- 1Department of Agricultural Economics and Rural Development (DARE), Georg-August-Universität Göttingen, Göttingen, Germany
- 2International Center for Tropical Agriculture (CIAT), Cali, Colombia
Coffee is a major commodity crop that shapes large shares of tropical landscapes. However, the sustainability of these landscapes is threatened by climate change. Whilst adopting climate-smart (CS) practices clearly offers direct benefits to local farmers, their greater benefits at landscape and global scales has not been studied for specific commodity crops so far. Our research uniquely outlines how local adoption of CS-practices in coffee-farming systems provides local, landscape and global benefits. We review literature on CS agriculture, CS landscapes, and coffee farming to firstly identify the different CS-practices applicable to coffee farming systems, and then group these into functional groups that represent the main functional trait targeted by different practices within coffee-farming systems. This allows identifying benefits provided at local, landscape and global scales. The seven functional groups identified are: soil characteristics; water management; crop and genetic diversity; climate buffer and adjustment; crop nutrient management; structural elements and natural habitats; and system functioning. Benefits offered at landscape and global scales (non-exhaustively) include improved water quality, biodiversity conservation and habitat connectivity, as well as stabilized regional climate patterns. Our review shows that regulating services are especially pronounced, although the extent of benefits provided depend on landscape coordination. We discuss considerations for managing possible conflicts, coordinating actions, financing and accommodating lead time. Local farmers, policy-makers and global donors must unite to improve uptake of CS coffee-production practices in a coordinated way, to thereby augment and safeguard coffee-farming's socio-ecological system along with associated local, landscape and global benefits.
Introduction
Coffee is a global commodity crop which shapes tropical landscapes through its particular agricultural system (Harvey et al., 2021). Coffee landscapes are highly valuable for global climate and biodiversity (Jha et al., 2014; Pendrill et al., 2019). Coffee farming and processing is a major source of income for local populations (Harvey et al., 2021). Depending on shade levels, its cultivation may preserve local ecosystems, but it can also degrade them (Jha et al., 2014; Martin et al., 2020). Climate change threatens to alter local land use and production systems. Adaptation to and mitigation of the effects of climate change will help secure local livelihoods, and have been shown to have global implications through teleconnections (Rahn et al., 2014; Pendrill et al., 2019; Verburg et al., 2019).
Climate-smart landscapes (Scherr et al., 2012) have been established as a general guiding framework for effective climate action, and include multifunctional landscapes. Multifunctional landscapes deliver multiple ecosystem services throughout each landscape, maximizing contributions to human and other species' well-being and achieving system sustainability (Fischer et al., 2006; Wu, 2013; Hölting et al., 2019). However, despite their relevance for climate action, a more finely-grained framework and review has yet to be developed. Such an approach should structure applicable practices and connect local action to benefits at local, landscape and global scales (Harvey et al., 2021).
We use coffee landscapes as an example of a tropical commodity crop adapting to climate change, as it is of major importance for local livelihoods and biodiversity impacts, especially in Latin America. This lays foundations for establishing more effective links with local policy and communities, and with international donors, when aiming to sustainably adapt commodity landscapes to climate change.
From Climate-Smart Agriculture to Climate-Smart Landscapes…
Globally, agricultural systems and surrounding nature are affected by the impacts of climate change, with agriculture simultaneously contributing to it as a major driver (Rosenzweig and Tubiello, 2007; IPCC WG III, 2014; Locatelli et al., 2015). Climate-smart agriculture (CSA) has been proposed as a solution to this dilemma, where climate actions aiming for adaptation and resilience assure livelihoods and provide opportunities for greenhouse gas (GHG) emissions reduction or carbon sequestration (Lipper et al., 2014). On the ground, climate smartness is achieved by implementing climate-smart (CS) practices. CS-practices address one or more of the three pillars of climate-smartness: (i) securing or increasing incomes, (ii) adapting to climate change, and increasing climate resilience, (iii) GHG emissions reduction or carbon sequestration (FAO, 2013). Additionally, CSA's potential for mitigating the effects of climate change helps to access further (particularly financial) resources, for example from climate compensation payments or ambitions from value chain, national or international actors, that aim to advance toward climate mitigation1 (FAO, 2013).
