- “La Sapienza” University of Rome, Botrugno, Italy
Several similarities between the embryo development and the cancer process suggest the para-embryonic nature of tumors. Starting from an initial cancer stem cell (i-CSC) as a para-embryonic stem cell (p-ESC), a hierarchic sequence of CSCs (CSC1s, CSC2s, CSC3s) and non-CSCs [cancer progenitor cells (CPCs), cancer differentiated cells (CDCs)] would be generated, mimicking an ectopic rudimentary ontogenesis. Such a proposed heterogeneous cell hierarchy within the tumor structure would suggest a tumor growth model consistent with experimental data reported for mammary tumors. By tabulating the theoretical data according to this model, it is possible to identify defined mathematical relationships between cancer cells (CSCs and non-CSCs) that are surprisingly similar to experimental data. Moreover, starting from this model, it is possible to speculate that, during progression, tumor growth would occur in a modular way that recalls the propagation of tumor spheres in vitro. All these considerations favor a comparison among normal blastocysts (as in vitro embryos), initial avascular tumors (as in vivo abnormal blastocysts) and tumor spheres (as in vitro abnormal blastocysts). In conclusion, this work provides further support for the para-embryonic nature of the cancer process, as recently theorized.
Introduction
It has been theorized recently that several similarities exist between the tumor process and the embryo development (Manzo, 2019). Starting from an initial cancer stem cell (i-CSC/CSC0), similar to an ESC without genomic homeostasis (para–ESC, p-ESC), after implantation in a niche, primary self-renewing cancer stem cells (CSC1s) would arise, corresponding to epiblast cells. CSC1s would then generate secondary proliferating CSCs (CSC2s), equivalent to hypoblast cells. CSC1s and CSC2s, with an epithelial phenotype, would generate, together, tertiary CSCs (CSC3s) with a mesenchymal phenotype, corresponding to mesodermal precursors at the primitive streak (PS). Under favorable stereotrophic conditions (normoxia), CSC3s would undergo asymmetric proliferation and pre-differentiation into cancer progenitor cells (CPCs) and then into cancer differentiated cells (CDCs), thus giving defined cell heterogeneity and hierarchy (Marjanovic et al., 2013; Singh et al., 2015), mimicking an ectopic rudimentary somito-histo-organogenesis process (Reya et al., 2001; Gibbs, 2009; Ma et al., 2010). In contrast, under unfavorable stereotrophic conditions (hypoxia), CSC3s would delaminate and migrate as quiescent micro-metastases, mimicking morphogenetic movements and localizing in metastatic niches (Cabrera et al., 2015; Singh et al., 2015; Yang et al., 2018). Here, specific signals, similar to those occurring in the gastrula inductions, would induce an EMT/MET switch (Thiery et al., 2009; Liu et al., 2014) reverting quiescent CSC3s to proliferating CSC1s. These cells would be able to generate macro-metastases with the same cell hierarchy as their primary tumors (Marjanovic et al., 2013). Now, I intend to show that the above-proposed tumor hierarchy, from CSCs to CDCs, allows the prediction of a tumor proliferation model that is in strong agreement with some experimental data reported for mammary tumors (Liu et al., 2014). Therefore, it is possible to identify specific mathematical relationships among cancer cells (CCs) occurring in the tumor mass. Moreover, this model suggests that during progression tumor growth might occur in a modular way, which recalls features of tumor spheres and pre-implantation blastocysts (Johnson et al., 2013; Vinnitsky, 2014).
Cell Heterogeneity, Hierarchy, and Plasticity in Cancer
The tumor bulk consists of several types of cells, encompassing Cancer cells (CCs), stroma cells, endothelial cells, and immune cells (Hanahan and Weinberg, 2011). In many tumors, phenotypic and functional heterogeneity among the various cells exists (Marjanovic et al., 2013; Singh et al., 2015), arising from different factors: endogenous, like genetic (mutations) and epigenetic (miRNA, HLA-G, HIF, TGF-beta, BMP); and exogenous, such as niche contact, microenvironment nutrients, pH, space, chemotherapeutic agents. Currently, three different theories try to explain the cell hierarchy and heterogeneity in tumors: (a) the clonal evolution model, (b) the classical CSC model, and (c) the plastic CSC model (Singh et al., 2015). The clonal evolution model proposes that stochastic accumulating mutational events create raw material for the selection of clones of novel cell populations in the same tumor. Each of these cells would be able to generate metastases with particular features, which are different for other metastases and primary tumors. Since it is generally shown that metastases recapitulate the cell hierarchy of the primary tumor in terms of cell type and percentage (Gupta et al., 2011; Marjanovic et al., 2013; Liu et al., 2014; Cabrera et al., 2015; Singh et al., 2015), the clonal model seems unrealistic. The classical CSC model proposes that tumor heterogeneity arises from CSCs that transit through different states (epithelial and mesenchymal) of stemness and differentiation (CPCs and CDCs) by unidirectional conversion from CSCs to non-CSCs (Singh et al., 2015). This model, where CSCs would be at the apex of the process, might better account for heterogeneity and hierarchy of cells in the same tumor, but it does not account for recent reports showing that non-CSCs might revert to CSCs (Chaffer et al., 2011; Gupta et al., 2011; Kim et al., 2013; Singh et al., 2015; Lu et al., 2020). The plastic CSC model proposes that bidirectional conversions are possible between CSCs and non-CSCs, suggesting that during the tumor process, non-CSCs might be induced into CSCs, thus creating new tumor populations (Chaffer et al., 2011; Kim et al., 2013; Singh et al., 2015; Lu et al., 2020). This model might account for both heterogeneity and hierarchy by plasticity of non-CSCs through a context-dependent behavior influenced by microenvironmental signals. Recently, I suggested that tumor heterogeneity and hierarchy might result from the para-embryonic nature of the i-CSC/CSC0 (Manzo, 2019), which, by the reactivation of an intrinsic genic program, would give rise to a sort of ectopic rudimentary somito-histo-organogenesis, tracing in some way that of the tissue of origin (Reya et al., 2001; Gibbs, 2009; Levings et al., 2009; Ma et al., 2010). Here, naturally epithelial, mesenchymal, progenitor, and differentiated tumor cells would be progressively generated (Bradshaw et al., 2016). Such a genic program would also be realized within related macro-metastases, accounting for the fact that, in general, metastatic cell heterogeneity and hierarchy recapitulate those of the primary tumor. On the other hand, stochastic mutations in the genic program of some CSCs or epigenetic and micro-environmental factors would also be responsible for metastases with a cell heterogeneity different from that in the primary tumor. The plasticity of non-CSCs reverting to a CSC state might be made possible by the genetic instability caused by the absence of genomic homeostasis in the i-CSC/CSC0 and handed down throughout all its progeny, including CDCs. This instability would allow non-CSCs to be de novo reactivated (neo-re-programmed) in their pluripotency gene regulatory network (OCT4, SOX2, NANONG, KLF4, MYC) by endogenous, niche and/or microenvironmental signals, probably in a different way from the original i-CSC/CSC0, thus generating new tumor cell populations (Iliopulos et al., 2011; Kim et al., 2013; Cabrera et al., 2015; Singh et al., 2015; Yang et al., 2018; Lu et al., 2020). Depending on its genetic, epigenetic and microenvironment conditions, a tumor cell could thus realize a defined genic program (“inductive gene chain”) that confers specific phenotypic and physio-pathological features, responsible for a peculiar cell heterogeneity and hierarchy.
