Possible Emergence of Zika Virus of African Lineage in Brazil and the Risk for New Outbreaks
- 1Laboratory of Genomic Medicine, Experimental Research Center, Hospital de Clínicas de Porto Alegre, Porto Alegre, Brazil
- 2Laboratory of Virology, Department of Microbiology, Immunology and Parasitology, Institute of Health Sciences, Universidade Federal do Rio Grande do Sul, Porto Alegre, Brazil
- 3Postgraduate Program in Genetics and Molecular Biology, Department of Genetics, Universidade Federal do Rio Grande do Sul, Porto Alegre, Brazil
- 4Teratogen Information Service, Hospital de Clínicas de Porto Alegre, Porto Alegre, Brazil
- 5Postgraduate Program in Bioscience, Universidade Federal de Ciências da Saúde de Porto Alegre, Porto Alegre, Brazil
- 6Department of Morphological Sciences, Institute of Health Sciences, Universidade Federal do Rio Grande do Sul, Porto Alegre, Brazil
- 7Postgraduate Program in Medicine: Medical Sciences, Universidade Federal do Rio Grande do Sul, Porto Alegre, Brazil
Introduction
In 2015, an outbreak of Zika virus (ZIKV) infection caused a disproportionate increase in the number of cases of microcephaly in Brazil (de Oliveira et al., 2017). Although two major ZIKV lineages referred to as African lineage (ZIKVAF) and Asian lineage (ZIKVAS) have been identified, phylogenetic and genomic analyses pointed to those cases as caused by a ZIKVAS (Faria et al., 2017). This link led scientists to not target ZIKVAF on their investigations. Due to this, information about ZIKVAF circulation in Brazil has been underestimated as well as its epidemic potential.
Recently, a study published by Kasprzykowski et al. (2020) identified ZIKVAF present among Brazilian ZIKV sequences. ZIKVAF was identified in non-human primates (NHPs) and mosquitoes in the South and Southeast Brazilian regions, respectively (Figure 1) (de Almeida et al., 2019– Preprint publication; Alencar et al., 2021). At the same time, Aubry et al. (2021) showed ZIKVAF being more transmissible in mosquitoes