Poor Unstable Midgut Microbiome of Hard Ticks Contrasts With Abundant and Stable Monospecific Microbiome in Ovaries
- 1Central European Institute of Technology (CEITEC), Center for Zoonoses, University of Veterinary and Pharmaceutical Sciences, Brno, Czechia
- 2Biology Centre, Institute of Parasitology, Czech Academy of Sciences, Ceske Budejovice, Czechia
- 3Department of Entomology, Kansas State University, Manhattan, KS, United States
- 4Faculty of Science, University of South Bohemia, Ceske Budejovice, Czechia
- 5Laboratório de Imunologia Aplicada a Sanidade Animal, Centro de Biotecnologia, Universidade Federal do Rio Grande do Sul, Porto Alegre, Brazil
- 6Faculdade de Veterinária, Universidade Federal do Rio Grande do Sul, Porto Alegre, Brazil
- 7Laboratório de Bioquímica de Artrópodes Hematófagos, Instituto de Bioquímica Médica, Universidade Federal do Rio de Janeiro, Rio de Janeiro, Brazil
- 8Department of Chemistry and Biochemistry, Mendel University, Brno, Czechia
Culture-independent metagenomic methodologies have enabled detection and identification of microorganisms in various biological systems and often revealed complex and unknown microbiomes. In many organisms, the microbiome outnumbers the host cells and greatly affects the host biology and fitness. Ticks are hematophagous ectoparasites with a wide host range. They vector a number of human and animal pathogens and also directly cause major economic losses in livestock. Although several reports on a tick midgut microbiota show a diverse bacterial community, in most cases the size of the bacterial population has not been determined. In this study, the microbiome was quantified in the midgut and ovaries of the ticks Ixodes ricinus and Rhipicephalus microplus before, during, and after blood feeding. Although the size of bacterial community in the midgut fluctuated with blood feeding, it was overall extremely low in comparison to that of other hematophagous arthropods. In addition, the tick ovarian microbiome of both tick species exceeded the midgut 16S rDNA copy numbers by several orders of magnitude. This indicates that the ratio of a tick midgut/ovary microbiome represents an exception to the general biology of other metazoans. In addition to the very low abundance, the tick midgut diversity in I. ricinus was variable and that is in contrast to that found in the tick ovary. The ovary of I. ricinus had a very low bacterial diversity and a very high and stable bacterial abundance with the dominant endosymbiont, Midichloria sp. The elucidation of this aspect of tick biology highlights a unique tissue-specific microbial-invertebrate host interaction.
Introduction
Ticks are obligate blood-feeding ectoparasites of a wide range of hosts including wild and domestic animals and people. They transmit a large number of pathogens and also directly cause great economic losses in livestock (Estrada-Peña, 2015). While the majority of studies focused on detection of the pathogens ticks transmit, several studies also explored the overall microbial diversity of ticks, including that of Ixodes ricinus and Rhipicephalus microplus (Andreotti et al., 2011; Carpi et al., 2011; Greay et al., 2018). However, the identification of the microbial taxa is only the first step toward the understanding of host-microbiota interactions. Till present, the main advance has been made in the functional characterization of endosymbionts housed in the tick ovary. Maternally-inherited bacteria Coxiella sp. and Francisella sp. were shown to be essential for tick molt and/or survival (Guizzo et al., 2017; Duron et al., 2018). In contrast, little is known about the microbiota residing in the tick midgut, its role in tick physiology and vector competence for pathogens as well as its potential origins (Narasimhan et al., 2014; Narasimhan and Fikrig, 2015; Vayssier-Taussat et al., 2015; Bonnet et al., 2017). In the past decade, several metagenomic studies using next-generation sequencing (NGS) have described the tick midgut as an organ harboring a diverse bacterial community (Moreno et al., 2006; Andreotti et al., 2011; Budachetri et al., 2014; Clayton et al., 2015). However, it is important to point that NGS methods are based on an amplification of the bacterial 16S rDNA gene fragment, allowing the detection of less abundant bacteria, but also potentially introduce false-positive results due to background contamination and also do not distinguish between viable bacteria and pieces of DNA from lysed cells (Goodrich et al., 2014; Huttenhower et al., 2014; Strong et al., 2014). Moreover, it was demonstrated that the sterilization protocols of the tick surface greatly impact results of the microbial diversity (Binetruy et al., 2019).
This study aimed to assess the bacterial diversity in the midgut and ovaries of I. ricinus, and to investigate its potential source from the host blood and skin by culture-independent approaches. Moreover, the dynamic of bacterial colonization was elucidated through the absolute quantification of the microbiome in the midgut and ovaries of I. ricinus and R. microplus during the feeding course on the vertebrate host.
Materials and Methods
Tick Rearing
Wild-caught unfed I. ricinus females were collected by flagging on grass around Ceske Budejovice, Czech Republic. Ticks were maintained in the rearing facility at the Institute of Parasitology, Biology Centre Czech Academy of Sciences (CAS) under controlled conditions (temperature: 24°C and humidity: 95%). To complete the life cycle, unfed female ticks were allowed to feed on guinea pigs until the natural detachment from the host. All laboratory animals were treated in accordance with the Animal Protection Law of the Czech Republic No. 246/1992 Sb., ethics approval No. 25/2018. The study was approved by the Institute of Parasitology, Biology Centre CAS and Central Committee for Animal Welfare, Czech Republic (Protocol No. 1/2015).
Rhipicephalus microplus (Porto Alegre strain) were maintained on Hereford cattle brought from a naturally tick-free area (Santa Vitória do Palmar, Brazil; 33° 32′2″ S, 53° 20′59″ W) and kept in individual tick-proof pens on a slatted floor at the Faculdade de Veterinária of Universidade Federal do Rio Grande do Sul (Brazil) (Reck et al., 2009). The colony is maintained without the introduction of field ticks. Calves were infested with 15-day-old tick larvae. Partially engorged and fully engorged females were collected directly from the host and after natural detachment, respectively. All animal care and experimental protocols were conducted following the guidelines of the institutional care and use committee (Ethics Committee on Animal Experimentation) and were approved under the registry 14403/protocol 07.
Vertebrate Host and Tick Samples
Ixodes ricinus females were collected at several time points during a blood-feeding course on a guinea pig host. Unfed ticks, ticks on day 1, 3, and 5 during blood feeding, fully fed ticks, and ticks on day 2 and 6 after natural detachment were dissected to remove the midgut. Ovaries were dissected out from fully fed ticks and from ticks on day 2 and 6 after detachment. Similarly, the midgut and ovaries from partially engorged (mean weight 75.0 mg/tick) and fully engorged females were dissected from R. microplus. Before dissection, ticks were surface sterilized with 70% ethanol for 1 min, followed by three washes in sterile phosphate buffered saline (PBS) (Sigma, St. Louis, Missouri). Ixodes ricinus and R. microplus were then subjected to the 16S rDNA gene absolute quantification through qPCR and I. ricinus to Illumina sequencing analysis.
