- Key Laboratory for Major Obstetric Diseases of Guangdong Province, Department of Obstetrics and Gynecology, Key Laboratory of Reproduction and Genetics of Guangdong Higher Education Institutes, The Third Affiliated Hospital of Guangzhou Medical University, Guangzhou, Guangdong, China
An increasing number of men require long-term drug therapy for various diseases. However, the effects of long-term drug therapy on male fertility are often not well evaluated in clinical practice. Meanwhile, the development of stem cell therapy and exosomes treatment methods may provide a new sight on treating male infertility. This article reviews the influence and mechanism of small molecule medications on male fertility, as well as progress of stem cell and exosomes therapy for male infertility with the purpose on providing suggestions (recommendations) for evaluating the effect of drugs on male fertility (both positive and negative effect on male fertility) in clinical application and providing strategies for diagnosis and treatment of male infertility.
1 Introduction
In clinical practice, many reproductive-aged men require long-term drug therapy for various diseases, such as cardiovascular disease, tumor and chemoradiotherapy, hyperlipemia, depressive disorder and so on. However, the effects of drug therapy on male fertility are often not well evaluated. Drugs may affect male fertility by direct gonadal toxicity, altering the hypothalamic-pituitary-gonadal axis, causing sexual dysfunction, and negative effects on libido. Whereas, some small molecule drugs may have a positive protective effect on germ cell genesis and fertility maintenance during use. Thus, it is necessary to evaluate the reproductive effects before drugs approved for clinical use. Stem cell therapy and exosomes therapy are promising treatment options for male infertility. Mesenchymal stem cells (MSCs) and their exosomes have attracted much attention due to their anti-inflammatory and immunomodulatory properties, trophic properties, and anti-apoptotic properties, as well as their easy availability. This article mainly discussed the direct effect of drugs on male germ cell and its mechanism and summarized the therapeutic effect of stem cells and extracellular vesicles in this regard.
2 Discussion
Different small molecule medications have different pharmacological mechanisms and have varying effects on male fertility. Among the effects on male fertility, small molecule medications may affect male fertility by influencing testicular microenvironment, spermatogenesis, sperm capacitation, fertilization. The mechanism may not be singular. This article attempts to review the research on the mechanisms of action of small molecule medications at different stages of sperm development to fertilization, and to summarize the prospects and obstacles of stem cell therapy and exosomes in male fertility.
3 Conclusion
The influence of clinical drugs on male fertility is complex and needs comprehensive evaluation. Further researches are needed to better understand the impact of drugs on male fertility and provide evidence-based recommendations for clinical use. Although there are many problems that remains to be solved when using MSCs and exosomes in clinical practice, the continuous deepening of research and treatment methods may become emerging methods for treating male infertility and fertility preservation.
4 Main text
4.1 The effects of small molecule medications on male fertility
Fertility is defined as the capacity to establish clinical pregnancy. According to WHO, infertility refers to the inability to conceive after 12 months of regular unprotected sexual intercourse (Zegers-Hochschild et al., 2017). Between 8% and 12% of reproductive-aged couples are affected by infertility. 50% of couples have male factors in infertility (Vander and Wyns., 2018). The cause of male infertility can be divided into congenital, acquired, and idiopathic. The use of small molecule medications is one of the causes of male infertility (Agarwal et al., 2021). Long term drug treatment may damage spermatogenesis, change semen parameters, and lead to sexual dysfunction (Fode et al., 2021). The quantity and quality of sperm are important indicators for evaluating male fertility (Goossens and Tournaye., 2014), so reproductive history and semen analysis are necessary for the initial evaluation of male fertility. For males with initial evaluation abnormalities, further comprehensive evaluation by reproductive experts may be required (Agarwal et al., 2021). The main methods for treating male infertility include medication to improve sperm quality, application of assisted reproductive technology, treatment of diseases that lead to infertility, and the therapeutic effect of stem cells and their extracellular vesicles on male infertility (Agarwal et al., 2021).
4.1.1 The effect of small molecule medications on testicular microenvironment
The main function of the scrotum-located testicles is to produce sperm and androgens. Spermatogenesis is a complex process of producing sperm, while the testicular microenvironment is crucial to the normal development of spermatogenesis (Horvath-Pereira et al., 2023). It regulates the proliferation, differentiation, and maturation of germ cell (Miyaso et al., 2022). The testicular microenvironment is composed of testicular cells and intercellular substance. Changes in immune homeostasis (Miyaso et al., 2022; Wang et al., 2018), somatic cells in testis (Zhao et al., 2020), blood–testis barrier, temperature, and the paracrine factors may all contribute to changes in the testicular microenvironment.
4.1.1.1 Testicular weight
During long-term drug therapy for various diseases, some medications may cause significant changes in testicular weight. The changes in testicular weight are also an indicator for evaluating the impact of drugs on male fertility (Figure 1A). In animal experiments, it has been found that fluoxetine, amlodipine, acetaminophen and nifedipine can decrease testicular weight (de Oliveira et al., 2013; Iranloye et al., 2009; Latif et al., 2008; Vieira et al., 2013; Wiger et al., 1995). Benzodiazepines as a sedative hypnotic drug have a negative impact on male fertility (Cook et al., 1979; Kar and Das., 1983; Means et al., 1982; Sanbuissho et al., 1995; Taher et al., 2019), but their impact on testicular weight is uncertain. Some experiments have shown that they do not cause changes in testicular weight (Chengelis et al., 1986), while others have shown that they can cause a decrease in testicular weight (Cook et al., 1979; Kar and Das., 1983; Means et al., 1982; Sanbuissho et al., 1995; Taher et al., 2019). Indometacin which used for pain relief and anti-inflammatory reduces or does not change testicular weight (Bagoji et al., 2017; Saksena et al., 1975). Statins which have lipid-lowering effect can improve testicular weight loss caused by hyperlipidemia (Shalaby et al., 2004), while Köhler-Samouilidis et al. found that Captopril which used to treat hypertension can increase testicular weight, although the study found that it has a negative impact on male fertility (Köhler-Samouilidis et al., 1997). The change of testicular weight is an indicator to evaluate the effect of drugs on male fertility in animal experiments, but it is not accurate, and needs to be evaluated together with other indicators. Table 1.

FIGURE 1. Effects and mechanisms of small molecule medications on male fertility. Small molecule medications effects on testicular weight (A). testicular microenvironment (B). spermatogenesis (C). sperm capacitation and fertilization (D). The targeted stages of medications, medicine categories, effects mechanisms, etc. are detailed in Tables 1–4.
4.1.1.2 Testicular microenvironment
Apart from the testicular weight changes, testicular microenvironment also has a series of impact on spermatogenesis (Figure 1B). The use of common clinical drugs may lead to changes in the testicular microenvironment and thus affect spermatogenesis. In animal studies, it was found that bupropion (Yardimci et al., 2019), paroxetine (Erdemir et al., 2014; Yakubu and Atoyebi., 2018; Yardimci et al., 2019), benzodiazepines (Chengelis et al., 1986; Means et al., 1982), nifedipine (Iranloye et al., 2009; Lee et al., 2006), ethosuximide (Lee et al., 2006), valproate (Alsemeh et al., 2022; Nishimura et al., 2000; RØste et al., 2001; Sukhorum and Lamsaard., 2017), pregabalin (Shokry et al., 2020; Taha et al., 2020), rosuvastatin (Leite et al., 2017), etc., had negative effects on the testicular microenvironment. Antidepressant bupropion can cause testicular structural damage and interstitial edema (Yardimci et al., 2019). As a kind of selective serotonin reuptake inhibitors (SSRI), paroxetine can damage testicular structure, induce degeneration of seminiferous tubules, and cause vacuolization of germinal epithelium (Erdemir et al., 2014; Yakubu and Atoyebi, 2018; Yardimci et al., 2019). Meanwhile, there are differences in results among different studies on benzodiazepines. This may be related to the dosage used in animal experiments. When the dosage is 75 mg/kg body weight (Chengelis et al., 1986), it will cause spermatogenesis arrest and the germinal epithelium degeneration, but it will not cause abnormalities in Sertoli cells and Leydig cells. However, when the dosage is 100 mg/kg (Means et al., 1982), it will cause degeneration and necrosis of seminiferous tubules, and vacuolization of interstitial cells.
Calcium channel blockers which used for cardiovascular diseases are commonly believed to cause male infertility (Drobnis and Nangia, 2017). Voltage-gated Ca2+ (CaV) channels can be divided into different types of voltage-gated channel according to pharmacology. As antihypertensive drugs, Calcium channel blockers include L-type and T-type voltage-gated calcium channel inhibitor (Elmslie, 2004). It found that L-type and T-type voltage-gated calcium channel exist in male germ cell. However, the specific regulatory mechanism for male germ cell is not clear. In a study on the effect of nifedipine on male reproductive function in rats, after continuous administration of 0.57 mg/kg nifedipine for 30 days, no significant effects were observed on testicular histology (Iranloye et al., 2009). However, another study on male mice showed that high doses (100 mg/kg) of nifedipine or ethosuximide could cause immature development of seminiferous tubules, spermatogenic stagnated in the elongating spermatid stage, and poorly developed lumen. In the study, it was found that the mRNA expression of the transcription factor cAMP-responsive element modulator (CREM) activator isoform in the testes of experimental mice was increased together with the mRNA expression of transition protein 2 and protamine 2. It is suggested that the spermatogenic disorders caused by calcium channel blockers may be related to the ectopic expression of CREM-dependent genes in the testes (Lee et al., 2006). The difference in these two study results may also be related to different drug doses and durations of use. As an Antiepileptic drugs (AEDs), Valproic acid can lead to spermatogenic disorders (RØste et al., 2001), evidenced by degeneration of seminiferous tubules and loss and shedding of spermatogenic cells under microscopy (Nishimura et al., 2000). In addition, collagen deposition, widening of interstitial spaces, congestion of the tunica propria and interstitial ducts (Alsemeh et al., 2022), an increase in the percentage of spermatozoa with abnormal acrosomes (Sukhorum and Lamsaard., 2017), and the generation of multinucleated giant cells have been observed (Iamsaard et al., 2017). Studies have shown that valproic acid can induce tissue pathological damage associated with autophagy by modulating the AMPK/mTOR signaling pathway (Alsemeh et al., 2022). And another type of AEDs Pregabalin can reduce the number of germ cells while increasing the number of interstitial cells, and cause a decrease in the number and diameter of convoluted tubules (Salem et al., 2020). It also induces cell apoptosis, increases caspase-3 expression in the testis (Shokry et al., 2020), upregulates pro-apoptotic genes BAX and p38 MAPK, and downregulates the anti-apoptotic gene BCL2 (Taha et al., 2020). The reproductive toxicity of pregabalin may be related to the p38 MAPK and BAX/BCL2 signaling pathways (Taha et al., 2020).
4.1.1.3 Oxidative stress
Oxidative stress is a cause of male infertility (Sharma et al., 2023). Increasing cellular oxidative stress is a pathway through which antiepileptic drugs damage male reproductive cells. Studies have shown that valproic acid, pregabalin, cannabidiol, and levetiracetam all increase oxidative stress in male reproductive cells (Baysal et al., 2017; Carvalho et al., 2022; Naderi et al., 2021; Ourique et al., 2016a; Ourique et al., 2016b; Shokry et al., 2020; Taha et al., 2020). Histologically, after administration of cannabidiol, the number of Sertoli cells will decrease (Carvalho et al., 2018), and testicular degeneration will occur (Patra and Wadsworth., 1991). The antidepressant drugs sertraline, fluoxetine, and citalopram also increase oxidative stress in the testes (Atli et al., 2017; Attia and Bakheet., 2013; Sakr et al., 2015). Taking fluoxetine during pregnancy and postpartum also has an impact on male reproductive health. Male offspring may exhibit reduced Sertoli cells in histology, decreased height of germinal epithelium, decreased diameter of seminiferous tubules, shorter epididymal tubules, and increased number of tubules without a lumen (de Oliveira et al., 2013; Ramos et al., 2015; Vieira et al., 2013). In animal experiments, it has been found that citalopram inhibits sperm production at different stages, leading to degeneration of seminiferous tubules, cell vacuolization, interstitial cell atrophy, and decreased sperm count in the lumen (Ilgin et al., 2017; Prasad et al., 2015). A 90-day animal study of long-term use of sertraline in rats showed that sertraline did not cause pathological changes in testicular tissue (Ghorbani et al., 2021). However, more animal studies have shown that long-term use of sertraline can cause histological damage to the testes (Atli et al., 2017; Hamdi, 2019). In animal studies, Antiviral drug such as lopinavir/ritonavir and nevirapine can increase oxidative stress in the testes (Adaramoye et al., 2012; 2015). Histologically, nevirapine causes extensive degeneration of the seminiferous tubules, necrosis and detachment of germ cells (Adaramoye et al., 2012). The animal study of Lopinavir/ritonavir found that the number of spermatogenic cells in the testis was reduced and the morphology was abnormal (Adaramoye et al., 2012; Adaramoye et al., 2015).