However, to accommodate the tight interconnectedness of farming with its surrounding landscape and related societies (Sayer et al., 2013; Reed et al., 2016), the concept of climate-smart landscapes (Scherr et al., 2012) was quickly proposed as an expansion. Climate-smart landscapes link local action to the landscape scale, consider interactions and land-use diversity, and adopt a process of integrated landscapes management to help achieve the goals of climate-smart agriculture (Scherr et al., 2012).
The concept of climate-smart landscapes has found especial resonance for tropical landscapes (Harvey et al., 2014; Chandra et al., 2018), as these still preserve a major share of global biodiversity and embrace a key region for carbon sequestration (IPBES, 2019; Pendrill et al., 2019). However, these landscapes are expected to be hardest hit by climate change, with local farmers often lacking financial options for adaptation actions, resulting in communities being skewed between international market forces and external earth-system changes that have been caused by the global north (McCarthy, 2014). Also, the landscapes' multiple functions are threatened by international market dynamics, especially from deforestation-risk commodity crops such as soy, cacao, palm oil or coffee (Pendrill et al., 2019).
…To Coffee…
Coffee landscapes have been subject to profound biophysical changes over the last 20 years (Harvey et al., 2021). Though sustainability certifications continue to gain ground, coffee and other landscapes still continue to be degraded (Harvey et al., 2021). Of particular concern are conventional intensification, with less cover crops and increased rate of fertilizer and pesticide use; encroachment on neighboring forested areas; but also abandonment of coffee farming, either for urban settlements or for other, often non-agroforest systems with higher environmental costs (Martin et al., 2020; Harvey et al., 2021).
Climate change is expected to increase these trends, with up to 50% of coffee farming estimated to fall out of production by 2050, causing major distortions to local production and farmers' livelihoods (Rahn et al., 2014; Bunn et al., 2015). The abiotic stresses of higher temperatures and greater variability in precipitation will constrain coffee productivity and quality, while increasing susceptibility to pests and diseases, alongside challenged pollination services (see Pham et al., 2019 for a detailed overview). Of the two major coffee species farmed, Arabica (Coffea arabica) is expected to suffer more from higher temperatures, while Robusta (C. canephora) will likely suffer most from higher intra-seasonal temperature variability (Bunn et al., 2015). Regions formerly known for their distinctive taste, and valued accordingly, may struggle to maintain price premiums, as changing climates alter coffee profiles (Läderach et al., 2011), in a similar fashion to the discourse on denomination of origin in changing climates in the wine sector (Mosedale et al., 2016).
Smallholder farmers will especially struggle to adapt successfully (McCarthy et al., 2011; Harvey et al., 2018). In some regions incremental adaptation action may be sufficient (Läderach et al., 2017; Verburg et al., 2019). However, where impacts of climate change are more severe, or production is located in already suboptimal areas, climate change will cause the need for systemic or even transformative adaptation (Verburg et al., 2019). Where farmers' adaptation capacity is surpassed, these producers will have to abandon farming and migrate. This will cause farmland degradation, where coffee agroforestry systems give way to open-land farming (Martin et al., 2020) or to a climate-migration nexus similar to that already seen, for example, in Central America (Kumari et al., 2018; Lynch, 2019). Considering the complex socio-ecological system that coffee farming constitutes, these changes are a threat not only to local farmers' livelihoods, but also to other related land-uses and general landscape sustainability, as also to global sustainability and climate action (Rahn et al., 2014; Capitani et al., 2019; Pendrill et al., 2019; Harvey et al., 2021).
…To Climate-Smart Coffee Landscapes
The adoption of the concept of climate-smart landscapes for managing coffee landscapes therefore constitutes an opportunity to preserve or even increase landscape social and economic sustainability, and at the same time deliver ecosystem services that are provided by local farming systems and valued translocally (Harvey et al., 2014).
However, operationalizing climate-smart coffee landscapes requires understanding of functional interactions between the adoption of field-based practices and landscape benefits. This will allow for targeted actions and effective communication with local communities and policy-makers, as well as potential donors in international development cooperation (Prager and Pfeifer, 2015).