Swab skin and blood samples were collected from the vertebrate host (guinea pig). The bacterial recovery from the host skin was performed through the homogenization of the swab skin in 500 μl of sterile PBS. Before blood collection, the host skin was cleaned with 70% ethanol and blood was collected from the jugular vein using a sterile syringe and needle.
Genomic DNA Extraction and Culture-Independent Analysis of the Bacterial Community in the Midgut and Ovaries of I. ricinus
The tick genomic DNA was isolated using the PowerSoil DNA isolation kit (MO BIO, Hilden, Germany) according to the manufacturer's instructions. The bacterial community of the midgut and ovary of wild I. ricinus, the midgut of laboratory-reared I. ricinus, host swab skin, and host blood was investigated by the 16S rDNA sequencing of the total DNA. For the midgut DNA extraction, the whole organ was used, including the lumen content. The protocol was adapted for fully fed females using a double volume of reaction adjusted at the final step. The sequencing library preparation was done at the Integrated Microbiome Resource (IMR) facility, Dalhousie University, Halifax, Nova Scotia, Canada as described by Comeau et al. (2017). Briefly, a dual-indexing one-step PCR strategy was applied using the extracted total DNA as a template to amplify the V6-V8 16S rDNA region (Comeau et al., 2011) with the Illumina Nextera adaptors plus the indices/barcodes. DNA-free water (Top-Bio, Praha, Czech Republic) was used as a negative control. PCR was performed in duplicates using two template dilutions, combined in one plate and visualized on an Invitrogen 96-well E-gel (Invitrogen, California, USA). Amplicons were cleaned-up and normalized using the high-throughput Invitrogen Sequal-Prep 96-well plate kit (Invitrogen, California, USA). Using the Invitrogen Qubit double-stranded DNA high sensitivity fluorescence-based method (Invitrogen, California, USA), the samples were pooled and quantified before the sequencing on an Illumina MiSeq machine.
The raw paired-end sequence reads obtained from the Illumina MiSeq sequencing platform were analyzed using the Mothur bioinformatic software pipeline (version 1.42.3) (Schloss et al., 2009). In short, primers were removed, and paired-end sequences were assembled into contigs. The quality of each contig (sequence hereafter) was checked. Sequence with low quality (q < 25), ambiguous base and ambiguous length (<200 and >450 bp) sequences were removed. High quality sequences were aligned with SSU rRNA SILVA reference alignment (Yilmaz et al., 2014) using Needleman-Wunsch global alignment algorithm (Needleman and Wunsch, 1970). VSEARCH (Rognes et al., 2016) was used to check chimera and removed chimeric sequences if present. High quality, non-chimeric sequences were clustered into operational taxonomic units (OTUs) with 97% sequence similarity using distance-based greedy cluster (DGC) algorithm. The representative sequence for each OTU was used to determine the phylogenetic identification of OTUs. These sequences were compared with SILVA reference datasets (Quast et al., 2013) using basic alignment search tool (BLAST) (Altschul et al., 1997). Erroneous OTUs (<2 sequence reads) were removed from the OTU table. Moreover, OTUs from background bacterial contamination were filtered from OTU table by removing OTUs that were clustered together with OTUs of the DNA-free water sample. The final OTU table was used for further statistical analyses.
Quantification of the Bacterial 16S rDNA and Tick Elongation Factor Genes
The tick genomic DNA was isolated as described above. The single copy gene for tick elongation factor-1α (ef-1α) was used as a reference for data normalization (Nijhof et al., 2009). Quantification of the bacterial 16S rDNA gene (Nadkarni et al., 2002) was carried out in a LightCycler® 480 (Roche, Basel, Switzerland) with 50 cycles of 95°C (10 s), 60°C (10 s), and 72°C (10 s) following an initial denaturation of 95°C (10 min). Each 20 μl reaction mixture contained 10 μl of FastStart universal probe master (Rox) (Roche), 10 pmol of each primer, 5 pmol of TaqMan probe (Nadkarni et al., 2002), 2 μl of genomic DNA, and 5 μl of DNA-free water (Top-Bio, Praha, Czech Republic). Quantification of ef-1α was performed on the same equipment as described above with 50 cycles of 95°C (10 s), 60°C (10 s) and 72°C (10 s) following an initial denaturation of 95°C (10 min). The amplification was performed with 12.5 μl of FastStart universal SYBR green master (Rox) (Roche), 10 pmol of each primer, 2 μl of genomic DNA, and DNA-free water (Top-Bio). In order to perform the absolute quantification, standard curves were plotted using serial dilutions (106-102) of a plasmid containing the fragment of a single copy tick specie-specific ef-1α gene or the bacterial 16S rDNA gene. The ef-1α gene for I. ricinus and the bacterial 16S rDNA gene were cloned into TOPO plasmid vector (Invitrogen, California, USA), while the ef-1α for R. microplus into the pGEM®-T easy (Promega, Wisconsin, USA). Bacterial 16S rDNA counts in DNA-free water (Top-Bio, Praha, Czech Republic) were used as a control for background contamination. Midgut and ovary from seven I. ricinus and six R. microplus ticks were analyzed at each time-point during a feeding course on a vertebrate host. The total 16S rDNA copy number was represented either by an organ or by the tick elongation factor gene. Primers and probe sequences are listed in Table 1.
Statistical Analysis
Statistical analysis of the microbiome quantification data was performed using the GraphPad Prism software (version 8). First, all the data were tested for normality using Shapiro-Wilk test. Differences among three or more groups were determined using Kruskal-Wallis for a non-parametric test. Other comparisons were performed using Mann Whitney test or unpaired t-test depending on the Gaussian distribution. The values were considered statistically significant with p < 0.05. The correlation coefficient was calculated using the Spearman's rank.
Statistical analysis of the microbiome data from 16S rDNA sequencing was performed in R v.3.4.4 (R Core Team, 2018). Alpha diversity (species richness, Shannon diversity index, and Pielou's evenness) were calculated using the vegan package in R (Oksanen et al., 2019). Prevalence of bacterial phyla and genera in individual samples were summarized in a bar plot.
Results
A High Midgut vs. Low Ovary Diversity in I. ricinus
In order to characterize the bacterial diversity of the I. ricinus midgut and ovary, the microbial community from wild and laboratory-reared ticks was investigated using Illumina sequencing. To elucidate the potential source, the microbiota from the host skin and blood was also assessed. The number of 16S rDNA good quality sequences obtained through bioinformatic analysis was used to determine two thresholds: sterility and reliability. The sterility threshold was used as a control for background contamination based on the bacterial counts obtained from DNA-free water (Top-Bio, Praha, Czech Republic). The reliability threshold was arbitrarily determined based on the number of good quality 16S rDNA sequences >1,000, which is directly related to the target DNA biomass and consequently to the DNA amplification. Only those samples above both thresholds were further analyzed (Figure 1 and Table S1).
