- 1Department of Endocrinology and Metabolism, First Affiliated Hospital of Nanchang University, Nanchang, China
- 2The First Clinical Medical College of Nanchang University, First Affiliated Hospital of Nanchang University, Nanchang, China
- 3The Second Clinical Medical College of Nanchang University, Second Affiliated Hospital of Nanchang University, Nanchang, China
- 4Jiangxi Clinical Research Center for Endocrine and Metabolic Disease, Nanchang, Jiangxi, China
- 5Jiangxi Branch of National Clinical Research Center for Metabolic Disease, Nanchang, Jiangxi, China
Obesity is a disease commonly associated with urbanization and can also be characterized as a systemic, chronic metabolic condition resulting from an imbalance between energy intake and expenditure. The World Health Organization (WHO) has identified obesity as the most serious chronic disease that is increasingly prevalent in the world population. If left untreated, it can lead to dangerous health issues such as hypertension, hyperglycemia, hyperlipidemia, hyperuricemia, nonalcoholic steatohepatitis, atherosclerosis, and vulnerability to cardiovascular and cerebrovascular events. The specific mechanisms by which obesity affects the development of these diseases can be refined to the effect on immune cells. Existing studies have shown that the development of obesity and its associated diseases is closely related to the balance or lack thereof in the number and function of various immune cells, of which neutrophils are the most abundant immune cells in humans, infiltrating and accumulating in the adipose tissues of obese individuals, whereas NETosis, as a newly discovered type of neutrophil-related cell death, its role in the development of obesity and related diseases is increasingly emphasized. The article reviews the significant role that NETosis plays in the development of obesity and related diseases, such as diabetes and its complications. It discusses the epidemiology and negative impacts of obesity, explains the mechanisms of NETosis, and examines its potential as a targeted drug to treat obesity and associated ailments.
1 Introduction
Currently, obesity has replaced malnutrition and diseases caused by infection as diseases with the highest mortality from its complications. Recent statistics indicate that the number of overweight or obese individuals globally continues to escalate, with over 200 million affected individuals, which accounts for approximately 30% of the world’s population. The Global Burden of Disease Panel reported that the prevalence of obesity had doubled in more than 70 countries since 1980 and continued to increase in most other countries in 2017 (Collaborators et al., 2017).
Studies have now been conducted that link obesity to a variety of diseases and related complications, such as nonalcoholic fatty liver disease, type 2 diabetes, and atherosclerosis. Obesity not only has a serious impact on people’s quality of life but can also have a negative impact on mental health. With obesity escalating at an alarming rate, it is imperative to conduct more research into the mechanisms that drive obesity to facilitate the development of preventative and remedial strategies for obesity and related health conditions.
Multiple studies have found that obesity-related diseases lead to an abnormal immune system and cell population (Fang et al., 2020a; Hildreth et al., 2021), such as CD4, CD8 (Yang et al., 2010), Th17, Th22 (Fabbrini et al., 2013), mononucleosis (Ilavská et al., 2012), macrophages (Wu et al., 2007), eosinophils (Moussa et al., 2019), neutrophils, and pathological enrichment of immune cell subsets. Among them, neutrophils play an important role in human immunity, which is an important role for the body to clear foreign threats and autoimmunity. The death modes of central granulocytes include pathogen-induced cell death, necrosis, pyrodeath, autophagy, aging and NETosis. NETosis is a specific type of neutrophil death mechanism that is different from apoptosis and pyrodeath. Its primary role is to enhance organismal immunity by lysing the nuclear membrane, decondensing DNA, rupturing the plasma membrane, and releasing reticular structures to capture and eliminate pathogens. Multiple studies have demonstrated the involvement of NETosis in the mechanisms and progression of various obesity-related diseases.
This review aims to provide an overview of the mechanisms of NETosis and its positive effects on obesity and related diseases. It will also list drugs commonly used in these diseases to guide future research on the mechanisms, as well as on the diagnosis and treatment of obesity and its complications.
2 Mechanisms of NETosis
2.1 Pathophysiological mechanism of NETosis
Neutrophils are the first immune cells recruited to the relevant site when the body is exposed to infection or injury and are the first line of defense against foreign microorganisms, playing an important role in internal immunity. Neutrophil extracellular trap networks (NETs) are a recently discovered anti-inflammatory mechanism. Unlike apoptosis and necrosis, NETosis is a special form of programmed cell death that is released when neutrophils are activated (Takei et al., 1996; Brinkmann et al., 2004), a process of NET release known as NETosis. In this process, the nucleus of the neutrophil loses its identity, its chromatin is stripped, and the nuclear membrane and particles breakdown and mix together. NET cells are subsequently released extracellularly by neutrophils and consist mainly of a double-stranded DNA complex, a reticulum complex consisting mainly of double-stranded DNA, along with a large number of granular proteins and various anti-inflammatory proteins, such as antimicrobial peptides, proteases, and guanosine histones (Fousert et al., 2020). The amount of each protein component depends on the proportion of NETs released by different neutrophil subpopulations (Pinegin et al., 2015; Hamam and Palaniyar, 2019). In contrast to other cell death processes, such as apoptosis, necrosis, or apoptosis, chromatin deperfusion is the main defining feature of NETosis. Chromatin decompression is thought to be mediated by histone posttranslational modifications, the most typical of which are PAD4-mediated citrullination and serine proteinase-mediated cleavage (Wong and Wagner, 2018). The study found that there are three main types of activation mechanisms during the anti-inflammatory immune response to NETosis (Figure 1). One is NOX-dependent NETosis, a collective term for the catalytic subunit of NADPH oxidase gp91phox/NOX2 and its homologs, where the key step in NETosis is the production of ROS by NADPH oxidase (Fousert et al., 2020). The most potent inducer is fosfomycin myristate (PMA) (de Bont et al., 2018). In addition to killing microorganisms, ROS also play an important role in the subsequent production of NETs, creating the conditions for the subsequent activation of myeloperoxidase (MPO) by H2O2 as a substrate, which in turn induces the release of neutrophil elastase (NE). Optimal conditions and environmental pH. After 60 min of stimulation, HOCI breaks down the cytoplasm and releases activated NE into the cytoplasm. One hundred 20 minutes later, NE degrades F-actin and enters the nucleus, breaking down histone H1 and finally reaching the core histones. Later, MPO, together with NE, promotes histone decondensation, the unfolding of additional chromatin and further disruption of the nuclear membrane. The nuclear membrane ruptures, gradually disintegrating into vesicles and releasing them into the cytoplasm, allowing the cytoplasm to mix with the nucleoplasm and decondensed chromatin to bind to cytoplasmic antimicrobial proteins, granulins and other proteins. Finally, with the rupture of the plasma membrane, NETs are released, followed by neutrophil death (Amulic et al., 2017; Bravo-Barrera et al., 2017; Manfredi et al., 2018; Volkman et al., 2019).
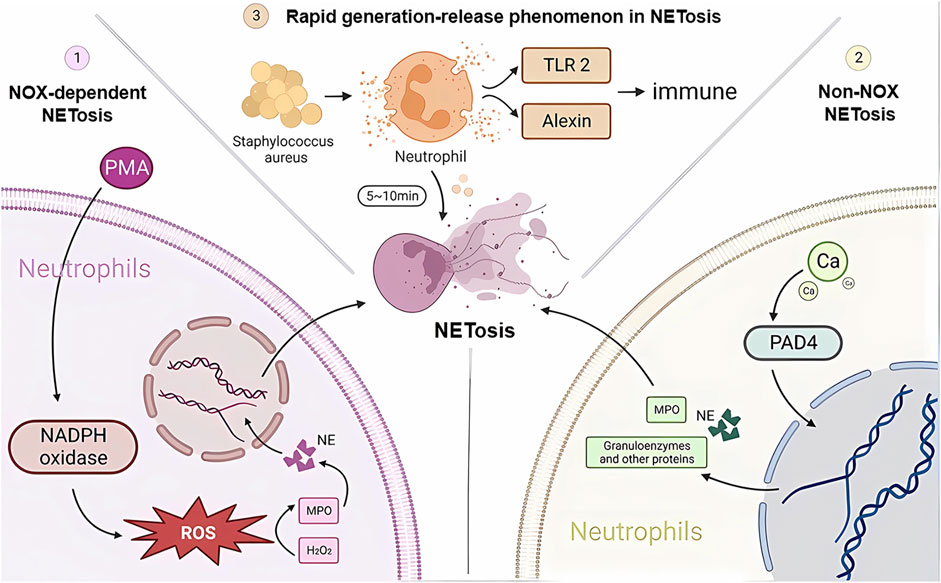
FIGURE 1. NETosis developmental mechanisms. Three different pathways of NETosis development are plotted in the figure. NADPH, nicotinamide adenine dinucleotide phosphate; NE, neutrophil elastase; MPO, myeloperoxidase; H2O2, hydrogen peroxide; TLR2, Toll-like receptor 2; PAD4, peptidylarginine deaminase 4.
Another type of NETosis does not depend on NADPH, and the occurrence of non-NOX-dependent NETosis depends on calcium inward flow, where the calcium carrier ionomycin, A23187, and pH elevation can induce non-NOX-dependent NETosis (Ravindran et al., 2019). As the concentration of calcium ions in the cytoplasm rises, peptidylarginine deaminase 4 (PAD4) activates, guanylating histones (mainly H3) and reducing the positive charge carrying capacity of histones, resulting in a weakened ability to bind proteins to DNA, thus causing chromatin decondensation. Chromatin decondenses into the cytoplasm and binds granzymes such as MPO, NE and other proteins, which are eventually released into the extracellular space to form NETs (Eghbalzadeh et al., 2019; Holmes et al., 2019). It has been shown that cells deprived of PAD4 will not be able to successfully release NETs (Sorensen and Borregaard, 2016). It has also been shown that in resting neutrophils, PAD4 is associated with the cytoplasmic subunit of NADPH oxidase and that this association affects ROS production. Thus, PAD4 may play a different and important role in NOX-dependent versus non-NOX-dependent NETosis (Zhou et al., 2018).
There is another rapid generation-release phenomenon in NETosis. In nitrogen-dependent and nonnitrogen-dependent cases, NET release takes 3–4 h (Ahmad et al., 2021) and eventually leads to death. In contrast, recent studies have shown that neutrophil skin infections such as Staphylococcus aureus produce within 10 min of stimulation, and even when NETosis occurs, some neutrophils do not die but remain active, showing an immune response through crawling Toll-like receptor 2 (TLR2) and the protein of the compliment, a granular release network that extends the unstable pseudopod and extends it through the skin. Transiently nucleated gran neutrophils are left behind and undergo hyperpolarization, which is the phenomenon of rapid opening of ion channels on the neutrophil cell membrane and a rapid increase in cell membrane potential, which changes the content of avidin on the cell membrane and promotes the secretion of microbial killer molecules, forming NETs (Hilscher and Shah, 2020; Nija et al., 2020; Alasmari, 2020). The rapid formation of NETs may also be an important mechanism for fighting infection, which may primarily act in the early stages of infection. In the human immune system, NETosis is a double-edged sword. On the one hand, NETosis has been described as a neutrophil defense mechanism of the organism that traps and destroys a wide range of pathogens involved in anti-inflammatory, antibacterial and antiviral immune responses, including Gram-positive bacteria (von Kockritz-Blickwede and Winstel, 2022), Gram-negative bacteria and their virulence factors (Sung et al., 2022), fungi (Huang et al., 2022), protozoa (Conejeros et al., 2022) and viruses (Kapoor and Shukla, 2023). NETosis is also involved in the normal regeneration and repair of blood vessels. On the other hand, there is increasing evidence from recent studies that NETosis is also involved in the pathophysiology of many diseases, such as autoimmune diseases (Alaygut et al., 2023), diabetes (Farhan et al., 2023), cardiovascular diseases (Fadini et al., 2016b), cancer (Tomas-Perez et al., 2023) and Alzheimer’s disease (Smyth et al., 2022). Overactivation and inappropriate recruitment of NETosis may lead to severe organismal damage and disease deterioration (Figure 2).
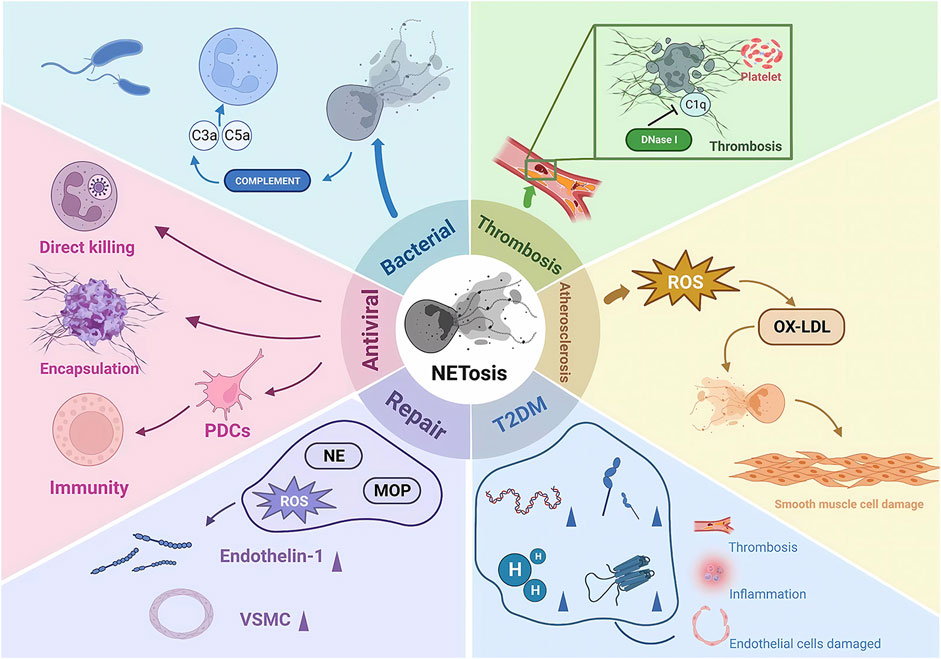
FIGURE 2. The pathophysiological role of NETosis. NE, neutrophil elastase; MOP, myeloperoxidase; ROS, reactive oxygen species; PDCs, plasmacytoid dendritic cells; ET1, endothelin 1; VSMCs, vascular smooth muscle cells; IL-6, interleukin 6; OX-LDL, oxidized low-density lipoprotein; DNase 1, deoxyribonuclease I.
Recent studies have also shown that NETosis is biased and that there is a specific subpopulation bias in the formation of NETosis by neutrophils (Denny et al., 2010; Ng et al., 2019). In some autoimmune diseases, such as systemic lupus erythematosus (SLE), the phenomenon of NETs resulting from an increase in this class of neutrophils may be a potential mechanism for the development of symptoms and complications of autoimmune diseases (Kaplan, 2011; Villanueva et al., 2011; Bengtsson et al., 2014; Wirestam et al., 2019). During the clearance of infection and immune defense by NETs, neutrophils also inevitably release their own antigens extracellularly, bringing a range of products into frequent contact with the body’s immune system and greatly increasing the chances of possible autoimmunity, making them an important target for immune responses. In addition, the large number of active mediators released by NETs, if combined with proinflammatory factors, will accelerate the inflammatory process and intensify the immune response (Lee et al., 2017b; Jorch and Kubes, 2017; He et al., 2018; Fousert et al., 2020).