Though discussed here for coffee in particular, the outlined interactions between local practices, landscapes and global benefits may also be applicable to other tropical commodity crops that can be produced in agroforestry systems, such as cacao (Tscharntke et al., 2015). We aim to create a basic understanding of elements and interactions needed for local, landscape and global benefits, addressing specific functional groups needed in the local context to guide actions on the ground.
Our research aims to show how the adoption of CS-practices in coffee farming systems contributes to local, landscape and global benefits. In the following sections, we first conceptualize coffee farming as complex socio-ecological systems, to illustrate the multiple interrelations such farming systems involve. We secondly outline the review methodology applied, and thirdly identify CS-practices applicable to coffee farming systems that are then structured according to functional groups. Based on these functional groups, we fourthly outline any derived potential benefits that climate-smart coffee landscapes may offer (i.e. in climate-smart landscapes with coffee as an anchor crop). Fifthly, we discuss further actions needed to establish climate-smart coffee landscapes, also highlighting any potential pitfalls. We conclude by articulating further policy recommendations and future research directions.
The Complex Socio-Ecological System of Coffee Landscapes
The socio-ecological systems framework describes human-nature interaction and sees human actions as strongly embedded in and interacting with local and global dynamics. For land systems, this framework integrates land use as a connection between social and ecological systems, whilst these elements are embedded in and interact with the global earth systems (GLP, 2005). Coffee farming systems can be seen as a complex socio-ecological systems (Schröter et al., 2018; Malik et al., 2019). Interactions occur between (i) the social system, embedding socio-cultural values and economics, (ii) the ecological system of tropical landscapes, and (iii) the local land-use system of coffee farming, forming and being formed by landscape and culture. Meanwhile, these arising coffee landscapes are coupled to (iv) the global earth system, especially through the impacts of climate change and the landscapes' importance for sustaining global biodiversity, whilst also being influenced through (v) international market dynamics.
The social system of coffee farming embeds the local economy, oftentimes strongly depending on this commodity crop, and the related local cultural values. Globally, for about 12.5 million farmers, coffee farming is the primary source of income (Browning, 2019), of whom many are smallholders (Pham et al., 2019). Coffee farming provides jobs and contributes to poverty alleviation and to the economies of the countries where it is produced (Pham et al., 2019). Including the farmers' families, an estimated 60–120 million people's livelihoods depends on coffee farming (Sachs et al., 2019). However, in many countries coffee farming is also deeply rooted in local culture and heritage: The coffee cultural landscape of Colombia, for example, was listed in the UNESCO World Heritage List2 in 2011 (Silva, 2017), showing its value as local and global cultural heritage. Likewise, coffee as a locally-produced crop can be deeply integrated in cultural rituals, as, for example, the Ethiopian coffee ceremony shows (Hirons et al., 2016).
The ecological system is partly characterized by the local abiotic and biotic conditions that interact with local coffee farming. Abiotic conditions such as temperature and precipitation influence coffee quality and yields (Pham et al., 2019). Moreover, altitude and climate influence which coffee species can be farmed: Robusta better withstands higher temperatures but needs sufficient precipitation or additional irrigation (Descroix and Snoeck, 2009). Meanwhile, Arabica coffee, which is more highly valued due to its milder taste, is generally farmed at higher altitudes (Descroix and Snoeck, 2009).
Land use connects the social system with the ecological tropical environment in which coffee farming is embedded, and also shapes the natural environment. Pollinators and pest control agents are important for coffee yield and quality, and at the same time their abundance and diversity is influenced by the way in which local farming systems are managed (Tscharntke et al., 2011). The characteristics of the land-use system always include the coffee plant as a woody perennial but vary with production intensity (Moguel and Toledo, 1999): In extensive systems, coffee is farmed as an understory crop in multispecies farming systems. However, in more intensive systems diversity of companion crops is gradually reduced and overstory trees (especially shade trees) are increasingly mono-species (Jha et al., 2014; Martin et al., 2020). In sun-grown coffee systems they are entirely absent. Systems with higher degrees of shade can store more carbon, are more climate-resilient, can deliver more diverse ecosystem services, and contribute more strongly to nature and biodiversity conservation (Tscharntke et al., 2011; van Rikxoort et al., 2014; Pham et al., 2019). However, lower levels of shade offer higher yields, at least if abundance of water is sufficient (Schnabel et al., 2018). Recognition of this tight socio-ecological interaction and its importance for biodiversity can be seen in various coffee production countries: Apart from the coffee cultural landscapes in Colombia, also Man and the Biosphere Reserves in Mexico3, El Salvador4, and Ethiopia (UNESCO, 2011) explicitly acknowledge the key influence of coffee farming on the landscape and its biodiversity.
