- 1Department of Biology, Avanqua-Oceanogràfic S.L, Valencia, Spain
- 2Department of Physiology, Faculty of Veterinary Science, University of Murcia, Murcia, Spain
- 3Department of Cell Biology and Histology, Faculty of Medicine, University of Murcia, Murcia, Spain
- 4Department of Veterinary Sciences, Faculty of Agrobiology, Food and Natural Resources, Czech University of Life Sciences Prague, Prague, Czechia
- 5Department of Animal Reproduction, National Agricultural and Food Research and Technology Institute (INIA), Madrid, Spain
- 6Department of Medicine and Surgery, Faculty of Veterinary Science, Madrid, Spain
- 7Research Department, Fundación Oceanogràfic, Valencia, Spain
Proteins play an important role in many reproductive functions such as sperm maturation, sperm transit in the female genital tract or sperm-oocyte interaction. However, in general, little information concerning reproductive features is available in the case of aquatic animals. The present study aims to characterize the proteome of both spermatozoa and seminal plasma of bottlenose dolphins (Tursiops truncatus) as a model organism for cetaceans. Ejaculate samples were obtained from two trained dolphins housed in an aquarium. Spermatozoa and seminal plasma were analyzed by means of proteomic analyses using an LC-MS/MS, and a list with the gene symbols corresponding to each protein was submitted to the DAVID database. Of the 419 proteins identified in spermatozoa and 303 in seminal plasma, 111 proteins were shared by both. Furthermore, 70 proteins were identified as involved in reproductive processes, 39 in spermatozoa, and 31 in seminal plasma. The five most abundant proteins were also identified in these samples: AKAP3, ODF2, TUBB, GSTM3, ROPN1 for spermatozoa and CST11, LTF, ALB, HSP90B1, PIGR for seminal plasma. In conclusion, this study provides the first characterization of the proteome in cetacean sperm and seminal plasma, opening the way to future research into new biomarkers, the analysis of conservation capacity or possible additional applications in the field of assisted reproductive technologies.
Introduction
Cetaceans, aquatic mammals from the Cetacea infraorder, are considered essential for marine ecosystems (Bowen, 1997; Uhen, 2007). Their populations are under enormous anthropogenic pressure, especially as a result of commercial overfishing, incidental captures in fisheries, and habitat degradation (Reeves et al., 2003; Parsons et al., 2014; Dolman and Brakes, 2018). Indeed, many cetacean species are threatened or in danger of extinction (Davidson et al., 2012). This is why cetaceans are protected under many national, regional and international legislations including Appendix II of the Convention on International Trade in Endangered Species of Wild Fauna and Flora (CITES), which limits the movement of animals or their products between countries. However, study of their populations in the wild involves many limitations (Bowen, 1997; O’Brien and Robeck, 2010) and, in most cases, it is not possible to keep individuals in captivity due to their size, their gregarious population structure or other peculiarities of their ethology that make their ex situ maintenance highly difficult or almost impossible. Only some species, such as Tursiops truncatus, the common bottlenose dolphin, have a docile character and the ability to adapt well to captivity, which makes them ideal model organisms (Barratclough et al., 2019). Moreover, important information can be obtained from ex situ populations in order to deepen our knowledge of their features for conservation programs, but also to extrapolate the obtained knowledge to address problems related with other cetacean species (Barratclough et al., 2019).
During recent decades, aquariums and animal conservation centers have actively contributed to the conservation and reproduction of bottlenose dolphins through joint breeding programs (Katsumata, 2010). Initially, the movement and exchange of animals for reproductive purposes between centers was necessary, but this activity entailed certain risks that could affect the integrity of the animals (O’Brien and Robeck, 2010). Subsequently, the progress made in assisted reproductive technologies (ARTs) allowed the collection and management of gametes, thus ensuring their quality to maximize fertilization (Beirão et al., 2019). In order to minimize any impact on animal welfare, voluntary semen collection techniques through animal training have been routinely established in dolphin populations under human care (Robeck and O’Brien, 2004; Robeck et al., 2005). The collection of semen facilitates research activity and the exchange of reproductive cells between geographically distant centers for further use in artificial insemination programs (Robeck et al., 2005). For that purpose, methods based on refrigeration (Takenaka et al., 2013; Ruiz-Díaz et al., 2020) and freezing (Robeck and O’Brien, 2004; Robeck et al., 2013; Sánchez-Calabuig et al., 2015b, 2017) have been developed for dolphins, including techniques for preservation of seminal samples that have allowed the creation of a gene bank of high biological value for use in the future (Holt and Pickard, 1999).
A knowledge of the semen characteristics of a species of interest is essential to gain new insights, either to widen fundamental basic studies or for further application in ARTs. Semen is composed of the cellular part, the spermatozoa, and the liquid part, the seminal plasma. Bottlenose dolphin spermatozoa are known to have a long tail, a short but hydrodynamic head shape and a bulky midpiece (van der Horst et al., 2018), these high quality features being kept after collection (van der Horst et al., 2018; Ruiz-Díaz et al., 2020). Seminal plasma is a fluid coming mostly from the prostate, the only sexual accessory gland described in cetaceans (Harrison et al., 1969; Rommel et al., 2007; Suárez-Santana et al., 2020). The complex composition of seminal plasma plays a key role in both the male and female reproductive features of mammals. In the case of males, seminal plasma is involved in sperm maturation, motility, transport, capacitation or acrosome reaction (reviewed by Juyena and Stelletta, 2012). When the seminal plasma is deposited in the female genital tract, it affects the inflammatory and immune responses (reviewed by Bromfield, 2018), protecting sperm (Kawano et al., 2014; Luongo et al., 2019), and even having an impact on the offspring (Bromfield et al., 2014). Furthermore, it has been demonstrated that the proteins of reproductive fluids (including oviductal and uterine fluids, and seminal plasma) are involved in the interaction with the sperm proteome (Luongo et al., 2020; Rickard and de Graaf, 2020).
Proteomic analysis has been used to describe the sperm and seminal plasma proteome either in non-mammal species, such as fish (Ciereszko et al., 2017; Dietrich et al., 2017), and in mammals, such as murine (Baker et al., 2008; Vicens et al., 2017), porcine (Perez-Patiño et al., 2016; Recuero et al., 2019; Luongo et al., 2020), equine (Swegen et al., 2015; Guasti et al., 2020), ovine (Cardozo et al., 2006; Pini et al., 2016), caprine (Pinto et al., 2019; Zhu et al., 2020; Martínez-Fresneda et al., 2021), bovine (Peddinti et al., 2008; Byrne et al., 2012), non-human primates (Skerget et al., 2013), and human (Martínez-Heredia et al., 2006), but not yet in any cetacean species. Furthermore, such studies have permitted the identification of proteins that can act as biomarkers of fertility (Dacheux et al., 2012; Li et al., 2016; Rahman et al., 2017; Druart and de Graaf, 2018; Pérez-Patiño et al., 2018; Druart et al., 2019), their relevance for sperm preservation (Soleilhavoup et al., 2014; Parrilla et al., 2019; Peris-Frau et al., 2019; Ryu et al., 2019; Bajuk et al., 2020; De Lazari et al., 2020) or involvement in sperm functional traits (Intasqui et al., 2016; Bezerra et al., 2019; De Lazari et al., 2019). Moreover, seminal plasma proteins are influenced by the social and competitive environment (Ramm, 2014; Mead, 2018; Hopkins et al., 2019). Therefore, knowing the protein profile of semen dolphins may be especially relevant to better understand the biology of the species in light of the complex multi-male mating strategy of bottlenose dolphins.
Finally, knowing the proteome of ejaculated spermatozoa and seminal plasma may contribute to obtaining comprehensive information and to understanding functional implications for reproductive processes not only for dolphin species but for cetaceans in general. For this reason, this study aims to identify, describe, and classify for the first time, the sperm and seminal plasma proteins of dolphins and to compare them with the protein profiles in other species (bovine, canine, and human) previously described in the literature.
Materials and Methods
Ethics of Experimentation
The samples were obtained from two trained dolphin males housed at the Oceanogràfic de Valencia following the Animal Care Protocol and policies of the aquarium. The animals were conditioned through positive reinforcement to participate in many different medical behaviors, including semen collection to provide basic husbandry care. Semen samples were obtained through training following the same approach as previously published (Sánchez-Calabuig et al., 2017). Furthermore, the regulations and policies of EU legislation, Directive 2010/63/EU1, were observed.
Animals
Semen samples were obtained from two adult males, who have lived in the same dolphin group since 2003. The estimated ages of both animals were 32 years (Male 1) and 27 years (Male 2) at the moment of the study. Both males live together with a stable social group in an outdoor pool that contains salty water at a temperature of 18–26°C. Both males successfully had previously sired several offspring. Their diet is based on frozen-thawed whole fish (herring-Clupea harengus, capelin-Mallotus villosus, blue whiting-Micromesistius poutassou, mackerel-Scomber scombrus, smelt-Osmeridae, squid-Loligo sp. and European sprat-Sprattus sprattus).
Semen Collection
Two ejaculates from each male were obtained by favoring extraction in the ventrum in an above water level position. The animals always collaborated with the trainers, who, through acoustic and tactile signals, favored extraction of the penis. The stimulation, which lasted a few seconds, ended in ejaculation. Sample was collected in a sterile LLDPE (linear low-density polyethylene) plastic bag (Sánchez-Calabuig et al., 2017). After collection, the following parameters were evaluated for each semen sample (as previously described by Ruiz-Díaz et al., 2020): pH, concentration (number of sperm/ml), total (%) and progressive motility (%) (evaluated by CASA system; 25 frames per second, and at least five random fields per sample), and viability (%) (200 spermatozoa per sample) (Table 1). All the samples were collected within 1 month (the days between sample extractions were 6 and 30 for Male 1 and Male 2, respectively).
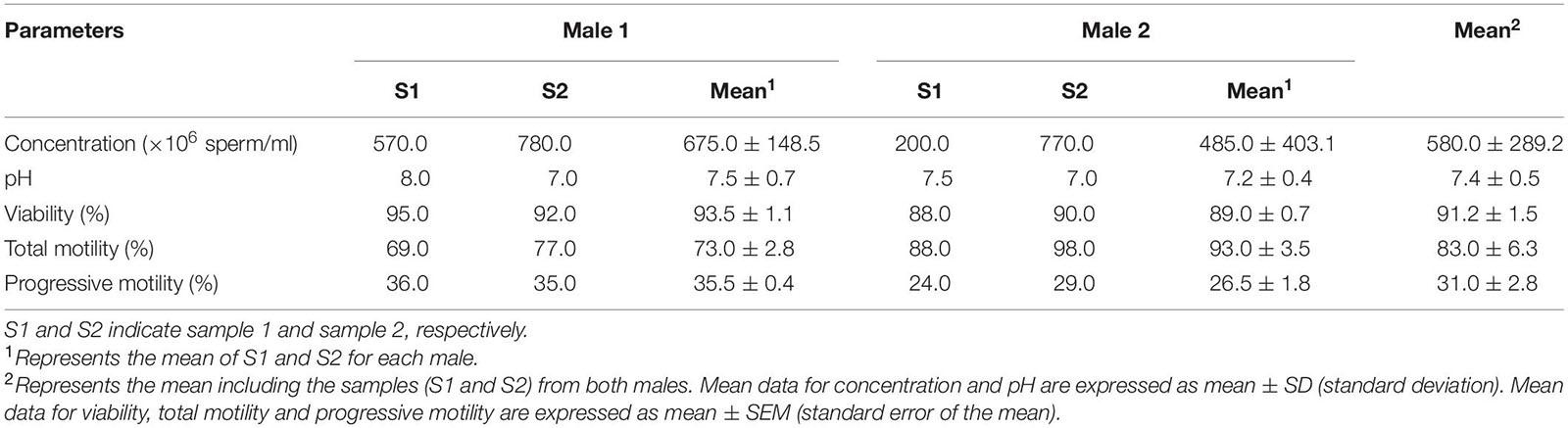
Table 1. Seminal parameters of bottlenose dolphin males (Tursiops truncatus) used for proteomic analysis.
Protein Extraction
The proteomic analysis was performed in the proteomics facility of SCSIE, University of Valencia which forms part of ProteoRed, PRB2-ISCIII, supported by grant PT13/0001. Semen samples were centrifugated at 800 × g for 5 min at 4°C to split the sample into two fractions (spermatozoa and seminal plasma). The fraction containing spermatozoa was washed twice (800 × g for 5 min, 4°C) in phosphate-buffered saline (PBS, Sigma-Aldrich®, Madrid, Spain) and the pellet was re-suspended in lysis buffer (50 mM Tris–HCl pH 8, 10 mM DTT and 2% SDS). The mixture was sonicated for 5 min, centrifuged 5 min at 15,870 × g and the resulting supernatant was transferred to a fresh microfuge tube (Figure 1). The seminal plasma was centrifuged twice (800 × g for 5 min, 4°C) to remove any remaining spermatozoa (microscopically verified).