There are also some clinical drugs that can reduce testicular oxidative stress while treating diseases. For diabetic rats, antihypertensive drugs enalapril can reduce oxidative stress and downregulate the expression of NFκB and COX-2 (Kushwaha and Jena, 2012). Studies on experimental varicocele rats and rats with adjuvant arthritis have shown that celecoxib can reduce oxidative stress in testicular tissue and improve sperm quality (Darwish et al., 2014; Mazhari et al., 2018). In clinical studies of patients with hypercholesterolemia, statins have no effect on sperm quality (Bernini et al., 1998; Cai et al., 2008; Dobs et al., 2000; Purvis et al., 1992). In studies on rats fed a high-fat diet, statins have a protective effect on male reproductive function. They improve semen quality, increase serum testosterone levels, improve testicular weight loss caused by hyperlipidemia, and increase fertility index (Abdulwahab et al., 2021; Esmail et al., 2020; Shalaby et al., 2004). These reproductive protective effects may be achieved by enhancing the mTOR signaling pathway and reducing oxidative stress (Cui et al., 2017; Farsani et al., 2018; Gurel et al., 2019). However, studies on statins have found that Rosuvastatin has a negative impact on male fertility. This may be related to the lower age of its research subjects. Rosuvastatin is commonly used in children with dyslipidemia (Leite et al., 2017). Its research focuses on pre-pubertal. Studies have shown that rosuvastatin reduces sperm quality, increases oxidative stress, increases DNA damage, damages testicular tissue, causes pathological changes in testicular and epididymal tissue, reduces testosterone levels, and damages the distribution of steroid receptors (Leite et al., 2019; Leite et al., 2017; Leite et al., 2018). Additionally, research on antihypertensive drugs has shown that irbesartan and carvedilol play a protective role in testicular injury caused by diseases through anti-inflammatory, antioxidant and anti-apoptotic effects (Abu-Risha et al., 2022; Eid et al., 2016; Eid et al., 2019; Kabel et al., 2020; Ramzy et al., 2014). Table 2.
4.1.2 The effect of small molecule medications on spermatogenesis
Spermatogenesis is through the proliferation of spermatogonia and differentiation to spermatocytes. After Meiosis, the spermatocytes that produce spermatozoa undergo round spermatids maturation, and finally form spermatozoa (Neto et al., 2016). Spermatogenesis is influenced by multiple factors, such as genetic disorders, environmental conditions, immune factors, etc. (Diemer and Desjardins, 1999; Chen et al., 2016; Gabrielsen and Tanrikut., 2016). For evaluating male fertility, semen analysis is an important indicator.
Small molecule medications can affect various germ cell during spermatogenesis (Figure 1C). The Angiotensin I-converting enzyme competitive inhibitor captopril reduces the proliferation rate of spermatogonial stem cells (Gao et al., 2018). Topiramate which used to patients with epilepsy can cause a decrease in the number of spermatogonia and spermatocytes (Otoom et al., 2004), resulting in the degeneration of spermatogenic cells and the formation of multinucleated giant cells (El et al., 2019), interstitial edema, and interstitial cell necrosis. Studies have found that topiramate downregulates the VEGFA gene, which promotes hormone entry into the vascular system in spermatogonia, and the SYCP3 gene, which plays an important role in meiosis in spermatocytes. Cannabidiol which used to treat neuropsychiatric disorders can impair spermatogenesis, affect the mitosis and meiosis of germ cells (Patra and Wadsworth., 1991), inhibit the G1/S phase cell cycle transition, inhibit DNA synthesis, and downregulate key cell cycle proteins (Li et al., 2022).
Drugs can cause damage to male fertility by damaging DNA. Antiviral drug abacavir and etravirine (Matuszewska et al., 2021), lipid-lowering drugs rosuvastatin (Leite et al., 2017), paracetamol (Smarr et al., 2017) will increase DNA damage. Several studies have shown that various antidepressants have a damaging effect on sperm DNA. Some scholars have found that antidepressants promote DNA damage centered on telomeres in germ cells (Sołek et al., 2021). Paroxetine causes abnormal DNA fragmentation in sperm (Tanrikut et al., 2010). Sertraline and escitalopram increase DNA damage (Akasheh et al., 2014; Atli et al., 2017; Ilgin et al., 2017; Hamdi, 2019). In vitro studies of sertraline have found that it negatively affects fertilization by inhibiting specific calcium ion channels in sperm, which affect calcium influx (Rahban et al., 2021). In the molecular mechanism study of reproductive cell toxicity of antidepressants, scholars have found that the mechanism is mediated by oxidative-reductive balance disorder, enzyme and non-enzyme cell protection mechanism failure, and mitochondrial dysfunction. Antidepressants can cause defects in spindle assembly and improper organelle segregation during in vitro cell division (Solek et al., 2021). For diabetic rats, enalapril can reduce sperm DNA damage caused by the disease (Kushwaha and Jena., 2012).
Small molecule drugs can also affect sperm quality by regulating gene expression. In research on antiepileptic drugs, it has been shown that they have a negative impact on sperm quality, but the mechanisms involved may vary. With the regard to carbamazepine, research has found that it downregulates the expression of potassium voltage-gated channel subfamily J member 11 (KCNJ11) in the testis, upregulates microRNA let-7a expression, and is statistically correlated with decreased sperm motility and increased sperm tail defects. Conversely, it upregulates cystic fibrosis transmembrane conductance regulator (CFTR) and microRNA 27a expression and is positively correlated with sperm tail defects (Tektemur et al., 2021). Previous studies have shown that decreased CFTR expression in sperm is associated with decreased sperm quality (Li et al., 2010). The authors speculate that the results contradicting previous research may be due to CFTR mRNA upregulation caused by CFTR channel dysfunction. The expression of CFTR and microRNA is related to sperm quality, but further experimental research is needed to confirm whether carbamazepine causes sperm damage through KCNJ11 or CFTR channels. Regarding valproic acid, research has found that the decreased expression of phosphorylated protein and Ki67 in the testis may affect the formation of the acrosome during sperm generation, leading to premature acrosome reactions and abnormal sperm heads. In clinical studies, the novel AED oxcarbazepine has been shown to increase sperm motility, concentration, and vitality (Guo et al., 2021; Wu et al., 2018). The authors speculate that this may have a positive effect on sperm production and maturation by raising FSH and LH levels. In addition to affecting sperm quality, small molecule drugs may also affect male fertility by affecting testosterone levels. Beta receptor antagonists such as propranolol and atenolol, also known as beta blockers, have been shown to reduce sperm motility, increase sperm abnormality rate, and lower testosterone levels, but they do not cause changes in the histology of the reproductive organs (El-Sayed et al., 1998; Fogari et al., 2002; Rosen et al., 1988; Suzuki et al., 1988). Studies on atenolol suggest that the reduction in testosterone levels may be due to the inhibition of testosterone release by reducing cAMP production in interstitial cells, rather than affecting steroidogenic enzyme activity (Khan et al., 2004). In vitro studies have shown that atenolol can increase sperm motility, and this increase in movement rate is downregulated by calcium channel blockers. Researchers speculate that this may be due to the fact that sperm adrenaline regulation may be calcium-dependent (Nelson and Cariello., 1989). Studies on propranolol have shown that it has a negative effect on male reproductive function. In the study on the mechanism of Propranolol inhibiting sperm motility, it was found that the isomer D-propranolol, which does not have beta receptor blocking activity, has a significant inhibitory effect on human sperm motility. Researchers speculate that propranolol’s inhibitory effect on sperm motility is due to its local anesthetic properties rather than beta receptor blockade (White et al., 1995). Other studies on propranolol have shown that it reduces sperm motility, increases sperm abnormality rate, lowers sperm concentration, reduces sperm count, inhibits sperm capacitation, acrosome reaction, and fertilization (De Turner et al., 1978; Khaled et al., 2020; Nusier et al., 2007; White et al., 1995). However, it does not cause chromosomal aberrations in germ cells (Aruna and Krishnamurthy, 1986). Table 3.
4.1.3 The effect of small molecule drugs on sperm capacitation and fertilization
Spermatozoa passing through female reproductive tract fertilizes egg and provides its genetic material. Before fertilization, spermatozoa require capacitation which is a process that sperm acquires the capacity to fertilize. This capacity includes hyperactivated movement, the acrosome reaction and fusion with oocytes (Bailey, 2010). The fertilization process is influenced by factors such as sperm motility and female reproductive tract environment (Fraser, 1992).
Male gametes must undergo the process of capacitation to meet the oocyte prior to fertilization (Figure 1D). Propranolol have shown a negative effect on male reproductive function as it inhibits sperm capacitation, acrosome reaction, and fertilization (White et al., 1995). Animal experiments with ibuprofen have shown that it weaken the ability of sperm to fertilize in vitro and reduces adult fertility potential (Barbosa et al., 2020; Martini et al., 2008). In vitro studies of sertraline have found that it negatively affects fertilization by inhibiting specific calcium ion channels in sperm, which affect calcium influx (Rahban et al., 2021). Animal experiments have shown that lisinopril reduces zona pellucida penetration and acrosome reaction (Saha et al., 2000a). However, there are also animal experiments and clinical studies that suggest a positive effect of lisinopril on male reproductive function. It can increase the number and quality of sperm and improve fertility (Mbah et al., 2012; Okeahialam et al., 2006). Calcium ions are important ions that trigger the acrosome reaction (Hong et al., 1984). Nimodipine and verapamil can cause a decrease in sperm density and motility and a decrease in acrosome reaction (Juneja et al., 1990; Saha et al., 2000b). It was found that captopril inhibits the acrosome reaction and reduces the proportion of penetrating oocytes (Foresta et al., 1991).
There are also drugs that have a positive effect on sperm capacitation and fertilization (Figure 1E). Many clinical studies have shown that indomethacin can improve sperm production in patients with oligo asthenoter atozoospermia, increase sperm motility and sperm count, and enhance fertilization ability. However, it can decrease testosterone levels (Aydin et al., 1995; Barkay et al., 1984; Conte et al., 1985; Guo et al., 2015). In studies on the effect of sodium ions on sperm motility, amiloride was found to increase the acrosome reaction and decrease sodium-induced sperm motility (Peris et al., 2000; Rai and Nirmal., 2003; Wong et al., 1981). Table 4.
4.2 Stem cell therapy in male infertility
With the rapid development of stem cell therapy these years, its application in male infertility has also received many attentions. Stem cells have the ability to self-renew and can differentiate into other special cell types (Ismail et al., 2023). In the treatment of male infertility, stem cells mainly play a therapeutic role through two pathways. One is to improve the microenvironment of spermatogenesis to restore the function of the remaining spermatogonial stem cells (SSCs). The other is to form germ cells through stem cells and further form sperm. At present, research on stem cell therapy mainly focuses on the application of MSCs (Ganjibakhsh et al., 2022; Ismail et al., 2023; Qiu et al., 2023). In addition, the vesicle system produced by stem cells, such as exosomes, also plays an important role in the field of stem cell therapy (Balistreri et al., 2020). The treatment methods of MSCs and exosomes in male infertility are illustrated in Figure 2.
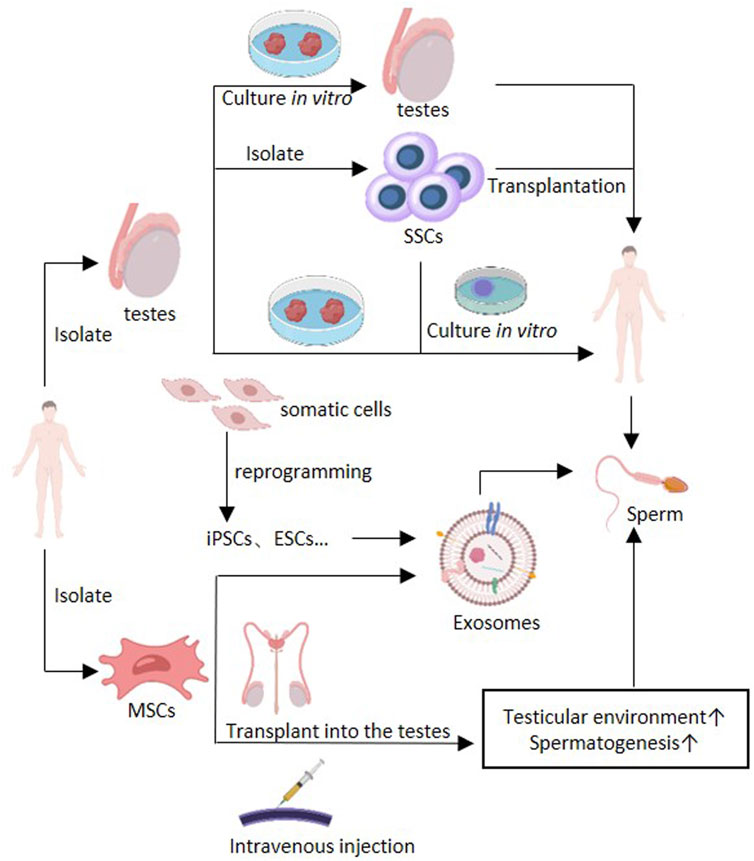
FIGURE 2. Application of stem cell therapy in male infertility. The separated MSCs can be divided into BM-MSCs, ADSC, AFSCs, USCs, UMSCs, etc., for therapy use. MSCs can improve testicular microenvironment and spermatogenesis by intravenous injection or testicular transplantation. Exosomes isolated from mesenchymal stem cells also have the same effect. Exosomes can also be isolated from ESCs and iPSCs, etc. Treatment may also be achieved through autologous transplantation of SSCs, autologous transplantation of testicular tissue and in vitro induction of spermatogenesis (culture testicular tissue or SSCs in vitro culture system to produce sperm). Abbreviations: Bone marrow derived MSCs (BM-MSCs), adipose derived stem cells (ADSCs), amniotic fluid-derived stem cells (AFSCs), Urine-derived stem cells (USCs), umbilical mesenchymal stem cells (UMSCs), embryonic stem cells (ESCs), induced pluripotent stem cells (iPSCs), spermatogonial stem cells (SSCs), ↑: improve.