Theoretical Proliferation Model in Cancer: The Tumor Growth Module
On the basis of the hypothesized p-ESC nature of the i-CSC/CSC0, I propose the following model for the establishment of cell heterogeneity and hierarchy within the tumor histological structure (Figure 1). In a merely theoretical way, considering an i-CSC/CSC0 and a niche able to contain only (for simplicity) two CSCs, the following events would occur: (a) allocation of i-CSC/CSC0 in a niche, at the apex of the entire process, and subsequent CSC0/CSC1 transition (Nichols and Smith, 2009); (b) initial expanding symmetrical (Morrison and Kimble, 2006; Norton and Popel, 2014) self-renewal of CSC1, yielding two epithelial CSC1s anchored to the niche (Niola et al., 2012); (c) committing asymmetrical (Knoblich, 2008; Pattabiraman and Weimberg, 2014) self-renewal of CSC1s, each yielding a maternal CSC1 at a central position in the niche and a committed epithelial daughter CSC2 in a sub-central position at the niche boundaries (Liu et al., 2014; Norton and Popel, 2014); (d) asymmetrical (Knoblich, 2008; Pattabiraman and Weimberg, 2014) autocrine/paracrine proliferation of CSC2s, each yielding a maternal epithelial CSC2 and (via EMT) a mesenchymal daughter CSC3 in a more peripheral position; (e) quiescence of more internal CSC3s and their migration externally at the tumor invasive front (Liu et al., 2014; Staneva et al., 2019); (f) asymmetrical (Knoblich, 2008; Norton and Popel, 2014; Pattabiraman and Weimberg, 2014) division of more external CSC3s, each yielding a maternal CSC3 and a CPC in a more peripheral position of the process (Liu et al., 2014; Staneva et al., 2019); and (g) asymmetrical (Knoblich, 2008; Pattabiraman and Weimberg, 2014) differentiation division of CPCs, each yielding a maternal CPC and a CDC, at the interface with the host normal tissues. Within this proliferation model, CSC1s-CSC2s-CSC3s-CPCs-CDCs would constitute a defined “tumor growth module.” It is possible that such a theoretical proliferation model might account for (1) the various types of CCs present in the bulk of mammary tumors (Liu et al., 2014); (2) the different (epithelial and mesenchymal) CSC phenotypes (ALDH1+ CD44+ Ki67+/hypothetical CSC1; ALDH1+ CD44– Ki67+/hypothetical CSC2; ALDH1– CD44+ Ki67–/hypothetical CSC3) detected in mammary tumors (Liu et al., 2014; Manzo, 2019); (3) the hierarchy of the various CSCs and non-CSCs present in a tumor (Liu et al., 2014); (4) the histological tumor structure, where CSCs would naturally remain internal, surrounded by more differentiated tumor cells (Liu et al., 2014; Singh et al., 2015); and (5) the position of CSC3s generated at early divisions, which would become progressively more external and proximal to the normoxic host tissues, where favorable micro-environmental conditions (space, oxygen, nutrients, pH) exist (Figure 1). Here, they could undergo EMT/MET switch, subsequent asymmetric division and differentiation in CPCs and then in CDCs, thus generating a growth module with a defined cell hierarchy, responsible for a peripheral finger-like morphology (Norton and Popel, 2014). With regards to the CDCs, the question arises of whether or not they are still proliferating: in general, proliferation and differentiation are mutually exclusive, as also it occurs in CCs (Ruijtenberg and van den Heuvel, 2016). However, coincident occurrence of cell division and a differentiated state have also been reported in CCs (Sage et al., 