- 1Department de Ciències Fisiològiques, Facultat de Medicina i Ciències de la Salut, Campus de Bellvitge, Universitat de Barcelona, L’Hospitalet de Llobregat, Spain
- 2Institut de Neurociències, Universitat de Barcelona, Barcelona, Spain
- 3Institut D'Investigació Biomèdica de Bellvitge, IDIBELL, L’Hospitalet de Llobregat, Spain
Hearing or balance loss are disabling conditions that have a serious impact in those suffering them, especially when they appear in children. Their ultimate cause is frequently the loss of function of mechanosensory hair cells in the inner ear. Hair cells can be damaged by environmental insults, like noise or chemical agents, known as ototoxins. Two of the most common ototoxins are life-saving medications: cisplatin against solid tumors, and aminoglycoside antibiotics to treat infections. However, due to their localization inside the temporal bone, hair cells are difficult to study in mammals. As an alternative animal model, zebrafish larvae have hair cells similar to those in mammals, some of which are located in a fish specific organ on the surface of the skin, the lateral line. This makes them easy to observe in vivo and readily accessible for ototoxins or otoprotective substances. These features have made possible advances in the study of the mechanisms mediating ototoxicity or identifying new potential ototoxins. Most importantly, the small size of the zebrafish larvae has allowed screening thousands of molecules searching for otoprotective agents in a scale that would be highly impractical in rodent models. The positive hits found can then start the long road to reach clinical settings to prevent hearing or balance loss.
Introduction
Hair cells are specialized mechanosensory epithelial cells responsible for the transduction of sound and accelerations, important for body orientation, vision stabilization and motor control. In mammals, they are found in the cochlea and the vestibular system. The transducing part of the hair cells, as indicated by its name, is a bundle of stereocilia in their apical side that bend in response to the vibration of sound waves or the movement of fluid inside the inner ear cavities. Cilia deflection opens ion channels that lead to the depolarization of the hair cell and activate synaptic transmission to afferent neurons from the spiral (auditory) or vestibular ganglia (Ó Maoiléidigh and Ricci, 2019). Hair cells contain in their basolateral membrane a specific type of presynaptic machinery, the ribbon synapse (also present in some retinal cells), adapted to constant activity (Moser et al., 2020). Hearing or vestibular impairment are disabling conditions that represent a major impact in the lives of those afflicted, particularly children (WHO).
Hair cells are exquisitely sensitive to insults and deficits in hair cell function cause hearing loss and imbalance. This loss is irreversible, as hair cells in adult mammals have a very limited, if any, regenerative capacity (Rubel et al., 2013; Lee and Waldhaus, 2022). These deficits can be triggered by age, viral infection, or environmental insults, such as noise or chemical substances (collectively known as ototoxins), by either accidental exposure or pharmacological treatment. Among the latter, two drugs can be highlighted due to their widespread use: the chemotherapeutic agent cisplatin and the aminoglycoside family of antibiotics (Schacht et al., 2012). Effects of ototoxins can lead to the death of the hair cells or to damage in their synaptic coupling to afferent neurons (Liberman and Kujawa, 2017). Therefore, potential ototoxicity of novel drugs should be assessed before or during clinical trials to prevent harmful side effects (Coffin et al., 2021), as well as the ototoxicity of environmental pollutants (Fábelová et al., 2019). Equally important, knowledge of the mechanisms that produce ototoxicity will help in obtaining drugs that would prevent or mitigate these known ototoxic effects when inevitable.
Many proteins essential for hair cell function (cilia components, ion channels, neurotransmitter release machinery, synaptic junction proteins, transcription factors) have been found through identifying the genes responsible for congenital deafness or generating deaf animal models (Taiber et al., 2022). However, research into the normal biology of hair cells or the pathophysiological mechanisms that lead to their dysfunction has been hampered in mammals by their localization inside the temporal bone, making them difficult to reach or sample, and its complex three-dimensional architecture. Additionally, clinically relevant mammalian models of ototoxicity have been difficult to develop due to overall toxicity after systemic administration of the ototoxin (Wu et al., 2001; Murillo-Cuesta et al., 2010; Fernandez et al., 2019). There are cell lines derived from the cochlea (Weir et al., 2000; Kalinec et al., 2003), but none of them is truly generating hair cells in vitro, which require specific physiological conditions (i.e., a potassium-rich extracellular environment) and supporting cells to be functional. Other experimental systems to study hair cells in mammals imply the use of neonatal or adult inner ear cultures (Cunningham, 2006; Ou et al., 2013; Ogier et al., 2019), which are laborious and offer limited throughput in toxicology studies (Lim et al., 2018). These issues could be solved by forthcoming advances in organoid research (Nist-Lund et al., 2022).
Mechanosensory cells are present throughout the animal kingdom, and hair cells homologous to those found in mammals have been observed in all vertebrate groups (Coffin et al., 2004). Ototoxicity has been studied in birds (Park and Cohen, 1982) and, unlike mammals, their hair cells show regenerative capacity (Cruz et al., 1987). The inner ears of birds are similar to those of mammals, and present the same problem: difficult access and limited throughput. Hair cells in zebrafish larvae are also able to regenerate after injury (Williams and Holder, 2000; Harris et al., 2003), and they offer two key advantages for ototoxicology research: accessible hair cells and a small size that allows upscaling.
Zebrafish hair cells are found in the inner ear and in a fish-specific organ, the lateral line (Figure 1A). This organ is responsible for sensing water flow in the surface of the fish (Suli et al., 2012), for detecting prey or predators (McHenry et al., 2009) and for schooling behavior (Venuto et al., 2022). The lateral line extends around the head (anterior lateral line, aLL) and along the rest of the body (posterior lateral line, pLL) and is formed by a series of structures called neuromasts, which consist of groups of hair cells and supporting cells (Ghysen and Dambly-Chaudière, 2007; Thomas and Raible, 2020). The lateral line is functional at 4–5 days post-fertilization and the biophysical properties of hair cells in zebrafish larvae at that stage have been characterized (Olt et al., 2014).
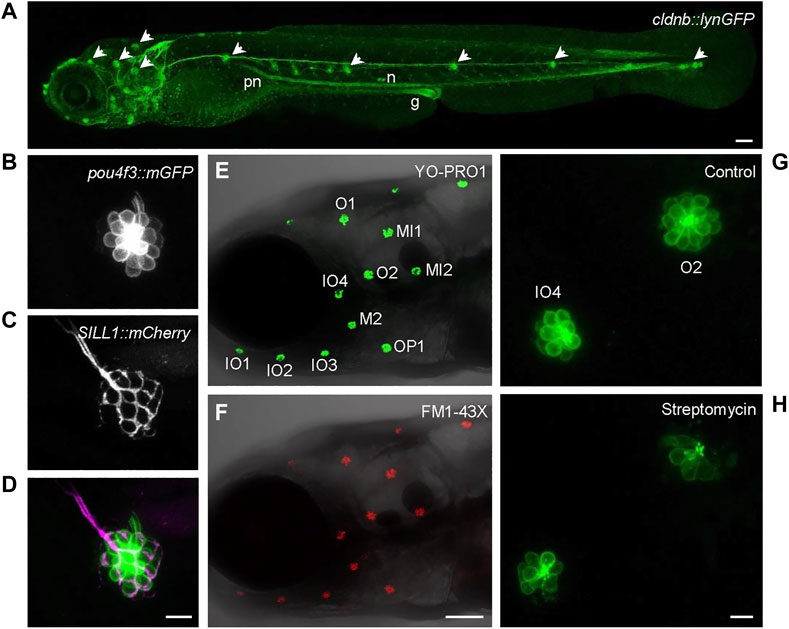
FIGURE 1. Images of hair cells in 5dpf zebrafish larvae. (A) Composite image obtained with laser scanning microscopy of a transgenic larva expressing cldnb::lynGFP (Haas and Gilmour, 2006), gift of Paola Bovolenta (CSIC, Madrid). Arrowheads point to some of the neuromast of the anterior (head) and posterior (trunk) lateral line. pn, pronephric duct; n, nephrons; g, distal gut. (B–D) Detail of an individual neuromast of the posterior lateral line showing (B) hair cells expressing the transgene pou4f3::mGFP (Obholzer et al., 2008), gift of Berta Alisa (Univ. Pompeu Fabra, Barcelona); (C) its afferent innervation expressing the transgene SILL1::mCherry (Pujol-Martí et al., 2012), gift of Hernán López-Schier (Helmholtz Zentrum, Munich); (D) overlay of the previous images. (E,F) Overview of the anterior lateral line showing hair cells labelled with (E) YO-PRO1 and (F) FM1-43X; neuromasts are labelled according to (Raible and Kruse, 2000). Inner ear hair cells are not labelled with these dyes; otoliths are visible behind neuromasts O2 and MI2. (G,H) Image of two anterior lateral line neuromasts labelled with the transgene pou4f3::mGFP under standard conditions (G) or after treatment with the aminoglycoside antibiotic streptomycin (H). Scale bar in A, E, (F) 100 μm; scale bar in (B,C,D,G,H) 10 μm. All images taken in the Microscopy Unit of Centres Científics i Tecnològics de la Universitat de Barcelona (CCiTUB) at the Campus de Bellvitge.
While the hair cells in the inner ear of the zebrafish larvae are located inside the otic capsule (Alsina and Whitfield, 2017), lateral line hair cells are exposed to the aquatic medium and are thus accessible for substances dissolved therein, like chemical agents whose toxic or protective effect on hair cells needs to be assessed (Ton and Parng, 2005). The superficial localization of the lateral line in the zebrafish larvae allows for the observation of hair cells in vivo. They can be labelled with fluorescent dyes added to the medium for a short time, like Yo-Pro1 (Figure 1E), FM1-43 (Figure 1F), DASPEI or DAPI (Baxendale and Whitfield, 2016). Alternatively, many transgenic zebrafish lines express fluorescent proteins or genetically-encoded indicators under the control of hair cell-specific promoters, such as pou4f3 (Figure 1B; Xiao et al., 2005), myo6b (Obholzer et al., 2008) or pavlb3a/b (McDermott et al., 2010), that allow their observation in vivo for extended periods (Zhang and Kindt, 2022). Lateral line hair cell function can be measured directly by stimulation with a micro water jet (De Faveri et al., 2021). Indirect measurements are possible as well, as perturbations of the lateral line function will alter zebrafish larvae swimming behavior in a water current (Suli et al., 2012; Niihori et al., 2015; Todd et al., 2017; Tantry et al., 2022), even in an ototoxin-specific manner (Newton et al., 2022). The lateral line is also responsible for the larval startle response, an avoidance reflex upon perturbation of their surroundings (Buck et al., 2012). Some of the first genes affecting hair cell function in zebrafish were identified because the defective larvae swam in circles, hence called circler mutants (Nicolson et al., 1998).