The earth system is mainly influenced by the impact of coffee farming on the global tropics, whilst impacting coffee landscapes through market dynamics and recently also climate change: The tropics harbor the highest biodiversity worldwide, where climate changes poses special risks for such biodiversity (Pimm et al., 2014; IPBES, 2019). More than 19 of the currently recognized 36 global biodiversity hotspots are situated in the tropics, and many of these in major coffee production countries such as Brazil, Vietnam, Colombia, and also the Mesoamerican region. At the same time, tropical rainforests are probably the most emblematic carbon sink globally. However, as coffee farming areas expand or shift to new areas, these forests and the biodiversity they preserve may be compromised through deforestation (Läderach et al., 2017).
International market demands and dynamics may induce such expansion of coffee farming areas (Pendrill et al., 2019; Harvey et al., 2021), and thereby illustrate a further important interaction within the socio-ecological system. The coffee market is buyer-driven, with power located in consuming countries, mainly located in the global north (Grabs and Ponte, 2019). Prices are volatile and pressure is passed on along the value chain to upstream actors, compromising profit margins and economic viability of coffee traders and growers (Grabs and Ponte, 2019). Examples for interactions of global trends in coffee production with local landscapes can be seen in the 1990's boom and bust cycles or also recent altitudinal migration due to climate change and consumers' preferences. The boom and bust cycles were caused by global market deregulation, combined with booms after local frosts in Brazil that caused global coffee prizes to surge, and induced overproduction on new cultivation areas, leading up to busts in later years (Eakin et al., 2009). More recently, the example of high-grown coffee illustrates the direct influence global markets can have on local ecosystems: This coffee is increasingly demanded and more highly valued due to a milder, more aromatic taste, incentivizing shifts of coffee farms within landscapes toward areas in higher altitudes, especially as, with climate change, lower areas become unsuitable for coffee farming (CIAT, 2017). However, such altitudinal migration thereby compromises local natural habitats and rainforests that remain in these higher altitudes (Läderach et al., 2017). Thus, we begin to see how climate change acts as a new and additional driver, causing great distortion in the socio-ecological system of coffee farming.
Methods
Our research will show how the adoption of CS-practices in coffee farming systems contributes to local, landscape and global benefits. However, considering the multitude of available practices, instead of trying to link each individual practice to different benefits, we classified practices in seven functional groups (for example “soil characteristics” or “water management”, see section Review findings), according to the functional traits of the farming system to which these practices contribute. Then, based on these functional groups, we established links to the broader benefits provided by the adoption of those different functional groups (for example “habitat connectivity” or “water quality”).
Our research design is based on an iterative, exploratory literature review (Arksey and O'Malley, 2005; Baumüller, 2018) and consists of three successive major steps: We (i) identified the different CS-practices applicable to coffee farming systems, (ii) grouped them into different functional groups that describe the main functional trait that these practices target within the farming system, to then (iii) perform an additional review, that identifies benefits that are provided at local, landscape and global scales when adopting CS-coffee practices.
In detail, in a first step, our iterative reviews started from the basic element of climate-smart landscapes: the adoption of CS-practices. We reviewed literature from the year range of 2010–2020, drawing on Web of Science, Scopus and Google Scholar as search engines and including scientific and gray literature. Our understanding of CS-practices is based on the official definition by the Food and Agricultural Organization by the United Nations (FAO, 2013), therefore including both, climate adaptation and climate mitigation practices, as long as positive livelihood effects for the farmer can be assumed.
