Defining the Roles of the Cation Diffusion Facilitators in Fe2+/Zn2+ Homeostasis and Establishment of Their Participation in Virulence in Pseudomonas aeruginosa
- Consejo Nacional de Investigaciones Científicas y Técnicas, Instituto de Investigación Médica Mercedes y Martín Ferreyra, Universidad Nacional de Córdoba, Córdoba, Argentina
Transporters of the cation diffusion facilitator (CDF) family form dimers that export transition metals from the cytosol. The opportunistic pathogen Pseudomonas aeruginosa encodes three homologous CDF genes, czcD (PA0397), aitP (PA1297), and yiiP (PA3963). The three proteins are required for virulence in a plant host model. Disruption of the aitP gene leads to higher Fe2+ and Co2+ sensitivity together with an intracellular accumulation of these ions and to a decreased survival in presence of H2O2. Strains lacking czcD and yiiP showed low Zn2+ sensitivity. However, in iron-rich media and in the presence of Zn2+ these strains secreted higher levels of the iron chelator pyoverdine. Disruption of czcD and yiiP in a non-pyoverdine producer strain and lacking the Zn2+-transporting ATPase, increased the Zn2+ sensitivity and the accumulation of this ion. Most importantly, independent of the pyoverdine production strains lacking CzcD or YiiP, presented lower resistance to imipenem, ciprofloxacin, chloramphenicol, and gentamicin. These observations correlated with a lower survival rate upon EDTA-lysozyme treatment and overexpression of OprN and OprD porins. We hypothesize that while AitP is an Fe2+/Co2+ efflux transporter required for Fe2+ homeostasis, and ultimately redox stress handling, CzcD, and YiiP export Zn2+ to the periplasm for proper Zn2+-dependent signaling regulating outer membrane stability and therefore antibiotic tolerance.
Introduction
Transition metals (TM) participate in vital physiological processes such as respiration, reactive oxygen species handling, and transcriptional regulation (Fraústo Da Silva and Williams, 2001). During infection processes, bacteria face host-driven changes in TM bioavailability, leading to their scarcity or abundance, both conditions detrimental for the bacteria. An excess of these nutrients leads to cellular toxicity, through mechanisms involving the displacement of cognate metals from catalytic and structural sites of metalloproteins (Ranquet et al., 2007), or by producing reactive oxygen species through Fenton-like reactions (Macomber and Imlay, 2009; Imlay, 2014). On the other hand, metal scarcity requires a highly regulated metabolic machinery to ensure metalloprotein synthesis (Argüello et al., 2013; Kehl-Fie et al., 2013). A large number of membrane transporter families participate specifically in metal efflux or uptake in order to maintain each individual TM quota and satisfy the metabolic demand.
The cation diffusion facilitator (CDF) family participates in TM efflux from the cytosol to the periplasm (Nies, 2003). TM efflux is coupled to H+ entry and thus is driven by the electrochemical H+-gradient across the bacterial plasma membrane (Guffanti et al., 2002; Gupta et al., 2014). Members of the family present six transmembrane segments (TMS) and a cytosolic C-terminal domain (CTD) involved in transport regulation and protein dimerization. In most cases, metal transport is accomplished by a homodimeric structure, although, in organisms bearing several CDF paralogs, molecular and phenotypic evidence suggests that functional heterodimerization occurs (Uebe et al., 2011). The crystal structure of the Zn2+ transporting E. coli member YiiP revealed three metal binding sites (A, B, and C; Lu et al., 2009). Site A is formed by residues located in TMS 2 and TMS 5 and is indispensable for transport, while sites B and C seem to be unrelated to this process. However, site C is involved in allosteric regulation by coupling the sensing of cytoplasmic Zn2+ to the binding of the ion at site A.
Cellular roles for CDF transporters have mostly been linked to transition metal tolerance, i.e., exporting metal surplus from the cell to avoid excessive accumulation and toxicity. In Streptococcus penumoniae, virulence is reduced when the function of the CDF transporter, MntE, is abolished (Rosch et al., 2009). The mutant strain accumulates Mn2+ specifically when grown in the presence of this ion. Similarly, in Sinorhizobium meliloti, the CDF YiiP plays a role in Mn2+ efflux and plants infected with this strain grow poorly due to a lesser infection capacity of the bacteria (Raimunda and Elso-Berberian, 2014). In humans, mutations in the gene coding for the ortholog ZnT10 lead to Parkinsonism with concomitant hypermanganesemia (Quadri et al., 2012).
More than half of whole genome-sequenced bacteria have two or more CDF paralog genes encoded in their genome (Ren et al., 2007). In these organisms, it is probable that different CDF proteins transport alternative substrates. Alternatively, the presence of CDF transporters sharing similar substrate specificity with members of other families in one organism opens the possibility to inquire about the different roles played among these, or support functional redundancy.
P. aeruginosa PAO1 has three uncharacterized CDF transporters in loci PA0397, PA1297, and PA3963. Two of them, PA0397 and PA3963, share high sequence similarity to the Zn2+-transporting E. coli member YiiP and thus a role in Zn2+ metal tolerance can be inferred. However, existing evidence indicating that the P. aeruginosa Zn2+-ATPase ZntA participates in the ion tolerance (M. González-Guerrero personal communication), argues against this possibility. PA1297 presents a poly-his stretch between TMS 4 and TMS 5 and belongs to the Zn2+ transporting sub-class (Montanini et al., 2007) although recent evidence supports also a role in Co2+ homeostasis (Cubillas et al., 2013). The absence of an Fe2+/Co2+ transporting ATPase in P. aeruginosa PAO1 suggests that PA1297 product may play a role in Fe2+/Co2+ homeostasis.
In this study we evaluated the specificity of transport, the cellular roles and the participation in infection of the P. aeruginosa CDF members. Our data suggest that they all are required for proper virulence and that this is linked to their roles in export of transition metals. One member, AitP (Alternative iron transport protein, PA1297), exports Fe2+/Co2+, while CzcD (PA0397) and YiiP (PA3963) are involved in Zn2+ efflux. Importantly, Fe2+ transport through AitP protects against redox stress, while Zn2+ transport through CzcD and YiiP is coupled to outer membrane permeability.
Materials and Methods
Bacterial Strains and Growth Conditions
Pseudomonas aeruginosa PAO1 wild-type strain, czcD::dTn5 (PW1733/PA0397 insertional mutant), aitP::dTn5 (PW3352/PA1297 insertional mutant) and yiiP::dTn5 (PW7707 /PA3963 insertional mutant) were obtained from the Comprehensive P. aeruginosa Transposon Mutant Library at the University of Washington Genome Center (Jacobs et al., 2003; Table 1). Strains lacking pyoverdine were generated from parental strain P. aeruginosa PAO1, czcD::dTn5 and yiiP::dTn5 by insertional mutation. An internal fragment corresponding to 600 bp of the gene PA2386/pvdA was cloned into pCHESIΩ-Km, between EcoRI and BamHI sites (Llamas et al., 2003). Subsequently, the plasmid was mobilized from E. coli DH5α cells into P. aeruginosa by triparental conjugation using E. coli HB101 (pRK2013) strain as helper. The resulting strains SM010 (pvdA mutant), DM011 (pvdA and czcD mutant) and DM013 (pvdA and yiiP mutant) were used to generate the mutants lacking the Zn2+ transporting ATPase, ZntA. An internal fragment corresponding to 450 bp of the gene PA3690/zntA was cloned into pJQ200SK-Gm (Quandt and Hynes, 1993) and mobilized into P. aeruginosa mutant strains as described above resulting in DM110 (zntA and pvdA mutant), TM111 (zntA, pvdA, and czcD mutant) and TM113 (zntA, pvdA, and yiiP mutant; Table 1). Complemented strains were obtained by cloning each CDF plus 500 bp of the putative promoter region in pUC18T-mini-Tn7T-Gm (Choi and Schweizer, 2006). Since yiiP is predicted to be part of a polycistronic operon, the 500 bp upstream region of the contiguous loci PA3962 was cloned and joined to yiiP. The megaprimer-PCR method was employed to obtain the fusion (Sambrook et al., 1989). The primers used in this and other procedures are listed in Table 1. The resulting vectors were inserted in the genome of P. aeruginosa by tetraparental mating conjugation method as described (Choi and Schweizer, 2006). Transconjugants were selected on selective Luria–Bertani (LB) agar plates. All constructs and mutants were confirmed by DNA sequencing. Cells were grown at 37°C in LB or Müller-Hinton media as indicated, supplemented with tetracycline (30–60 μg/ml), gentamicin (30 μg/ml), and kanamycin (300 μg/ml) as required.