Zebrafish offer other advantages as an experimental animal model, such as being more amenable to genetic manipulation, allowing loss-of-function analysis or generation of transgenic animals (Holtzman et al., 2016). An individual healthy female zebrafish can lay more than a hundred eggs on a weekly basis, and systems for multiple spawning exist that can yield thousands of eggs simultaneously (Adatto et al., 2011); therefore, obtaining embryos for toxicological testing is not an issue, even in small facilities. As the use of animals in experimental research should adhere to the 3Rs (Replacement, Reduction and Refinement) principle following Directive 2010/63/EU in the European Union, there is another advantage on the use zebrafish larvae for toxicological studies: they are not constricted by the regulation on animal experimentation. It should be noted, however, that these criteria might vary between countries and even different institutions in the same country (i.e., US). Zebrafish larvae are considered experimental animals in the European Union only from 5 days post fertilization (dpf) on, when they can feed on their own (Strähle et al., 2012; Moulder, 2016), and by that moment, the hair cells of the lateral line are already developed and functional. Therefore, zebrafish larvae represent an in vivo model with the advantages of an in vitro system for high-throughput toxicological studies focused on hair cells or other organs (Cassar et al., 2020; MacRae and Peterson, 2022).
This work will review recent advances in ototoxicology using the zebrafish as experimental model and its potential as a tool for the discovery of drugs with ototoxicity and drugs that would prevent hair cell damage.
Cisplatin ototoxicity in zebrafish
Cisplatin (cis-dichlorodiammineplatinum (II)) is an antineoplasic agent widely used in adults and children (Ranasinghe et al., 2022). Upon entering the cell, it binds to DNA, creating platinum covalent adducts which damage DNA, triggering DNA-repair response, oxidative stress and subsequent apoptosis (Riddell and Lippard, 2018; Gentilin et al., 2019). Despite its effectiveness against solid tumors, cisplatin presents with high prevalence dose-limiting adverse side effects due to its action in non-proliferating cells; it is toxic to liver, kidney, nervous system and the cochlea, affecting hair cells and spiral ganglion neurons (Callejo et al., 2015; Rybak et al., 2019; Prayuenyong et al., 2021). Close to half a million cases of hearing loss associated to the treatment with cisplatin per year globally have been estimated (Dillard et al., 2022).
Cisplatin was found to cause hair cell death in the lateral line of zebrafish larvae in a dose-dependent manner (Ton and Parng, 2005; Ou et al., 2007). Cisplatin effects on adult zebrafish have been less studied, but it does produce hair cell damage in the lateral line and changes in their swimming behavior (Kim et al., 2014). In addition to hair cells, cisplatin also affects the embryonic kidney as in mammals (Hentschel et al., 2005) and other cell types in the skin, the ionocytes, responsible for ionic homeostasis (Hung et al., 2019). Ionocytes have been found recently to migrate inside the neuromasts of zebrafish larvae in certain environmental conditions, highlighting the relevance of ionic homeostasis for hair cell function (Peloggia et al., 2021). On the other hand, mutations in two genes that play a role in ionic homeostasis, the chloride/bicarbonate exchanger slc4a1b and the transcription factor gcm2, provide some resistance to cisplatin- and aminoglycoside-induced damage to hair cells (Hailey et al., 2012; Stawicki et al., 2014).
The manner in which cisplatin enters the hair cells is not completely elucidated. It had been proposed to involve passive diffusion through the plasma membrane after substitution of the two chloride atoms by hydroxyl groups, and through two transporters at the plasma membrane, copper transport 1 (CRT1) and organic cation transporter 2 (OCT2) (Riddell and Lippard, 2018; Gentilin et al., 2019). Zebrafish hair cells lack Oct2 expression and show widespread expression of Crt1 (McDermott et al., 2007; Thomas et al., 2013). Using a fluorescently-labeled cisplatin analog (Rho-Pt) in zebrafish larvae, and a mix of pharmacological and genetic tools to inhibit mechanotransduction in hair cells, it was demonstrated that the mechanotransduction channel (MET) is required for cisplatin uptake and death of hair cells (Thomas et al., 2013). Recently, this has been proved the case also in mice; cochlear explants from mice lacking Tmie (one of the proteins that form the mechanotransduction channel) are partially resistant to cisplatin treatment and Oct2 inhibition further increase their protection (Li et al., 2022).
Upon entering the hair cell, cisplatin causes vacuolization, condensation of chromatin and, specially, degeneration of mitochondria (Giari et al., 2012). Mitochondria have a central role in ototoxin induced hair cell death, because of their energy-demanding activity and the production of reactive oxygen species (ROS) (Holmgren and Sheets, 2020). Treatment with mdiv-1, a proposed inhibitor of mitochondrial fission, a process indicative of mitochondrial dysfunction and excess production of ROS (Smith and Gallo, 2017), reduced cisplatin damage to hair cells in zebrafish (Vargo et al., 2017). Cisplatin treatment of zebrafish larvae labeled with fluorescent indicators of mitochondrial membrane potential or a genetically-encoded calcium indicator showed that cisplatin increased mitochondrial membrane hyperpolarization, calcium levels and ROS production in hair cells, leading to the activation of caspase 3 and apoptosis (Lee et al., 2022). These authors noted a heterogeneous response to cisplatin even among hair cells of the same neuromast, that could be related to the fact that not all hair cells are active simultaneously, and some are synaptically silent and would show less energy consumption (Zhang et al., 2018). Elevated ROS levels from dysfunctional mitochondria lead to an increase in protein nitration (Nakamura and Lipton, 2020), and cisplatin also induced this process in zebrafish hair cells, with a reduction in the levels of Lmo4, a transcriptional regulator (Shahab et al., 2021).
Besides the evidences linking cisplatin to mitochondria dysfunction, other pathways have been implicated in cisplatin-induced hair cell damage. Cisplatin activates Toll-like receptor 4 (TLR4) at the plasma membrane in a similar manner to the lipopolysaccharide (LPS) found in Gram-negative bacteria (Babolmorad et al., 2021). Although fish show a different response to LPS than mammals, and some teleost species have lost the Tlr4 gene, zebrafish has two ortholog genes, tlr4a and tlr4b (Sepulcre et al., 2009) and reducing their expression with morpholino antisense oligonucleotides attenuates cisplatin effects on hair cells (Babolmorad et al., 2021).
Aminoglycoside ototoxicity in zebrafish
The aminoglycoside family of antibiotic comprises a series of structurally related compounds of both natural and semisynthetic origin; to name a few: gentamicin, streptomycin, kanamycin and neomycin. They are used primarily to treat infections by Gram-negative bacteria, like those linked to cystic fibrosis, urinary tract or neonatal infections. Due to their cationic nature, aminoglycosides have poor gastrointestinal absorption, and they are administered intravenously or intramuscularly (Krause et al., 2016; Kim et al., 2022). Inside bacteria, aminoglycoside antibiotics bind to ribosomes and interfere with translation, leading to defective proteins and death of the bacteria (Hermann, 2007). Aminoglycoside antibiotics have been long known for their side effects, mainly nephrotoxicity and ototoxicity (Kim et al., 2022). While it has been proposed that aminoglycosides would enter renal cells through endocytosis mediated by megalin (Nagai and Takano, 2014), entry into hair cells depends on the activity of the mechanotransduction channel (Marcotti et al., 2005; Alharazneh et al., 2011). Nevertheless, megalin has been implicated in aminoglycoside transport into the cochlea as well (Kim and Ricci, 2022). Once inside the cell, aminoglycosides accumulate in lysosomes (Hashino et al., 1997) and cause mitochondrial dysfunction and production of ROS, leading to hair cell death (Böttger and Schacht, 2013), although the precise mechanism remains elusive.
Aminoglycoside ototoxic effects on hair cells of the zebrafish larvae were identified more than 20 years ago (Seiler and Nicolson, 1999; Williams and Holder, 2000), as well as the potential of this system to study its rapid hair cell regeneration after injury (Harris et al., 2003). Zebrafish hair cell susceptibility to neomycin depends on their developmental or maturation stage, being more sensitive at 5dpf than at 4dpf (Murakami et al., 2003; Santos et al., 2006). Another factor to be considered is the calcium concentration in the medium used to incubate the zebrafish larvae: elevated calcium concentrations protect to some extent from neomycin and gentamicin-induced hair cell damage (Coffin et al., 2009). Strikingly, and relevant for the reproducibility of toxicological research, there are differences in aminoglycoside sensitivity among wild-type zebrafish strains (Wiedenhoft et al., 2017).
Members of the aminoglycoside family of antibiotics differ in their effects on zebrafish hair cells, in terms of doses and time required to induce their death: neomycin is faster and more effective than gentamicin, streptomycin (Figures 1G,H) or kanamycin; also, gentamicin-induced hair cell death persisted after washout, unlike with neomycin (Owens et al., 2009). These data suggested that there may be multiple pathways to aminoglycoside-induced hair cell death. This difference between neomycin and gentamicin was also shown using pharmacological and genetic modulators of cell death (Coffin et al., 2013a; Coffin et al., 2013b).
