Ixodes ricinus ticks have a functional association with Midichloria mitochondrii
- 1Institute of Parasitology, Biology Centre of the Czech Academy of Sciences, České Budějovice, Czechia
- 2Faculty of Science, University of South Bohemia, Ceske Budejovice, Czechia
- 3CEITEC, University of Veterinary Sciences, Brno, Czechia
- 4Department of Microbiology, Nutrition and Dietetics/CINeZ, Czech University of Life Sciences, Prague, Czechia
- 5Department of Chemistry and Biochemistry, Mendel University, Brno, Czechia
In addition to being vectors of pathogenic bacteria, ticks also harbor intracellular bacteria that associate with ticks over generations, aka symbionts. The biological significance of such bacterial symbiosis has been described in several tick species but its function in Ixodes ricinus is not understood. We have previously shown that I. ricinus ticks are primarily inhabited by a single species of symbiont, Midichloria mitochondrii, an intracellular bacterium that resides and reproduces mainly in the mitochondria of ovaries of fully engorged I. ricinus females. To study the functional integration of M. mitochondrii into the biology of I. ricinus, an M. mitochondrii-depleted model of I. ricinus ticks was sought. Various techniques have been described in the literature to achieve dysbiosed or apo-symbiotic ticks with various degrees of success. To address the lack of a standardized experimental procedure for the production of apo-symbiotic ticks, we present here an approach utilizing the ex vivo membrane blood feeding system. In order to deplete M. mitochondrii from ovaries, we supplemented dietary blood with tetracycline. We noted, however, that the use of tetracycline caused immediate toxicity in ticks, caused by impairment of mitochondrial proteosynthesis. To overcome the tetracycline-mediated off-target effect, we established a protocol that leads to the production of an apo-symbiotic strain of I. ricinus, which can be sustained in subsequent generations. In two generations following tetracycline administration and tetracycline-mediated symbiont reduction, M. mitochondrii was gradually eliminated from the lineage. Larvae hatched from eggs laid by such M. mitochondrii-free females repeatedly performed poorly during blood-feeding, while the nymphs and adults performed similarly to controls. These data indicate that M. mitochondrii represents an integral component of tick ovarian tissue, and when absent, results in the formation of substandard larvae with reduced capacity to blood-feed.
1. Highlights
● ex vivo membrane feeding of ticks is a powerful tool for the production of apo-symbiotic ticks
● Midichloria mitochondrii-free I. ricinus females produce larvae that are less successful during blood-feeding
● use of tetracycline shows off-target effects on proteosynthesis in tick mitochondria
2. Introduction
The co-existence of microbiota and eukaryotic hosts underpins much of the complexity of the natural world and experimental studies demonstrate that these associations confer benefits to the hosting eukaryote (Douglas, 2014). Arthropods, too, commonly display stable associations with microbial symbionts and rarely exist as symbiont-independent entities (Hammer et al., 2019). The wide distribution of microbial-arthropod associations often results in a nutritional advantage (synthesis or digestion) to the host (Douglas, 2009) and symbiont-dependent species have often evolved specialized tissues or organs with micro-niches where the microbiota flourish (Douglas, 2020). Such microbial association is often essential only at a specific point in ontogeny, e.g. Daphnia egg (Mushegian et al., 2018).
Experimental axenic or dysbiosed variants of blood-feeding arthropod species have been instrumental in understanding the functional integration of microbes into the physiology of their hosts (Narasimhan et al., 2014; Romoli et al., 2021; Gilliland et al., 2022). While mosquitoes are inhabited by a substantial amount of bacterial biomass, its functional significance in mosquito physiology and development appears to be minor, if any, under the conditions examined (Steven et al., 2021). Ticks, on the other hand, associate with very few, probably transient, microbes in the digestive tract (Ross et al., 2018; Guizzo et al., 2020) but generally harbor ovary-resident bacterial symbionts (Bonnet et al., 2017; Buysse et al., 2021). The functional significance of the microbiota in tick physiology and development has typically been investigated using antibiotic treatment (Duron et al., 2018; Zhong et al., 2021).
Midichloria mitochondrii is the dominant tick-associated bacterium (Epis et al., 2008), and was originally reported to be associated with 100% of Ixodes ricnus wild-caught females (Lo et al., 2006), although it now appears that the symbiont species differs geographically across Europe (Krawczyk et al., 2022). Midichloria mitochondrii occupies primarily the mitochondrial inter-membrane space in ovarian cells (Buysse and Duron, 2018), where it multiplies upon the tick engorging a blood meal (Sassera et al., 2008). Transmission electron microscopy images and mathematical simulation, however, did not support predatory behavior of M. mitochondrii on I. ricinus mitochondria (Comandatore et al., 2021), contrasting with the original assumption (Sacchi et al., 2004). If M. mitochondrii is not predatory in nature, its function or benefit might be sought. In I. ricinus, however, there are no established protocols for the production of apo-symbiotic ticks depleted of M. mitochondrii, which would be key for obtaining functional data on M. mitochondrii - I. ricinus symbiosis.
To bridge this gap, we have established such a protocol using artificial ex vivo blood membrane feeding of I. ricinus. We show that oral antibiotic administration is clearly superior to micro-injection, being able to profoundly reduce levels of M. mitochondrii in generation zero. In generations subsequent to tetracycline administration, a complete elimination of M. mitochondrii was achieved in the lineage but the larvae produced from these apo-symbiotic females have a reduced fitness, pointing towards a functional significance of M. mitochondrii.
3. Materials and methods
3.1. Tick rearing
Wild-caught unfed I. ricinus females were collected by flagging around Ceske Budejovice, Czech Republic. All tick life stages were maintained under controlled conditions (temperature: 24°C and humidity: 95%) in the rearing facility at the Institute of Parasitology, Biology Centre of the Czech Academy of Sciences. All laboratory animals were treated in accordance with the Animal Protection Law of the Czech Republic No. 246/1992 Sb., ethics approval No. 25/2018. The study was approved by the Institute of Parasitology, Biology Centre CAS and Central Committee for Animal Welfare, Czech Republic (Protocol No. 1/2015).