It is worth noting that NETosis may possess both anti-inflammatory and proinflammatory effects, which are mainly related to the timing and concentration of NETs (Schauer et al., 2014). The mediators released by NETs in the early stages of inflammation contribute to the recruitment and attachment of platelets, macrophages and neutrophils, promoting an inflammatory immune response. In contrast, the dense reticulum structure in later stages may serve to confine areas of inflammation, and serine proteases attached to the reticulum are able to block neutrophil chemotaxis and contribute to the regression of inflammation by degrading inflammatory factors such as IL-1β and chemokines (Joosten et al., 2010; Mitroulis et al., 2011). Two conditions are necessary for the onset of acute gout: monosodium urate (MSU) deposition and the body’s inflammatory response to crystals. Both of these utilities play a significant role in the onset and spontaneous remission of acute gout (Steiger and Harper, 2014; Dalbeth et al., 2021).
In the context of the proinflammatory and prothrombotic phenomenon of excessive NETosis, several studies have shown the involvement of NETosis in the development and progression of many disease complications (Fadini et al., 2016b; Smyth et al., 2022; Alaygut et al., 2023; Farhan et al., 2023; Tomas-Perez et al., 2023). It has been suggested that the hyperglycemic environment of diabetic patients may induce the formation of NETs and NETosis (Menegazzo et al., 2015). In addition, patients with type 2 diabetes have increased circulating NETosis products such as histones, DNA, elastase and circulating mono- and oligonucleosomes and produce more IL-6 (Avogaro et al., 2003). This set of changes may lead to thrombosis, inflammation and endothelial dysfunction, which can affect the development of diabetic complications such as diabetic macular degeneration, diabetic nephropathy, cardiovascular disease and diabetic foot. The mechanism by which the high-glucose environment promotes the development of NETosis is not yet clear but may be related to the glucose-induced increase in NADPH oxidase, which requires further study (Almyroudis et al., 2013; Keshari et al., 2013).
2.2 Detection method
Currently, fluorescence microscopy is the most widely accepted method for visualizing and quantifying NETs. However, this approach has its drawbacks; it is subjective, time-consuming, and yields a small sample size of analyzed polymorphonuclear cells (PMN) per sample. There is now a growing interest in using flow cytometry techniques to identify NETs. However, flow cytometry analysis of NETs requires special attention to sample preparation to obtain reproducible data. The assay uses a combination of fluorescently labeled antibodies against cellular markers on PMNs and nucleic acid stains to measure NETosis in whole blood (WB) and purified PMNs using the plasma membrane impermeable DNA binding dye SYTOX Orange (SO), which is positive for SO and DAPI in the cell-attached DNA of NETting PMNs. The combination of optimally diluted antibody and nucleic acid dye requires no washing and produces low background fluorescence. Because WB and purified PMNs correlate significantly with NETosis, the assay can be validated by comparison with time-lapse live cell fluorescence microscopy. The assay can be well applied to diseases associated with NETosis, such as systemic lupus erythematosus (SLE). The assay is observer-independent and allows rapid assessment of large numbers of PMNs per sample, does not require sophisticated microscopic equipment such as imaging flow cytometry, and can be used as a starting point for analyzing the formation of extracellular traps (traps) in immune cells other than PMNs (Zharkova et al., 2019). Another novel imaging flow cytometry (IFC) allows specific detection and quantification of histone 4 (H4cit3) guanylation as a marker of NETs in whole neutrophils before the release of DNA and cytoplasmic protein chains into the extracellular space. Other analytical parameters looking at nuclear and cellular morphological changes (nuclear decondensation and hypercontraction, lobulated nuclei and cell membrane damage) can provide additional information about the behavior of the analyzed neutrophil population (Barbu et al., 2021). Another representative innovative and efficient analytical method is the imaging and computational algorithm of the High Context Screening (HCS) cytomics platform, which employs membrane permeable and impermeable DNA dyes to identify NET-forming cells in situ. Automated algorithm-driven single-cell analysis of nuclear morphology changes, nuclear area increases and intensity changes provides accurate detection of NET-forming cells and eliminates user bias against other cell death patterns. It is further combined with in situ staining for membrane-linked protein V to detect specific death pathways, such as apoptosis, and thus distinguishes between NET, apoptosis and necrosis. The method does not utilize fixation and permeabilization steps that interfere with NETs, thus allowing monitoring of NETs over time, and this imaging-based method for specific high-throughput NET analysis may provide a good platform for discovering potential inhibitors of NET formation and/or drugs that regulate neutrophil death (e.g., NETosis-apoptosis switch) as an alternative strategy for enhancing inflammatory resolution (Singhal et al., 2022). In addition, convolutional neural networks (CNNs) can also be used to accurately quantify neutrophil NETosis. CNNs have achieved >94% performance accuracy in distinguishing NETosis from non-NETosis cells and have greatly facilitated dose‒response analysis of NETosis responses in patients’ neutrophils and screening. Using only features learned from nuclear morphology, the CNN can distinguish between NETosis and necrosis and different NETosis signaling pathways, making it an accurate tool for NETosis detection (Elsherif et al., 2019). Other detection methods include ELISA, immunoblotting, and image flow-based methods, but all of them exhibit the disadvantages of subjectivity, nonspecificity, error proneness and low throughput.
3 NETosis in obesity and obesity-related diseases
3.1 Obesity
Before fully describing the relationship between obesity and NETosis, it is important to note that obesity is a heterogeneous disease, with variations in definitions of obesity and assessment criteria across populations and assessment methods. This heterogeneity can be explored through body mass index (BMI), waist circumference, and other relevant measures, and the potential impact of obesity on different disease mechanisms and the development of various conditions (e.g., nonalcoholic fatty liver disease, type 2 diabetes mellitus, and atherosclerosis) can be discussed. Body mass index (BMI), calculated by dividing body weight (kilograms) by the square of height (meters), is a commonly used indicator to define obesity. BMI is the most basic way of defining obesity; however, there is a U- or J-shaped relationship between BMI-defined obesity and mortality, which has led to the creation of other measures (Flegal et al., 2013). Waist circumference (WC) is another indicator used to assess central obesity, which is strongly associated with metabolic complications, which may be related to the fact that WC is more able to characterize the fat levels of visceral tissues (Armstrong et al., 2022).
In addition to BMI and WC, a number of other measures, such as waist-to-hip ratio (WHtR) and fat mass index (FMI), have also been used to define obesity. The waist-to-height ratio (WHtR) was shown to be superior to WC and BMI in detecting cardiometabolic risk factors in both sexes in multiple cohorts of large samples (Ashwell et al., 2012). A research trial showed that fat mass index (FMI) and WC were more useful in identifying the risk of obesity-associated neuropathy compared to BMI in patients with T2DM (Gao et al., 2021). The diversity of obesity definitions and measurements can greatly influence our understanding of disease mechanisms and associated disease progression. For example, differences in obesity criteria may influence the prevalence and severity of NAFLD, type 2 diabetes, and atherosclerosis. By exploring these associations, we can gain insight into potential interventions and personalized treatment strategies for obesity-related diseases.
The different measures also imply that the development of obesity is influenced by a variety of factors. The prevailing view is that chronic energy imbalance is the main factor in the development of obesity (Rohm et al., 2022). Obesity occurs when caloric expenditure in the body decreases and most of the energy is stored in fat cells as triglycerides. Triglycerides continue to accumulate in adipocytes, which limits blood flow in adipose tissue, and the oxygen supply is reduced as a result (Maurizi et al., 2018). As obesity progresses, intestinal mucosal permeability increases, and under the influence of a variety of factors, including intestinal microbes and their metabolites, obese patients develop mild chronic inflammation throughout the body.
Thus, the molecular and cellular mechanisms by which obesity leads to inflammation and insulin resistance involve several complex processes: 1) Release of inflammatory factors by adipocytes: adipocytes release a variety of inflammatory factors, such as tumor necrosis factor-alpha (TNF-α), interleukin-6 (IL-6), and C-reactive protein (CRP). These inflammatory factors can stimulate the inflammatory response and interfere with insulin signaling, leading to insulin resistance (Ahmed et al., 2021). 2) Oxidative stress in adipose tissue: oxidative stress can lead to the production of excessive reactive oxides in the cells, which can damage cellular structure and function and in turn trigger the inflammatory response and insulin resistance (Ouchi et al., 2011). 3) Lipid accumulation in adipose tissue: lipid accumulation can lead to the abnormal function of adipocytes, which release more inflammatory factors and interfere with insulin signaling, leading to insulin resistance. 4) Inflammatory cell infiltration in adipose tissue: the number of inflammatory cells such as neutrophils, macrophages and T-lymphocytes increases. These inflammatory cells release inflammatory factors, creating an inflammatory environment that further exacerbates insulin resistance (De Lorenzo et al., 2016). It is currently believed that in the early stages of obesity, adipose tissue triggers an inflammatory response, leading to the activation of inflammatory cells (e.g., macrophages and neutrophils) and the release of NETs, which induces NETosis. The formation of NETs may further exacerbate the inflammatory response and insulin resistance, creating a vicious cycle. Obesity is associated with oxidative stress and lipid accumulation, which may also be involved in the induction of NETosis.
Studies have shown that obesity increases the number of low-density neutrophils with inflammatory genetic features (Sanchez-Pino et al., 2022). This low-density neutrophil population showed proinflammatory, degranulation, and immunosuppressive functions. Furthermore, the study also showed that bariatric surgery, as an effective treatment for severe obesity, can reduce circulating LDNs and improve immunity and metabolism in obese patients. This also provides another idea for research: to improve multiple adverse effects in obese patients by modulating NETosis (Metzemaekers et al., 2020). Neutrophils are immune cells that infiltrate adipose tissue. The low-grade chronic inflammation produced by obesity plays an important role in the activation of neutrophils, and its mechanism and role in the formation of NETosis remain to be explored in greater depth. However, the findings regarding the mechanisms of NETosis in obesity are unclear or even contradictory (Figure 3).
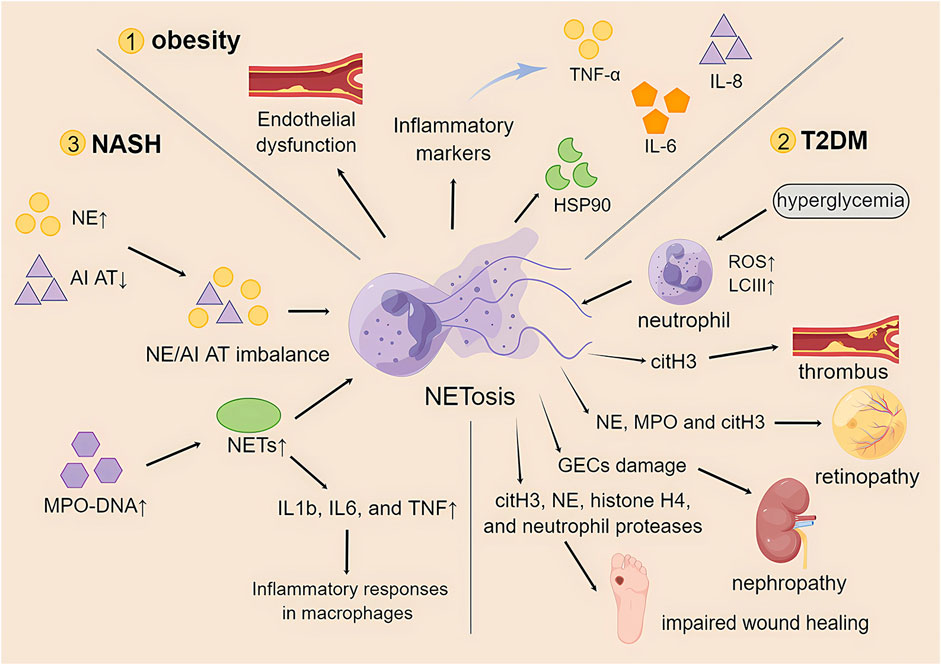
FIGURE 3. The role of NETosis in obesity and its associated metabolic diseases. TNF-α, tumor necrosis factor-α; IL-6, interleukin-6; IL-8, interleukin-8; HSP90, heat shock protein 90; T2DM, type 2 diabetes mellitus; ROS, reactive oxygen species; LCIII, microtubule-associated protein light chain III; citH3, citrullinated histone 3; NE, neutrophil elastase; MPO, myeloperoxidase; GECs, glomerular endothelial cells; NASH, nonalcoholic steatohepatitis; AI AT, α1-antitrypsin; NETs, neutrophil extracellular traps; IL1b, interleukin 1b.
Several studies point to an association of obesity-induced inflammation with high levels of NETosis. In a study in a high-fat diet (HFD) mouse model of obesity, endothelial dysfunction and high expression of NETosis in the mesenteric artery wall were reported (Wang et al., 2018a). When NETosis was suppressed, endothelial function was subsequently restored. Therefore, the occurrence of NETosis in obesity may be responsible for endothelial dysfunction. Recent database analysis studies have also revealed a strong relationship between inflammatory markers such as TNF-α, IL-6, IL-8, and heat shock protein 90 (HSP90) and NETosis formation in obese patients (Valeria Oliveira de Sousa et al., 2021; Freitas et al., 2022). It has also been reported that obese patients can reduce NETosis levels and thus improve the occurrence of adverse events through exercise (Valeria Oliveira de Sousa et al., 2021).
However, in other studies, low levels of NETosis were instead demonstrated in obese patients. A study that examined purified neutrophils from obese mice found that although neutrophils exhibited elevated ROS expression, suggesting neutrophil activation, they had lower NETosis than nonobese mice (Roberts et al., 2018). Similarly, when the hepatic vascular system of HFD mice was observed using in vivo microscopy, it was found that NETosis levels in obese mice remained lower than normal (Cichon et al., 2021).
Another class of studies notes that the direct causal relationship between NETosis and obesity is unclear. There is even an early study showing that inhibition of NETosis formation may not be clinically relevant to obesity-mediated adverse events such as adipose tissue inflammation and insulin resistance. A recent study has shown that severe obesity is associated with increased production of NETs (Rosales et al., 2017) and that NETosis in turn alters the systemic inflammatory status of patients (Rhee et al., 2018). Although bariatric surgery removed some of the adipose tissue, it failed to correct NETosis. Moreover, patients with the highest risk of cardiovascular events are suspected to be associated with the persistence of NETosis (D'Abbondanza et al., 2019).
The differences between the above studies may be related to the fact that different methods of assessing NETosis were used. Most of them used the following methods: a) detection of some NET components in plasma or serum; b) direct in vitro assessment of NETosis formation with purified neutrophils; and c) live microscopy to detect NETosis (none of them even confirmed the occurrence of NETosis). Elevated circulating DNA or neutrophil granulocyte protein does not equate to NETosis, and in vitro assays using purified neutrophils are more reliable. The results and conclusions are not only influenced by the limitations of the methods used but likewise depend on the differences in the study population. Therefore, future studies will require more rigorous assays and modeling criteria.