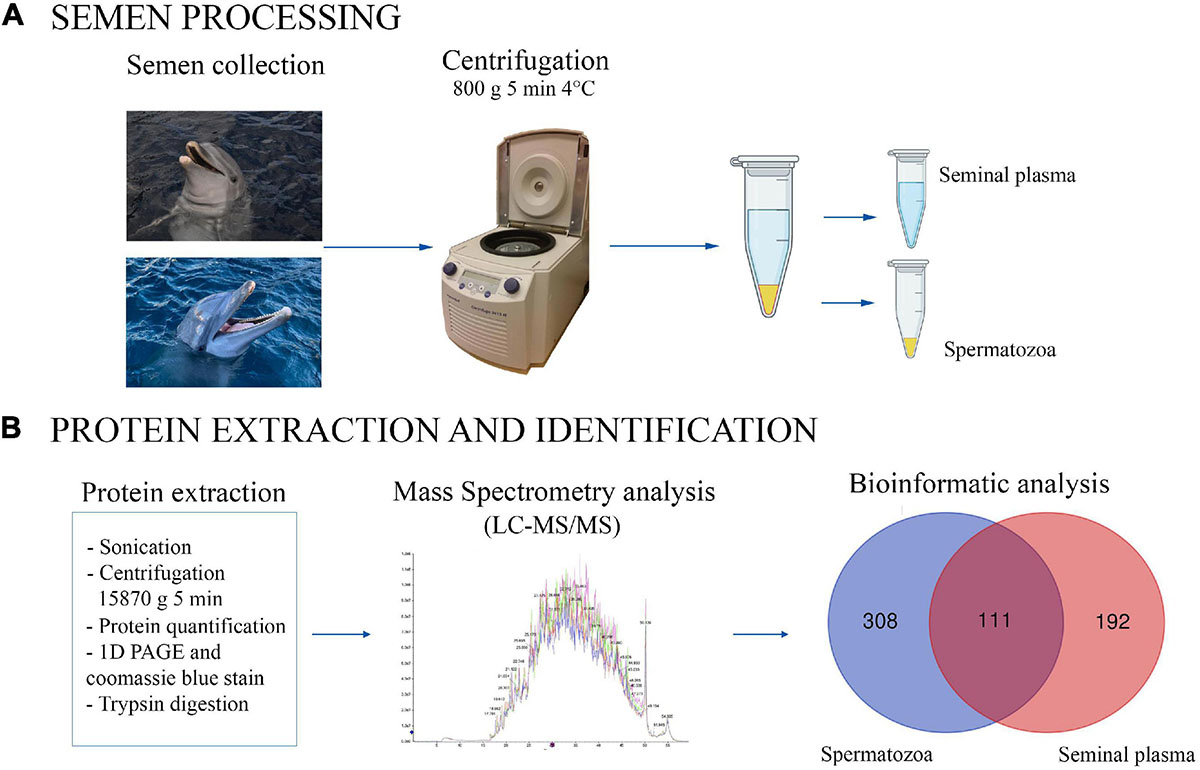
Figure 1. Graphical representation of experimental process for bottlenose dolphin semen processing (A) and protein extraction and identification (B). The Venn diagram shows the proteins found to be common (or not) to seminal plasma and spermatozoa.
The protein concentration obtained from both, spermatozoa and seminal plasma samples, was determined by Protein Quantification Assay Kit (Macherey-Nagel, Düren, Germany) according to the manufacturer’s instructions. Twenty micrograms of protein were adjusted to a 40 μl final volume with Laemmli Sample buffer (Bio-Rad, Hercules, CA, United States) with 2–5% β-mercaptoethanol.
1D PAGE
The sample was denatured at 95°C for 5 min and then loaded into a 1D PAGE. Electrophoresis was performed using a 12% precast gel (Bio-Rad, Hercules, CA, United States) at 200 V for 5 min. Then, the gel was fixed with 40% ethanol/10% acetic acid for 1 h, stained with colloidal Coomassie (Bio-Rad, Hercules, CA, United States) for 1 h and scanned by Image Scanner (GE Healthcare).
In Gel Protein Digestion
The gel slide was digested with sequencing grade trypsin (Promega, Madison, WI, United States) as described elsewhere (Shevchenko et al., 1996). Briefly, isolated proteins were digested with trypsin (500 ng) at 37°C overnight. The digestion was quenched with 10% trifluoroacetic acid (TFA) at final concentration of 1%. And, after double extraction with neat acetonitrile (ACN), the peptide mixtures were dried in a rotary evaporator and resuspended with 20 μl of 2% ACN, 0.1% TFA.
Mass Spectrometry Analysis and Protein Identification
For liquid chromatography and tandem mass spectrometry (LC–MS/MS), 3 μl of a peptide mixture sample was loaded onto a trap column (3 μm C18-CL, 350 μm × 0.5 mm; Eksigent, CA, United States) and desalted with 0.1% TFA at 5 μl/min for 5 min. The peptides were then loaded onto an analytical column (3 μm C18-CL 120 Å, 0.075 × 150 mm; Eksigent, CA, United States) equilibrated with 5% ACN and 0.1% formic acid (FA). Elution was carried out with a linear gradient of 5–40% B in A for 45 min (A: 0.1% FA; B: ACN, 0.1% FA) at a flow rate of 300 nl/min. Peptides were analyzed in a nanoESIqQTOF mass spectrometer (6600plus TripleTOF, ABSciex, MA, United States).
Samples were ionized in a Source Type: Optiflow < 1 μl Nanoapplying 3.0 kV to the spray emitter at 200°C. Analysis was carried out in data-dependent mode. Survey MS1 scans were acquired from 350 to 1,400 m/z for 250 ms. The quadrupole resolution was set to “LOW” for MS2 experiments, which were acquired at 100–1,500 m/z for 25 ms in “high sensitivity” mode. The following switch criteria were used: charge 2+ to 4+; minimum intensity, 250 counts per second (cps). Up to 100 ions were selected for fragmentation after each survey scan. Dynamic exclusion was set to 15 s. The system sensitivity was controlled by analyzing 0.5 μg of K562 trypsin digestion (Sciex). Two thousand, eight hundred and seventy proteins were identified (FDR < 1%) in these conditions in a 45 min gradient.
Data were processed with ProteinPilot v. 5.0 (AB Sciex, Framingham, MA, United States), using the default parameters to generate a peak list directly from 6600 plus TripleTofwiff files. The Paragon algorithm (Shilov et al., 2007) of ProteinPilot v. 5.0 was used to search the Uniprot Delphinidae database (v 02.2020; 3513001 proteins in database) with the following parameters: Trypsin enzyme specificity, taxonomy non-restricted, and the search effort was set to rapid.
Protein Identification
Protein Orthologs Identification
Uncharacterized proteins with no properly assigned gene symbol accounted for 15–25% of the proteins within each sample. To identify a direct ortholog for each uncharacterized protein, the proteins were filtered with a custom script written in Python (Supplementary File 1) making use of libraries Bio.Blast (Cock et al., 2009) SeqIO, StrinGIO and pandas. In essence, the algorythm performed parallel queries with the uncharacterized proteins against a database made with “makeblastdb” from a UniProt fasta database made by merging the Swissprot curated database with the T. truncatus TrEMBL dataset (release 2020_02). Candidate orthologs were then ranked from maximum to minimum sequence identity and those with a sequence identity within 5% of the maximum were selected. The results were then manually curated, reducing the uncharacterized proteins to 0.2–1% of the proteins within each sample. In some cases, it was not possible to distinguish the direct ortholog within the candidates (the case with several keratins) and thus the proteins where omitted from the biological annotation.
Biological Annotation
For the biological annotation, only the proteins identified in all the samples (seminal plasma or spermatozoa) were analyzed. A list with the gene symbols corresponding to each protein was submitted to DAVID [v6.8 (Huang et al., 2009)]. Because of the performance querying versus the T. truncatus database was very poor, the queries were made with the Homo sapiens database, as it is the best annotated organism. Unmapped IDs were checked for synonyms in GeneCard in order to avoid losing information by selecting those identifiers present in DAVID (which were not always the HGNC ones). All the Gene Ontology terms with a FDR < 0.01 were selected and classified into the three categories “biological process,” “cellular component,” and “molecular process.”
The mass spectrometry proteomics data have been deposited to the ProteomeXchange Consortium via the PRIDE (Perez-Riverol et al., 2019) partner repository with the dataset identifier PXD024588.
Results
A total of 722 different proteins in seminal plasma and spermatozoa were identified in each of the four samples analyzed): 419 proteins in spermatozoa, and 303 in seminal plasma, of which 111 proteins were common to both, spermatozoa and seminal plasma (Figure 1). The total list of identified proteins for each analyzed sample (two per male) is shown in Supplementary File 2 (sperm proteins) and Supplementary File 3 (seminal plasma proteins). Moreover, the Supplementary File 4 shows the list of the common proteins between spermatozoa and seminal plasma.
Since bovids and cetaceans are evolutionarily related (Vislobokova, 2013), belonging to the order Cetartiodactyla, a bovine dataset (Ramesha et al., 2020) was compared with dolphin. In addition, human as a biological model (Batruch et al., 2011; Baker et al., 2013; Amaral et al., 2014) and dogs (canids) (Aquino-Cortez et al., 2017; Araujo et al., 2020), because their only accessory sex gland is the prostate, as in dolphins, were also included in the comparison. The four species were therefore retrieved to evaluate the proteome profile of bottlenose dolphin spermatozoa and seminal plasma, resulting in the Venn diagram of Figure 2. The analysis revealed that 42 were identified in the spermatozoa of all four species, and 30 in the seminal plasma of the same. The number of co-present sperm proteins between animals were the following: (i) bull and dolphin, 362; (ii) man and dolphin, 375; and (iii) dog and dolphin, 58. The number of co-present seminal proteins were: (i) bull and dolphin, 218; (ii) man and dolphin, 213; and (iii) dog and dolphin, 51. These proteins are listed in Supplementary File 5 (sperm) and Supplementary File 6 (seminal plasma).
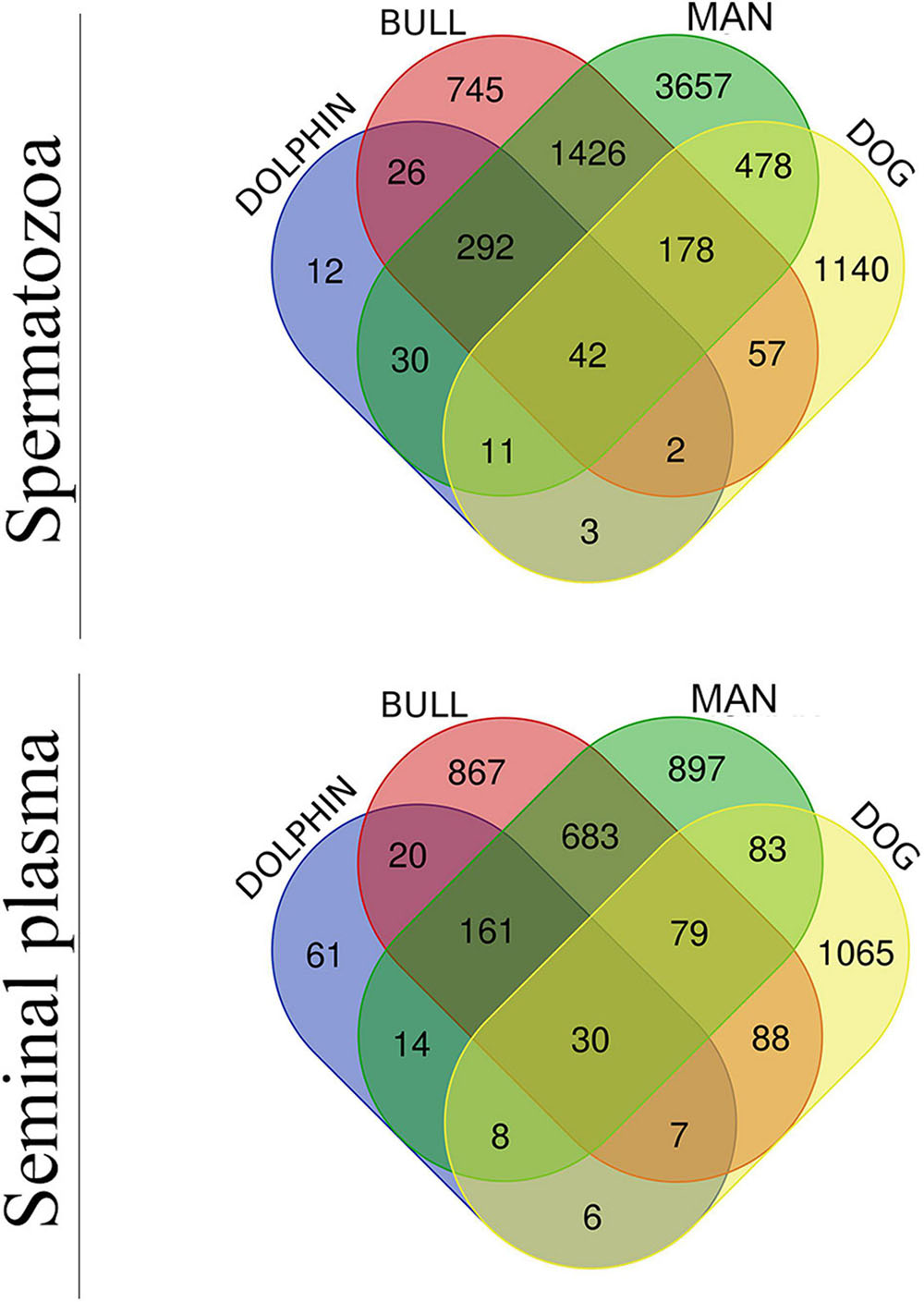
Figure 2. Venn diagram of sperm (upper diagram) and seminal plasma (lower diagram) proteomes of man, bull, dog and dolphin.