A specific search for CS-practices in coffee yielded very few results: results were limited to individual case studies and gray literature, without taking further into account interactions between practices or landscape effects. Therefore, we defined the search scope somewhat more broadly: on the one hand, we broadened the agricultural field, including also CS-practices (search terms: “climate-smart practices”; “climate-smart agriculture” AND practices) in agriculture in general, and then subsequently refined listings to only include practices applicable to coffee farming systems. We checked for duplicates and then reviewed title, and if relevant, abstract of the papers, before reading the papers more in detail. For Google Scholar, we only reviewed the first 300 results for each query. To refine CS-practices to be applicable to coffee farming systems, the specific characteristics of coffee-farming systems were taken into account, including its characteristic as a woody perennial that can be farmed as an understory crop. Furthermore, practices had to be applicable in the tropics, were coffee is farmed.
On the other hand, we broadened the type of practice, including also climate mitigation (“climate change” AND mitigation AND coffee) or climate adaptation practices (“climate change” AND adaptation AND coffee) but here only including sources specifically aiming at coffee farming systems. We identified seven papers as especially relevant to list CS-practices or practices that offer combined adaptation and mitigation potential in coffee farming (find listing of practices in the Supplementary Material S1) and from which then our subsequent identification of functional groups started from.
In the second step, we distilled major functional groups from the listings of CS-practices for coffee-farming systems. These groups correspond to the main functional trait that the practices address within the coffee farming system. Functional groups (such as “Natural habitats”) then allowed us to link functional traits that are improved through the adoption of CS-practices (such as “Structural elements and natural habitats”) to broader benefits of coffee-farming systems that have been reported throughout scientific literature on coffee-farming systems.
Therefore, in a third step, and based on these functional groups, we then performed another review, to identify contributions to local, landscape and global benefits. For each functional group we checked for corresponding papers that report on elements in coffee-farming systems or similar farming systems (for example cacao) presenting connections between the identified functional groups and benefits at local, landscape or global scale. Here, we based our work in the principles of landscape ecology (especially Tscharntke et al., 2005, 2012; Fischer et al., 2006). Also, we took into account the conceptual advances on landscape benefits and landscape multifunctionality (Scherr et al., 2012; and for landscape services Termorshuizen and Opdam, 2009; Bastian et al., 2014). These benefits were then reported in a structured form, applying the Millennium Ecosystem Assessment (MEA) framework for ecosystem service assessment, distinguishing between regulating, provisioning and cultural ecosystem services (MEA, 2003).
Review Findings
Functional Groups of Climate-Smart Practices in Coffee Farming
CS-practices, or practices that contribute to climate adaptation or mitigation, were listed in various sources (see also listing in Supplementary Material S1). Scherr et al. (2012) and FAO (2013) list CS-practices for agriculture in general, as well as Harvey et al. (2014) who offer an overview on practices that contribute to climate adaptation and mitigation and possible synergistic potential. For coffee cultivation in particular, possible climate adaptation measures are listed in c&c (2015) and by Pham et al. (2019) and Verburg et al. (2019). Combined adaptation and mitigation practices are listed in Rahn et al. (2014) for the region of Nicaragua, whereas in CIAT (2017) an extensive list of specific CS-practices for coffee farming in Honduras is provided.
For CS-practices in coffee farming, we identified seven functional groups contributing to the overarching goals of CSA: improved and sustainable livelihoods, climate adaptation, and climate mitigation (see also Figure 1):