4.2.1 MSCs in male infertility
MSCs are adult stem cells that can be isolated from many tissue such as adipose tissue, bone marrow or cord blood.It is still unclear whether the therapeutic effect of MSCs on male infertility is achieved by improving the testicular microenvironment or by differentiating into germ cell (Badawy et al., 2020). MSCs have the advantages of relatively easy access and low transplant rejection reaction. Many scholars have attempted to differentiate MSCs into male germ-like cells (Behzadi et al., 2019; Luo et al., 2019) and even haploid specialized like cells (Shlush et al., 2017). However, it has not yet been achieved to differentiate sperm from MSCs and produce offspring. Further investigation is still needed in this regard (Zhang et al., 2014). The different sources of MSCs can be divided into bone marrow derived MSCs (BM-MSCs), adipose derived stem cells (ADSCs), amniotic fluid-derived stem cells (AFSCs), Urine-derived stem cells (USCs), umbilical mesenchymal stem cells (UMSCs), etc., The research content mainly focuses on the improvement of MSCs on the damaged testicular microenvironment and spermatogenesis, and on the differentiation of MSCs into germ cell. In the research, the cells that have received much attention include BM-MSCs and UMSCs (Fazeli et al., 2018). However, obtaining BM-MSCs is more difficult compared to other types of MSCs. USCs and their exosomes isolated from the urine of healthy men have been found to promote the recovery of spermatogenesis in azoospermic mice (Deng et al., 2019). This non-invasive and simpler method of obtaining MSCs has advantages in widespread clinical applications.
In the study of mice, Co-transplantation of MSCs improves SSCs transplantation efficiency (Kadam et al., 2018). Transplantation of BM-MSCs alone can upregulate the expression of target genes related to spermatogenesis and improve fertility of azoospermic rats (Badawy et al., 2020) In vitro studies, it can also better maintain spermatogenesis in co-culture with SSCs (Önen et al., 2023). For the administration method, intravenous injection of MSCs can alleviate the testicular toxicity caused by gonadal toxic substances through antioxidant, anti-inflammatory, and anti-apoptotic effects (Abdelaziz et al., 2019; Elbaghdady et al., 2018; Sherif et al., 2018), and testicular injection also has an improvement effect (Hsiao et al., 2015; SM et al., 2017). ADSCs and AFSCs can reduce oxidative stress in the testes and promote sperm production, (Eliyasi et al., 2020; Ibrahim et al., 2021; Qian et al., 2020; Siregar et al., 2021), and can improve the damage of chemotherapy drugs to testes (Meligy et al., 2019). Intratesticular injection of ADSCs can improve the spermatogenesis of azoospermia (Ganjibakhsh et al., 2022; Hajihoseini et al., 2018; Karimaghai et al., 2018), while xenotransplantation of human umbilical cord blood derived MSCs into azoospermia mice also has the same effect (Abd et al., 2017). Animal experiments have shown that after transplantation of BM-MSCs into the testis, in addition to influencing male reproductive function through paracrine action, they will also relocate to a new niche to form colonies, thus realizing the reconstruction of the damaged testicular germinal epithelium (Ghasemzadeh-Hasankolaei et al., 2016; Monsefi et al., 2013). Scholars try to differentiate different types of MSCs into germ cell, hoping that they can become a source of germ cell for the treatment of male infertility (Dissanayake et al., 2018; Ghaem et al., 2018; Kumar et al., 2018; Liu et al., 2018; Shlush et al., 2017). However, this goal has not yet been achieved. Table 5.
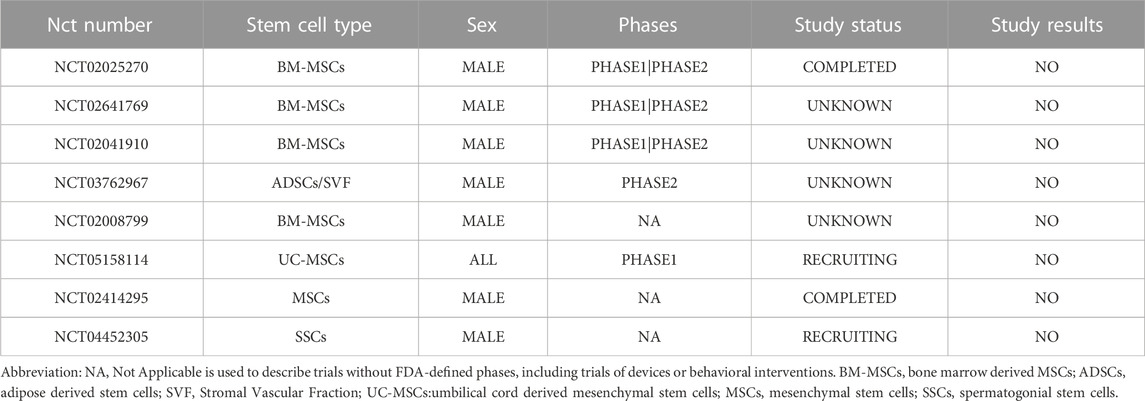
TABLE 5. Clinical trials on stem cell therapy for male infertility (U. S. National Library of Medicine).
In addition to tissue-originated MSCs, pluripotent stem cells MSCs (PSC-MSCs) are another pathway to obtain MSCs. PSC-MSCs have attracted attention due to their easier availability, more convenience quality control, and possibly large-scale production compared to tissue-originated MSCs (Abdal et al., 2019; Sabapathy and Kumar., 2016). In the research of PSC-MSCs in the treatment of diseases, PSC-MSCs having anti-inflammatory and immunomodulatory properties, like tissue-originated MSCs, can play a therapeutic role via paracrine, exosomes (Qu et al., 2022). However, it has the same safety issue of tumorigenicity as tissue-originated MSCs and iPSCs (Jiang et al., 2019). Although PSC-MSCs have been studied to varying degrees in the treatment of other diseases, there is still a lack of research in the treatment of male infertility.
MSCs have anti-inflammatory and immunomodulatory properties, trophic properties, and anti-apoptotic properties (Murphy et al., 2013). It may improve male infertility in multiple ways. More research is needed on the mechanism of its action. From the perspective of clinical treatment, different types of MSCs have their own advantages, and more research and analysis are needed to determine which type of MSCs treatment is more effective and in what manner (Zhankina et al., 2021). The safety of various mesenchymal cell applications also needs further exploration (Pendleton et al., 2013).
4.2.2 iPSC/ESC in male infertility
In addition, embryonic stem cells (ESCs) and human induced pluripotent stem cells (iPSCs) have also received attention in this field. Human ESCs and iPSCs are two pluripotent populations that can self-renew and differentiate into any type of cell (Zhao et al., 2021). iPSCs were discovered in 2006 by Takahashi and Yamanaka as pluripotent stem cells that exhibit the morphological and growth characteristics of ESCs and express ESCs marker genes (Takahashi and Yamanka., 2006). The male germ cell differentiated from iPSCs is a germ cell with the patient’s own genetic material, which is the advantage of iPSCs as a cell therapy. However, iPSCs also reduces the immune system response of patients after transplantation. The disadvantage of iPSCs lies in the possibility of tumor formation, and its safety still needs to be considered (Sojoudi et al., 2023). Although it has been found in research that human iPSCs can directly differentiate into haploid spermatogenic cells, it is still not possible to ultimately form personalized human gametes in vitro (Easley et al., 2012; Eguizabal et al., 2011). Improving the efficiency of differentiation, inducing iPSC to produce personalized human gametes and identify markers for successful differentiation of germ cell are the difficulties that need to be broken through (Zhao et al., 2021). Although in the research of mice, embryonic stem cells can complete Meiosis in vitro and produce offspring through assisted reproduction technology (Nayernia et al., 2006; Zhou et al., 2016). However, this technology has not yet been achieved in the study of human ESCs. And there are differences in the genetic material between the germ cell obtained from ESCs and the recipient, which will lead to ethical disputes (Meligy et al., 2019).
4.2.3 SSCs in male infertility
The use of patients’ own germ cell for treatment can mainly be achieved through autologous transplantation of SSCs, autologous transplantation of testicular tissue and in vitro induction of spermatogenesis (Figure 2), but these approaches have not yet been applied to clinical practice, are still in the experimental stage, and there are many problems to be solved (Dong et al., 2019). SSCs can maintain self-renewal and differentiate into sperm, providing genetic material for the next-generation. SSCs can differentiate into spermatozoa in vitro and generate mature spermatozoa through Spermatogenesis by transplantation in vivo, thus restoring male fertility (Diao et al., 2022). However, there is still no systematic method for long-term cultivation of human SSCs in vitro. Whether the epigenetic stability can be maintained in long-term in vitro culture still needs further exploration, although some studies have found that the sperm DNA methylation of the offspring of long-term in vitro cultured mouse SSCs after transplantation is stable (Serrano et al., 2023), and the genetic and epigenetics stability of human spermatogonial stem cells in long-term culture (Nickkholgh et al., 2014). SSCs transplantation was first achieved in mice in 1994 (Brinster and Zimmermann, 1994). In recent years, Whelan et al. have found that transplanting rat spermatogonial stem cells that have been frozen for over 20 years into mice can still produce sperm (Whelan et al., 2022). However, there is still limited research on spermatogonial stem cell transplantation in humans. Up to now, it has not been possible for human SSCs to form gametes in vitro, nor for cryopreserved human testicular tissue to initiate spermatogenesis in vitro (Portela et al., 2019). Autologous testicular tissue transplantation may be an option for men who want to receive gonadal toxicity treatment before puberty (Duca et al., 2019). Re transplantation of frozen testicular tissue into patients can achieve spermatogenesis in vivo, thus restoring the fertility of patients. In the study of primates, scholars have restored male fertility and produced offspring through cryopreserved autologous testicular tissue transplantation (Fayomi et al., 2019; Jahnukainen et al., 2012). Although many centers have also cryopreserved testicular tissue from pre-adolescent patients, testicular tissue transplantation has not yet been achieved in humans (Goossens et al., 2020; Valli-Pulaski et al., 2019; Zarandi et al., 2018). And for patients with cancer, there may be a risk of cancer cells in the tissue (Abdelaal et al., 2021; Sanou et al., 2022). Transplantation may lead to tumor recurrence in the recipient (Hou et al., 2007; Jahnukainen et al., 2001). Therefore, the safety of frozen testicular tissue autotransplantation for tumor patients should be comprehensively evaluated. For prepubertal patients, the testicular tissue is relatively small and the number of spermatogonial stem cells is limited. Therefore, expanding the number of spermatogonial stem cells in vitro before transplantation is also a challenge that needs to be overcame (Zarandi et al., 2018). In addition, some studies have shown that the germ cell in the male testicular tissue before puberty may have abnormal maturation of germ cell, which may lead to the failure to restore fertility after reimplantation of testicular tissue (Pietzak et al., 2015). Further exploration is needed on the conditions for long-term stable cryopreservation of testicular tissue and the location of transplantation (Moraveji et al., 2019; Sharma et al., 2019). The above technologies have not yet reached the clinical stage (Diao et al., 2022; Martin-Inaraja et al., 2021; Sanou et al., 2022).
Different stem cells have their own advantages and disadvantages when applied to male infertility. MSCs and SSCs are the existing stem cell options for male infertility. According to the data of U. S. National Library of Medicine, there are 8 clinical trials on stem cell therapy for male infertility (Table 5), but none of them has submitted any results yet. No relevant clinical trial results were found in PubMed. Further exploration of its mechanism of action and individualized selection of stem cell therapy regimens may be necessary.
Consequently, MSCs and SSCs may have more applications in the treatment of male infertility compared to other types of stem cell. The reason why MSCs have attracted much attention from researchers is that they are easy to obtain and cultivate and can achieve therapeutic effects through autologous cell transplantation. Different types of MSCs have different advantages. Choosing which MSCs to use in treatment may require more research and individualized treatment programs. Forming sperm from stem cells may be a potential therapeutic treatment for male infertility, although it has not yet been achieved. SSCs play an important role for male fertility preservation, although more research is needed in areas such as cryopreservation and in vitro culture. The application of stem cells in the treatment of male infertility still requires more extensive research.
4.3 The application of exosomes in male infertility
Exosomes are extracellular vesicles that are released from most cell types. Exosome play an important role in intercellular connections. Its diameter is 60–180 nm, which can be considered as an extracellular organelle mainly composed of proteins, lipids, and nucleic acids that act through endocytosis, phagocytosis, and membrane fusion (Sojoudi et al., 2023; Wang, 2021). Stem cell exosomes are another important pathway for stem cell therapy. A cell free therapy can be achieved by obtaining stem cell exosomes (Balistreri et al., 2020). In recent years, the function of exosomes in male reproduction has received widespread attention. The miRNA expression of extracellar microvessels in oligozoospermia and necropermia patients is different from that in healthy men (Abu-Halima et al., 2016). Other studies have shown that harmful substances can cause damage to sperm development by damaging exosomal secretion associated with spermatozoa (Ali et al., 2023). In terms of treatment, exosomes have advantages such as low immunogenicity, easy management, and no risk of tumor development, and can be used as a cell-free therapeutic approach (Taheri et al., 2019). Exosomes may be applied as novel drugs in the clinical treatment of male infertility.
Various types of cells in the testes secrete extracellular vesicles, with the extracellular vesicles secreted by the epididymis and prostate being the most concerned. The signal pathway proteins and miRNAs related to spermatogenesis in these exosomes may become a new therapeutic method for male reproduction and a means of disease diagnosis (Amiri et al., 2023; Candenas and Chianese, 2020; Eikmans et al., 2020; Lal et al., 2022; Zhu et al., 2020). Semen extracellular vesicles from the epididymis and prostate are known as epididymosomes and prostasomes, respectively. Sperm motility activation, capacitation and acrosome reaction are related to epididymosomes and prostasomes (Simon et al., 2018). In current research, it has been found that epididymosomes are involved in sperm maturation and have the function of reducing oxidative stress, regulating gene expression in sperm, regulating sperm morphology and motility. Prostasomes play an important role in regulating sperm motility and sperm capacitation (Baskaran et al., 2020; Ma et al., 2023). In addition, exosomes that sertoli cells secretion also play an important role in male fertility, although the small molecules it plays are still not very clear (Gao et al., 2023) Sertoli-derived exosomes can reduce oxidative stress and improve the microenvironment of spermatogenesis (Salek et al., 2021). Some studies on pigs have shown that the exosomes secreted by Sertoli cells can promote the proliferation of spermatogonial cells. This means that exosomes may be used for feeder-free layer culture systems (Thiageswaran et al., 2022). Studies have found that extracellular vesicles in semen also play an important role in embryonic development before implantation (Chen et al., 2020). In addition, exosomes derived from MSCs also have good prospects in the treatment of male infertility.