Bioinformatics Analyses
All sequences in the study were retrieved from the NCBI database. Alignments were performed in MUSCLE (Edgar, 2004), and analyzed with ESPript software (Gouet et al., 1999). E. coli YiiP, B. subtilis CzcD, and M. smegmatis ZitA were included in the alignment for comparison. The proteins that were not on KEGG were named with a two-letter key to denote their organismal origin, as indicated in Figure 1. Evolutionary analyses were conducted in MEGA6 (Tamura et al., 2013). Searches for poly-his CDF and Fe2+/Co2+-ATPases were performed in BlastP (PHI-Blast) using as query the protein sequence of P. aeruginosa AitP (accession number NP_249988.1) and M. semgmatis CtpD (accession number AFP41699.1) and against bacterial reference genomes database. Searches were oriented with H-H-H-[HD] or H-E-G-[GS]-T and the sequences downloaded were inspected visually. A list with the bacterial reference organism names containing CDF poly-his, PIB4-ATPase or both was generated and used to create a Venn diagram.
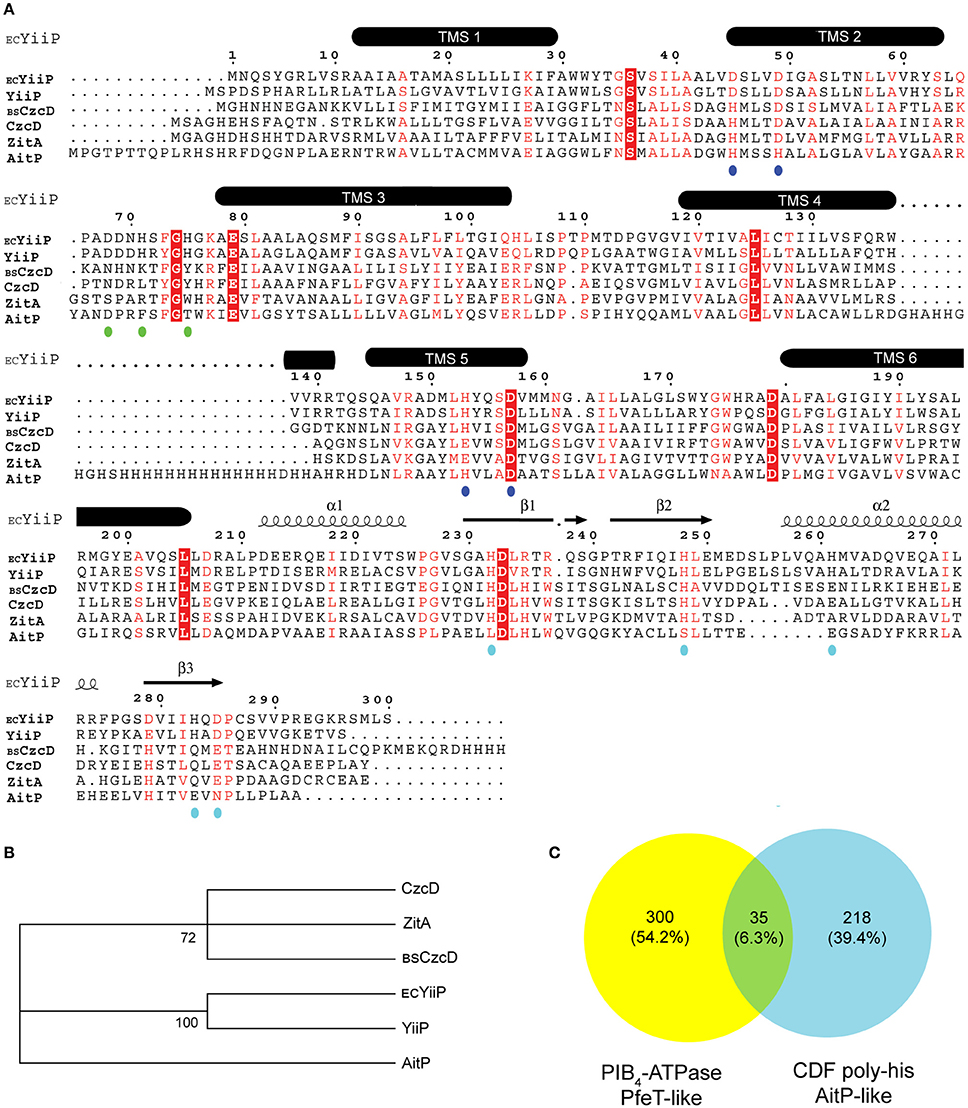
Figure 1. Multiple protein sequence alignment of CDF members in Pseudomonas aeruginosa PAO1 with previously characterized homologous members and analysis of the presence of Fe2+ exporters in reference bacterial genomes. Organisms and corresponding loci/protein names used are: P. aeruginosa PAO1, PA3963/YiiP, PA0397/CzcD, and PA1297/AitP; Escherichia coli K12-MG1655, fieF/ECYiiP; Bacillus subtilis 168, czcD/BSCzcD; Mycobacterium smegmatis mc2155, MSMEG_0755/ZitA. TMS, transmembrane segments. Putative residues in metal binding sites A, B, and C are indicated with blue, green, and cyan dots, respectively. Conserved residues are shown in white letters and red boxes. Red letters indicate conservative substitution. E. coli YiiP 3D structure PDB 3H90 was used to depict TMS (A). Molecular phylogenetic analysis by Maximum Likelihood method is shown. The percentage of trees in which the associated sequences clustered together is shown next to the branches (B). Venn diagram depicting the number of reference bacterial genomes having a PIB4-ATPase (yellow), a CDF poly-his (cyan), or both (green) (C).
Metal Sensitivity Determination
Metal sensitivity was assessed by inhibition halo experiments and the agar dilution method. In the first case aliquots of 5 ml molten LB-soft agar media (agar 0.6%) containing approximately 5.107 cfu/ml (OD600 nm = 0.05) were laid on LB-agar plates. After solidification four dried filter paper discs (7-mm diameter) previously embedded with 10 μl of different metal solutions (1 M, 0.5, 0.2, and 0.1 M as chloride salts except for FeSO4) were placed on top of the agar. Sensitivity was determined as zones of clearing surrounding each disc and scored after 18 h incubation at 37°C. For the agar dilution method LB liquid cultures were grown till OD600 nm of 1.0 from an overnight culture and 10 μl of serial dilution were spotted on LB-agar plates supplemented with the desired metal concentration as indicated in the figure.
Pyoverdine Detection
LB soft-agar plates with P. aeruginosa strains grown for 16 h from OD600 nm = 0.005 were exposed to white light (300–700 nm) and UV light (<300 nm; UVP, CA). Images were obtained with a digital camera and the settings controlled by an image acquisition software (Doc-It, UVP). ROIs were selected and analyzed with ImageJ. ROIs were defined as the surrounding area of the Zn-loaded filter paper disc where a fluorescent signal is detected and there is no inhibition of growth. Optical density signals were obtained from mean gray value intensities of the fluorescent signal area, normalized by those obtained from white light (300–700 nm) exposed plates. The value from WT strain was subtracted in all cases. Strains with the pvdA gene disrupted showed no fluorescent signal after 2 days of incubation in cetrimide-agar plates. This was interpreted as incapability to produce the siderophore pyoverdine in the strains.
Metal Accumulation
Fifty milliliters of liquid LB cultures in late exponential phase were supplemented with 0.1 mM CoCl2, ZnCl2 or FeSO4 and incubated for 2 h. End point inhibitory growth curves showed these concentrations to be in the sub-lethal range. After this incubation, OD600 nm was determined, cells harvested, and washed with 0.9% NaCl. Pellets were digested with 0.5 ml of HNO3 (trace metal grade) for 1 h at 80°C and then overnight at 20°C. Digestions were stopped by the addition of 0.1 ml of 30% H2O2 and dilution to 10 ml with water. Metal contents in samples were measured by ICP–MS.
Membrane Permeability Assays
The LB liquid cultures were inoculated at OD600 nm of 0.1 from overnight cultures. When an OD600 nm value of 0.6 was reached, cultures were washed and resuspended in 5 mM HEPES-Na, pH 7.2. Three fractions were used for different treatments. One was supplemented with 1 mg/ml lysozyme, another was supplemented with 1 mg/ml lysozyme plus 1 mM EDTA and the rest was left unamended. Cells were counted at 0, 5, 10, and 15 min after addition of the reagents. Survival rate was estimated as the ratio of number of cells in the presence and the absence of any treatment.
Antibiotic Susceptibility Determination
Antibiotic susceptibility was assessed by the agar dilution method in Müller–Hinton medium. For imipenem minimal inhibitory concentration (MIC) was corroborated by the Epsilon test (Biomerieux Diagnostics) in Müller–Hinton medium and according to manufacturer instructions.