One of the advantages of the use of zebrafish to study hair cells is their accessibility for observation. Fluorescently-labelled aminoglycosides have been used to show their entry in hair cells in vivo through endocytic and non-endocytic pathways, the involvement of the mechanotransduction channel, and accumulation in lysosomes (Wang and Steyger, 2009; Hailey et al., 2017). It is worth noting that labelled gentamicin, which is less rapid in killing hair cells, accumulates faster in lysosomes than labelled neomycin, which induces hair cell death more quickly (Hailey et al., 2017), suggesting aminoglycoside handling inside the cell has a role in their ototoxicity. Another interesting difference between neomycin and gentamicin modes of action in hair cells is found in their transport from the proposed point of entry, the tips of the sterocilia, to the cytoplasm. Mutations inactivating proteins involved in retrograde intraflagellar transport provide resistance towards neomycin-induced hair cell death, but not so much against gentamicin (Stawicki et al., 2016; Stawicki et al., 2019).
The role of mitochondria in hair cell death after aminoglycoside treatment has been extensively studied in zebrafish larvae. Morphological alterations in mitochondria appear already at low concentrations and short exposures to neomycin, even at subtoxical doses; loss of mitochondrial potential was observed at higher doses (Owens et al., 2007). A mutation on the mitochondrial protein Mpv17 impairs mitochondrial calcium levels and raises their ROS production; although these fish are viable and show no behavioral phenotype, their hair cells are more sensitive to aminoglycoside-induced damage (Holmgren and Sheets, 2021). Other studies used a collection of genetically encoded calcium indicators specifically expressed in hair cells to follow the changes in the concentration of this ion in vivo after aminoglycoside treatment; the data showed that neomycin caused a rise in the calcium levels in the mitochondria and the cytosol (Esterberg et al., 2013; Esterberg et al., 2014) and then, an increased oxidation and the production of ROS (Esterberg et al., 2016). Hair cell function is energy demanding, and the associated mitochondrial sustained activity to supply the required energy imposes a toll on hair cell susceptibility to aminoglycosides, altering mitochondrial turnover and repair (Pickett et al., 2018). One cellular process that is responsible for energy demand is synaptic transmission between hair cells and their afferent neurons, as genetic or pharmacological blocking of synaptic transmission in hair cells provides protection from aminoglycoside (both neomycin and gentamicin)-induced cell death, precisely by reducing mitochondrial oxidation and accumulation of ROS (Lukasz et al., 2022).
Screening for potential ototoxins
Beyond studying the mechanism behind well-known ototoxins, zebrafish larvae are useful to discover potential ototoxic effects of other molecules more rapidly than other animal studies assessing auditory end points. A systematic approach was taken to screen more than a 1000 compounds already approved by the American Food and Drug Administration (FDA) for medical use, and it found fourteen new potential ototoxins (Chiu et al., 2008). Nevertheless, this study had some limitations, as it tested only one relatively short exposure time (1 h) and some ototoxins need more time to cause detectable hair cell damage; precisely one of such is gentamicin, which failed to test positive in this screen. Automation of the inspection of stained hair cells would allow to refine such high throughput screening process to acquire more sensitivity (Philip et al., 2018).
More focused approaches targeting specific classes of drugs have been performed using the zebrafish larvae. In one of the most recent examples, the COVID-19 global pandemic has brought up the necessity of rapidly developing therapies to reduce its effects. This need for speed may lead to the use of drugs without the proper consideration of potential side effects, like ototoxicity. Some of the proposed therapies for COVID-19 (having real benefits or not) have been found to be toxic for zebrafish larvae hair cells, like hydroxichloroquine or ivermectin (Davis et al., 2020; Coffin et al., 2022). A screen of more than 500 natural products that can be found on dietary herbal supplements found nine new potential ototoxins (Neveux et al., 2017). Although cisplatin is an antitumoral drug well known for its ototoxic effects, another five drugs used to treat cancer have been shown to have similar ototoxic side-effects, as well as potential synergistic effects among them (Hirose et al., 2016). The issue of the potential synergistic effects of drugs being administered simultaneously is important, as many patients present comorbidities that need treatment. Glucocorticoids have been proposed to treat hearing loss (Meltser and Canlon, 2011). However, using zebrafish larvae it was demonstrated that previous exposure to glucocorticoids like cortisol or dexamethasone increased neomycin damage to hair cells (Hayward et al., 2019; Saettele et al., 2022). On a positive note, testing the ototoxicity of new semisynthetic aminoglycoside antibiotics has shown that etimicin exerts less damage to lateral line hair cells in zebrafish larvae than gentamicin (Shao et al., 2021), so this antibiotic could become an alternative for the ototoxic members of the family.
Another potential field of application is the detection of ototoxicity in environmental samples, contamination of which could be relevant for both human health and aquatic habitats. Heavy metals like copper, cadmium or gadolinium are known to be toxic to zebrafish hair cells (Hernández et al., 2006; Linbo et al., 2009; Rah et al., 2018; Schmid et al., 2020). Other chemical compounds that have been found to induce hair cell damage in zebrafish larvae include: pesticides (Nasri et al., 2016; Wu et al., 2021), bisphenol A, a well-known endocrine disruptor (Hayashi et al., 2015), tris (1-chloro-2-propyl) phosphate, a flame retardant (Xia et al., 2021) or urban waters (McIntyre et al., 2014; Young et al., 2018).
Screening for hair cell protectors
Testing for the ototoxicity of a new molecule is quite straightforward, either in zebrafish larvae or in mammalian inner ear. Searching for substances that protect hair cells from cisplatin or aminoglycoside-induced damage is a more complex matter. These molecules will have to fulfill a series of requirements: must have a protective effect without compromising anti-cancer or anti-microbial activities, be devoid of other toxic effects on their own, and be able to reach hair cells in the mammalian inner ear after systemic administration, eliminating the need for intratympanic administration.
Knowing that damage to hair cells is exerted through dysfunctional mitochondria and ROS production, a number of drugs among the antioxidant class and ROS scavengers have been proposed for the treatment or prevention of ototoxicity: D-methionine, N-acetylcysteine, selenium compound (Ebselen), alpha lipoic acid, coenzyme Q10; however, none of them have performed well in clinical trials (Hammill and Campbell, 2018). Therefore, the need for effective otoprotectants exists, and the hair cells of the zebrafish larvae are a useful model to speed up that goal (Ton and Parng, 2005). Some of the mentioned antioxidant compounds have been positively tested against ototoxins in zebrafish larvae, like glutathione, allopurinol, N-acetylcysteine, D-methionine (Ton and Parng, 2005), L-Serine (Monroe et al., 2021), a curcuminoid (Monroe et al., 2018), flavonoids, like naringin (Li et al., 2021) or quercetin (Lee et al., 2015; Hirose et al., 2016), a small peptide (Xiao et al., 2018), or ferrostatin (Hu et al., 2020).
The small size of zebrafish larvae, and the large number of them that can be obtained, have made possible unbiased systematic approaches to search for otoprotective agents among large collections of chemical compounds. Such studies are not practical using other model systems. Owens et al. (2008) screened 10960 compounds from the Diverset E library against neomycin toxicity. One of the two positive hits, initially called PROTO-1, was further chemically refined following a structure-activity relationship program and, after more than 300 modifications, ORC-13661 was obtained; this compound was orally administered and successful in protecting hair cells in rats treated with amikacin (Chowdhury et al., 2018). ORC-13661 has been shown to be a mechanotransduction channel blocker, protecting hair cells against both aminoglycosides and cisplatin-induced damage, unlike the original compound (Kitcher et al., 2019).
As the process to introduce a new drug into clinical practice can be very long, it is worthy to screen approved drugs for a novel use. Ou et al. (2007) screened a library of 1042 FDA-approved compounds against neomycin toxicity. Seven compounds were found to be effective in zebrafish hair cells, and two of them were tested on neomycin-treated mouse utricle preparations; one was toxic and the other one, tacrine, an anticholinergic agent, showed protective effects. Re-evaluation of the molecules that showed lesser degree of protection in this screen, revealed that eight of them shared a common quinoline scaffold (Ou et al., 2012). Quinoxaline, another quinoline derivative, was found to provide protection against cisplatin, but only partially against aminoglycosides (Rocha-Sanchez et al., 2018). An additional set of 68 quinoxaline derivatives was tested, finding one of them effective against both cisplatin and aminoglycosides in zebrafish larvae and neonatal mouse cochlear explants (Zallocchi et al., 2021). This molecule prevented the ototoxic effects by inhibiting NF-κB, upstream of ROS production (Zallocchi et al., 2021).
We have mentioned earlier that there are differences in the toxic effects and death pathways induced of different aminoglycoside antibiotics (Coffin et al., 2013b). Thus, a molecule found to protect from one aminoglycoside may not be effective against another one. Vlasits et al. approached this problem by screening a library of 640 FDA-approved natural compounds against three aminoglycosides: neomycin, gentamicin and kanamycin, and also cisplatin (Vlasits et al., 2012). Ten of them protected from at least two of the ototoxins, but only two of those were effective against aminoglycosides and cisplatin: bezamil, a diuretic, and paroxetine, a selective serotonin reuptake inhibitor. Recent not yet per-reviewed data point to a role of environmental serotonin in hair cell function (Desban et al., 2022), and several serotonin receptors are expressed in the mammalian inner ear (gEAR Expression Analysis Resource, Hertzano and Mahurkar, 2022).
A similar approach, using neomycin and gentamicin, was used to screen a library of 502 natural products (Kruger et al., 2016). They found four otoprotective molecules that shared a common structure, being derivatives of bisbenzylisoquinoline (Kruger et al., 2016), and nine further derivatives of one of them, berbamine, offered protection against both types of aminoglycoside (Hudson et al., 2020).
Using a more comprehensive strategy using zebrafish larvae and mouse cochlear cultures, Kenyon et al. screened the 10240 compounds from the Life Chemicals Diversity Set. First against neomycin and then against gentamicin in zebrafish larvae, obtaining 64 otoprotective molecules, of which 20 were effective protecting mouse cochlear cultures and only eight of them did not damage hair bundles (Kenyon et al., 2021). What these eight molecules had in common was the ability to block the MET channel, the proposed entry pathway into the hair cell for aminoglycosides (Hailey et al., 2017). In fact, the same group had performed a previous screen focused on a set of 160 ion channel modulators and found 13 positive hits active in zebrafish larvae and mouse cochlear cultures, six of which are MET channel blockers (Kenyon et al., 2017). Therefore, temporary blocking the MET channel to prevent the entry of aminoglycosides on hair cells seems a promising strategy to prevent their ototoxic effects during the treatment course, in order to limit the expected loss of hearing and balance.