and more lethal in mice when compared to ZIKVAS. In addition, it was suggested that there was a high epidemic potential of ZIKVAF. Since South and Southeast Brazilian regions are geographically distant (> 1,500 km) and climatically different, and the ZIKVAF was found from different sources, here we raise and bring concerns about the circulation of this lineage in Brazil and the risk it might represent as the cause of a new outbreak of disease in humans potentially associated with birth defects.
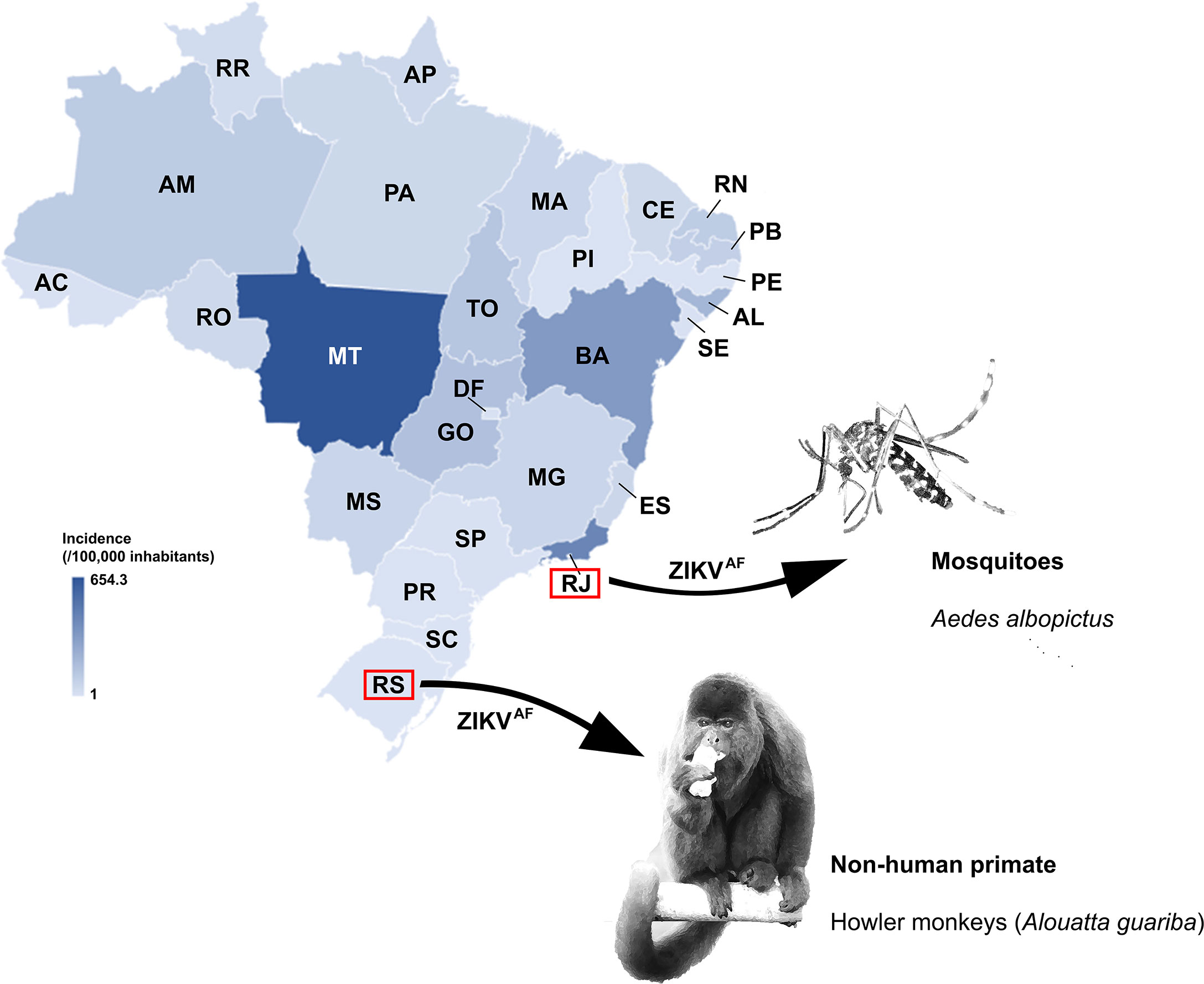
Figure 1 Incidence per 100,000 inhabitants of Zika cases in Brazil reported in 2016. Color gradient representing high (darker blue) to low ZIKV incidence (lighter blue). The Brazilian states where ZIKVAF was identified in sylvatic area are also highlighted. Zika virus incidence data were recovered from Brazil Ministry of Health (2018). AC, Acre; AL, Alagoas; AP, Amapá; AM, Amazonas; BA, Bahia; CE, Ceará; DF, Distrito Federal; ES, Espírito Santo; GO, Goiás; MA, Maranhão; MT, Mato Grosso; MS, Mato Grosso do Sul; MG, Minas Gerais; PA, Pará; PB, Paraíba; PR, Paraná; PE, Pernambuco; PI, Piauí; RJ, Rio de Janeiro; RN, Rio Grande do Norte; RS, Rio Grande do Sul; RO, Rondônia; RR, Roraima; SC, Santa Catarina; SP, São Paulo; SE, Sergipe; TO, Tocantins.
Zika Virus: Epidemiological Profile and a Threat of New Outbreaks
The Zika virus epidemic spread quickly through South America, extending its range beyond the areas of recurrent transmission of arboviruses, including areas restricted by climatic barriers or low population density (Lowe et al., 2018). After the outbreak in 2015/2016, at least two smaller waves associated with ZIKVAS were reported in 2017 and 2018 in two geographically distant and climatically different Brazilian regions, Southeast and Northern (Giovanetti et al., 2020; Iani et al., 2021). These sporadic outbreaks highlight the ongoing virus circulation, despite the considerable decrease in incidence of ZIKV-associated disease in human.