2005; Ajioka et al., 2007); moreover, the eventual occurrence of dividing pre-differentiated CCs must be considered. In contrast, CSC3s generated at later divisions would remain more internal and thus under unfavorable hypoxic conditions. Consequently, in an attempt to survive, they would migrate externally in spatially coordinated migration patterns (Thiery et al., 2009; Tiwari et al., 2012; Staneva et al., 2019), at the interface with normal vascularized host tissues, where better stereo-trophic conditions exist, thus creating an invasive front. Here, they could install in metastatic niches as dormant CSC3s by EMT signals (WNT, TGFb) and eventually revert to self-renewing CSC1s by MET signals (BMP, LIF) (Thiery et al., 2009; Liu et al., 2014; Grosse-Wilde et al., 2015).
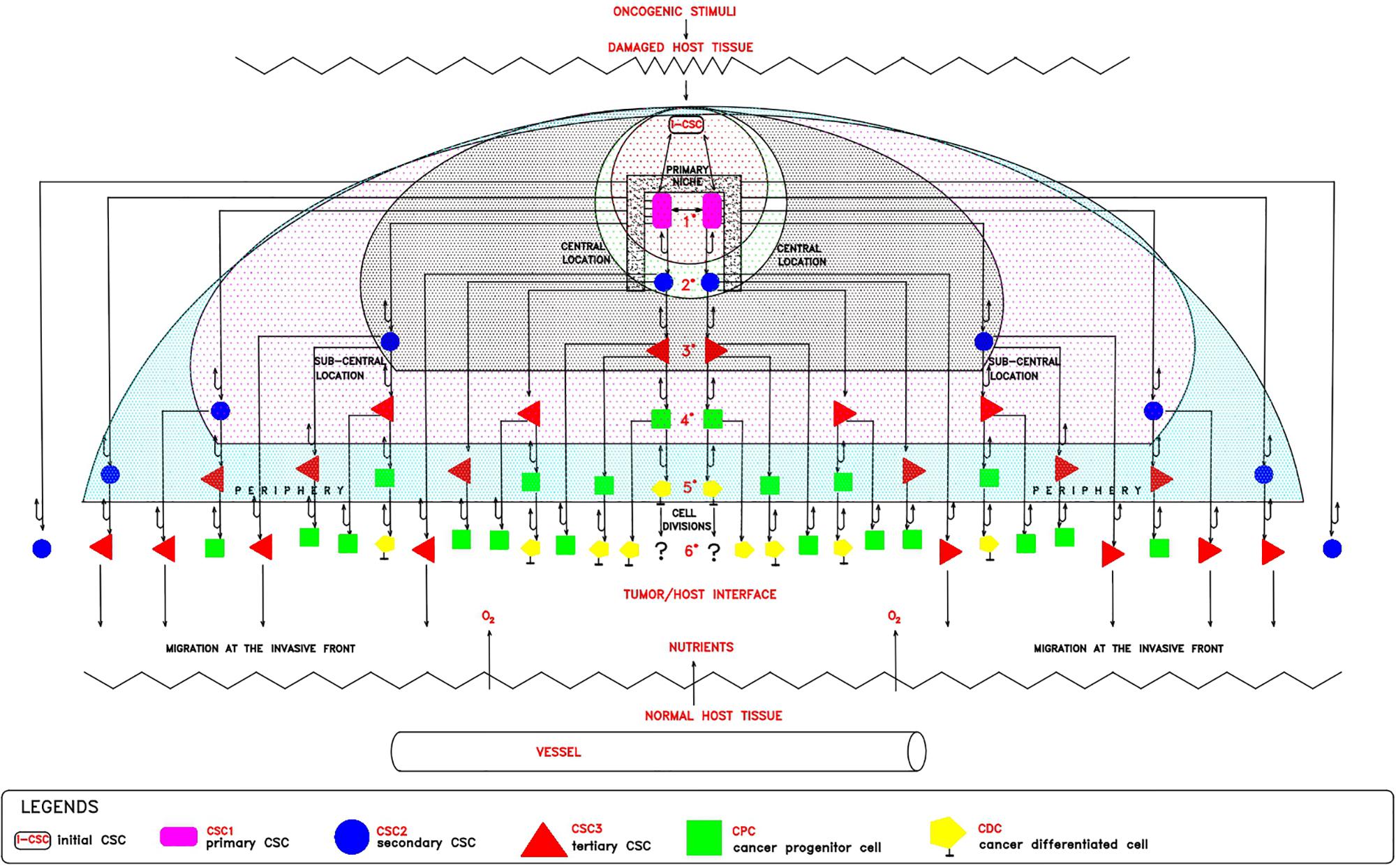
Figure 1. Theoretical tumor growth model: cell hetrogeneity and hierarchy. In a damaged host tissue, the initial cancer stem cell (iCSC/CSCo, white rectangle) would install itself in a primary niche (black square), where, by expanding symmetrical self-renewal, would generate 2 epithelial CSC1s (violet rectangles) located at a central position. Each CSC1, by committing asymmetrical self-renewal, would generate 1 maternal CSC1, remaining at a central position in the niche, and 1 epithelial committed CSC2 (blue circles), located at a peri-niche sub-central position. Each CSC2, by asymmetrical autocrine/paracrine division, would generate 1 maternal CSC2 and 1 mesenchymal CSC3 (red triangles), located in a more peripheral position. After a certain division number, CSC3s generated at early stages, would become more external and proximal to the normoxic host tissue, where they proliferate by asymmetrical division, yielding 1 maternal CSC3 and 1 pre-differentiated CPC (green squares) located at the border of the process, at the interface between the tumor and the host. CSC3s generated at later stages, more internal and thus under hypoxic conditions, would remain quiescent and migrate at the invasive front in search of survival conditions in metastatic niches. Each CPC, by asymmetrical division, would yield 1 maternal CPC and 1 differentiated CDC (yellow pentagons). At this point, the question arises as to whether or not CDCs, differentiated but genetically unstable, could further proliferate.
Modular Growth in Avascular Tumors
The proposed proliferation model would generate tumor growth modules (CSC1-CSC2-CSC3-CPC-CDC) that might be at the basis of and account for the structure and features of the avascular tumor bulk. In particular, mesenchymal CSC3s generated early in a tumor growth module would lie in favorable stereotrophic conditions, so they could proliferate, yielding a progeny of CPCs and then CDCs. This progeny could form a hierarchic histological structure that might appear as growth-cordfingers (Norton and Popel, 2014; Figure 2). On the other hand, mesenchymal CSC3s generated later within a growth module would lie in unfavorable stereotrophic conditions, so they would be induced to migrate externally for survival. If they find a new niche, they would self-seed (Norton and Popel, 2014) and, by specific signals, undergo EMT/MET switch, becoming self-renewing CSC1s able to generate new tumor modules. In such a way, tumor growth could occur by reiterated production of defined cell modules, generating a spherical avascular mass. This might expand until it reaches a diameter of approximately 400 microns, since