3.2 Type 2 diabetes
Type 2 diabetes mellitus (T2DM) is a chronic multifactorial disease closely associated with overweight and obesity. Its characteristic is relative insulin deficiency caused by pancreatic beta-cell dysfunction and target organ insulin resistance. In the presence of ß-cell dysfunction, insulin secretion is unable to maintain glucose homeostasis, resulting in abnormally high glucose levels in the blood (Kahn et al., 1993; Stumvoll et al., 2005). On the other hand, insulin resistance increases glucose production in the liver and decreases glucose uptake in skeletal muscle, liver, and adipose tissue (Petersen and Shulman, 2002; Leclercq et al., 2007; Czech, 2020). When these two pathological states coexist, they interact and may synergistically exacerbate T2DM (Cerf, 2013). Neutrophil elastase (NE) is a key effector involved in the inflammatory response to obesity, and its additivity in hepatocytes or adipocytes leads to cellular insulin resistance (Talukdar et al., 2012). In addition, the absence of NE leads to less tissue inflammation accompanied by improved glucose tolerance and increased insulin sensitivity. These results indicate that neutrophils may be directly involved in inflammation-induced metabolic diseases such as T2DM (Talukdar et al., 2012). Neutrophils may function as amplifiers of inflammatory signals and chemotactic agents for other inflammatory subpopulations, playing a crucial role in the regulation of insulin resistance in insulin-sensitive tissues.
Extensive evidence indicates the production of NETs and the circulation of NET markers, including nucleosomal DNA, HNE-DNA complexes, MPO-DNA complexes, and cell-free DNA (cfDNA). HNE and the expression of PAD4 on neutrophils are increased in patients with T2DM (Menegazzo et al., 2015; Wong et al., 2015; Carestia et al., 2016; Miyoshi et al., 2016; Wang et al., 2018b). A study found that the percentage of glucose-controlled markers HbA1c was positively correlated with circulating NET markers, including mononucleosomes and oligonucleotides. Hyperglycemia increased the release of circulating NET markers and NETosis. This finding provides evidence for a link between diabetes and NETs (Menegazzo et al., 2015). In addition, it has been demonstrated that hyperglycemia leads to increased levels of ROS in neutrophils of diabetic patients, which leads to an increase in LCIII, a marker of autophagy, and downstream NETosis (Farhan et al., 2023).
However, the role of NETosis in the pathogenesis of T2DM remains debated. A recent study provided evidence that increased formation of NETs (especially PAD4) is strongly associated with leaky gut in T1DM but unrelated to T2DM (You et al., 2019). A report showed impaired NET production under hyperglycemic conditions in vitro (Joshi et al., 2013). To explore the link between glycemic control and NETosis in T2DM patients, researchers found that increased NETosis in T2DM patients appeared to be due to proinflammatory cytokines rather than the result of impaired glycemic control when assessing whether NET levels changed after treatment with diabetic medications (Carestia et al., 2016).
Although the role of NETosis in the pathogenesis of T2DM remains controversial, there is growing evidence that NETs play an important role in diabetes-related complications. The presence of NETs in atherosclerotic plaques in mice and humans was demonstrated a decade ago (Megens et al., 2012), and a subsequent study showed that the NET component is significantly higher in patients with severe coronary artery disease (CAD) than in healthy controls (Borissoff et al., 2013) and that NETs may also impair plaque dissolution (Josefs et al., 2020), but the clinical use of NET markers in diabetic patients at risk of CAD has not been proven. By immunohistochemical staining analysis, citrullinated histone 3 (citH3), a marker of NETs, was detected in thrombi of almost all ischemic stroke patients (Laridan et al., 2017), and increased NETs in thrombus and peri-infarct brain tissue were associated with hyperglycemia (Deng et al., 2020).
Circulating DNA-histone complexes and polymorphonuclear neutrophil elastase were significantly increased in diabetic patients with retinopathy. These markers are significant independent risk factors for retinopathy (Park et al., 2016). A subsequent study injected IL-8 or TNF-α into mouse eyes and found neutrophil infiltration that produced NETs. This is evidenced by immunohistochemically positive staining of NE, MPO, and citH3. These markers were also elevated in vitreous samples from patients with PDR and significantly correlated with their severity (Barliya et al., 2017). NE has been shown to contribute to vascular injury in DR (Liu et al., 2019). There is a large body of data linking NETs to inflammatory kidney disease (Odobasic et al., 2007; Lood et al., 2016; Liu and Dong, 2018). A recent study found increased NET deposition in glomeruli in diabetic nephropathy patients and diabetic mice. The predisposition of diabetic mice to glomerulopathy and glomerular endothelial cell (GEC) damage was attenuated by degrading NETs with DNase I (Zheng et al., 2022). Diabetes predisposes neutrophils to the formation of NETs, which impairs wound healing (Wong et al., 2015). Proteomic analysis of diabetic plantar ulcers in the unhealed group showed enrichment of NET-related proteins, including NE, histone H4, and neutrophil proteases. In addition, this study found a positive correlation between protease-3 and the odds of wound infection (Fadini et al., 2016a). CitH3 was identified as a risk factor for impaired wound healing and amputation (Yang et al., 2020).
Although a formal role for neutrophils in the pathogenesis of T2DM has been demonstrated in preclinical modules of diabetes, further studies in humans are required to elucidate whether neutrophils drive the development of T2DM or whether they are a consequence of obesity and hyperglycemia. The controversy over the generation of NETosis in the pathogenesis of T2DM may be due to the heterogeneity of NETs. NETosis may be associated with patient subgroups representing specific T2DM endotypes. Different types of NETs arise in response to different stimuli, have different components, may contribute differently to diabetic complications, and respond differently to anti-NET therapies. Therefore, researchers still need to continue to investigate the exact mechanisms by which NET components contribute to T2DM and its complications.
3.3 Nonalcoholic steatohepatitis
Nonalcoholic steatohepatitis (NASH) is an inflammatory subtype of nonalcoholic fatty liver disease (NAFLD), and it is associated with steatosis as well as hepatocellular injury and inflammation accompanied by varying degrees of liver fibrosis. Accumulation of fat in hepatocytes occurs when fat input or synthesis exceeds fat output or degradation, which is known as steatosis (Machado and Cortez-Pinto, 2014). Triglycerides are the most obvious type of fat in fatty liver (Puri et al., 2007); however, they are not inherently hepatotoxic (Yamaguchi et al., 2007). Other types of lipids that accumulate in the fatty liver may damage hepatocytes (Puri et al., 2007). Hepatocyte damage and death are key features of NASH (Younossi et al., 2011). Damaged hepatocytes can release factors that promote the accumulation of immune cells, produce hepatotoxic substances, and trigger further injury and inflammation. Conversely, factors that promote inflammation also increase hepatocyte exposure to cytokines, gut-derived products, and other inflammatory mediators with hepatotoxic properties (Arrese et al., 2016; Heymann and Tacke, 2016).
Several studies in humans and mice point to a role for neutrophils in NASH. Hepatic neutrophil infiltration is an early event in NASH and can promote inflammation and liver injury (Zhao et al., 2020). Circulating neutrophils correlate with the severity of NASH patients (Antonucci et al., 2020). Patients with NAFLD showed elevated serum NE concentrations and reduced levels of its inhibitor α1-antitrypsin A1AT, resulting in an NE/A1AT imbalance, a ratio associated with NASH progression (Zang et al., 2016). In an NE knockout mouse model fed a Western diet, hepatic steatosis and inflammation are reduced, and NASH is ameliorated (Chen et al., 2019). In addition to NE, plasma MPO levels were higher in NASH mice. MPO deficiency attenuated NASH progression and reduced HFD-induced liver inflammation and fibrosis. This result also suggests an important role of neutrophils in NASH (Rensen et al., 2012).
Several studies have shown that levels of MPO-DNA, markers of NETs, are elevated in patients with NASH and in mouse models of NASH (van der Windt et al., 2018; Du et al., 2022). Another study also found significantly elevated levels of NET markers in the plasma of NASH patients. In addition, this study demonstrated that NETs enhance procoagulant activity in NASH patients (Du et al., 2022). NETs are formed, and the process of NETosis is prolonged in mice fed an MCD-HFD diet for 3 weeks. NETs have also been detected in clinical NASH patients. Inhibition of NETosis can alleviate liver inflammation and liver injury (Wu et al., 2023). In addition, this study demonstrated that NETs did not directly kill neighboring hepatocytes; however, they triggered inflammatory responses in macrophages by upregulating the expression of inflammatory cytokines, including IL-1b, IL-6, and TNF (Wu et al., 2023). A study showed that the progression of hepatocellular carcinoma was also reduced after NETosis inhibition in mice (van der Windt et al., 2018).
3.4 Atherosclerosis
NETs are involved in the formation of arterial thrombosis and venous thrombosis (Baptista de Barros Ribeiro Dourado et al., 2022). Platelets are the most important cells involved in hemostasis and thrombosis. A growing body of literature now expresses the view that there is a complex interaction between neutrophils, platelets and NETs in relation to coagulation (de Bont et al., 2019). NETs act as an adhesion meshwork where platelets are recruited and activated by histones on the net, attaching to the net and to the fibrin coagulation meshwork with larger pore sizes, which becomes the basis for thrombus formation. In turn, fibrin clots mixed with NETs are more resistant to fibrinolytic enzymes in the fibrinolytic system, and NETs are themselves prevented from degrading NET structures due to associated complement binding, such as C1q, by the endogenous DNA lysozyme DNase I, exacerbating the formation of thrombi and associated disease (Joiner et al., 1984; Fujihara et al., 2012; Berends et al., 2014; Morgan, 2016; Dhawan et al., 2021).
In contrast, atherosclerosis model mice show that damage to vascular smooth muscle cells induces neutrophil migration, leading to NETosis production (Silvestre-Roig et al., 2019). In contrast, histone H4 in NETs damages smooth muscle cells, leading to plaque instability and an increased risk of detachment (Silvestre-Roig et al., 2019). NETosis is also exacerbated by atherosclerosis, and the main key points of action may be related to oxidized low-density lipoprotein (ox-LDL) and cholesterol crystals (Awasthi et al., 2016). ox-LDL is produced by ROS and has a pro-NET effect. In turn, NETosis, which is stimulated by cholesterol crystals, prompts macrophages to synthesize precursor molecules for IL (interleukin)-1β (Awasthi et al., 2016). At the same time, cholesterol crystals are endocytosed by macrophages, thereby promoting the maturation of endogenous IL-1β, which is thought to be an intracellular mediator of ox-LDL-induced NETosis. Meanwhile, multiple mechanisms of plaque formation have been shown in case studies related to atherosclerosis to have possible involvement of NETs (Warnatsch et al., 2015). NETs contain related components, such as carbon monoxide synthase, NADPH oxidase and MPO, that oxidatively modify high-density lipoprotein (HDL), affecting the body’s lipid-regulating function and contributing to plaque formation (Warnatsch et al., 2015).
4 Potential targeted drugs
Targeting NETosis therapy is currently the focus of research. Most targeted drugs are still only attempting to target COVID-19 (Zhu et al., 2022). However, there are also a few experiments trying to explain the possibility of modulating NETosis in obesity and its related diseases. This review subsequently explores the targeted therapeutic strategies that are currently being attempted for the treatment of obesity and its related diseases (Table 1).
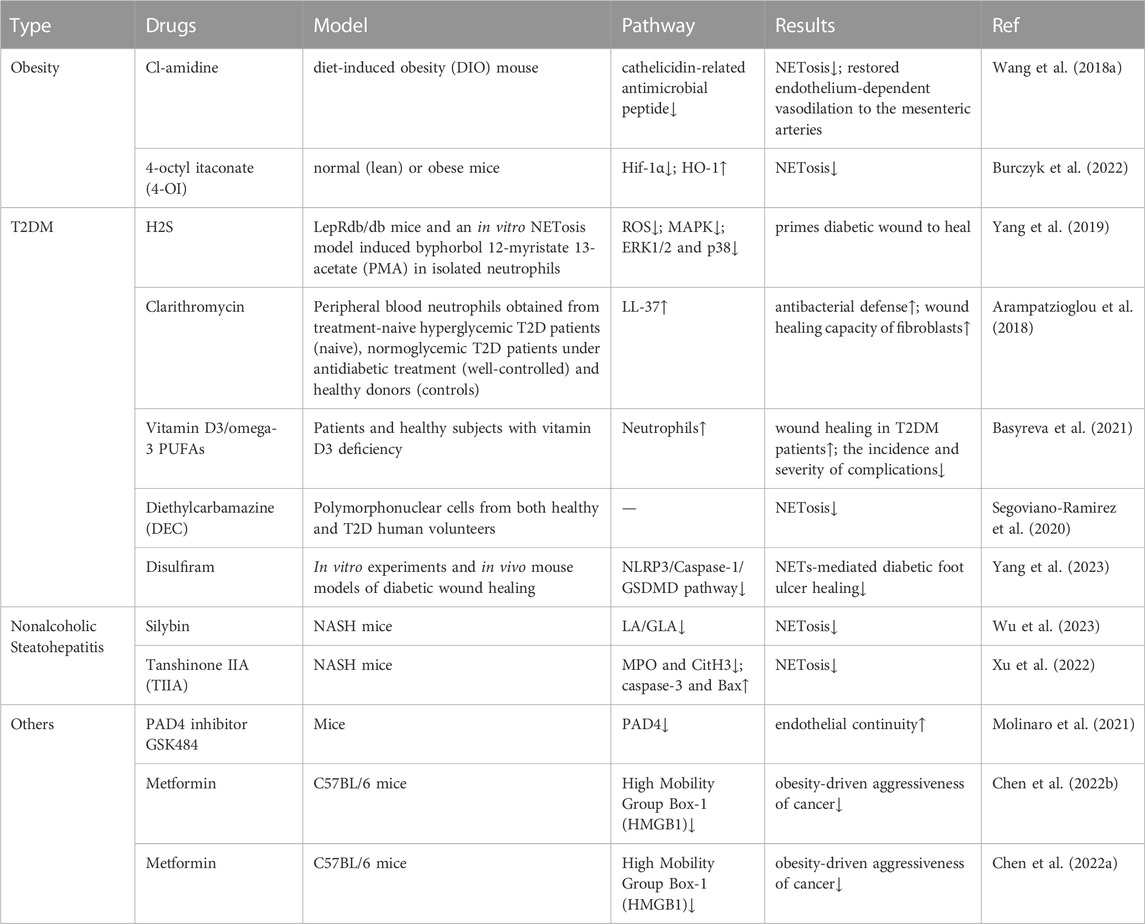
TABLE 1. Drugs for the treatment of obesity and its related metabolic diseases by targeting NETosis.