It is possible that the decline in prevalence of ZIKV disease is due to a buildup in herd immunity (Masmejan et al., 2020). Investigations performed in a city of Northeastern Brazil, a region highly affected by the virus, revealed that 63%–73% of the population had been exposed to the virus by the end of the 2015 outbreak (Netto et al., 2017). This provides substantial evidence to support the idea that the resulting herd immunity led ZIKV disease to become a rare event. Early epidemiological modeling investigations estimated that ZIKV infection rates during the pandemic in the Americas provided sufficient herd immunity to mitigate the risk of another large epidemic for at least another decade (Ferguson et al., 2016). However, a serological surveillance is essential to monitor ZIKV seroprevalence and to confirm this estimative. The persistency of neutralizing antibodies should be monitored through time to determine if there is or not a decline of immunity (Henderson et al., 2020). In addition, the degree of re-infection that humans might experience over time, in areas where infection is prevalent in mosquitoes, remains to be determined. The imbalance between the circulation of ZIKV in mosquitoes and the decline in herd immunity might dictate the risk for new outbreaks or for the reemergence of ZIKV. Herd immunity might not explain the decrease in ZIKV disease in humans in regions displaying low incidence. Other factors such as climatic condition, demographics, vector incidence, and mutation in the viral genome might also be influential (Masmejan et al., 2020; Liu et al., 2021).
In Brazil, ZIKV disease displayed uneven epidemiological outcomes across the regions (PAHO/WHO, 2017; Brazil Ministry of Health, 2018) (Figure 1), thus the epidemic potential of ZIKV to cause disease in humans and new outbreaks cannot be discarded. In 2016, the Northeast, Southeast, and Central-West regions were severely hit by the ZIKV epidemic accounting for 93.5% of the nationwide reported cases (16.11, 42.98 and 15.8%, respectively), whereas in Southern Brazil, only few cases of ZIKV infection (< 0.5%) had been reported (PAHO/WHO, 2017; Brazil Ministry of Health, 2018; Lowe et al., 2018) (Figure 1). Regions where most of the population has not had contact with the ZIKV may be especially vulnerable. Moreover, the official diagnostic tests for ZIKV infection have been performed by immunoassay (ELISA test) and/or by molecular assay (PCR), and none of these usual approaches allows for the determination of ZIKV lineages. Thus, all positive results are considered ZIKVAS, and it might contribute to ZIKVAF being unnoticed by public health surveillance systems. Clearly, diagnostic methods should be revised to include strain differential tests, since the proportion of ZIKVAF and ZIKVAS circulating in Brazil is as yet unknown. Additionally, asymptomatic infections or absence of severe infection outcomes such as microcephaly or Congenital Zika Syndrome could have contributed to unnoticed ZIKV detection, regardless of the lineage.
In addition to uneven epidemiological outcomes of ZIKV disease, Brazil is a tropical country that displays favorable environmental and climatic conditions to sustain multiplication of mosquitoes as well as alternative reservoirs. Eventually, these vectors may be carrying ZIKV, regardless of the lineages and supporting a possible establishment of a sylvatic cycle, critical for the arbovirus maintenance (Lowe et al., 2018; Giovanetti et al., 2020). Recently, ZIKVAF circulation in mosquitoes and NHPs in sylvatic areas in Brazil was evidenced (Figure 1) (de Almeida et al., 2019– Preprint publication; Alencar et al., 2021). This lineage displayed higher transmissibility in Aedes aegypti than ZIKVAS, and it was also verified to mosquitoes from South American (Aubry et al., 2021). Alencar et al. (2021) found Ae. albopictus naturally infected with ZIKVAF in the Southeast of Brazil, recovered from the Atlantic Forest. The ZIKV sequence from Ae. albopictus was classified as ZIKVAF by an automated sub-typing screening system developed by Kasprzykowski et al. (2020). This species is a recognized vector for arboviruses (Vazeille et al., 2010) and has been detected in all Brazilian urban and peri-urban areas (Carvalho et al., 2014), highlighting its high adaptability to different environmental conditions (Figueiredo, 2019). Unfortunately, in Brazil, the ongoing national entomological surveillance program reports on Ae. aegypti only, because this species is considered the main vector of transmission between humans and it is widely spread across Brazilian regions (Boyer et al., 2018). Moreover, the program targets exclusively the incidence of the vector and does not provide the virological investigation. Consequently, viruses circulating Ae aegypti as well as in other mosquito species that might act as potential vectors for arboviruses are not officially reported.