diffusion and the supply of nutrients and oxygen at the core cells is not possible beyond about 200 microns (Hamilton and Rath, 2019). Assuming for the module cells a middle diameter of about 15 microns, this fact would imply that an avascular tumor bulk might contain about 13 tumor cell layers. Beyond this limit, tumor avascular growth could occur only externally with a simultaneous death of core cells. In such a way, an advanced avascular tumor mass could be a sphere made of (a) an anoxic central zone with necrotic tumor cells, presumably the earlier tumor modules; (b) a sub-central hypoxic zone with the later generated quiescent CSC3s that try to migrate externally (Staneva et al., 2019) in search of niches to self-seed around or for metastasizing elsewhere (Norton and Popel, 2014); and (c) a peripheral normoxic zone with the earlier generated proliferating CSC3s and their numerous progeny of CPCs and CDCs, resulting together in a cord-finger morphology (Norton and Popel, 2014; Figures 2, 3). Thus, this tumor proliferation model would generate structures that appear to be very similar to real initial avascular tumors and multicellular tumor spheroids (MCTS) (Millard et al., 2017; Hamilton and Rath, 2019; Scientific Reports and Nature Research, 2019; Figure 4).
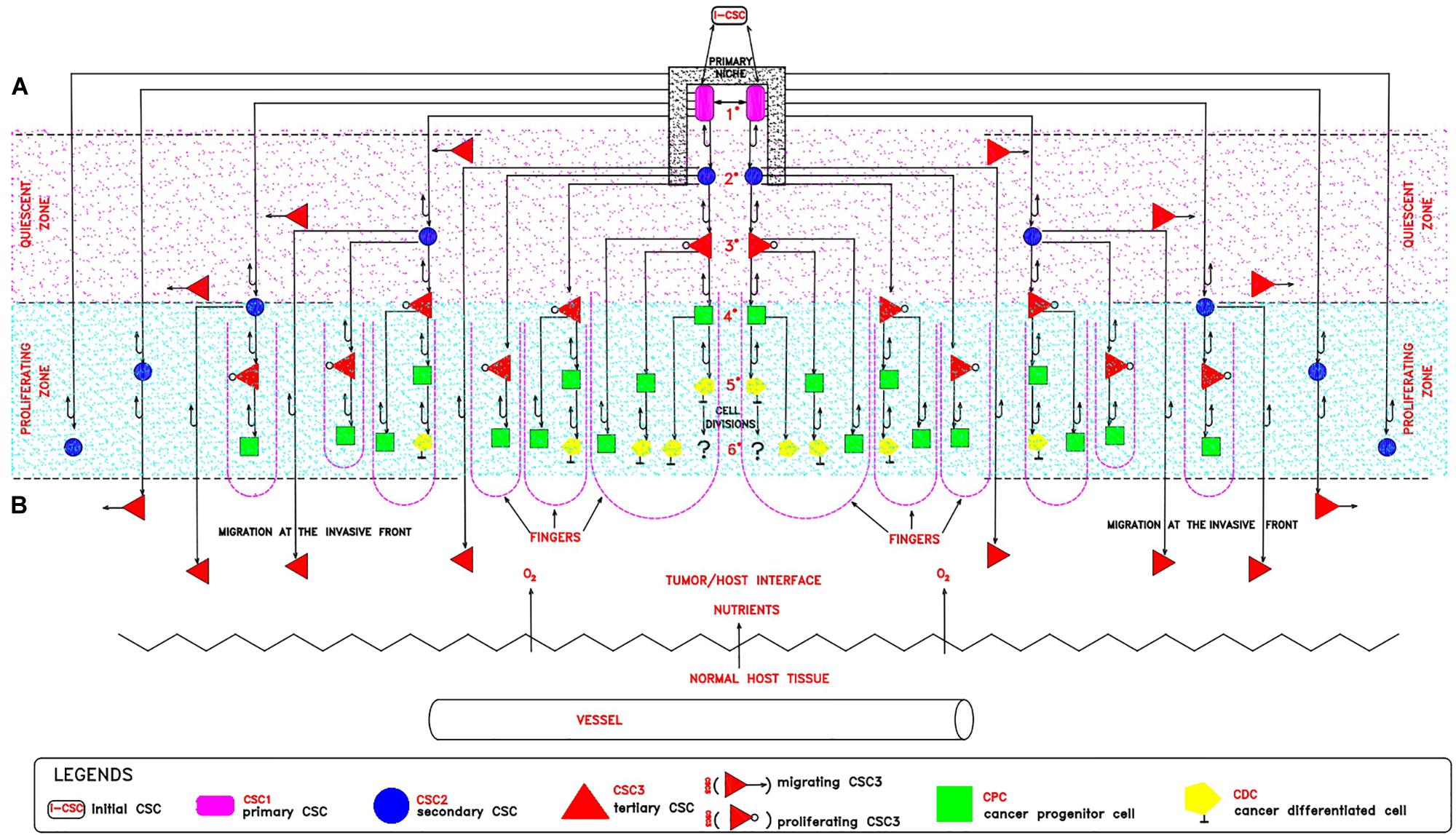
Figure 2. Theoretical tumor growth module: a cord-finger structure. In a tumor growth module, it is possible to distinguish: (A) An internal hypoxic zone (pink color) where quiescent CSC3s lie, generated at later divisions and migrating externally in spatially coordinated patterns, toward the tumor/host interface, endowed with more favorable stereo-trophic conditions, thus creating an invasive front. Here, they could install in metastatic niches as dormant CSC3s by EMT environmental signals (WNT, TGFb) and eventually revert to self-renewing CSC1s by MET signals (BMP, LIF). (B) An external normoxic zone (light-blue color), where CSC3s lie, generated at earlier divisions and thus more proximal to the tumor/host interface, where favorable micro-environmental conditions (space, oxygen, nutrients, pH) exist. Here, these cells could undergo EMT/MET switch, subsequent asymmetric division and differentiation in CPCs and then in CDCs, thus generating a growth module with a defined cell hierarchy, responsible for a peripheral finger-like morphology (violet broken lines). With regards to the CDCs, the question arises of whether or not they are still proliferating.
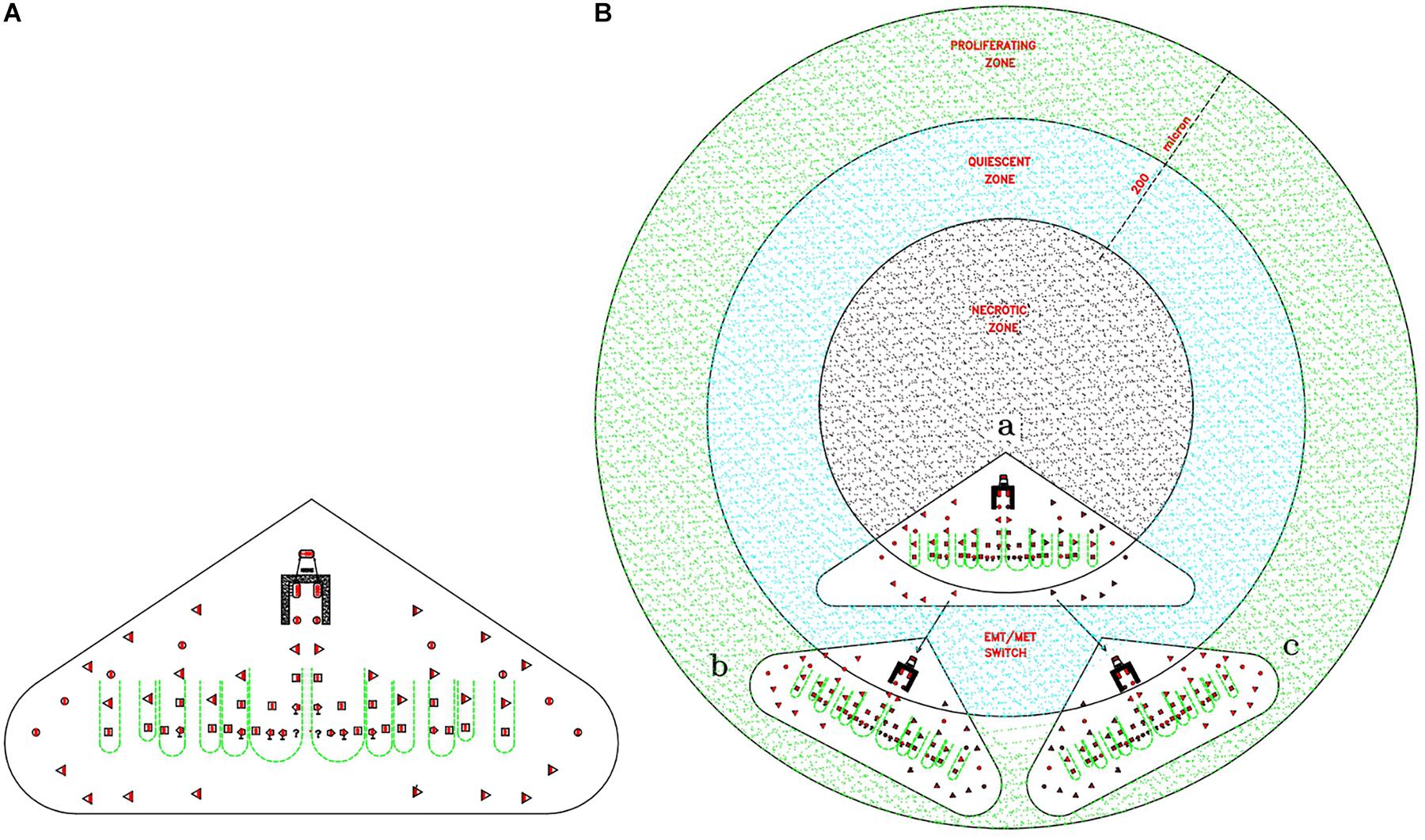
Figure 3. Theoretical relationships between tumor gowth modules and avascular tumors. (A) Cord-finger structure of a tumor growth module. This image is directly extrapolated from the Figure 2B. (B) Theoretical modular growth in an avascular tumor: (a) Initial tumor growth module, located in the central zone, the future necrotic zone (black color). (b) and (c) Secondary tumor growth modules, arising from migrating CSC3s, seeding via EMT/MET switch in surrounding niches, located in the sub-central zone, the future quiescent zone (blue color). Since nutrient diffusion limits are about 200 microns, the primary module comes to lie in the necrotic zone and dies, whereas the later modules located in the quiescent/proliferating zones (blue/green zones) grow, conferring a peripheral finger morphology on the structure. This structure could grow beyond defined limits only if its vascularization occurs, able to supply the necessary nutrients.