Fewer studies have focused on identifying molecules that can protect from cisplatin-induced hair cell damage in zebrafish larvae. The screening of the 1280 compounds from the Sigma LOPAC library against cisplatin toxic effects on both hair cells and pronephros found 22 molecules effective on both assays, and two of the more active ones were members of the dopaminergic pathway, dopamine and L-mimosine, an inhibitor of dopamine β-hydroxylase structurally related to dopamine (Wertman et al., 2020). Interestingly, the known antioxidant N-acetylcisteine was found to be otoprotective, but not nephroprotective (Wertman et al., 2020).
The rise of zebrafish as an animal model has been driven by the ability to perform genetic screens looking for genes affecting embryonic development (Driever et al., 1996; Haffter et al., 1996), including the lateral line (Whitfield et al., 1996). This approach was also taken in search for genes than could prevent aminoglycoside-induced hair cell damage (Owens et al., 2008; Stawicki et al., 2016). The first of these screens yielded five recessive mutations that conferred resistance to neomycin-induced damage and two of the genes were identified: cc2d2a, a cilia protein (Owens et al., 2008), and gcm2, a transcription factor important for the development of ionocytes in the skin of teleosts, mentioned previously (Stawicki et al., 2014). The second screen resulted in the identification of six additional cilia proteins, revealing different roles for intraflagellar transport proteins and transition zone proteins in aminoglycoside-induced hair cell damage (Stawicki et al., 2016).
Limitations on the use of zebrafish for hair cell toxicology
Studies conducted over the past 20 years have shown that zebrafish larvae are a useful model for hair cell toxicology, due to the easy access to their hair cells to study in vivo ototoxin mechanisms, and their small overall size, making possible high throughput screens. However, we have to consider the limitations of the model as well. First, lateral line hair cells are homologous to mammalian inner ear hair cells, but there are no known differences among them as in mammals, where we can distinguish inner and outer hair cells in the cochlea, or type I and type II hair cells in the vestibular system. These different hair cells have been shown to present distinct sensitivities to ototoxins, even between regions of the same epithelia (Forge and Schacht, 2000; Hirvonen et al., 2005; Fettiplace and Nam, 2019). Second, lateral line hair cells are exposed to the environment and drugs can readily reach them. Mammalian inner ear hair cells are isolated from the rest of the body by the blood-labyrinth barrier (Glueckert et al., 2018). Current therapies to prevent hair cell loss are based on invasive methods to introduce the drug into the inner ear (Patel et al., 2019). Related to this fact, otoprotective drug doses effective on zebrafish lateral line hair cells could be different to the levels of that drug found in plasma when used in mammals. Third, lateral line hair cells have the ability to regenerate rapidly after the initial insult, preventing the study of ototoxin exposures beyond 24 h, while ototoxicity in patients takes longer periods to develop.
Despite these limitations, research on ototoxicity using zebrafish hair cells may offer new insights into the underlying mechanisms that could be translated into a way to prevent it. On the other hand, it is hoped that at least one of the otoprotective molecules that have been discovered using zebrafish lateral line hair cells reaches the stage when it could be used in clinical settings to preserve the hearing and balance of patients, making an impact in their lives. The recent elucidation of the structure of the main component of the mechanotransduction channel will surely bring a new wave of focused screens (Jeong et al., 2022), as well as the implementation of machine and deep learning to discover new ototoxins (Zhou et al., 2014; Huang et al., 2021) or new otoprotective agents to be tested rapidly on zebrafish larvae.
Author contributions
AB-G and JL wrote the manuscript. All authors contributed to the article and approved the submitted version.
Funding
Work in the lab of AB-G and JL is funded by MCInn grants RTI2018-096452-B-100 and PID2021-124678OB-100 (MCIN/AEI/10.13039/501100011033/FEDER, EU), and by grant SGR 2017 621 (Agència de Gestió d’Ajuts Universitaris i de Recerca, Generalitat de Catalunya).
Acknowledgments
AB-G is a Serra Húnter fellow. We thank Benjamin Torrejón from the Centres Científics i Tecnològics de la Universitat de Barcelona (CCiTUB) at the Campus de Bellvitge for his help with laser scanning microscopy.
Conflict of interest
The authors declare that the research was conducted in the absence of any commercial or financial relationships that could be construed as a potential conflict of interest.
Publisher’s note
All claims expressed in this article are solely those of the authors and do not necessarily represent those of their affiliated organizations, or those of the publisher, the editors and the reviewers. Any product that may be evaluated in this article, or claim that may be made by its manufacturer, is not guaranteed or endorsed by the publisher.
References
Adatto, I., Lawrence, C., Thompson, M., and Zon, L. I. (2011). A new system for the rapid collection of large numbers of developmentally staged zebrafish embryos. PLoS ONE 6, e21715. doi:10.1371/journal.pone.0021715
Alharazneh, A., Luk, L., Huth, M., Monfared, A., Steyger, P. S., Cheng, A. G., et al. (2011). Functional hair cell mechanotransducer channels are required for aminoglycoside ototoxicity. PloS ONE 6, e22347. doi:10.1371/journal.pone.0022347
Alsina, B., and Whitfield, T. T. (2017). Sculpting the labyrinth: Morphogenesis of the developing inner ear. Semin. Cell Dev. Biol. 65, 47–59. doi:10.1016/j.semcdb.2016.09.015
Babolmorad, G., Latif, A., Domingo, I. K., Pollock, N. M., Delyea, C., Rieger, A. M., et al. (2021). Toll-like receptor 4 is activated by platinum and contributes to cisplatin-induced ototoxicity. EMBO Rep. 22, e51280. doi:10.15252/embr.202051280
Baxendale, S., and Whitfield, T. T. (2016). Methods to study the development, anatomy, and function of the zebrafish inner ear across the life course. Methods Cell Biol. 134, 165–209. doi:10.1016/bs.mcb.2016.02.007
Böttger, E. C., and Schacht, J. (2013). The mitochondrion: A perpetrator of acquired hearing loss. Hear. Res. 303, 12–19. doi:10.1016/j.heares.2013.01.006
Buck, L. M. J., Winter, M. J., Redfern, W. S., and Whitfield, T. T. (2012). Ototoxin-induced cellular damage in neuromasts disrupts lateral line function in larval zebrafish. Hear. Res. 284, 67–81. doi:10.1016/j.heares.2011.12.001
Callejo, A., Sedó-Cabezón, L., Juan, I. D., and Llorens, J. (2015). Cisplatin-induced ototoxicity: Effects, mechanisms and protection strategies. Toxics 3, 268–293. doi:10.3390/toxics3030268
Cassar, S., Adatto, I., Freeman, J. L., Gamse, J. T., Iturria, I., Lawrence, C., et al. (2020). Use of zebrafish in drug discovery toxicology. Chem. Res. Toxicol. 33, 95–118. doi:10.1021/acs.chemrestox.9b00335
Chiu, L. L., Cunningham, L. L., Raible, D. W., Rubel, E. W., and Ou, H. C. (2008). Using the zebrafish lateral line to screen for ototoxicity. J. Assoc. Res. Otolaryngol. 9, 178–190. doi:10.1007/s10162-008-0118-y
Chowdhury, S., Owens, K. N., Herr, R. J., Jiang, Q., Chen, X., Johnson, G., et al. (2018). Phenotypic optimization of urea-thiophene carboxamides to yield potent, well tolerated, and orally active protective agents against aminoglycoside-induced hearing loss. J. Med. Chem. 61, 84–97. doi:10.1021/acs.jmedchem.7b00932
Coffin, A. B., Boney, R., Hill, J., Tian, C., and Steyger, P. S. (2021). Detecting novel ototoxins and potentiation of ototoxicity by disease settings. Front. Neurol. 12, 725566. doi:10.3389/fneur.2021.725566
Coffin, A. B., Dale, E., Doppenberg, E., Fearington, F., Hayward, T., Hill, J., et al. (2022). Putative COVID-19 therapies imatinib, lopinavir, ritonavir, and ivermectin cause hair cell damage: A targeted screen in the zebrafish lateral line. Front. Cell. Neurosci. 16, 941031. doi:10.3389/fncel.2022.941031
Coffin, A. B., Kelley, M., Manley, G. A., and Popper, A. A. (2004). “Evolution of sensory hair cells,” in Evolution of the vertebrate auditory system (Berlin, Germany: Springer Science+Bussiness Media), 55. doi:10.1007/978-1-4419-8957-4