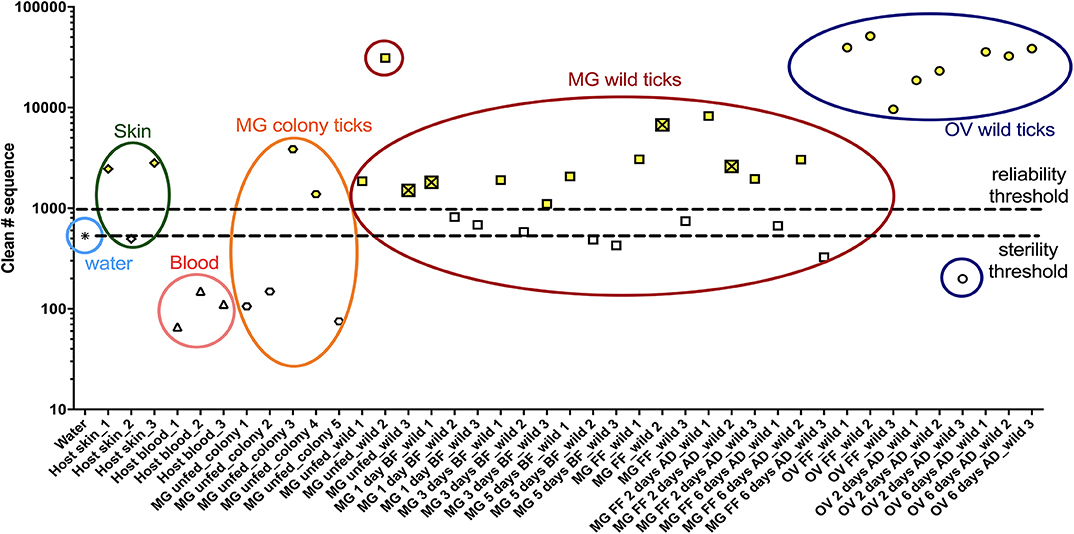
Figure 1. Numbers of good quality 16S rDNA gene sequences obtained through bioinformatic analysis. Samples above both thresholds (yellow) were selected to bacterial diversity analysis. The crossed square represents a tick infected with Borreliella sp. MG, midgut; OV, ovary; UF, unfed; 1D, 1-day; 3D, 3-days; 5D, 5-days; FF, fully fed; AD, after detachment.
A total of 300,206 good quality sequences were obtained and these were clustered into 1,138 OTUs at a similarity level 97% (Table 2 and Table S2). The bacterial diversity in the midgut was higher and variable comparing to that of the ovary, depending on the individual variation and the tick-borne pathogen infection status. Bacteria from the phyla Bacterioidetes and Proteobacteria, Prevotella sp., and Neisseria sp. respectively, were identified from the host skin (Figures 2A,D) as well as from midguts of all blood fed ticks (Figures 2B,E). However, any bacterial genus was found in all the midgut samples irrespective of the tick feeding status (Figure 2E and Table S2). As expected, only wild ticks were infected with tick-borne pathogens. Four out of twelve wild ticks were positive for Borreliella sp., the bacterial agent causing Lyme disease. Spiroplasma sp. was the dominant bacterial taxon in the midgut of two blood fed females. Other bacterial genera including Escherichia sp., Neisseria sp. and Porphyromonas sp. were found in a high frequency in the tick midgut (Figure 2E and Table S2). On average, more bacterial genera were found in the midgut of unfed wild ticks in comparison to that of the laboratory-reared ticks (Figure 2E and Table S2). The dominant bacterial taxon in ovaries was the endosymbiont Midichloria sp. (phylum Proteobacteria) regardless of the tick feeding stage (Figures 2C,F). These results are also reflected in the alpha diversity indices, including Shannon and Pielou's evenness, which are lower for the ovarian samples (Table 2). Midichloria sp. was also identified in the midgut from two unfed wild and two colony ticks, as well as in one blood fed tick (Figure 2E).
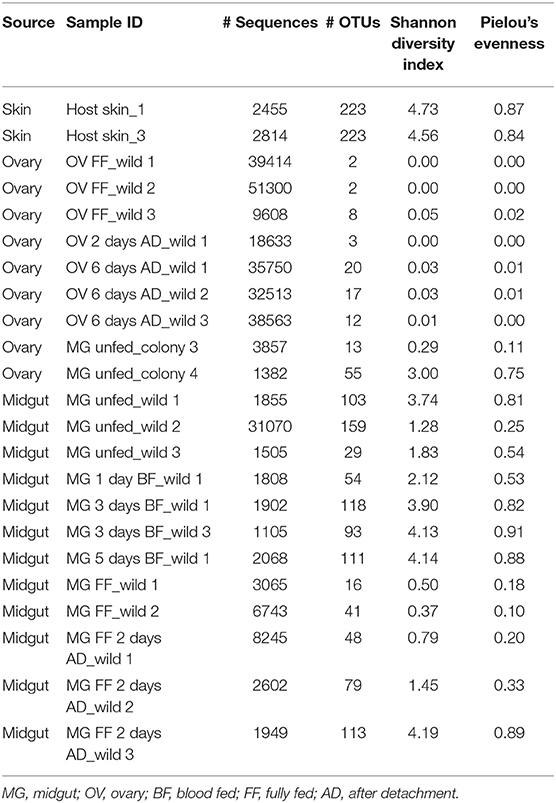
Table 2. Number of good quality sequences and operational taxonomic units (OTUs), and alpha diversity indices of the bacterial community in the ovary and the midgut of Ixodes ricinus.
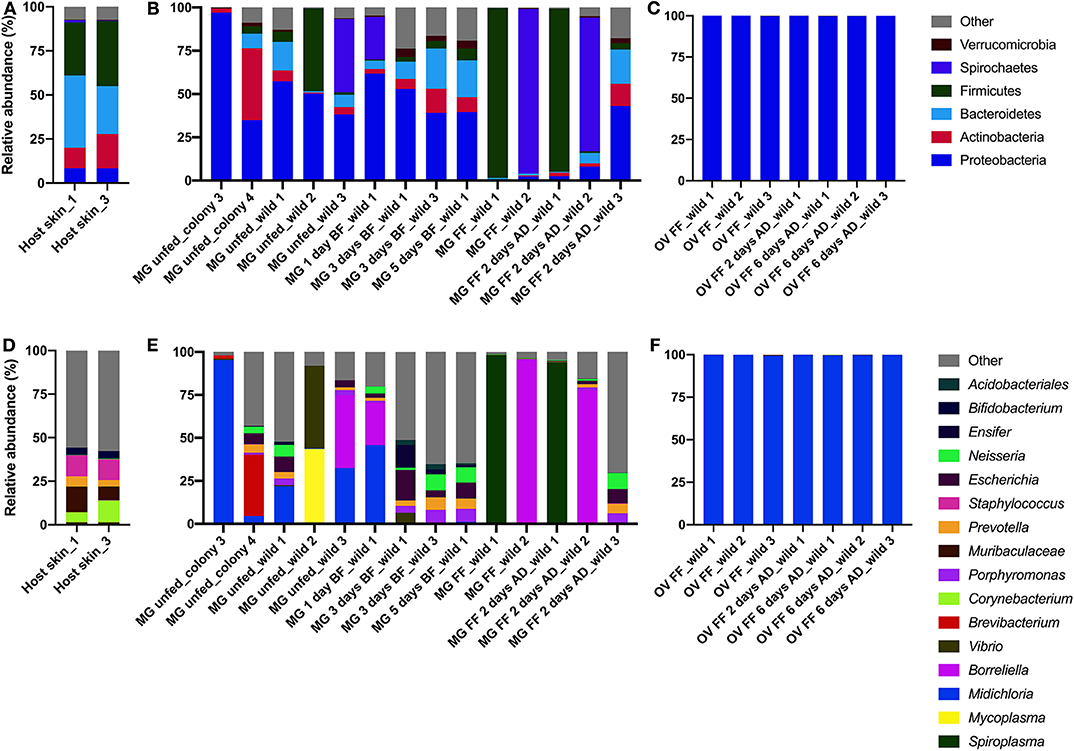
Figure 2. Bacterial diversity in the host skin (A,D) and the midgut (B,E) and ovary (C,F) of Ixodes ricinus on phylum (A–C) and genus (D–F) levels. MG, midgut; OV, ovary; BF, blood fed; FF, fully fed female; AD, after detachment.