Research has shown that the exosomes of MSCs from different sources have an improvement effect on testicular injury (Mobarak et al., 2021; Sagaradze et al., 2019; Zhang et al., 2018b), which may be due to anti-inflammatory, antioxidant, and anti-apoptotic effects (Zhang et al., 2018a) (Table 6). In general, exosomes can play an anti-inflammatory, anti-hypoxic, anti-apoptotic and other roles by transmitting miRNA and other substances, improve the testicular microenvironment, and thus improve spermatogenesis, fertilization, implantation, and other aspects. However, no clinical trial was found in the U. S. National Library of Medicine or PubMed for the treatment of testicular injury with exosomes currently. There are animal studies on the treatment of testicular injury with exosomes. Radiation-induced testicular injury can be alleviated by macrophage-derived exosomes which contain protective components G-CSF and MIP-2 (Liu et al., 2020). The advantage of exosome is to avoid the degradation of active ingredient and the disadvantage of cell therapy. It is easy to detect the exosomes released by testicular cells in seminal plasma. With the deepening of research on its mechanism of action and its role as clinical biomarkers, exosomes may become an important diagnostic and treatment method for male fertility in the future.
5 Conclusion and discussion
The effects of drugs on male germ cells can affect testosterone production, spermatogenesis, sperm capacitation, alter genetic material of germ cells, increase oxidative stress, and regulate signaling pathways. Most drugs have negative effects on male germ cells. Although germ cells can recover after most drugs are discontinued, the negative impact of drugs on germ cells should also be taken into consideration when choosing drugs for clinical use. Drugs with obvious toxicity to germ cells should be used with caution. More research should be invested in drugs that need to be taken long-term to maintain treatment, in order to clarify their mechanisms of action and explore how to cope with the damage to germ cells caused by these drugs.
Stem cell therapy and exosomes therapy may be a new type of treatment for iatrogenic male infertility or male infertility caused by diseases in the future. The advantages of exosomes application include avoiding the degradation of active ingredients and the drawbacks of cell therapy, but their specific molecular mechanisms of action still need to be further explored. The advantage of stem cell therapy lies in self-renewal and the ability to differentiate into other special cell types. The application of different types of stem cells in the treatment of male infertility has different difficulties that need to be overcome. Therefore, while exploring its mechanism of action and applying it to regenerative medicine, we may also consider the personalized choice of its treatment scheme and the safety of its application.
Author contributions
PM: Writing–original draft. ZZ: Validation, Visualization, Writing–original draft. XK: Validation, Visualization, Writing–original draft. YF: Funding acquisition, Supervision, Writing–review and editing. CL: Funding acquisition, Project administration, Supervision, Validation, Writing–review and editing.
Funding
The author(s) declare financial support was received for the research, authorship, and/or publication of this article. This work was supported by grants from National Key Research and Development Program of China (2019YFA0110804, 2021YFC2700904 to YF); Guangzhou Science and technology planning project (2023A03J0043 to CL); the National Natural Science Foundation of China (3210060082 to CL) and Guangdong Basic and Applied Basic Research Foundation (2020A1515010877 to CL).
Acknowledgments
We would like to thank the support of Student Innovation Ability Project plan of Guangzhou Medical University.
Conflict of interest
The authors declare that the research was conducted in the absence of any commercial or financial relationships that could be construed as a potential conflict of interest.
Publisher’s note
All claims expressed in this article are solely those of the authors and do not necessarily represent those of their affiliated organizations, or those of the publisher, the editors and the reviewers. Any product that may be evaluated in this article, or claim that may be made by its manufacturer, is not guaranteed or endorsed by the publisher.
References
Abd, A. S., Pasha, H. F., Abdelrahman, A. A., and Mazen, N. F. (2017). Molecular effect of human umbilical cord blood cd34-positive and cd34-negative stem cells and their conjugate in azoospermic mice. Mol. Cell. Biochem. 428 (1-2), 179–191. doi:10.1007/s11010-016-2928-2
Abdal, D. A., Lee, S. B., Kim, K., Lim, K. M., Jeon, T. I., Seok, J., et al. (2019). Production of mesenchymal stem cells through stem cell reprogramming. Int. J. Mol. Sci. 20 (8), 1922. doi:10.3390/ijms20081922
Abdelaal, N. E., Tanga, B. M., Abdelgawad, M., Allam, S., Fathi, M., Saadeldin, I. M., et al. (2021). Cellular therapy via spermatogonial stem cells for treating impaired spermatogenesis, non-obstructive azoospermia. Cells 10 (7), 1779. doi:10.3390/cells10071779
Abdelaziz, M. H., Salah, E. E., El-Dakdoky, M. H., and Ahmed, T. A. (2019). The impact of mesenchymal stem cells on doxorubicin-induced testicular toxicity and progeny outcome of male prepubertal rats. Birth Defects Res. 111 (13), 906–919. doi:10.1002/bdr2.1535
Abdulwahab, D. K., Ibrahim, W. W., Abd, E. R., Abdel-Latif, H. A., and Abdelkader, N. F. (2021). Grape seed extract improved the fertility-enhancing effect of atorvastatin in high-fat diet-induced testicular injury in rats: involvement of antioxidant and anti-apoptotic effects. J. Pharm. Pharmacol. 73 (3), 366–376. doi:10.1093/jpp/rgaa002
Abu-Halima, M., Ludwig, N., Hart, M., Leidinger, P., Backes, C., Keller, A., et al. (2016). Altered micro-ribonucleic acid expression profiles of extracellular microvesicles in the seminal plasma of patients with oligoasthenozoospermia. Fertil. Steril. 106 (5), 1061–1069. doi:10.1016/j.fertnstert.2016.06.030
Abu-Risha, S. E., Mousa, M. A., and Elsisi, A. E. (2022). Protective role of irbesartan against cyclophosphamide-induced testicular damage in rats via up-regulating ppar-γ signaling and ameliorating nf-κb/nlrp3/il-18 inflammatory axis. Life Sci. 289, 120218. doi:10.1016/j.lfs.2021.120218
Adaramoye, O. A., Adesanoye, O. A., Adewumi, O. M., and Akanni, O. (2012). Studies on the toxicological effect of nevirapine, an antiretroviral drug, on the liver, kidney and testis of male wistar rats. Hum. Exp. Toxicol. 31 (7), 676–685. doi:10.1177/0960327111424304
Adaramoye, O. A., Akanni, O. O., Adewumi, O. M., and Owumi, S. E. (2015). Lopinavir/ritonavir, an antiretroviral drug, lowers sperm quality and induces testicular oxidative damage in rats. Tokai J. Exp. Clin. Med. 40 (2), 51–57.
Agarwal, A., Baskaran, S., Parekh, N., Cho, C. L., Henkel, R., Vij, S., et al. (2021). Male infertility. Lancet 397 (10271), 319–333. doi:10.1016/S0140-6736(20)32667-2
Akasheh, G., Sirati, L., Noshad, K. A., and Sepehrmanesh, Z. (2014). Comparison of the effect of sertraline with behavioral therapy on semen parameters in men with primary premature ejaculation. Urology 83 (4), 800–804. doi:10.1016/j.urology.2013.12.004
Ali, W., Bian, Y., Ali, H., Sun, J., Zhu, J., Ma, Y., et al. (2023). Cadmium-induced impairment of spermatozoa development by reducing exosomal-mvbs secretion: A novel pathway. Aging (Albany NY) 15 (10), 4096–4107. doi:10.18632/aging.204675
Alsemeh, A. E., Ahmed, M. M., Fawzy, A., Samy, W., Tharwat, M., and Rezq, S. (2022). Vitamin e rescues valproic acid-induced testicular injury in rats: role of autophagy. Life Sci. 296, 120434. doi:10.1016/j.lfs.2022.120434
Amiri, N., Mohammadi, P., Allahgholi, A., Salek, F., and Amini, E. (2023). The potential of sertoli cells (scs) derived exosomes and its therapeutic efficacy in male reproductive disorders. Life Sci. 312, 121251. doi:10.1016/j.lfs.2022.121251
Aruna, N., and Krishnamurthy, N. B. (1986). Mutagenic evaluation of propranolol in somatic and germ cells of mice. Mutat. Res.-Fundam. Mol. Mech. Mutagen. 173 (3), 207–210. doi:10.1016/0165-7992(86)90037-0
Atli, O., Baysal, M., Aydogan-Kilic, G., Kilic, V., Ucarcan, S., Karaduman, B., et al. (2017). Sertraline-induced reproductive toxicity in male rats: evaluation of possible underlying mechanisms. Asian J. Androl. 19 (6), 672–679. doi:10.4103/1008-682X.192637
Attia, S. M., and Bakheet, S. A. (2013). Citalopram at the recommended human doses after long-term treatment is genotoxic for male germ cell. Food Chem. Toxicol. 53, 281–285. doi:10.1016/j.fct.2012.11.051
Aydin, S., Inci, O., and Alagöl, B. (1995). The role of arginine, indomethacin and kallikrein in the treatment of oligoasthenospermia. Int. Urol. Nephrol. 27 (2), 199–202. doi:10.1007/BF02551320
Badawy, A. A., El-Magd, M. A., AlSadrah, S. A., and Alruwaili, M. M. (2020). Altered expression of some mirnas and their target genes following mesenchymal stem cell treatment in busulfan-induced azoospermic rats. Gene 737, 144481. doi:10.1016/j.gene.2020.144481
Bagoji, I. B., Hadimani, G. A., Yendigeri, S. M., and Das, K. K. (2017). Sub-chronic indomethacin treatment and its effect on the male reproductive system of albino rats: possible protective role of black tea extract. J. Basic Clin. Physiol. Pharmacol. 28 (3), 201–207. doi:10.1515/jbcpp-2016-0168
Bailey, J. L. (2010). Factors regulating sperm capacitation. Syst. Biol. Reprod. Med. 56 (5), 334–348. doi:10.3109/19396368.2010.512377
Balistreri, C. R., De Falco, E., Bordin, A., Maslova, O., Koliada, A., and Vaiserman, A. (2020). Stem cell therapy: old challenges and new solutions. Mol. Biol. Rep. 47 (4), 3117–3131. doi:10.1007/s11033-020-05353-2
Barbosa, M. G., Jorge, B. C., Stein, J., Santos, F. D., Barreto, A., Reis, A., et al. (2020). Pre-pubertal exposure to ibuprofen impairs sperm parameters in male adult rats and compromises the next generation. J. Toxicol. Env. Health Part A. 83 (15-16), 559–572. doi:10.1080/15287394.2020.1786483
Barkay, J., Harpaz-Kerpel, S., Ben-Ezra, S., Gordon, S., and Zuckerman, H. (1984). The prostaglandin inhibitor effect of antiinflammatory drugs in the therapy of male infertility. Fertil. Steril. 42 (3), 406–411. doi:10.1016/s0015-0282(16)48081-7
Baskaran, S., Panner, S. M., and Agarwal, A. (2020). Exosomes of male reproduction. Advan. Clin. Chem. 95, 149–163. doi:10.1016/bs.acc.2019.08.004
Baysal, M., Ilgin, S., Kilic, G., Kilic, V., Ucarcan, S., and Atli, O. (2017). Reproductive toxicity after levetiracetam administration in male rats: evidence for role of hormonal status and oxidative stress. PLoS One 12 (4), e0175990. doi:10.1371/journal.pone.0175990
Behzadi, F. S., Mazaheri, Z., Ghorbanmehr, N., Movahedin, M., Behzadi, F. M., and Gholampour, M. A. (2019). Analysis of mirna-17 and mirna-146 expression during differentiation of spermatogonial stem like cells derived from mouse bone marrow mesenchymal stem cells. Int. J. Mol. Cell. Med. 8 (1), 14–23. doi:10.22088/IJMCM.BUMS.8.1.14
Bernini, G. P., Brogi, G., Argenio, G. F., Moretti, A., and Salvetti, A. (1998). Effects of long-term pravastatin treatment on spermatogenesis and on adrenal and testicular steroidogenesis in male hypercholesterolemic patients. J. Endocrinol. Investig. 21 (5), 310–317. doi:10.1007/BF03350334
Brinster, R. L., and Zimmermann, J. W. (1994). Spermatogenesis following male germ-cell transplantation. Proc. Natl. Acad. Sci. U. S. A. 91 (24), 11298–11302. doi:10.1073/pnas.91.24.11298
Cai, T., Mondaini, N., Mazzoli, S., and Bartoletti, R. (2008). A possible negative effect of co-administered amlodipine and atorvastatin on semen volume and spermatozoa in men. J. Pharm. Pharmacol. 60 (11), 1431–1432. doi:10.1211/jpp/60.11.0002
Candenas, L., and Chianese, R. (2020). Exosome composition and seminal plasma proteome: A promising source of biomarkers of male infertility. Int. J. Mol. Sci. 21 (19), 7022. doi:10.3390/ijms21197022
Carvalho, R. K., Rocha, T. L., Fernandes, F. H., Gonçalves, B. B., Souza, M. R., Araújo, A. A., et al. (2022). Decreasing sperm quality in mice subjected to chronic cannabidiol exposure: new insights of cannabidiol-mediated male reproductive toxicity. Chem.-Biol. Interact. 351, 109743. doi:10.1016/j.cbi.2021.109743
Carvalho, R. K., Santos, M. L., Souza, M. R., Rocha, T. L., Guimarães, F. S., Anselmo-Franci, J. A., et al. (2018). Chronic exposure to cannabidiol induces reproductive toxicity in male swiss mice. J. Appl. Toxicol. 38 (9), 1215–1223. doi:10.1002/jat.3631
Chen, Q., Deng, T., and Han, D. (2016). Testicular immunoregulation and spermatogenesis. Semin. Cell. Dev. Biol. 59, 157–165. doi:10.1016/j.semcdb.2016.01.019
Chen, X., Zheng, Y., Lei, A., Zhang, H., Niu, H., Li, X., et al. (2020). Early cleavage of preimplantation embryos is regulated by trna(gln-ttg)-derived small rnas present in mature spermatozoa. J. Biol. Chem. 295 (32), 10885–10900. doi:10.1074/jbc.RA120.013003
Chengelis, C. P., Dodd, D. C., and Kotsonis, F. N. (1986). Testicular toxicity of a novel 1,4-benzodiazepine (sc-32855) in rats and dogs. Res. Commun. Chem. Pathol. Pharmacol. 51 (1), 23–36.