Coffin, A. B., Reinhart, K. E., Owens, K. N., Raible, D. W., and Rubel, E. W. (2009). Extracellular divalent cations modulate aminoglycoside-induced hair cell death in the zebrafish lateral line. Hear. Res. 253, 42–51. doi:10.1016/j.heares.2009.03.004
Coffin, A. B., Rubel, E. W., and Raible, D. W. (2013a). Bax, Bcl2, and p53 differentially regulate neomycin- and gentamicin-induced hair cell death in the zebrafish lateral line. J. Assoc. Res. Otolaryngol. 14, 645–659. doi:10.1007/s10162-013-0404-1
Coffin, A. B., Williamson, K. L., Mamiya, A., Raible, D. W., and Rubel, E. W. (2013b). Profiling drug-induced cell death pathways in the zebrafish lateral line. Apoptosis 18, 393–408. doi:10.1007/s10495-013-0816-8
Cruz, R. M., Lambert, P. R., and Rubel, E. W. (1987). Light microscopic evidence of hair cell regeneration after gentamicin toxicity in chick cochlea. Arch. Otolaryngol. Head. Neck Surg. 113, 1058–1062. doi:10.1001/archotol.1987.01860100036017
Cunningham, L. L. (2006). The adult mouse utricle as an in vitro preparation for studies of ototoxic-drug-induced sensory hair cell death. Brain Res. 1091, 277–281. doi:10.1016/j.brainres.2006.01.128
Davis, S. N., Wu, P., Camci, E. D., Simon, J. A., Rubel, E. W., and Raible, D. W. (2020). Chloroquine kills hair cells in zebrafish lateral line and murine cochlear cultures: Implications for ototoxicity. Hear. Res. 395, 108019. doi:10.1016/j.heares.2020.108019
De Faveri, F., Marcotti, W., and Ceriani, F. (2021). Sensory adaptation at ribbon synapses in the zebrafish lateral line. J. Physiology 599, 3677–3696. doi:10.1113/JP281646
Desban, L., Roussel, J., Mirat, O., Lejeune, F.-X., Keiser, L., Michalski, N., et al. (2022). Lateral line hair cells integrate mechanical and chemical cues to orient navigation. bioRxiv. doi:10.1101/2022.08.31.505989
Dillard, L. K., Lopez-Perez, L., Martinez, R. X., Fullerton, A. M., Chadha, S., and McMahon, C. M. (2022). Global burden of ototoxic hearing loss associated with platinum-based cancer treatment: A systematic review and meta-analysis. Cancer Epidemiol. 79, 102203. doi:10.1016/j.canep.2022.102203
Driever, W., Solnica-Krezel, L., Schier, A. F., Neuhauss, S. C., Malicki, J., Stemple, D. L., et al. (1996). A genetic screen for mutations affecting embryogenesis in zebrafish. Development 123, 37–46. doi:10.1242/dev.123.1.37
Esterberg, R., Hailey, D. W., Coffin, A. B., Raible, D. W., and Rubel, E. W. (2013). Disruption of intracellular calcium regulation is integral to aminoglycoside-induced hair cell death. J. Neurosci. 33, 7513–7525. doi:10.1523/JNEUROSCI.4559-12.2013
Esterberg, R., Hailey, D. W., Rubel, E. W., and Raible, D. W. (2014). ER-mitochondrial calcium flow underlies vulnerability of mechanosensory hair cells to damage. J. Neurosci. 34, 9703–9719. doi:10.1523/JNEUROSCI.0281-14.2014
Esterberg, R., Linbo, T., Pickett, S. B., Wu, P., Ou, H. C., Rubel, E. W., et al. (2016). Mitochondrial calcium uptake underlies ROS generation during aminoglycoside-induced hair cell death. J. Clin. Invest. 126, 3556–3566. doi:10.1172/JCI84939
Fábelová, L., Loffredo, C. A., Klánová, J., Hilscherová, K., Horvat, M., Tihányi, J., et al. (2019). Environmental ototoxicants, a potential new class of chemical stressors. Environ. Res. 171, 378–394. doi:10.1016/j.envres.2019.01.042
Fernandez, K., Wafa, T., Fitzgerald, T. S., and Cunningham, L. L. (2019). An optimized, clinically relevant mouse model of cisplatin-induced ototoxicity. Hear. Res. 375, 66–74. doi:10.1016/j.heares.2019.02.006
Fettiplace, R., and Nam, J.-H. (2019). Tonotopy in calcium homeostasis and vulnerability of cochlear hair cells. Hear. Res. 376, 11–21. doi:10.1016/j.heares.2018.11.002
Forge, A., and Schacht, J. (2000). Aminoglycoside antibiotics. Audiol. Neurootol. 5, 3–22. doi:10.1159/000013861
Gentilin, E., Simoni, E., Candito, M., Cazzador, D., and Astolfi, L. (2019). Cisplatin-induced ototoxicity: Updates on molecular targets. Trends Mol. Med. 25, 1123–1132. doi:10.1016/j.molmed.2019.08.002
Ghysen, A., and Dambly-Chaudière, C. (2007). The lateral line microcosmos. Genes Dev. 21, 2118–2130. doi:10.1101/gad.1568407
Giari, L., Dezfuli, B. S., Astolfi, L., and Martini, A. (2012). Ultrastructural effects of cisplatin on the inner ear and lateral line system of zebrafish (Danio rerio) larvae. J. Appl. Toxicol. 32, 293–299. doi:10.1002/jat.1691
Glueckert, R., Johnson Chacko, L., Rask-Andersen, H., Liu, W., Handschuh, S., and Schrott-Fischer, A. (2018). Anatomical basis of drug delivery to the inner ear. Hear. Res. 368, 10–27. doi:10.1016/j.heares.2018.06.017
Haas, P., and Gilmour, D. (2006). Chemokine signaling mediates self-organizing tissue migration in the zebrafish lateral line. Dev. Cell 10, 673–680. doi:10.1016/j.devcel.2006.02.019
Haffter, P., Granato, M., Brand, M., Mullins, M. C., Hammerschmidt, M., Kane, D. A., et al. (1996). The identification of genes with unique and essential functions in the development of the zebrafish, Danio rerio. Development 123, 1–36. doi:10.1242/dev.123.1.1
Hailey, D. W., Esterberg, R., Linbo, T. H., Rubel, E. W., and Raible, D. W. (2017). Fluorescent aminoglycosides reveal intracellular trafficking routes in mechanosensory hair cells. J. Clin. Invest. 127, 472–486. doi:10.1172/JCI85052
Hailey, D. W., Roberts, B., Owens, K. N., Stewart, A. K., Linbo, T., Pujol, R., et al. (2012). Loss of Slc4a1b chloride/bicarbonate exchanger function protects mechanosensory hair cells from aminoglycoside damage in the zebrafish mutant persephone. PLoS Genet. 8, e1002971. doi:10.1371/journal.pgen.1002971
Hammill, T. L., and Campbell, K. C. (2018). Protection for medication-induced hearing loss: The state of the science. Int. J. Audiol. 57, S67–S75. doi:10.1080/14992027.2018.1455114
Harris, J. A., Cheng, A. G., Cunningham, L. L., MacDonald, G., Raible, D. W., and Rubel, E. W. (2003). Neomycin-induced hair cell death and rapid regeneration in the lateral line of zebrafish (Danio rerio). J. Assoc. Res. Otolaryngol. 4, 219–234. doi:10.1007/s10162-002-3022-x
Hashino, E., Shero, M., and Salvi, R. J. (1997). Lysosomal targeting and accumulation of aminoglycoside antibiotics in sensory hair cells. Brain Res. 777, 75–85. doi:10.1016/s0006-8993(97)00977-3
Hayashi, L., Sheth, M., Young, A., Kruger, M., Wayman, G. A., and Coffin, A. B. (2015). The effect of the aquatic contaminants bisphenol-A and PCB-95 on the zebrafish lateral line. Neurotoxicology 46, 125–136. doi:10.1016/j.neuro.2014.12.010
Hayward, T., Young, A., Jiang, A., Crespi, E. J., and Coffin, A. B. (2019). Glucococorticoid receptor activation exacerbates aminoglycoside-induced damage to the zebrafish lateral line. Hear. Res. 377, 12–23. doi:10.1016/j.heares.2019.03.002
Hentschel, D. M., Park, K. M., Cilenti, L., Zervos, A. S., Drummond, I., and Bonventre, J. V. (2005). Acute renal failure in zebrafish: A novel system to study a complex disease. Am. J. Physiol. Ren. Physiol. 288, F923–F929. doi:10.1152/ajprenal.00386.2004
Hermann, T. (2007). Aminoglycoside antibiotics: Old drugs and new therapeutic approaches. Cell. Mol. Life Sci. 64, 1841–1852. doi:10.1007/s00018-007-7034-x
Hernández, P. P., Moreno, V., Olivari, F. A., and Allende, M. L. (2006). Sub-lethal concentrations of waterborne copper are toxic to lateral line neuromasts in zebrafish (Danio rerio). Hear. Res. 213, 1–10. doi:10.1016/j.heares.2005.10.015
Hertzano, R., and Mahurkar, A. (2022). Advancing discovery in hearing research via biologist-friendly access to multi-omic data. Hum. Genet. 141, 319–322. doi:10.1007/s00439-022-02445-w
Hirose, Y., Sugahara, K., Kanagawa, E., Takemoto, Y., Hashimoto, M., and Yamashita, H. (2016). Quercetin protects against hair cell loss in the zebrafish lateral line and Guinea pig cochlea. Hear. Res. 342, 80–85. doi:10.1016/j.heares.2016.10.001
Hirvonen, T. P., Minor, L. B., Hullar, T. E., and Carey, J. P. (2005). Effects of intratympanic gentamicin on vestibular afferents and hair cells in the chinchilla. J. Neurophysiol. 93, 643–655. doi:10.1152/jn.00160.2004
Holmgren, M., and Sheets, L. (2021). Influence of mpv17 on hair-cell mitochondrial homeostasis, synapse integrity, and vulnerability to damage in the zebrafish lateral line. Front. Cell. Neurosci. 15, 693375. doi:10.3389/fncel.2021.693375
Holmgren, M., and Sheets, L. (2020). Using the zebrafish lateral line to understand the roles of mitochondria in sensorineural hearing loss. Front. Cell Dev. Biol. 8, 628712. doi:10.3389/fcell.2020.628712
Holtzman, N. G., Iovine, M. K., Liang, J. O., and Morris, J. (2016). Learning to fish with genetics: A primer on the vertebrate model Danio rerio. Genetics 203, 1069–1089. doi:10.1534/genetics.116.190843