Protein Profile of Bottlenose Dolphin Spermatozoa
As already mentioned, a total of 419 proteins were present in the spermatozoa of the four samples analyzed (two from each male). A complete protein list (ordered by the number of peptides) for the spermatozoa accompanied by the protein name, String ID dolphin, peptides, mean protein score (±SD), preferred name and annotation (H. sapiens) is presented in Supplementary File 7. A detailed search was carried out in the STRING database v 11.02 to obtain a description of the functions related with each of the proteins. The following cases were observed: (i) Proteins described for T. truncatus (269 proteins, 63.9%); (ii) Proteins described for H. sapiens (416 proteins, 99.3%); and (iii) Proteins not described in either of the above (3 proteins, 0.7%). Of the proteins belonging to the third case, one is described for Mus musculus (ADAM5), one in Pan paniscus (LRRC37A5P) and the other one has no description for any species (ATP6V1FNB).
A functional annotation of the proteins from H. sapiens sperm was peformed using DAVID software and divided into different categories: “Biological processes” (BP), “Cellular components” (CC), and “Molecular functions” (MF) (Figure 3 and Supplementary File 8). Although there are different categories for each of the divisions made, the most abundant were those involved in oxidation-reduction processes (8.4%), spermatogenesis (6%), and protein folding (6%) in the case of BP (Figure 3A); extracellular exosome (41.6%), nucleus (36.3%), and mitochondrion (35.3%) in the case of CC (Figure 3B); and ATPbinding proteins (14.2%), ATPase activity (5.5%), and unfolded protein binding (5.3%) for MF (Figure 3C).
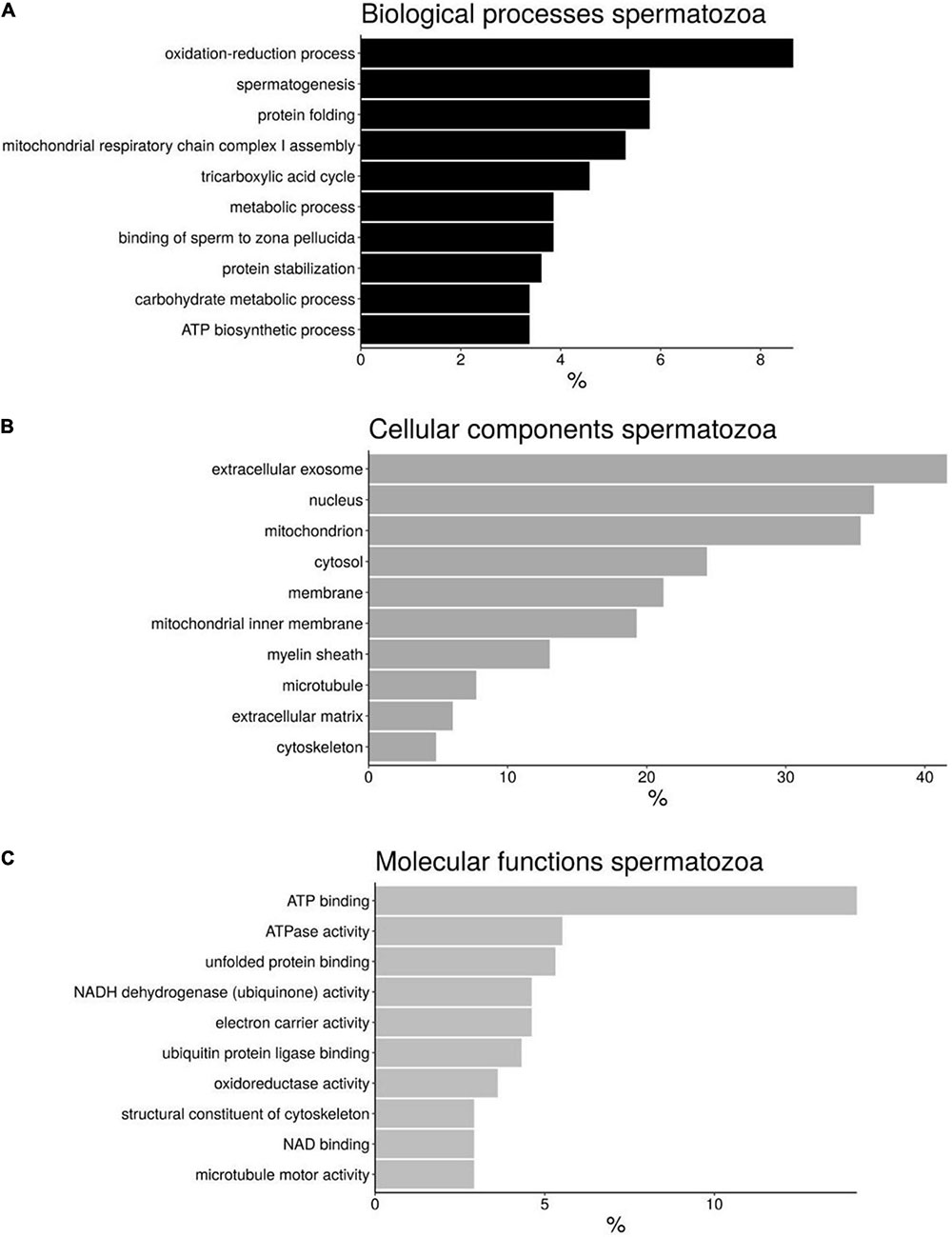
Figure 3. Bar chart representing the gene ontology annotations of protein identified in bottlenose dolphin spermatozoa according to biological processes (A), cellular components (B), and molecular functions (C).
Of the total of 419 proteins identified in the spermatozoa, 39 are linked to sperm function categories (Table 2) such as cilia/flagela (17 proteins, 43.6%), sperm motility (10 proteins, 25.6%), capacitation and acrosome reaction (4 proteins, 10.3%), sperm-egg fusion (4 proteins, 10.3%), spermatogenesis and fertilization (2 proteins, 5.1%), and other functions (2 proteins, 5.1%). Not all 39 proteins involved in sperm functions are identified and/or described in the STRING program for dolphins, so we divided them into three different categories: (1) Proteins described for T. truncatus (27 proteins, 69.2%); (2) Proteins described for H. sapiens (35 proteins, 89.7%); and (3) Proteins described for M. musculus (1 protein, 2.6%). Twenty-four proteins were common to both categories (1 and 2).
Protein Profile of Bottlenose Dolphin Seminal Plasma
A total of 303 proteins were identified in the seminal plasma of the four samples obtained, two from each male. A complete protein list (ordered by the number of peptides) for the seminal plasma with protein name, String ID dolphin, peptides, mean protein score (±SD), preferred name and annotation (H. sapiens) is presented in Supplementary File 9. A detailed search was carried out in the STRING database v 11.0 (see text foot note 2) to obtain a description of the functions related to each of the proteins, finding the following: (i) Proteins described for T. truncatus (201 proteins, 66.1%); (ii) Proteins described for H. sapiens (298 proteins, 98.0%); (iii) Proteins not described in any of above (4 proteins, 1.3%). Of the proteins belonging to the third case, all are described for M. musculus (ADAM1, ADAM5, DNAJB3 y MUC19), an animal with poliandric reproduction and sperm competition.
The proteins present in seminal plasma described in H. sapiens were distributed into 3 categories according DAVID software: “Biological process” (BP), “Cellular components” (CC), and “Molecular Function” (MF) (Figure 4 and Supplementary File 8). Although there are different categories for each of the divisions made the most abundant are those involved in protein folding (10.6%), proteolysis (8%), and cell–cell adhesion (7.6%) for BP (Figure 4A); extracellular exosome (71.1%), cytosol (36.9%), and extracellular space (29.9%) for CC (Figure 4B); and protein binding (61.5%), unfolded protein binding (8.3%) and cadherin binding involved in cell-cell adhesion (7.6%) for MF (Figure 4C).
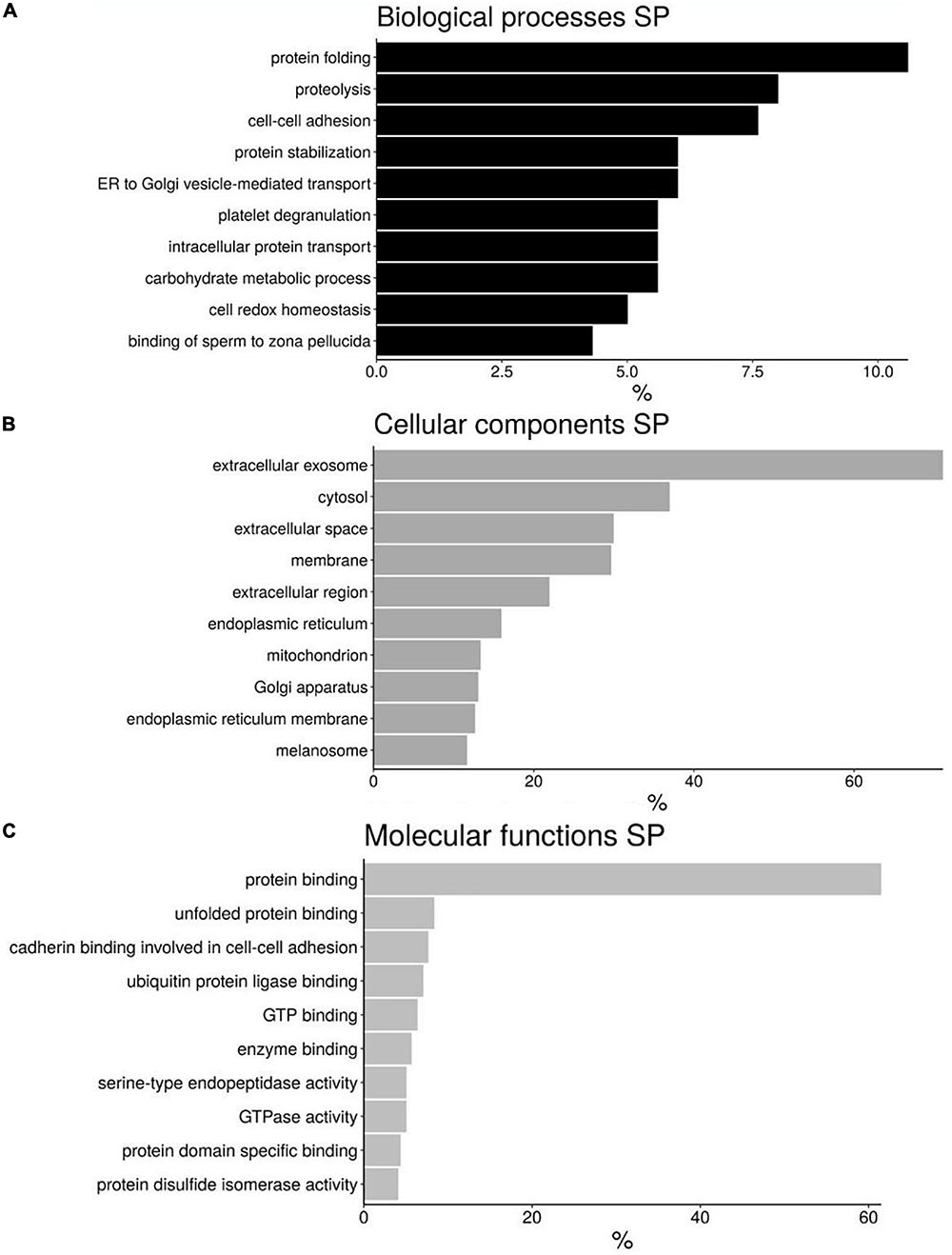
Figure 4. Bar chart representing the gene ontology annotations of protein identified in bottlenose dolphin seminal plasma (SP) according to biological processes (A), cellular components (B), and molecular functions (C).
Of the 303 proteins identified in seminal plasma, 31 are involved in reproductive processes (Table 3) such as adhesion with zona pellucida (8 proteins, 25.8%), spermatogenesis (6 proteins, 19.3%), sperm motility process (4 proteins, 12.9%), the formation of microtubules involved in flagellar development (4 proteins, 12.9%), sperm maturation/capacitation (4 proteins, 12.9%), epididymal proteins (2 proteins, 6.5%), muellerian inhibitor (1 protein, 3.2%), oocyte maturation (1 protein, 3.2%), and testis protein (1 protein, 3.2%). Not all the 31 reproductive proteins are identified and/or described in the STRING program for dolphins, so we divided them into three different categories: (1) Those described in T. truncatus (29 proteins, 93.5%); (2) Those described in H. sapiens (17 proteins, 54.8%); and (3) Those described in M. musculus (2 proteins, 6.7%). All the proteins described in H. sapiens are also present in T. truncatus.
Protein–Protein Interaction Networks
All proteins were searched using STRING software. A total of 266 nodes and 1,452 edges were identified in T. truncatus spermatozoa (Figure 5), as well as 201 nodes and 804 edges in seminal plasma (Figure 6) for the protein–protein interaction (PPI) networks. Network nodes represent proteins and the line color indicates the type of interaction evident, light blue color for known interactions from curated databases, and pink color for interactions experimentally determined. The Predicted Interactions are represented by different colors: green for gene neighborhood, red for gene fusions and dark blue for gene co-occurrence. Other relations between proteins are identified with light green for text mining, black for co-expression and blue for protein homology. Query proteins are represented as colored nodes and the second shell of interactors are represented as white nodes. Empty nodes represent proteins of unknown 3D structure and filled nodes represent predicted or known structures.