1. Soil characteristics
2. Water management
3. Crop and genetic diversity
4. Climate buffer and adjustment
5. Nutrient management
6. Structural elements and natural habitats
7. System functioning.
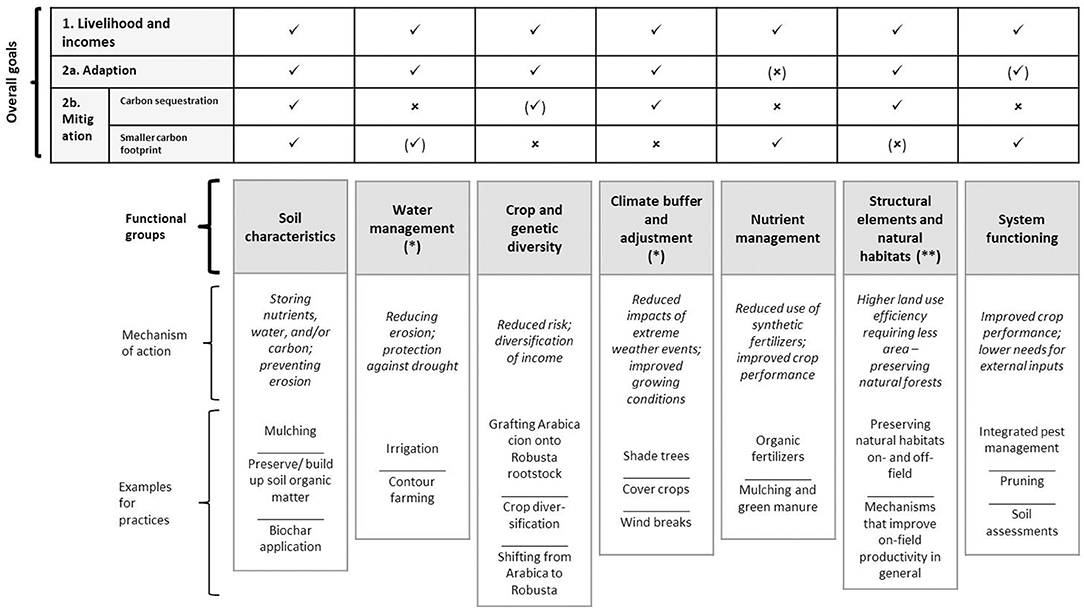
Figure 1. Functional groupings of climate-smart practices in coffee farming. Top part of the table describes contribution to overall goals of climate-smartness. Mitigation action is additionally differentiated in carbon sequestration and carbon footprint reduction. In the bottom part, mechanism of action describes how functional groups deliver farm, landscape, and global benefits. Asterisk additional mark where landscape-wide adoption is of special importance for emerging effects to occur: (*) = improved effective scale is landscape wide adoption; (**) = optimum effective scale is landscape-wide adoption. Own elaboration.
These contribute to improved livelihoods, adaptation and/or mitigation through the following interactions:
(1) Soil characteristics: Soil is vital for coffee crop performance as plants extract water and nutrients from it, with the top 30 cm being of special importance for coffee plants (Wintgens and Descroix, 2009). Any loss of soil or degradation of soil biota should be avoided (Wintgens and Descroix, 2009). Soil organic matter improves system resilience and contributes to important nutrient and hydrological cycles and poses important adaptation and mitigation benefits (Scherr et al., 2012; Harvey et al., 2014). Measures like application of biochar or mulching contribute to building up soil organic matter and help to improve soil structure, whilst at the same time sequestering carbon (CIAT, 2017). Furthermore, mulching keeps the soil covered, contributing to reduced soil erosion, and can also reduce soil water loss (CIAT, 2017).
(2) Water management: Improving water management to reduce the impacts of precipitation extremes. Minimizing water erosion and run-off lead to better water availability for the plant and reduced nutrient loss (Harvey et al., 2014; Verburg et al., 2019). Improving irrigation efficiency and water use efficiency in general are important elements in developing sound climate adaptation strategies, maintaining viability of coffee farming also in regions with increased drought frequency (Pham et al., 2019). When wastewater treatment is introduced in wet processing of coffee beans, GHG-emissions can also be reduced (Rahn et al., 2014).
(3) Crop and genetic diversity: Higher genetic diversity and use of more adequate genotypes (e.g., for high-temperature- or drought-tolerance) lowers production risks by increasing resilience to environmental shocks and maximizing crop suitability under the given climate (DaMatta et al., 2018), with better-adapted crops less susceptible to new pests and diseases entering the regions when climate changes (Verburg et al., 2019). More diverse systems generally show higher resilience, not only in the face of climate-change, but also for food security and when subject to market shocks (Scherr et al., 2012; Tscharntke et al., 2012). Also, if woody crops are introduced for diversification, in-field biomass increases, and thus higher in-field carbon stocks are created, thereby contributing to climate mitigation.
(4) Climate buffer and adjustment: Elements that buffer extreme climatic conditions avoid or reduce stress, which would otherwise compromise crop performance and would increase the susceptibility of coffee plants to pests and diseases (Pham et al., 2019). Shade trees, for example, can reduce air temperatures and lower wind speeds (Pham et al., 2019). Moreover, they can serve as an additional source of income and reduce dependency on coffee (Harvey et al., 2014). However, shade level and species of shade trees should be adjusted to local conditions as they might also compete with coffee for light, nutrients and water when chosen wrong (Pham et al., 2019; Verburg et al., 2019).