Hard Ticks Have Very Low Levels of the Midgut Microbiota During Blood Feeding Stage
The size of the bacterial community in the midgut was followed through a feeding time course of I. ricinus wild females on guinea pigs. The total 16S rDNA level increased slightly but not significantly (p > 0.99) on day 1 of blood feeding and then started decline with statistically significant decreases on day 5 of blood-feeding (p = 0.03) and at the full engorgement (p = 0.009) (Figure 3A and Figure S1A). The total 16S rDNA level in the midgut negatively correlated (r = −0.88) with the tick weight during the feeding course (Figure S2).
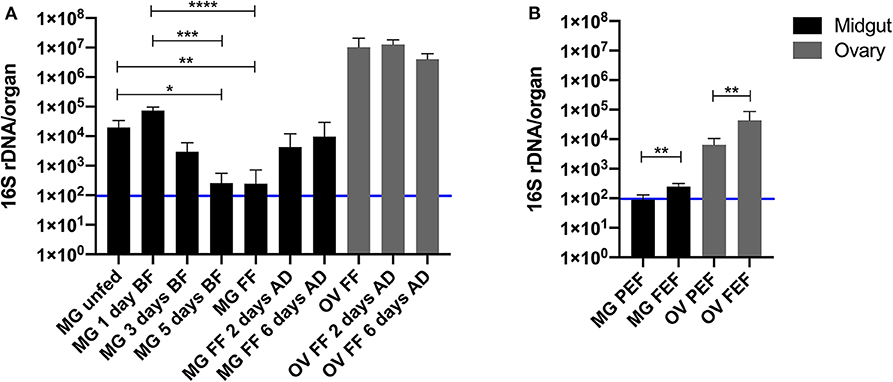
Figure 3. Quantification of the 16S rDNA gene per midgut (black) and ovary (gray) of Ixodes ricinus (A) and Rhipicephalus microplus (B) during blood feeding on a vertebrate host. MG, midgut; OV, ovary; BF, blood fed; FF, fully fed; AD, after detachment; PEF, partially engorged female; FEF, fully engorged female. The number of biological replicates analyzed in each time-point was 7 for I. ricinus and 6 for R. microplus. Error bars indicate standard deviation. The blue line represents the sterility threshold (16S rDNA counts in DNA-free water). Stars indicate statistically significant differences. *p < 0.05; **p < 0.01; ***p < 0.001; and ****p < 0.0001.
Similarly to I. ricinus, very low levels of midgut microbiota were found in R. microplus revealing that only few bacterial cells were found in the whole digestive tract (~100 or less cells). However, due to nature of maintenance of this tick species on cattle, only two time points of blood feeding were examined in these ticks (Figure 3B and Figure S1C). The bacterial 16S rDNA gene copy number in the tick midgut during the blood feeding significantly increased (p = 0.002).
Ovarian Microbial Abundance Greatly Exceeds That of the Midgut in Hard Ticks
In view of the low level of the total midgut microbiota in fully fed females of both, I. ricinus and R. microplus, we were interested in determination of assessment of the total microbial community in tick ovaries. The same fully fed female ticks from which the midguts were used for the total genomic DNA isolation had the ovarian DNA extracted and subjected to the bacterial quantification. For both tick species, I. ricinus and R. microplus, the 16S rDNA gene copy numbers per ovary or per house keeping gene levels exceeded that of the midgut by several orders of magnitude (Figures 3A,B and Figures S1B,D). Ixodes ricinus had 2–5 times order of magnitude higher levels of the bacterial 16S rDNA copy number in the ovary than in the midgut when expressed per organ (Figure 3A) and even higher when expressed per the house keeping gene (Figure S1B). The 16S rDNA absolute number in the ovary of I. ricinus decreased comparing 2–6 days after tick detachment from the host (Figure S1B).
Interestingly, the level of the bacterial 16S rDNA copy number in the ovary of R. microplus significantly increased when represented per organ (p = 0.004) or the house keeping gene (p = 0.02) during the blood feeding (Figure 3B and Figure S1D).
Discussion
The digestive tract of metazoans is typically colonized by a numerous and diverse microbial community with an important role in the host physiology and overall fitness and survival (Engel and Moran, 2013; Heintz-Buschart and Wilmes, 2018). Several exceptions to this rule have been recently reported including some microbiome-free arthropod taxa (Hammer et al., 2019); however, blood-feeding arthropods generally possess a gut microbiota that affects their vector competence for pathogens (Weiss and Aksoy, 2011; Dennison et al., 2014; Narasimhan et al., 2014; Narasimhan and Fikrig, 2015). For hematophagous arthropods, blood is the main source of nutrients and is essential for embryo development and molting of juvenile stages. The influx of blood into the digestive system typically leads to an expansion of the midgut bacterial community in blood feeding arthropods as shown, for example, for mosquitoes and triatomine bugs (Eichler and Schaub, 2002; Oliveira et al., 2011). In Aedes aegypti the blood uptake increased the culturable midgut bacterial population by about three orders of magnitude (from 103 to 106 per insect) (Oliveira et al., 2011). In the triatomine bug, Rhodnius prolixus, the level of the gut bacterial symbiont Rhodococcus rhodnii increased 15-fold in the fifth instars after 10 days of blood feeding and resulted in the population of 108 bacteria per insect (Eichler and Schaub, 2002).
During the feeding course on a vertebrate host, hard ticks increase their size 100–1,000 times reflecting a great influx of blood which represents a nutritionally rich substrate for ticks, potentially available also for bacteria present in the tick midgut. Nevertheless, unlike reported for other hematophagous arthropods, we showed here that in the hard ticks, I. ricinus and R. microplus, the blood intake is not associated with bacterial growth in the midgut. In fact, our results show that there is a negative correlation between the amount of blood consumed and the midgut bacterial level in I. ricinus; 16S rDNA levels per organ ranged from 104 in unfed females to 102 in fully fed females. In R. microplus, due the one-host life cycle for all tick stages, the unfed female could not be obtained for analysis. The bacterial levels in this tick species, regardless the blood influx, remained very low with approximately 102 16S rDNA copies per midgut in partially and fully engorged females. In both cases, these bacterial levels were close to the sterility technical threshold. Taken together, the numbers do not allow to define if the few bacterial cells found in I. ricinus and R. microplus midgut are just a transient population or constitute a resident community.