Cl-Amidine, an inhibitor of peptidyl arginine deiminase 4 (PAD4), specifically inhibits Net (Willis et al., 2011; Knight et al., 2013), and its action is required for histone citrullination during NET formation (Causey et al., 2011; Leshner et al., 2012; Rohrbach et al., 2012). In studying the effects of obesity-associated endothelial dysfunction, researchers evaluated endothelium-dependent vasorelaxation function with angiotensin-converting enzyme (Ach) using a diet-induced obesity (DIO) mouse model. The concentration of plasma monocyte chemotactic protein-1 (MCP-1) was elevated after DIO induction, and Ach-induced vasorelaxation in small mesenteric arteries was impaired. Mice in the DIO group had significantly higher levels of cathelicidin-related antimicrobial peptide (CRIMP), a marker of NET formation, than mice in the standard chow group. Obese mice were treated with Cl-amidine for 2 weeks, and ACh-induced vasorelaxation was significantly improved in DIO mice on chloropyrimidine compared with untreated DIO mice. Therefore, targeting NET formation by Cl-squint may be a feasible approach to treating obesity-associated endothelial dysfunction and inflammation (Wang et al., 2018a).
Itaconic acid is produced endogenously by macrophages in the “broken Krebs cycle” as an intermediate metabolite of lipopolysaccharide processing (Strelko et al., 2011). By exogenously adding 4-octyl itaconate (4-OI), a derivative of itaconate, to normal (lean) or obese mice, researchers found that hypoxia-inducible factor-1α (Hif-1α) was inhibited and heme oxygenase (HO-1) was induced, reducing the formation of Net in normal (lean) or obese mice. This confirms the importance and potential of itaconic acid as a target for NET formation. Detailed testing of the metabolites in vivo animal disease models is still needed (Burczyk et al., 2022).
Hydrogen sulfide (H2S) is widely recognized as an endogenous signaling molecule, and many of its actions are related to antioxidants (Zhao et al., 2013). H2S inhibits the mitogen-activated protein kinase (MAPK) pathway activated by oxidative and reactive oxygen species (ROS) in H9c2 cardiomyogenic myocytes (Dong et al., 2012). Furthermore, in aspirin-treated gastric tissues, H2S inhibited neutrophil aggregation and activation, as well as MPO and ROS production (Yang et al., 2017). In a recent study, researchers used an analog of PKC activation by endogenous diacylglycerol (DAG) to treat human neutrophils with phorbol 12-myristate 13-acetate (PMA), which is significantly increased in diabetic conditions, as a model and found that H2S pretreatment in the form of Na2S resulted in elevated ROS levels. In addition, H2S treatment blocked PMA-induced ERK1/2 and p38 phosphorylation, confirming that H2S treatment could inhibit NET metabolism and NET release from diabetic wounds, thus promoting wound healing and providing clues for H2S targeting NETosis for the treatment of diabetic skin complications. However, in this study, the in vitro study model could not fully mimic the real NETosis process in diabetic mice or patients. This may be a limitation given the differences between humans and mice (Yang et al., 2019).
The macrolide antibiotic clarithromycin has been shown to be a potential immunomodulator and a known inducer of LL-37-bearing NETs (Konstantinidis et al., 2016). A recent study further demonstrated its ability to induce antimicrobial activity in diabetic NET structures in vitro. Researchers collected peripheral blood neutrophils from hyperglycemic T2DM patients at the beginning of treatment (NAIVE), normoglycemic T2DM patients after glucose-lowering therapy (well-controlled), and healthy blood donors (controls) and performed E. coli NCTC 9001 cultures in the presence or absence of neutrophils from naive and well-controlled T2DM patients. After stimulation of NET production with clarithromycin in vitro, LL-37 was found to be significantly more externalized on NET produced by well-controlled T2DM neutrophils. This suggests that clarithromycin may enhance the antimicrobial defense and wound-healing capacity of fibroblasts by upregulating LL-37 expression on NET structures, which may provide a further advantage to patients with well-controlled T2D (Arampatzioglou et al., 2018).
Data reported in the literature suggest that vitamin D3/omega-3 PUFA supplementation significantly reduces ROS/RHS production and increases total serum antioxidant capacity (Razzaghi et al., 2017; Soleimani et al., 2017; Lu et al., 2018; Heshmati et al., 2019). A recent study was conducted by combining vitamin D3 with omega-3 PUFAs (vitamin D3/omega-3 PUFAs) in T2DM patients with septic necrotizing lesions of the lower extremities and healthy subjects, both of whom are deficient in vitamin D3. Using phorbol-12-myristate-13-acetate (PMA) activation of neutrophils in isolated blood as a model of NETosis, it was found that after in vitro whole blood PMA activation of neutrophils, administration of vitamin D3/omega-3 PUFAs completely “turned off “NETosis and neutrophil death. This suggests that supplementation with vitamin D3/omega-3 PUFAs reduces the ability of neutrophils to produce NETs and has a positive effect on wound healing in patients with T2DM, reducing the incidence and severity of complications. However, this preliminary study included only a small number of patients and healthy subjects and should be followed by a double-blind placebo-controlled study with a sufficiently large number of participants (Basyreva et al., 2021).
Decamazin (DEC), a water-soluble derivative of piperazine, is an antiparasitic drug. Some studies have reported the dose-dependent immunomodulatory effects of DEC on phagocytosis in animal models (García-Hernández et al., 2014; Medina-De la Garza et al., 2012). In a recent study, researchers incubated purified polymorphonuclear cells (PMNs) from both healthy and DM2 volunteers with DEC followed by induction of NETosis with PMA and administered DEC 1 h prior to the PMA challenge. It was found that DEC had an immunomodulatory effect in both healthy and DM2 populations by reducing and delaying the formation of NETs from PMNs. This phenomenon reveals that DEC may have clinical application in the control of wounds and plantar ulcers in DM2 patients and provide them with benefits in terms of treatment cost and quality of life (Segoviano-Ramirez et al., 2020).
Disulfiram has been proven to inhibit acetaldehyde dehydrogenase and has been used to treat alcoholism (Koppaka et al., 2012). Previous studies have shown that neutrophils use inflammatory vesicles and GSDMD-dependent mechanisms to activate NETosis as a defense response against cytoplasmic bacteria (Chen et al., 2018). Disulfiram is a potent inhibitor of GSDMD (Hu et al., 2020). In a recent study, researchers found that inhibition of GSDMD by disulfiram eliminated NET formation and thus accelerated diabetic wound healing through in vitro experiments and in vivo diabetic wound healing mouse models. The possible mechanism is through inhibition of the NLRP3/Caspase-1/GSDMD pathway, thereby inhibiting NETs-mediated diabetic foot ulcer healing injury (Yang et al., 2023).
Silybin, derived from silymarin, has multiple pharmacological effects and may alleviate nonalcoholic steatohepatitis (NASH) by reducing lipid accumulation (Sun et al., 2020; Zhang et al., 2021b). In a recent study, researchers intragastrically gavaged (ig) with vehicle or 50 mg/kg silybin every day since the induction of NASH. The results revealed for the first time that silybin-pretreated neutrophils were resistant to linoleic acid (LA)- or γ-linolenic acid (GLA)-mediated NETosis, possibly due to the inhibition of ROS production. Silymarin also inhibited the formation of NETs in NASH animals and alleviated MCD-HFD-induced liver inflammation, steatosis and fibrosis. These results suggest that silybin can alleviate inflammation in NASH while inhibiting NETs. This demonstrates its potential as an important inhibitor of NETs and its therapeutic potential in the treatment of NETosis-related diseases (Wu et al., 2023).
Tanshinone IIA (TIIA) is one of the main active components of the traditional Chinese medicine Salvia miltiorrhiza Bunge. It has been reported to have several pharmacological effects (Tan et al., 2019; Fang et al., 2020b; Fu et al., 2021) and has been widely used in the treatment of cardiovascular diseases (Ansari et al., 2021). Recently, some researchers investigated the therapeutic effects and mechanisms of TIIA on methionine choline deficiency (MCD) diet-induced nonalcoholic steatohepatitis (NASH) in mice. TIIA attenuated inflammatory progression and reduced hepatocyte apoptosis in a mouse model by inhibiting the formation of MPO and CitH3 in the neutrophil extracellular trap network and suppressing caspase-3 and Bax-mediated apoptosis in hepatocytes. These results suggest the potential applicability of TIIA as a therapeutic agent for NASH (Xu et al., 2022).
In the treatment of atherosclerosis, some researchers have developed collagen type IV-targeted nanoparticles (Col IV NPs) that selectively deliver PAD4 inhibitors to areas of endothelial cell collapse and type IV collagen-rich basement membrane exposure. Delivery of the PAD4 inhibitor GSK484 reduced NET accumulation at sites of endothelial injury and maintained endothelial continuity. This result demonstrates a new therapeutic approach to limit plaque erosion in endothelial injury and further supports the role of PAD4 and NETs in superficial erosion, suggesting that the PAD4 inhibitor GSK484 may be a targeted agent for the treatment of atherosclerosis (Molinaro et al., 2021).
In addition to the known hypoglycemic and antidiabetic effects of metformin, studies have shown that metformin has other therapeutic applications in terms of anticancer potential (Zhang et al., 2021a; Kang et al., 2021; Sun et al., 2022). In a recent study, researchers investigated the anticancer effects of metformin using a homologous tumor model established in mouse LLC cells and C57BL/6 mice fed a high-fat diet with obese mice. The results revealed that data from neutrophil studies confirmed the inhibitory effect of metformin on HMGB1-induced network formation. In addition, HMGB1 was identified as a facilitator molecule that promotes the transition process to NETS. These results suggest that metformin plays a role in obesity-induced cancer aggressiveness by inhibiting HMGB1-induced NET formation and may be a potential target drug (Chen et al., 2022b).
5 The role of lifestyle, diet, microbiome, genetics, and environmental factors
NETosis is mediated not only by drugs but also by a variety of other factors. Lifestyle, diet, microbiome, genetics, and environmental factors have been found to play multiple roles in regulating NETosis in obesity and its related diseases and are therefore explored in the following sections. factors are discussed below.
In recent years, the impact of lifestyle-derived risk factors on NETosis has received increasing attention. Poor lifestyles such as unbalanced diet, physical inactivity, smoking, and inadequate sleep quality are associated with NETosis and increase the risk of related diseases. Lack of regular physical activity affects whole immune system function. It has been shown that the levels of lactic acid (LA) can increase up to 20-fold in blood plasma after an acute bout of exhausting exercise (Abebayehu et al., 2016). LA negatively regulates neutrophil NET capacity. In vitro, increased LA concentrations are also associated with reduced rates of NET release and decreased ROS formation (Shi et al., 2019). It is well known that smoking induces oxidative stress in endothelial cells. However, it was recently proposed that smoking also affects the function of immune cell populations. Studies have shown that exposure of mouse and human neutrophils to chronic tobacco smoke induces massive NET formation (Qiu et al., 2017; Albrengues et al., 2018), and neutrophils isolated from smoking subjects showed elevated rates of spontaneous and PMA-induced NETosis (Lee et al., 2017a). Isolated human neutrophils are capable of NETosis via nicotine-stimulated nicotinic acetylcholine receptor (nAChR) stimulation, which may exacerbate inflammatory pathways and induce tissue damage (Hosseinzadeh et al., 2016). Sleep deprivation is believed to be an important risk factor for immune disorders. It has been proven that shortened sleep duration and sleep fragmentation in humans increase neutrophil and monocyte counts in blood and peripheral tissues (Beiter et al., 2014; Carreras et al., 2014; Vallat et al., 2020). However, there are no scientific reports delving into the link between NETs and sleep deprivation, and the mechanisms linking the two remain to be investigated.
High-fat diets have been noted to be associated with NET formation. Studies have shown that neutrophils from high-fat diet (HFD)-fed mice are more likely to spontaneously form NETs in the lungs than neutrophils from low-fat diet-fed animals (Moorthy et al., 2016). Feeding an HFD induces neutrophil recruitment into adipose tissue and increases NET formation (Moorthy et al., 2016; Wang et al., 2018a). NOD1 in HFD-fed mice drives NETosis, which contributes to the regulation of atherogenesis progression (Fernández-García et al., 2022). In recent years, the gut microbiota was found to be strongly associated with NETosis formation. Gut microbiota and its metabolite butyrate significantly reduced neutrophil infiltration and NOX2-dependent NETosis, inflammation, and abnormal phenotypic switching of vascular smooth muscle cells in the aortic wall, which resulted in significant attenuation of abdominal aortic aneurysm development (Tian et al., 2022). In addition, oral butyrate significantly improves and ameliorates mucosal inflammation in inflammatory bowel disease by inhibiting NET formation (Li et al., 2021). It has also been found that gut flora can exacerbate cardiac ischemia/reperfusion (I/R) injury by modulating the formation of NETs (Chen et al., 2022a). Gut flora inhibits NET neutrophil hyperreactivity in mesenteric I/R injury (Ascher et al., 2020).
Increasing evidence suggests that single nucleotide polymorphisms (SNPs) play an important role in the formation of NETs. It was found that neutrophil ROS and NET formation are regulated by the NCF1-339 genotype and that the NCF1-339 SNP-mediated decrease in NADPH oxidase function is associated with high interferon activity and impaired formation of NETs in SLE, permitting a dependence on mitochondrial ROS (Linge et al., 2020). In another study, the C1858T SNP was found to be associated with an increased tendency to form NETs in peripheral blood monocytes with excess citrulline and an increased propensity for spontaneous NET formation and increased the risk of RA by enhancing the spontaneous formation of protein citrulline and NETs (Chang et al., 2015). LNK (R262 W) decreases LNK function in human platelets and neutrophils, promotes NETosis, and increases the risk of coronary artery disease in carriers (Dou et al., 2021). Mendelian randomization is also likely to explain the causal relationship between NETosis and obesity.
The role of environmental factors in regulating NETosis should not be overlooked. Chronic exposure to high levels of fluoride can lead to severe neuropathological damage in the brain. A recent study has shown that fluoride leads to the opening of calcium channels by causing a calcium imbalance in neutrophils, which in turn leads to the opening of L-type calcium channels (LTCCs). Extracellular free iron enters the cell from the open LTCC, leading to neutrophil iron death, which releases NETs and exacerbates neuronal cell inflammation (Wang et al., 2023). UVA and UVB radiation have been shown to induce NET formation in human polymorphonuclear cells (Zawrotniak et al., 2019). NET formation is activated not only by UV-A but also by the three visible light spectra, blue, green, and orange, in a dose-dependent manner. Light-induced NETosis was found to proceed via NADPH oxidase and PAD4 (Arzumanyan et al., 2023). Furthermore, it has been revealed that in an asthma model, PM2.5 aggravates NQO1-induced mucus hypersecretion through the release of NETs (He et al., 2021).
In conclusion, the existing research on the regulation of NETosis in obesity and its related diseases has shed light on the multifaceted roles played by lifestyle, diet, microbiome, genetics, and environmental factors. However, it is important to note that there are certain limitations and gaps in our current understanding. Most studies have primarily focused on individual factors and their impact on NETosis regulation. Future research should aim to investigate the complex interplay and synergistic effects of these factors, as they are likely to interact and influence NETosis in a more comprehensive manner. In the future, a multidisciplinary approach integrating genetics, microbiome, immunology, and environmental sciences will be essential to unravel the complexities of NETosis regulation. This will enable the development of personalized interventions and therapeutic strategies for individuals suffering from obesity and its associated diseases.