Conte, D., Nordio, M., Romanelli, F., Manganelli, F., Giovenco, P., Dondero, F., et al. (1985). Role of seminal prostaglandins in male fertility. Ii. Effects of prostaglandin synthesis inhibition on spermatogenesis in man. J. Endocrinol. Investig. 8 (4), 289–291. doi:10.1007/BF03348497
Cook, P. S., Notelovitz, M., Kalra, P. S., and Kalra, S. P. (1979). Effect of diazepam on serum testosterone and the ventral prostate gland in male rats. Arch. Androl. 3 (1), 31–35. doi:10.3109/01485017908985045
Cui, X., Long, C., Zhu, J., and Tian, J. (2017). Protective effects of fluvastatin on reproductive function in obese male rats induced by high-fat diet through enhanced signaling of mtor. Cell. Physiol. Biochem. 41 (2), 598–608. doi:10.1159/000457881
Darwish, H. A., Arab, H. H., and Abdelsalam, R. M. (2014). Chrysin alleviates testicular dysfunction in adjuvant arthritic rats via suppression of inflammation and apoptosis: comparison with celecoxib. Toxicol. Appl. Pharmacol. 279 (2), 129–140. doi:10.1016/j.taap.2014.05.018
de Oliveira, W. M., de Sá, I. R., de Torres, S. M., de Morais, R. N., Andrade, A. M., Maia, F. C., et al. (2013). Perinatal exposure to fluoxetine via placenta and lactation inhibits the testicular development in male rat offspring. Syst. Biol. Reprod. Med. 59 (5), 244–250. doi:10.3109/19396368.2013.796021
De Turner, E., Aparicio, N. J., Turner, D., and Schwarzstein, L. (1978). Effect of two phosphodiesterase inhibitors, cyclic adenosine 3':5'-monophosphate, and a beta-blocking agent on human sperm motility. Fertil. Steril. 29 (3), 328–331. doi:10.1016/s0015-0282(16)43161-4
Deng, C., Xie, Y., Zhang, C., Ouyang, B., Chen, H., Lv, L., et al. (2019). Urine-derived stem cells facilitate endogenous spermatogenesis restoration of busulfan-induced nonobstructive azoospermic mice by paracrine exosomes. Stem Cells Dev. 28 (19), 1322–1333. doi:10.1089/scd.2019.0026
Diao, L., Turek, P. J., John, C. M., Fang, F., and Reijo, P. R. (2022). Roles of spermatogonial stem cells in spermatogenesis and fertility restoration. Front. Endocrinol. 13, 895528. doi:10.3389/fendo.2022.895528
Diemer, T., and Desjardins, C. (1999). Developmental and genetic disorders in spermatogenesis. Hum. Reprod. Update. 5 (2), 120–140. doi:10.1093/humupd/5.2.120
Dissanayake, D., Patel, H., and Wijesinghe, P. S. (2018). Differentiation of human male germ cells from wharton's jelly-derived mesenchymal stem cells. Clin. Exp. Reprod. Med.-CERM. 45 (2), 75–81. doi:10.5653/cerm.2018.45.2.75
Dobs, A. S., Miller, S., Neri, G., Weiss, S., Tate, A. C., Shapiro, D. R., et al. (2000). Effects of simvastatin and pravastatin on gonadal function in male hypercholesterolemic patients. Metab.-Clin. Exp. 49 (1), 115–121. doi:10.1016/s0026-0495(00)90938-7
Dong, L., Gul, M., Hildorf, S., Pors, S. E., Kristensen, S. G., Hoffmann, E. R., et al. (2019). Xeno-free propagation of spermatogonial stem cells from infant boys. Int. J. Mol. Sci. 20 (21), 5390. doi:10.3390/ijms20215390
Drobnis, E. Z., and Nangia, A. K. (2017). Cardiovascular/pulmonary medications and male reproduction. Adv. Exp. Med. Biol. 1034, 103–130. doi:10.1007/978-3-319-69535-8_9
Duca, Y., Di Cataldo, A., Russo, G., Cannata, E., Burgio, G., Compagnone, M., et al. (2019). Testicular function of childhood cancer survivors: who is worse? J. Clin. Med. 8 (12), 2204. doi:10.3390/jcm8122204
Easley, C. T., Phillips, B. T., McGuire, M. M., Barringer, J. M., Valli, H., Hermann, B. P., et al. (2012). Direct differentiation of human pluripotent stem cells into haploid spermatogenic cells. Cell. Rep. 2 (3), 440–446. doi:10.1016/j.celrep.2012.07.015
Eguizabal, C., Montserrat, N., Vassena, R., Barragan, M., Garreta, E., Garcia-Quevedo, L., et al. (2011). Complete meiosis from human induced pluripotent stem cells. Stem Cells 29 (8), 1186–1195. doi:10.1002/stem.672
Eid, A. H., Abdelkader, N. F., Abd, E. O., Fawzy, H. M., and El-Denshary, E. S. (2016). Carvedilol alleviates testicular and spermatological damage induced by cisplatin in rats via modulation of oxidative stress and inflammation. Arch. Pharm. Res. 39 (12), 1693–1702. doi:10.1007/s12272-016-0833-6
Eid, A. H., Gad, A. M., Fikry, E. M., and Arab, H. H. (2019). Venlafaxine and carvedilol ameliorate testicular impairment and disrupted spermatogenesis in rheumatoid arthritis by targeting ampk/erk and pi3k/akt/mtor pathways. Toxicol. Appl. Pharmacol. 364, 83–96. doi:10.1016/j.taap.2018.12.014
Eikmans, M., D, H. A. J., Blijleven, L., Meuleman, T., van Beelen, E., van der Hoorn, M. P., et al. (2020). Optimization of microrna acquirement from seminal plasma and identification of diminished seminal microrna-34b as indicator of low semen concentration. Int. J. Mol. Sci. 21 (11), 4089. doi:10.3390/ijms21114089
El, M. A., Ibrahim, F. M., Mabrouk, D. M., Ahmed, K. A., and Fawzy, R. M. (2019). Effect of antiepileptic drug (topiramate) and cold pressed ginger oil on testicular genes expression, sexual hormones and histopathological alterations in mice. Biomed. Pharmacother. 110, 409–419. doi:10.1016/j.biopha.2018.11.146
El-Sayed, M. G., El-Sayed, M. T., Elazab, A. E. S., Hafeiz, M. H., El-Komy, A. A., and Hassan, E. (1998). Effects of some beta-adrenergic blockers on male fertility parameters in rats. Dtsch. Tierarztl Wochenschr 105 (1), 10–12.
Elbaghdady, H., Alwaili, M. A., and El-Demerdash, R. S. (2018). Amelioration of cadmium-induced testes' damage in rats by the bone marrow mesenchymal stem cells. Ecotox. Environ. Safe. 148, 763–769. doi:10.1016/j.ecoenv.2017.10.016
Eliyasi, D. M., Hemadi, M., Saki, G., Mohammadiasl, J., and Khodadadi, A. (2020). Spermatogenesis recovery potentials after transplantation of adipose tissue-derived mesenchymal stem cells cultured with growth factors in experimental azoospermic mouse models. Cell. J. 21 (4), 401–409. doi:10.22074/cellj.2020.6055
Elmslie, K. S. (2004). Calcium channel blockers in the treatment of disease. J. Neurosci. Res. 75 (6), 733–741. doi:10.1002/jnr.10872
Erdemir, F., Atilgan, D., Firat, F., Markoc, F., Parlaktas, B. S., and Sogut, E. (2014). The effect of sertraline, paroxetine, fluoxetine and escitalopram on testicular tissue and oxidative stress parameters in rats. Int. Braz J. Urol. 40 (1), 100–108. doi:10.1590/S1677-5538.IBJU.2014.01.15
Esmail, M., Kandeil, M., El-Zanaty, A. M., and Abdel-Gabbar, M. (2020). The ameliorative effect of atorvastatin on serum testosterone and testicular oxidant/antioxidant system of hfd-fed male albino rats. F1000Res 9, 1300. doi:10.12688/f1000research.25926.1
Farsani, B. E., Karimi, S., and Mansouri, E. (2018). Pravastatin attenuates testicular damage induced by doxorubicin - a stereological and histopatological study. J. Basic Clin. Physiol. Pharmacol. 30 (1), 103–109. doi:10.1515/jbcpp-2018-0073
Fayomi, A. P., Peters, K., Sukhwani, M., Valli-Pulaski, H., Shetty, G., Meistrich, M. L., et al. (2019). Autologous grafting of cryopreserved prepubertal rhesus testis produces sperm and offspring. Science 363 (6433), 1314–1319. doi:10.1126/science.aav2914
Fazeli, Z., Abedindo, A., Omrani, M. D., and Ghaderian, S. M. H. (2018). Mesenchymal stem cells (mscs) therapy for recovery of fertility: A systematic review. Stem Cell. Rev. Rep. 14 (1), 1–12. doi:10.1007/s12015-017-9765-x
Fode, M., Joensen, U. N., Wiborg, M. H., Fojecki, G., Jørgensen, N., and Jensen, C. (2021). Diagnosis and treatment of male infertility. Ugeskr. Laeger 183 (2), V07200565.
Fogari, R., Preti, P., Derosa, G., Marasi, G., Zoppi, A., Rinaldi, A., et al. (2002). Effect of antihypertensive treatment with valsartan or atenolol on sexual activity and plasma testosterone in hypertensive men. Eur. J. Clin. Pharmacol. 58 (3), 177–180. doi:10.1007/s00228-002-0456-3
Foresta, C., Mioni, R., Rossato, M., Varotto, A., and Zorzi, M. (1991). Evidence for the involvement of sperm angiotensin converting enzyme in fertilization. Int. J. Androl. 14 (5), 333–339. doi:10.1111/j.1365-2605.1991.tb01101.x
Fraser, L. R. (1992). Requirements for successful mammalian sperm capacitation and fertilization. Arch. Pathol. Lab. Med. 116 (4), 345–350.