(5) Nutrient management: Nutrient supply is crucial for crop performance. Sustaining an adequate level of nutrients maximizes yields while lowering susceptibility to pests and diseases (Muller et al., 2009). Moreover, when using green manure or similar organic fertilizers, production costs for the farmer may be lowered (Scherr et al., 2012). Also, using organic instead of mineral fertilizers avoids production-related GHG-emissions. Nitrogen-fixing shade trees may improve crop performance while lowering the need for fertilizers (Scherr et al., 2012).
(6) Structural elements and natural habitats: Protection and restoration of structural elements and natural habitats on and off farms can secure the deliverance of important ecosystem services like pest control, pollination, or improved climate to coffee production (Tscharntke et al., 2011; Avelino et al., 2012; Verburg et al., 2019). They also act as important carbon sinks (van Rikxoort et al., 2014). In general, increasing productivity and climate resilience in-field helps to take pressure from natural habitats still under protection, likewise preserving ecosystem functions delivered by them (FAO, 2013).
(7) System functioning: Improved system functioning mainly refers to better crop performance or lowered management costs. Requiring less external input such as herbicides or synthetic fertilizers reduces GHG-emissions and lowers management costs, while improved crop performance increases productivity (Verburg et al., 2019). Likewise, soil assessment techniques can combine optimized fertilizer use with reduced nitrogen leaching as well as improved overall plant performance (Mueller et al., 2011; FAO, 2013; Ball et al., 2017). Examples of practices are integrated pest management with lowered costs for pesticides, and also pruning which enhances crop performance and lowers susceptibility to pests and diseases, while clippings can be used as fuelwood avoiding the need for fossil fuels (c&c, 2015).
Scale matters when studying the benefits of different CS-practices (Öborn et al., 2015). On-farm practices can strongly influence the surrounding landscape, while at the same time the efficiency of practices adopted will be influenced strongly by the surrounding landscape mosaic. Likewise, measures may imply optimum scales. From the groups named above, water management and climate buffer and climate adjustment are greatly enhanced when applied at a landscape scale (Scherr et al., 2012; Harvey et al., 2014). The delivery of vital ecosystem services of natural habitats may partly be achieved through climate buffer and adjustment elements already, however with conservation of natural habitats and structural elements on a larger scale, superadditive effects may also be achieved (Freeman, 2015; Verburg et al., 2019).
Multiscale Benefits and the Importance of Spatial Patterns
As previously mentioned, our research explores how the adoption of CS-practices in coffee farming systems contributes to local, landscape and global benefits. Having identified CS-practices in coffee farming, and classified these in functional groups, we are able to link local action to larger scale benefits. In the following, we first report how adopting CS-coffee practices can offer benefits at local, landscape and global scale (see Figure 2). Then we additionally report benefits under the MEA framework, for provisioning, regulating, and cultural ecosystem services. Here, it becomes clear that regulating services are especially pronounced, and additionally provisioning and cultural services can also be enhanced.
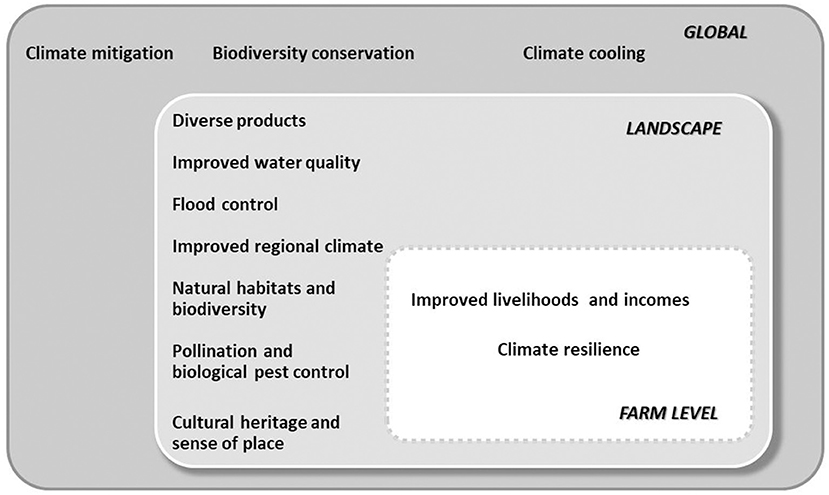
Figure 2. Multiscale benefits offered by implementation of climate-smart coffee practices. Farm-level benefits are summarized in view of the overall goal of the implementation of the climate-smart coffee practice on field. Own elaboration.