In free-living NHPs, ZIKVAF was identified in Alouatta guariba in Southern Brazil (de Almeida et al., 2019 – Preprint publication). The viral genome was sequenced and it was observed to display 98% similarity with the MR766 strain, isolated in Uganda, a representative of the ZIKVAF. Alouatta guariba is a fairly common NHP in the Atlantic Forest (Culot et al., 2019). The species was hit hard in past yellow fever outbreaks as the genus is one of the most vulnerable to the disease (Moreno et al., 2015; dos Santos et al., 2020). The susceptibility of this species to ZIKV is yet to be described in detail, and predictive modeling systems estimated an alarming risk for the genus to be potentially involved as reservoirs in future reemergence of arboviruses events to humans (Han et al., 2019), not excluding ZIKVAF. In 2016, Brazilian research identified ZIKVAS in marmosets and capuchin monkeys captured in Northeastern Brazil, a region largely affected by the virus (Favoretto et al., 2019). These peri-domestic animals were captured in proximity to humans in areas with reports of ZIKV-associated microcephaly cases during the epidemic period. Considering that ZIKV can circulate in NHPs, surveillance programs should include these potential reservoirs in wild environments, since the role of these animals in the epidemiology and prevalence of ZIKV remains unknown.
Since in Brazil there are temporally-consistent reports about the incidence of the vectors in all regions (Brazil Ministry of Health, 2018), and potential reservoirs, along with ZIKVAF detection, even if only detected in the sylvatic area, the risk of a new outbreak caused by this lineage may not be discarded. Due to scarcity of data and testing negligence it is possible that the ZIKVAF is also circulating in the country and could be spread, since its transmission efficiency in Ae aegypti is better than ZIKVAS.
African Zika Virus Lineage: Experimental Findings
Experimental evidence in vitro and in vivo points towards higher transmissibility and pathogenicity of ZIKVAF causing more productive and lethal infections than ZIKVAS in cell culture (Anfasa et al., 2017; Gabriel et al., 2017; Sheridan et al., 2018; Smith et al., 2018) and more severe pathology in mice (Shao et al., 2017; Smith et al., 2018; Jaeger et al., 2019). In human cells, ZIKVAF was able to infect and replicate in neural stem cells and astrocytes more efficiently than ZIKVAS (Simonin et al., 2016). A clear disruption of normal gene expression levels was also observed in human prostate cells when infected by ZIKVAF (Machado et al., 2021).
The ZIKVAF has been reported for its high pathogenicity and teratogenic potential in animals model (Tripathi et al., 2017; Nunes et al., 2020). ZIKVAF caused stronger infection, inflammation and transmission between fetuses than ZIKVAS in a porcine model (Udenze et al., 2019). A comparison between the lineages also showed that ZIKVAF induces higher embryo mortality in chicken embryos than ZIKVAS (Willard et al., 2017). Moreover, Aubry et al. (2021) observed that ZIKVAF was more pathogenic in immunocompromised adult mice, and in embryos of mice it was observed that intraplacental infection with ZIKVAF caused subcutaneous edema, high levels of infection, and more severe phenotypes, leading to death. A study showed that vertical transmission of ZIKVAF in mice leads to diminished fetal viability and viral RNA can be detected in different fetal tissues, including those related to brain/neurologic development (Vermillion et al., 2017).
Although Aubry et al. (2021) has shown ZIKVAF having higher transmissibility and pathogenicity, so far, only ZIKVAS has been related to disease and congenital anomalies in humans. Indeed, since ZIKVAF has been reported as more virulent with the potential to cause serious and unrelated outcomes to life, including miscarriages and stillbirths, its effects in humans would be harder to be identified (Masmejan et al., 2020; Aubry et al., 2021; Lambrechts, 2021). However, taking into account ZIKV neurotropic ability and vertical transmission capacity (Aubry et al., 2021; Lambrechts, 2021), we cannot rule out that new outbreaks related to ZIKVAF could increase the rate of malformations. Thus, such as apparently conflicting situation requires further investigation, mainly in areas where ZIKVAF may be circulating.