3.2. Generation of Midichloria mitochondrii-free ticks by ex vivo membrane blood feeding
Wild-caught Ixodes ricinus adult females were fed defibrinated bovine blood via an ex vivo membrane feeding system (Kröber and Guerin, 2007). Every 12 hours for a period of 10 days, the feeding chambers were washed with a multipurpose disinfectant, 0.5% (w/v) Virkon S (Chemours), followed by a sterile solution NaCl (0.9%) and placed in clean cell culture plate-wells filled with 3.1 mL of fresh blood supplemented with 50 µg/mL or 5 µg/mL tetracycline (Sigma-Aldrich: T3383). The antibiotic low- and high-dosage is very similar to the previously published amounts of antibiotics used for blood meal supplementation (Oliver et al., 2021). These working concentrations were obtained by 1000 × dilution from their stock solutions prepared in DMSO. The blood was freshly supplemented with every 12h blood exchange regime. Individual I. ricinus females were thus dosed with 100 µmol or 10 µmol of tetracycline, respectively, assuming a 1mL uptake of blood meal. Fully fed females that spontaneously detached from the membrane were randomly placed into two groups: in one of these, the females had their ovaries dissected out for DNA isolation, and in the other, the females were placed individually into glass vials kept at 24°C and 95% humidity to allow egg laying and subsequent hatching of larvae. The first generation was initiated by placing 15 mg of unfed I. ricinus larvae from M. mitochondrii-reduced (from I. ricinus females dosed with 100 µmol of tetracycline) and control females on guinea pigs, and the second generation was initiated by placing 20 mg of unfed I. ricinus larvae from M. mitochondrii-free and control females. For comparison, wild-caught I. ricinus females were fed naturally on laboratory guinea pigs, and freshly engorged females were micro-injected into the haemoceol with 350 nL of tetracycline, which was solubilized in MilliQ H2O as 150 mg/mL, 15 mg/mL, or 1.5 mg/mL stock solutions. Individual I. ricinus females were thus dosed with 112 nmol, 11.2 nmol, and 1.12 nmol of tetracycline, respectively.
3.3. Evaluation of tick fitness by natural feeding on a host
Larvae, nymphs and adult ticks from control and Midichloria-free groups were naturally fed to repletion on guinea pigs in separated compartments. Control ticks used in this study originated from the progeny of wild-caught adult females ticks naturally fed on lab-reared hosts. Guinea pigs were examined once a day for the presence of fully fed ticks. Fully fed larvae and nymphs were placed in glass vials to molt to the following life stage. Fully engorged females were allowed to perform egg laying and subsequent hatching of larvae. Larval engorgement was calculated by the number of larvae that fully engorged and dropped off the host. Nymphal and adult female engorgement successes were evaluated by the percentage of ticks that fed until repletion, detaching spontaneously from the host. Nymphal and adult molting successes were calculated by the percentage of nymphs and adults molted from engorged larva and nymphs, respectively.
3.4. Genomic DNA isolation and quantification of Midichloria mitochondrii
Total DNA was isolated from either dissected ovary (from individual adult females under sterile PBS), eggs (n = 30), or the whole tick at different life stages using the PowerSoil Kit (Mobio) according to the manufacturer’s instructions. DNA was eluted in 100 μL with concentrations ranging from 1.8 ng/μL to 14.7 ng/μL. Midichloria mitochondrii was quantified as described by (Sassera et al., 2008). The real-time PCR reaction mixture contained 2 μL of DNA, 10 μL of SYBR green (Roche, 4913914001), 1 μL of each primer (10 pmol) (F: 5’CTTGAGAGCAGAACCACCTA 3’; R: 5’CAAGCTCTGCC GAAATATCTT 3’) and DNA-free water (Top-Bio, Praha, Czech Republic) to 20 μL. The I. ricinus single-copy gene elongation factor-1α (ef-1α; ID: GU074769.1) was used as a reference for data normalization and also as a control for the DNA isolation procedure (F: 5’ACGAGGCTCTGACGGAAG 3’; R: 5’CACGACGCAACTCCTTCAC 3’). The reaction was carried out in a QuantStudio 6 qPCR instrument (Applied Biosystems) with 50 cycles at 95 °CC (10 s), 60 °CC (10 s), and 72 °CC (10 s) following an initial denaturation at 95 °CC (10 min). The samples were considered free of M. mitochondrii when no specific amplification of the bacterium could be detected within 50 cycles.
3.5. Medium preparation and cell cultures
The I. ricinus embryonic cell line (IRE/CTVM19) (Bell-Sakyi et al., 2007) was propagated in Leibovitz L-15 medium (Biosera, LM-L1050), supplemented with 20% (v/v) human serum (Sigma- Aldrich, H4522), Tryptose Phosphate Broth (10% w/v) (Sigma-Aldrich, T8159), Antibiotic and Antimycotic (1% w/v; Biosera XC-A4110), and L-glutamine (1% w/v; Biowest, X0550). To test the sensitivity of tick mitochondrial proteosynthesis to individual antibiotics, 6mL cell cultures (6 × 105 cells/mL media) were transferred to a medium without the antibiotic and antimycotic supplement and were instead supplemented with 400 µg/mL (final concentration) of tetracycline, chloramphenicol (Sigma-Aldrich: C0378), or gentamicin (Sigma-Aldrich: G1914) according to (Moullan et al., 2015). Cells were cultured for 72 hours and then centrifuged at 160 × g for 5 min, rinsed with 1× phosphate-buffered saline (PBS), and again centrifuged at 160 × g for 5 min. Cellular pellets were homogenized and proteins were solubilized in 500 µL of 1× PBS pH 7.4, 1mM EDTA, 1µM E-64 (Merck: E3132) by three freeze-thaw cycles in liquid nitrogen. The homogenate was clarified by centrifuging at 10 000 × g, 10 min, 4°C. The consequent soluble fraction was discarded and the membrane protein-enriched pellets were solubilized in reducing Laemmli buffer.
3.6. SDS-PAGE and western blotting
Ovaries from fully engorged I. ricinus females were dissected under DEPC-treated PBS. Three individual ovaries were used for RNA extraction and three individual ovaries were homogenized and used for protein solubilization as described above. Protein homogenates of tick ovarian tissue or embryonic cell line were adjusted to the equal protein load and separated by reducing SDS-PAGE in 4–20% Stain-free gels (BioRad: 4568095). Protein separation was visualized in the stain-free mode and proteins were transferred to 0.2µm pore PVDF membrane (BioRad: 1704272) for Western blotting. The membrane was blocked in non-fat milk suspended in PBS-Tween for 1 hour at room temperature. Primary antibodies anti-ATP5A (Abcam: ab14748) and anti-MTCO1(Thermo Fisher Scientific: 459600) were diluted in PBS-Tween 1:500 and 1:1000, respectively, and incubated with the membrane overnight. Immunodetection was performed by anti-mouse peroxidase-conjugated immunoglobulin (Sigma-Aldrich: A9044), diluted 1: 10 000 in PBS-Tween, and the signal was visualized by Clarity™ Western ECL substrate (BioRad: 170-5061) using ChemiDoc (BioRad).
3.7. RNA extraction, cDNA synthesis, dsRNA synthesis, and RNAi evaluation by RT-qPCR
RNA was extracted from ovaries using a NucleoSpinRNA II kit (Macherey-Nagel, Germany). The quality of RNA was checked by gel electrophoresis on an agarose gel (1%, w/vol), and stored at -80 °C prior to cDNA synthesis. cDNA preparations were made from 0.5 μg of total RNA in independent triplicates using the Transcriptor High-Fidelity cDNA Synthesis Kit (Roche Diagnostics, Germany). The cDNA served as templates for subsequent quantitative expression analyses by RT-qPCR. Samples were analyzed by a LightCycler 480 (Roche) using Fast Start Universal SYBR Green Master Kit (Roche). Relative expressions were calculated using the ΔΔCt method (Livak and Schmittgen, 2001). The expression profiles were normalized to I. ricinus elongation factor 1α (ef-1α; ID: GU074769.1). Primers used were: ir-atp5A (F: 5’GCTACCTGGACAAGTTGGACC 3’, R: 5’CTTCTGTGTCGACCTGATGTG 3’), ir-mtco1 (F: 5’GTTTTTGATTATTACCCCCTTCTTT 3’, R: 5’ACTGTTCATCCAGTTCCTGCC 3’).