Several hypotheses were formulated for the primary cause of low bacterial levels in the midgut of ticks. First, it is hypothesized that hemoglobin fragments generated during blood digestion and the complement system from the host blood (Fogaça et al., 1999; Nakajima et al., 2009; Kopáček et al., 2010) negatively impact the midgut bacterial community and cause its rapid decline. The second hypothesis is that the epithelial immunity regulates the bacterial population in the tick midgut by antimicrobial peptides and reactive oxygen species (ROS) (Sonenshine and Macaluso, 2017). For example, it was demonstrated in the I. ricinus midgut transcriptome that the immune genes such as defensin, lysozyme, and microplusin were substantially upregulated at the end of tick feeding (Perner et al., 2016). Moreover, the capillary feeding of Dermacentor variabilis with Escherichia coli resulted in expression of defensin-like peptides in tick midgut (Sonenshine et al., 2002). In the majority of hematophagous animals, the digestion is an extracellular process and takes place in the gut lumen. Ticks, however, represent an exception as they digest blood intracellularly in the specialized digestive midgut cells that take up blood components by means of massive endocytosis (Sojka et al., 2013). The third hypothesis is that the midgut microbiota is reduced in part, by the tick digestive cells. Some midgut microbiota might be endocytosed by the tick digestive epithelial cells along with the vertebrate blood components (Lara, 2005) and consequently digested.
Andreotti et al. (2011) reported that the R. microplus midgut harbors a highly diverse bacterial community belonging to 11 bacterial genera. Similarly, we showed here that the I. ricinus midgut carries a variable bacterial population that differs depending on individual ticks and the tick-borne pathogen infection status. The host skin as a potential source of bacteria in the midgut of I. ricinus is indicated by detecting the same bacterial taxa (e.g., Prevotella sp. and Neisseria sp.) from both, host skin and in the midgut, of blood fed females, regardless the time-point of the blood feeding course. Prevotella sp. has been identified as part of the healthy skin microbiome and Neisseria sp. is common in the mucosal surface of vertebrate hosts of I. ricinus (Elliott et al., 2005; Dimitriu et al., 2019). In contrast to the ovary, the midgut did not show a core microbiome, as we did not detect any common bacterial genera in all midgut samples. It is likely that most of identified bacteria in the midgut originated from the environment; they were accidentally ingested and constitute just a transient population. The hypothesis that the source of the midgut bacteria is the environment is further supported by the finding that wild ticks have a higher bacterial diversity than colony ticks maintained under laboratory-controlled conditions.
Bacterium from the genus Midichloria sp. found in the midgut of unfed and in 1-day blood fed I. ricinus corroborated the study of Olivieri et al. (2019). Vibrio sp. detected in the midgut of five out from fourteen ticks is not usually reported in metagenomic analysis of ticks and it likely represents a bacterium of the environmental origin. Mycoplasma sp. found in the midgut of one unfed I. ricinus has been reported from ticks previously (Hornok et al., 2019) but its significance in tick biology as well as from the clinical perspective is unknown. The midgut of two blood fed ticks were dominated by Spiroplasma sp. Different species in the genus Spiroplasma were described in arthropods and plants in commensal, mutualist, and pathogenic associations (Regassa and Gasparich, 2006). In ticks, this taxon was detected in several species and described as a facultative symbiont (Henning et al., 2006; Duron et al., 2017). Four out of twelve wild ticks were infected with a bacterium from the genus Borreliella, which make part of a complex of species that are vectored by ticks and cause Lyme disease in humans (Lane et al., 1991). Borreliella sp. are acquired by ticks during blood feeding from an infected host at the early tick life-stages and persist in the midgut after tick molting (Benach et al., 1987). Presence of Borreliella sp. in I. ricinus in some parts of Central Europe can be very high (Strnad et al., 2017) and it is therefore not surprising that it was found in the midgut of 33% of the wild ticks analyzed. It appears that both, Spiroplasma sp. and Borreliella sp., are capable of outcompeting other bacteria in the midgut during tick blood feeding and blood digestion and become the dominant member of the tick midgut microbiome. However, our data are limited and studies with larger number of specimen are needed to confirm these results.
In contrast to the tick midgut, we found an abundant and stable bacterial community with very low diversity in the ovary of I. ricinus with the dominant endosymbiont, Midichloria sp. This result corroborates with the finding of high levels of Midichloria sp. in fully fed I. ricinus females (Sassera et al., 2008). In the R. microplus ovary, it was shown that the dominant endosymbiont is Coxiella sp. representing 98.2% of the 16S rDNA sequences in fully engorged females (Andreotti et al., 2011). The presence of Midichloria sp. and Coxiella sp. in tick ovaries and eggs points out to vertical transmission, typical for maternally inherited symbionts (Sassera et al., 2008; Andreotti et al., 2011).
The abundant bacterial population in the tick ovaries and the negligible microbial community in the tick midgut indicate that the physiological role of the gut bacterial community, usually exercised in most metazoan, is accomplished in ticks by the obligate intracellular symbionts housed in the ovary. Indeed, it has been shown that genomes of both, Midichloria mitochondrii and Coxiella sp., encode genes that participate in the metabolic pathways for the biosynthesis of vitamins and cofactors suggested to be missed in the host blood (Sassera et al., 2011; Guizzo et al., 2017). Coxiella sp. in the ovary of R. microplus was demonstrated to play an important role in the tick development and reproductive fitness (Zhong et al., 2007; Guizzo et al., 2017; Zhang et al., 2017). Although the role of M. mitochondrii in biology of I. ricinus is likely similar to that of Coxiella sp. in R. microplus, further studies are needed to demonstrate its specific role.
In conclusion, using the quantification of the total 16S rDNA gene, we report here very low levels of the midgut microbiota in I. ricinus and R. microplus, without a core microbiome in I. ricinus. This corroborates the findings of a limited and unstable midgut microbiota in Ixodes scapularis (Ross et al., 2018). In contrast, several magnitudes higher levels of 16S rDNA were found in the ovaries of both tick species represented in I. ricinus by an endosymbiont Midichloria sp. The bacterial distribution in tick internal organs is an exception of the general biology of metazoans, characterized by a high ratio of a tick midgut/ovary microbiome.
Data Availability Statement
Sequenced OTUs were deposited in the Genbank database under accession numbers MT255253- MT256050.
Ethics Statement
This animal study was reviewed and approved by the Animal Protection Law of the Czech Republic No. 246/1992 Sb., ethics approval No. 25/2018. This study was approved by the Institute of Parasitology, Biology Centre CAS and Central Committee for Animal Welfare, Czech Republic (Protocol No. 1/2015). Ethics Committee on Animal Experimentation and were approved under the registry 14403/protocol 07.