Gabrielsen, J. S., and Tanrikut, C. (2016). Chronic exposures and male fertility: the impacts of environment, diet, and drug use on spermatogenesis. Andrology 4 (4), 648–661. doi:10.1111/andr.12198
Ganjibakhsh, M., Mehraein, F., Koruji, M., and Bashiri, Z. (2022). The therapeutic potential of adipose tissue-derived mesenchymal stromal cells in the treatment of busulfan-induced azoospermic mice. J. Assist. Reprod. Genet. 39 (1), 153–163. doi:10.1007/s10815-021-02309-8
Gao, H., Cao, H., Li, Z., Li, L., Guo, Y., Chen, Y., et al. (2023). Exosome-derived small rnas in mouse sertoli cells inhibit spermatogonial apoptosis. Theriogenology 200, 155–167. doi:10.1016/j.theriogenology.2023.02.011
Gao, T., Zhao, X., Liu, C., Shao, B., Zhang, X., Li, K., et al. (2018). Somatic angiotensin i-converting enzyme regulates self-renewal of mouse spermatogonial stem cells through the mitogen-activated protein kinase signaling pathway. Stem Cells Dev. 27 (15), 1021–1032. doi:10.1089/scd.2017.0287
Ghaem, M. R., Mirzapour, T., and Bayrami, A. (2018). Differentiation of mesenchymal stem cells to germ-like cells under induction of sertoli cell-conditioned medium and retinoic acid. Andrologia 50 (3), e12887. doi:10.1111/and.12887
Ghasemzadeh-Hasankolaei, M., Batavani, R., Eslaminejad, M. B., and Sayahpour, F. (2016). Transplantation of autologous bone marrow mesenchymal stem cells into the testes of infertile male rats and new germ cell formation. Int. J. Stem Cells. 9 (2), 250–263. doi:10.15283/ijsc16010
Ghorbani, H., Akhavanrezayat, A., Jarahi, L., Memar, B., Amouian, S., Attaranzadeh, A., et al. (2021). Effects of sertraline on spermatogenesis of male rats and its reversibility after terminating the drug. Urol. J. 18 (4), 434–438. doi:10.22037/uj.v18i.6458
Goossens, E., Jahnukainen, K., Mitchell, R. T., van Pelt, A., Pennings, G., Rives, N., et al. (2020). Fertility preservation in boys: recent developments and new insights (†). Hum. Reprod. Open. 2020 (3), hoaa016. doi:10.1093/hropen/hoaa016
Goossens, E., and Tournaye, H. (2014). Male fertility preservation, where are we in 2014? Ann. Endocrinol. 75 (2), 115–117. doi:10.1016/j.ando.2014.03.011
Guo, L., Jing, J., Feng, Y. M., and Yao, B. (2015). Tamoxifen is a potent antioxidant modulator for sperm quality in patients with idiopathic oligoasthenospermia. Int. Urol. Nephrol. 47 (9), 1463–1469. doi:10.1007/s11255-015-1065-2
Guo, Y., Chen, L., Wu, D., Yu, L., Sun, H., and Zhu, Q. (2021). A comparative study of the effects of valproate and oxcarbazepine on sexual function, sperm quality, and sex hormones in males with epilepsy. Biomed. Res. Int. 2021, 6624101. doi:10.1155/2021/6624101
Gurel, C., Kuscu, G. C., Buhur, A., Dagdeviren, M., Oltulu, F., Karabay, Y. N., et al. (2019). Fluvastatin attenuates doxorubicin-induced testicular toxicity in rats by reducing oxidative stress and regulating the blood-testis barrier via mtor signaling pathway. Hum. Exp. Toxicol. 38 (12), 1329–1343. doi:10.1177/0960327119862006
Hajihoseini, M., Mehrabani, D., Vahdati, A., Hosseini, S. E., Tamadon, A., Dianatpour, M., et al. (2018). Spermatogenesis after transplantation of adipose tissue-derived stem cells in azoospermic Guinea pigs: A histological and histomorphometric study. Galen. Med. J. 7, e1000. doi:10.22086/gmj.v0i0.1000
Hamdi, H. (2019). The preventive role of wheat germ oil against sertraline-induced testicular damage in male albino rats. Andrologia 51 (10), e13369. doi:10.1111/and.13369
Hong, C. Y., Chiang, B. N., and Turner, P. (1984). Calcium ion is the key regulator of human sperm function. Lancet 2 (8417-8418), 1449–1451. doi:10.1016/s0140-6736(84)91634-9
Horvath-Pereira, B. O., Almeida, G., Da, S. J. L., Do, N. P., Horvath, P. B., Fireman, J., et al. (2023). Biomaterials for testicular bioengineering: how far have we come and where do we have to go? Front. Endocrinol. 14, 1085872. doi:10.3389/fendo.2023.1085872
Hou, M., Andersson, M., Eksborg, S., Söder, O., and Jahnukainen, K. (2007). Xenotransplantation of testicular tissue into nude mice can be used for detecting leukemic cell contamination. Hum. Reprod. 22 (7), 1899–1906. doi:10.1093/humrep/dem085
Hsiao, C. H., Ji, A. T., Chang, C. C., Cheng, C. J., Lee, L. M., and Ho, J. H. (2015). Local injection of mesenchymal stem cells protects testicular torsion-induced germ cell injury. Stem Cell. Res. Ther. 6 (1), 113. doi:10.1186/s13287-015-0079-0
Iamsaard, S., Sukhorum, W., Arun, S., Phunchago, N., Uabundit, N., Boonruangsri, P., et al. (2017). Valproic acid induces histologic changes and decreases androgen receptor levels of testis and epididymis in rats. Int. J. Reprod. Biomed. 15 (4), 217–224. doi:10.29252/ijrm.15.4.217
Ibrahim, H. F., Safwat, S. H., Zeitoun, T. M., El, M. K., and Medwar, A. Y. (2021). The therapeutic potential of amniotic fluid-derived stem cells on busulfan-induced azoospermia in adult rats. Tissue Eng. Regen. Med. 18 (2), 279–295. doi:10.1007/s13770-020-00309-w
Ilgin, S., Kilic, G., Baysal, M., Kilic, V., Korkut, B., Ucarcan, S., et al. (2017). Citalopram induces reproductive toxicity in male rats. Birth Defects Res. 109 (7), 475–485. doi:10.1002/bdr2.1010
Iranloye, B. O., Morakinyo, A. O., Uwah, J., Bello, O., and Daramola, O. A. (2009). Effect of nifedipine on reproductive functions in male rats. Nig Q. J. Hosp. Med. 19 (3), 165–168. doi:10.4314/nqjhm.v19i3.54506
Ismail, H. Y., Hussein, S., Shaker, N. A., Rizk, H., and Wally, Y. R. (2023). Stem cell treatment trials for regeneration of testicular tissue in laboratory animals. Reprod. Sci. 30 (6), 1770–1781. doi:10.1007/s43032-022-01152-1
Jahnukainen, K., Ehmcke, J., Nurmio, M., and Schlatt, S. (2012). Autologous ectopic grafting of cryopreserved testicular tissue preserves the fertility of prepubescent monkeys that receive sterilizing cytotoxic therapy. Cancer Res. 72 (20), 5174–5178. doi:10.1158/0008-5472.CAN-12-1317
Jahnukainen, K., Hou, M., Petersen, C., Setchell, B., and Söder, O. (2001). Intratesticular transplantation of testicular cells from leukemic rats causes transmission of leukemia. Cancer Res. 61 (2), 706–710.
Jiang, B., Yan, L., Wang, X., Li, E., Murphy, K., Vaccaro, K., et al. (2019). Concise review: mesenchymal stem cells derived from human pluripotent cells, an unlimited and quality-controllable source for therapeutic applications. Stem Cells 37 (5), 572–581. doi:10.1002/stem.2964
Juneja, R., Gupta, I., Wali, A., Sanyal, S. N., Chakravarti, R. N., and Majumdar, S. (1990). Effect of verapamil on different spermatozoal functions in Guinea pigs-a preliminary study. Contraception 41 (2), 179–187. doi:10.1016/0010-7824(90)90146-m
Kabel, A. M., Salama, S. A., Alghorabi, A. A., and Estfanous, R. S. (2020). Amelioration of cyclosporine-induced testicular toxicity by carvedilol and/or alpha-lipoic acid: role of tgf-β1, the proinflammatory cytokines, nrf2/ho-1 pathway and apoptosis. Clin. Exp. Pharmacol. Physiol. 47 (7), 1169–1181. doi:10.1111/1440-1681.13281
Kadam, P., Ntemou, E., Baert, Y., Van Laere, S., Van Saen, D., and Goossens, E. (2018). Co-transplantation of mesenchymal stem cells improves spermatogonial stem cell transplantation efficiency in mice. Stem Cell. Res. Ther. 9 (1), 317. doi:10.1186/s13287-018-1065-0
Kar, R. N., and Das, R. K. (1983). Induction of sperm head abnormalities in mice by three tranquilizers. Cytobios 36 (141), 45–51.
Karimaghai, N., Tamadon, A., Rahmanifar, F., Mehrabani, D., Raayat, J. A., Zare, S., et al. (2018). Spermatogenesis after transplantation of adipose tissue-derived mesenchymal stem cells in busulfan-induced azoospermic hamster. Iran. J. Basic Med. Sci. 21 (7), 660–667. doi:10.22038/IJBMS.2018.29040.7010
Khaled, E., Abo, E. F., El-Nesr, K. A., Zanaty, M. I., El-Banna, H. A., and El-Shahawy, A. (2020). An herbal nanohybrid formula of epigallocatechin gallate-chitosan-alginate efficiently restrict the sexual dysfunction adverse effect of β-adrenergic antagonist drug: propranolol. J. Biomed. Nanotechnol. 16 (4), 505–519. doi:10.1166/jbn.2020.2915
Khan, U. A., Aslam, M., and Saeed, S. A. (2004). Effect of beta adrenergic antagonist on the production of testosterone by rat’s leydig cells. J. Ayub Med. Coll. Abbottabad 16 (4), 26–28.
Köhler-Samouilidis, G., Schmidt-Adamopoulou, B., Samouilidis, S., Papaioannou, N., and Kotsaki-Kovatsi, V. P. (1997). Effects of captopril on the male reproductive organs and various semen parameters of rabbits. Berl. Munch Tierarztl Wochenschr 110 (6), 201–205.
Kumar, K., Das, K., Ap, M., Kumar, A., Singh, P., Mondal, T., et al. (2018). Rat bone marrow derived mesenchymal stem cells differentiate to germ cell like cells. Cold Spring Harbor. Germany: Cold Spring Harbor Laboratory Press. (Reprinted.
Kushwaha, S., and Jena, G. B. (2012). Enalapril reduces germ cell toxicity in streptozotocin-induced diabetic rat: investigation on possible mechanisms. Schmiedeb. Arch. Pharmacol. 385 (2), 111–124. doi:10.1007/s00210-011-0707-x
Lal, A., Pike, J., Polley, E. L., Huang, S., Sanni, M., Hailat, T., et al. (2022). Comparison of rna content from hydrophobic interaction chromatography-isolated seminal plasma exosomes from intrauterine insemination (iui) pregnancies. Andrologia 54 (2), e14325. doi:10.1111/and.14325
Latif, R., Lodhi, G. M., and Aslam, M. (2008). Effects of amlodipine on serum testosterone, testicular weight and gonado-somatic index in adult rats. J. Ayub Med. Coll. Abbottabad 20 (4), 8–10.
Lee, J. H., Kim, H., Kim, D. H., and Gye, M. C. (2006). Effects of calcium channel blockers on the spermatogenesis and gene expression in peripubertal mouse testis. Arch. Androl. 52 (4), 311–318. doi:10.1080/01485010600664024
Leite, G., de Barros, J., Martins, A. J., Anselmo-Franci, J. A., Barbosa, F. J., and Kempinas, W. G. (2019). Ascorbic acid supplementation ameliorates testicular hormonal signaling, sperm production and oxidative stress in male rats exposed to rosuvastatin during pre-puberty. J. Appl. Toxicol. 39 (2), 305–321. doi:10.1002/jat.3720
Leite, G., Figueiredo, T. M., Pacheco, T. L., Sanabria, M., Silva, P., Fernandes, F. H., et al. (2017). Vitamin c partially prevents reproductive damage in adult male rats exposed to rosuvastatin during prepuberty. Food Chem. Toxicol. 109 (1), 272–283. doi:10.1016/j.fct.2017.09.003
Leite, G., Sanabria, M., Cavariani, M. M., Anselmo-Franci, J. A., Pinheiro, P., Domeniconi, R. F., et al. (2018). Lower sperm quality and testicular and epididymal structural impairment in adult rats exposed to rosuvastatin during prepuberty. J. Appl. Toxicol. 38 (6), 914–929. doi:10.1002/jat.3599
Li, C. Y., Jiang, L. Y., Chen, W. Y., Li, K., Sheng, H. Q., Ni, Y., et al. (2010). Cftr is essential for sperm fertilizing capacity and is correlated with sperm quality in humans. Hum. Reprod. 25 (2), 317–327. doi:10.1093/humrep/dep406
Li, Y., Wu, Q., Li, X., Von Tungeln, L. S., Beland, F. A., Petibone, D., et al. (2022). In vitro effects of cannabidiol and its main metabolites in mouse and human sertoli cells. Food Chem. Toxicol. 159, 112722. doi:10.1016/j.fct.2021.112722
Liu, H., Chen, M., Liu, L., Ren, S., Cheng, P., and Zhang, H. (2018). Induction of human adipose-derived mesenchymal stem cells into germ lineage using retinoic acid. Cell. Reprogr. 20 (2), 127–134. doi:10.1089/cell.2017.0063
Liu, Z., Cao, K., Liao, Z., Chen, Y., Lei, X., Wei, Q., et al. (2020). Monophosphoryl lipid a alleviated radiation-induced testicular injury through tlr4-dependent exosomes. J. Cell. Mol. Med. 24 (7), 3917–3930. doi:10.1111/jcmm.14978
Luo, Y., Xie, L., Mohsin, A., Ahmed, W., Xu, C., Peng, Y., et al. (2019). Efficient generation of male germ-like cells derived during co-culturing of adipose-derived mesenchymal stem cells with sertoli cells under retinoic acid and testosterone induction. Stem Cell. Res. Ther. 10 (1), 91. doi:10.1186/s13287-019-1181-5
Ma, Y., Ma, Q., Sun, Y., and Chen, X. (2023). The emerging role of extracellular vesicles in the testis. Hum. Reprod. 38 (3), 334–351. doi:10.1093/humrep/dead015
Martin-Inaraja, M., Ferreira, M., Taelman, J., Eguizabal, C., and Chuva, D. S. L. S. (2021). Improving in vitro culture of human male fetal germ cells. Cells 10 (8), 2033. doi:10.3390/cells10082033
Martini, A. C., Vincenti, L. M., Santillán, M. E., Stutz, G., Kaplan, R., Ruiz, R. D., et al. (2008). Chronic administration of nonsteroidal-antiinflammatory drugs (nsaids): effects upon mouse reproductive functions. Rev. Fac. Cien Med. Univ. Nac. Cordoba 65 (2), 41–51.
Matuszewska, A., Nowak, B., Niżański, W., Eberhardt, M., Domrazek, K., Nikodem, A., et al. (2021). Long-term administration of abacavir and etravirine impairs semen quality and alters redox system and bone metabolism in growing male wistar rats. Oxidative Med. Cell. Longev. 2021, 5596090. doi:10.1155/2021/5596090
Mazhari, S., Razi, M., and Sadrkhanlou, R. (2018). Silymarin and celecoxib ameliorate experimental varicocele-induced pathogenesis: evidences for oxidative stress and inflammation inhibition. Int. Urol. Nephrol. 50 (6), 1039–1052. doi:10.1007/s11255-018-1862-5
Mbah, A. U., Ndukwu, G. O., Ghasi, S. I., Shu, E. N., Ozoemena, F. N., Mbah, J. O., et al. (2012). Low-dose lisinopril in normotensive men with idiopathic oligospermia and infertility: A 5-year randomized, controlled, crossover pilot study. Clin. Pharmacol. Ther. 91 (4), 582–589. doi:10.1038/clpt.2011.265
Means, J. R., Chengelis, C. P., and Jasty, V. (1982). Testicular toxicity induced by oral administration of sc-32855, a 1,4-benzodiazepine, in the dog. Res. Commun. Chem. Pathol. Pharmacol. 37 (2), 317–320.