Outer Membrane Proteins Purification and MS-MS Analysis
Cells were harvested from 200 ml LB cultures during the late exponential growth phase via centrifugation (6,000 × g, 10 min, 4°C) and washed twice with 10 ml of 10 mM Tris-HCl (pH 7.5). The cell suspensions were then sonicated using 2 cycles of 20 s bursts at 80–120 W on ice. Any intact cells were removed via 10 min of centrifugation at 6,000 × g at 4°C. The supernatants were centrifuged for 30 min at 100,000 × g at 4°C. After washing the pellets with 10 mM Tris-Cl pH 7.5, they were resuspended in 30 ml of 10 mM Tris-Cl pH 7.5, Triton X-100 was added to a final concentration of 2% and incubated for 10 min at room temperature. The Triton X-100- insoluble fraction was then separated via high-speed centrifugation (100,000 × g, 1 h). The pellets were washed in 30 ml 10 mM Tris-Cl pH 7.5 once and finally resuspended in 200 μl 10 mM Tris-Cl pH 7.5. Protein was measured in accordance to Bradford (1976) and resolved in SDS-PAGE 8%. Gels were stained with Coomassie Brillant Blue and bands analyzed visually. A 50 kDa band showing higher intensity in the mutant strains was excised for tryptic digestion and MS/MS identification. MS/MS determinations were performed at the Institut Pasteur–Analytical Biochemistry and Proteomics Unit- Montevideo, Uruguay.
Hydrogen Peroxide Sensitivity Test
The LB liquid cultures were inoculated at OD600 nm of 0.1 from overnight cultures. When an OD600 nm value of 0.6 was reached, cultures were split, a half was supplemented with 30 mM H2O2 and the other left unamended. Cells were counted at 0, 15, 30, and 45 min after adding H2O2. Survival rate was estimated as the ratio of number of cells in the presence and the absence H2O2.
Infectivity Assay
Arabidopsis thaliana ecotype Columbia was infected with P. aeruginosa as described by Baldini et al. (2014). Briefly, 3-week-old plants were inoculated by leaf infiltration with approximately 2 × 106 colony-forming units (cfu) per milliliter (OD600 nm 0.002). Two leaf discs were taken from each infected plant at 0, 3, and 4 days post infection (d.p.i.) and homogenized in 10 mM MgSO4. Serial dilutions of this homogenate were plated in selected media and cfu were counted after 16 h growth at 37°C. Four plants were infected per each bacterial strain.
Results
Bioinformatics Analyses
The transport mechanism of CDF transporters requires TM binding at the intracellular exposed site A for subsequent export. Considering that optimal coordination at the transport site depends on lateral amino acids side chain the specificity for metal transport can be predicted a priori in silico. P. aeruginosa PAO1 CDF transporter sequences were analyzed by multiple sequence alignment including the archetypical Zn/Fe/Cu CDF transporters, YiiP from E. coli and CzcD from Bacillus subtilis (Grass et al., 2005; Moore et al., 2005). The alignment indicates conservative substitutions at the transport metal binding site A in the three P. aeruginosa paralogous genes with lateral amino acids chains having O (Asp and Glu) or N (His) ligands (Figure 1A, blue dots). PA3963/YiiP has the highest similarity with the archetypical member E. coli YiiP showing strict identity for residues forming sites A, B, and C (Figure 1A, blue, green, and cyan dots). Residues in sites A, B, and C of PA0397/CzcD are similar to residues found in the previously characterized B. subtilis CDF member CzcD with a conservative substitution at TMS 5 (His153-Glu153). In B. subtilis, CzcD confers resistance to Zn2+, Co2+, Ni2+, and Cu+/2+ (Moore et al., 2005). Another characterized ortholog, zitA, was included in the analyses as there is evidence pointing to a role for this gene in Zn2+ homeostasis in Mycobacterium smegmatis (Grover and Sharma, 2006; Raimunda et al., 2012). P. aeruginosa CzcD shares strict identity at the metal transport site with zitA (Figure 1A) and these clustered together (Figure 1B) indicating a likely participation in Zn2+ transport. The presence of a cytosolic poly-histidine stretch between TMS 4 and TMS 5 in PA1297/AitP suggests that this CDF is involved in Co2+ fluxes as similar low complexity histidine-rich sequences are found in Co2+ transporting members (Montanini et al., 2007; Podar et al., 2012). Interestingly, P. aeruginosa PAO1 genome lacks a PIB4-ATPase. Members of this sub-family have been characterized to participate in Co2+/Fe2+ transport in bacterial pathogens (Patel et al., 2016). This led us to inquire whether the presence of a CDF showing a poly-his stretch between TMS 4 and TMS 5 conditions negatively the presence of a PIB4-ATPase in other bacteria. The analysis of 4,430 bacterial reference genomes indicated that 553 contained at least one of the two proteins (Supplementary Table 1), i.e., a CDF with a poly-his stretch or an Fe2+/Co2+-ATPase and that a low percentage (6.3%) of these genomes contained both a CDF with a poly-his stretch and an Fe2+/Co2+-ATPase (Figure 1C). Taken together, these analyses suggested that AitP could play a role in Fe2+ export.
Metal Sensitivity Screening of P. aeruginosa CDFs Mutants
We initially screened the TM sensitivity of the loss-of-function mutants for CzcD, AitP and YiiP vs. WT, by inhibition halo experiments. An increased Co2+ sensitivity was observed for the strain aitP::dTn5 (Figure 2). A subtle Fe2+ sensitivity was detected in this strain also. Unexpectedly, the strains czcD::dTn5 and yiiP::dTn5 showed Zn2+ resistance while no changes were detected with other metals. Ni2+ sensitivity was apparent for strains czcD::dTn5 and aitP::dTn5 only at higher metal concentrations. This and the fact that total Ni2+ concentrations in biological systems are much lower than Fe2+ and Zn2+ precluded us to test further the participation of CzcD and AitP in Ni2+ homeostasis.
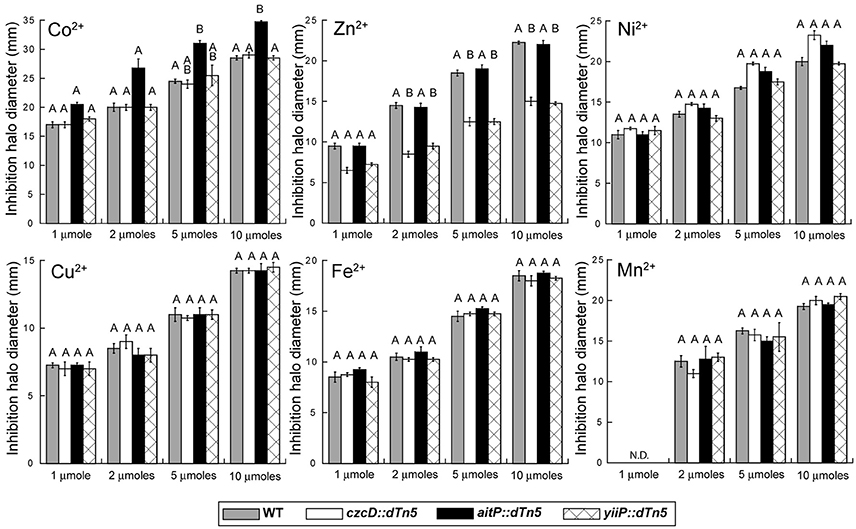
Figure 2. Screening of P. aeruginosa CDF transporters roles in transition metal homeostasis and transport. Metal toxicity was evaluated by inhibition halo experiments in strains PAO1 (gray bars), czcD::dTn5 (empty bars), aitP::dTn5 (black bars) and yiiP::dTn5 (cross-hatched bar). 7-mm filter paper discs loaded with several amounts (x-axis) of metal (upper left in panels) were dried and then laid on top of a soft-agar inoculated with each strain (OD600 nm = 0.05). Plates were incubated 16 h at 37°C and inhibition halo diameters measured. N.D. = not detected. Bars indicate mean ± SE of three independent experiments. Different letters designate significantly different means as informed by a Bonferroni post hoc (p < 0.05) test of an ANOVA.
AitP is Involved in Fe2+ and Co2+ Homeostasis
Inhibition halo experiments in soft LB-agar showed that the insertional mutant of aitP was sensitive to Co2+ and probably to Fe2+ compared to the WT strain. These phenotypes were confirmed by the dilution agar method (Figure 3A) and reverted in the complemented strain C-aitP discarding polar effects. Growth inhibition could be the consequence of intracellular Fe2+ and Co2+ accumulation due to the lack of transport in this loss-of-function mutant. To test this intracellular Fe2+ and Co2+ content was measured in cells incubated at the exponential phase in presence of a sub-lethal concentration of 100 μM Fe2+ or Co2+ for 2 h. Compared to WT, the strain aitP::dTn5 showed a 60–70% increase in Fe2+ and Co2+ accumulation (Figure 3B). This suggests a direct participation of AitP in these ions homeostasis as an Fe2+/Co2+ exporter.