Hu, B., Liu, Y., Chen, X., Zhao, J., Han, J., Dong, H., et al. (2020). Ferrostatin-1 protects auditory hair cells from cisplatin-induced ototoxicity in vitro and in vivo. Biochem. Biophys. Res. Commun. 533, 1442–1448. doi:10.1016/j.bbrc.2020.10.019
Huang, X., Tang, F., Hua, Y., and Li, X. (2021). In silico prediction of drug-induced ototoxicity using machine learning and deep learning methods. Chem. Biol. Drug Des. 98, 248–257. doi:10.1111/cbdd.13894
Hudson, A. M., Lockard, G. M., Namjoshi, O. A., Wilson, J. W., Kindt, K. S., Blough, B. E., et al. (2020). Berbamine analogs exhibit differential protective effects from aminoglycoside-induced hair cell death. Front. Cell. Neurosci. 14, 234. doi:10.3389/fncel.2020.00234
Hung, G.-Y., Wu, C.-L., Chou, Y.-L., Chien, C.-T., Horng, J.-L., and Lin, L.-Y. (2019). Cisplatin exposure impairs ionocytes and hair cells in the skin of zebrafish embryos. Aquat. Toxicol. 209, 168–177. doi:10.1016/j.aquatox.2019.02.006
Jeong, H., Clark, S., Goehring, A., Dehghani-Ghahnaviyeh, S., Rasouli, A., Tajkhorshid, E., et al. (2022). Structures of the TMC-1 complex illuminate mechanosensory transduction. Nature 610, 796–803. doi:10.1038/s41586-022-05314-8
Kalinec, G. M., Webster, P., Lim, D. J., and Kalinec, F. (2003). A cochlear cell line as an in vitro system for drug ototoxicity screening. Audiol. Neurootol. 8, 177–189. doi:10.1159/000071059
Kenyon, E. J., Kirkwood, N. K., Kitcher, S. R., Goodyear, R. J., Derudas, M., Cantillon, D. M., et al. (2021). Identification of ion-channel modulators that protect against aminoglycoside-induced hair cell death. JCI Insight 6, e96773. doi:10.1172/jci.insight.96773
Kenyon, E. J., Kirkwood, N. K., Kitcher, S. R., O’Reilly, M., Derudas, M., Cantillon, D. M., et al. (2017). Identification of ion-channel modulators that protect against aminoglycoside-induced hair cell death. JCI Insight 2, 96773. doi:10.1172/jci.insight.96773
Kim, J., Hemachandran, S., Cheng, A. G., and Ricci, A. J. (2022). Identifying targets to prevent aminoglycoside ototoxicity. Mol. Cell. Neurosci. 120, 103722. doi:10.1016/j.mcn.2022.103722
Kim, J., and Ricci, A. J. (2022). In vivo real-time imaging reveals megalin as the aminoglycoside gentamicin transporter into cochlea whose inhibition is otoprotective. Proc. Natl. Acad. Sci. U. S. A. 119, e2117946119. doi:10.1073/pnas.2117946119
Kim, M. J., Choi, J., Kim, N., and Han, G. C. (2014). Behavioral changes of zebrafish according to cisplatin-induced toxicity of the balance system. Hum. Exp. Toxicol. 33, 1167–1175. doi:10.1177/0960327114521046
Kitcher, S. R., Kirkwood, N. K., Camci, E. D., Wu, P., Gibson, R. M., Redila, V. A., et al. (2019). ORC-13661 protects sensory hair cells from aminoglycoside and cisplatin ototoxicity. JCI Insight 4, e126764. doi:10.1172/jci.insight.126764
Krause, K. M., Serio, A. W., Kane, T. R., and Connolly, L. E. (2016). Aminoglycosides: An overview. Cold Spring Harb. Perspect. Med. 6, a027029. doi:10.1101/cshperspect.a027029
Kruger, M., Boney, R., Ordoobadi, A. J., Sommers, T. F., Trapani, J. G., and Coffin, A. B. (2016). Natural bizbenzoquinoline derivatives protect zebrafish lateral line sensory hair cells from aminoglycoside toxicity. Front. Cell. Neurosci. 10, 83. doi:10.3389/fncel.2016.00083
Lee, D. S., Schrader, A., Warchol, M., and Sheets, L. (2022). Cisplatin exposure acutely disrupts mitochondrial bioenergetics in the zebrafish lateral-line organ. Hear. Res. 2022, 108513. doi:10.1016/j.heares.2022.108513
Lee, M. P., and Waldhaus, J. (2022). In vitro and in vivo models: What have we learnt about inner ear regeneration and treatment for hearing loss? Mol. Cell. Neurosci. 120, 103736. doi:10.1016/j.mcn.2022.103736
Lee, S. K., Oh, K. H., Chung, A. Y., Park, H. C., Lee, S. H., Kwon, S. Y., et al. (2015). Protective role of quercetin against cisplatin-induced hair cell damage in zebrafish embryos. Hum. Exp. Toxicol. 34, 1043–1052. doi:10.1177/0960327114567766
Li, J., Liu, C., Kaefer, S., Youssef, M., and Zhao, B. (2022). The mechanotransduction channel and organic cation transporter are critical for cisplatin ototoxicity in murine hair cells. Front. Mol. Neurosci. 15, 835448. doi:10.3389/fnmol.2022.835448
Li, M., Liu, J., Liu, D., Duan, X., Zhang, Q., Wang, D., et al. (2021). Naringin attenuates cisplatin- and aminoglycoside-induced hair cell injury in the zebrafish lateral line via multiple pathways. J. Cell. Mol. Med. 25, 975–989. doi:10.1111/jcmm.16158
Liberman, M. C., and Kujawa, S. G. (2017). Cochlear synaptopathy in acquired sensorineural hearing loss: Manifestations and mechanisms. Hear. Res. 349, 138–147. doi:10.1016/j.heares.2017.01.003
Lim, H. W., Pak, K., Ryan, A. F., and Kurabi, A. (2018). Screening mammalian cochlear hair cells to identify critical processes in aminoglycoside-mediated damage. Front. Cell. Neurosci. 12, 179. doi:10.3389/fncel.2018.00179
Linbo, T. L., Baldwin, D. H., McIntyre, J. K., and Scholz, N. L. (2009). Effects of water hardness, alkalinity, and dissolved organic carbon on the toxicity of copper to the lateral line of developing fish. Environ. Toxicol. Chem. 28, 1455–1461. doi:10.1897/08-283.1
Lukasz, D., Beirl, A., and Kindt, K. (2022). Chronic neurotransmission increases the susceptibility of lateral-line hair cells to ototoxic insults. eLife 11, e77775. doi:10.7554/eLife.77775
MacRae, C. A., and Peterson, R. T. (2022). Zebrafish as a mainstream model for in vivo systems pharmacology and toxicology. Annu. Rev. Pharmacol. Toxicol. doi:10.1146/annurev-pharmtox-051421-105617
Marcotti, W., van Netten, S. M., and Kros, C. J. (2005). The aminoglycoside antibiotic dihydrostreptomycin rapidly enters mouse outer hair cells through the mechano-electrical transducer channels. J. Physiol. 567, 505–521. doi:10.1113/jphysiol.2005.085951
McDermott, B. M., Asai, Y., Baucom, J. M., Jani, S. D., Castellanos, Y., Gomez, G., et al. (2010). Transgenic labeling of hair cells in the zebrafish acousticolateralis system. Gene Expr. Patterns 10, 113–118. doi:10.1016/j.gep.2010.01.001
McDermott, B. M., Baucom, J. M., and Hudspeth, A. J. (2007). Analysis and functional evaluation of the hair-cell transcriptome. Proc. Natl. Acad. Sci. U. S. A. 104, 11820–11825. doi:10.1073/pnas.0704476104
McHenry, M. J., Feitl, K. E., Strother, J. A., and Van Trump, W. J. (2009). Larval zebrafish rapidly sense the water flow of a predator’s strike. Biol. Lett. 5, 477–479. doi:10.1098/rsbl.2009.0048
McIntyre, J. K., Davis, J. W., Incardona, J. P., Stark, J. D., Anulacion, B. F., and Scholz, N. L. (2014). Zebrafish and clean water technology: Assessing soil bioretention as a protective treatment for toxic urban runoff. Sci. Total Environ. 501, 173–180. doi:10.1016/j.scitotenv.2014.08.066
Meltser, I., and Canlon, B. (2011). Protecting the auditory system with glucocorticoids. Hear. Res. 281, 47–55. doi:10.1016/j.heares.2011.06.003
Monroe, J. D., Millay, M. H., Patty, B. G., and Smith, M. E. (2018). The curcuminoid, EF-24, reduces cisplatin-mediated reactive oxygen species in zebrafish inner ear auditory and vestibular tissues. J. Clin. Neurosci. 57, 152–156. doi:10.1016/j.jocn.2018.09.002
Monroe, J. D., Moolani, S. A., Irihamye, E. N., Johnston, A. M., and Smith, M. E. (2021). Effects of L-Serine against cisplatin-mediated reactive oxygen species generation in zebrafish vestibular tissue culture and HEI-OC1 auditory hybridoma cells. Neurotox. Res. 39, 36–41. doi:10.1007/s12640-020-00188-y
Moser, T., Grabner, C. P., and Schmitz, F. (2020). Sensory processing at ribbon synapses in the retina and the cochlea. Physiol. Rev. 100, 103–144. doi:10.1152/physrev.00026.2018
Moulder, G. L. (2016). Hatching fish—When should animal tracking begin? Zebrafish 13, S150–S152. doi:10.1089/zeb.2016.1243
Murakami, S. L., Cunningham, L. L., Werner, L. A., Bauer, E., Pujol, R., Raible, D. W., et al. (2003). Developmental differences in susceptibility to neomycin-induced hair cell death in the lateral line neuromasts of zebrafish (Danio rerio). Hear. Res. 186, 47–56. doi:10.1016/s0378-5955(03)00259-4
Murillo-Cuesta, S., Contreras, J., Cediel, R., and Varela-Nieto, I. (2010). Comparison of different aminoglycoside antibiotic treatments to refine ototoxicity studies in adult mice. Lab. Anim. 44, 124–131. doi:10.1258/la.2009.009046
Nagai, J., and Takano, M. (2014). Entry of aminoglycosides into renal tubular epithelial cells via endocytosis-dependent and endocytosis-independent pathways. Biochem. Pharmacol. 90, 331–337. doi:10.1016/j.bcp.2014.05.018