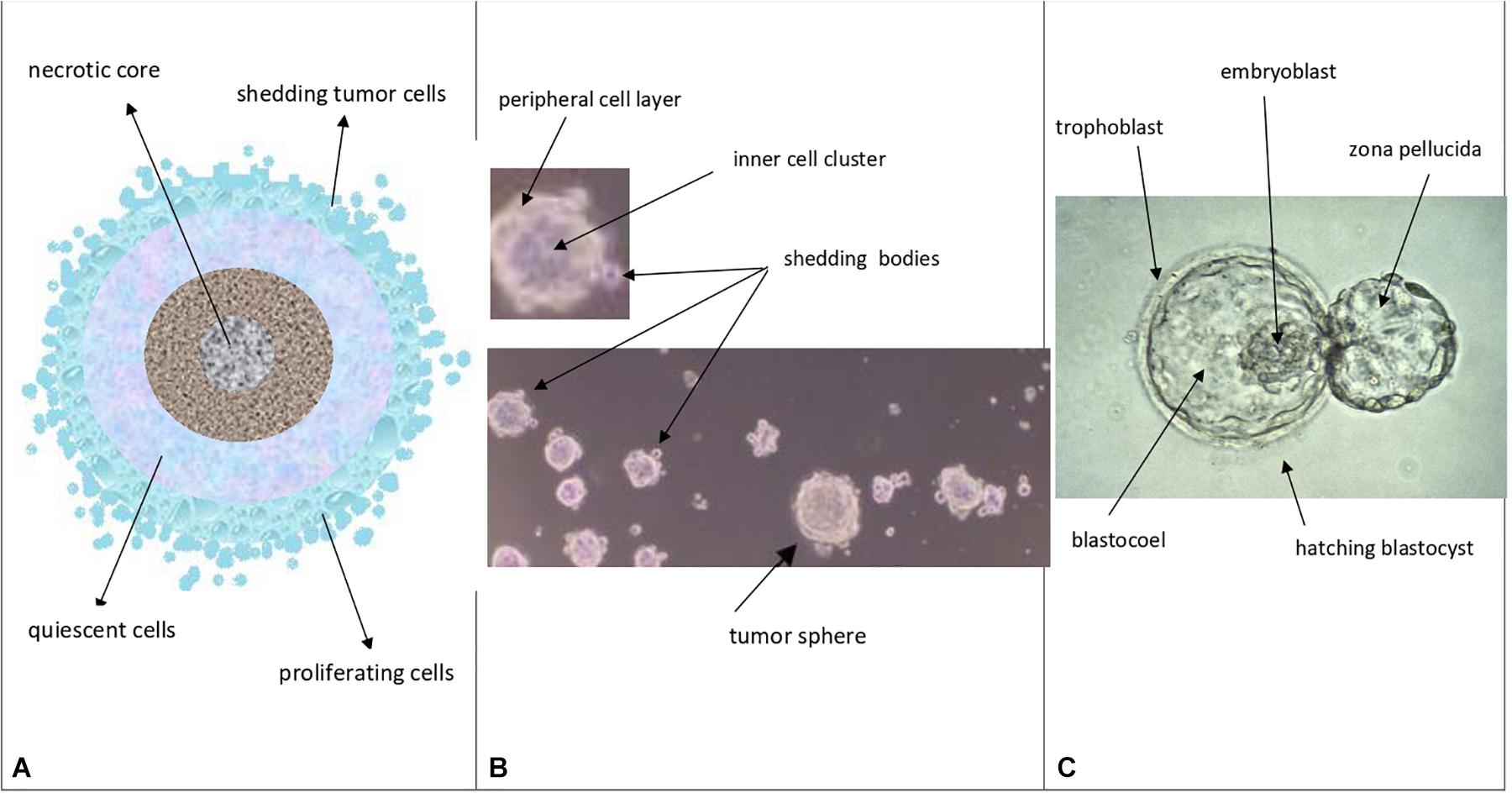
Figure 4. Theoretical comparison among avascular tumors (A), tumor spheres (B), and normal blastocysts (C) A. Avascular tumors (as in vivo abnormal blastocysts): external proliferating cell layers (light-blue color), middle quiescent cells (gray color) and necrotic core cells (dark colors) are depicted. B. Tumor spheres (as in vitro abnormal blastocysts): a layered cell distribution, like in avascular tumors, a peripheral cell layer, similar to the blastocyst trophectoderm, and an inner cell cluster, similar to the blastocyst ICM can be observed; shedding bodies from the sphere surfaces can also be noted (modified and adapted from Bond et al., Plos One. 2013). C. Normal hatching blastocysts (as in vitro embryos): trophectoderm, ICM (embryoblast) and blastocoel cavity are indicated, together to the “zona pellucida” (modified from: Human blastocyst hatching. Credit: K. Hardy. CC BY).
Mathematical Relationships Among Cancer Cells
In the model proposed in Figure 1 it is possible to detect numerical relationships among all the CC typologies in a tumor, which surprisingly agree with experimental data shown in a study on 45 primary breast tumors (Liu et al., 2014). By tabulating the theoretical data proposed in Figure 1, it is possible to find well-defined mathematical relationships between CSCs (CSC1s, CSC2s, CSC3s) and non-CSCs (CPCs, CDCs) at each (n) cell division. Starting from a hypothetical low and stable number (two, for simplicity) of CSC1s in a niche, for each (n) division, it is possible to define the following relationships (Table 1):
These relationships theoretically allow us to know, at each (n) division, a presumed total CC number as a sum of the number of each cell typology. In particular, it might be noted that (a) for values of (n) from 0 to 5, the total CC number is expressed as a numerical doubling (2n) (Table 1h,i) (b) from a value (n) of 6, the total CC number decreases progressively compared with (2n) (Table 1h,i), because of missing CDCs (mCDCs), in a quantity expressed by the relationship (Table 1g):
(c) at a value (n) of 10, the presumed CC number is exactly half of (2n), at a value (n) of 11, about one third, and at a value (n) of 12, a little more than one fourth (Table 1h,i). Thus, the total CC number appears to become progressively more self-limiting; nevertheless, the CSC quantity decreases gradually compared with the total CCs, according to the experimental ratio reported in the literature (Table 1j).
(d) At a value (n) of 10, the percentages of CSC1s, CSC2s, and CSC3s result, respectively, in 0.39, 3.51, and 14.06%, with a total of 17.96% CSCs. Surprisingly, these theoretical data, concerning a single niche, are strongly similar to the experimental data found in the mammary tumor mass, namely: 0.084% for ALDH1+ CD44+ Ki67+ CSCs (hypothetical CSC1s); 5.54% for ALDH1+ CD44– Ki67+ CSCs (hypothetical CSC2s); 12.87% for ALDH1– CD44+ Ki67– CSCs (hypothetical CSC3s); and 18.494% for total CSCs (Liu et al., 2014). These similar (presumed/experimental) percentages (about 18%) also occur starting from a niche with a different (3, 4,…) initial CSC1 number. Inside these percentages, the discrepancy for CSC1s (0.39 to 0.084%, about 5 to 1) and CSC2s (3.51 to 5.54%, about 1 to 2) might be due to the fact that the proliferation rate for a single theoretical niche is assumed as defined, while in the tumor bulk many niches could have asynchronous growth and a variable proliferation rate. Moreover, in a computational model, stem cell percentages have been found to be between 0.2 and 15%, depending on the simulation parameters (Norton and Popel, 2014). These similarities might thus indicate a true correspondence between ALDH1+ CD44+ Ki67+CSCs and CSC1s, ALDH1+ CD44– Ki67+CSCs and CSC2s, ALDH1– CD44+ Ki67– CSCs and CSC3s, and, consequently, a possible real existence of the hypothesized CSC1s, CSC2s, and CSC3s (Manzo, 2019).