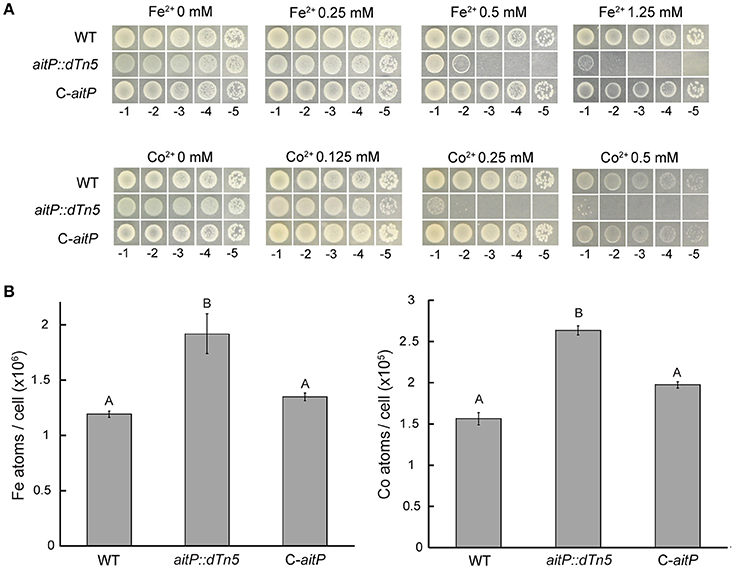
Figure 3. AitP is involved in Fe2+ and Co2+ homeostasis. Serial dilutions (10 μl) of P. aeruginosa PAO1 (WT), aitP::dTn5 and complemented C-aitP cells initially at OD600 nm = 1.0 were spotted on LB-agar supplemented with the indicated Fe2+ (as ) or Co2+ (as Cl−) concentration and incubated for 16 h at 37°C (A). Iron and cobalt content was measured in WT, aitP::dTn5 and C-aitP after incubation with 0.1 mM FeSO4 or CoCl2 for 2 h in LB medium during early exponential phase growth. Metal contents are shown as atoms/cell (B). Data are the mean ± SE of three independent experiments. Different letters designate significantly different means as informed by a Bonferroni post hoc (p < 0.05) test of an ANOVA.
CzcD and YiiP Export Zn2+
Inhibition halo experiments showed that czcD::dTn5 and yiiP::dTn5 are more resistant to Zn2+ (Figures 2, 4A). When the Zn2+ disc-containing plates were observed under UV light a fluorescent halo was detected surrounding the inhibition zone (Figure 4A). This halo had higher fluorescent intensities in the cases of these strains (Figure 4A). Although there was a variation in the fluorescence signal of the strains tested with Ni2+, Cu2+, Fe2+, and Mn2+ (not shown), only Zn2+ produced a consistently defined fluorescent halo throughout the experiments. It is well known that P. aeruginosa secretes the green fluorescent molecule, pyoverdine, in order to acquire Fe2+ (Vasil and Ochsner, 1999). Intracellular iron levels were similar to the WT in both strains grown in presence of FeSO4 (not shown), discarding a putative role in Fe2+ import. However, it has previously been described that the presence of elevated Zn2+ in the media induces pyoverdine production in P. aeruginosa (Hofte et al., 1993; Rossbach et al., 2000). Mutation of pvdA in the background mutants suppresses the Zn2+-dependent response supporting that pyoverdine and no other fluorophore yields the fluorescent halo (Supplementary Figure 1). Considering this and the fact that pyoverdine is able to chelate divalent TM other than Fe2+ with high affinities (Schalk and Guillon, 2013), the Zn2+ resistant phenotypes of czcD::dTn5 and yiiP::dTn5 may be explained by the overproduction of the chelator in response to a transient cytosolic Zn2+ increase. Although we observed a subtle increased in Zn2+ sensitivity when czcD or yiiP where disrupted in a non-pyoverdine producer strain, the existence of the gene zntA codiyng for a Zn2+-ATPase in P. aeruginosa genome precluded us to asses phenotypically a direct role in Zn2+ transport of CzcD and YiiP. Thus, we created the triple mutants lacking ZntA, PvdA and each of the CDF, CzcD, or YiiP (Table 1, TM111 and TM113), and compared the Zn2+ sensitivity of these vs. the double mutant lacking ZntA and PvdA (Table 1, DM110). We observed an increased sensitivity in both cases (Figure 4B) which correlated with an increased intracellular accumulation of Zn2+ (Figure 4C).
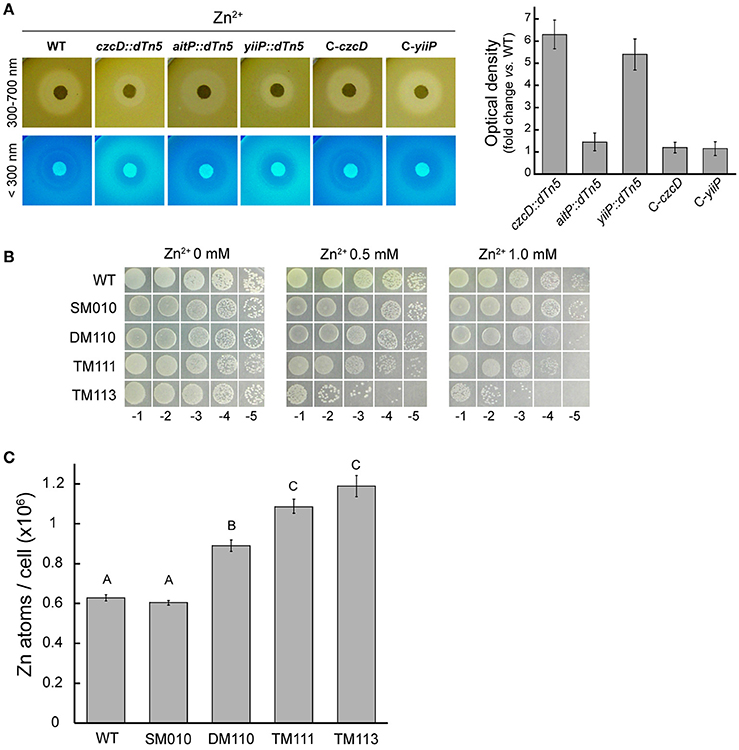
Figure 4. CzcD and YiiP play a role in Zn2+ transport. Differential Zn2+-dependent pyoverdine production was estimated by relating optical densities of images acquired using UV light (<300 nm) to those acquired using white light (300–700 nm) as described in detail in the Materials and Methods section. Strains were grown for 16 h at 37°C in soft-agar plates containing Zn2+-loaded filter discs (5 μmoles). Bars indicate fold increase in fluorescence vs. WT. Note the increased resistance to Zn2+ and the increased fluorescent signal in strains czcD::dTn5 and yiiP::dTn5 vs. WT (A). Serial dilutions (10 μl) of P. aeruginosa strains initially at OD600 nm = 1.0 were spotted on LB-agar supplemented with the indicated Zn2+ concentration (as Cl−) and incubated for 16 h at 37°C (B). Zinc content was determined in P. aeruginosa strains after incubation with 0.1 mM ZnCl2 in LB medium for 2 h during early exponential phase growth. Metal contents are shown as atoms/cell (C). WT (PAO1), SM010 (pvdA mutant), DM110 (zntA and pvdA mutant), TM111 (zntA, pvdA, and czcD mutant) and TM113 (zntA, pvdA, and yiiP mutant). Data are the mean ± SE of three independent experiments. Different letters designate significantly different means as informed by a Bonferroni post hoc (p < 0.05) test of an ANOVA.
CzcD and YiiP Confers Resistance to a Wide Range of Antibiotics
The increased sensitivity to Zn2+ observed in the triple mutant strains suggests that the roles of both CDF in this bacterium are linked to Zn2+ efflux. However, the presence of a Zn2+ transporting PIB-ATPase in the P. aeruginosa genome argues against the participation of CzcD and YiiP in Zn2+ tolerance. We noticed that of the three Tcr transposon mutant strains, yiiP::dTn5 and czcD::dTn5 had a MIC tetracycline concentration decreased by half. Evaluation of MICs for several antibiotics showed a 4–8-fold increased sensitivity to imipenem and ciprofloxacin and a 2-fold increased sensitivity to chloramphenicol and gentamicin for yiiP::dTn5 and czcD::dTn5 (Table 2). Interestingly, the MIC-values remained unchanged after pvdA was disrupted in these mutants discarding a possible participation of pyoverdine in the mechanism leading to increased antibiotic sensitivity.