Author Contributions
MG conducted the experiments, analyzed the results, and wrote the manuscript. SN analyzed the results of 16S rDNA survey. MK and HF assisted with the experiments. JP, IS, PK, PO, and LZ conceived the experiments and wrote the manuscript. All authors reviewed the manuscript.
Funding
This study was mainly funded by the Czech Science Foundation (GACR) Grant No: 19-04301S to LZ and PK; MG, JP, and PK were additionally supported by the Centre for research of pathogenicity and virulence of parasites (No. CZ.02.1.01/0.0/0.0/16_019/0000759) funded by European Regional Development Fund (ERDF) and Ministry of Education, Youth and Sport (MEYS). We would like to acknowledge the support from the Conselho Nacional de Desenvolvimento Científico e Tecnológico CNPq: 573959/2008-0, Coordenação de Aperfeiçoamento de Pessoal de Nível Superior (CAPES: Finance Code 001) and Instituto Nacional de Ciência e Tecnologia em Entomologia Molecular (INCT-EM) to MG, IS, and PO. We are grateful to Radek Sima for the generation of 16S rDNA standard curve and also Jan Erhart and Tereza Hatalová for excellent technical support.
Conflict of Interest
The authors declare that the research was conducted in the absence of any commercial or financial relationships that could be construed as a potential conflict of interest.
Supplementary Material
The Supplementary Material for this article can be found online at: https://www.frontiersin.org/articles/10.3389/fcimb.2020.00211/full#supplementary-material
Figure S1. Quantification of the 16S rDNA gene per the elongation ef-1α in the midgut (A) and ovary (B) of Ixodes ricinus and in the midgut (C) and ovary (D) of Rhipicephalus microplus during blood feeding on a vertebrate host. MG, midgut; OV, ovary; BF, blood fed; FF, fully fed; AD, after detachment; PEF, partially engorged female; FEF, fully engorged female. Each time-point represents the median of 7 biological replicates for I. ricinus and 6 for R. microplus. Error bars indicate standard deviation. Stars indicate statistically significant differences. *p < 0.05; **p < 0.01; and ****p < 0.0001.
Figure S2. Negative correlation between 16S rDNA gene in the midgut per the ef-1α and Ixodes ricinus weight during blood feeding on a vertebrate host. The results represent the median for 7 organs. Spearman's rank correlation coefficient = −0.88.
Table S1. Number of good quality 16S rDNA gene sequences obtained through bioinformatic analysis for the analyzed samples. MG, midgut; OV, ovary; BF, blood fed; FF, fully fed; AD, after detachment. Samples in yellow are above both sterility and reliability threshold. Samples in red were removed from the final analysis.
Table S2. Overview of the identified OTUs, and its classification into bacterial phylum and genera.
References
Altschul, S. F., Madden, T. L., Schäffer, A. A., Zhang, J., Zhang, Z., Miller, W., et al. (1997). Gapped BLAST and PSI-BLAST: a new generation of protein database search programs. Nucleic Acids Res. 25, 3389–402. doi: 10.1093/nar/25.17.3389
Andreotti, R., Pérez de León, A. A., Dowd, S. E., Guerrero, F. D., Bendele, K. G., and Scoles, G. A. (2011). Assessment of bacterial diversity in the cattle tick Rhipicephalus (Boophilus) microplus through tag-encoded pyrosequencing. BMC Microbiol. 11:6. doi: 10.1186/1471-2180-11-6
Benach, J. L., Coleman, J. L., Skinner, R. A., and Rosler, E. M. (1987). Adult ixodes dammini on rabbits: a hypothesis for the development and transmission of borrelia burgdorferi. J. Infect. Dis. 155, 1300–6. doi: 10.1093/infdis/155.6.1300
Binetruy, F., Dupraz, M., Buysse, M., and Duron, O. (2019). Surface sterilization methods impact measures of internal microbial diversity in ticks. Parasit. Vectors 12:268. doi: 10.1186/s13071-019-3517-5
Bonnet, S. I., Binetruy, F., Hernández-Jarguín, A. M., and Duron, O. (2017). The tick microbiome: why non-pathogenic microorganisms matter in tick biology and pathogen transmission. Front. Cell. Infect. Microbiol. 7:236. doi: 10.3389/fcimb.2017.00236
Budachetri, K., Browning, R. E., Adamson, S. W., Dowd, S. E., Chao, C. C., Ching, W. M., et al. (2014). An insight into the microbiome of the Amblyomma maculatum (Acari: Ixodidae). J. Med. Entomol. 51, 119–29. doi: 10.1603/me12223
Carpi, G., Cagnacci, F., Wittekindt, N. E., Zhao, F., Qi, J., Tomsho, L. P., et al. (2011). Metagenomic profile of the bacterial communities associated with Ixodes ricinus ticks. PLoS ONE 6:e25604. doi: 10.1371/journal.pone.0025604
Clayton, K. A., Gall, C. A., Mason, K. L., Scoles, G. A., and Brayton, K. A. (2015). The characterization and manipulation of the bacterial microbiome of the Rocky mountain wood tick, Dermacentor andersoni. Parasit. Vectors 8:632. doi: 10.1186/s13071-015-1245-z
Comeau, A. M., Douglas, G. M., and Langille, M. G. I. (2017). Microbiome helper: a custom and streamlined workflow for microbiome research. mSystems 2:e00127-16. doi: 10.1128/msystems.00127-16
Comeau, A. M., Li, W. K. W., Tremblay, J. É., Carmack, E. C., and Lovejoy, C. (2011). Arctic ocean microbial community structure before and after the 2007 record sea ice minimum. PLoS ONE 6:e27492. doi: 10.1371/journal.pone.0027492
Dennison, N. J., Jupatanakul, N., and Dimopoulos, G. (2014). The mosquito microbiota influences vector competence for human pathogens. Curr. Opin. Insect Sci. 3, 6–13. doi: 10.1016/j.cois.2014.07.004
Dimitriu, P. A., Iker, B., Malik, K., Leung, H., Mohn, W. W., and Hillebrand, G. G. (2019). New insights into the intrinsic and extrinsic factors that shape the human skin microbiome. MBio 10:e00839-19. doi: 10.1128/mBio.00839-19