The target sequence for RNAi was obtained by PCR using the following primers: ir-atp5A (F: 5’ atgggccCGACAGGCAGACTGGTAAG 3’, R: 5’ attctaGAGAGATCGTCGTAGATGATAAG 3’), ir-mtco1 (F: 5’atgggccCTCTTCCTGTTCTTGCTGGAG 3’, R: 5’attctagaCTCGAGCTTACTTTACAGCTGC 3’). dsRNA of ir-atp5A (GenBank: GIYG01000932.1), ir-mtco1 (GIXL01030069.1), or gfp (green fluorescent protein) used as a control were synthesized using the MEGAscript T7 transcription kit (Ambion, Lithuania) according to the previously described protocol (Hajdusek et al., 2009; Perner et al., 2018). This resulted in a synthesis and hybridization of dsRNA products of 309 bp (ir-atp5a) and 331 bp (ir-mtco1). I. ricinus females were injected into the haemocoel through to the coxae with these dsRNAs (0.5 μL; 3 μg/μL) or control gfp dsRNA (0.5 μL; 3 μg/μL) using a glass capilary of the outer dimater of 20 – 25 µm and a microinjector (Narishige), allowed to rest for one day and then fed naturally for 7 days on guinea pigs. The efficiency of RNA-mediated silencing of ir-atp5A gene expression was verified both at the transcript level by RT-qPCR and at the protein level by Western blot analysis using αATP5A antibodies. In this way, we validated specificity of commercial antibodies against non-tick immunogen to recognize its tick homologue.
3.8. Statistical analysis
Statistical analyses were performed using GraphPad Prism software (version 8). Differences in DNA amplicon abundances, tick engorged weights, or weights of egg clutches were calculated using the t-test. The values were considered statistically significant with p<0.05 denoted as *, p<0.005 denoted as **, and p<0.0001 denoted as ****.
4. Results
4.1. Reduction of Midichloria mitochondrii abundance in Ixodes ricinus ovaries is achieved by feeding ticks tetracycline during ex vivo membrane blood feeding
We previously determined that ovaries of I. ricinus females were virtually exclusively colonized by a single bacterial species, M. mitochondrii (Guizzo et al., 2020). As a proxy for the effectiveness of bacterial clearance from fully engorged I. ricinus females, we determined the relative amounts of M. mitochondrii in tick ovaries (Figure 1A). First, we performed a one-time microinjection of fully engorged I. ricinus females with tetracycline in a concentration series, and quantified levels of M. mitochondrii three and six days after feeding (and injection). At both time-points, there was no reduction in levels of M. mitochondrii in tick ovaries (Figure 1B). To increase the overall dosage of tetracycline achieved in ticks, we performed ex vivo tick membrane blood feeding supplemented with two different concentrations (50 µg/mL and 5 µg/mL; final concentrations) of tetracycline. With this approach, we achieved a significant reduction in M. mitochondrii levels in tick ovaries, three and six days after engorgement (Figure 1C). We noted, however, that at higher concentration of tetracycline, these I. ricinus females were less successful than control ticks in their capacity to fully engorge (see below).
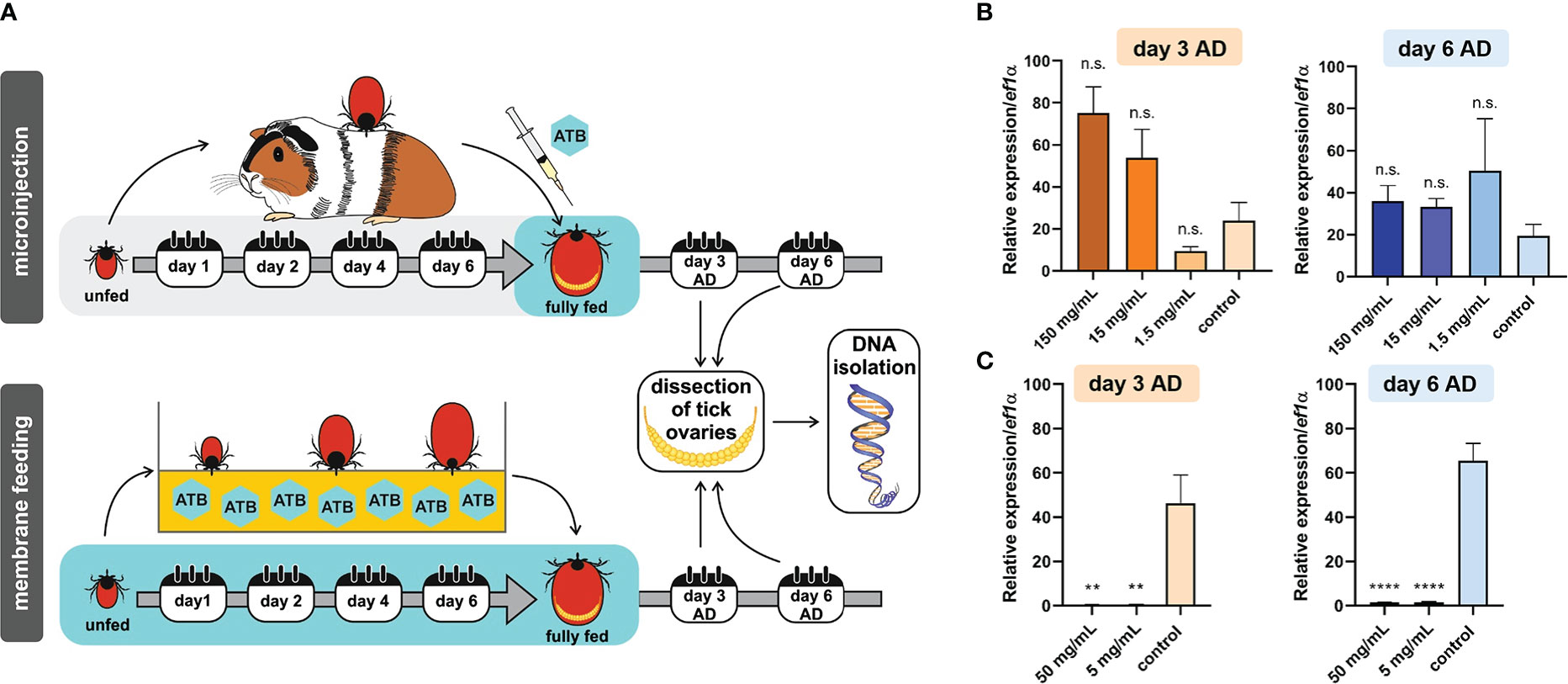
Figure 1 (A) Scheme of tetracycline antibiotics (ATB) administration (microinjection or ex vivo membrane feeding) and a timeline of DNA extraction from tick ovaries after tick detachment (AD). (B) Microinjection. Real-time PCR on DNA extracts from individual tick ovaries determining the levels of Mitochondria mitochondrii upon tetracycline microinjection into hemolymph of Ixodes ricinus females fully engorged in vivo. (C) Membrane feeding. Real-time PCR on DNA extracts from individual tick ovaries determining the levels of M. mitochondrii upon tetracycline supplementation of blood meal during ex vivo membrane feeding of I. ricinus ticks. (B, C) Stock concentrations of tetracycline are shown. Mean and SEM are shown, (B) n = 3 ticks, (C) n ≥ 9 ticks. T-test analysis: **p < 0.005; ****p < 0.0001; n.s., not significant.