Outer Membrane Stability Depends on CzcD and YiiP
Hydrophobic and hydrophilic antibiotics can permeate the outer membrane via lipid-mediated pathway or via diffusion porins, respectively (Delcour, 2009). Thus, the observed sensitivity toward several hydrophobic antibiotics in czcD::dTn5 and yiiP::dTn5 might reflect alterations in the outer membrane as this is constituted mostly by lipopolysaccharides (LPS). To test this we analyzed the survival rate of grown cells incubated in presence of lysozyme alone or lysozyme plus EDTA in a low osmolarity buffer. This divalent cation chelator is hypothesized to alter the LPS distribution in the outer membrane allowing lysozyme to disrupt the cell wall with consequent cell lysis. A two-way ANOVA analysis indicated that in all cases the time variable may affect cell survival (two-way ANOVA, p < 0.05). Furthermore, the same analysis showed that the treatment variable significantly changed the cell growth rates of czcD::dTn5, yiiP::dTn5 (two-way ANOVA, p < 0.0001) and C-yiiP strains (two-way ANOVA, p < 0.002). Multiple comparisons at each time points by corrected post hoc test showed that significant differences in the survival rate of these strains were only attained when cells were incubated with lysozyme plus EDTA (Figure 5). The same analysis pointed to no significant differences between samples of the same treatment for WT, aitP::dTn5 and C-czcD. The complemented strains C-czcD and C-yiiP become resistant similar to the control treatment levels except in the C-yiiP strain where a partial restoration of the phenotype was observed (Figures 5E,F). These results indicate that the outer membrane permeability toward hydrophobic compounds and large macromolecules might be affected in czcD::dTn5 and yiiP::dTn5.
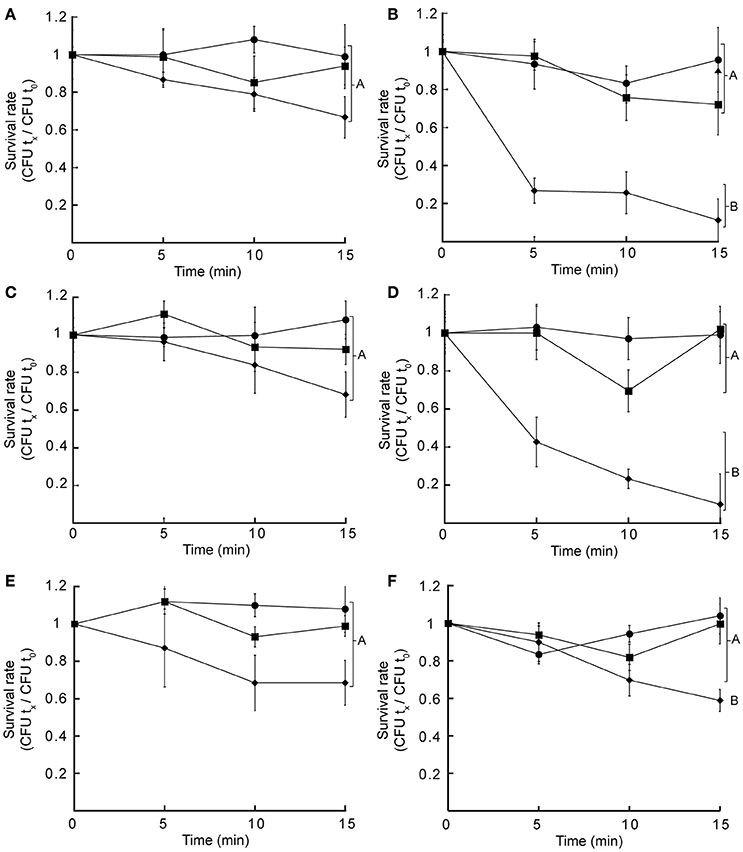
Figure 5. Insertional mutant strains of CzcD and YiiP show altered permeability of the outer membrane. Wild type (A), czcD::dTn5 (B), aitP::dTn5 (C), yiiP::dTn5 (D), C-czcD (E), or C-yiiP (F) at mid-exponential phase were incubated in a low osmolarity buffer alone (•), supplemented with 1 mg/ml lysozyme (■) or with 1 mg/ml lysozyme plus 1 mM EDTA (♦). After the indicated time cells were estimated and normalized to cell number at time = 0 (CFU tx/CFU t0). Data are the mean ± SE of three independent experiments. Different letters designate significantly different means as informed by a Bonferroni post hoc (p < 0.05) test of a two-way ANOVA.
CzcD or YiiP Mutation Results in Altered OprN and OprD Expression
The increased sensitivity toward more hydrophobic antibiotics such as chloramphenicol, gentamicin and ciprofloxacin can be explained considering that the outer membrane permeability is compromised in the CzcD and YiiP mutant stains (Figure 5). However, small hydrophilic compounds, like imipenem, require outer membrane porins for cell entry (Eren et al., 2013). In P. aeruginosa, the OprD porin enables the entry of positively charged aminoacids and imipenem (Trias and Nikaido, 1990). OprD expression is negatively regulated in response to incremental Zn2+ levels in the growth media through the two component system CzcRS (Perron et al., 2004; Dieppois et al., 2012). Also the global regulator factor MvaT negatively affects oprD expression (Westfall et al., 2006; Lister et al., 2009). In order to evaluate changes in outer membrane protein expression leading to increased antibiotic sensitivity, we analyzed the protein profile of this fraction in all strains by SDS-PAGE. A 50–55 KDa band was differentially expressed in both czcD::dTn5 and yiiP::dTn5 (Figure 6A). The band was analyzed by MS/MS and the peptides identified matched with OprN and OprD (Figure 6B). These proteins could not be detected in aitP::dTn5 and WT samples. In P. aeruginosa, together with MexE and MexF, OprN forms an RND (resistance-nodulation-division) efflux pump characterized to participate in ciprofloxacin and the Pseudomonas quinolone signal (PQS, 2-heptyl-3-hydroxy-4-quinolone) efflux. The increased expression of OprD correlates with the imipenem sensitivity shown by czcD::dTn5 and yiiP::dTn5. Although the OprN overexpression does not correlate with the ciprofloxacin sensitivity observed in these strains (Table 2), it may be a consequence of outer membrane permeability changes leading to intracellular accumulation of hydrophobic PQS molecules, the natural substrate of the MexEF-OprN RND efflux system.
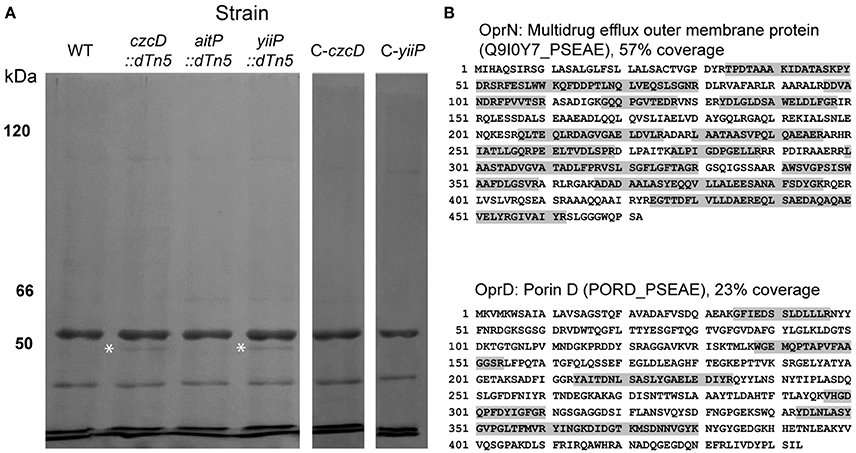
Figure 6. Insertional mutation of CzcD and YiiP induces overexpression of outer membrane proteins OprN and OprD. SDS-PAGE analysis of outer membrane protein fractions from P. aeruginosa strains. Proteins were resolved in an 8% gel and stained with Coomassie Brillant Blue. White asterisks indicate the position of the bands observed only in fractions of czcD::dTn5 and yiiP::dTn5 (A). The bands were excised and identified by MS/MS. Detected OprN and OprD peptides (gray highlighted letters) and maximal sequence coverage are shown (B).
P. aeruginosa CDFs Participates in Virulence
It was previously shown that members of the CDF family responsible for Mn2+ homeostasis play a crucial role in bacterial virulence (Rosch et al., 2009; Raimunda and Elso-Berberian, 2014). To evaluate possible roles in virulence of CzcD, AitP, and YiiP a simple assay that takes advantage of P. aeruginosa capability to infect plants (Baldini et al., 2014) was used. Figure 7A shows that the three P. aeruginosa CDFs were required for host infection. As oxidative burst with generation of H2O2 and free radicals is part of the defense mechanism in plants (Wojtaszek, 1997) we tested in vitro the resistant to H2O2. Only aitP::dTn5 showed decreased survival in presence of the stressor (Figure 7B). This indicates that their roles are achieved by different mechanisms probably related to the transport specificity. Complementation with the wild-type genes conferred the strains normal virulent phenotypes and normal levels of resistance to H2O2.