Duron, O., Binetruy, F., Noël, V., Cremaschi, J., McCoy, K. D., Arnathau, C., et al. (2017). Evolutionary changes in symbiont community structure in ticks. Mol. Ecol. 26, 2905–2921. doi: 10.1111/mec.14094
Duron, O., Morel, O., Noël, V., Buysse, M., Binetruy, F., Lancelot, R., et al. (2018). Tick-bacteria mutualism depends on B vitamin synthesis pathways. Curr. Biol. 28, 1896–1902.e5. doi: 10.1016/j.cub.2018.04.038
Eichler, S., and Schaub, G. A. (2002). Development of symbionts in triatomine bugs and the effects of infections with trypanosomatids. Exp. Parasitol. 100, 17–27. doi: 10.1006/expr.2001.4653
Elliott, D. R., Wilson, M., Buckley, C. M. F., and Spratt, D. A. (2005). Cultivable oral microbiota of domestic dogs. J. Clin. Microbiol. 43, 5470–6. doi: 10.1128/JCM.43.11.5470-5476.2005
Engel, P., and Moran, N. A. (2013). The gut microbiota of insects - diversity in structure and function. FEMS Microbiol. Rev. 37, 699–735. doi: 10.1111/1574-6976.12025
Estrada-Peña, A. (2015). Ticks as vectors: taxonomy, biology and ecology. Rev. Sci. Tech. 34, 53–65. doi: 10.20506/rst.34.1.2345
Fogaça, A. C., Da Silva, P. I., Miranda, M. T. M., Bianchi, A. G., Miranda, A., Ribolla, P. E. M., et al. (1999). Antimicrobial activity of a bovine hemoglobin fragment in the tick Boophilus microplus. J. Biol. Chem. 274, 25330–4. doi: 10.1074/jbc.274.36.25330
Goodrich, J. K., Di Rienzi, S. C., Poole, A. C., Koren, O., Walters, W. A., Caporaso, J. G., et al. (2014). Conducting a microbiome study. Cell 158, 250–262. doi: 10.1016/j.cell.2014.06.037
Greay, T. L., Gofton, A. W., Paparini, A., Ryan, U. M., Oskam, C. L., and Irwin, P. J. (2018). Recent insights into the tick microbiome gained through next-generation sequencing. Parasit. Vectors 11:12. doi: 10.1186/s13071-017-2550-5
Guizzo, M. G., Parizi, L. F., Nunes, R. D., Schama, R., Albano, R. M., Tirloni, L., et al. (2017). A Coxiella mutualist symbiont is essential to the development of Rhipicephalus microplus. Sci. Rep. 7:17554. doi: 10.1038/s41598-017-17309-x
Hammer, T. J., Sanders, J. G., and Fierer, N. (2019). Not all animals need a microbiome. FEMS Microbiol. Lett. 366:fnz117. doi: 10.1093/femsle/fnz117
Heintz-Buschart, A., and Wilmes, P. (2018). Human gut microbiome: function matters. Trends Microbiol. 26, 563–574. doi: 10.1016/j.tim.2017.11.002
Henning, K., Greiner-Fischer, S., Hotzel, H., Ebsen, M., and Theegarten, D. (2006). Isolation of spiroplasma sp. from an ixodes tick. Int. J. Med. Microbiol. 40, 157–61. doi: 10.1016/j.ijmm.2006.01.012
Hornok, S., Szoke, K., Meli, M. L., Sándor, A. D., Görföl, T., Estók, P., et al. (2019). Molecular detection of vector-borne bacteria in bat ticks (acari: ixodidae, argasidae) from eight countries of the old and new worlds 06 biological sciences 0604 genetics. Parasit. Vectors. 12:50. doi: 10.1186/s13071-019-3303-4
Huttenhower, C., Knight, R., Brown, C. T., Caporaso, J. G., Clemente, J. C., Gevers, D., et al. (2014). Advancing the microbiome research community. Cell 159, 227–30. doi: 10.1016/j.cell.2014.09.022
Kopáček, P., Hajdušek, O., Burešová, V., and Daffre, S. (2010). Tick innate immunity. Adv. Exp. Med. Biol. 708, 137–162. doi: 10.1007/978-1-4419-8059-5-8
Lane, R. S., Piesman, J., and Burgdorfer, W. (1991). Lyme borreliosis: relation of its causative agent to its vectors and hosts in North America and Europe. Annu. Rev. Entomol. 36, 587–609. doi: 10.1146/annurev.ento.36.1.587
Lara, F. A. (2005). Tracing heme in a living cell: hemoglobin degradation and heme traffic in digest cells of the cattle tick Boophilus microplus. J. Exp. Biol. 208, 3093–3101. doi: 10.1242/jeb.01749
Moreno, C. X., Moy, F., Daniels, T. J., Godfrey, H. P., and Cabello, F. C. (2006). Molecular analysis of microbial communities identified in different developmental stages of Ixodes scapularis ticks from westchester and dutchess counties, New York. Environ. Microbiol. 8, 761–72. doi: 10.1111/j.1462-2920.2005.00955.x
Nadkarni, M. A., Martin, F. E., Jacques, N. A., and Hunter, N. (2002). Determination of bacterial load by real-time PCR using a broad-range (universal) probe and primers set. Microbiology 148(Pt 1), 257–266. doi: 10.1099/00221287-148-1-257
Nakajima, Y., Ogihara, K., Taylor, D., and Yamakawa, M. (2009). Antibacterial hemoglobin fragments from the midgut of the soft tick, Ornithodoros moubata (Acari: Argasidae). J. Med. Entomol. 40, 78–81. doi: 10.1603/0022-2585-40.1.78
Narasimhan, S., and Fikrig, E. (2015). Tick microbiome: the force within. Trends Parasitol. 31, 315–323. doi: 10.1016/j.pt.2015.03.010
Narasimhan, S., Rajeevan, N., Liu, L., Zhao, Y. O., Heisig, J., Pan, J., et al. (2014). Gut microbiota of the tick vector Ixodes scapularis modulate colonization of the Lyme disease spirochete. Cell Host Microbe 15, 58–71. doi: 10.1016/j.chom.2013.12.001
Needleman, S. B., and Wunsch, C. D. (1970). A general method applicable to the search for similarities in the amino acid sequence of two proteins. J. Mol. Biol. 48, 443–53. doi: 10.1016/0022-2836(70)90057-4
Nijhof, A. M., Balk, J. A., Postigo, M., and Jongejan, F. (2009). Selection of reference genes for quantitative RT-PCR studies in Rhipicephalus (Boophilus) microplus and Rhipicephalus appendiculatus ticks and determination of the expression profile of Bm86. BMC Mol. Biol. 10:112. doi: 10.1186/1471-2199-10-112
Oksanen, J., Blanchet, F. G., Friendly, M., Kindt, R., Legendre, P., McGlinn, D., et al. (2019). vegan: Community Ecology Package. R package version 2.5-2. Cran R.