4.2. Tetracycline inhibits proteosynthesis in tick mitochondria
In order to evaluate the potential of off-target tetracycline activity to inhibit mitochondrial proteosynthesis, as reported in other eukaryotes (Moullan et al., 2015), we designed a Western blot assay detecting two mitochondrial proteins; one encoded by the nuclear genome and the other by the mitochondrial genome. ATP synthase F1 subunit alpha (ATP5A) is encoded by the nuclear genome, is translated in the cytosol, and only then is transported into the mitochondria, while the Mitochondrially Encoded Cytochrome C Oxidase I (MTCO1) is encoded in the genome of mitochondria, where it is also synthesized by the mitochondrial-based translation apparatus (Figure 2A). Upon specificity validation of the commercial antibodies against non-tick homologues (Figure 2B), we noted that tetracycline treatment reduced the levels of MTCO1 in tick mitochondria while the levels of ATP5A remained unchanged (Figure 2C). These data show that tetracycline inhibited tick mitochondrial proteosynthesis and thus may elicit activity affecting tick fitness. Using RNAi, we could only validate specificity against ATP5A, as we could not achieve RNAi silencing for the mitochondrial protein MTCO1 (Supplementary Figure S1). Instead, we confirmed the anti-MTCO1 specificity of the commercial antibodies using chloramphenicol as a specific inhibitor of mitochondrial proteosynthesis (Richter et al., 2019) (Figure 2C).
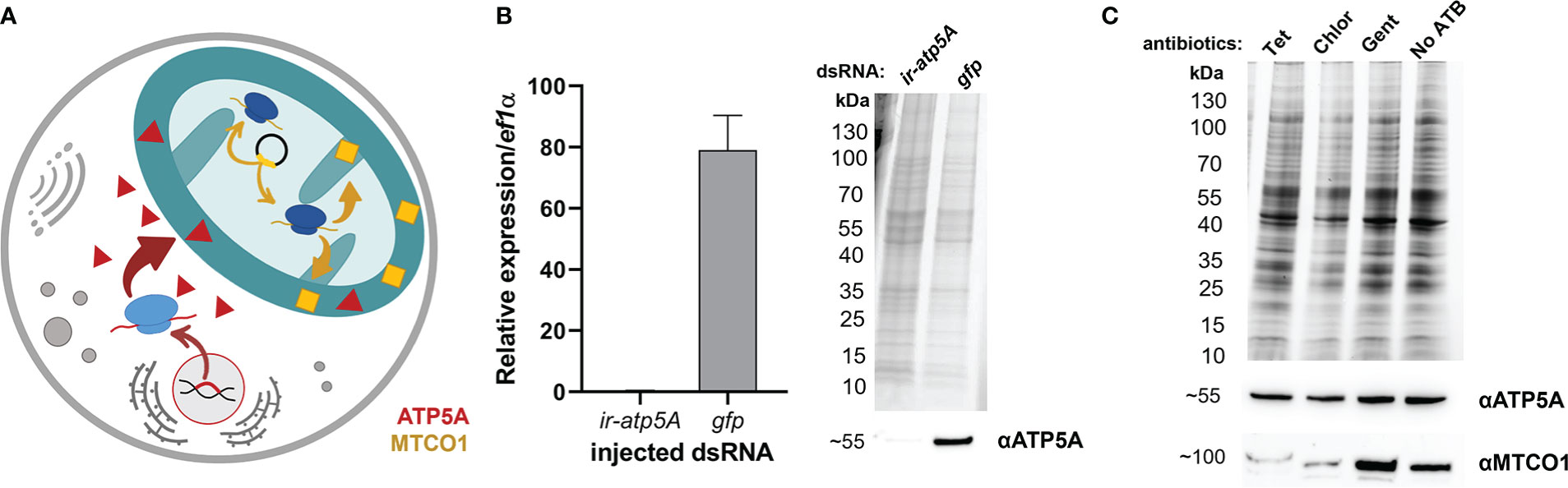
Figure 2 (A) A scheme depicting two distinct genomic origins of mitochondrial proteins, one from the nuclear gene (red) and one from mitochondria (yellow). (B) Validation of anti-ATP5A antibody by transcript knockdown of ir-atp5a using RNAi. RT-qPCR on cDNA from ovaries (left) and Western blotting on protein homogenates of ovaries (right) from fully engorged I. ricinus females are shown. (C) Western blot analysis detecting two mitochondrial proteins ATP synthase subunit alpha (ATP5A) and Mitochondrially Encoded Cytochrome C Oxidase I (MTCO1) in a homogenate of tick cells. Tet, Tetracyclin; Chlor, Chloramphenicol; Gent, Gentamicin; No ATB, no antibiotics. (B, C) Proteins were separated using reducing SDS-PAGE and visualized by the stain-free system (BioRad). Below, western blot analyses are shown using commercial antibodies against ATP5A (αATP5A) and MTCO1 (αMTCO1).
4.3. An ex vivo membrane platform for tick blood feeding as a tool for generation of a Midichloria mitochondrii-free strain of I. ricinus
In order to avoid possible immediate mitochondrial toxicity of tetracycline, we set out to produce a strain of I. ricinus ticks that would be distanced in time from the administered tetracycline (Figure 3A). Ixodes ricinus females were fed tetracycline-supplemented blood meal (Figure 3B) via the ex vivo membrane feeding system, and the larvae were used as starting progenies for the M. mitochondrii-free strain. These progenies were monitored for two following generations and assessed for their capacity to blood-feed, develop, and reproduce. In a generation subsequent to adult bacterial clearance by feeding, larvae exhibited a reduced capacity to engorge in vivo on laboratory animals. Engorgement was reduced to 57% in the first generation and to 61% in the second generation (Figure 3C). The failure of the cohort to normally blood-feed was manifested in profoundly reduced numbers of larvae reaching engorgement in 48 hours (Supplementary Figure S2). Larvae that fed successfully then developed into nymphs with a rate similar to that of the control group (Figure 3D). Furthermore, nymphs also displayed no deviation from controls (Figures 3E, F). While we observed an impairment of females during the clearance feeding itself (Figure 3B) with a longer time to engorge and lower engorged weight, we did not observe any reduced capacity of adult females to blood-feed in the generation after clearance (Figures 3G, H), strongly suggesting an off-target effect of the higher dose of tetracycline. The egg mass produced by M. mitochondrii-free and control groups of the second generation of I. ricinus females were not statistically significant either (Figure 3I). The extent of reduction of M. mitochondrii levels through the two generations cycle was monitored by qPCR (Supplementary Figure S3). These data clearly indicated that the use of tetracycline in the ex vivo blood feeding system led to an immediate reduction in M. mitochondrii in this parenting generation (F0), but eventually reached complete elimination (i.e. beyond the limits of real time PCR detection) of M. mitochondrii in the following generation of I. ricinus females (F1) (Supplementary Figure S3). These data show a clear correlation between the partial failure of larvae to blood-feed and the M. mitochondrii-free status of their female ancestor. The causative link, however, still remains to be unambiguously determined.