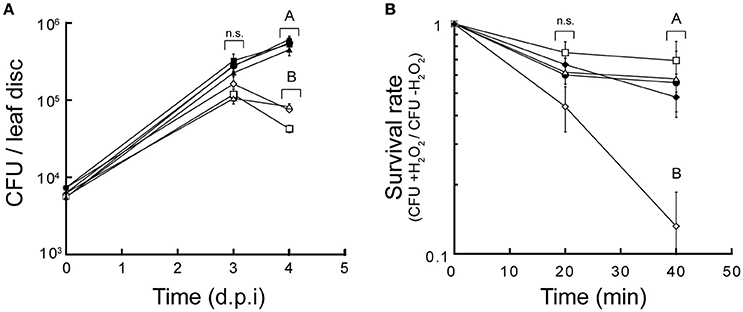
Figure 7. P. aeruginosa CDF transporters are required for virulence playing different roles. Growth rate of P. aeruginosa PAO1 (•), czcD::dTn5 (□), aitP::dTn5 (♢), and yiiP::dTn5 (Δ) in A. thaliana leaves. Complemented strains of the CDF mutants are shown (C-czcD, ■; C-aitP, ♦; C-yiiP, ▲) (A). Cell survival of P. aeruginosa PAO1 (•), czcD::dTn5 (□), aitP::dTn5 (♢), yiiP::dTn5 (Δ) and aitP complemented (C-aitP, ♦) after treatment with 30 mM H2O2 in LB medium for various lengths of time. Cell viability is shown as the ratio of CFU of treated/untreated cultures (B). Data are the mean ± SE of three independent experiments. Different letters designate significantly different means as informed by a Bonferroni post hoc (p < 0.05) test of an ANOVA. n.s, not statistically significant.
Discussion
Previous studies have shown that bacterial CDF transporters are involved in TM tolerance establishing their participation in TM export to avoid cellular toxicity through intracellular metal accumulation (Grass et al., 2005; Grover and Sharma, 2006; Rosch et al., 2009). Although their roles during bacterial infection was also described (Rosch et al., 2009), in P. aeruginosa these were not identify as relevant factors for wound infection or for intrinsic antibiotic resistance (Turner et al., 2014; Murray et al., 2015). However, a study of the genes required to colonize the murine gastrointestinal tract showed that a CzcD homolog plays an important role in P. aeruginosa PA14 during this process (Skurnik et al., 2013). Independent of the methodological aspects leading to these differences, our results show that the three members of CDF family in P. aeruginosa PAO1 are required for proper infection fitness in the A. thaliana infection model. This has led us to evaluate a possible correlation between CDF specificity of transport and virulence. Another aim of this work was to test a possible functional redundancy among them or members of different TM transport protein families with similar specificity of transport, i.e., PIB-ATPases.
Protein sequence alignments of the P. aeruginosa CDF with previous archetypical characterized members, B. subtilis CzcD and E. coli YiiP, showed a high degree of conservation in amino acids at positions of the metal transport site. Positions of the cytosolic regulatory site C also showed a high degree of conservation except for the member AitP. A histidine-rich domain located between TMS 4 and TMS 5 in this member suggested a role in Co2+ transport (Montanini et al., 2007; Podar et al., 2012). As expected, the mutant strain lacking AitP showed increased sensitivity to Co2+ and Ni2+, although the last was observed only at higher Ni2+ concentrations. Supporting a role in Co2+ homeostasis this mutant accumulated more Co2+ vs. WT. Analysis of the P. aeruginosa genome indicates a lack of PIB4-ATPase. This sub-group of transporters has been characterized as Fe2+/Co2+-transporting ATPases (Guan et al., 2015; Patel et al., 2016) and to be required for virulence in pathogenic bacteria (Raimunda et al., 2014). The mutant lacking AitP showed increased sensitivity to Fe2+ and accumulated higher intracellular levels of this ion vs. WT. The nutritional immunity paradigm points to a lessen bioavailability of Fe2+ in the bacterial environment during infection (Hood and Skaar, 2012). A role for an Fe2+-transporting CDF emerges when considering Fe-S cluster Fe2+ released induced by the H2O2 generation in the activated macrophage with subsequent intracellular reactive oxygen radicals formation through Fenton reaction (Imlay, 2008). A sequence analysis of organisms having a PIB4-ATPases or a CDF poly-his stretches between TMS 4 and TMS 5 in their genome resulted in only 6% of the total organisms having both proteins (35/553). The fact that 78% of the reference genomes analyzed (553/4430) lacking a PIB4-ATPase and a CDF with a poly-his stretch raises the question on whether other transporters from these or other families participates in the Fe2+ efflux process.
Based on sequence similarity, the alignment shows a closer phylogenetic relationship between B. subtilis CzcD and P. aeruginosa CzcD and between E. coli YiiP and P. aeruginosa YiiP. We also included the only Zn2+-transporting CDF member present in M. smegmatis ZitA (Grover and Sharma, 2006; Raimunda et al., 2012). The same alignment shows that ZitA is phylogenetically related to CzcD. These results suggested that CzcD and YiiP are Zn2+ transporters. We demonstrated that CzcD and YiiP export intracellular Zn2+. The triple mutant strains lacking pyoverdine, the putative Zn2+-ATPase, ZntA, and CzcD or YiiP showed increased Zn2+ sensitivity and accumulated the ion at higher levels vs. the double mutant strains lacking pyoverdine and ZntA but expressing CzcD or YiiP. Considering the higher apparent Zn2+ accumulation of the triple mutants, vs. the double mutant, CzcD and YiiP would have access to different intracellular Zn2+ pools other than ZntA. The Zn2+-resistant phenotypes of the czcD::dTn5 and yiiP::dTn5 were unexpected and a parsimonious model explaining this would imply a Zn2+ importer function for both CDF members. However, the concomitant pyoverdine overproduction seen in these mutants in the presence of Zn2+ leads us to hypothesize a role in the efflux of this metal. It is known that pyoverdine synthesis genes are up-regulated in the presence of Zn2+ (Rossbach et al., 2000). Independent of the mechanism leading to pyoverdine overproduction, we hypothesize that Zn2+ toxicity is decreased in both mutants due to a lesser bioavailability of the metal in the media from the pyoverdine-Zn complex formation. Several conditions are required for this scenario to be possible. A transient rise in the cytosolic Zn2+ concentration would be necessary to induce pyoverdine synthesis in these strains. Moreover, although normal intracellular Zn2+ pools could be restored eventually, the binding of pyoverdine-Zn complexes to FpvA could amplify the signal in a positive feedback for pyoverdine synthesis (Braud et al., 2009).
Considering the Zn2+ transport specificity of CzcD and YiiP and the phenotypes observed in czcD::dTn5 and yiiP::dTn5 indicating antibiotic susceptibility, outer membrane permeability changes and overexpression of outer membrane proteins, two likely scenarios are hypothesized. First, CzcD and YiiP may participate in Zn2+ efflux to fine tune the TM intracellular homeostasis. Changes in Zn2+ levels could trigger pleiotropic effects, leading to outer membrane defects and instability. Alternatively, Zn2+ transport could be coupled to periplasmic Zn2+ sensing via two component systems. This supposes a protein-protein interaction and a Zn2+ transfer reaction from the CDF to the sensing component at the periplasmic side of the inner membrane. In P. aeruginosa the two component systems CzcRS and ColRS have been described as participating in Zn2+ sensing leading to Zn2+ and antibiotic resistance and LPS modifications, respectively (Perron et al., 2004; Nowicki et al., 2015). Transcription of oprD is regulated in the periplasm via CzcRS. When Zn2+ efflux is downregulated, as in the czcD::dTn5 and yiiP::dTn5, an increase in oprD transcription is expected. A direct positive effect on oprD expression by extracellular pyoverdine-Zn2+ formation is discarded as the non-pyoverdine producers showed the same sensitivity toward imipenem and the others antibiotics (Table 2). The Zn2+ content in our media (5 μM by ICP-MS) is sufficient to suppress orpD expression in the WT (Perron et al., 2004). Although purely speculative, this would not be the case in the czcD::dTn5 and yiiP::dTn5 strains, where OprD is overexpressed relative to the WT and aitP::dTn5 strains.
How can the twin phenotypes shown by the single mutant strains czcD::dTn5 and yiiP::dTn5 be explained? Since the CDF functional unit is a dimer, we hypothesize that CzcD and YiiP form a functional heterodimer. Residues at positions forming the charge interlock at the cytosol-membrane interface in E. coli YiiP, i.e., Lys77 and Asp207, are well conserved in CzcD and YiiP and thus the heterodimer formation is plausible (Kolaj-Robin et al., 2015). Recent studies proposed that in the magnetotactic bacteria Magnetospirillum gryphiswaldense two CDF genes, mamB and mamM, interact to form a heterodimer functional unit (Uebe et al., 2011).
In conclusion, this study provides evidence for new roles of CDF transporters required during bacterial infection. More specifically, our results show that Zn2+ efflux mediated by YiiP and CzcD is required in P. aeruginosa for proper outer membrane permeability. A third CDF member, AitP, is required for Fe2+/Co2+ homeostasis.
Author Contributions
Conceived and designed the experiments: DR; Performed the experiments: AS, DR; Analyzed the data: AS, DR; Contributed reagents/samples/analysis/tools: DR. Wrote or edited the manuscript: AS, DR; All authors read and approved the final manuscript.