Conclusion
ZIKV has circulated for several decades with silent transmission causing sporadic outbreaks. So far, only ZIKVAS has been associated with birth defects in humans. However, experimental data have also shown the teratogenic potential of ZIKVAF. Thus, in order to prevent new human outbreaks and potentially associated birth defects, close epidemiological vigilance and identification of ZIKV lineages circulating in mosquitoes, humans, and NPHs is of high importance. The detection of ZIKVAF in mosquitoes and NHPs in a sylvatic environment in Brazil warns of the possibility of occurrence of a sylvatic cycle involving ZIKVAF. Mosquitoes and NHPs, which naturally inhabit the wild, can be easily introduced into urban and peri-urban regions, where half of the total Brazilian population resides. Additional studies and development of appropriate diagnostic assay capable of distinguishing viral lineages would be fundamental in establishing the role of such lineages in human and NHPs disease. Effective vector control programs should also be encouraged. These could aid in building a better understanding of the viral dynamics in urban and sylvatic environments, and prevent future ZIKV outbreaks. It is expected that such interventions may mitigate the impact of ZIKV on public health, avoiding disastrous outcomes, such as those of the 2016 ZIKV epidemic.
Author Contributions
SMSM, AFH, GEW, and BDR performed the writing—draft. APMV and LRF led the manuscript and performed the writing—review. LS-F and PMR provided important comments. All authors contributed to the article and approved the submitted version.
Funding
Study funded by Conselho Nacional de Desenvolvimento Científico e Tecnológico (CNPq)—Universal MCTIC/CNPq 2018 (project 424362/2018-0); and by the Fundo de Incentivo à Pesquisa e Eventos do Hospital de Clínicas de Porto Alegre (FIPE/HCPA) (Grant 2019-0649).
Conflict of Interest
The authors declare that the research was conducted in the absence of any commercial or financial relationships that could be construed as a potential conflict of interest.
Publisher’s Note
All claims expressed in this article are solely those of the authors and do not necessarily represent those of their affiliated organizations, or those of the publisher, the editors and the reviewers. Any product that may be evaluated in this article, or claim that may be made by its manufacturer, is not guaranteed or endorsed by the publisher.
Acknowledgments
The authors acknowledge the Brazilian research funding agencies Conselho Nacional de Desenvolvimento Científico e Tecnológico (CNPq), Coordenação de Aperfeiçoamento de Pessoal de Nível Superior (CAPES—Brazil), Fundação de Amparo à Pesquisa do Estado do Rio Grande do Sul (FAPERGS) and Universidade Federal do Rio Grande do Sul for students’ scholarships. LS-F and PMR are a CNPq research fellow. We thank Julia do Amaral Gomes, João Matheus Bremm for providing the epidemiological data and Fernanda Sales Luiz Vianna for discussions and assistance on epidemiological interpretation.
References
Alencar, J., de Mello, C. F., Marcondes, C. B., Guimarães, A. É., Toma, H. K., Bastos, A. Q., et al. (2021). Natural Infection and Vertical Transmission of Zika Virus in Sylvatic Mosquitoes Aedes albopictus and Haemagogus leucocelaenus from Rio de Janeiro, Brazil. Trop. Med. Infect. Dis. 6 (99), 1–10. doi: 10.3390/tropicalmed6020099
Anfasa, F., Siegers, J., van der Kroeg, M., Mumtaz, N., Stalin Raj, V., de Vrij, F., et al. (2017). Phenotypic Differences Between Asian and African Lineage Zika Viruses in Human Neural Progenitor Cells. mSphere 2 (4), 1–1. doi: 10.1128/mSphere.00292-17
Aubry, F., Jacobs, S., Darmuzey, M., Lequime, S., Delang, L., Fontaine, A., et al. (2021). Recent African Strains of Zika Virus Display Higher Transmissibility and Fetal Pathogenicity Than Asian Strains. Nat. Commun. 12 (1), 1–14. doi: 10.1038/s41467-021-21199-z
Boyer, S., Calvez, E., Chouin-Carneiro, T., Diallo, D., Failloux, A. B. (2018). An Overview of Mosquito Vectors of Zika Virus. Microbes Infect. 20, 646–660. doi: 10.1016/j.micinf.2018.01.006
Brazil Ministry of Health (2018) Monitoramento Dos Casos De Dengue, Febre De Chikungunya E Febre Pelo Vírus Zika Até a Semana Epidemiológica 52. Available at: https://antigo.saude.gov.br/images/pdf/2018/janeiro/23/Boletim-2018-001-Dengue.pdf (Accessed April, 2021).