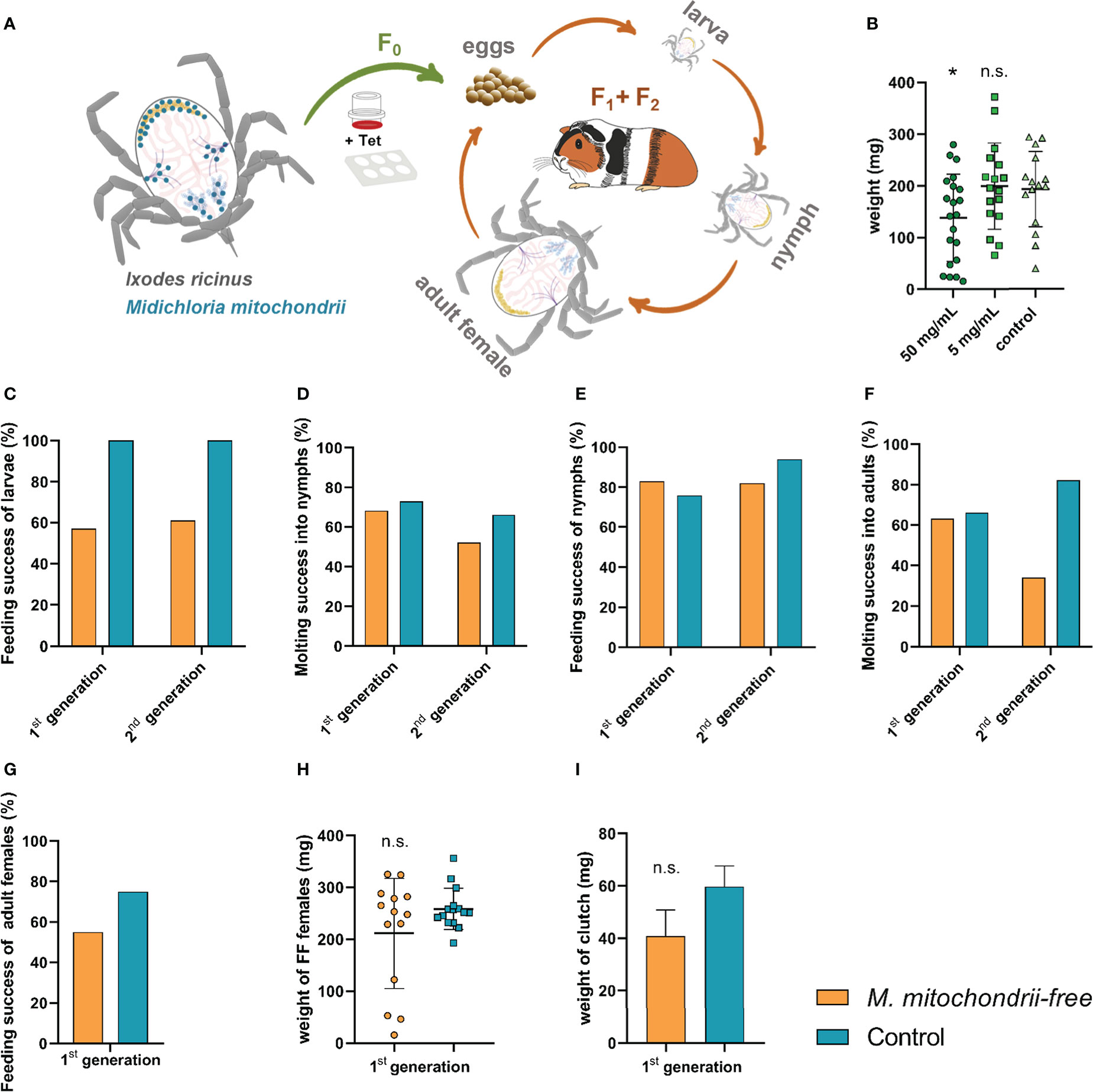
Figure 3 Effect of the absence of Midichloria mitochondrii on tick engorgement and development across two generations. (A) A scheme depicting the production of M. mitochondrii-free strain of I. ricinus ticks. The ex vivo membrane feeding is indicated by a feeder and a 6-well plate; Tet, tetracycline. The green arrow indicates a reduction in M. mitochondrii levels in generation zero, while the orange arrows indicate the propagation of apo-symbiotic I.ricinus lineage upon M. mitochondrii elimination. The blue dots in the cartoon of unfed I. ricinus female (top left) indicate tissue tropism of M. midichloria, according to (Olivieri et al., 2019). (B) Weights of fully engorged I. ricinus females upon blood feeding tetracycline-supplemented blood meal via ex vivo membrane feeding. Stock concentrations of tetracycline are shown. Mean and SEM are shown, n ≥ 15; *p < 0.05; n.s., not significant. (C) Success feeding rate of M. mitochondrii-free larvae in two generations subsequent to adult I. ricinus clearance. (D) Molting success of these larvae into nymphs. (E) Feeding success rate of M. mitochondrii-free nymphs in two generations subsequent to adult I. ricinus clearance. (F) Molting success of these nymphs into adults. (G) Feeding success rate of M. mitochondrii-free adults in a generation subsequent to adult I. ricinus clearance. (H) Weights of these M. mitochondrii-free I. ricinus adult females upon in vivo engorgement. (I) Weights of egg clutches laid by 1st generation M. mitochondrii-free I. ricinus females. (B, H, I) Mean and SEM are shown. Original numbers to the bar graphs are shown in Supplementary Table S1.
5. Discussion
The increasing number of studies on the diversity and quantity of tick microbiota starts creating a distinct sub-field of tick biology. Functional studies stemming from experimental models of ticks with manipulated inner bacterial ecosystems only start to appear, keeping pace with other symbiotic systems (see below). In mosquito research, two main protocols have been developed describing the development of axenic mosquitoes: (i) the use of disinfectants on oviposited eggs (Coon et al., 2014; Correa et al., 2018) and (ii) using a transient colonization/decolonization approach (Romoli et al., 2021). It has been demonstrated that axenic mosquitoes do not show any developmental retardation, reach the same size as controls (Correa et al., 2018; Romoli et al., 2021), and can therefore be reared under axenic conditions for multiple generations, similarly to mice and fruit flies (Steven et al., 2021).