Funding
This work was supported by the Agencia Nacional de Promoción Científica y Tecnológica (PICT2013–2258 and PICT2015–2897 to DR). DR is a Consejo Nacional de Investigaciones Científicas y Tecnológicas (CONICET) investigator.
Conflict of Interest Statement
The authors declare that the research was conducted in the absence of any commercial or financial relationships that could be construed as a potential conflict of interest.
The reviewer MLV and handling Editor declared their shared affiliation and the handling Editor states that the process nevertheless met the standards of a fair and objective review.
Acknowledgments
We thank Dra. Graciela Elso-Berberián for helpful discussions and critical reading of early versions of the manuscript, to Dr. Claudio Valverde (Universidad Nacional de Quilmes, Argentina) for providing us with a bacterial strain for transconjugation and Myriam Siravegna for technical support.
Supplementary Material
The Supplementary Material for this article can be found online at: https://www.frontiersin.org/article/10.3389/fcimb.2017.00084/full#supplementary-material
Abbreviations
TM, transition metal; CDF, cation diffusion facilitator; TMS, transmembrane segment; CTD, C-terminal domain; ICP-MS, inductively coupled plasma mass spectrometry.
References
Argüello, J. M., Raimunda, D., and Padilla-Benavides, T. (2013). Mechanisms of copper homeostasis in bacteria. Front. Cell. Infect. Microbiol. 3:73. doi: 10.3389/fcimb.2013.00073
Baldini, R. L., Starkey, M., and Rahme, L. G. (2014). Assessing Pseudomonas virulence with the nonmammalian host model: Arabidopsis thaliana. Methods Mol. Biol. 1149, 689–697. doi: 10.1007/978-1-4939-0473-0_53
Bradford, M. M. (1976). A rapid and sensitive method for the quantitation of microgram quantities of protein utilizing the principle of protein-dye binding. Anal. Biochem. 72, 248–254. doi: 10.1016/0003-2697(76)90527-3
Braud, A., Hoegy, F., Jezequel, K., Lebeau, T., and Schalk, I. J. (2009). New insights into the metal specificity of the Pseudomonas aeruginosa pyoverdine-iron uptake pathway. Environ. Microbiol. 11, 1079–1091. doi: 10.1111/j.1462-2920.2008.01838.x
Choi, K. H., and Schweizer, H. P. (2006). mini-Tn7 insertion in bacteria with single attTn7 sites: example Pseudomonas aeruginosa. Nat. Protoc. 1, 153–161. doi: 10.1038/nprot.2006.24
Cubillas, C., Vinuesa, P., Tabche, M. L., and Garcia-de los Santos, A. (2013). Phylogenomic analysis of cation diffusion facilitator proteins uncovers Ni2+/Co2+ transporters. Metallomics 5, 1634–1643. doi: 10.1039/c3mt00204g
Delcour, A. H. (2009). Outer membrane permeability and antibiotic resistance. Biochim. Biophys. Acta 1794, 808–816. doi: 10.1016/j.bbapap.2008.11.005
Dieppois, G., Ducret, V., Caille, O., and Perron, K. (2012). The transcriptional regulator CzcR modulates antibiotic resistance and quorum sensing in i. PLoS ONE 7:e38148. doi: 10.1371/journal.pone.0038148
Edgar, R. C. (2004). MUSCLE: multiple sequence alignment with high accuracy and high throughput. Nucleic Acids Res. 32, 1792–1797. doi: 10.1093/nar/gkh340
Eren, E., Parkin, J., Adelanwa, A., Cheneke, B., Movileanu, L., Khalid, S., et al. (2013). Toward understanding the outer membrane uptake of small molecules by Pseudomonas aeruginosa. J. Biol. Chem. 288, 12042–12053. doi: 10.1074/jbc.M113.463570
Fraústo Da Silva, J. J. R., and Williams, R. J. P. (2001). The Biological Chemistry of the Elements: The Inorganic Chemistry of Life. New York, NY: Oxford University Press.
Gouet, P., Courcelle, E., Stuart, D. I., and Metoz, F. (1999). ESPript: analysis of multiple sequence alignments in PostScript. Bioinformatics 15, 305–308. doi: 10.1093/bioinformatics/15.4.305
Grass, G., Otto, M., Fricke, B., Haney, C. J., Rensing, C., Nies, D. H., et al. (2005). FieF (YiiP) from Escherichia coli mediates decreased cellular accumulation of iron and relieves iron stress. Arch. Microbiol. 183, 9–18. doi: 10.1007/s00203-004-0739-4
Grover, A., and Sharma, R. (2006). Identification and characterization of a major Zn(II) resistance determinant of Mycobacterium smegmatis. J. Bacteriol. 188, 7026–7032. doi: 10.1128/JB.00643-06
Guan, G., Pinochet-Barros, A., Gaballa, A., Patel, S. J., Argüello, J. M., and Helmann, J. D. (2015). PfeT, a PIB4 -type ATPase, effluxes ferrous iron and protects Bacillus subtilis against iron intoxication. Mol. Microbiol. 98, 787–803. doi: 10.1111/mmi.13158
Guffanti, A. A., Wei, Y., Rood, S. V., and Krulwich, T. A. (2002). An antiport mechanism for a member of the cation diffusion facilitator family: divalent cations efflux in exchange for K+ and H+. Mol. Microbiol. 45, 145–153. doi: 10.1046/j.1365-2958.2002.02998.x
Gupta, S., Chai, J., Cheng, J., D'Mello, R., Chance, M. R., and Fu, D. (2014). Visualizing the kinetic power stroke that drives proton-coupled zinc(II) transport. Nature 512, 101–104. doi: 10.1038/nature13382
Hofte, M., Buysens, S., Koedam, N., and Cornelis, P. (1993). Zinc affects siderophore-mediated high affinity iron uptake systems in the rhizosphere Pseudomonas aeruginosa 7NSK2. Biometals 6, 85–91. doi: 10.1007/BF00140108
Hood, M. I., and Skaar, E. P. (2012). Nutritional immunity: transition metals at the pathogen-host interface. Nat. Rev. Microbiol. 10, 525–537. doi: 10.1038/nrmicro2836
Imlay, J. A. (2008). Cellular defenses against superoxide and hydrogen peroxide. Annu. Rev. Biochem. 77, 755–776. doi: 10.1146/annurev.biochem.77.061606.161055
Imlay, J. A. (2014). The mismetallation of enzymes during oxidative stress. J. Biol. Chem. 289, 28121–28128. doi: 10.1074/jbc.R114.588814
Jacobs, M. A., Alwood, A., Thaipisuttikul, I., Spencer, D., Haugen, E., Ernst, S., et al. (2003). Comprehensive transposon mutant library of Pseudomonas aeruginosa. Proc. Natl. Acad. Sci. U.S.A. 100, 14339–14344. doi: 10.1073/pnas.2036282100
Kehl-Fie, T. E., Zhang, Y., Moore, J. L., Farrand, A. J., Hood, M. I., Rathi, S., et al. (2013). MntABC and MntH contribute to systemic Staphylococcus aureus infection by competing with calprotectin for nutrient manganese. Infect. Immun. 81, 3395–3405. doi: 10.1128/IAI.00420-13
Kolaj-Robin, O., Russell, D., Hayes, K. A., Pembroke, J. T., and Soulimane, T. (2015). Cation diffusion facilitator family: structure and function. FEBS Lett. 589, 1283–1295. doi: 10.1016/j.febslet.2015.04.007
Lister, P. D., Wolter, D. J., and Hanson, N. D. (2009). Antibacterial-resistant Pseudomonas aeruginosa: clinical impact and complex regulation of chromosomally encoded resistance mechanisms. Clin. Microbiol. Rev. 22, 582–610. doi: 10.1128/CMR.00040-09
Llamas, M. A., Rodriguez-Herva, J. J., Hancock, R. E., Bitter, W., Tommassen, J., and Ramos, J. L. (2003). Role of Pseudomonas putida tol-oprL gene products in uptake of solutes through the cytoplasmic membrane. J. Bacteriol. 185, 4707–4716. doi: 10.1128/JB.185.16.4707-4716.2003
Lu, M., Chai, J., and Fu, D. (2009). Structural basis for autoregulation of the zinc transporter YiiP. Nat. Struct. Mol. Biol. 16, 1063–1067. doi: 10.1038/nsmb.1662
Macomber, L., and Imlay, J. A. (2009). The iron-sulfur clusters of dehydratases are primary intracellular targets of copper toxicity. Proc. Natl. Acad. Sci. U.S.A. 106, 8344–8349. doi: 10.1073/pnas.0812808106