Carvalho, R. G., Lourenço-de-Oliveira, R., Braga, I. A. (2014). Updating the Geographical Distribution and Frequency of Aedes Albopictus in Brazil With Remarks Regarding Its Range in the Americas. Memórias do Instituto Oswaldo Cruz 109 (6), 787–796. doi: 10.1590/0074-0276140304
Culot, L., Pereira, L. A., Agostini, I., de Almeida, M. A. B., Alves, R. S. C., Aximov, I., et al. (2019). Atlantic-Primates: A Dataset of Communities and Occurrences of Primates in the Atlantic Forests of South America. Ecology 100 (1). doi: 10.1002/ecy.2525
de Almeida, P. R., Ehlers, L. P., Demoliner, M., Eisen, A. K. A., Girardi, V., de Lorenzo, C., et al. (2019). Detection of a Novel African-Lineage-Like Zika Virus Naturally Infecting Freeliving Neotropical Primates in Southern Brazil. bioRxiv. doi: 10.1101/828871
de Oliveira, W. K., de França, G. V. A., Carmo, E. H., Duncan, B. B., de Souza Kuchenbecker, R., Schmidt, M. I. (2017). Infection-Related Microcephaly After the 2015 and 2016 Zika Virus Outbreaks in Brazil: A Surveillance-Based Analysis. Lancet 390 (10097), 861–870. doi: 10.1016/S0140-6736(17)31368-5
dos Santos, D. O. D., de Oliveira, A. R., de Lucena, F. P., de Mattos, S. A., de Carvalho, T. P., Costa, F. B., et al. (2020). Histopathologic Patterns and Susceptibility of Neotropical Primates Naturally Infected With Yellow Fever Virus. Vet. Pathol. 57 (5), 681–686. doi: 10.1177/0300985820941271
Faria, N. R., Quick, J., Claro, I. M., Thézé, J., De Jesus, J. G., Giovanetti, M., et al. (2017). Establishment and Cryptic Transmission of Zika Virus in Brazil and the Americas. Nature 546 (7658), 406–410. doi: 10.1038/nature2240
Favoretto, S. R., Araujo, D. B., Duarte, N. F. H., Oliveira, D. B. L., da Crus, N. G., Mesquita, F., et al. (2019). Zika Virus in Peridomestic Neotropical Primates, Northeast Brazil. Ecohealth 16 (1), 61–69. doi: 10.1007/s10393-019-01394-7
Ferguson, N. M., Cucunubá, Z. M., Dorigatti, I., Nedjati-Gilani, G. L., Donnelly, C. A., Basáñez, M. G., et al. (2016). Epidemiology. Countering the Zika Epidemic in Latin America. Science 353 (6297), 353–354. doi: 10.1126/science.aag0219
Figueiredo, L. T. M. (2019). Human Urban Arboviruses Can Infect Wild Animals and Jump to Sylvatic Maintenance Cycles in South America. Front. Cell. Infect. Microbiol. 9, 259. doi: 10.3389/fcimb.2019.00259
Gabriel, E., Ramani, A., Karow, U., Gottardo, M., Natarajan, K., Gooi, L. M., et al. (2017). Recent Zika Virus Isolates Induce Premature Differentiation of Neural Progenitors in Human Brain Organoids. Cell Stem Cell. 20, 397–406.e5. doi: 10.1016/j.stem.2016.12.005
Giovanetti, M., Faria, N. R., Lourenço, J., Goes de Jesus, J., Xavier, J., Claro, I., et al. (2020). Genomic and Epidemiological Surveillance of Zika Virus in the Amazon Region. Cell Rep. 30 (7), 2275–2283.e7. doi: 10.1016/j.celrep.2020.01.085
Han, B. A., Majumdar, S., Calmon, F. P., Glicksberg, B. S., Horesh, R., et al. (2019). Confronting Data Sparsity to Identify Potential Sources of Zika Virus Spillover Infection Among Primates. Epidemics 27, 59–65. doi: 10.1016/j.epidem.2019.01.005