In this study, we used two different administrations of tetracycline, the antibiotic that has been used in previous reports of successful elimination of other Gram-negative intracellular symbionts in several tick species (Zhong et al., 2007; Guizzo et al., 2017; Zhong et al., 2021). While we, and others (Ninio et al., 2015), failed to reduce M. mitochondrii loads in I. ricinus ticks through tetracycline micro-injection, both microinjection as well as membrane feeding of I. scapularis ticks with ciprofloxacin was effective in depleting or eliminating Rickettsia buchneri (Oliver et al., 2021). We thus believe that the membrane feeding platform, which is available for multiple species of hard ticks (Gonzalez et al., 2021), represents a universal and powerful tool for the production of apo-symbiotic ticks.
Midichloria mitochondrii is the dominant bacterium in ovaries of fully engorged I. ricinus females (Guizzo et al., 2020) and it is vertically transmitted from the parent to the offspring (Sassera et al., 2006). Therefore, a reduction in this bacterium in the tick ovaries enabled us to produce a following generation of I. ricinus in which M. mitochondrii was eliminated, while minimizing the confounding off-target effect of tetracycline. This allowed us to determine the effect of tick endogenous bacteria on development and fitness of its host I. ricinus. We found that larval feeding success was equally negatively impacted in both the first and second generations of bacteria-free I. ricinus ticks. These data are in line with those of the obligate symbionts Coxiella sp. in Rhipicephalus microplus (Guizzo et al., 2017) and Francisella sp. in Ornithodoros moubata (Duron et al., 2018). In these cases, bacterial elimination caused an interruption in the regular course of tick development in the short-term, where it impaired the feeding of nymphs of O. moubata, or it stopped feeding and development at the nymphal stage of R. microplus. Larval feeding success was also inhibited in Rhipicephalus sanguineus when Coxiella sp. was eliminated (Ben-Yosef et al., 2020). In R. microplus, the comparative transcriptomic analysis of Coxiella-free nymphs revealed a substantial under-expression of tick transcripts involved in their blood-feeding capacity (Guizzo et al., 2022).
The functional aspects of the bacterial microbiota of blood-feeding arthropods, integrating their metabolic products with their physiology, has started to emerge, as reviewed by (Song et al., 2022). The metabolic interaction between bacteria and ticks, with a distinct impact on development and/or physiology, seems to be very species-specific (Duron et al., 2018; Zhong et al., 2021). In addition, the methods used across the studies are not unified, which prevents us formulating general statements on this topic. Overall, the administration of antibiotics has been applied to manipulate bacterial levels and/or diversity in order to study the role of bacteria in tick biology (Narasimhan et al., 2014; Guizzo et al., 2017; Zhang et al., 2017; Duron et al., 2018; Zhong et al., 2021). These reports mainly comprise microinjection into the tick haemocoel, administration of antibiotics into vertebrate hosts, or ex vivo membrane blood feeding as tools to effectively deliver antibiotics into ticks and thus disturb the microbial community. A wide range of negative impacts on tick fitness has been reported as a result of disturbance in the abundance of Coxiella sp. and Francisella sp. in their tick hosts (Zhong et al., 2007; Guizzo et al., 2017; Zhang et al., 2017; Duron et al., 2018; Ben-Yosef et al., 2020), thus indicating a functional interaction between maternally-inherited bacteria and their tick hosts. Having said that, we are far from a full appreciation of the functional derivation of bacterial symbiosis and integration of bacterial metabolites into physiology of ticks. For a successful endosymbiosis to occur in nature, a flux of carbon and nitrogen in the form of amino acids (Esser et al., 2019), which represent the “molecular currency” providing the housing eukaryote a benefit. The pilot study in identifying such molecular currency in tick host symbiosis was recently published, demonstrating the key involvement of Coxiella-produced chorismate in the metabolism of amino acids (tryptophan) of the Haemophysalis longicornis ticks (Zhong et al., 2021).
Altogether, our data suggest a functional integration of bacterial symbiosis, most likely with M. mitochondrii, into developmental and physiological processes of I. ricinus ticks. With M. mitochondrii being unculturable, it is technically not feasible to rescue the apo-symbiotic I. ricinus females with the bacterium to restore the physiology of maternal ovaries and thus the production of fully fit larvae. This represents a clear limitation of our study. The fact that we cannot link the observed phenotype of slow larval feeding and the overall lower feeding success rate of larvae (from tetracycline-treated ancestors) with M. mitochondrii leaves open the possibility of the significance of another bacterial species or bacterial mass regardless of the bacterial taxon. Having said that, the maternal inheritance of M. mitochondrii, may qualify this bacterium as a prime bacterial entity candidate, facilitating the formation of more successful larvae. Another interpretation of our data might be that the functional association is not key during embryogenesis and larval formation, but that the functional association with bacteria occurs in tick larvae themselves. On another technical note, the ability of tetracycline to inhibit proteosynthesis in tick mitochondria warrants caution. We advise to only use tetracycline as a means of producing apo-symbiotic strains of ticks but discourage using it as an anti-bacterial agent for phenotypisation of the organism immediately after receiving tetracycline treatment. We argue that the trans-stadial and trans-generational distance between the use of tetracycline and phenotypisation legitimizes its use.
Data availability statement
The datasets presented in this study can be found in online repositories. The names of the repository/repositories and accession number(s) can be found in the article/Supplementary material.
Author contributions
MG, conceived the study, overseen data analysis, perfomed experiments, and wrote a first manuscript draft. TH, performed experiments, analyzed data, and prepared figures. HF, performed experiments. LZ, edited manuscript and funding acquisition PK, edited manuscript, funding acquisition, and data analysis. JP, conceptualized the work, wrote the final manuscript version, and funding acquisition. All authors contributed to the article and approved the submitted version.
Funding
This study was funded by the Czech Science Foundation (GAČR), numbers: 19-04301S (LZ, PK), and 22-12648J (PK, LZ), 22-18424M (JP). JP, MG, and PK were also funded by the “Centre for research of pathogenicity and virulence of parasites” (no. CZ.02.1.01/0.0/0.0/16_019/0000759) funded by the European Regional Development Fund (ERDF) and Ministry of Education, Youth, and Sport (MEYS).
Acknowledgments
We are grateful to Jan Erhart for tick rearing, Dr. Alena Krejčí (University of South Bohemia, Czech Republic) for kindly providing us with the aliquot of anti-ATP5A antibody (ab14748), Dr. Lesley Bell-Sakyi (University of Liverpool, Great Britain) and the Tick Cell Biobank and Dr. Martin Palus (Biology Centre, Czech Republic) for kindly providing us with aliquots of I. ricinus cell line, and Dr. Martina Hajdušková for graphical visualization (https://www.biographix.cz).
Conflict of interest
The authors declare that the research was conducted in the absence of any commercial or financial relationships that could be construed as a potential conflict of interest.