Nakamura, T., and Lipton, S. A. (2020). Nitric oxide-dependent protein post-translational modifications impair mitochondrial function and metabolism to contribute to neurodegenerative diseases. Antioxid. Redox Signal. 32, 817–833. doi:10.1089/ars.2019.7916
Nasri, A., Valverde, A. J., Roche, D. B., Desrumaux, C., Clair, P., Beyrem, H., et al. (2016). Neurotoxicity of a biopesticide analog on zebrafish larvae at nanomolar concentrations. Int. J. Mol. Sci. 17, E2137. doi:10.3390/ijms17122137
Neveux, S., Smith, N. K., Roche, A., Blough, B. E., Pathmasiri, W., and Coffin, A. B. (2017). Natural compounds as occult ototoxins? Ginkgo biloba flavonoids moderately damage lateral line hair cells. J. Assoc. Res. Otolaryngol. 18, 275–289. doi:10.1007/s10162-016-0604-6
Newton, K. C., Kacev, D., Nilsson, S. R. O., Saettele, A. L., Golden, S. A., and Sheets, L. (2022). Lateral line ablation by ototoxic compounds results in distinct rheotaxis profiles in larval zebrafish. bioRxiv 15, 468723. doi:10.1101/2021.11.15.468723
Nicolson, T., Rüsch, A., Friedrich, R. W., Granato, M., Ruppersberg, J. P., Nüsslein-Volhard, C., et al. (1998). Genetic analysis of vertebrate sensory hair cell mechanosensation: The zebrafish circler mutants. Neuron 20, 271–283. doi:10.1016/s0896-6273(00)80455-9
Niihori, M., Platto, T., Igarashi, S., Hurbon, A., Dunn, A. M., Tran, P., et al. (2015). Zebrafish swimming behavior as a biomarker for ototoxicity-induced hair cell damage: A high-throughput drug development platform targeting hearing loss. Transl. Res. 166, 440–450. doi:10.1016/j.trsl.2015.05.002
Nist-Lund, C., Kim, J., and Koehler, K. R. (2022). Advancements in inner ear development, regeneration, and repair through otic organoids. Curr. Opin. Genet. Dev. 76, 101954. doi:10.1016/j.gde.2022.101954
Ó Maoiléidigh, D., and Ricci, A. J. (2019). A bundle of mechanisms: Inner-ear hair-cell mechanotransduction. Trends Neurosci. 42, 221–236. doi:10.1016/j.tins.2018.12.006
Obholzer, N., Wolfson, S., Trapani, J. G., Mo, W., Nechiporuk, A., Busch-Nentwich, E., et al. (2008). Vesicular glutamate transporter 3 is required for synaptic transmission in zebrafish hair cells. J. Neurosci. 28, 2110–2118. doi:10.1523/JNEUROSCI.5230-07.2008
Ogier, J. M., Burt, R. A., Drury, H. R., Lim, R., and Nayagam, B. A. (2019). Organotypic culture of neonatal murine inner ear explants. Front. Cell. Neurosci. 13, 170. doi:10.3389/fncel.2019.00170
Olt, J., Johnson, S. L., and Marcotti, W. (2014). In vivo and in vitro biophysical properties of hair cells from the lateral line and inner ear of developing and adult zebrafish. J. Physiol. 592, 2041–2058. doi:10.1113/jphysiol.2013.265108
Ou, H. C., Keating, S., Wu, P., Simon, J. A., Raible, D. W., and Rubel, E. W. (2012). Quinoline ring derivatives protect against aminoglycoside-induced hair cell death in the zebrafish lateral line. J. Assoc. Res. Otolaryngol. 13, 759–770. doi:10.1007/s10162-012-0353-0
Ou, H. C., Lin, V., and Rubel, E. W. (2013). In-bone” utricle cultures-a simplified, atraumatic technique for in situ cultures of the adult mouse (Mus musculus) utricle. Otol. Neurotol. 34, 353–359. doi:10.1097/mao.0b013e31827ca330
Ou, H. C., Raible, D. W., and Rubel, E. W. (2007). Cisplatin-induced hair cell loss in zebrafish (Danio rerio) lateral line. Hear. Res. 233, 46–53. doi:10.1016/j.heares.2007.07.003
Owens, K. N., Coffin, A. B., Hong, L. S., Bennett, K. O., Rubel, E. W., and Raible, D. W. (2009). Response of mechanosensory hair cells of the zebrafish lateral line to aminoglycosides reveals distinct cell death pathways. Hear. Res. 253, 32–41. doi:10.1016/j.heares.2009.03.001
Owens, K. N., Cunningham, D. E., MacDonald, G., Rubel, E. W., Raible, D. W., and Pujol, R. (2007). Ultrastructural analysis of aminoglycoside-induced hair cell death in the zebrafish lateral line reveals an early mitochondrial response. J. Comp. Neurol. 502, 522–543. doi:10.1002/cne.21345
Owens, K. N., Santos, F., Roberts, B., Linbo, T., Coffin, A. B., Knisely, A. J., et al. (2008). Identification of genetic and chemical modulators of zebrafish mechanosensory hair cell death. PLoS Genet. 4, e1000020. doi:10.1371/journal.pgen.1000020
Park, J. C., and Cohen, G. M. (1982). Vestibular ototoxicity in the chick: Effects of streptomycin on equilibrium and on ampullary dark cells. Am. J. Otolaryngol. 3, 117–127. doi:10.1016/s0196-0709(82)80042-2
Patel, J., Szczupak, M., Rajguru, S., Balaban, C., and Hoffer, M. E. (2019). Inner ear therapeutics: An overview of middle ear delivery. Front. Cell. Neurosci. 13, 261. doi:10.3389/fncel.2019.00261
Peloggia, J., Münch, D., Meneses-Giles, P., Romero-Carvajal, A., Lush, M. E., Lawson, N. D., et al. (2021). Adaptive cell invasion maintains lateral line organ homeostasis in response to environmental changes. Dev. Cell 56, 1296–1312.e7. e7. doi:10.1016/j.devcel.2021.03.027
Philip, R. C., Rodriguez, J. J., Niihori, M., Francis, R. H., Mudery, J. A., Caskey, J. S., et al. (2018). Automated high-throughput damage scoring of zebrafish lateral line hair cells after ototoxin exposure. Zebrafish 15, 145–155. doi:10.1089/zeb.2017.1451
Pickett, S. B., Thomas, E. D., Sebe, J. Y., Linbo, T., Esterberg, R., Hailey, D. W., et al. (2018). Cumulative mitochondrial activity correlates with ototoxin susceptibility in zebrafish mechanosensory hair cells. eLife 7, e38062. doi:10.7554/eLife.38062
Prayuenyong, P., Baguley, D. M., Kros, C. J., and Steyger, P. S. (2021). Preferential cochleotoxicity of cisplatin. Front. Neurosci. 15, 695268. doi:10.3389/fnins.2021.695268
Pujol-Martí, J., Zecca, A., Baudoin, J.-P., Faucherre, A., Asakawa, K., Kawakami, K., et al. (2012). Neuronal birth order identifies a dimorphic sensorineural map. J. Neurosci. 32, 2976–2987. doi:10.1523/JNEUROSCI.5157-11.2012
Rah, Y. C., Han, E. J., Park, S., Rhee, J., Koun, S., Park, H.-C., et al. (2018). In vivo assay of the potential gadolinium-induced toxicity for sensory hair cells using a zebrafish animal model. J. Appl. Toxicol. 38, 1398–1404. doi:10.1002/jat.3656
Raible, D. W., and Kruse, G. J. (2000). Organization of the lateral line system in embryonic zebrafish. J. Comp. Neurol. 421, 189–198. doi:10.1002/(sici)1096-9861(20000529)421:2<189:aid-cne5>3.0.co;2-k
Ranasinghe, R., Mathai, M. L., and Zulli, A. (2022). Cisplatin for cancer therapy and overcoming chemoresistance. Heliyon 8, e10608. doi:10.1016/j.heliyon.2022.e10608
Riddell, I. A., and Lippard, S. J. (2018). “1. Cisplatin and oxaliplatin: Our current understanding of their actions,” in Metallo-Drugs: Development and action of anticancer agents (Berlin, Germany: De Gruyter), 1–42. doi:10.1515/9783110470734-001
Rocha-Sanchez, S. M., Fuson, O., Tarang, S., Goodman, L., Pyakurel, U., Liu, H., et al. (2018). Quinoxaline protects zebrafish lateral line hair cells from cisplatin and aminoglycosides damage. Sci. Rep. 8, 15119. doi:10.1038/s41598-018-33520-w
Rubel, E. W., Furrer, S. A., and Stone, J. S. (2013). A brief history of hair cell regeneration research and speculations on the future. Hear. Res. 297, 42–51. doi:10.1016/j.heares.2012.12.014
Rybak, L. P., Mukherjea, D., and Ramkumar, V. (2019). Mechanisms of cisplatin-induced ototoxicity and prevention. Semin. Hear. 40, 197–204. doi:10.1055/s-0039-1684048
Saettele, A. L., Wong, H.-T. C., Kindt, K. S., Warchol, M. E., and Sheets, L. (2022). Prolonged dexamethasone exposure enhances zebrafish lateral-line regeneration but disrupts mitochondrial homeostasis and hair cell function. J. Assoc. Res. Otolaryngol. doi:10.1007/s10162-022-00875-x
Santos, F., MacDonald, G., Rubel, E. W., and Raible, D. W. (2006). Lateral line hair cell maturation is a determinant of aminoglycoside susceptibility in zebrafish (Danio rerio). Hear. Res. 213, 25–33. doi:10.1016/j.heares.2005.12.009
Schacht, J., Talaska, A. E., and Rybak, L. P. (2012). Cisplatin and aminoglycoside antibiotics: Hearing loss and its prevention. Anat. Rec. 295, 1837–1850. doi:10.1002/ar.22578
Schmid, C., Alampi, I., Briggs, J., Tarcza, K., and Stawicki, T. M. (2020). Mechanotransduction activity facilitates hair cell toxicity caused by the heavy metal cadmium. Front. Cell. Neurosci. 14, 37. doi:10.3389/fncel.2020.00037
Seiler, C., and Nicolson, T. (1999). Defective calmodulin-dependent rapid apical endocytosis in zebrafish sensory hair cell mutants. J. Neurobiol. 41, 424–434. doi:10.1002/(sici)1097-4695(19991115)41:3<424:aid-neu10>3.0.co;2-g
Sepulcre, M. P., Alcaraz-Pérez, F., López-Muñoz, A., Roca, F. J., Meseguer, J., Cayuela, M. L., et al. (2009). Evolution of lipopolysaccharide (LPS) recognition and signaling: Fish TLR4 does not recognize LPS and negatively regulates NF-kappaB activation. J. Immunol. 182, 1836–1845. doi:10.4049/jimmunol.0801755