Henderson, A. D., Aubry, M., Kama, M., Vanhomwegen, J., Teissier, A., Mariteragi-Helle, T., et al. (2020). Zika Seroprevalence Declines and Neutralizing Antibodies Wane in Adults Following Outbreaks in French Polynesia and Fiji. Elife 9, 1–17. doi: 10.7554/eLife.48460
Iani, F. C. M., Giovanetti, M., Fonseca, V., Souza, W. M., Adelino, T. E. R., Xavier, J., et al. (2021). Epidemiology and Evolution of Zika Virus in Minas Gerais, Southeast Brazil. Infect. Genet. Evol. 91, 104785. doi: 10.1016/j.meegid.2021.104785
Jaeger, A. S., Murrieta, R. A., Goren, L. R., Crooks, C. M., Moriarty, R. V., Weiler, A. M., et al. (2019). Zika Viruses of African and Asian Lineages Cause Fetal Harm in a Mouse Model of Vertical Transmission. PloS Negl. Trop. Dis. 13, e0007343. doi: 10.1371/journal.pntd.0007343
Kasprzykowski, J. I., Fukutani, K. F., Fabio, H., Fukutani, E. R., Costa, L. C., Andrade, B. B., et al. (2020). A Recursive Sub-Typing Screening Surveillance System Detects the Appearance of the ZIKV African Lineage in Brazil: Is There a Risk of a New Epidemic? Int. J. Infect. Dis. 96, 579–581. doi: 10.1016/j.ijid.2020.05.090
Lambrechts, L. (2021). Did Zika Virus Attenuation or Increased Virulence Lead to the Emergence of Congenital Zika Syndrome? J. Travel Med., 1–3. doi: 10.1093/jtm/taab041
Liu, J., Liu, Y., Shan, C., Nunes, B. T. D., Yun, R., Haller, S. L., et al. (2021). Role of Mutational Reversions and Fitness Restoration in Zika Virus Spread to the Americas. Nat. Commun. 12 (1), 595. doi: 10.1038/s41467-020-20747-3
Lowe, R., Barcellos, C., Brasil, P., Cruz, O. G., Honório, N. A., Kuper, H., et al. (2018). The Zika Virus Epidemic in Brazil: From Discovery to Future Implications. Int. J. Environ. Res. Public Health 15 (1)96, 1–18. doi: 10.3390/ijerph15010096
Machado, F. C., Bittar, C., Rahal, P., Calmon, M. F. (2021). Identification of Differentially Expressed miRNAs in Human Cells Infected With Different Zika Virus Strains. Arch. Virol. 166, 1681–1689. doi: 10.1007/s00705-021-05051-9
Masmejan, S., Musso, D., Vouga, M., Pomar, L., Dashraath, P., Stojanov, M., et al. (2020). Zika Virus. Pathogens 9, 898. doi: 10.3390/pathogens9110898
Moreno, E. S., Agostini, I., Holzmann, I., Di Bitetti, M. S., Oklander, L. I., et al. (2015). Yellow Fever Impact on Brown Howler Monkeys (Alouatta Guariba Clamitans) in Argentina: A Metamodelling Approach Based on Population Viability Analysis and Epidemiological Dynamics. Mem. Inst. Oswaldo Cruz Rio Janeiro 110 (7), 865–876. doi: 10.1590/0074-02760150075
Netto, E. M., Moreira-Soto, A., Pedroso, C., Hoser, C., Funk, S., Kucharski, A. J., et al. (2017). High Zika Virus Seroprevalence in Salvador, Northeastern Brazil Limits the Potential for Further Outbreaks. MBio 8 (6), 1–14. doi: 10.1128/mBio.01390-17
Nunes, B. T. D., Fontes-Garfias, C. R., Shan, C., Muruato, A. E., Nunes, J. G. C., Burbano, R. M. R., et al. (2020). Zika Structural Genes Determine the Virulence of African and Asian Lineages. Emerg. Microbes Infect. 9 (1), 1023–1033. doi: 10.1080/22221751.2020.1753583
Pan American Health Organization/World Health Organization (2017) Zika - Epidemiological Report Brazil. March 2017 (Washington, D.C: PAHO/WHO). Available at: http://paho.org/hq/dmdocuments/2017/2017-phe-zika-situation-report-bra.pdf (Accessed April, 2021).