Publisher’s note
All claims expressed in this article are solely those of the authors and do not necessarily represent those of their affiliated organizations, or those of the publisher, the editors and the reviewers. Any product that may be evaluated in this article, or claim that may be made by its manufacturer, is not guaranteed or endorsed by the publisher.
Supplementary material
The Supplementary Material for this article can be found online at: https://www.frontiersin.org/articles/10.3389/fcimb.2022.1081666/full#supplementary-material
References
Bell-Sakyi, L., Zweygarth, E., Blouin, E. F., Gould, E. A., Jongejan, F. (2007). Tick cell lines: tools for tick and tick-borne disease research. Trends Parasitol. 23, 450–457. doi: 10.1016/j.pt.2007.07.009
Ben-Yosef, M., Rot, A., Mahagna, M., Kapri, E., Behar, A., Gottlieb, Y. (2020). Coxiella-like endosymbiont of Rhipicephalus sanguineus is required for physiological processes during ontogeny. Front. Microbiol. 11. doi: 10.3389/fmicb.2020.00493
Bonnet, S. I., Binetruy, F., Hernández-Jarguín, A. M., Duron, O. (2017). The tick microbiome: Why non-pathogenic microorganisms matter in tick biology and pathogen transmission. Front. Cell. Infect. Microbiol. 7. doi: 10.3389/fcimb.2017.00236
Buysse, M., Duron, O. (2018). Multi-locus phylogenetics of the midichloria endosymbionts reveals variable specificity of association with ticks. Parasitology 145, 1969–1978. doi: 10.1017/s0031182018000793
Buysse, M., Floriano, A. M., Gottlieb, Y., Nardi, T., Comandatore, F., Olivieri, E., et al. (2021). A dual endosymbiosis supports nutritional adaptation to hematophagy in the invasive tick Hyalomma marginatum. eLife 10, e72747. doi: 10.7554/eLife.72747
Comandatore, F., Radaelli, G., Montante, S., Sacchi, L., Clementi, E., Epis, S., et al. (2021). Modeling the life cycle of the intramitochondrial bacterium "Candidatus Midichloria mitochondrii" using electron microscopy data. mBio 12, e0057421. doi: 10.1128/mBio.00574-21
Coon, K. L., Vogel, K. J., Brown, M. R., Strand, M. R. (2014). Mosquitoes rely on their gut microbiota for development. Mol. Ecol. 23, 2727–2739. doi: 10.1111/mec.12771
Correa, M. A., Matusovsky, B., Brackney, D. E., Steven, B. (2018). Generation of axenic Aedes aegypti demonstrate live bacteria are not required for mosquito development. Nat Commun 9, 4464. doi: 10.1038/s41467-018-07014-2
Douglas, A. E. (2009). The microbial dimension in insect nutritional ecology. Funct. Ecol. 23, 38–47. doi: 10.1111/j.1365-2435.2008.01442.x
Douglas, A. E. (2014). Symbiosis as a general principle in eukaryotic evolution. Cold Spring Harbor Perspect. Biol. 6, a016113–a016113. doi: 10.1101/cshperspect.a016113
Douglas, A. E. (2020). Housing microbial symbionts: evolutionary origins and diversification of symbiotic organs in animals. Philos. Trans. R. Soc. B: Biol. Sci. 375, 20190603. doi: 10.1098/rstb.2019.0603
Duron, O., Morel, O., Noël, V., Buysse, M., Binetruy, F., Lancelot, R., et al. (2018). Tick-bacteria mutualism depends on b vitamin synthesis pathways. Curr. Biol. 28, 1896–1902.e1895. doi: 10.1016/j.cub.2018.04.038
Epis, S., Sassera, D., Beninati, T., Lo, N., Beati, L., Piesman, J., et al. (2008). Midichloria mitochondrii is widespread in hard ticks (Ixodidae) and resides in the mitochondria of phylogenetically diverse species. Parasitology 135, 485–494. doi: 10.1017/S0031182007004052
Esser, D., Lange, J., Marinos, G., Sieber, M., Best, L., Prasse, D., et al. (2019). Functions of the microbiota for the physiology of animal metaorganisms. J. Innate Immun. 11, 393–404. doi: 10.1159/000495115
Gilliland, C.A., Patel, V., Dombrowski, A.C., Mackett, B.M., Vogel, K.J. (2022), Using axenic and gnotobiotic insects to examine the role of different microbes on the development and reproduction of the kissing bug Rhodnius prolixus (Hemiptera: Reduviidae). Mol Ecol. doi: 10.1111/mec.16800
Gonzalez, J., Bickerton, M., Toledo, A. (2021). Applications of artificial membrane feeding for ixodid ticks. Acta Trop. 215, 105818. doi: 10.1016/j.actatropica.2020.105818
Guizzo, M. G., Neupane, S., Kucera, M., Perner, J., Frantová, H., Da Silva Vaz, I., et al. (2020). Poor unstable midgut microbiome of hard ticks contrasts with abundant and stable monospecific microbiome in ovaries. Front. Cell. Infect. Microbiol. 10. doi: 10.3389/fcimb.2020.00211
Guizzo, M. G., Parizi, L. F., Nunes, R. D., Schama, R., Albano, R. M., Tirloni, L., et al. (2017). A coxiella mutualist symbiont is essential to the development of Rhipicephalus microplus. Sci Rep 7, 17554. doi: 10.1038/s41598-017-17309-x
Guizzo, M. G., Tirloni, L., Gonzalez, S. A., Farber, M. D., Braz, G., Parizi, L. F., et al. (2022). Coxiella endosymbiont of Rhipicephalus microplus modulates tick physiology with a major impact in blood feeding capacity. Front. Microbiol. 13. doi: 10.3389/fmicb.2022.868575
Hajdusek, O., Sojka, D., Kopacek, P., Buresova, V., Franta, Z., Sauman, I., et al. (2009). Knockdown of proteins involved in iron metabolism limits tick reproduction and development. Proc. Natl. Acad. Sci. 106, 1033–1038. doi: 10.1073/pnas.0807961106
Hammer, T. J., Sanders, J. G., Fierer, N. (2019). Not all animals need a microbiome. FEMS Microbiol. Lett. 366(10), fnz117. doi: 10.1093/femsle/fnz117
Krawczyk, A. I., Röttjers, L., Fonville, M., Takumi, K., Takken, W., Faust, K., et al. (2022). Quantitative microbial population study reveals geographical differences in bacterial symbionts of Ixodes ricinus. Microbiome 10, 120. doi: 10.1186/s40168-022-01276-1
Kröber, T., Guerin, P. M. (2007). In vitro feeding assays for hard ticks. Trends Parasitol. 23, 445–449. doi: 10.1016/j.pt.2007.07.010
Livak, K. J., Schmittgen, T. D. (2001). Analysis of relative gene expression data using real-time quantitative PCR and the 2–ΔΔCT method. Methods 25, 402–408. doi: 10.1006/meth.2001.1262