Similarities Among Avascular Tumors, Tumor Spheres, and Blastocysts
Notably, at (n) = 10, many important events seem to occur, as described above. At (n) > 10, the correspondence (about 18%) between presumed (17.96%) and experimental (18.494%) CSCs for a single niche tends to diminish progressively. Since the experimental data refer to tumor bulks, certainly with more than 10 cell divisions, it would be possible to question how this correspondence might be conserved in the tumor mass. I therefore hypothesize that it might occur through the proposed “modular growth,” which is able to maintain these percentages throughout tumor progression. In particular, this might be possible if, as proposed earlier, CSC1s-CSC2s-CSC3s-CPCs-CDCs together constituted a tumor growth module (Manzo, 2019; Figure 2). This would self-generate after about 10 division cycles, when the cell number would become presumably too large to survive under unfavorable stereo-trophic conditions (Hamilton and Rath, 2019). For this reason, some CSC3s would be induced to delaminate, migrate, and localize in new local or distant niches, where, after EMT/MET switch, they would revert to CSC1s (O’Brien et al., 2012; Liu et al., 2014; Grosse-Wilde et al., 2015; Yang et al., 2018; Manzo, 2019) and repeat the modular growth process, thus generating structures with a defined cell heterogeneity and hierarchy (Knoblich, 2008; Johnson et al., 2013; Vinnitsky, 2014). A modular growth process appears to occur also when CSCs cultured in vitro under defined conditions form solid, round cellular structures with a diameter of about 50–250 microns, named tumor spheres, through joining of smaller aggregates (spheroids), similar to -single tumor modules (Hamilton and Rath, 2019). Spheroids are also found in vivo, as circulating tumor clusters, in ascitic fluid of ovarian cancer and pleural effusions of lung cancers, arising by collective detachment from the tumor bulk (Hamilton and Rath, 2019). These spheroids have a smaller size without the hypoxia and necrotic regions observed in larger 3D structures (Hamilton and Rath, 2019). Tumor spheres are enriched in CSCs, but they also contain non-CSCs, less or more differentiated (CPCs, CDCs) (Cao et al., 2011; Johnson et al., 2013; Liu et al., 2013). The CSCs are endowed with persistent self-renewal, stemness gene expression, high invasiveness, increased tumorigenic potential, and chemo-resistance (Cao et al., 2011; Liu et al., 2013). In such CSCs, expression of NANOG, OCT4, and SOX2 is present, as well as that of ALDH1 and KLF4 (epithelial markers) and CD44 (mesenchymal marker) (Liu et al., 2013). Tumor spheres are tridimensional and mimic the micro-environmental conditions and growth of real tumors. Tumor-sphere cultivation is widely used to analyze the self-renewal capability of CSCs and to enrich these cells from bulk CCs, thus providing a reliable platform for screening potential anti-CSC agents (Knoblich, 2008; Nunes et al., 2018). Large spheroids (400–500 microns in diameter) display a layered cell distribution, also observed in solid avascular tumors: the outer layers are enriched with highly proliferating cells, the middle zone exhibits quiescent cells, and the core contains necrotic cells and acellular regions with hypoxia and nutrient depletion (Norton and Popel, 2014; Galateanu et al., 2016; Millard et al., 2017). Very large tumor spheroids can reach 650 microns in diameter (Zanoni et al., 2016). Morphologically, tumor spheres appear to be defined by a cell layer that resembles the trophectoderm in blastocysts and a cluster of inner cells that resembles the ICM, just like in a preimplantation blastocyst (Johnson et al., 2013; Vinnitsky, 2014) (Figures 4B,C). I suggest that tumor spheres could be an artificial condition mimicking in vitro the natural conditions of normal pre-implantation blastocysts (Cao et al., 2011; Vinnitsky, 2014; Nunes et al., 2018), as well as those of in vivo avascular tumors (Figures 4A–C and Table 2). Thus, I hypothesize that tumor spheres might be a sort of artificial rudimentary (abnormal) blastocysts which, cultured in vitro onto ultralow attachment surfaces in the absence of implantation conditions, display a modular growth behavior similar to that of avascular tumors in vivo (Vinnitsky, 2014). This modular growth would also be confirmed by the images of small “shedding” structures, similar to single tumor modules, recently shown on the tumor-sphere surface and released in the surrounding micro-environment (Johnson et al., 2013; Hamilton and Rath, 2019; Figure 4B). The release of such structures resembles and could reflect in some way the “hatching” phenomenon of expanded pre-implantation blastocysts, by which these emerge from the zona pellucida to acquire a condition fit for subsequent implantation (Hardy et al., 1989; Figure 4C). Larger tumor spheres could maintain their state by inducing the release of cells exceeding a cell number (250–280) which would be optimal for eventual implantation. In the absence of micro-environmental conditions that favor implantation, normal blastocysts in the uterus die, while tumor spheres with defined in vitro conditions survive and spread, producing shedding growth modules. These would be presumably similar to in vivo initial avascular tumors (Vinnitsky, 2014), which could survive as such (dormant) in the absence of suitable implantation conditions or progress in the presence of such conditions. Multicellular tumor spheroid models closely mimic small avascular tumors in vivo, with the presence of proliferative cells (about 40%) surrounding quiescent cells and a necrotic core, and with similar gradients of oxygen, pH, and nutrients (Millard et al., 2017; Hamilton and Rath, 2019) (Figure 4B). It has been proposed that tumor spheres fulfill the precondition for a protected niche for dormant tumor cells as an hypoxic niche protected by the outer layers, which exhibit continuous shedding of tumor cells and fragments (Johnson et al., 2013; Hamilton and Rath, 2019).