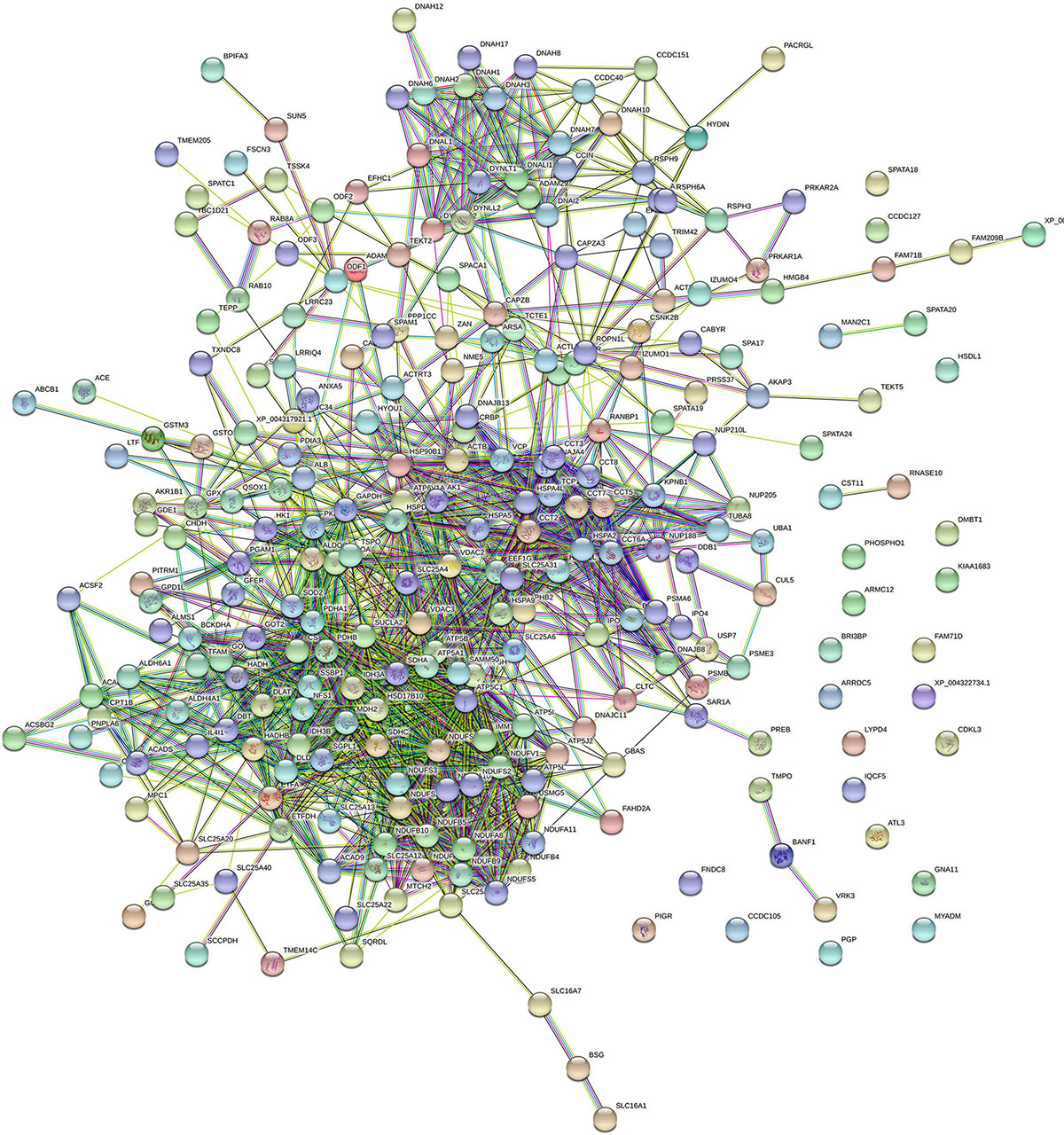
Figure 5. STRING protein–protein interaction network showing the interactions of the spermatozoa proteins identified in bottlenose dolphin.
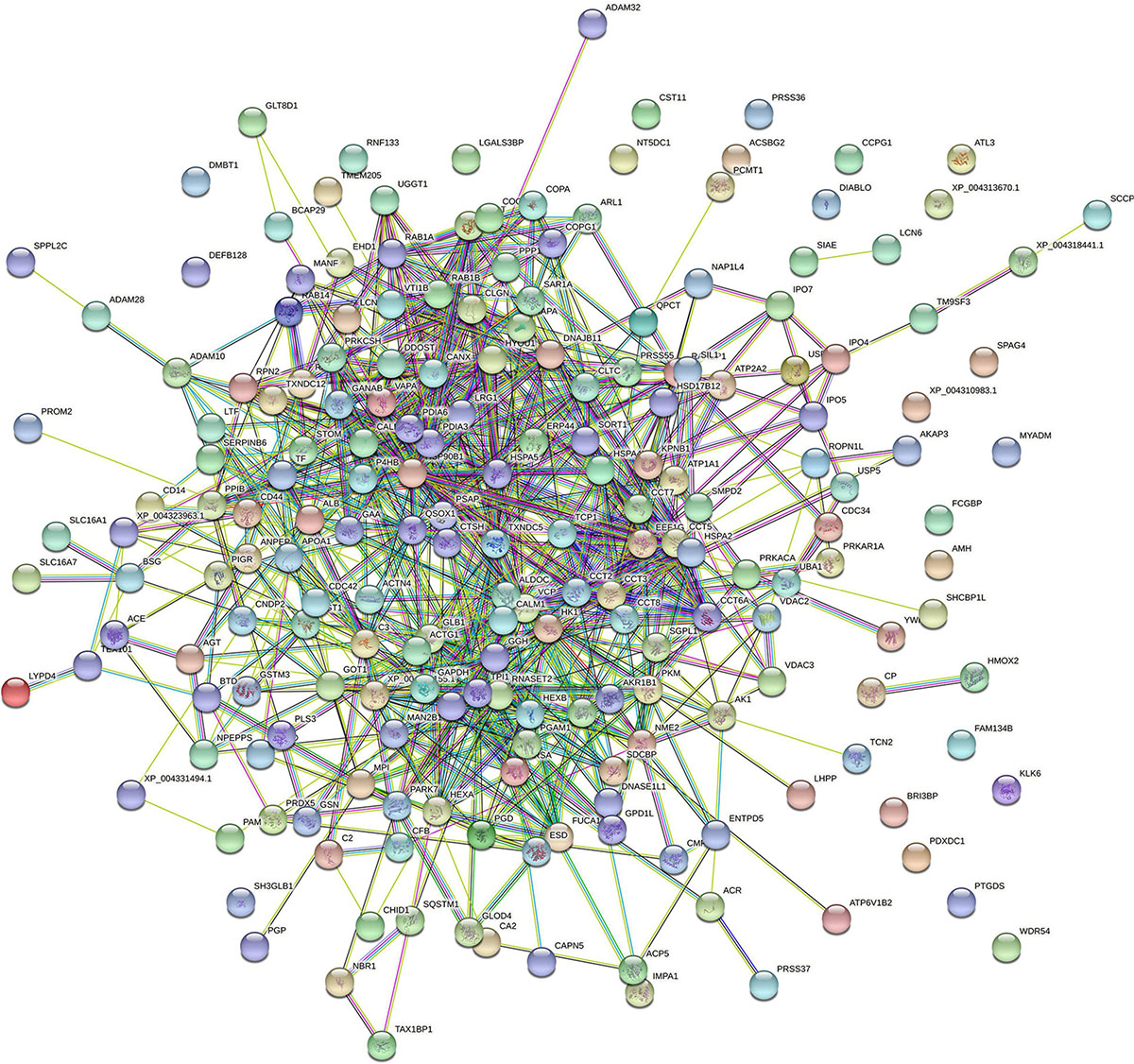
Figure 6. STRING protein–protein interaction network showing the interactions of the seminal plasma proteins identified in bottlenose dolphin.
Discussion
Dolphins are one of the most studied marine mammals, mainly due to their easy maintenance in ex situ conditions compared to other cetaceans. Although dolphins can reproduce in captivity, the main problem is the inbreeding that occurs in small groups of animals. For this reason, their reproductive characteristics and subsequent improvements in ARTs are essential in these animals to ensure genetic spread and conservation. In this respect, studies of the semen protein composition has determined the possibility of using sperm freezing in different species (Soleilhavoup et al., 2014; Kasimanickam et al., 2019; Gaitskell-Phillips et al., 2021; Martínez-Fresneda et al., 2021), the identification of presumptive fertility biomarkers (Dacheux et al., 2012; Li et al., 2016; Rahman et al., 2017; Druart and de Graaf, 2018; Pérez-Patiño et al., 2018; Druart et al., 2019) or sperm traits (Intasqui et al., 2016; Bezerra et al., 2019; De Lazari et al., 2019). The present study describes the proteins in spermatozoa and seminal plasma of bottlenose dolphin. To the best of our knowledge, this study represents the first description of the semen proteome in any cetacean. A total of 722 proteins (common to the 4 samples analyzed) were described for sperm (419) and seminal plasma (303).
Proteins Identified in the Spermatozoa of Bottlenose Dolphin
The five most abundant proteins found in dolphin spermatozoa (based on the number of peptides identified) were the following: AKAP3, ODF2, TUBB, GSTM3, and ROPN1. Four of these proteins (AKAP3, ODF2, TUBB, and GSTM3) are also in the top five of highly abundant proteins in the spermatozoa of buffalo (Fu et al., 2019). AKAP3 protein was the most abundant sperm protein according to our data. This protein is not only essential for the formation of the subcellular structure of the sperm flagellum, sperm motility and male fertility in mice, but also for sperm capacitation. As is known, sperm capacitation is a series of biochemical and physiological changes that mammalian sperm must undergo to become fertile (Gervasi and Visconti, 2016). AKAP3 null mice sperm has low sperm motility, leading to sperm morphology abnormalities, the displacement of PKA subunits and misregulated PKA activity, a key factor during sperm capacitation, and in male sterility (Xu et al., 2020). Moreover, other proteins identified in our study, such as CABYR and ROPN1, interact with AKAP3 (Carr et al., 2001; Li et al., 2011). CABYR protein is a fibrous sheath calcium-binding tyrosine phosphorylation-regulated protein (Li et al., 2007), which associates with AKAP3 in human spermatozoa (Li et al., 2011). CABYR is located in the principal piece of the sperm flagellum (Li et al., 2007; Zhang et al., 2016) and it is involved in calcium-binding when phosphorylated during capacitation (Naaby-Hansen et al., 2002). Moreover, this protein is expressed in the oviduct of several species (Hanlon Newell et al., 2008; Martinez et al., 2020), where its expression increases in response to sperm entry. ODF2 is the second most abundant protein found in the spermatozoa of bottlenose dolphin. In mammalian sperm, the flagellum presents complex accessory structures surrounding the central axoneme, the outer dense fibers (ODFs) being part of these structures (Zhao et al., 2018). Dolphin sperm is not an exception, and ODFs are almost of similar form and size as in most other mammals (van der Horst et al., 2018). ODFs play a role in the protection of the sperm tail against shear forces during epididymal transport and ejaculation (Baltz et al., 1990). More than 14 polypeptides from mammalian ODFs have been identified, including four major proteins (ODF1, ODF2, ODF3, and ODF4) (reviewed by Zhao et al., 2018). Our analysis identified ODF1, ODF2, and ODF3 in dolphin sperm, the most abundant being ODF2 protein. The disruption of ODF2 expression in mice reduced sperm motility, and is compatible with asthenozoospermia characteristics (Zhao et al., 2018). Moreover, ODF2 is indispensable for the neck midpiece connection, which is composed of a centrosome-derived component and a flagellar component (Ito et al., 2019). Tubulins are a family of proteins in which α- and β-tubulin are the major components of microtubules in spermatozoa (Kierszenbaum, 2002). TUBB and TUBB4B proteins are two of the tubulin proteins previously be found in the sperm of our analyzed dolphin samples. These proteins are important in the development of cilia and flagellum, but there is nothing in the bibliography which explains in detail the relation between this fact and the presence/absence of this group as fertility biomarkers, although TUBB protein exhibits clear differences in expression according to porcine litter size (Kwon et al., 2015b). GSTM3 is another protein situated among the five most abundant sperm proteins identified in our study. GSTM3 is a detoxification protein, which has been reported to play a key role in oocyte binding (Gopalakrishnan et al., 1998; Petit et al., 2013) and their interaction with the zona pellucida (Petit et al., 2013). Moreover, sperm GSTM3 has been proposed as a quality (Llavanera et al., 2020b), fertility (Kwon et al., 2015a) and cryotolerance (Llavanera et al., 2019) biomarker for pig sperm. Our data also report the presence of this protein in the seminal plasma of dolphins. Recently, it has been demonstrated that low concentration of GSTM3 in pig seminal plasma is related to an increased percentage of sperm abnormalities (Llavanera et al., 2020a). ROPN1 protein is found in sperm flagellum as part of the fibrous sheath, specifically located in the principal piece and end piece of the flagellum (Fujita et al., 2000; Chen et al., 2011). The expression level of ROPN1 was found to be significantly lower in asthenozoospermic men than in normozoospermic suggesting its involvement in sperm motility (Chen et al., 2011). When mice lacking ROPN1 were analyzed, the sperm exhibited altered motility, the same males being subfertile, and producting fewer and smaller litters (Fiedler et al., 2012). Indeed, a positive correlation between motility (progressive and total motile sperm number) and ROPN1/CABYR gene expression has been observed (Pelloni et al., 2018).
One interesting sperm protein identified in our data was IZUMO1. Sperm-egg fusion is accomplished through the interaction of a specific membrane proteins in sperm, IZUMO1 (Inoue et al., 2005), and oocyte, JUNO (Bianchi et al., 2014). During acrosome reaction, IZUMO1 relocates from the anterior head of the sperm to the site where fusion takes place and which was the first site to be shown as critical for gamete fusion (Inoue et al., 2005, 2011). Thus, our data suggest that gamete fusion is probably mediated by the same mechanism as that described in mouse.