Oliveira, J. H. M., Gonçalves, R. L. S., Lara, F. A., Dias, F. A., Gandara, A. C. P., Menna-Barreto, R. F. S., et al. (2011). Blood meal-derived heme decreases ROS levels in the midgut of Aedes aegypti and allows proliferation of intestinal microbiota. PLoS Pathog. 7:e1001320. doi: 10.1371/journal.ppat.1001320
Olivieri, E., Epis, S., Castelli, M., Varotto Boccazzi, I., Romeo, C., Desirò, A., et al. (2019). Tissue tropism and metabolic pathways of Midichloria mitochondrii suggest tissue-specific functions in the symbiosis with Ixodes ricinus. Ticks Tick. Borne. Dis. 10, 1070–1077. doi: 10.1016/j.ttbdis.2019.05.019
Perner, J., Provazník, J., Schrenková, J., Urbanová, V., Ribeiro, J. M. C., and Kopáček, P. (2016). RNA-seq analyses of the midgut from blood- and serum-fed Ixodes ricinus ticks. Sci. Rep. 6:36695. doi: 10.1038/srep36695
Quast, C., Pruesse, E., Yilmaz, P., Gerken, J., Schweer, T., Yarza, P., et al. (2013). The SILVA ribosomal RNA gene database project: Improved data processing and web-based tools. Nucleic Acids Res. 41, D590–D596. doi: 10.1093/nar/gks1219
R Core Team (2018). R: A language and environment for statistical computing. R Foundation for Statistical Computing, Vienna. Available online at: https://www.R-project.org/
Reck, J., Berger, M., Terra, R. M. S., Marks, F. S., da Silva Vaz, I., Guimarães, J. A., et al. (2009). Systemic alterations of bovine hemostasis due to Rhipicephalus (Boophilus) microplus infestation. Res. Vet. Sci. 86, 56–62. doi: 10.1016/j.rvsc.2008.05.007
Regassa, L. B., and Gasparich, G. E. (2006). Spiroplasmas: evolutionary relationships and biodiversity. Front. Biosci. 11, 2983–3002. doi: 10.2741/2027
Rognes, T., Flouri, T., Nichols, B., Quince, C., and Mahé, F. (2016). VSEARCH: a versatile open source tool for metagenomics. PeerJ. 4:e2584. doi: 10.7717/peerj.2584
Ross, B. D., Hayes, B., Radey, M. C., Lee, X., Josek, T., Bjork, J., et al. (2018). Ixodes scapularis does not harbor a stable midgut microbiome. ISME J. 12, 2596–2607. doi: 10.1038/s41396-018-0161-6
Sassera, D., Lo, N., Bouman, E. A. P., Epis, S., Mortarino, M., and Bandi, C. (2008). “Candidatus midichloria” endosymbionts bloom after the blood meal of the host, the hard tick Ixodes ricinus. Appl. Environ. Microbiol. 74, 6138–40. doi: 10.1128/AEM.00248-08
Sassera, D., Lo, N., Epis, S., D'Auria, G., Montagna, M., Comandatore, F., et al. (2011). Phylogenomic evidence for the presence of a flagellum and cbb 3 oxidase in the free-living mitochondrial ancestor. Mol. Biol. Evol. 28, 3285–96. doi: 10.1093/molbev/msr159
Schloss, P. D., Westcott, S. L., Ryabin, T., Hall, J. R., Hartmann, M., Hollister, E. B., et al. (2009). Introducing mothur: open-source, platform-independent, community-supported software for describing and comparing microbial communities. Appl. Environ. Microbiol. 75, 7537–7541. doi: 10.1128/AEM.01541-09
Sojka, D., Franta, Z., Horn, M., Caffrey, C. R., Mareš, M., and Kopáček, P. (2013). New insights into the machinery of blood digestion by ticks. Trends Parasitol. 29, 276–285. doi: 10.1016/j.pt.2013.04.002
Sonenshine, D. E., Ceraul, S. M., Hynes, W. E., Macaluso, K. R., and Azad, A. F. (2002). Expression of defensin-like peptides in tick hemolymph and midgut in response to challenge with Borrelia burgdorferi, Escherichia coli and Bacillus subtilis. Exp. Appl. Acarol. 28, 127–34. doi: 10.1023/A:1025354326877
Sonenshine, D. E., and Macaluso, K. R. (2017). Microbial invasion vs. tick immune regulation. Front. Cell. Infect. Microbiol. 7:390. doi: 10.3389/fcimb.2017.00390
Strnad, M., Hönig, V., RuŽek, D., Grubhoffer, L., and Rego, R. O. M. (2017). Europe-wide meta-analysis of Borrelia burgdorferi sensu lato prevalence in questing Ixodes ricinus ticks. Appl. Environ. Microbiol. 83:e00609-17. doi: 10.1128/AEM.00609-17
Strong, M. J., Xu, G., Morici, L., Splinter Bon-Durant, S., Baddoo, M., Lin, Z., et al. (2014). Microbial contamination in next generation sequencing: implications for sequence-based analysis of clinical samples. PLoS Pathog. 10:e1004437. doi: 10.1371/journal.ppat.1004437
Vayssier-Taussat, M., Kazimirova, M., Hubalek, Z., Hornok, S., Farkas, R., Cosson, J. F., et al. (2015). Emerging horizons for tick-borne pathogens: From the “one pathogen-one disease” vision to the pathobiome paradigm. Future Microbiol. 10, 2033–43. doi: 10.2217/fmb.15.114
Weiss, B., and Aksoy, S. (2011). Microbiome influences on insect host vector competence. Trends Parasitol. 27, 514–22. doi: 10.1016/j.pt.2011.05.001
Yilmaz, P., Parfrey, L. W., Yarza, P., Gerken, J., Pruesse, E., Quast, C., et al. (2014). The SILVA and “all-species living tree project (LTP)” taxonomic frameworks. Nucleic Acids Res. 42, D643–D648. doi: 10.1093/nar/gkt1209
Zhang, C. M., Li, N. X., Zhang, T. T., Qiu, Z. X., Li, Y., Li, L. W., et al. (2017). Endosymbiont CLS-HI plays a role in reproduction and development of Haemaphysalis longicornis. Exp. Appl. Acarol. 73, 429–438. doi: 10.1007/s10493-017-0194-y
Keywords: tick, Ixodes ricinus, Rhipicephalus microplus, midgut microbiome, ovary microbiome, symbiosis, Midichloria mitochondrii
Citation: Guizzo MG, Neupane S, Kucera M, Perner J, Frantová H, da Silva Vaz I, Oliveira PL, Kopacek P and Zurek L (2020) Poor Unstable Midgut Microbiome of Hard Ticks Contrasts With Abundant and Stable Monospecific Microbiome in Ovaries. Front. Cell. Infect. Microbiol. 10:211. doi: 10.3389/fcimb.2020.00211
Received: 28 November 2019; Accepted: 17 April 2020;
Published: 08 May 2020.
Edited by:
Alejandro Cabezas-Cruz, Institut National de la Recherche Agronomique (INRA), FranceReviewed by:
Travis Bourret, Creighton University, United StatesDavide Sassera, University of Pavia, Italy
Sandra Antunes, New University of Lisbon, Portugal
Copyright © 2020 Guizzo, Neupane, Kucera, Perner, Frantová, da Silva Vaz, Oliveira, Kopacek and Zurek. This is an open-access article distributed under the terms of the Creative Commons Attribution License (CC BY). The use, distribution or reproduction in other forums is permitted, provided the original author(s) and the copyright owner(s) are credited and that the original publication in this journal is cited, in accordance with accepted academic practice. No use, distribution or reproduction is permitted which does not comply with these terms.
*Correspondence: Melina Garcia Guizzo, melinaguizzo@gmail.com; Ludek Zurek, zureklu@vfu.cz