Shahab, M., Rosati, R., Meyer, D. N., Shields, J. N., Crofts, E., Baker, T. R., et al. (2021). Cisplatin-induced hair cell loss in zebrafish neuromasts is accompanied by protein nitration and Lmo4 degradation. Toxicol. Appl. Pharmacol. 410, 115342. doi:10.1016/j.taap.2020.115342
Shao, W., Zhong, D., Jiang, H., Han, Y., Yin, Y., Li, R., et al. (2021). A new aminoglycoside etimicin shows low nephrotoxicity and ototoxicity in zebrafish embryos. J. Appl. Toxicol. 41, 1063–1075. doi:10.1002/jat.4093
Smith, G., and Gallo, G. (2017). To mdivi-1 or not to mdivi-1: Is that the question? Dev. Neurobiol. 77, 1260–1268. doi:10.1002/dneu.22519
Stawicki, T. M., Hernandez, L., Esterberg, R., Linbo, T., Owens, K. N., Shah, A. N., et al. (2016). Cilia-associated genes play differing roles in aminoglycoside-induced hair cell death in zebrafish. G3 6, 2225–2235. doi:10.1534/g3.116.030080
Stawicki, T. M., Linbo, T., Hernandez, L., Parkinson, L., Bellefeuille, D., Rubel, E. W., et al. (2019). The role of retrograde intraflagellar transport genes in aminoglycoside-induced hair cell death. Biol. Open 8, bio038745. doi:10.1242/bio.038745
Stawicki, T. M., Owens, K. N., Linbo, T., Reinhart, K. E., Rubel, E. W., and Raible, D. W. (2014). The zebrafish merovingian mutant reveals a role for pH regulation in hair cell toxicity and function. Dis. Model. Mech. 7, 847–856. doi:10.1242/dmm.016576
Strähle, U., Scholz, S., Geisler, R., Greiner, P., Hollert, H., Rastegar, S., et al. (2012). Zebrafish embryos as an alternative to animal experiments-a commentary on the definition of the onset of protected life stages in animal welfare regulations. Reprod. Toxicol. 33, 128–132. doi:10.1016/j.reprotox.2011.06.121
Suli, A., Watson, G. M., Rubel, E. W., and Raible, D. W. (2012). Rheotaxis in larval zebrafish is mediated by lateral line mechanosensory hair cells. PloS One 7, e29727. doi:10.1371/journal.pone.0029727
Taiber, S., Gwilliam, K., Hertzano, R., and Avraham, K. B. (2022). The genomics of auditory function and disease. Annu. Rev. Genomics Hum. Genet. 23, 275–299. doi:10.1146/annurev-genom-121321-094136
Tantry, M. S. A., Harini, V. s., and Santhakumar, K. (2022). Simple and high-throughput rheotaxis behavioral assay for zebrafish larva. Zebrafish 19, 114–118. doi:10.1089/zeb.2021.0087
Thomas, A. J., Hailey, D. W., Stawicki, T. M., Wu, P., Coffin, A. B., Rubel, E. W., et al. (2013). Functional mechanotransduction is required for cisplatin-induced hair cell death in the zebrafish lateral line. J. Neurosci. 33, 4405–4414. doi:10.1523/JNEUROSCI.3940-12.2013
Thomas, E. D., and Raible, D. W. (2020). “Chapter 22 - the mechanosensory lateral line system,” in The zebrafish in biomedical research American college of laboratory animal medicine. Editors S. C. Cartner, J. S. Eisen, S. C. Farmer, K. J. Guillemin, M. L. Kent, and G. E. Sanders (Cambridge: Academic Press), 245–253. doi:10.1016/B978-0-12-812431-4.00022-1
Todd, D. W., Philip, R. C., Niihori, M., Ringle, R. A., Coyle, K. R., Zehri, S. F., et al. (2017). A fully automated high-throughput zebrafish behavioral ototoxicity assay. Zebrafish 14, 331–342. doi:10.1089/zeb.2016.1412
Ton, C., and Parng, C. (2005). The use of zebrafish for assessing ototoxic and otoprotective agents. Hear. Res. 208, 79–88. doi:10.1016/j.heares.2005.05.005
Vargo, J. W., Walker, S. N., Gopal, S. R., Deshmukh, A. R., McDermott, B. M., Alagramam, K. N., et al. (2017). Inhibition of mitochondrial division attenuates cisplatin-induced toxicity in the neuromast hair cells. Front. Cell. Neurosci. 11, 393. doi:10.3389/fncel.2017.00393
Venuto, A., Smith, C. P., Cameron-Pack, M., and Erickson, T. (2022). Alone in a crowd: Effect of a nonfunctional lateral line on expression of the social hormone parathyroid hormone 2. Biol. Open 11, 059432. doi:10.1242/bio.059432
Vlasits, A. L., Simon, J. A., Raible, D. W., Rubel, E. W., and Owens, K. N. (2012). Screen of FDA-approved drug library reveals compounds that protect hair cells from aminoglycosides and cisplatin. Hear Res. 294, 153–165. doi:10.1016/j.heares.2012.08.002
Wang, Q., and Steyger, P. S. (2009). Trafficking of systemic fluorescent gentamicin into the cochlea and hair cells. J. Assoc. Res. Otolaryngol. 10, 205–219. doi:10.1007/s10162-009-0160-4
Weir, J., Rivolta, M., and Holley, M. (2000). Identification of differentiating cochlear hair cells in vitro. Am. J. Otol. 21, 130–134. doi:10.1016/s0196-0709(00)80087-3
Wertman, J. N., Melong, N., Stoyek, M. R., Piccolo, O., Langley, S., Orr, B., et al. (2020). The identification of dual protective agents against cisplatin-induced oto- and nephrotoxicity using the zebrafish model. eLife 9, e56235. doi:10.7554/eLife.56235
Whitfield, T. T., Granato, M., van Eeden, F. J., Schach, U., Brand, M., Furutani-Seiki, M., et al. (1996). Mutations affecting development of the zebrafish inner ear and lateral line. Development 123, 241–254. doi:10.1242/dev.123.1.241
Wiedenhoft, H., Hayashi, L., and Coffin, A. B. (2017). PI3K and inhibitor of apoptosis proteins modulate gentamicin- induced hair cell death in the zebrafish lateral line. Front. Cell. Neurosci. 11, 326. doi:10.3389/fncel.2017.00326
Williams, J. A., and Holder, N. (2000). Cell turnover in neuromasts of zebrafish larvae. Hear. Res. 143, 171–181. doi:10.1016/s0378-5955(00)00039-3
Wu, C.-H., Lu, C.-W., Hsu, T.-H., Wu, W.-J., and Wang, S.-E. (2021). Neurotoxicity of fipronil affects sensory and motor systems in zebrafish. Pestic. Biochem. Physiol. 177, 104896. doi:10.1016/j.pestbp.2021.104896
Wu, W. J., Sha, S. H., McLaren, J. D., Kawamoto, K., Raphael, Y., and Schacht, J. (2001). Aminoglycoside ototoxicity in adult CBA, C57BL and BALB mice and the Sprague-Dawley rat. Hear. Res. 158, 165–178. doi:10.1016/s0378-5955(01)00303-3
Xia, M., Wang, X., Xu, J., Qian, Q., Gao, M., and Wang, H. (2021). Tris (1-chloro-2-propyl) phosphate exposure to zebrafish causes neurodevelopmental toxicity and abnormal locomotor behavior. Sci. Total Environ. 758, 143694. doi:10.1016/j.scitotenv.2020.143694
Xiao, K., Yanhui, S., Zhenjie, W., Shuang, Z., and Hongzhuo, L. (2018). A mitochondrial targeting tetrapeptide Bendavia protects lateral line hair cells from gentamicin exposure. J. Appl. Toxicol. 38, 376–384. doi:10.1002/jat.3547
Xiao, T., Roeser, T., Staub, W., and Baier, H. (2005). A GFP-based genetic screen reveals mutations that disrupt the architecture of the zebrafish retinotectal projection. Development 132, 2955–2967. doi:10.1242/dev.01861
Young, A., Kochenkov, V., McIntyre, J. K., Stark, J. D., and Coffin, A. B. (2018). Urban stormwater runoff negatively impacts lateral line development in larval zebrafish and salmon embryos. Sci. Rep. 8, 2830. doi:10.1038/s41598-018-21209-z
Zallocchi, M., Hati, S., Xu, Z., Hausman, W., Liu, H., He, D. Z., et al. (2021). Characterization of quinoxaline derivatives for protection against iatrogenically induced hearing loss. JCI Insight 6, 141561. doi:10.1172/jci.insight.141561
Zhang, Q., and Kindt, K. S. (2022). Using light-sheet microscopy to study spontaneous activity in the developing lateral-line system. Front. Cell Dev. Biol. 10, 819612. doi:10.3389/fcell.2022.819612
Zhang, Q., Li, S., Wong, H.-T. C., He, X. J., Beirl, A., Petralia, R. S., et al. (2018). Synaptically silent sensory hair cells in zebrafish are recruited after damage. Nat. Commun. 9, 1388. doi:10.1038/s41467-018-03806-8
Keywords: hair cell, zebrafish, ototoxicity, otoprotection, cisplatin, aminoglycoside antibiotic
Citation: Barrallo-Gimeno A and Llorens J (2022) Hair cell toxicology: With the help of a little fish. Front. Cell Dev. Biol. 10:1085225. doi: 10.3389/fcell.2022.1085225
Received: 31 October 2022; Accepted: 28 November 2022;
Published: 13 December 2022.
Edited by:
Emma J. Kenyon, University of Sussex, United KingdomReviewed by:
Yingzi He, Fudan University, ChinaMark Warchol, Washington University in St. Louis, United States
Copyright © 2022 Barrallo-Gimeno and Llorens. This is an open-access article distributed under the terms of the Creative Commons Attribution License (CC BY). The use, distribution or reproduction in other forums is permitted, provided the original author(s) and the copyright owner(s) are credited and that the original publication in this journal is cited, in accordance with accepted academic practice. No use, distribution or reproduction is permitted which does not comply with these terms.
*Correspondence: Alejandro Barrallo-Gimeno, YWJhcnJhbGxvQHViLmVkdQ==