Proteins Identified in the Seminal Plasma of Bottlenose Dolphin
As mentioned above, the seminal plasma of dolphins comes from the testis, epididymis and the prostate, the only accessory sex gland in this species (Harrison et al., 1969; Rommel et al., 2007; Suárez-Santana et al., 2020). Seminal plasma is not simply a transport fluid for sperm but also modulates the female genital tract environment (Bromfield, 2014). It also protects sperm during their journey toward the oocyte (Kawano et al., 2014; Luongo et al., 2019) and interacts with other reproductive fluids modifying the sperm proteome (Luongo et al., 2020). The five most abundant proteins (based on the number of peptides identified) represented in seminal plasma of dolphin were CST11, LTF, ALB, HSP90B1, and PIGR. Of these CST11 (Cystatin11) was seen to be the most abundant protein in the seminal plama of dolphin (and was also detected in the sperm). CST11 belongs to the cystatin type 2 family of cysteine protease inhibitor and exhibits antimicrobial activity in vitro (Magister and Kos, 2013), suggesting that cystatins defend the male reproductive tract against invading pathogens (Hamil et al., 2002; Fan et al., 2014). This protein has been detected throughout the epididymis (particularly in the initial segment) and in ejaculated human sperm (Hamil et al., 2002). LTF is a glycoprotein with antioxidant and antibacterial activities that is synthesized in the epididymis in mammals (Pearl and Roser, 2008) and has been detected in the prostate and seminal vesicles in humans (Wichmann et al., 1989). It has been reported that the treatment of asthenoteratozoospermic males with LTF, combined with other natural antioxidants, significantly improves the motility of sperm cells (Piomboni et al., 2008). Moreover, a positive correlation between seminal plasma LFT concentration and sperm density was established in dogs (Kikuchi et al., 2003a) and horses (Kikuchi et al., 2003b). The addition of LTF to freezing sperm extender protected stallion sperm, increasing the percentage of sperm with functional membranes and decreased lipid oxidizing agents (Martins et al., 2018). ALB protein is positively related with sperm concentration, total sperm count and the percentage of morphologically normal spermatozoa, but negatively related with semen volume in humans (Elzanaty et al., 2007). In a study developed in Holstein bulls, high concentrations of this protein correlated with animals whose semen was classified as highly fertile (Kasimanickam et al., 2019), so this protein could be used as a biomarker of fertility. HSP90B1 is a molecular chaperone member of the heat shock protein 90 (HSP90) family. This protein is involved in the immune response (Graustein et al., 2018) in the suppression of cell apoptosis and autophagy (Sun et al., 2015). This function suggests a putative role for HSP90B1 once it is in the female genital tract after insemination, since it is known that seminal plasma modulates maternal immunity (Bromfield, 2018). PIGR is involved in the tissue homeostasis pathway (Panner Selvam et al., 2019) and its expression is regulated by cytokines, which show high levels in the seminal plasma of varicocele patients due to an inflammatory response (Zeinali et al., 2017). The presence of PIGR was previously reported in the semen of bulls (Rego et al., 2014) and cats (Mogielnicka-Brzozowska et al., 2020). In humans, PIGR expression was observed in the prostate (Sirigu et al., 1995) which agrees with our results as the prostate is the only accessory sex gland in dolphins.
NPC2 is one of the proteins found in abundance in the seminal plasma of bottlenose dolphin (the sixth most abundant protein). NPC2 has also been described to be among the most abundant secreted proteins in the epididymis in bovine (Belleannée et al., 2011). In the epididymal fluid, NPC2 participates in cholesterol efflux from the spermatozoa during epididymal sperm maturation (Légaré et al., 2006). Furthermore, capacitated NPC2(–/–) mice spermatozoa exhibited defective tyrosine phosphorylation patterns and a reduced ability to fertilize cumulus-oocyte complexes compared with wild-type spermatozoa, supporting the relevance of epididymal NPC2 in male mouse fertility (Busso et al., 2014). Recently, relative levels of two isoforms of NPC2 were found to be higher in the porcine seminal plasma of poor freezability ejaculates than in that with good freezability, suggesting that the NPC2 content may be useful for predict ejaculate freezability (Valencia et al., 2020).
Proteome Profile of Dolphin Spermatozoa and Seminal Plasma Compared to Other Species (Human, Bovine, and Canine)
Comparative analyses and a Venn diagram were made relating dolphin to other three species: bovine, canine and human. Bovine and porcine species are even-toed ungulates (artiodactyls), and closest living relatives of dolphin (Thewissen et al., 2007; Spaulding et al., 2009). Actually, both species (bovine and delphinus) share 362 of the 419 sperm proteins detected in the analysis (86.4%) and 218 of the 304 detected in seminal plasma protein (71.7%), appointing to the high number of conserved proteins between these animals. Likewise, dolphin sperm are able to attach and penetrate bovine ZP, even triggering the blockage of polyspermy (Sánchez-Calabuig et al., 2015a, 2017). The dog was also compared to dolphin because they both share the fact that of having only one accessory sex gland, the prostate. Dog and dolphin share 51 seminal plasma proteins, only 6 of which are exclusive to these animals (NBR1, BCAP29, KLK6, SQSTM1, SLC16A7, and MUC19). This suggests that, although prostate is the only accessory sex gland common to dogs and dolphins, the protein composition is not well conserved between species, and less than 25% of the seminal plasma proteins are shared. Moreover, as an outgroup, we also included human in the comparative analysis because of the availability proteomic data. The results indicated that 42 sperm proteins and 30 seminal plasma proteins are common to the four species.
FSIP2 is one of the sperm proteins identified as common to all four compared species (Supplementary File 5) (sixth position in the sperm protein list based on the number of identified peptides; Supplementary File 7). FISP2 is one of the main genes involved in the multiple morphological abnormalities of sperm flagella syndrome (Martinez et al., 2018). Moreover, mutations in FSIP2 lead to the absence of A-kinase anchoring protein 4 (AKAP4), a protein also detected in dolphin sperm. DNAH8 has also been found also to be common to the four species analyzed (Supplementary File 5) (tenth position in sperm protein list based on the number of identified peptides; Supplementary File 7). Loss-of-function mutation in DNAH8 is suggested to cause male infertility because of the multiple morphological abnormalities of sperm flagella syndrome, DNAH8 being essential for sperm flagellum formation (Yang et al., 2020). There are 12 sperm proteins that have only been identified in dolphin: LRRC37A5P, CFAP61, HOGA1, CCDC127, UBB, C6orf58, CST11, DEFB130, HEL-S-80P, ADAM10, ATP6V1FNB, and GCAT.
In the case of seminal plasma, LTF and ALB are present in all the compared species. ENO1, which has been recognized as a candidate for fertility marker in bulls (Park et al., 2019) is another protein conserved in the four species and a good freezability predictor in human (Jiang et al., 2015). It would be interesting to analyze ENO1 in dolphins with different degrees of fertility and as a possible component of an extender during preservation. A total of 61 proteins were exclusively detected in the sperm of dolphin but not in dogs, humans or bulls.
To evaluate whether the most abundant protein found in dolphin are also in the semen of other species (human and bovine) the most abundant protein situated in the first decile of the lists for sperm and seminal plasma of these species were analyzed. In the case of sperm proteins, 16 of them were shared across the three species (DNAH8, ODF2, NDUFS1, HSPA2, TUBB4B, HK1, TEKT5, DLAT, AKAP3, TEKT2, EFHC1, LTF, GSTM3, SDHA, TEKT3, and UQCRC2). Four of them are included in the top ten of the most abundant proteins detected in dolphin sperm (DNAH8, ODF2, AKAP3, and GSTM3). In the case of seminal plasma, 13 proteins were shared between the three species (ACE, QSOX1, PDIA3, P4HB, HYOU1, HSPA5, ALB, GLB1, PIGR, LTF, HSP90B1, HSPA8, and HSP90AA1). Seven of them are included in the top ten of the most abundant proteins detected in the seminal plasma of dolphin (ACE, PDIA3, ALB, PIGR, LTF, HSP90B1, HSPA8, and HSP90AA1).
Protein differences between the species could be a result of different requirements for spermatozoa to interact with the female tract (Druart and de Graaf, 2018). The species here compared (human, bovine, canine, and dolphin) have vaginal semen deposition which can explain a similar protein profile between species for helping with cervical migration. Moreover, cross-species comparison of mammalian seminal plasma proteomes performed in seven species (bovine, sheep, goat, pig, horse, camel, and alpaca) revealed that the phylogenetic proximity between species could be related to the similarity of seminal plasma proteome (Druart et al., 2013).
Conclusion
In conclusion, this study provides an inventory of 722 proteins present in bottlenose dolphin semen. As demonstrated in most mammalian species, bottlenose dolphin semen proteome includes proteins that are essential to the sperm structure and function. Comparison of this proteome with other physio-pathologies enable the identification of semen markers for reproduction purposes. Moreover, this work opens the door to future research aimed at investigating a new molecular basis of sperm, proteins that are involved in sperm conservation, or those that can be used as biomarkers of sperm quality and fertility.
Data Availability Statement
The mass spectrometry proteomics data have been deposited to the ProteomeXchange Consortium via the PRIDE partner repository with the dataset identifier PXD024588.
Ethics Statement
The samples were obtained from two trained dolphin males housed at the Oceanogr fic de Valencia following the Animal Care Protocol and policies of the aquarium. The animals were conditioned through positive reinforcement to participate in many different medical behaviors, including semen collection to provide basic husbandry care. Semen samples were obtained through training following the same approach as previously published (Sánchez-Calabuig et al., 2017). Furthermore, the regulations and policies of EU 143 legislation, Directive 2010/63/EU (https://eur-lex.europa.eu/legal144 content/EN/TXT/?uri=CELEX:32010L0063), were observed.
Author Contributions
MI and FG-V: conceptualization. M-CF-A, LG-B, PC, CL, SA-S, JR-S, EP, SR-D, CB-G, and DG-P: methodology. FG-V: validation and supervision. LG-B: formal analysis and data curation. M-CF-A, LG-B, CL, SA-S, JR-S, EP, SR-D, CB-G, M-JS-C, DG-P, MA, MI, and FG-V: investigation. M-CF-A, CB-G, and DG-P: resources. M-CF-A and FG-V: writing – original draft preparation. M-CF-A, LG-B, PC, CL, SA-S, JR-S, EP, SR-D, CB-G, M-JS-C, DG-P, MA, MI, and FG-V: review and editing. DG-P, MA, and FG-V: funding acquisition. All authors have read and agreed to the published version of the manuscript.
Funding
This research was supported by the Spanish Ministry of Science and Innovation (PID2019-106380RB-I00/AEI/10.1303/501100011033) and PGC2018-094781-B-I00 (MCINN/AEI/FEDER, UE), and Oceanogràfic Foundation.
Conflict of Interest
The authors declare that the research was conducted in the absence of any commercial or financial relationships that could be construed as a potential conflict of interest.
Acknowledgments
We are grateful to the aquarium Oceanogràfic Valencia staff, dolphin trainers and veterinarians for their assistance and commitment with animal care and for facilitating semen sample collections. We also thank M. Luz Valero of the Proteomic Service of the University of Valencia for her useful help and advice.
Supplementary Material
The Supplementary Material for this article can be found online at: https://www.frontiersin.org/articles/10.3389/fcell.2021.673961/full#supplementary-material
Supplementary File 1 | Python code used to identify the most similar orthologs for dolphin proteins without an official name. The code performs blast queries against a database composed of curated entries from Swissprot plus the Uniprot entries associated with T. truncatus (release 2020_02). Candidates for each query are selected within the top 5 matches with an official name, e-value of 0.001 and sequence identity of 60%. If the top match has over 99% identity its name is assigned to the problem protein of dolphin. Otherwise, all candidates within a margin of 5% identity are selected and their names compared. If there is no consensus for the name, the program writes their names and sequence identity and the alignment results are manually checked. If the program does not find any proteins matching the requisites a message is returned: “Check this protein, there are no near orthologs.”
Supplementary File 2 | Individual lists of proteins identified in each of the sperm samples analyzed in bottlenose dolphin (Tursiops truncatus).
Supplementary File 3 | Individual lists of proteins identified in each of the seminal plasma samples analyzed in bottlenose dolphin (Tursiops truncatus).
Supplementary File 4 | List of common proteins to spermatozoa and seminal plasma in bottlenose dolphin (Tursiops truncatus).
Supplementary File 5 | List of sperm proteins common (or not) to bull, man, dog, and bottlenose dolphin.
Supplementary File 6 | List seminal plasma proteins common (or not) to bull, man, dog, and bottlenose dolphin.
Supplementary File 7 | Proteins identified in the sperm (common to all of the analyzed samples) of bottlenose dolphin (Tursiops truncatus).
Supplementary File 8 | Functional distribution of bottlenose dolphin (Tursiops truncatus) spermatozoa and seminal plasma from DAVID Bioinformatics Resources.
Supplementary File 9 | Proteins identified in the seminal plasma (common to all of the analyzed samples) of bottlenose dolphin (Tursiops truncatus).