6 Conclusion
Extensive research has been conducted on the discovery of NETosis, its mechanism, and its effects on various diseases. Presently, three principal mechanisms of NETosis have been recognized: NOX-dependent NETosis, non-NOX-dependent NETosis, and rapid production-release NETosis. The formation of different types of NETosis may have varying effects depending on the scenario. Although NETosis can be beneficial by entrapping and eliminating pathogens, there is increasing evidence indicating that it also plays a crucial role in diseases such as obesity, T2DM, and NASH. Excessive activation and inappropriate recruitment of NETosis may cause severe organismal damage and disease progression.
However, current research on NETosis is limited, and many challenges remain that require attention. For example, in the study of NEtoSis-related diseases, a standardized method for detecting NETosis is needed. In addition, many clinical studies have small samples, and it is necessary to collect more clinical evidence to confirm the universality of the pathogenic effects of NETosis. In addition, the mechanism of the interaction between NETosis and chronic metabolic diseases is not fully understood, and there is a lack of complete mechanistic studies. Future studies should focus on clarifying and improving the investigation of the mechanism of NETosis in various diseases and how to control the generation and interruption of NETosis. These mechanisms.
As research on NETosis continues to advance, controlling NETs is rapidly becoming a therapeutic target for managing a wide range of diseases. Therefore, the importance of developing targeted drugs cannot be overemphasized. These drugs can regulate NETs by disrupting the formation of NETs or by targeting compounds in NET components such as NNA or NE. Therefore, when developing NETosis-targeted drugs, attention must be paid to the types of NETosis stimulation and changes in signaling pathways under different pathological conditions to more specifically target the disease. It is important to note that the phagocytosis of neutrophils is central to host defense, and any targeted drug against NET formation must avoid impairing the physiological function of these cells. Clinical trials could be designed to test the efficacy of NET interfering drugs in obese patients.
Author contributions
Conceptualization and design, KY and JX; writing–original draft preparation, JL, LY, and SC; writing–review and editing, JD and JW; project administration, KY and JX; funding acquisition, JX. Manuscript revised: JX; All authors contributed to the article and approved the submitted version.
Funding
This work was supported by the Jiangxi Provincial Natural Science Foundation Key Project (20224ACB206010); Key Project of Jiangxi Province Science and Technology Key R&D Plan (20201BBG71006); and Jiangxi Provincial Science and Technology Innovation Base Plan (2020BCG74001 and 20221ZDG02011).
Acknowledgments
The figures were created using online BioRender software (BioRender.com).
Conflict of interest
The authors declare that the research was conducted in the absence of any commercial or financial relationships that could be construed as a potential conflict of interest.
Publisher’s note
All claims expressed in this article are solely those of the authors and do not necessarily represent those of their affiliated organizations, or those of the publisher, the editors and the reviewers. Any product that may be evaluated in this article, or claim that may be made by its manufacturer, is not guaranteed or endorsed by the publisher.
References
Abebayehu, D., Spence, A. J., Qayum, A. A., Taruselli, M. T., Mcleod, J. J., Caslin, H. L., et al. (2016). Lactic acid suppresses IL-33-mediated mast cell inflammatory responses via hypoxia-inducible factor-1α-dependent miR-155 suppression. J. Immunol. 197, 2909–2917. doi:10.4049/jimmunol.1600651
Ahmed, B., Sultana, R., and Greene, M. W. (2021). Adipose tissue and insulin resistance in obese. Biomed. Pharmacother. 137, 111315. doi:10.1016/j.biopha.2021.111315
Alasmari, S. Z. (2020). In Vivo imaging of neutrophil extracellular traps (NETs): visualization methods and outcomes Biomed. Res. Int., 4192745.
Alaygut, D., Ozturk, I., Ulu, S., and Gungor, O. (2023). NETosis and kidney disease: what do we know? Int. Urol. Nephrol. 55, 1985–1994. doi:10.1007/s11255-023-03527-y
Albrengues, J., Shields, M. A., Ng, D., Park, C. G., Ambrico, A., Poindexter, M. E., et al. (2018). Neutrophil extracellular traps produced during inflammation awaken dormant cancer cells in mice. Science 361, eaao4227. doi:10.1126/science.aao4227
Almyroudis, N. G., Grimm, M. J., Davidson, B. A., Rohm, M., Urban, C. F., and Segal, B. H. (2013). NETosis and NADPH oxidase: at the intersection of host defense, inflammation, and injury. Front. Immunol. 4, 45. doi:10.3389/fimmu.2013.00045
Amulic, B., Knackstedt, S. L., Abu Abed, U., Deigendesch, N., Harbort, C. J., Caffrey, B. E., et al. (2017). Cell-cycle proteins control production of neutrophil extracellular traps. Dev. Cell. 43, 449–462 e5. doi:10.1016/j.devcel.2017.10.013
Ansari, M. A., Khan, F. B., Safdari, H. A., Almatroudi, A., Alzohairy, M. A., Safdari, M., et al. (2021). Prospective therapeutic potential of Tanshinone IIA: an updated overview. Pharmacol. Res. 164, 105364. doi:10.1016/j.phrs.2020.105364
Antonucci, L., Porcu, C., Timperi, E., Santini, S. J., Iannucci, G., and Balsano, C. (2020). Circulating neutrophils of nonalcoholic steatohepatitis patients show an activated phenotype and suppress T lymphocytes activity. J. Immunol. Res. 2020, 4570219. doi:10.1155/2020/4570219
Arampatzioglou, A., Papazoglou, D., Konstantinidis, T., Chrysanthopoulou, A., Mitsios, A., Angelidou, I., et al. (2018). Clarithromycin enhances the antibacterial activity and wound healing capacity in type 2 diabetes mellitus by increasing LL-37 load on neutrophil extracellular traps. Front. Immunol. 9, 2064. doi:10.3389/fimmu.2018.02064
Armstrong, A., Jungbluth Rodriguez, K., Sabag, A., Mavros, Y., Parker, H. M., Keating, S. E., et al. (2022). Effect of aerobic exercise on waist circumference in adults with overweight or obesity: a systematic review and meta-analysis. Obes. Rev. 23, e13446. doi:10.1111/obr.13446
Arrese, M., Cabrera, D., Kalergis, A. M., and Feldstein, A. E. (2016). Innate immunity and inflammation in NAFLD/NASH. Dig. Dis. Sci. 61, 1294–1303. doi:10.1007/s10620-016-4049-x
Arzumanyan, G., Mamatkulov, K., Arynbek, Y., Zakrytnaya, D., Jevremović, A., and Vorobjeva, N. (2023). Radiation from UV-A to red light induces ROS-dependent release of neutrophil extracellular traps. Int. J. Mol. Sci. 24, 5770. doi:10.3390/ijms24065770
Ascher, S., Wilms, E., Pontarollo, G., Formes, H., Bayer, F., MüLLER, M., et al. (2020). Gut microbiota restricts NETosis in acute mesenteric ischemia‒reperfusion injury. Arterioscler. Thromb. Vasc. Biol. 40, 2279–2292. doi:10.1161/ATVBAHA.120.314491
Ashwell, M., Gunn, P., and Gibson, S. (2012). Waist-to-height ratio is a better screening tool than waist circumference and BMI for adult cardiometabolic risk factors: systematic review and meta-analysis. Obes. Rev. 13, 275–286. doi:10.1111/j.1467-789X.2011.00952.x
Avogaro, A., Pagnin, E., and Calo, L. (2003). Monocyte NADPH oxidase subunit p22(phox) and inducible hemeoxygenase-1 gene expressions are increased in type II diabetic patients: relationship with oxidative stress. J. Clin. Endocrinol. Metab. 88, 1753–1759. doi:10.1210/jc.2002-021025
Awasthi, D., Nagarkoti, S., Kumar, A., Dubey, M., Singh, A. K., Pathak, P., et al. (2016). Oxidized LDL induced extracellular trap formation in human neutrophils via TLR-PKC-IRAK-MAPK and NADPH-oxidase activation. Free Radic. Biol. Med. 93, 190–203. doi:10.1016/j.freeradbiomed.2016.01.004
Baptista De Barros Ribeiro Dourado, L. P., Santos, M., and Moreira-Goncalves, D. (2022). Nets, pulmonary arterial hypertension, and thrombo-inflammation. J. Mol. Med. Berl. 100, 713–722. doi:10.1007/s00109-022-02197-0
Barbu, E. A., Dominical, V. M., Mendelsohn, L., and Thein, S. L. (2021). An imaging flow cytometry method to measure citrullination of H4 histone as a read-out for neutrophil extracellular traps formation. Bio Protoc. 11, e3927. doi:10.21769/BioProtoc.3927
Barliya, T., Dardik, R., Nisgav, Y., Dachbash, M., Gaton, D., Kenet, G., et al. (2017). Possible involvement of NETosis in inflammatory processes in the eye: evidence from a small cohort of patients. Mol. Vis. 23, 922–932.
Basyreva, L. Y., Vakhrusheva, T. V., Letkeman, Z. V., Maximov, D. I., Fedorova, E. A., Panasenko О, M., et al. (2021). Effect of vitamin D3 in combination with omega-3 polyunsaturated fatty acids on NETosis in type 2 diabetes mellitus patients. Oxid. Med. Cell. Longev. 2021, 8089696. doi:10.1155/2021/8089696
Beiter, T., Fragasso, A., Hudemann, J., Schild, M., Steinacker, J., Mooren, F. C., et al. (2014). Neutrophils release extracellular DNA traps in response to exercise. J. Appl. Physiol. (1985) 117, 325–333. doi:10.1152/japplphysiol.00173.2014
Bengtsson, A. A., Pettersson, A., Wichert, S., Gullstrand, B., Hansson, M., Hellmark, T., et al. (2014). Low production of reactive oxygen species in granulocytes is associated with organ damage in systemic lupus erythematosus. Arthritis Res. Ther. 16, R120. doi:10.1186/ar4575
Berends, E. T., Kuipers, A., Ravesloot, M. M., Urbanus, R. T., and Rooijakkers, S. H. (2014). Bacteria under stress by complement and coagulation. FEMS Microbiol. Rev. 38, 1146–1171. doi:10.1111/1574-6976.12080
Borissoff, J. I., Joosen, I. A., Versteylen, M. O., Brill, A., Fuchs, T. A., Savchenko, A. S., et al. (2013). Elevated levels of circulating DNA and chromatin are independently associated with severe coronary atherosclerosis and a prothrombotic state. Arterioscler. Thromb. Vasc. Biol. 33, 2032–2040. doi:10.1161/ATVBAHA.113.301627
Bravo-Barrera, J., Kourilovitch, M., and Galarza-Maldonado, C. (2017). Neutrophil extracellular traps, antiphospholipid antibodies and treatment. Antibodies (Basel) 6, 4. doi:10.3390/antib6010004
Brinkmann, V., Reichard, U., Goosmann, C., Fauler, B., Uhlemann, Y., Weiss, D. S., et al. (2004). Neutrophil extracellular traps kill bacteria. Science 303, 1532–5.