Meligy, F. Y., Abo, E. A., and Alghareeb, S. M. (2019). Therapeutic effect of adipose-derived mesenchymal stem cells on cisplatin induced testicular damage in adult male albino rat. Ultrastruct. Pathol. 43 (1), 28–55. doi:10.1080/01913123.2019.1572256
Miyaso, H., Ogawa, Y., and Itoh, M. (2022). Microenvironment for spermatogenesis and sperm maturation. Histochem. Cell. Biol. 157 (3), 273–285. doi:10.1007/s00418-021-02071-z
Mobarak, H., Heidarpour, M., Rahbarghazi, R., Nouri, M., and Mahdipour, M. (2021). Amniotic fluid-derived exosomes improved spermatogenesis in a rat model of azoospermia. Life Sci. 274, 119336. doi:10.1016/j.lfs.2021.119336
Monsefi, M., Fereydouni, B., Rohani, L., and Talaei, T. (2013). Mesenchymal stem cells repair germinal cells of seminiferous tubules of sterile rats. Iran. J. Reprod. Med. 11 (7), 537–544.
Moraveji, S. F., Esfandiari, F., Sharbatoghli, M., Taleahmad, S., Nikeghbalian, S., Shahverdi, A., et al. (2019). Optimizing methods for human testicular tissue cryopreservation and spermatogonial stem cell isolation. J. Cell. Biochem. 120 (1), 613–621. doi:10.1002/jcb.27419
Murphy, M. B., Moncivais, K., and Caplan, A. I. (2013). Mesenchymal stem cells: environmentally responsive therapeutics for regenerative medicine. Exp. Mol. Med. 45 (11), e54. doi:10.1038/emm.2013.94
Naderi, M., Ahangar, N., Badakhshan, F., Ghasemi, M., and Shaki, F. (2021). Zinc and selenium supplement mitigated valproic acid-induced testis toxicity by modulating the oxidative redox balance in male rats. Anat. Cell. Biol. 54 (3), 387–394. doi:10.5115/acb.20.280
Nayernia, K., Nolte, J., Michelmann, H. W., Lee, J. H., Rathsack, K., Drusenheimer, N., et al. (2006). In vitro-differentiated embryonic stem cells give rise to male gametes that can generate offspring mice. Dev. Cell. 11 (1), 125–132. doi:10.1016/j.devcel.2006.05.010
Nelson, L., and Cariello, L. (1989). Adrenergic stimulation of sea urchin sperm cells. Gamete Res. 24 (3), 291–302. doi:10.1002/mrd.1120240306
Neto, F. T., Bach, P. V., Najari, B. B., Li, P. S., and Goldstein, M. (2016). Spermatogenesis in humans and its affecting factors. Semin. Cell. Dev. Biol. 59, 10–26. doi:10.1016/j.semcdb.2016.04.009
Nickkholgh, B., Mizrak, S. C., van Daalen, S. K., Korver, C. M., Sadri-Ardekani, H., Repping, S., et al. (2014). Genetic and epigenetic stability of human spermatogonial stem cells during long-term culture. Fertil. Steril. 102 (6), 1700–1707. doi:10.1016/j.fertnstert.2014.08.022
Nishimura, T., Sakai, M., and Yonezawa, H. (2000). Effects of valproic acid on fertility and reproductive organs in male rats. J. Toxicol. Sci. 25 (2), 85–93. doi:10.2131/jts.25.85
Nusier, M. K., Bataineh, H. N., and Daradka, H. M. (2007). Adverse effects of propranolol on reproductive function in adult male mice. Pak J. Biol. Sci. 10 (16), 2728–2731. doi:10.3923/pjbs.2007.2728.2731
Okeahialam, B. N., Amadi, K., and Ameh, A. S. (2006). Effect of lisnopril, an angiotensin converting enzyme (ace) inhibitor on spermatogenesis in rats. Arch. Androl. 52 (3), 209–213. doi:10.1080/01485010500398012
Önen, S., Atik, A. C., Gizer, M., Köse, S., Yaman, Ö., Külah, H., et al. (2023). A pumpless monolayer microfluidic device based on mesenchymal stem cell-conditioned medium promotes neonatal mouse in vitro spermatogenesis. Stem Cell. Res. Ther. 14 (1), 127. doi:10.1186/s13287-023-03356-x
Otoom, S., Batieneh, H., Hassan, Z., and Daoud, A. (2004). Effects of long-term use topiramate on fertility and growth parameter in adult male rats. Neuroendocrinol. Lett. 25 (5), 351–355.
Ourique, G. M., Pês, T. S., Saccol, E. M., Finamor, I. A., Glanzner, W. G., Baldisserotto, B., et al. (2016a). Resveratrol prevents oxidative damage and loss of sperm motility induced by long-term treatment with valproic acid in wistar rats. Exp. Toxicol. Pathol. 68 (8), 435–443. doi:10.1016/j.etp.2016.07.001
Ourique, G. M., Saccol, E. M., Pês, T. S., Glanzner, W. G., Schiefelbein, S. H., Woehl, V. M., et al. (2016b). Protective effect of vitamin e on sperm motility and oxidative stress in valproic acid treated rats. Food Chem. Toxicol. 95, 159–167. doi:10.1016/j.fct.2016.07.011
Patra, P. B., and Wadsworth, R. M. (1991). Quantitative evaluation of spermatogenesis in mice following chronic exposure to cannabinoids. Andrologia 23 (2), 151–156. doi:10.1111/j.1439-0272.1991.tb02520.x
Pendleton, C., Li, Q., Chesler, D. A., Yuan, K., Guerrero-Cazares, H., and Quinones-Hinojosa, A. (2013). Mesenchymal stem cells derived from adipose tissue vs bone marrow: in vitro comparison of their tropism towards gliomas. PLoS One 8 (3), e58198. doi:10.1371/journal.pone.0058198
Peris, S., Solanes, D., Peña, A., Enric-Rodríguez-Gil, J., and Riga, T. (2000). Ion-mediated resistance to osmotic changes of ram spermatozoa: the role of amiloride and ouabain. Theriogenology 54 (9), 1453–1467. doi:10.1016/s0093-691x(00)00467-2
Pietzak, E. R., Tasian, G. E., Tasian, S. K., Brinster, R. L., Carlson, C., Ginsberg, J. P., et al. (2015). Histology of testicular biopsies obtained for experimental fertility preservation protocol in boys with cancer. J. Urol. 194 (5), 1420–1424. doi:10.1016/j.juro.2015.04.117
Portela, J., de Winter-Korver, C. M., van Daalen, S., Meißner, A., de Melker, A. A., Repping, S., et al. (2019). Assessment of fresh and cryopreserved testicular tissues from (pre)pubertal boys during organ culture as a strategy for in vitro spermatogenesis. Hum. Reprod. 34 (12), 2443–2455. doi:10.1093/humrep/dez180
Prasad, P., Ogawa, S., and Parhar, I. S. (2015). Serotonin reuptake inhibitor citalopram inhibits gnrh synthesis and spermatogenesis in the male zebrafish. Biol. Reprod. 93 (4), 102. doi:10.1095/biolreprod.115.129965
Purvis, K., Tollefsrud, A., Rui, H., Haug, E., Norseth, J., Viksmoen, L., et al. (1992). Short-term effects of treatment with simvastatin on testicular function in patients with heterozygous familial hypercholesterolaemia. Eur. J. Clin. Pharmacol. 42 (1), 61–64. doi:10.1007/BF00314921
Qian, C., Meng, Q., Lu, J., Zhang, L., Li, H., and Huang, B. (2020). Human amnion mesenchymal stem cells restore spermatogenesis in mice with busulfan-induced testis toxicity by inhibiting apoptosis and oxidative stress. Stem Cell. Res. Ther. 11 (1), 290. doi:10.1186/s13287-020-01803-7
Qiu, J., Ma, H., and Pei, Y. (2023). Efficacy of cellular therapy for azoospermia in animal models: A systematic review and meta-analysis. Exp. Clin. Transpl. 21 (3), 197–210. doi:10.6002/ect.2022.0327
Qu, Y., He, Y., Meng, B., Zhang, X., Ding, J., Kou, X., et al. (2022). Apoptotic vesicles inherit sox2 from pluripotent stem cells to accelerate wound healing by energizing mesenchymal stem cells. Acta Biomater. 149, 258–272. doi:10.1016/j.actbio.2022.07.009
Rahban, R., Rehfeld, A., Schiffer, C., Brenker, C., Egeberg, P. D., Wang, T., et al. (2021). The antidepressant sertraline inhibits catsper ca2+ channels in human sperm. Hum. Reprod. 36 (10), 2638–2648. doi:10.1093/humrep/deab190
Rai, U., and Nirmal, B. K. (2003). Significance of regional difference in ion concentrations in lizard, hemidactylus flaviviridis (rüppell): assessment of ionic influence on sperm motility in vitro. Indian J. Exp. Biol. 41 (12), 1431–1435.
Ramos, A. C., H, D. S. A., Silveira, K. M., Kiss, A. C., Mesquita, S. F., and Gerardin, D. C. (2015). Maternal treatment with fluoxetine promotes testicular alteration in male rat pups. Reprod. Fertil. Dev. doi:10.1071/RD14199
Ramzy, M. M., El-Sheikh, A. A., Kamel, M. Y., Abdelwahab, S. A., and Morsy, M. A. (2014). Mechanism of testicular protection of carvedilol in streptozotocin-induced diabetic rats. Indian J. Pharmacol. 46 (2), 161–165. doi:10.4103/0253-7613.129307
Rosen, R. C., Kostis, J. B., and Jekelis, A. W. (1988). Beta-blocker effects on sexual function in normal males. Arch. Sex. Behav. 17 (3), 241–255. doi:10.1007/BF01541742
Røste, L. S., TaubØll, E., Berner, A., Berg, K. A., Aleksandersen, M., and Gjerstad, L. (2001). Morphological changes in the testis after long-term valproate treatment in male wistar rats. Seizure 10 (8), 559–565. doi:10.1053/seiz.2001.0545
Sabapathy, V., and Kumar, S. (2016). Hipsc-derived imscs: nextgen mscs as an advanced therapeutically active cell resource for regenerative medicine. J. Cell. Mol. Med. 20 (8), 1571–1588. doi:10.1111/jcmm.12839
Sagaradze, G. D., Basalova, N. A., Kirpatovsky, V. I., Ohobotov, D. A., Grigorieva, O. A., Balabanyan, V. Y., et al. (2019). Application of rat cryptorchidism model for the evaluation of mesenchymal stromal cell secretome regenerative potential. Biomed. Pharmacother. 109, 1428–1436. doi:10.1016/j.biopha.2018.10.174
Saha, L., Bhargava, V. K., Garg, S. K., and Majumdar, S. (2000a). Effect of nimodipine on male reproductive functions in rats. Indian J. Physiol. Pharmacol. 44 (4), 449–455.
Saha, L., Garg, S. K., Bhargava, V. K., and Mazumdar, S. (2000b). Role of angiotensin-converting enzyme inhibitor, lisinopril, on spermatozoal functions in rats. Methods Find. Exp. Clin. Pharmacol. 22 (3), 159–162. doi:10.1358/mf.2000.22.3.796102
Sakr, H. F., Abbas, A. M., Elsamanoudy, A. Z., and Ghoneim, F. M. (2015). Effect of fluoxetine and resveratrol on testicular functions and oxidative stress in a rat model of chronic mild stress-induced depression. J. Physiol. Pharmacol. 66 (4), 515–527.