Montanini, B., Blaudez, D., Jeandroz, S., Sanders, D., and Chalot, M. (2007). Phylogenetic and functional analysis of the Cation Diffusion Facilitator (CDF) family: improved signature and prediction of substrate specificity. BMC Genomics 8:107. doi: 10.1186/1471-2164-8-107
Moore, C. M., Gaballa, A., Hui, M., Ye, R. W., and Helmann, J. D. (2005). Genetic and physiological responses of Bacillus subtilis to metal ion stress. Mol. Microbiol. 57, 27–40. doi: 10.1111/j.1365-2958.2005.04642.x
Murray, J. L., Kwon, T., Marcotte, E. M., and Whiteley, M. (2015). Intrinsic antimicrobial resistance determinants in the superbug Pseudomonas aeruginosa. mBio 6:e01603–01615. doi: 10.1128/mBio.01603-15
Nies, D. H. (2003). Efflux-mediated heavy metal resistance in prokaryotes. FEMS Microbiol. Rev. 27, 313–339. doi: 10.1016/S0168-6445(03)00048-2
Nowicki, E. M., O'Brien, J. P., Brodbelt, J. S., and Trent, M. S. (2015). Extracellular zinc induces phosphoethanolamine addition to Pseudomonas aeruginosa lipid A via the ColRS two-component system. Mol. Microbiol. 97, 166–178. doi: 10.1111/mmi.13018
Patel, S. J., Lewis, B. E., Long, J. E., Nambi, S., Sassetti, C. M., Stemmler, T. L., et al. (2016). Fine-tuning of substrate affinity leads to alternative roles of Mycobacterium tuberculosis Fe2+-ATPases. J. Biol. Chem. 291, 11529–11539. doi: 10.1074/jbc.M116.718239
Perron, K., Caille, O., Rossier, C., Van Delden, C., Dumas, J. L., and Kohler, T. (2004). CzcR-CzcS, a two-component system involved in heavy metal and carbapenem resistance in Pseudomonas aeruginosa. J. Biol. Chem. 279, 8761–8768. doi: 10.1074/jbc.M312080200
Podar, D., Scherer, J., Noordally, Z., Herzyk, P., Nies, D., and Sanders, D. (2012). Metal selectivity determinants in a family of transition metal transporters. J. Biol. Chem. 287, 3185–3196. doi: 10.1074/jbc.M111.305649
Quadri, M., Federico, A., Zhao, T., Breedveld, G. J., Battisti, C., Delnooz, C., et al. (2012). Mutations in SLC30A10 cause parkinsonism and dystonia with hypermanganesemia, polycythemia, and chronic liver disease. Am. J. Hum. Genet. 90, 467–477. doi: 10.1016/j.ajhg.2012.01.017
Quandt, J., and Hynes, M. F. (1993). Versatile suicide vectors which allow direct selection for gene replacement in gram-negative bacteria. Gene 127, 15–21. doi: 10.1016/0378-1119(93)90611-6
Raimunda, D., and Elso-Berberian, G. (2014). Functional characterization of the CDF transporter SMc02724 (SmYiiP) in Sinorhizobium meliloti: roles in manganese homeostasis and nodulation. Biochim. Biophys. Acta 1838, 3203–3211. doi: 10.1016/j.bbamem.2014.09.005
Raimunda, D., Long, J. E., Padilla-Benavides, T., Sassetti, C. M., and Argüello, J. M. (2014). Differential roles for the Co2+/Ni2+ transporting ATPases, CtpD and CtpJ, in Mycobacterium tuberculosis virulence. Mol. Microbiol. 91, 185–197. doi: 10.1111/mmi.12454
Raimunda, D., Long, J. E., Sassetti, C. M., and Argüello, J. M. (2012). Role in metal homeostasis of CtpD, a Co2+ transporting PIB4-ATPase of Mycobacterium smegmatis. Mol. Microbiol. 84, 1139–1149. doi: 10.1111/j.1365-2958.2012.08082.x
Ranquet, C., Ollagnier-de-Choudens, S., Loiseau, L., Barras, F., and Fontecave, M. (2007). Cobalt stress in Escherichia coli. The effect on the iron-sulfur proteins. J. Biol. Chem. 282, 30442–30451. doi: 10.1074/jbc.M702519200
Ren, Q., Chen, K., and Paulsen, I. T. (2007). TransportDB: a comprehensive database resource for cytoplasmic membrane transport systems and outer membrane channels. Nucleic Acids Res. 35, D274–D279. doi: 10.1093/nar/gkl925
Rosch, J. W., Gao, G., Ridout, G., Wang, Y. D., and Tuomanen, E. I. (2009). Role of the manganese efflux system mntE for signalling and pathogenesis in Streptococcus pneumoniae. Mol. Microbiol. 72, 12–25. doi: 10.1111/j.1365-2958.2009.06638.x
Rossbach, S., Wilson, T. L., Kukuk, M. L., and Carty, H. A. (2000). Elevated zinc induces siderophore biosynthesis genes and a zntA-like gene in Pseudomonas fluorescens. FEMS Microbiol. Lett. 191, 61–70. doi: 10.1111/j.1574-6968.2000.tb09320.x
Sambrook, J., Fritsch, E. F., and Maniatis, T. (1989). Molecular Cloning: a Laboratory Manual. New York, NY: Cold Spring Harbor Laboratory Press.
Schalk, I. J., and Guillon, L. (2013). Pyoverdine biosynthesis and secretion in Pseudomonas aeruginosa: implications for metal homeostasis. Environ. Microbiol. 15, 1661–1673. doi: 10.1111/1462-2920.12013
Skurnik, D., Roux, D., Aschard, H., Cattoir, V., Yoder-Himes, D., Lory, S., et al. (2013). A comprehensive analysis of in vitro and in vivo genetic fitness of Pseudomonas aeruginosa using high-throughput sequencing of transposon libraries. PLoS Pathog. 9:e1003582. doi: 10.1371/journal.ppat.1003582
Tamura, K., Stecher, G., Peterson, D., Filipski, A., and Kumar, S. (2013). MEGA6: Molecular evolutionary genetics analysis version 6.0. Mol. Biol. Evol. 30, 2725–2729. doi: 10.1093/molbev/mst197
Trias, J., and Nikaido, H. (1990). Outer membrane protein D2 catalyzes facilitated diffusion of carbapenems and penems through the outer membrane of Pseudomonas aeruginosa. Antimicrob. Agents Chemother. 34, 52–57. doi: 10.1128/AAC.34.1.52
Turner, K. H., Everett, J., Trivedi, U., Rumbaugh, K. P., and Whiteley, M. (2014). Requirements for Pseudomonas aeruginosa acute burn and chronic surgical wound infection. PLoS Genet. 10:e1004518. doi: 10.1371/journal.pgen.1004518
Uebe, R., Junge, K., Henn, V., Poxleitner, G., Katzmann, E., Plitzko, J. M., et al. (2011). The cation diffusion facilitator proteins MamB and MamM of Magnetospirillum gryphiswaldense have distinct and complex functions, and are involved in magnetite biomineralization and magnetosome membrane assembly. Mol. Microbiol. 82, 818–835. doi: 10.1111/j.1365-2958.2011.07863.x
Vasil, M. L., and Ochsner, U. A. (1999). The response of Pseudomonas aeruginosa to iron: genetics, biochemistry and virulence. Mol. Microbiol. 34, 399–413. doi: 10.1046/j.1365-2958.1999.01586.x
Westfall, L. W., Carty, N. L., Layland, N., Kuan, P., Colmer-Hamood, J. A., and Hamood, A. N. (2006). mvaT mutation modifies the expression of the Pseudomonas aeruginosa multidrug efflux operon mexEF-oprN. FEMS Microbiol. Lett. 255, 247–254. doi: 10.1111/j.1574-6968.2005.00075.x
Keywords: cation diffusion facilitator, transition metal homeostasis, efflux, iron, zinc, virulence
Citation: Salusso A and Raimunda D (2017) Defining the Roles of the Cation Diffusion Facilitators in Fe2+/Zn2+ Homeostasis and Establishment of Their Participation in Virulence in Pseudomonas aeruginosa Front. Cell. Infect. Microbiol. 7:84. doi: 10.3389/fcimb.2017.00084
Received: 07 December 2016; Accepted: 02 March 2017;
Published: 20 March 2017.
Edited by:
Michael J. Schurr, University of Colorado Denver, USAReviewed by:
Pierre Cornelis, Vrije Universiteit Brussel, BelgiumMichael L. Vasil, University of Colorado Denver School of Medicine, USA
Michael John Franklin, Montana State University, USA
Matthew J. Wargo, University of Vermont, USA
Copyright © 2017 Salusso and Raimunda. This is an open-access article distributed under the terms of the Creative Commons Attribution License (CC BY). The use, distribution or reproduction in other forums is permitted, provided the original author(s) or licensor are credited and that the original publication in this journal is cited, in accordance with accepted academic practice. No use, distribution or reproduction is permitted which does not comply with these terms.
*Correspondence: Daniel Raimunda, draimunda@immf.uncor.edu