Burczyk, G., Cichon, I., and Kolaczkowska, E. (2022). Itaconate suppresses formation of neutrophil extracellular traps (NETs): involvement of hypoxia-inducible factor 1α (Hif-1α) and heme oxygenase (HO-1). Front. Immunol. 13, 864638. doi:10.3389/fimmu.2022.864638
Carestia, A., Frechtel, G., Cerrone, G., Linari, M. A., Gonzalez, C. D., Casais, P., et al. (2016). NETosis before and after hyperglycemic control in type 2 diabetes mellitus patients. PLoS One 11, e0168647. doi:10.1371/journal.pone.0168647
Carreras, A., Zhang, S. X., Peris, E., Qiao, Z., Gileles-Hillel, A., Li, R. C., et al. (2014). Chronic sleep fragmentation induces endothelial dysfunction and structural vascular changes in mice. Sleep 37, 1817–1824. doi:10.5665/sleep.4178
Causey, C. P., Jones, J. E., Slack, J. L., Kamei, D., Jones, L. E., Subramanian, V., et al. (2011). The development of N-α-(2-carboxyl)benzoyl-N(5)-(2-fluoro-1-iminoethyl)-l-ornithine amide (o-F-amidine) and N-α-(2-carboxyl)benzoyl-N(5)-(2-chloro-1-iminoethyl)-l-ornithine amide (o-Cl-amidine) as second generation protein arginine deiminase (PAD) inhibitors. J. Med. Chem. 54, 6919–6935. doi:10.1021/jm2008985
Cerf, M. E. (2013). Beta cell dysfunction and insulin resistance. Front. Endocrinol. (Lausanne) 4, 37. doi:10.3389/fendo.2013.00037
Chang, H. H., Dwivedi, N., Nicholas, A. P., and Ho, I. C. (2015). The W620 polymorphism in PTPN22 disrupts its interaction with peptidylarginine deiminase type 4 and enhances citrullination and NETosis. Arthritis Rheumatol. 67, 2323–2334. doi:10.1002/art.39215
Chen, C. J., Wu, C. C., Chang, C. Y., Li, J. R., Ou, Y. C., Chen, W. Y., et al. (2022b). Metformin mitigated obesity-driven cancer aggressiveness in tumor-bearing mice. Int. J. Mol. Sci. 23, 9134. doi:10.3390/ijms23169134
Chen, C., Zhang, H., Xie, R., Wang, Y., and Ma, Y. (2022a). Gut microbiota aggravate cardiac ischemia-reperfusion injury via regulating the formation of neutrophils extracellular traps. Life Sci. 303, 120670. doi:10.1016/j.lfs.2022.120670
Chen, J., Liang, B., Bian, D., Luo, Y., Yang, J., Li, Z., et al. (2019). Knockout of neutrophil elastase protects against western diet induced nonalcoholic steatohepatitis in mice by regulating hepatic ceramides metabolism. Biochem. Biophys. Res. Commun. 518, 691–697. doi:10.1016/j.bbrc.2019.08.111
Chen, K. W., Monteleone, M., Boucher, D., Sollberger, G., Ramnath, D., Condon, N. D., et al. (2018). Noncanonical inflammasome signaling elicits gasdermin D-dependent neutrophil extracellular traps. Sci. Immunol. 3, eaar6676. doi:10.1126/sciimmunol.aar6676
Cichon, I., Ortmann, W., Santocki, M., Opydo-Chanek, M., and Kolaczkowska, E. (2021). Scrutinizing mechanisms of the 'obesity paradox in sepsis': obesity is accompanied by diminished formation of neutrophil extracellular traps (NETs) due to restricted neutrophil-platelet interactions. Cells 10, 384. doi:10.3390/cells10020384
Collaborators, G. B. D. O., Afshin, A., Forouzanfar, M. H., Reitsma, M. B., Sur, P., Estep, K., et al. (2017). Health effects of overweight and obesity in 195 countries over 25 years. N. Engl. J. Med. 377, 13–27. doi:10.1056/NEJMoa1614362
Conejeros, I., Lopez-Osorio, S., Zhou, E., Velasquez, Z. D., Del Rio, M. C., Burgos, R. A., et al. (2022). Glycolysis, monocarboxylate transport, and purinergic signaling are key events in Eimeria bovis-induced NETosis. Front. Immunol. 13, 842482. doi:10.3389/fimmu.2022.842482
Czech, M. P. (2020). Mechanisms of insulin resistance related to white, beige, and brown adipocytes. Mol. Metab. 34, 27–42. doi:10.1016/j.molmet.2019.12.014
D'Abbondanza, M., Martorelli, E. E., Ricci, M. A., De Vuono, S., Migliola, E. N., Godino, C., et al. (2019). Increased plasmatic NETs byproducts in patients in severe obesity. Sci. Rep. 9, 14678. doi:10.1038/s41598-019-51220-x
Dalbeth, N., Gosling, A. L., Gaffo, A., and Abhishek, A. (2021). Gout. Lancet 397, 1843–1855. doi:10.1016/S0140-6736(21)00569-9
De Bont, C. M., Boelens, W. C., and Pruijn, G. J. M. (2019). NETosis, complement, and coagulation: a triangular relationship. Cell. Mol. Immunol. 16, 19–27. doi:10.1038/s41423-018-0024-0
De Bont, C. M., Koopman, W. J. H., Boelens, W. C., and Pruijn, G. J. M. (2018). Stimulus-dependent chromatin dynamics, citrullination, calcium signalling and ROS production during NET formation. Biochim. Biophys. Acta Mol. Cell. Res. 1865, 1621–1629. doi:10.1016/j.bbamcr.2018.08.014
De Lorenzo, A., Soldati, L., Sarlo, F., Calvani, M., Di Lorenzo, N., and Di Renzo, L. (2016). New obesity classification criteria as a tool for bariatric surgery indication. World J. Gastroenterol. 22, 681–703. doi:10.3748/wjg.v22.i2.681
Deng, J., Zhao, F., Zhang, Y., Zhou, Y., Xu, X., Zhang, X., et al. (2020). Neutrophil extracellular traps increased by hyperglycemia exacerbate ischemic brain damage. Neurosci. Lett. 738, 135383. doi:10.1016/j.neulet.2020.135383
Denny, M. F., Yalavarthi, S., Zhao, W., Thacker, S. G., Anderson, M., Sandy, A. R., et al. (2010). A distinct subset of proinflammatory neutrophils isolated from patients with systemic lupus erythematosus induces vascular damage and synthesizes type I IFNs. J. Immunol. 184, 3284–3297. doi:10.4049/jimmunol.0902199
Dhawan, U. K., Bhattacharya, P., Narayanan, S., Manickam, V., Aggarwal, A., and Subramanian, M. (2021). Hypercholesterolemia impairs clearance of neutrophil extracellular traps and promotes inflammation and atherosclerotic plaque progression. Arterioscler. Thromb. Vasc. Biol. 41, 2598–2615. doi:10.1161/ATVBAHA.120.316389
Dong, X. B., Yang, C. T., Zheng, D. D., Mo, L. Q., Wang, X. Y., Lan, A. P., et al. (2012). Inhibition of ROS-activated ERK1/2 pathway contributes to the protection of H2S against chemical hypoxia-induced injury in H9c2 cells. Mol. Cell. Biochem. 362, 149–157. doi:10.1007/s11010-011-1137-2
Dou, H., Kotini, A., Liu, W., Fidler, T., Endo-Umeda, K., Sun, X., et al. (2021). Oxidized phospholipids promote NETosis and arterial thrombosis in LNK(SH2B3) deficiency. Circulation 144, 1940–1954. doi:10.1161/CIRCULATIONAHA.121.056414
Du, J., Zhang, J., Chen, X., Zhang, S., Zhang, C., Liu, H., et al. (2022). Neutrophil extracellular traps induced by pro-inflammatory cytokines enhance procoagulant activity in NASH patients. Clin. Res. Hepatol. Gastroenterol. 46, 101697. doi:10.1016/j.clinre.2021.101697
Eghbalzadeh, K., Georgi, L., Louis, T., Zhao, H., Keser, U., Weber, C., et al. (2019). Compromised anti-inflammatory action of neutrophil extracellular traps in PAD4-deficient mice contributes to aggravated acute inflammation after myocardial infarction. Front. Immunol. 10, 2313. doi:10.3389/fimmu.2019.02313
Elsherif, L., Sciaky, N., Metts, C. A., Modasshir, M., Rekleitis, I., Burris, C. A., et al. (2019). Machine learning to quantitate neutrophil NETosis. Sci. Rep. 9, 16891. doi:10.1038/s41598-019-53202-5
Fabbrini, E., Cella, M., Mccartney, S. A., Fuchs, A., Abumrad, N. A., Pietka, T. A., et al. (2013). Association between specific adipose tissue CD4+ T-cell populations and insulin resistance in obese individuals. Gastroenterology 145, 366–374.e1-3. doi:10.1053/j.gastro.2013.04.010
Fadini, G. P., Menegazzo, L., Rigato, M., Scattolini, V., Poncina, N., Bruttocao, A., et al. (2016a). NETosis delays diabetic wound healing in mice and humans. Diabetes 65, 1061–1071. doi:10.2337/db15-0863
Fadini, G. P., Menegazzo, L., Scattolini, V., Gintoli, M., Albiero, M., and Avogaro, A. (2016b). A perspective on NETosis in diabetes and cardiometabolic disorders. Nutr. Metab. Cardiovasc Dis. 26, 1–8. doi:10.1016/j.numecd.2015.11.008
Fang, X., Henao-Mejia, J., and Henrickson, S. E. (2020a). Obesity and immune status in children. Curr. Opin. Pediatr. 32, 805–815. doi:10.1097/MOP.0000000000000953
Fang, Z. Y., Zhang, M., Liu, J. N., Zhao, X., Zhang, Y. Q., and Fang, L. (2020b). Tanshinone IIA: a review of its anticancer effects. Front. Pharmacol. 11, 611087. doi:10.3389/fphar.2020.611087
Farhan, A., Hassan, G., Ali, S. H. L., Yousaf, Z., Shafique, K., Faisal, A., et al. (2023). Spontaneous NETosis in diabetes: a role of hyperglycemia mediated ROS and autophagy. Front. Med. (Lausanne) 10, 1076690. doi:10.3389/fmed.2023.1076690
FernáNDEZ-GarcíA, V., GonzáLEZ-Ramos, S., AvendañO-Ortiz, J., MartíN-Sanz, P., GóMEZ-Coronado, D., Delgado, C., et al. (2022). High-fat diet activates splenic NOD1 and enhances neutrophil recruitment and neutrophil extracellular traps release in the spleen of ApoE-deficient mice. Cell. Mol. Life Sci. 79, 396. doi:10.1007/s00018-022-04415-x
Flegal, K. M., Kit, B. K., Orpana, H., and Graubard, B. I. (2013). Association of all-cause mortality with overweight and obesity using standard body mass index categories: a systematic review and meta-analysis. Jama 309, 71–82. doi:10.1001/jama.2012.113905
Fousert, E., Toes, R., and Desai, J. (2020). Neutrophil extracellular traps (NETs) take the central stage in driving autoimmune responses. Cells 9, 915. doi:10.3390/cells9040915
Freitas, D. F., ColóN, D. F., Silva, R. L., Santos, E. M., GuimarãES, V. H. D., Ribeiro, G. H. M., et al. (2022). Neutrophil extracellular traps (NETs) modulate inflammatory profile in obese humans and mice: adipose tissue role on NETs levels. Mol. Biol. Rep. 49, 3225–3236. doi:10.1007/s11033-022-07157-y
Fu, K., Feng, C., Shao, L., Mei, L., and Cao, R. (2021). Tanshinone IIA exhibits anti-inflammatory and antioxidative effects in LPS-stimulated bovine endometrial epithelial cells by activating the Nrf2 signaling pathway. Res. Vet. Sci. 136, 220–226. doi:10.1016/j.rvsc.2021.03.004
Fujihara, J., Yasuda, T., Ueki, M., Iida, R., and Takeshita, H. (2012). Comparative biochemical properties of vertebrate deoxyribonuclease I. Comp. Biochem. Physiol. B Biochem. Mol. Biol. 163, 263–273. doi:10.1016/j.cbpb.2012.07.002
Gao, S., Zhang, H., Long, C., and Xing, Z. (2021). Association between obesity and microvascular diseases in patients with type 2 diabetes mellitus. Front. Endocrinol. (Lausanne) 12, 719515. doi:10.3389/fendo.2021.719515
GarcíA-HernáNDEZ, M., Castro-Corona, M. A., Segoviano-RamíREZ, J. C., Brattig, N. W., and Medina-De La Garza, C. E. (2014). Immunomodulatory effect of diethylcarbamazine in mice infected with Nocardia brasiliensis. Int. Immunopharmacol. 23, 113–120. doi:10.1016/j.intimp.2014.08.004
Hamam, H. J., and Palaniyar, N. (2019). Histone deacetylase inhibitors dose-dependently switch neutrophil death from netosis to apoptosis. Biomolecules 9.
He, X., Zhang, L., Xiong, A., Ran, Q., Wang, J., Wu, D., et al. (2021). PM2.5 aggravates NQO1-induced mucus hypersecretion through release of neutrophil extracellular traps in an asthma model. Ecotoxicol. Environ. Saf. 218, 112272. doi:10.1016/j.ecoenv.2021.112272
He, Y., Yang, F. Y., and Sun, E. W. (2018). Neutrophil extracellular traps in autoimmune diseases. Chin. Med. J. Engl. 131, 1513–1519. doi:10.4103/0366-6999.235122
Heshmati, J., Morvaridzadeh, M., Maroufizadeh, S., Akbari, A., Yavari, M., Amirinejad, A., et al. (2019). Omega-3 fatty acids supplementation and oxidative stress parameters: a systematic review and meta-analysis of clinical trials. Pharmacol. Res. 149, 104462. doi:10.1016/j.phrs.2019.104462
Heymann, F., and Tacke, F. (2016). Immunology in the liver-from homeostasis to disease. Nat. Rev. Gastroenterol. Hepatol. 13, 88–110. doi:10.1038/nrgastro.2015.200
Hildreth, A. D., Ma, F., Wong, Y. Y., Sun, R., Pellegrini, M., and O'Sullivan, T. E. (2021). Single-cell sequencing of human white adipose tissue identifies new cell states in health and obesity. Nat. Immunol. 22, 639–653. doi:10.1038/s41590-021-00922-4
Hilscher, M. B., and Shah, V. H. (2020). Small but mighty: platelets in NASH and other chronic liver diseases. Hepatology 71, 1501–1504.
Holmes, C. L., Shim, D., Kernien, J., Johnson, C. J., Nett, J. E., and Shelef, M. A. (2019). Insight into neutrophil extracellular traps through systematic evaluation of citrullination and peptidylarginine deiminases. J. Immunol. Res. 2019, 2160192. doi:10.1155/2019/2160192
Hosseinzadeh, A., Thompson, P. R., Segal, B. H., and Urban, C. F. (2016). Nicotine induces neutrophil extracellular traps. J. Leukoc. Biol. 100, 1105–1112. doi:10.1189/jlb.3AB0815-379RR
Hu, J. J., Liu, X., Xia, S., Zhang, Z., Zhang, Y., Zhao, J., et al. (2020). FDA-approved disulfiram inhibits pyroptosis by blocking gasdermin D pore formation. Nat. Immunol. 21, 736–745. doi:10.1038/s41590-020-0669-6
Huang, J., Hong, W., Wan, M., and Zheng, L. (2022). Molecular mechanisms and therapeutic target of NETosis in diseases. MedComm (2020) 3, e162. doi:10.1002/mco2.162
Ilavská, S., HorváTHOVá, M., Szabová, M., NemessáNYI, T., Jahnová, E., Tulinská, J., et al. (2012). Association between the human immune response and body mass index. Hum. Immunol. 73, 480–485. doi:10.1016/j.humimm.2012.02.023
Joiner, K. A., Brown, E. J., and Frank, M. M. (1984). Complement and bacteria: chemistry and biology in host defense. Annu. Rev. Immunol. 2, 461–491. doi:10.1146/annurev.iy.02.040184.002333
Joosten, L. A., Netea, M. G., Mylona, E., Koenders, M. I., Malireddi, R. K., Oosting, M., et al. (2010). Engagement of fatty acids with Toll-like receptor 2 drives interleukin-1β production via the ASC/caspase 1 pathway in monosodium urate monohydrate crystal-induced gouty arthritis. Arthritis Rheum. 62, 3237–3248. doi:10.1002/art.27667
Jorch, S. K., and Kubes, P. (2017). An emerging role for neutrophil extracellular traps in noninfectious disease. Nat. Med. 23, 279–287. doi:10.1038/nm.4294
Josefs, T., Barrett, T. J., Brown, E. J., Quezada, A., Wu, X., Voisin, M., et al. (2020). Neutrophil extracellular traps promote macrophage inflammation and impair atherosclerosis resolution in diabetic mice. JCI Insight 5, e134796. doi:10.1172/jci.insight.134796
Joshi, M. B., Lad, A., Bharath Prasad, A. S., Balakrishnan, A., Ramachandra, L., and Satyamoorthy, K. (2013). High glucose modulates IL-6 mediated immune homeostasis through impeding neutrophil extracellular trap formation. FEBS Lett. 587, 2241–2246. doi:10.1016/j.febslet.2013.05.053
Kahn, S. E., Prigeon, R. L., Mcculloch, D. K., Boyko, E. J., Bergman, R. N., Schwartz, M. W., et al. (1993). Quantification of the relationship between insulin sensitivity and beta-cell function in human subjects. Evidence for a hyperbolic function. Diabetes 42, 1663–1672. doi:10.2337/diab.42.11.1663
Kang, J., Jeong, S. M., Shin, D. W., Cho, M., Cho, J. H., and Kim, J. (2021). The associations of aspirin, statins, and metformin with lung cancer risk and related mortality: a time-dependent analysis of population-based nationally representative data. J. Thorac. Oncol. 16, 76–88. doi:10.1016/j.jtho.2020.08.021
Kaplan, M. J. (2011). Neutrophils in the pathogenesis and manifestations of SLE. Nat. Rev. Rheumatol. 7, 691–699. doi:10.1038/nrrheum.2011.132