Saksena, S. K., Lau, I. F., Bartke, A., and Chang, M. C. (1975). Effect of indomethacin on blood plasma levels of lh and testosterone in male rats. J. Reprod. Fertil. 42 (2), 311–317. doi:10.1530/jrf.0.0420311
Salek, F., Baharara, J., Shahrokhabadi, K. N., and Amini, E. (2021). The guardians of germ cells; Sertoli-derived exosomes against electromagnetic field-induced oxidative stress in mouse spermatogonial stem cells. Theriogenology 173, 112–122. doi:10.1016/j.theriogenology.2021.08.001
Salem, H. M., Mohamed, T. K., Badary, D. M., Ahmed, M. W., and Mohamed, M. E. (2020). Pregabalin administration and withdrawal affect testicular structure and functions in rats. Andrologia 52 (11), e13808. doi:10.1111/and.13808
Sanbuissho, A., Terada, S., Suzuki, K., Masuda, N., Teranishi, M., and Masuda, H. (1995). Male reproductive toxicity study of nitrazepam in rats. J. Toxicol. Sci. 20 (3), 319–328. doi:10.2131/jts.20.319
Sanou, I., van Maaren, J., Eliveld, J., Lei, Q., Meißner, A., de Melker, A. A., et al. (2022). Spermatogonial stem cell-based therapies: taking preclinical research to the next level. Front. Endocrinol. 13, 850219. doi:10.3389/fendo.2022.850219
Serrano, J. B., Tabeling, N. C., de Winter-Korver, C. M., van Daalen, S., van Pelt, A., and Mulder, C. L. (2023). Sperm dna methylation is predominantly stable in mice offspring born after transplantation of long-term cultured spermatogonial stem cells. Clin. Epigenetics. 15 (1), 58. doi:10.1186/s13148-023-01469-x
Shalaby, M. A., El-Zorba, H. Y., and Kamel, G. M. (2004). Effect of alpha-tocopherol and simvastatin on male fertility in hypercholesterolemic rats. Pharmacol. Res. 50 (2), 137–142. doi:10.1016/j.phrs.2003.10.013
Sharma, P., Kaushal, N., Saleth, L. R., Ghavami, S., Dhingra, S., and Kaur, P. (2023). Oxidative stress-induced apoptosis and autophagy: balancing the contrary forces in spermatogenesis. Biochim. Biophys. Acta-Mol. Basis Dis. 1869 (6), 166742. doi:10.1016/j.bbadis.2023.166742
Sharma, S., Wistuba, J., Pock, T., Schlatt, S., and Neuhaus, N. (2019). Spermatogonial stem cells: updates from specification to clinical relevance. Hum. Reprod. Update. 25 (3), 275–297. doi:10.1093/humupd/dmz006
Sherif, I. O., Sabry, D., Abdel-Aziz, A., and Sarhan, O. M. (2018). The role of mesenchymal stem cells in chemotherapy-induced gonadotoxicity. Stem Cell. Res. Ther. 9 (1), 196. doi:10.1186/s13287-018-0946-6
Shlush, E., Maghen, L., Swanson, S., Kenigsberg, S., Moskovtsev, S., Barretto, T., et al. (2017). In vitro generation of sertoli-like and haploid spermatid-like cells from human umbilical cord perivascular cells. Stem Cell. Res. Ther. 8 (1), 37. doi:10.1186/s13287-017-0491-8
Shokry, D. A., El, N. N., Yassa, H. D., Gaber, S. S., Batiha, G. E., and Welson, N. N. (2020). Pregabalin induced reproductive toxicity and body weight changes by affecting caspase3 and leptin expression: protective role of wheat germ oil. Life Sci. 260, 118344. doi:10.1016/j.lfs.2020.118344
Simon, C., Greening, D. W., Bolumar, D., Balaguer, N., Salamonsen, L. A., and Vilella, F. (2018). Extracellular vesicles in human reproduction in health and disease. Endocr. Rev. 39 (3), 292–332. doi:10.1210/er.2017-00229
Siregar, S., Noegroho, B. S., Adriansjah, R., Mustafa, A., and Bonar, A. (2021). The effect of intratesticular injection of human adipose-derived mesenchymal cell on testicular oxidative stress and spermatogenesis process in the varicocele rat model. Res. Rep. Urol. 13, 759–765. doi:10.2147/RRU.S330634
Sm, M., Nm, E., Sf, M., Ni, H., Sy, S., Mr, B., et al. (2017). Effect of stem cell therapy on gentamicin induced testicular dysfunction in rats. J. Health & Med. Inf. 08 (03). doi:10.4172/2157-7420.1000263
Smarr, M. M., Kannan, K., Chen, Z., Kim, S., and Buck, L. G. (2017). Male urinary paracetamol and semen quality. Andrology 5 (6), 1082–1088. doi:10.1111/andr.12413
Sojoudi, K., Azizi, H., and Skutella, T. (2023). A review of the potential of induced pluripotent stem cell-derived exosome as a novel treatment for male infertility. Biotechnol. Genet. Eng. Rev., 1–26. doi:10.1080/02648725.2023.2193772
Sołek, P., Mytych, J., Tabęcka-Łonczyńska, A., and Koziorowski, M. (2021). Molecular consequences of depression treatment: A potential in vitro mechanism for antidepressants-induced reprotoxic side effects. Int. J. Mol. Sci. 22 (21), 11855. doi:10.3390/ijms222111855
Solek, P., Mytych, J., Tabecka-Lonczynska, A., Sowa-Kucma, M., and Koziorowski, M. (2021). Toxic effect of antidepressants on male reproductive system cells: evaluation of possible fertility reduction mechanism. J. Physiol. Pharmacol. 72 (3). doi:10.26402/jpp.2021.3.06
Sukhorum, W., and Iamsaard, S. (2017). Changes in testicular function proteins and sperm acrosome status in rats treated with valproic acid. Reproduction, Fertil. Dev. 29 (8), 1585–1592. doi:10.1071/RD16205
Suzuki, H., Tominaga, T., Kumagai, H., and Saruta, T. (1988). Effects of first-line antihypertensive agents on sexual function and sex hormones. J. Hypertens. Suppl. 6 (4), S649–S651. doi:10.1097/00004872-198812040-00204
Taheri, B., Soleimani, M., Fekri, A. S., Esmaeili, E., Bazi, Z., and Zarghami, N. (2019). Induced pluripotent stem cell-derived extracellular vesicles: A novel approach for cell-free regenerative medicine. J. Cell. Physiol. 234 (6), 8455–8464. doi:10.1002/jcp.27775
Taha, S., Zaghloul, H. S., Ali, A., Rashed, L. A., Sabry, R. M., and Gaballah, I. F. (2020). Molecular and hormonal changes caused by long-term use of high dose pregabalin on testicular tissue: the role of p38 mapk, oxidative stress and apoptosis. Mol. Biol. Rep. 47 (11), 8523–8533. doi:10.1007/s11033-020-05894-6
Taher, M. A., Zainab, N. H., and Anber, H. (2019). Effect of diazepam on the reproductive system in male rats.
Takahashi, K., and Yamanaka, S. (2006). Induction of pluripotent stem cells from mouse embryonic and adult fibroblast cultures by defined factors. Cell. 126 (4), 663–676. doi:10.1016/j.cell.2006.07.024
Tanrikut, C., Feldman, A. S., Altemus, M., Paduch, D. A., and Schlegel, P. N. (2010). Adverse effect of paroxetine on sperm. Fertil. Steril. 94 (3), 1021–1026. doi:10.1016/j.fertnstert.2009.04.039
Tektemur, A., Etem, Ö. E., Kaya, T. N., Dayan, C. S., Kılınçlı, Ç. A., Tekedereli, 0., et al. (2021). Carbamazepine-induced sperm disorders can be associated with the altered expressions of testicular kcnj11/mir-let-7a and spermatozoal cftr/mir-27a. Andrologia 53 (2), e13954. doi:10.1111/and.13954
Thiageswaran, S., Steele, H., Voigt, A. L., and Dobrinski, I. (2022). A role for exchange of extracellular vesicles in porcine spermatogonial co-culture. Int. J. Mol. Sci. 23 (9), 4535. doi:10.3390/ijms23094535
Valli-Pulaski, H., Peters, K. A., Gassei, K., Steimer, S. R., Sukhwani, M., Hermann, B. P., et al. (2019). Testicular tissue cryopreservation: 8 years of experience from a coordinated network of academic centers. Hum. Reprod. 34 (6), 966–977. doi:10.1093/humrep/dez043
Vander, B. M., and Wyns, C. (2018). Fertility and infertility: definition and epidemiology. Clin. Biochem. 62, 2–10. doi:10.1016/j.clinbiochem.2018.03.012
Vieira, M. L., Hamada, R. Y., Gonzaga, N. I., Bacchi, A. D., Barbieri, M., Moreira, E. G., et al. (2013). Could maternal exposure to the antidepressants fluoxetine and st. John's wort induce long-term reproductive effects on male rats? Reprod. Toxicol. 35, 102–107. doi:10.1016/j.reprotox.2012.07.006
Wang, A. (2021). Human induced pluripotent stem cell-derived exosomes as a new therapeutic strategy for various diseases. Int. J. Mol. Sci. 22 (4), 1769. doi:10.3390/ijms22041769
Wang, M., Liu, X., Chang, G., Chen, Y., An, G., Yan, L., et al. (2018). Single-cell rna sequencing analysis reveals sequential cell fate transition during human spermatogenesis. Cell. Stem Cell. 23 (4), 599–614. doi:10.1016/j.stem.2018.08.007
Whelan, E. C., Yang, F., Avarbock, M. R., Sullivan, M. C., Beiting, D. P., and Brinster, R. L. (2022). Reestablishment of spermatogenesis after more than 20 years of cryopreservation of rat spermatogonial stem cells reveals an important impact in differentiation capacity. PLoS. Biol. 20 (5), e3001618. doi:10.1371/journal.pbio.3001618
White, D. R., Clarkson, J. S., Ratnasooriya, W. D., and Aitken, R. J. (1995). Complementary effects of propranolol and nonoxynol-9 upon human sperm motility. Contraception 52 (4), 241–247. doi:10.1016/0010-7824(95)00190-l
Wiger, R., Hongslo, J. K., Evenson, D. P., De Angelis, P., Schwarze, P. E., and Holme, J. A. (1995). Effects of acetaminophen and hydroxyurea on spermatogenesis and sperm chromatin structure in laboratory mice. Reprod. Toxicol. 9 (1), 21–33. doi:10.1016/0890-6238(94)00052-x
Wong, P. Y., Lee, W. M., and Tsang, A. Y. (1981). The effects of sodium and amiloride on the motility of the caudal epididymal spermatozoa of the rat. Experientia 37 (1), 69–71. doi:10.1007/BF01965575
Wu, D., Chen, L., Ji, F., Si, Y., and Sun, H. (2018). The effects of oxcarbazepine, levetiracetam, and lamotrigine on semen quality, sexual function, and sex hormones in male adults with epilepsy. Epilepsia 59 (7), 1344–1350. doi:10.1111/epi.14450
Yakubu, M. T., and Atoyebi, A. R. (2018). Brysocarpus coccineus (schum & thonn) root reinstates sexual competence and testicular function in paroxetine-induced sexual dysfunction in male wistar rats. Andrologia 50, e12980. doi:10.1111/and.12980
Yardimci, A., Ulker, N., Bulmus, O., Kaya, N., Colakoglu, N., Ozcan, M., et al. (2019). Effects of long-term paroxetine or bupropion treatment on puberty onset, reproductive and feeding parameters in adolescent male rats. Andrologia 51 (6), e13268. doi:10.1111/and.13268
Zarandi, N. P., Galdon, G., Kogan, S., Atala, A., and Sadri-Ardekani, H. (2018). Cryostorage of immature and mature human testis tissue to preserve spermatogonial stem cells (sscs): A systematic review of current experiences toward clinical applications. Stem Cells Cloning Adv. Appl. 11, 23–38. doi:10.2147/SCCAA.S137873
Zegers-Hochschild, F., Adamson, G. D., Dyer, S., Racowsky, C., de Mouzon, J., Sokol, R., et al. (2017). The international glossary on infertility and fertility care, 2017. Fertil. Steril. 108 (3), 393–406. doi:10.1016/j.fertnstert.2017.06.005
Zhang, D., Liu, X., Peng, J., He, D., Lin, T., Zhu, J., et al. (2014). Potential spermatogenesis recovery with bone marrow mesenchymal stem cells in an azoospermic rat model. Int. J. Mol. Sci. 15 (8), 13151–13165. doi:10.3390/ijms150813151
Zhang, W., Cheng, Y., Guo, W., Guo, X., Bian, J., Zhou, Q., et al. (2018b). rotective effect of bone marrow mesenchymal stem cells-derived exosomes against testicular ischemia-reperfusion injury in rats. J. South Med. Univ. 38 (8), 910–916. doi:10.3969/j.issn.1673-4254.2018.08.02
Zhang, W., Yang, C., Guo, W., Guo, X., Bian, J., Zhou, Q., et al. (2018a). Rotective effect of bone marrow mesenchymal stem cells-derived exosomes against testicular ischemia-reperfusion injury in rats. Nan Fang. Yi Ke Da Xue Xue Bao 38 (8), 910–916. doi:10.3969/j.issn.1673-4254.2018.08.02
Zhankina, R., Baghban, N., Askarov, M., Saipiyeva, D., Ibragimov, A., Kadirova, B., et al. (2021). Mesenchymal stromal/stem cells and their exosomes for restoration of spermatogenesis in non-obstructive azoospermia: A systemic review. Stem Cell. Res. Ther. 12 (1), 229. doi:10.1186/s13287-021-02295-9
Zhao, L., Yao, C., Xing, X., Jing, T., Li, P., Zhu, Z., et al. (2020). Single-cell analysis of developing and azoospermia human testicles reveals central role of sertoli cells. Nat. Commun. 11 (1), 5683. doi:10.1038/s41467-020-19414-4
Zhao, N., Sheng, M., Wang, X., Li, Y., and Farzaneh, M. (2021). Differentiation of human induced pluripotent stem cells into male germ cells. Curr. Stem Cell. Res. Ther. 16 (5), 622–629. doi:10.2174/1574888X15666200705214223
Zhou, Q., Wang, M., Yuan, Y., Wang, X., Fu, R., Wan, H., et al. (2016). Complete meiosis from embryonic stem cell-derived germ cells in vitro. Cell. Stem Cell. 18 (3), 330–340. doi:10.1016/j.stem.2016.01.017
Keywords: male fertility, clinical medications, spermatogenesis, stem cell therapy, exosomes therapy
Citation: Mo P, Zhao Z, Ke X, Fan Y and Li C (2023) Effects of clinical medications on male fertility and prospects for stem cell therapy. Front. Cell Dev. Biol. 11:1258574. doi: 10.3389/fcell.2023.1258574
Received: 14 July 2023; Accepted: 07 September 2023;
Published: 18 September 2023.
Edited by:
Bin Jiang, Shenzhen Second People’s Hospital, ChinaReviewed by:
Dejin Zheng, Michigan State University, United StatesXufeng Fu, Ningxia Medical University, China
Li Yan, University of Maryland, College Park, United States
Copyright © 2023 Mo, Zhao, Ke, Fan and Li. This is an open-access article distributed under the terms of the Creative Commons Attribution License (CC BY). The use, distribution or reproduction in other forums is permitted, provided the original author(s) and the copyright owner(s) are credited and that the original publication in this journal is cited, in accordance with accepted academic practice. No use, distribution or reproduction is permitted which does not comply with these terms.
*Correspondence: Yong Fan, eW9uZ2ZhbjAxMUBnemhtdS5lZHUuY24=; Chaohui Li, Y2hhb2h1aWxpMjAyMUBnemhtdS5lZHUuY24=