Shao, Q., Herrlinger, S., Zhu, Y. N., Yang, M., Goodfellow, F., Stice, S. L., et al. (2017). The African Zika Virus MR-766 Is More Virulent and Causes More Severe Brain Damage Than Current Asian Lineage and Dengue Virus. Development 144 (22), 4114–4124. doi: 10.1242/dev.156752
Sheridan, M. A., Balaraman, V., Schust, D. J., Ezashi, T., Michael Roberts, R., Franz, A. W. E. (2018). African and Asian Strains of Zika Virus Differ in Their Ability to Infect and Lyse Primitive Human Placental Trophoblast. PloS One 13 (7), 1–18. doi: 10.1371/journal.pone.0200086
Simonin, Y., Loustalot, F., Desmetz, C., Foulongne, V., Constant, O., Fournier-Wirth, C., et al. (2016). Zika Virus Strains Potentially Display Different Infectious Profiles in Human Neural Cells. EBioMedicine 12, 161–169. doi: 10.1016/j.ebiom.2016.09.020
Smith, D. R., Sprague, T. R., Hollidge, B. S., Valdez, S. M., Padilla, S. L., Bellanca, S. A., et al. (2018). African and Asian Zika Virus Isolates Display Phenotypic Differences Both In Vitro and In Vivo. Am. J. Trop. Med. Hyg. 98 (2), 432–444. doi: 10.4269/ajtmh.17-0685
Tripathi, S., Balasubramaniam, V. R. M. T., Brown, J. A., Mena, I., Grant, A., Bardina, S. V., et al. (2017). A Novel Zika Virus Mouse Model Reveals Strain Specific Differences in Virus Pathogenesis and Host Inflammatory Immune Responses. PloS Pathog. 13 (3), 1–19. doi: 10.1371/journal.ppat.1006258
Udenze, D., Trus, I., Berube, N., Gerdts, V., Karniychuk, U. (2019). The African Strain of Zika Virus Causes More Severe In Utero Infection Than Asian Strain in a Porcine Fetal Transmission Model. Emerging Microbes Infections 1, 1098–1107. doi: 10.1080/22221751.2019.1644967
Vazeille, M., Mousson, L., Martin, E., Failloux, A. B. (2010). Orally Co-Infected Aedes Albopictus From La Reunion Island, Indian Ocean, can Deliver Both Dengue and Chikungunya Infectious Viral Particles in Their Saliva. PloS Negl. Trop. Dis. 4 (6), e706. doi: 10.1371/journal.pntd.0000706
Vermillion, M. S., Lei, J., Shabi, Y., Baxter, V. K., Crilly, N. P., McLane, M., et al. (2017). Intrauterine Zika Virus Infection of Pregnant Immunocompetent Mice Models Transplacental Transmission and Adverse Perinatal Outcomes. Nat. Commun. 8, 1–14. doi: 10.1038/ncomms14575
Keywords: Congenital Zika Syndrome, epidemic, teratogenesis, animal models, viral emergence, birth defects
Citation: de Matos SMS, Hennigen AF, Wachholz GE, Rengel BD, Schuler-Faccini L, Roehe PM, Varela APM and Fraga LR (2021) Possible Emergence of Zika Virus of African Lineage in Brazil and the Risk for New Outbreaks. Front. Cell. Infect. Microbiol. 11:680025. doi: 10.3389/fcimb.2021.680025
Received: 12 March 2021; Accepted: 25 May 2021;
Published: 23 July 2021.
Edited by:
Jeremy V. Camp, Medical University of Vienna, AustriaReviewed by:
Philippe Desprès, Université de la Réunion, FranceFabien Aubry, UPR9022 Réponse immunitaire et développement chez les insectes (RIDI), France
Copyright © 2021 de Matos, Hennigen, Wachholz, Rengel, Schuler-Faccini, Roehe, Varela and Fraga. This is an open-access article distributed under the terms of the Creative Commons Attribution License (CC BY). The use, distribution or reproduction in other forums is permitted, provided the original author(s) and the copyright owner(s) are credited and that the original publication in this journal is cited, in accordance with accepted academic practice. No use, distribution or reproduction is permitted which does not comply with these terms.
*Correspondence: Lucas Rosa Fraga, lrfraga@ufrgs.br; Ana Paula Muterle Varela, anapaulamut@gmail.com
†These authors have contributed equally to this work and share first authorship
‡These authors have contributed equally to this work and share last authorship