Discussion and Conclusion
The hypothesis that oncogenesis might be a sort of ectopic rudimentary ontogenesis (Manzo, 2019) would permit us to formulate some considerations and potential explanations for several phenomena: a-Tumor cell heterogeneity and hierarchy, similar in primary and metastatic tumors, might be a natural consequence of the developmental genic program of a de-re-programmed i-CSC/CSC0 endowed with para-embryonic features (p-ESC) (Manzo, 2019). b-The plasticity of non-CSCs in the CSC conversion might be made possible by the early genomic instability of the i-CSC/CSC0, handed down throughout all its progeny. Therefore, thanks to this condition, a non-CSC could be newly re-programmed in CSC by intrinsic and/or extrinsic signals, eventually also in a different way to the original i-CSC, thus potentially giving rise to a new tumor cell population, with new co-existent heterogeneity and hierarchy arising in the same primary tumor. c-The proposed cell hierarchy model (Figure 1) might account for the global tumor structure shown in mammary tumors (Liu et al., 2014), namely the distinction between CSCs and non-CSCs, the different detected CSC phenotypes, the reciprocal allocation of the different CSCs in the tumor mass, the internal position of CSCs to the external position of the non-CSCs. d-Tabulation of the above proposed cell hierarchy model (Figure 1) permits the elaboration of well-defined formulae for calculating the presumed number of each CC typology and, consequently, the presumed total number of CCs and the CSCs/CCs ratio after (n) cell division (Table 1j). This presumed ratio clearly appears to decrease progressively, in agreement with the experimental data reported in the literature. e-However, the presumed total number of CCs seems to be self-limiting for the occurrence of mCDCs. mCDCs could be the result of a lack of further proliferation of CDCs; but, if CDCs were still proliferating, this fact could be due to a natural apoptotic cell death, similarly to what occurs in embryos throughout ontogenesis (Hardy et al., 1989) and in multicellular spheroids (Nunes et al., 2018). In the embryo, widespread cell death by apoptosis in both TE and ICM normally occurs, increasing substantially by about day 7 (Hardy et al., 1989), namely from the 6/7° “one per day” division. Surprisingly, in Table 1, the onset of mCDC occurred just by the 6°cell division and then increased progressively. f-The major indication, resulting from Table 1, is the surprising similarity between the presumed and experimental percentage values for CSCs (ALDH1+ CD44+ Ki67+/CSC1; ALDH1+ CD44– Ki67+/CSC2, ALDH1– CD44+ Ki67–/CSC3), totaling approximately 18% for mammary tumors. g-Such a quantitative correspondence (about 18%) for CSCs could not be a simple coincidence and, if so, constitute a strong indication for the real existence of CSC1s, CSC2s, and CSC3s (Liu et al., 2014; Manzo, 2019). h-CSC1-CSC2s-CSC3s-CPCs-CDCs, together, could constitute a real tumor progression module that determines modular growth able to maintain a substantially constant ratio of about 18% for CSCs in the tumor mass, as detected in mammary tumors (Liu et al., 2014). In conclusion, I believe this work might contain and supply further indications sustaining the para-embryonic nature of the cancer process, as recently theorized (Manzo, 2019).
Author Contributions
GM conceived the theory and wrote the manuscript.
Conflict of Interest
The author declares that the research was conducted in the absence of any commercial or financial relationships that could be construed as a potential conflict of interest.
References
Ajioka, I., Martins, R. A. P., Bayazitov, I. T., Donovan, S., Johnson, D. A., Frase, S., et al. (2007). Differentiated horizontal interneurons clonally expand to form metastatic retinoblastoma in mice. Cell 131, 378–390. doi: 10.1016/j.cell.2007.09.036
Bradshaw, A., Wickremsekera, A., Tan, S. T., Peng, L., Davis, P. F., and Itinteang, T. (2016). Cancer stem cell hierarchy in glioblastoma multiforme. Front. Surg. 3:21. doi: 10.3389/fsurg.2016.00021
Cabrera, M. C., Hollingsworth, R. E., and Hurt, E. M. (2015). Cancer stem cell plasticity and tumor hierarchy. World J. Stem Cells 7, 27–36. doi: 10.4252/wjsc.v7.i1.27
Cao, L., Zhou, Y., Zhai, B., Liao, J., Xu, W., Zhang, R., et al. (2011). Sphere-forming cell subpopulations with cancer stem cell properties in human hepatoma cell lines. BMC Gastroenterol. 11:71. doi: 10.1186/1471-230X-11-71
Chaffer, C. L., Brueckmann, I., Scheel, C., Kaestli, A. J., Wiggins, P. A., Rodrigues, L. O., et al. (2011). Normal and neoplastic non-stem cells can spontaneously convert to a stem-like state. Proc. Natl. Acad. Sci. U S A. 108, 7950–7955.
Galateanu, B., Hudita, A., Negrei, C., Ion, R. M., Costache, M., Stan, M., et al. (2016). Impact of multicellular tumor spheroids as an in vivo-like tumor model on anticancer drug response. Int. J. Oncol. 48, 2295–2302. doi: 10.3892/ijo.2016.3467
Gibbs, C. P. (2009). Cancer Stem Cells in Sarcoma. V6N4 ESUN Editorial. New York, NY: Liddy Shriver sarcoma initiative.
Grosse-Wilde, A., Fouquier, d’Herouel, A., McIntosh, E., Ertaylan, G., Skupin, A., et al. (2015). Stemness of the hybrid epithelial/mesenchymal state in breast cancer and its association with poor survival. PloS One 10:e0126522. doi: 10.1371/journal.pone.0126522
Gupta, P. B., Fillmore, C. M., Jiang, G., Shapira, S. D., Tao, K., Kuperwasser, C., et al. (2011). Stochastic state transitions give rise to phenotypic equilibrium in populations of cancer cells. Cell 146, 633–644. doi: 10.1016/j.cell.2011.07.026
Hamilton, G., and Rath, B. (2019). Role of circulating tumor cell spheroids in drug resistance. Cancer Drug Resist. 2, 762–772. doi: 10.20517/cdr.2019.47
Hanahan, D., and Weinberg, R. A. (2011). Hallmarks of cancer: the next generation. Cell 144, 646–674. doi: 10.1016/j.cell.2011.02.013
Hardy, K., Handyside, A. H., and Winston, R. M. L. (1989). The human blastocyst: cell number, death and allocation during late preimplantation development in vitro. Development 107, 597–604.
Iliopulos, D., Hirsch, H. A., Wang, G., and Struhl, K. (2011). Inducible formation of breast cancer stem cells and their dynamic equilibrium with non-stem cancer cells via IL6 secretion. Proc. Natl. Acad. Sci. U S A. 108, 1397–1402. doi: 10.1073/pnas.1018898108
Johnson, S., Chen, H., and Lo, P. K. (2013). In vitro tumor-sphere formation assays. Bio-protocol 3:e325. doi: 10.21769/Bio-Protoc.3
Kim, S. Y., Kang, J. W., Song, X., Kim, B. K., Yoo, I. D., Kwon, Y. T., et al. (2013). Role of the IL6-JAK1- STAT3-OCT4 pathway in the conversion of non-stem cancer cells into cancer stem-like cells. Cell Signal 25, 961–969. doi: 10.1016/j.cellsig.2013.01.007
Knoblich, J. A. (2008). Mechanisms of asymmetric stem cell division. Cell 132, 583–597. doi: 10.1016/j.cell.2008.02.007
Levings, P. P., Mcgarry, S. V., Currie, T. P., Nickerson, D. M., McClellan, S., Ghivizzani, S. C., et al. (2009). Expression of an exogenous OCT-4 promoter identifies tumor-initiating cells in osteosarcoma. Cancer Res. 69, 5648–5655. doi: 10.1158/0008-5472.can-08-3580
Liu, J., Ma, L., Xu, J., Liu, C., Zhang, J., Liu, J., et al. (2013). Spheroid body-forming cells in the human gastric cancer cell lineMKN-45 possess cancer stem cell properties. Int. J. Oncol. 42, 453–459. doi: 10.3892/ijo.2012.1720
Liu, S., Cong, Y., Wang, D., Sun, Y., Deng, L., Liu, Y., et al. (2014). Breast cancer stem cells transition between epithelial and mesenchymal states reflective of their normal counterparts. Stem Cell Rep. 2, 78–91. doi: 10.1016/j.stemcr.2013.11.009
Lu, H., Xie, Y., Tran, L., Lan, J., Yang, Y., Murugan, N. L., et al. (2020). Chemotherapy-induced S100A10 Recruits KDM6A to Facilitate OCT4-mediated Breast Cancer Stemness. J. Clin. Invest. doi: 10.1172/JCI138577
CrossRef Full Text Online ahead of print. | PubMed Abstract | Google Scholar
Ma, Y., Zhang, P., Wang, F., Yang, J., Yang, Z., and Qin, H. (2010). The relationship between early embryo development and tumourigenesis. J. Cell. Mol. Med 14, 2697–2701. doi: 10.1111/j.1582-4934.2010.01191.x
Manzo, G. (2019). Similarities between embryo development and cancer process suggest new strategies for research and therapy of tumors: a new point of view. Front. Cell Dev. Biol. 7:20. doi: 10.3389/fcell.2019.00020
Marjanovic, N. D., Weinberg, R. A., and Chaffer, C. L. (2013). Cell plasticity and heterogeneity in cancer. Clin. Chem. 59, 168–179. doi: 10.1373/clinchem.2012.184655.