Lo, N., Beninati, T., Sassera, D., Bouman, E. A. P., Santagati, S., Gern, L., et al. (2006). Widespread distribution and high prevalence of an alpha-proteobacterial symbiont in the tick Ixodes ricinus. Environ. Microbiol. 8, 1280–1287. doi: 10.1111/j.1462-2920.2006.01024.x
Moullan, N., Mouchiroud, L., Wang, X., Ryu, D., Williams, E. G., Mottis, A., et al. (2015). Tetracyclines disturb mitochondrial function across eukaryotic models: A call for caution in biomedical research. Cell Rep. 10, 1681–1691. doi: 10.1016/j.celrep.2015.02.034
Mushegian, A. A., Walser, J.-C., Sullam, K. E., Ebert, D., Hoye, B. (2018). The microbiota of diapause: How host-microbe associations are formed after dormancy in an aquatic crustacean. J. Anim. Ecol. 87, 400–413. doi: 10.1111/1365-2656.12709
Narasimhan, S., Rajeevan, N., Liu, L., Zhao, Y. O., Heisig, J., Pan, J., et al. (2014). Gut microbiota of the tick vector Ixodes scapularis modulate colonization of the Lyme disease spirochete. Cell Host Microbe 15, 58–71. doi: 10.1016/j.chom.2013.12.001
Ninio, C., Plantard, O., Serra, V., Pollera, C., Ferrari, N., Cafiso, A., et al. (2015). Antibiotic treatment of the hard tick Ixodes ricinus: Influence on Midichloria mitochondrii load following blood meal. Ticks Tick-borne Dis. 6, 653–657. doi: 10.1016/j.ttbdis.2015.05.011
Oliver, J. D., Price, L. D., Burkhardt, N. Y., Heu, C. C., Khoo, B. S., Thorpe, C. J., et al. (2021). Growth dynamics and antibiotic elimination of symbiotic Rickettsia buchneri in the tick Ixodes scapularis (Acari: Ixodidae). Appl. Environ. Microbiol. 87, e01672-20. doi: 10.1128/aem.01672-20
Olivieri, E., Epis, S., Castelli, M., Varotto Boccazzi, I., Romeo, C., Desirò, A., et al. (2019). Tissue tropism and metabolic pathways of Midichloria mitochondrii suggest tissue-specific functions in the symbiosis with Ixodes ricinus. Ticks Tick-borne Dis. 10, 1070–1077. doi: 10.1016/j.ttbdis.2019.05.019
Perner, J., Kotál, J., Hatalová, T., Urbanová, V., Bartošová-Sojková, P., Brophy, P. M., et al. (2018). Inducible glutathione s-transferase (IrGST1) from the tick Ixodes ricinus is a haem-binding protein. Insect Biochem. Mol. Biol. 95, 44–54. doi: 10.1016/j.ibmb.2018.02.002
Richter, U., Ng, K. Y., Suomi, F., Marttinen, P., Turunen, T., Jackson, C., et al. (2019). Mitochondrial stress response triggered by defects in protein synthesis quality control. Life Sci. Alliance 2, e201800219. doi: 10.26508/lsa.201800219
Romoli, O., Schönbeck, J. C., Hapfelmeier, S., Gendrin, M. (2021). Production of germ-free mosquitoes via transient colonisation allows stage-specific investigation of host–microbiota interactions. Nat Commun 12, 942. doi: 10.1038/s41467-021-21195-3
Ross, B. D., Hayes, B., Radey, M. C., Lee, X., Josek, T., Bjork, J., et al. (2018). Ixodes scapularis does not harbor a stable midgut microbiome. ISME J. 12, 2596–2607. doi: 10.1038/s41396-018-0161-6
Sacchi, L., Bigliardi, E., Corona, S., Beninati, T., Lo, N., Franceschi, A. (2004). A symbiont of the tick Ixodes ricinus invades and consumes mitochondria in a mode similar to that of the parasitic bacterium Bdellovibrio bacteriovorus. Tissue Cell 36, 43–53. doi: 10.1016/j.tice.2003.08.004
Sassera, D., Beninati, T., Bandi, C., Bouman, E. A. P., Sacchi, L., Fabbi, M., et al. (2006). ‘Candidatus Midichloria mitochondrii’, an endosymbiont of the tick Ixodes ricinus with a unique intramitochondrial lifestyle. Int. J. Systemat. Evolution. Microbiol. 56, 2535–2540. doi: 10.1099/ijs.0.64386-0
Sassera, D., Lo, N., Bouman, E. A. P., Epis, S., Mortarino, M., Bandi, C. (2008). “Candidatus Midichloria” endosymbionts bloom after the blood meal of the host, the hard tick Ixodes ricinus. Appl. Environ. Microbiol. 74, 6138–6140. doi: 10.1128/aem.00248-08
Song, X., Zhong, Z., Gao, L., Weiss, B. L., Wang, J. (2022). Metabolic interactions between disease-transmitting vectors and their microbiota. Trends Parasitol. 38, 697–708. doi: 10.1016/j.pt.2022.05.002
Steven, B., Hyde, J., Lareau, J. C., Brackney, D. E. (2021). The axenic and gnotobiotic mosquito: Emerging models for microbiome host interactions. Front. Microbiol. 12. doi: 10.3389/fmicb.2021.714222
Zhang, C.-M., Li, N.-X., Zhang, T.-T., Qiu, Z.-X., Li, Y., Li, L.-W., et al. (2017). Endosymbiont CLS-HI plays a role in reproduction and development of Haemaphysalis longicornis. Exp. Appl. Acarol. 73, 429–438. doi: 10.1007/s10493-017-0194-y
Zhong, J., Jasinskas, A., Barbour, A. G. (2007). Antibiotic treatment of the tick vector Amblyomma americanum reduced reproductive fitness. PloS One 2, e405. doi: 10.1371/journal.pone.0000405
Keywords: Ixodes ricinus, ticks, symbionts, Midichloria, membrane feeding, tetracycline, mitochondria
Citation: Guizzo MG, Hatalová T, Frantová H, Zurek L, Kopáček P and Perner J (2023) Ixodes ricinus ticks have a functional association with Midichloria mitochondrii. Front. Cell. Infect. Microbiol. 12:1081666. doi: 10.3389/fcimb.2022.1081666
Received: 27 October 2022; Accepted: 12 December 2022;
Published: 09 January 2023.
Edited by:
Jeff Grabowski, Foundation for Advanced Education in the Sciences, United StatesReviewed by:
Jonathan D. Oliver, University of Minnesota Twin Cities, United StatesBenjamin Cull, University of Minnesota Twin Cities, United States
Copyright © 2023 Guizzo, Hatalová, Frantová, Zurek, Kopáček and Perner. This is an open-access article distributed under the terms of the Creative Commons Attribution License (CC BY). The use, distribution or reproduction in other forums is permitted, provided the original author(s) and the copyright owner(s) are credited and that the original publication in this journal is cited, in accordance with accepted academic practice. No use, distribution or reproduction is permitted which does not comply with these terms.
*Correspondence: Jan Perner, perner@paru.cas.cz
†These authors have contributed equally to this work