Footnotes
References
Amaral, A., Castillo, J., Ramalho-Santos, J., and Oliva, R. (2014). The combined human sperm proteome: cellular pathways and implications for basic and clinical science. Hum. Reprod. Update 20, 40–62. doi: 10.1093/humupd/dmt046
Aquino-Cortez, A., Pinheiro, B. Q., Lima, D. B. C., Silva, H. V. R., Mota-Filho, A. C., Martins, J. A. M., et al. (2017). Proteomic characterization of canine seminal plasma. Theriogenology 95, 178–186. doi: 10.1016/j.theriogenology.2017.03.016
Araujo, M. S., de Oliveira Henriques, Paulo, O. L., Paranzini, C. S., Scott, C., Codognoto, V. M., et al. (2020). Proteomic data of seminal plasma and spermatozoa of four purebred dogs. Data Br. 30:105498. doi: 10.1016/j.dib.2020.105498
Bajuk, B. P., Zrimšek, P., Pipan, M. Z., Tilocca, B., Soggiu, A., Bonizzi, L., et al. (2020). Proteomic analysis of fresh and liquid-stored boar spermatozoa. Animals 10:553. doi: 10.3390/ani10040553
Baker, M. A., Hetherington, L., Reeves, G. M., and Aitken, R. J. (2008). The mouse sperm proteome characterized via IPG strip prefractionation and LC-MS/MS identification. Proteomics 8, 1720–1730. doi: 10.1002/pmic.200701020
Baker, M. A., Naumovski, N., Hetherington, L., Weinberg, A., Velkov, T., and Aitken, R. J. (2013). Head and flagella subcompartmental proteomic analysis of human spermatozoa. Proteomics 13, 61–74. doi: 10.1002/pmic.201200350
Baltz, J. M., Williams, P. O., and Cone, R. A. (1990). Dense fibers protect mammalian sperm against damage. Biol. Reprod. 43, 485–491. doi: 10.1095/biolreprod43.3.485
Barratclough, A., Wells, R. S., Schwacke, L. H., Rowles, T. K., Gomez, F. M., Fauquier, D. A., et al. (2019). Health assessments of common bottlenose dolphins (Tursiops truncatus): past, present, and potential conservation applications. Front. Vet. Sci. 6:444. doi: 10.3389/fvets.2019.00444
Batruch, I., Lecker, I., Kagedan, D., Smith, C. R., Mullen, B. J., Grober, E., et al. (2011). Proteomic analysis of seminal plasma from normal volunteers and post-vasectomy patients identifies over 2000 proteins and candidate biomarkers of the urogenital system. J. Proteome Res. 10, 941–953. doi: 10.1021/pr100745u
Beirão, J., Boulais, M., Gallego, V., O’Brien, J. K., Peixoto, S., Robeck, T. R., et al. (2019). Sperm handling in aquatic animals for artificial reproduction. Theriogenology 133, 161–178. doi: 10.1016/j.theriogenology.2019.05.004
Belleannée, C., Labas, V., Teixeira-Gomes, A. P., Gatti, J. L., Dacheux, J. L., and Dacheux, F. (2011). Identification of luminal and secreted proteins in bull epididymis. J. Proteomics 74, 59–78. doi: 10.1016/j.jprot.2010.07.013
Bezerra, M. J. B., Arruda-Alencar, J. M., Martins, J. A. M., Viana, A. G. A., Viana Neto, A. M., Rêgo, J. P. A., et al. (2019). Major seminal plasma proteome of rabbits and associations with sperm quality. Theriogenology 128, 156–166. doi: 10.1016/j.theriogenology.2019.01.013
Bianchi, E., Doe, B., Goulding, D., and Wright, G. J. (2014). Juno is the egg Izumo receptor and is essential for mammalian fertilization. Nature 508, 483–487. doi: 10.1038/nature13203
Bowen, W. D. (1997). Role of marine mammals in aquatic ecosystems. Mar. Ecol. Prog. Ser. 158, 267–274. doi: 10.3354/meps158267
Bromfield, J. J. (2014). Seminal fluid and reproduction: much more than previously thought. J. Assist. Reprod. Genet. 31, 627–636. doi: 10.1007/s10815-014-0243-y
Bromfield, J. J. (2018). Review: the potential of seminal fluid mediated paternal-maternal communication to optimise pregnancy success. Animal 12, s104–s109. doi: 10.1017/S1751731118000083
Bromfield, J. J., Schjenken, J. E., Chin, P. Y., Care, A. S., Jasper, M. J., and Robertson, S. A. (2014). Maternal tract factors contribute to paternal seminal fluid impact on metabolic phenotype in offspring. Proc. Natl. Acad. Sci. U S A. 111, 2200–2205. doi: 10.1073/pnas.1305609111
Busso, D., Oñate-Alvarado, M. J., Balboa, E., Castro, J., Lizama, C., Morales, G., et al. (2014). Spermatozoa from mice deficient in Niemann-Pick disease type C2 (NPC2) protein have defective cholesterol content and reduced in vitro fertilising ability. Reprod. Fertil. Dev. 26, 609–621. doi: 10.1071/RD12059
Byrne, K., Leahy, T., Mcculloch, R., Colgrave, M. L., and Holland, M. K. (2012). Comprehensive mapping of the bull sperm surface proteome. Proteomics 12, 3559–3579. doi: 10.1002/pmic.201200133
Cardozo, J. A., Fernández-Juan, M., Forcada, F., Abecia, A., Muiño-Blanco, T., and Cebrián-Pérez, J. A. (2006). Monthly variations in ovine seminal plasma proteins analyzed by two-dimensional polyacrylamide gel electrophoresis. Theriogenology 66, 841–850. doi: 10.1016/j.theriogenology.2006.01.058
Carr, D. W., Fujita, A., Stentz, C. L., Liberty, G. A., Olson, G. E., and Narumiya, S. (2001). Identification of sperm-specific proteins that interact with a-kinase anchoring proteins in a manner similar to the Type II regulatory subunit of PKA. J. Biol. Chem. 276, 17332–17338. doi: 10.1074/jbc.M011252200
Chen, J., Wang, Y., Wei, B., Lai, Y., Yan, Q., Gui, Y., et al. (2011). Functional expression of ropporin in human testis and ejaculated spermatozoa. J. Androl. 32, 26–32. doi: 10.2164/jandrol.109.009662
Ciereszko, A., Dietrich, M. A., and Nynca, J. (2017). Fish semen proteomics — new opportunities in fish reproductive research. Aquaculture 472, 81–92. doi: 10.1016/j.aquaculture.2016.03.005
Cock, P. J. A., Antao, T., Chang, J. T., Chapman, B. A., Cox, C. J., Dalke, A., et al. (2009). Biopython: freely available python tools for computational molecular biology and bioinformatics. Bioinformatics 25, 1422–1423. doi: 10.1093/bioinformatics/btp163
Dacheux, J. L., Belleannée, C., Guyonnet, B., Labas, V., Teixeira-Gomes, A. P., Ecroyd, H., et al. (2012). The contribution of proteomics to understanding epididymal maturation of mammalian spermatozoa. Syst. Biol. Reprod. Med. 58, 197–210. doi: 10.3109/19396368.2012.663233
Davidson, A. D., Boyer, A. G., Kim, H., Pompa-Mansilla, S., Hamilton, M. J., Costa, D. P., et al. (2012). Drivers and hotspots of extinction risk in marine mammals. Proc. Natl. Acad. Sci. U S A. 109, 3395–3400. doi: 10.1073/pnas.1121469109
De Lazari, F. L., Sontag, E. R., Schneider, A., Araripe Moura, A. A., Vasconcelos, F. R., Nagano, C. S., et al. (2020). Proteomic identification of boar seminal plasma proteins related to sperm resistance to cooling at 17°C. Theriogenology 147, 135–145. doi: 10.1016/j.theriogenology.2019.11.023
De Lazari, F. L., Sontag, E. R., Schneider, A., Moura, A. A. A., Vasconcelos, F. R., Nagano, C. S., et al. (2019). Seminal plasma proteins and their relationship with sperm motility and morphology in boars. Andrologia 51:e13222. doi: 10.1111/and.13222
Dietrich, M. A., Irnazarow, I., and Ciereszko, A. (2017). Proteomic identification of seminal plasma proteins related to the freezability of carp semen. J. Proteomics 162, 52–61. doi: 10.1016/j.jprot.2017.04.015
Dolman, S. J., and Brakes, P. (2018). Sustainable fisheries management and the welfare of bycaught and entangled cetaceans. Front. Vet. Sci. 5:287. doi: 10.3389/fvets.2018.00287
Druart, X., and de Graaf, S. (2018). Seminal plasma proteomes and sperm fertility. Anim. Reprod. Sci. 194, 33–40. doi: 10.1016/j.anireprosci.2018.04.061
Druart, X., Rickard, J. P., Mactier, S., Kohnke, P. L., Kershaw-Young, C. M., Bathgate, R., et al. (2013). Proteomic characterization and cross species comparison of mammalian seminal plasma. J. Proteomics. 91, 13–22. doi: 10.1016/j.jprot.2013.05.029
Druart, X., Rickard, J. P., Tsikis, G., and de Graaf, S. P. (2019). Seminal plasma proteins as markers of sperm fertility. Theriogenology 137, 30–35. doi: 10.1016/j.theriogenology.2019.05.034
Elzanaty, S., Erenpreiss, J., and Becker, C. (2007). Seminal plasma albumin: origin and relation to the male reproductive parameters. Andrologia 39, 60–65. doi: 10.1111/j.1439-0272.2007.00764.x
Fan, K., Jiang, J., Wang, Z., Fan, R., Yin, W., Sun, Y., et al. (2014). Expression and purification of soluble porcine cystatin 11 in pichia pastoris. Appl. Biochem. Biotechnol. 174, 1959–1968. doi: 10.1007/s12010-014-1148-z
Fiedler, S. E., Sisson, J. H., Wyatt, T. A., Pavlik, J. A., Gambling, T. M., Carson, J. L., et al. (2012). Loss of ASP but not ROPN1 reduces mammalian ciliary motility. Cytoskeleton 69, 22–32. doi: 10.1002/cm.20539
Fu, Q., Pan, L., Huang, D., Wang, Z., Hou, Z., and Zhang, M. (2019). Proteomic profiles of buffalo spermatozoa and seminal plasma. Theriogenology 134, 74–82. doi: 10.1016/j.theriogenology.2019.05.013
Fujita, A., Nakamura, K. I., Kato, T., Watanabe, N., Ishizaki, T., Kimura, K., et al. (2000). Ropporin, a sperm-specific binding protein of rhophilin, that is localized in the fibrous sheath of sperm flagella. J. Cell Sci. 113, 103–112. doi: 10.1242/jcs.113.1.103
Gaitskell-Phillips, G., Martín-Cano, F. E., Ortiz-Rodríguez, J. M., Silva-Rodríguez, A., Gil, M. C., Ortega-Ferrusola, C., et al. (2021). Differences in the proteome of stallion spermatozoa explain stallion-to-stallion variability in sperm quality post thaw†. Biol. Reprod. 104, 1097–1113. doi: 10.1093/biolre/ioab003
Gervasi, M. G., and Visconti, P. E. (2016). Chang’s meaning of capacitation: a molecular perspective. Mol. Reprod. Dev. 83, 860–874. doi: 10.1002/mrd.22663
Gopalakrishnan, B., Aravinda, S., Pawshe, C. H., Totey, S. M., Nagpal, S., Salunke, D. M., et al. (1998). Studies on glutathione S-transferases important for sperm function: evidence of catalytic activity-independent functions. Biochem. J. 329, 231–241. doi: 10.1042/bj3290231
Graustein, A. D., Misch, E. A., Musvosvi, M., Shey, M., Shah, J. A., Seshadri, C., et al. (2018). Toll-like receptor chaperone HSP90B1 and the immune response to mycobacteria. PLoS One 13:e0208940. doi: 10.1371/journal.pone.0208940
Guasti, P. N., Souza, F. F., Scott, C., Papa, P. M., Camargo, L. S., Schmith, R. A., et al. (2020). Equine seminal plasma and sperm membrane: functional proteomic assessment. Theriogenology 156, 70–81. doi: 10.1016/j.theriogenology.2020.06.014
Hamil, K. G., Liu, Q., Sivashanmugam, P., Yenugu, S., Soundararajan, R., Grossman, G., et al. (2002). Cystatin 11: a new member of the cystatin type 2 family. Endocrinology 143, 2787–2796. doi: 10.1210/endo.143.7.8925
Hanlon Newell, A. E., Fiedler, S. E., Ruan, J. M., Pan, J., Wang, P. J., Deininger, J., et al. (2008). Protein kinase A RII-like (R2D2) proteins exhibit differential localization and AKAP interaction. Cell Motil. Cytoskeleton 65, 539–552. doi: 10.1002/cm.20279
Harrison, R. J., Boice, R. C., and Brownell, R. L. (1969). Reproduction in wild and captive dolphins. Nature 222, 1143–1147. doi: 10.1038/2221143b0
Holt, W. V., and Pickard, A. R. (1999). Role of reproductive technologies and genetic resource banks in animal conservation. Rev. Reprod. 4, 143–150. doi: 10.1530/ror.0.0040143
Hopkins, B. R., Sepil, I., Thézénas, M. L., Craig, J. F., Miller, T., Charles, P. D., et al. (2019). Divergent allocation of sperm and the seminal proteome along a competition gradient in Drosophila melanogaster. Proc. Natl. Acad. Sci. U S A. 116, 17925–17933. doi: 10.1073/pnas.1906149116
Huang, D. W., Sherman, B. T., and Lempicki, R. A. (2009). Bioinformatics enrichment tools: paths toward the comprehensive functional analysis of large gene lists. Nucleic Acids Res. 37, 1–13. doi: 10.1093/nar/gkn923
Inoue, N., Ikawa, M., Isotani, A., and Okabe, M. (2005). The immunoglobulin superfamily protein Izumo is required for sperm to fuse with eggs. Nature 434, 234–238. doi: 10.1038/nature03362
Inoue, N., Ikawa, M., and Okabe, M. (2011). The mechanism of sperm-egg interaction and the involvement of IZUMO1 in fusion. Asian J. Androl. 13, 81–87. doi: 10.1038/aja.2010.70
Intasqui, P., Camargo, M., Antoniassi, M. P., Cedenho, A. P., Carvalho, V. M., Cardozo, K. H. M., et al. (2016). Association between the seminal plasma proteome and sperm functional traits. Fertil. Steril. 105, 617–628. doi: 10.1016/j.fertnstert.2015.11.005