Kapoor, D., and Shukla, D. (2023). Neutrophil extracellular traps and their possible implications in ocular herpes infection. Pathogens 12, 209. doi:10.3390/pathogens12020209
Keshari, R. S., Verma, A., Barthwal, M. K., and Dikshit, M. (2013). Reactive oxygen species-induced activation of ERK and p38 MAPK mediates PMA-induced NETs release from human neutrophils. J. Cell. Biochem. 114, 532–540. doi:10.1002/jcb.24391
Knight, J. S., Zhao, W., Luo, W., Subramanian, V., O'Dell, A. A., Yalavarthi, S., et al. (2013). Peptidylarginine deiminase inhibition is immunomodulatory and vasculoprotective in murine lupus. J. Clin. Invest. 123, 2981–2993. doi:10.1172/JCI67390
Konstantinidis, T., Kambas, K., Mitsios, A., Panopoulou, M., Tsironidou, V., Dellaporta, E., et al. (2016). Immunomodulatory role of clarithromycin in acinetobacter baumannii infection via formation of neutrophil extracellular traps. Antimicrob. Agents Chemother. 60, 1040–1048. doi:10.1128/AAC.02063-15
Koppaka, V., Thompson, D. C., Chen, Y., Ellermann, M., Nicolaou, K. C., Juvonen, R. O., et al. (2012). Aldehyde dehydrogenase inhibitors: a comprehensive review of the pharmacology, mechanism of action, substrate specificity, and clinical application. Pharmacol. Rev. 64, 520–539. doi:10.1124/pr.111.005538
Laridan, E., Denorme, F., Desender, L., FrançOIS, O., Andersson, T., Deckmyn, H., et al. (2017). Neutrophil extracellular traps in ischemic stroke thrombi. Ann. Neurol. 82, 223–232. doi:10.1002/ana.24993
Leclercq, I. A., Da Silva Morais, A., Schroyen, B., Van Hul, N., and Geerts, A. (2007). Insulin resistance in hepatocytes and sinusoidal liver cells: mechanisms and consequences. J. Hepatol. 47, 142–156. doi:10.1016/j.jhep.2007.04.002
Lee, J., Luria, A., Rhodes, C., Raghu, H., Lingampalli, N., Sharpe, O., et al. (2017a). Nicotine drives neutrophil extracellular traps formation and accelerates collagen-induced arthritis. Rheumatol. Oxf. 56, 644–653. doi:10.1093/rheumatology/kew449
Lee, K. H., Kronbichler, A., Park, D. D., Park, Y., Moon, H., Kim, H., et al. (2017b). Neutrophil extracellular traps (NETs) in autoimmune diseases: a comprehensive review. Autoimmun. Rev. 16, 1160–1173. doi:10.1016/j.autrev.2017.09.012
Leshner, M., Wang, S., Lewis, C., Zheng, H., Chen, X. A., Santy, L., et al. (2012). PAD4 mediated histone hypercitrullination induces heterochromatin decondensation and chromatin unfolding to form neutrophil extracellular trap-like structures. Front. Immunol. 3, 307. doi:10.3389/fimmu.2012.00307
Li, G., Lin, J., Zhang, C., Gao, H., Lu, H., Gao, X., et al. (2021). Microbiota metabolite butyrate constrains neutrophil functions and ameliorates mucosal inflammation in inflammatory bowel disease. Gut Microbes 13, 1968257. doi:10.1080/19490976.2021.1968257
Linge, P., Arve, S., Olsson, L. M., Leonard, D., SjöWALL, C., Frodlund, M., et al. (2020). NCF1-339 polymorphism is associated with altered formation of neutrophil extracellular traps, high serum interferon activity and antiphospholipid syndrome in systemic lupus erythematosus. Ann. Rheum. Dis. 79, 254–261. doi:10.1136/annrheumdis-2019-215820
Liu, H., Lessieur, E. M., Saadane, A., Lindstrom, S. I., Taylor, P. R., and Kern, T. S. (2019). Neutrophil elastase contributes to the pathological vascular permeability characteristic of diabetic retinopathy. Diabetologia 62, 2365–2374. doi:10.1007/s00125-019-04998-4
Liu, J., and Dong, Z. (2018). Neutrophil extracellular traps in ischemic AKI: new way to kill. Kidney Int. 93, 303–305. doi:10.1016/j.kint.2017.09.031
Lood, C., Blanco, L. P., Purmalek, M. M., Carmona-Rivera, C., De Ravin, S. S., Smith, C. K., et al. (2016). Neutrophil extracellular traps enriched in oxidized mitochondrial DNA are interferogenic and contribute to lupus-like disease. Nat. Med. 22, 146–153. doi:10.1038/nm.4027
Lu, L., Lu, Q., Chen, W., Li, J., Li, C., and Zheng, Z. (2018). Vitamin D(3) protects against diabetic retinopathy by inhibiting high-glucose-induced activation of the ROS/TXNIP/NLRP3 inflammasome pathway. J. Diabetes Res. 2018, 8193523. doi:10.1155/2018/8193523
Machado, M. V., and Cortez-Pinto, H. (2014). Nonalcoholic fatty liver disease: what the clinician needs to know. World J. Gastroenterol. 20, 12956–12980. doi:10.3748/wjg.v20.i36.12956
Manfredi, A. A., Ramirez, G. A., Rovere-Querini, P., and Maugeri, N. (2018). The neutrophil's choice: phagocytose vs make neutrophil extracellular traps. Front. Immunol. 9, 288. doi:10.3389/fimmu.2018.00288
Maurizi, G., Della Guardia, L., Maurizi, A., and Poloni, A. (2018). Adipocytes properties and crosstalk with immune system in obesity-related inflammation. J. Cell. Physiol. 233, 88–97. doi:10.1002/jcp.25855
Medina-De La Garza, C. E., Guerrero-RamíREZ, G., GarcíA-HernáNDEZ, M., Castro-Corona, M. A., Torres-LóPEZ, E., Brattig, N. W., et al. (2012). Immunomodulatory activity of diethylcarbamazine on humoral, cellular cytokine response and respiratory burst in BALB/c mice. Immunopharmacol. Immunotoxicol. 34, 477–483. doi:10.3109/08923973.2011.630008
Megens, R. T., Vijayan, S., Lievens, D., DöRING, Y., Van Zandvoort, M. A., Grommes, J., et al. (2012). Presence of luminal neutrophil extracellular traps in atherosclerosis. Thromb. Hemost. 107, 597–598. doi:10.1160/TH11-09-0650
Menegazzo, L., Ciciliot, S., Poncina, N., Mazzucato, M., Persano, M., Bonora, B., et al. (2015). NETosis is induced by high glucose and associated with type 2 diabetes. Acta Diabetol. 52, 497–503. doi:10.1007/s00592-014-0676-x
Metzemaekers, M., Gouwy, M., and Proost, P. (2020). Neutrophil chemoattractant receptors in health and disease: double-edged swords. Cell. Mol. Immunol. 17, 433–450. doi:10.1038/s41423-020-0412-0
Mitroulis, I., Kambas, K., Chrysanthopoulou, A., Skendros, P., Apostolidou, E., Kourtzelis, I., et al. (2011). Neutrophil extracellular trap formation is associated with IL-1β and autophagy-related signaling in gout. PLoS One 6, e29318. doi:10.1371/journal.pone.0029318
Miyoshi, A., Yamada, M., Shida, H., Nakazawa, D., Kusunoki, Y., Nakamura, A., et al. (2016). Circulating neutrophil extracellular trap levels in well-controlled type 2 diabetes and pathway involved in their formation induced by high-dose glucose. Pathobiology 83, 243–251. doi:10.1159/000444881
Molinaro, R., Yu, M., Sausen, G., Bichsel, C. A., Corbo, C., Folco, E. J., et al. (2021). Targeted delivery of protein arginine deiminase-4 inhibitors to limit arterial intimal NETosis and preserve endothelial integrity. Cardiovasc Res. 117, 2652–2663. doi:10.1093/cvr/cvab074
Moorthy, A. N., Tan, K. B., Wang, S., Narasaraju, T., and Chow, V. T. (2016). Effect of high-fat diet on the formation of pulmonary neutrophil extracellular traps during influenza pneumonia in BALB/c mice. Front. Immunol. 7, 289. doi:10.3389/fimmu.2016.00289
Morgan, B. P. (2016). The membrane attack complex as an inflammatory trigger. Immunobiology 221, 747–751. doi:10.1016/j.imbio.2015.04.006
Moussa, K., Gurung, P., Adams-Huet, B., Devaraj, S., and Jialal, I. (2019). Increased eosinophils in adipose tissue of metabolic syndrome. J. Diabetes Complicat. 33, 535–538. doi:10.1016/j.jdiacomp.2019.05.010
Ng, L. G., Ostuni, R., and Hidalgo, A. (2019). Heterogeneity of neutrophils. Nat. Rev. Immunol. 19, 255–265. doi:10.1038/s41577-019-0141-8
Nija, R. J., Sanju, S., Sidharthan, N., and Mony, U. (2020). Extracellular trap by blood cells: clinical implications. Tissue Eng. Regen. Med. 17, 141–153.
Odobasic, D., Kitching, A. R., Semple, T. J., and Holdsworth, S. R. (2007). Endogenous myeloperoxidase promotes neutrophil-mediated renal injury, but attenuates T-cell immunity inducing crescentic glomerulonephritis. J. Am. Soc. Nephrol. 18, 760–770. doi:10.1681/ASN.2006040375
Ouchi, N., Parker, J. L., Lugus, J. J., and Walsh, K. (2011). Adipokines in inflammation and metabolic disease. Nat. Rev. Immunol. 11, 85–97. doi:10.1038/nri2921
Park, J. H., Kim, J. E., Gu, J. Y., Yoo, H. J., Park, S. H., Kim, Y. I., et al. (2016). Evaluation of circulating markers of neutrophil extracellular trap (NET) formation as risk factors for diabetic retinopathy in a case‒control association study. Exp. Clin. Endocrinol. Diabetes 124, 557–561. doi:10.1055/s-0042-101792
Petersen, K. F., and Shulman, G. I. (2002). Pathogenesis of skeletal muscle insulin resistance in type 2 diabetes mellitus. Am. J. Cardiol. 90, 11–18. doi:10.1016/s0002-9149(02)02554-7
Pinegin, B., Vorobjeva, N., and Pinegin, V. (2015). Neutrophil extracellular traps and their role in the development of chronic inflammation and autoimmunity. Autoimmun. Rev. 14, 633–40.
Puri, P., Baillie, R. A., Wiest, M. M., Mirshahi, F., Choudhury, J., Cheung, O., et al. (2007). A lipidomic analysis of nonalcoholic fatty liver disease. Hepatology 46, 1081–1090. doi:10.1002/hep.21763
Qiu, S. L., Zhang, H., Tang, Q. Y., Bai, J., He, Z. Y., Zhang, J. Q., et al. (2017). Neutrophil extracellular traps induced by cigarette smoke activate plasmacytoid dendritic cells. Thorax 72, 1084–1093. doi:10.1136/thoraxjnl-2016-209887
Ravindran, M., Khan, M. A., and Palaniyar, N. (2019). Neutrophil extracellular trap formation: physiology, pathology, and pharmacology. Biomolecules 9, 365. doi:10.3390/biom9080365
Razzaghi, R., Pourbagheri, H., Momen-Heravi, M., Bahmani, F., Shadi, J., Soleimani, Z., et al. (2017). The effects of vitamin D supplementation on wound healing and metabolic status in patients with diabetic foot ulcer: a randomized, double-blind, placebo-controlled trial. J. Diabetes Complicat. 31, 766–772. doi:10.1016/j.jdiacomp.2016.06.017
Rensen, S. S., Bieghs, V., Xanthoulea, S., Arfianti, E., Bakker, J. A., Shiri-Sverdlov, R., et al. (2012). Neutrophil-derived myeloperoxidase aggravates nonalcoholic steatohepatitis in low-density lipoprotein receptor-deficient mice. PLoS One 7, e52411. doi:10.1371/journal.pone.0052411
Rhee, H., Love, T., and Harrington, D. (2018). Blood neutrophil count is associated with body mass index in adolescents with asthma. JSM Allergy Asthma 3, 1019.
Roberts, H. M., Grant, M. M., Hubber, N., Super, P., Singhal, R., and Chapple, I. L. C. (2018). Impact of bariatric surgical intervention on peripheral blood neutrophil (PBN) function in obesity. Obes. Surg. 28, 1611–1621. doi:10.1007/s11695-017-3063-1
Rohm, T. V., Meier, D. T., Olefsky, J. M., and Donath, M. Y. (2022). Inflammation in obesity, diabetes, and related disorders. Immunity 55, 31–55. doi:10.1016/j.immuni.2021.12.013
Rohrbach, A. S., Slade, D. J., Thompson, P. R., and Mowen, K. A. (2012). Activation of PAD4 in NET formation. Front. Immunol. 3, 360. doi:10.3389/fimmu.2012.00360
Rosales, C., Lowell, C. A., Schnoor, M., and Uribe-Querol, E. (2017). Neutrophils: their role in innate and adaptive immunity 2017. J. Immunol. Res. 2017, 9748345. doi:10.1155/2017/9748345
Sanchez-Pino, M. D., Richardson, W. S., Zabaleta, J., Puttalingaiah, R. T., Chapple, A. G., Liu, J., et al. (2022). Increased inflammatory low-density neutrophils in severe obesity and effect of bariatric surgery: results from case‒control and prospective cohort studies. EBioMedicine 77, 103910. doi:10.1016/j.ebiom.2022.103910
Schauer, C., Janko, C., Munoz, L. E., Zhao, Y., Kienhofer, D., Frey, B., et al. (2014). Aggregated neutrophil extracellular traps limit inflammation by degrading cytokines and chemokines. Nat. Med. 20, 511–517. doi:10.1038/nm.3547
Segoviano-Ramirez, J. C., Lopez-Altamirano, D. F., Garcia-Juarez, J., Aguirre-Garza, J. E. S., CáRDENAS-Estrada, E., and Ancer-Rodriguez, J. (2020). The diethylcarbamazine delays and decreases the NETosis of polymorphonuclear cells of humans with DM type 2. J. Diabetes Res. 2020, 4827641. doi:10.1155/2020/4827641
Shi, Y., Shi, H., Nieman, D. C., Hu, Q., Yang, L., Liu, T., et al. (2019). Lactic acid accumulation during exhaustive exercise impairs release of neutrophil extracellular traps in mice. Front. Physiol. 10, 709. doi:10.3389/fphys.2019.00709
Silvestre-Roig, C., Braster, Q., Wichapong, K., Lee, E. Y., Teulon, J. M., Berrebeh, N., et al. (2019). Externalized histone H4 orchestrates chronic inflammation by inducing lytic cell death. Nature 569, 236–240. doi:10.1038/s41586-019-1167-6
Singhal, A., Yadav, S., Chandra, T., Mulay, S. R., Gaikwad, A. N., and Kumar, S. (2022). An imaging and computational algorithm for efficient identification and quantification of neutrophil extracellular traps. Cells 11, 191. doi:10.3390/cells11020191
Smyth, L. C. D., Murray, H. C., Hill, M., Van Leeuwen, E., Highet, B., Magon, N. J., et al. (2022). Neutrophil-vascular interactions drive myeloperoxidase accumulation in the brain in Alzheimer's disease. Acta Neuropathol. Commun. 10, 38. doi:10.1186/s40478-022-01347-2
Soleimani, Z., Hashemdokht, F., Bahmani, F., Taghizadeh, M., Memarzadeh, M. R., and Asemi, Z. (2017). Clinical and metabolic response to flaxseed oil omega-3 fatty acids supplementation in patients with diabetic foot ulcer: a randomized, double-blind, placebo-controlled trial. J. Diabetes Complicat. 31, 1394–1400. doi:10.1016/j.jdiacomp.2017.06.010
Sorensen, O. E., and Borregaard, N. (2016). Neutrophil extracellular traps - the dark side of neutrophils. J. Clin. Invest. 126, 1612–1620. doi:10.1172/JCI84538
Steiger, S., and Harper, J. L. (2014). Mechanisms of spontaneous resolution of acute gouty inflammation. Curr. Rheumatol. Rep. 16, 392. doi:10.1007/s11926-013-0392-5
Strelko, C. L., Lu, W., Dufort, F. J., Seyfried, T. N., Chiles, T. C., Rabinowitz, J. D., et al. (2011). Itaconic acid is a mammalian metabolite induced during macrophage activation. J. Am. Chem. Soc. 133, 16386–16389. doi:10.1021/ja2070889
Stumvoll, M., Goldstein, B. J., and Van Haeften, T. W. (2005). Type 2 diabetes: principles of pathogenesis and therapy. Lancet 365, 1333–1346. doi:10.1016/S0140-6736(05)61032-X
Sun, R., Xu, D., Wei, Q., Zhang, B., Aa, J., Wang, G., et al. (2020). Silybin ameliorates hepatic lipid accumulation and modulates global metabolism in an NAFLD mouse model. Biomed. Pharmacother. 123, 109721. doi:10.1016/j.biopha.2019.109721
Sun, X., Dong, M., Gao, Y., Wang, Y., Du, L., Liu, Y., et al. (2022). Metformin increases the radiosensitivity of non-small cell lung cancer cells by destabilizing NRF2. Biochem. Pharmacol. 199, 114981. doi:10.1016/j.bcp.2022.114981
Sung, P. S., Peng, Y. C., Yang, S. P., Chiu, C. H., and Hsieh, S. L. (2022). CLEC5A is critical in Pseudomonas aeruginosa-induced NET formation and acute lung injury. JCI Insight 7, e156613. doi:10.1172/jci.insight.156613
Talukdar, S., Oh, D. Y., Bandyopadhyay, G., Li, D., Xu, J., Mcnelis, J., et al. (2012). Neutrophils mediate insulin resistance in mice fed a high-fat diet through secreted elastase. Nat. Med. 18, 1407–1412. doi:10.1038/nm.2885
Takei, S., Fernandez, D., Redford, A., and Toyoda, H. (1996). Methylation status of 5'-regulatory region of tumor necrosis factor alpha gene correlates with differentiation stages of monocytes. Biochem. Biophys. Res. Commun. 220, 606–12.