Millard, M., Yakavets, I., Zorin, V., Kulmukhamedova, A., Marchal, S., and Besdetnaya, L. (2017). Drug delivery to solid tumors: the predictive value of the multicellular tumor spheroid model for nanomedicine screening. Int. J. Nanomed. 12, 7993–8007. doi: 10.2147/IJN.S146927
Morrison, S. J., and Kimble, J. (2006). Asymmetric and symmetric stem cell divisions in development and cancer. Nature 441, 1068–1074. doi: 10.1038/nature04956
Nichols, J., and Smith, A. (2009). Naive and primed pluripotent states. Cell Stem Cell 4, 487–492. doi: 10.1016/j.stem.2009.05.015
Niola, F., Zhao, X., Singh, D., Castano, A., Sullivan, R., Lauria, M., et al. (2012). Id proteins synchronize stemness and anchorage to the niche of neural stem cells. Nat. Cell Biol. 14, 477–487. doi: 10.1038/ncb2490
Norton, K.-A., and Popel, A. S. (2014). An agent-based model of cancer stem cell initiated avascular tumor growth and metastasis: the effect of seeding frequency and location. J. R. Soc. Interface 11:20140640. doi: 10.1098/rsif.2014.0640
Nunes, A. S., Barros, A. S., Costa, E. C., Moreira, A. F., and Correia, I. J. (2018). 3D tumor spheroids as in vitro models to mimic in vivo human solid tumors resistance to therapeutic drugs. Biotechnol. Bioeng. 116, 206–226. doi: 10.1002/bit.26845
O’Brien, C. A., Kreso, A., Ryan, P., Hermans, K. G., Gibson, L., Wang, Y., et al. (2012). ID1 and ID3 regulate the self-renewal capacity of human colon cancer-initiating cells through p21. Cancer Cell. 21, 777–792. doi: 10.1016/j.ccr.2012.04.036
Pattabiraman, D. R., and Weimberg, R. A. (2014). Tackling the cancer stem cells – what challenges do they pose? Nat. Rev. Drug Disc. 13, 497–512. doi: 10.1038/nrd4253
Reya, T., Morrison, S. J., Clarke, M. F., and Weissman, I. L. (2001). Stem cells, cancer and cancer stem cells. Nature 414, 105–111. doi: 10.1038/35102167
Ruijtenberg, S., and van den Heuvel, S. (2016). Coordinating cell proliferation and differentiation: antagonism between cell cycle regulators and cell type-specific gene expression. Cell Cycle 15, 196–212. doi: 10.1080/15384101.2015.1120925
Sage, C., Huang, M., Karimi, K., Gutierrez, G., Vollrath, M. A., Zang, D.-S., et al. (2005). Proliferation of functional hair cells in vivo in the absence of the retinoblastoma protein. Science 307, 1114–1118. doi: 10.1126/science.1106642
Scientific Reports and Nature Research (2019). Growth and characterization of MTS. Berlin: Springer Nature Publishin AG.
Singh, A. K., Arya, R. K., Maheshwari, S., Singh, A., Meena, S., Pandey, P., et al. (2015). Tumor heterogeneity and cancer stem cell paradigm: updates in concept, controversies and clinical relevance. Int. J. Cancer 136, 1991–2000. doi: 10.1002/ijc.28804
Staneva, R., El Marjou, F., Barbazan, J., Krndija, D., Richon, S., Clark, A. G., et al. (2019). Cancer cells in the tumor core exhibit spatially coordinated migration patterns. J. Cell Sci. 132:jcs220277. doi: 10.1242/jcs.220277
Thiery, J. P., Acloque, H., Huang, R. J. Y., and Nieto, M. A. (2009). Epithelial-mesenchymal transitions in development and disease. Cell 139, 871–890. doi: 10.1016/j.cell.2009.11.007
Tiwari, N., Gheldof, A., Tatari, M., and Christofori, G. (2012). EMT as the ultimate survival mechanism of cancer cells. Semin. Cancer Biol. 22, 194–207. doi: 10.1016/j.semcancer2012.02.013
Vinnitsky, V. (2014). The development of a malignant tumor is due to a desperate asexual self-cloning process in which cancer stem cells develop the ability to mimic the genetic program of germline cells. Intrinsically Disord. Proteins 2:e29997. doi: 10.4161/idp.29997
Yang, X., Liang, X., Zheng, M., and Tang, Y. (2018). Cellular phenotype plasticity in cancer dormancy and metastasis. Front. Oncol. 8:505. doi: 10.3389/fonc.2018.00505
Keywords: tumor propagation, tumor hierarchy, cancer stem cell (CSC), tumor sphere, embryo
Citation: Manzo G (2020) Defined Mathematical Relationships Among Cancer Cells Suggest Modular Growth in Tumor Progression and Highlight Developmental Features Consistent With a Para-Embryonic Nature of Cancer. Front. Cell Dev. Biol. 8:804. doi: 10.3389/fcell.2020.00804
Received: 19 January 2020; Accepted: 29 July 2020;
Published: 28 August 2020.
Edited by:
Isabel Pires, University of Hull, United KingdomReviewed by:
Bèla Ózsvàri, University of Salford, United KingdomAamir Ahmad, Mitchell Cancer Institute, United States
Max Seymour Wicha, University of Michigan, United States
Copyright © 2020 Manzo. This is an open-access article distributed under the terms of the Creative Commons Attribution License (CC BY). The use, distribution or reproduction in other forums is permitted, provided the original author(s) and the copyright owner(s) are credited and that the original publication in this journal is cited, in accordance with accepted academic practice. No use, distribution or reproduction is permitted which does not comply with these terms.
*Correspondence: Giovanni Manzo, Z2lvdmFubmltYW56b2J0ckBnbWFpbC5jb20=