Ito, C., Akutsu, H., Yao, R., Yoshida, K., Yamatoya, K., Mutoh, T., et al. (2019). Odf2 haploinsufficiency causes a new type of decapitated and decaudated spermatozoa. Odf2-DDS, in mice. Sci. Rep. 9:14249. doi: 10.1038/s41598-019-50516-50512
Jiang, X. P., Wang, S. Q., Wang, W., Xu, Y., Xu, Z., Tang, J. Y., et al. (2015). Enolase1 (ENO1) and glucose-6-phosphate isomerase (GPI) are good markers to predict human sperm freezability. Cryobiology 71, 141–145. doi: 10.1016/j.cryobiol.2015.04.006
Juyena, N. S., and Stelletta, C. (2012). Seminal plasma: an essential attribute to spermatozoa. J. Androl. 33, 536–551. doi: 10.2164/jandrol.110.012583
Kasimanickam, R. K., Kasimanickam, V. R., Arangasamy, A., and Kastelic, J. P. (2019). Sperm and seminal plasma proteomics of high- versus low-fertility holstein bulls. Theriogenology 126, 41–48. doi: 10.1016/j.theriogenology.2018.11.032
Katsumata, E. (2010). Study on reproduction of captive marine mammals. J. Reprod. Dev. 56, 1–8. doi: 10.1262/jrd.09-212E
Kawano, N., Araki, N., Yoshida, K., Hibino, T., Ohnami, N., Makino, M., et al. (2014). Seminal vesicle protein SVS2 is required for sperm survival in the uterus. Proc. Natl. Acad. Sci. U S A. 111, 4145–4150. doi: 10.1073/pnas.1320715111
Kierszenbaum, A. L. (2002). Sperm axoneme: a tale of tubulin posttranslation diversity. Mol. Reprod. Dev. 62, 1–3. doi: 10.1002/mrd.10139
Kikuchi, M., Mizoroki, S., Kubo, T., Ohiwa, Y., Kubota, M., Yamada, N., et al. (2003a). Seminal plasma lactoferrin but not transferrin reflects gonadal function in dogs. J. Vet. Med. Sci. 65, 679–684. doi: 10.1292/jvms.65.679
Kikuchi, M., Takao, Y., Tokuda, N., Ohnami, Y., Orino, K., and Watanabe, K. (2003b). Relationship between seminal plasma lactoferrin and gonadal function in horses. J. Vet. Med. Sci. 65, 1273–1274. doi: 10.1292/jvms.65.1273
Kwon, W. S., Oh, S. A., Kim, Y. J., Rahman, M. S., Park, Y. J., and Pang, M. G. (2015a). Proteomic approaches for profiling negative fertility markers in inferior boar spermatozoa. Sci. Rep. 5:13821. doi: 10.1038/srep13821
Kwon, W. S., Rahman, M. S., Ryu, D. Y., Park, Y. J., and Pang, M. G. (2015b). Increased male fertility using fertility-related biomarkers. Sci. Rep. 5:15654. doi: 10.1038/srep15654
Légaré, C., Thabet, M., Gatti, J. L., and Sullivan, R. (2006). HE1/NPC2 status in human reproductive tract and ejaculated spermatozoa: consequence of vasectomy. Mol. Hum. Reprod. 12, 461–468. doi: 10.1093/molehr/gal050
Li, C. J., Wang, D., and Zhou, X. (2016). Sperm proteome and reproductive technologies in mammals. Anim. Reprod. Sci. 173, 1–7. doi: 10.1016/j.anireprosci.2016.08.008
Li, Y. F., He, W., Jha, K. N., Klotz, K., Kim, Y. H., Mandal, A., et al. (2007). FSCB, a novel protein kinase A-phosphorylated calcium-binding protein, is a CABYR-binding partner involved in late steps of fibrous sheath biogenesis. J. Biol. Chem. 282, 34104–34119. doi: 10.1074/jbc.M702238200
Li, Y. F., He, W., Mandal, A., Kim, Y. H., Digilio, L., Klotz, K., et al. (2011). CABYR binds to AKAP3 and ropporin in the human sperm fibrous sheath. Asian J. Androl. 13, 266–274. doi: 10.1038/aja.2010.149
Llavanera, M., Delgado-Bermúdez, A., Fernandez-Fuertes, B., Recuero, S., Mateo, Y., Bonet, S., et al. (2019). GSTM3, but not IZUMO1, is a cryotolerance marker of boar sperm. J. Anim. Sci. Biotechnol. 10:61. doi: 10.1186/s40104-019-0370-375
Llavanera, M., Delgado-Bermúdez, A., Mateo-Otero, Y., Padilla, L., Romeu, X., Roca, J., et al. (2020a). Exploring seminal plasma GSTM3 as a quality and in vivo fertility biomarker in pigs—relationship with sperm morphology. Antioxidants 9:741. doi: 10.3390/antiox9080741
Llavanera, M., Delgado-bermúdez, A., Olives, S., Mateo-otero, Y., Recuero, S., Bonet, S., et al. (2020b). Glutathione S-transferases play a crucial role in mitochondrial function, plasma membrane stability and oxidative regulation of mammalian sperm. Antioxidants 9:100. doi: 10.3390/antiox9020100
Luongo, C., Abril-Sánchez, S., Hernández, J. G., and García-Vázquez, F. A. (2019). Seminal plasma mitigates the adverse effect of uterine fluid on boar spermatozoa. Theriogenology 136, 28–35. doi: 10.1016/j.theriogenology.2019.06.018
Luongo, C., González-Brusi, L., Cots-Rodríguez, P., Izquierdo-Rico, M. J., Avilés, M., and García-Vázquez, F. A. (2020). Sperm proteome after interaction with reproductive fluids in porcine: from the ejaculation to the fertilization site. Int. J. Mol. Sci. 21, 1–27. doi: 10.3390/ijms21176060
Magister, Š, and Kos, J. (2013). Cystatins in immune system. J. Cancer 4, 45–56. doi: 10.7150/jca.5044
Martinez, C. A., Alvarez-Rodriguez, M., Wright, D., and Rodriguez-Martinez, H. (2020). Does the pre-ovulatory pig oviduct rule sperm capacitation in vivo mediating transcriptomics of catsper channels? Int. J. Mol. Sci. 21:1840. doi: 10.3390/ijms21051840
Martinez, G., Kherraf, Z. E., Zouari, R., Mustapha, S. F., Ben, Saut, A., et al. (2018). Whole-exome sequencing identifies mutations in FSIP2 as a recurrent cause of multiple morphological abnormalities of the sperm flagella. Hum. Reprod. 33, 1973–1984. doi: 10.1093/humrep/dey264
Martínez-Fresneda, L., Sylvester, M., Shakeri, F., Bunes, A., Del Pozo, J. C., García-Vázquez, F. A., et al. (2021). Differential proteome between ejaculate and epididymal sperm represents a key factor for sperm freezability in wild small ruminants. Cryobiology 99, 64–77. doi: 10.1016/j.cryobiol.2021.01.012
Martínez-Heredia, J., Estanyol, J. M., Ballescà, J. L., and Oliva, R. (2006). Proteomic identification of human sperm proteins. Proteomics 6, 4356–4369. doi: 10.1002/pmic.200600094
Martins, H. S., da Silva, G. C., Cortes, S. F., Paes, F. O., Martins Filho, O. A., Araujo, M. S. S., et al. (2018). Lactoferrin increases sperm membrane functionality of frozen equine semen. Reprod. Domest. Anim. 53, 617–623. doi: 10.1111/rda.13148
Mead, J. G. (2018). Shepherd’s beaked whale. Encyclopedia Mar. Mamm. 2018, 853–854. doi: 10.1016/B978-0-12-804327-1.00227-222
Mogielnicka-Brzozowska, M., Prochowska, S., Niżański, W., Bromke, M. A., Wiśniewski, J., Olejnik, B., et al. (2020). Proteome of cat semen obtained after urethral catheterization. Theriogenology 141, 68–81. doi: 10.1016/j.theriogenology.2019.09.003
Naaby-Hansen, S., Mandal, A., Wolkowicz, M. J., Sen, B., Westbrook, V. A., Shetty, J., et al. (2002). CABYR, a novel calcium-binding tyrosine phosphorylation-regulated fibrous sheath protein involved in capacitation. Dev. Biol. 242, 236–254. doi: 10.1006/dbio.2001.0527
O’Brien, J., and Robeck, T. (2010). The value of Ex situ cetacean populations in understanding reproductive physiology and developing assisted reproductive technology for Ex Situ and in Situ species management and conservation efforts. Int. J. Comp. Psychol. 23, 227–248. doi: 10.1017/cbo9781139051927.012
Panner Selvam, M., Agarwal, A., and Baskaran, S. (2019). Proteomic analysis of seminal plasma from bilateral varicocele patients indicates an oxidative state and increased inflammatory response. Asian J. Androl. 21, 544–550. doi: 10.4103/aja.aja_121_18
Park, Y. J., Pang, W. K., Ryu, D. Y., Song, W. H., Rahman, M. S., and Pang, M. G. (2019). Optimized combination of multiple biomarkers to improve diagnostic accuracy in male fertility. Theriogenology 139, 106–112. doi: 10.1016/j.theriogenology.2019.07.029
Parrilla, I., Perez-Patiño, C., Li, J., Barranco, I., Padilla, L., Rodriguez-Martinez, H., et al. (2019). Boar semen proteomics and sperm preservation. Theriogenology 137, 23–29. doi: 10.1016/j.theriogenology.2019.05.033
Parsons, E. C. M., Favaro, B., Aguirre, A. A., Bauer, A. L., Blight, L. K., Cigliano, J. A., et al. (2014). Seventy-one important questions for the conservation of marine biodiversity. Conserv. Biol. 28, 1206–1214. doi: 10.1111/cobi.12303
Pearl, C. A., and Roser, J. F. (2008). Expression of lactoferrin in the boar epididymis: effects of reduced estrogen. Domest. Anim. Endocrinol. 34, 153–159. doi: 10.1016/j.domaniend.2007.01.001
Peddinti, D., Nanduri, B., Kaya, A., Feugang, J. M., Burgess, S. C., and Memili, E. (2008). Comprehensive proteomic analysis of bovine spermatozoa of varying fertility rates and identification of biomarkers associated with fertility. BMC Syst. Biol. 2:19. doi: 10.1186/1752-0509-2-19
Pelloni, M., Paoli, D., Majoli, M., Pallotti, F., Carlini, T., Lenzi, A., et al. (2018). Molecular study of human sperm RNA: ropporin and CABYR in asthenozoospermia. J. Endocrinol. Invest. 41, 781–787. doi: 10.1007/s40618-017-0804-x
Perez-Patiño, C., Barranco, I., Parrilla, I., Martinez, E. A., Rodriguez-Martinez, H., and Roca, J. (2016). Extensive dataset of boar seminal plasma proteome displaying putative reproductive functions of identified proteins. Data Br. 8, 1370–1373. doi: 10.1016/j.dib.2016.07.037
Pérez-Patiño, C., Parrilla, I., Barranco, I., Vergara-Barberán, M., Simó-Alfonso, E. F., Herrero-Martínez, J. M., et al. (2018). New in-depth analytical approach of the porcine seminal plasma proteome reveals potential fertility biomarkers. J. Proteome Res. 17, 1065–1076. doi: 10.1021/acs.jproteome.7b00728
Perez-Riverol, Y., Csordas, A., Bai, J., Bernal-Llinares, M., Hewapathirana, S., Kundu, D. J., et al. (2019). The PRIDE database and related tools and resources in 2019: improving support for quantification data. Nucleic Acids Res. 47, D442–D450. doi: 10.1093/nar/gky1106
Peris-Frau, P., Martín-Maestro, A., Iniesta-Cuerda, M., Sánchez-Ajofrín, I., Mateos-Hernández, L., Garde, J. J., et al. (2019). Freezing-thawing procedures remodel the proteome of ram sperm before and after in vitro capacitation. Int. J. Mol. Sci. 20:4596. doi: 10.3390/ijms20184596
Petit, F. M., Serres, C., Bourgeon, F., Pineau, C., and Auer, J. (2013). Identification of sperm head proteins involved in zona pellucida binding. Hum. Reprod. 28, 852–865. doi: 10.1093/humrep/des452
Pini, T., Leahy, T., Soleilhavoup, C., Tsikis, G., Labas, V., Combes-Soia, L., et al. (2016). Proteomic investigation of ram spermatozoa and the proteins conferred by seminal plasma. J. Proteome Res. 15, 3700–3711. doi: 10.1021/acs.jproteome.6b00530
Pinto, T. M. F., Moreira, R. F., Matos, M. N. C., Soares, V. V. M., de Almeida Aguiar, M. V., de Aragão, P., et al. (2019). Evaluation of the proteomic profiles of ejaculated spermatozoa from Saanen bucks (Capra hircus). Anim. Reprod. 16, 902–913. doi: 10.21451/1984-3143-AR2019-0001
Piomboni, P., Gambera, L., Serafini, F., Campanella, G., Morgante, G., and De Leo, V. (2008). Sperm quality improvement after natural anti-oxidant treatment of asthenoteratospermic men with leukocytospermia. Asian J. Androl. 10, 201–206. doi: 10.1111/j.1745-7262.2008.00356.x
Rahman, M. S., Kwon, W. S., and Pang, M. G. (2017). Prediction of male fertility using capacitation-associated proteins in spermatozoa. Mol. Reprod. Dev. 84, 749–759. doi: 10.1002/mrd.22810
Ramesha, K. P., Mol, P., Kannegundla, U., Thota, L. N., Gopalakrishnan, L., Rana, E., et al. (2020). Deep proteome profiling of semen of indian indigenous malnad gidda (Bos indicus) cattle. J. Proteome Res. 19, 3364–3376. doi: 10.1021/acs.jproteome.0c00237
Ramm, S. A. (2014). Sperm competition and the evolution of reproductive systems. Mol. Hum. Reprod. 20, 1159–1160. doi: 10.1093/molehr/gau076
Recuero, S., Fernandez-Fuertes, B., Bonet, S., Barranco, I., and Yeste, M. (2019). Potential of seminal plasma to improve the fertility of frozen-thawed boar spermatozoa. Theriogenology 137, 36–42. doi: 10.1016/j.theriogenology.2019.05.035
Reeves, R. R., Smith, B. D., Crespo, E. A., and Notarbartolo di Sciara, G. (2003). Dolphins, Whales and Porpoises: 2002–2010. Conservation Action Plan for the World’s Cetaceans. IUCN/SSC Cetacean Specialist Group. Gland: IUCN.
