-3
Zhang, X., Yuan, X., Zhu, W., Qian, H., and Xu, W. (2015). SALL4: an emerging cancer biomarker and target. Cancer Lett. 357, 55–62. doi: 10.1016/j.canlet.2014.11.037
Zhang, X., Zhang, P., Shao, M., Zang, X., Zhang, J., Mao, F., et al. (2018b). SALL4 activates TGF-β/SMAD signaling pathway to induce EMT and promote gastric cancer metastasis. Cancer Manag. Res. 10, 4459–4470. doi: 10.2147/cmar.S177373
Zhang, Y., and Chen, H. (2011). Genistein attenuates WNT signaling by up-regulating sFRP2 in a human colon cancer cell line. Exp. Biol. Med. 236, 714–722. doi: 10.1258/ebm.2011.010347
Zhao, P., Li, Y., and Lu, Y. (2010). Aberrant expression of CD133 protein correlates with Ki-67 expression and is a prognostic marker in gastric adenocarcinoma. BMC Cancer 10:218. doi: 10.1186/1471-2407-10-218
Zhao, R., Liu, Z., Xu, W., Song, L., Ren, H., Ou, Y., et al. (2020). Helicobacter pylori infection leads to KLF4 inactivation in gastric cancer through a TET1-mediated DNA methylation mechanism. Cancer Med. 9, 2551–2563. doi: 10.1002/cam4.2892
Zhao, W., Li, Y., and Zhang, X. (2017). Stemness-related markers in cancer. Cancer Transl. Med. 3, 87–95. doi: 10.4103/ctm.ctm_69_16
Zhi, Q. M., Chen, X. H., Ji, J., Zhang, J. N., Li, J. F., Cai, Q., et al. (2011). Salinomycin can effectively kill ALDH(high) stem-like cells on gastric cancer. Biomed. Pharmacother. 65, 509–515. doi: 10.1016/j.biopha.2011.06.006
Keywords: gastric cancer, colorectal cancer, transcription factors, cancer stem cells, signaling pathways, targeted therapy
Citation: Pádua D, Figueira P, Ribeiro I, Almeida R and Mesquita P (2020) The Relevance of Transcription Factors in Gastric and Colorectal Cancer Stem Cells Identification and Eradication. Front. Cell Dev. Biol. 8:442. doi: 10.3389/fcell.2020.00442
Received: 20 March 2020; Accepted: 11 May 2020;
Published: 18 June 2020.
Edited by:
Susana Solá, University of Lisbon, PortugalReviewed by:
Asfar S. Azmi, Wayne State University Karmanos Cancer Institute, United StatesBorhane Guezguez, German Cancer Research Center, Germany
Copyright © 2020 Pádua, Figueira, Ribeiro, Almeida and Mesquita. This is an open-access article distributed under the terms of the Creative Commons Attribution License (CC BY). The use, distribution or reproduction in other forums is permitted, provided the original author(s) and the copyright owner(s) are credited and that the original publication in this journal is cited, in accordance with accepted academic practice. No use, distribution or reproduction is permitted which does not comply with these terms.
*Correspondence: Patrícia Mesquita, cG1lc3F1aXRhQGlwYXRpbXVwLnB0