Tan, Y. L., Ou, H. X., Zhang, M., Gong, D., Zhao, Z. W., Chen, L. Y., et al. (2019). Tanshinone IIA promotes macrophage cholesterol efflux and attenuates atherosclerosis of apoE-/- mice by omentin-1/ABCA1 pathway. Curr. Pharm. Biotechnol. 20, 422–432. doi:10.2174/1389201020666190404125213
Tian, Z., Zhang, Y., Zheng, Z., Zhang, M., Zhang, T., Jin, J., et al. (2022). Gut microbiome dysbiosis contributes to abdominal aortic aneurysm by promoting neutrophil extracellular trap formation. Cell. Host Microbe 30, 1450–1463.e8. doi:10.1016/j.chom.2022.09.004
Tomas-Perez, S., Oto, J., Aghababyan, C., Herranz, R., Cuadros-Lozano, A., Gonzalez-Canto, E., et al. (2023). Increased levels of NETosis biomarkers in high-grade serous ovarian cancer patients' biofluids: potential role in disease diagnosis and management. Front. Immunol. 14, 1111344. doi:10.3389/fimmu.2023.1111344
Valeria Oliveira De Sousa, B., De Freitas, D. F., Monteiro-Junior, R. S., Mendes, I. H. R., Sousa, J. N., GuimarãES, V. H. D., et al. (2021). Physical exercise, obesity, inflammation and neutrophil extracellular traps (NETs): a review with bioinformatics analysis. Mol. Biol. Rep. 48, 4625–4635. doi:10.1007/s11033-021-06400-2
Vallat, R., Shah, V. D., Redline, S., Attia, P., and Walker, M. P. (2020). Broken sleep predicts hardened blood vessels. PLoS Biol. 18, e3000726. doi:10.1371/journal.pbio.3000726
Van Der Windt, D. J., Sud, V., Zhang, H., Varley, P. R., Goswami, J., Yazdani, H. O., et al. (2018). Neutrophil extracellular traps promote inflammation and development of hepatocellular carcinoma in nonalcoholic steatohepatitis. Hepatology 68, 1347–1360. doi:10.1002/hep.29914
Villanueva, E., Yalavarthi, S., Berthier, C. C., Hodgin, J. B., Khandpur, R., Lin, A. M., et al. (2011). Netting neutrophils induce endothelial damage, infiltrate tissues, and expose immunostimulatory molecules in systemic lupus erythematosus. J. Immunol. 187, 538–552. doi:10.4049/jimmunol.1100450
Volkman, R., Ben-Zur, T., Kahana, A., Garty, B. Z., and Offen, D. (2019). Myeloperoxidase deficiency inhibits cognitive decline in the 5XFAD mouse model of Alzheimer's disease. Front. Neurosci. 13, 990. doi:10.3389/fnins.2019.00990
Von Kockritz-Blickwede, M., and Winstel, V. (2022). Molecular prerequisites for neutrophil extracellular trap formation and evasion mechanisms of Staphylococcus aureus. Front. Immunol. 13, 836278. doi:10.3389/fimmu.2022.836278
Wang, D., Yin, K., Zhang, Y., Lu, H., Hou, L., Zhao, H., et al. (2023). Fluoride induces neutrophil extracellular traps and aggravates brain inflammation by disrupting neutrophil calcium homeostasis and causing ferroptosis. Environ. Pollut. 331, 121847. doi:10.1016/j.envpol.2023.121847
Wang, H., Wang, Q., Venugopal, J., Wang, J., Kleiman, K., Guo, C., et al. (2018a). Obesity-induced endothelial dysfunction is prevented by neutrophil extracellular trap inhibition. Sci. Rep. 8, 4881. doi:10.1038/s41598-018-23256-y
Wang, L., Zhou, X., Yin, Y., Mai, Y., Wang, D., and Zhang, X. (2018b). Hyperglycemia induces neutrophil extracellular traps formation through an NADPH oxidase-dependent pathway in diabetic retinopathy. Front. Immunol. 9, 3076. doi:10.3389/fimmu.2018.03076
Warnatsch, A., Ioannou, M., Wang, Q., and Papayannopoulos, V. (2015). Inflammation. Neutrophil extracellular traps license macrophages for cytokine production in atherosclerosis. Science 349, 316–320. doi:10.1126/science.aaa8064
Willis, V. C., Gizinski, A. M., Banda, N. K., Causey, C. P., Knuckley, B., Cordova, K. N., et al. (2011). N-α-Benzoyl-N5-(2-Chloro-1-Iminoethyl)-l-Ornithine amide, a protein arginine deiminase inhibitor, reduces the severity of murine collagen-induced arthritis. J. Immunol. 186, 4396–4404. doi:10.4049/jimmunol.1001620
Wirestam, L., Arve, S., Linge, P., and Bengtsson, A. A. (2019). Neutrophils-important communicators in systemic lupus erythematosus and antiphospholipid syndrome. Front. Immunol. 10, 2734. doi:10.3389/fimmu.2019.02734
Wong, S. L., Demers, M., Martinod, K., Gallant, M., Wang, Y., Goldfine, A. B., et al. (2015). Diabetes primes neutrophils to undergo NETosis, which impairs wound healing. Nat. Med. 21, 815–819. doi:10.1038/nm.3887
Wong, S. L., and Wagner, D. D. (2018). Peptidylarginine deiminase 4: a nuclear button triggering neutrophil extracellular traps in inflammatory diseases and aging. Faseb j. 32, fj201800691R.
Wu, H., Ghosh, S., Perrard, X. D., Feng, L., Garcia, G. E., Perrard, J. L., et al. (2007). T-cell accumulation and regulated on activation, normal T-cell expressed and secreted upregulation in adipose tissue in obesity. Circulation 115, 1029–1038. doi:10.1161/CIRCULATIONAHA.106.638379
Wu, J., Zhang, C., He, T., Zhang, S., Wang, Y., Xie, Z., et al. (2023). Polyunsaturated fatty acids drive neutrophil extracellular trap formation in nonalcoholic steatohepatitis. Eur. J. Pharmacol. 945, 175618. doi:10.1016/j.ejphar.2023.175618
Xu, L., Liu, X., Jia, T., Sun, Y., Du, Y., Wei, S., et al. (2022). Tanshinone IIA ameliorates nonalcoholic steatohepatitis in mice by modulating neutrophil extracellular traps and hepatocyte apoptosis. Evid. Based Complement. Altern. Med. 2022, 5769350. doi:10.1155/2022/5769350
Yamaguchi, K., Yang, L., Mccall, S., Huang, J., Yu, X. X., Pandey, S. K., et al. (2007). Inhibiting triglyceride synthesis improves hepatic steatosis but exacerbates liver damage and fibrosis in obese mice with nonalcoholic steatohepatitis. Hepatology 45, 1366–1374. doi:10.1002/hep.21655
Yang, C. T., Chen, L., Chen, W. L., Li, N., Chen, M. J., Li, X., et al. (2019). Hydrogen sulfide primes diabetic wound to close through inhibition of NETosis. Mol. Cell. Endocrinol. 480, 74–82. doi:10.1016/j.mce.2018.10.013
Yang, C. T., Lai, Z. Z., Zheng, Z. H., Kang, J. M., Xian, M., Wang, R. Y., et al. (2017). A novel pH-controlled hydrogen sulfide donor protects gastric mucosa from aspirin-induced injury. J. Cell. Mol. Med. 21, 2441–2451. doi:10.1111/jcmm.13166
Yang, H., Youm, Y. H., Vandanmagsar, B., Ravussin, A., Gimble, J. M., Greenway, F., et al. (2010). Obesity increases the production of proinflammatory mediators from adipose tissue T cells and compromises TCR repertoire diversity: implications for systemic inflammation and insulin resistance. J. Immunol. 185, 1836–1845. doi:10.4049/jimmunol.1000021
Yang, S., Feng, Y., Chen, L., Wang, Z., Chen, J., Ni, Q., et al. (2023). Disulfiram accelerates diabetic foot ulcer healing by blocking NET formation via suppressing the NLRP3/Caspase-1/GSDMD pathway. Transl. Res. 254, 115–127. doi:10.1016/j.trsl.2022.10.008
Yang, S., Gu, Z., Lu, C., Zhang, T., Guo, X., Xue, G., et al. (2020). Neutrophil extracellular traps are markers of wound healing impairment in patients with diabetic foot ulcers treated in a multidisciplinary setting. Adv. Wound Care (New Rochelle) 9, 16–27. doi:10.1089/wound.2019.0943
You, Q., He, D. M., Shu, G. F., Cao, B., Xia, Y. Q., Xing, Y., et al. (2019). Increased formation of neutrophil extracellular traps is associated with gut leakage in patients with type 1 but not type 2 diabetes. J. Diabetes 11, 665–673. doi:10.1111/1753-0407.12892
Younossi, Z. M., Stepanova, M., Rafiq, N., Makhlouf, H., Younoszai, Z., Agrawal, R., et al. (2011). Pathologic criteria for nonalcoholic steatohepatitis: interprotocol agreement and ability to predict liver-related mortality. Hepatology 53, 1874–1882. doi:10.1002/hep.24268
Zang, S., Ma, X., Zhuang, Z., Liu, J., Bian, D., Xun, Y., et al. (2016). Increased ratio of neutrophil elastase to α1-antitrypsin is closely associated with liver inflammation in patients with nonalcoholic steatohepatitis. Clin. Exp. Pharmacol. Physiol. 43, 13–21. doi:10.1111/1440-1681.12499
Zawrotniak, M., Bartnicka, D., and Rapala-Kozik, M. (2019). UVA and UVB radiation induce the formation of neutrophil extracellular traps by human polymorphonuclear cells. J. Photochem Photobiol. B 196, 111511. doi:10.1016/j.jphotobiol.2019.111511
Zhang, K., Bai, P., Dai, H., and Deng, Z. (2021a). Metformin and risk of cancer among patients with type 2 diabetes mellitus: a systematic review and meta-analysis. Prim. Care Diabetes 15, 52–58. doi:10.1016/j.pcd.2020.06.001
Zhang, R., Xu, D., Zhang, Y., Wang, R., Yang, N., Lou, Y., et al. (2021b). Silybin restored CYP3A expression through the sirtuin 2/nuclear factor κ-B pathway in mouse nonalcoholic fatty liver disease. Drug Metab. Dispos. 49, 770–779. doi:10.1124/dmd.121.000438
Zhao, X., Yang, L., Chang, N., Hou, L., Zhou, X., Yang, L., et al. (2020). Neutrophils undergo switch of apoptosis to NETosis during murine fatty liver injury via S1P receptor 2 signaling. Cell. Death Dis. 11, 379. doi:10.1038/s41419-020-2582-1
Zhao, Y., Bhushan, S., Yang, C., Otsuka, H., Stein, J. D., Pacheco, A., et al. (2013). Controllable hydrogen sulfide donors and their activity against myocardial ischemia‒reperfusion injury. ACS Chem. Biol. 8, 1283–1290. doi:10.1021/cb400090d
Zharkova, O., Tay, S. H., Lee, H. Y., Shubhita, T., Ong, W. Y., Lateef, A., et al. (2019). A flow cytometry-based assay for high-throughput detection and quantification of neutrophil extracellular traps in mixed cell populations. Cytom. A 95, 268–278. doi:10.1002/cyto.a.23672
Zheng, F., Ma, L., Li, X., Wang, Z., Gao, R., Peng, C., et al. (2022). Neutrophil extracellular traps induce glomerular endothelial cell dysfunction and pyroptosis in diabetic kidney disease. Diabetes 71, 2739–2750. doi:10.2337/db22-0153
Zhou, Y., An, L. L., Chaerkady, R., Mittereder, N., Clarke, L., Cohen, T. S., et al. (2018). Evidence for a direct link between PAD4-mediated citrullination and the oxidative burst in human neutrophils. Sci. Rep. 8, 15228. doi:10.1038/s41598-018-33385-z
Keywords: neutrophil extracellular trap networks, NETosis, obesity, nonalcoholic steatohepatitis, diabetes, atherosclerosis
Citation: Li J, Yin L, Chen S, Li Z, Ding J, Wu J, Yang K and Xu J (2023) The perspectives of NETosis on the progression of obesity and obesity-related diseases: mechanisms and applications. Front. Cell Dev. Biol. 11:1221361. doi: 10.3389/fcell.2023.1221361
Received: 12 May 2023; Accepted: 04 August 2023;
Published: 15 August 2023.
Edited by:
Yilei Zhang, Xi’an Jiaotong University, ChinaReviewed by:
Triet M. Bui, Dana–Farber Cancer Institute, United StatesMadankumar Ghatge, The University of Iowa, United States
Copyright © 2023 Li, Yin, Chen, Li, Ding, Wu, Yang and Xu. This is an open-access article distributed under the terms of the Creative Commons Attribution License (CC BY). The use, distribution or reproduction in other forums is permitted, provided the original author(s) and the copyright owner(s) are credited and that the original publication in this journal is cited, in accordance with accepted academic practice. No use, distribution or reproduction is permitted which does not comply with these terms.
*Correspondence: Kangping Yang, eWFuZ2thbmdwaW5nMDkxM0AxNjMuY29t; Jixiong Xu, aml4aW9uZy54dUBuY3UuZWR1LmNu
†These authors have contributed equally to this work and share first authorship