Rego, J. P. A., Crisp, J. M., Moura, A. A., Nouwens, A. S., Li, Y., Venus, B., et al. (2014). Seminal plasma proteome of electroejaculated Bos indicus bulls. Anim. Reprod. Sci. 148, 1–17. doi: 10.1016/j.anireprosci.2014.04.016
Rickard, J. P., and de Graaf, S. P. (2020). Sperm surface changes and their consequences for sperm transit through the female reproductive tract. Theriogenology 150, 96–105. doi: 10.1016/j.theriogenology.2020.02.018
Robeck, T. R., Montano, G. A., Steinman, K. J., Smolensky, P., Sweeney, J., Osborn, S., et al. (2013). Development and evaluation of deep intra-uterine artificial insemination using cryopreserved sexed spermatozoa in bottlenose dolphins (Tursiops truncatus). Anim. Reprod. Sci. 139, 168–181. doi: 10.1016/j.anireprosci.2013.04.004
Robeck, T. R., and O’Brien, J. K. (2004). Effect of cryopreservation methods and precryopreservation storage on bottlenose dolphin (Tursiops truncatus) spermatozoa. Biol. Reprod. 70, 1340–1348. doi: 10.1095/biolreprod.103.025304
Robeck, T. R., Steinman, K. J., Yoshioka, M., Jensen, E., O’Brien, J. K., Katsumata, E., et al. (2005). Estrous cycle characterisation and artificial insemination using frozen-thawed spermatozoa in the bottlenose dolphin (Tursiops truncatus). Reproduction 129, 659–674. doi: 10.1530/rep.1.00516
Rommel, S., Pabst, D., and McLellan, W. (2007). “Functional anatomy of the cetacean reproductive system, with comparisons to the domestic dog,” in Reproductive Biology and Phylogeny of Cetacea, eds B. G. M. Jamieson, and D. Miller (Boca Raton, FL: CRC Press), doi: 10.1201/b11001-5
Ruiz-Díaz, S., Luongo, C., Fuentes-Albero, M. C., Abril-Sánchez, S., Sánchez-Calabuig, M. J., Barros-García, C., et al. (2020). Effect of temperature and cell concentration on dolphin (Tursiops truncatus) spermatozoa quality evaluated at different days of refrigeration. Anim. Reprod. Sci. 212:106248. doi: 10.1016/j.anireprosci.2019.106248
Ryu, D. Y., Song, W. H., Pang, W. K., Yoon, S. J., Rahman, M. S., and Pang, M. G. (2019). Freezability biomarkers in bull epididymal spermatozoa. Sci. Rep. 9:12797. doi: 10.1038/s41598-019-49378-49375
Sánchez-Calabuig, M. J., de la Fuente, J., Laguna-Barraza, R., Beltrán-Breña, P., Martínez-Nevado, E., Johnston, S. D., et al. (2015a). Heterologous murine and bovine IVF using bottlenose dolphin (Tursiops truncatus) spermatozoa. Theriogenology 84, 983–994. doi: 10.1016/j.theriogenology.2015.06.001
Sánchez-Calabuig, M. J., López-Fernández, C., Johnston, S. D., Blyde, D., Cooper, J., Harrison, K., et al. (2015b). Effect of cryopreservation on the sperm DNA fragmentation dynamics of the bottlenose dolphin (Tursiops truncatus). Reprod. Domest. Anim. 50, 227–235. doi: 10.1111/rda.12474
Sánchez-Calabuig, M. J., García-Vázquez, F. A., Laguna-Barraza, R., Barros-García, C., García-Parraga, D., Rizos, D., et al. (2017). Bottlenose dolphin (Tursiops truncatus) spermatozoa: collection, cryopreservation, and heterologous in vitro fertilization. J. Vis. Exp. 2017:55237. doi: 10.3791/55237
Shevchenko, A., Jensen, O. N., Podtelejnikov, A. V., Sagliocco, F., Wilm, M., Vorm, O., et al. (1996). Linking genome and proteome by mass spectrometry: large-scale identification of yeast proteins from two dimensional gels. Proc. Natl. Acad. Sci. U S A. 93, 14440–14445. doi: 10.1073/pnas.93.25.14440
Shilov, I. V., Seymourt, S. L., Patel, A. A., Loboda, A., Tang, W. H., Keating, S. P., et al. (2007). The paragon algorithm, a next generation search engine that uses sequence temperature values sequence temperature values and feature probabilities to identify peptides from tandem mass spectra. Mol. Cell. Proteomics 6, 1638–1655. doi: 10.1074/mcp.T600050-MCP200
Sirigu, P., Perra, M. T., and Turno, F. (1995). Immunohistochemical study of secretory IGA in the human male reproductive tract. Andrologia 27, 335–339. doi: 10.1111/j.1439-0272.1995.tb01368.x
Skerget, S., Rosenow, M., Polpitiya, A., Petritis, K., Dorus, S., and Karr, T. L. (2013). The rhesus macaque (macaca mulatta) sperm proteome. Mol. Cell. Proteomics 12, 3052–3067. doi: 10.1074/mcp.M112.026476
Soleilhavoup, C., Tsikis, G., Labas, V., Harichaux, G., Kohnke, P. L., Dacheux, J. L., et al. (2014). Ram seminal plasma proteome and its impact on liquid preservation of spermatozoa. J. Proteomics 109, 245–260. doi: 10.1016/j.jprot.2014.07.007
Spaulding, M., O’Leary, M. A., and Gatesy, J. (2009). Relationships of cetacea (Artiodactyla) among mammals: increased taxon sampling alters interpretations of key fossils and character evolution. PLoS One 4:e7062. doi: 10.1371/journal.pone.0007062
Suárez-Santana, C. M., Fernández, A., Sierra, E., Arbelo, M., Bernaldo, de Quirós, Y., et al. (2020). Comparative morphology, histology, and cytology of odontocete cetaceans prostates. Anat. Rec. 303, 2036–2053. doi: 10.1002/ar.24285
Sun, Y., Xiao, S., Chen, J., Wang, M., Zheng, Z., Song, S., et al. (2015). Heat shock protein 90 mediates the apoptosis and autophage in nicotinic-mycoepoxydienetreated HeLa cells. Acta Biochim. Biophys. Sin. (Shanghai). 47, 451–458. doi: 10.1093/abbs/gmv034
Swegen, A., Curry, B. J., Gibb, Z., Lambourne, S. R., Smith, N. D., and Aitken, R. J. (2015). Investigation of the stallion sperm proteome by mass spectrometry. Reproduction 149, 235–244. doi: 10.1530/REP-14-0500
Takenaka, A., Kashiwagi, N., Maezono, Y., Nakao, T., Wano, Y., Kakizoe, Y., et al. (2013). Study on the ejaculate characteristics and liquid storage of semen in the common bottlenose dolphin (Tursiops truncatus). Japanese J. Zoo Wildl. Med. 18, 107–114. doi: 10.5686/jjzwm.18.107
Thewissen, J. G. M., Cooper, L. N., Clementz, M. T., Bajpai, S., and Tiwari, B. N. (2007). Whales originated from aquatic artiodactyls in the Eocene epoch of India. Nature 450, 1190–1194. doi: 10.1038/nature06343
Uhen, M. D. (2007). Evolution of marine mammals: back to the sea after 300 million years. Anat. Rec. 290, 514–522. doi: 10.1002/ar.20545
Valencia, J., Yeste, M., Quintero-Moreno, A., Niño-Cardenas, C., del, P., and Henao, F. J. (2020). Relative content of Niemann-Pick C2 protein (NPC2) in seminal plasma, but not that of spermadhesin AQN-1, is related to boar sperm cryotolerance. Theriogenology 14, 181–189. doi: 10.1016/j.theriogenology.2019.10.023
van der Horst, G., Medger, K., Steckler, D., Luther, I., and Bartels, P. (2018). Bottlenose dolphin (Tursiops truncatus) sperm revisited: motility, morphology and ultrastructure of fresh sperm of consecutive ejaculates. Anim. Reprod. Sci. 195, 309–320. doi: 10.1016/j.anireprosci.2018.06.009
Vicens, A., Borziak, K., Karr, T. L., Roldan, E. R. S., and Dorus, S. (2017). Comparative sperm proteomics in mouse species with divergent mating systems. Mol. Biol. Evol. 34, 1403–1416. doi: 10.1093/molbev/msx084
Vislobokova, I. A. (2013). On the origin of cetartiodactyla: comparison of data on evolutionary morphology and molecular biology. Paleontol. J. 47, 321–334. doi: 10.1134/S003103011303012X
Wichmann, L., Vaalasti, A., Vaalasti, T., and Tuohimaa, P. (1989). Localization of lactoferrin in the male reproductive tract. Int. J. Androl. 12, 179–186. doi: 10.1111/j.1365-2605.1989.tb01302.x
Xu, K., Yang, L., Zhang, L., and Qi, H. (2020). Lack of AKAP3 disrupts integrity of the subcellular structure and proteome of mouse sperm and causes male sterility. Development 147:dev181057. doi: 10.1242/dev.181057
Yang, Y., Jiang, C., Zhang, X., Liu, X., Li, J., Qiao, X., et al. (2020). Loss-of-function mutation in DNAH8 induces asthenoteratospermia associated with multiple morphological abnormalities of the sperm flagella. Clin. Genet. 98, 396–401. doi: 10.1111/cge.13815
Zeinali, M., Hadian Amree, A., Khorramdelazad, H., Karami, H., and Abedinzadeh, M. (2017). Inflammatory and anti-inflammatory cytokines in the seminal plasma of infertile men suffering from varicocele. Andrologia 49, e12685. doi: 10.1111/and.12685
Zhang, X., Chen, M., Yu, R., Liu, B., Tian, Z., and Liu, S. (2016). FSCB phosphorylation regulates mouse spermatozoa capacitation through suppressing SUMOylation of ROPN1/ROPN1L. Am. J. Transl. Res. 8, 2776–2782.
Zhao, W., Li, Z., Ping, P., Wang, G., Yuan, X., and Sun, F. (2018). Outer dense fibers stabilize the axoneme to maintain sperm motility. J. Cell. Mol. Med. 22, 1755–1768. doi: 10.1111/jcmm.13457
Zhu, W., Cheng, X., Ren, C., Chen, J., Zhang, Y., Chen, Y., et al. (2020). Proteomic characterization and comparison of ram (Ovis aries) and buck (Capra hircus) spermatozoa proteome using a data independent acquisition mass spectometry (DIA-MS) approach. PLoS One 15:e0228656. doi: 10.1371/journal.pone.0228656
Keywords: cetacean, proteomic analyses, semen, sperm cells, seminal plasma
Citation: Fuentes-Albero M-C, González-Brusi L, Cots P, Luongo C, Abril-Sánchez S, Ros-Santaella JL, Pintus E, Ruiz-Díaz S, Barros-García C, Sánchez-Calabuig M-J, García-Párraga D, Avilés M, Izquierdo Rico MJ and García-Vázquez FA (2021) Protein Identification of Spermatozoa and Seminal Plasma in Bottlenose Dolphin (Tursiops truncatus). Front. Cell Dev. Biol. 9:673961. doi: 10.3389/fcell.2021.673961
Received: 28 February 2021; Accepted: 28 May 2021;
Published: 16 July 2021.
Edited by:
Ana Josefa Soler, University of Castilla-La Mancha, SpainReviewed by:
Micaela Cerletti, National University of Mar del Plata, ArgentinaLucia Zalazar, Instituto de Investigaciones Biológicas, Consejo Nacional de Investigaciones Científicas y Técnicas, Argentina
Copyright © 2021 Fuentes-Albero, González-Brusi, Cots, Luongo, Abril-Sánchez, Ros-Santaella, Pintus, Ruiz-Díaz, Barros-García, Sánchez-Calabuig, García-Párraga, Avilés, Izquierdo Rico and García-Vázquez. This is an open-access article distributed under the terms of the Creative Commons Attribution License (CC BY). The use, distribution or reproduction in other forums is permitted, provided the original author(s) and the copyright owner(s) are credited and that the original publication in this journal is cited, in accordance with accepted academic practice. No use, distribution or reproduction is permitted which does not comply with these terms.
*Correspondence: Francisco Alberto García-Vázquez, ZmFnYXJjaWFAdW0uZXM=