Farm Level Benefits
When adopting CS-practices, farmers can benefit in multiple ways. CS-smart practices may increase productivity, for example, when growing conditions are optimized through the use of shade trees. Management costs are lowered, for example with mulching suppressing weeds, decreasing the necessity of manual weed control or use of herbicides, while offering additional fertilization to the plants. Likewise, nitrogen-fixing plants decrease quantities of fertilizer needed. In parallel with adaptation, climate resilience increases. Additionally, ecosystem services preserved in the landscape may have beneficial effects for the farmer as well.
Furthermore, farmers may profit from new sources of income: secondary crops (introduced as climate buffers and adjustment elements or for enhanced crop diversity) can be used for self-supply, livestock fodder, or be sold at local or international markets. Prunings can be used as fuelwood. Protection of ecosystem services may be rewarded through national or international payments for ecosystem services and carbon mitigation through carbon compensation payments. Additionally, a label such as “climate-smart coffee landscape” may achieve higher selling prices at the market, raising farmers' incomes further and cross-financing adaptation measures to increase climate resilience further.
Landscape Benefits—Importance of Spatial Patterns
Coffee farms consist of different structural elements whose diversity is improved when CS-practices are adopted. Structural elements include the soil that is used, the coffee plant, different layers of overstory plants, and plants that are actively or coincidently intercropped with coffee plants. Coffee plants might be intercropped with secondary crops or cover crops, as well as shade trees, windbreaks or hedges, providing an additional structural level. Together, those elements can deliver different provisioning, regulating, and cultural services to the landscape and influence different aspects of human well-being, with benefits emerging through the interaction at the greater, the landscape scale. To illustrate mechanisms, we provide an example outline of options to contribute to some of these landscape ecosystem services as follows:
• Improved water quality Contribution of climate-smart on-farm practices to improved water quality within the region takes place by three different mechanisms: Firstly, minimizing erosion lowers nutrient discharge from the field and avoids overload of nutrients and suspended particles in local river basins. Functional groups that deliver these positive side effects are improving soil and water management, diversifying production, and integrating elements to buffer climate extremes. Secondly, when protecting local natural habitats, their water filtering function and positive contribution to local water quality are preserved. Thirdly, improving system functioning reduces need for agro-chemicals mitigating negative effects on water quality as well and reduces leaching of harmful substances (Jha et al., 2011; Verburg et al., 2019).
• Biodiversity Conservation and Natural Habitats Increasing the range of crop varieties and species, and using shading elements provides multiple microhabitats and niches for different organisms, hence contributing to biodiversity conservation. This also reduces susceptibilities to pests and diseases. Habitat connectivity is increased, as well as landscape permeability. Improved productivity and climate resilience in-field may reduce deforestation by agricultural expansion, though adversely with more financial resources available business expansion to those areas could also be a hypothetical consequence (Fischer et al., 2006; Jha et al., 2011; Tscharntke et al., 2012; Verburg et al., 2019).
• Improved regional climate Regional climate can be improved by introducing shade trees and climate-buffering elements throughout the landscape, and preserving natural habitats. Forests and increased ground-cover and cover-crops are able to buffer against extreme weather conditions like heavy rainfalls or strong winds. Moreover, higher rates of evapotranspiration can have positive impacts on cloud formation and therefore reduce the risk of drought within the landscape, though when groundwater recharge is low, increased evapotranspiration may also lower overall water availability. Trees and shrubs augment landscape roughness, lowering wind speed and reducing the risk of wind erosion (Jha et al., 2011; Ellison et al., 2017; Verburg et al., 2019).
• Habitats for pollinators and pest control agents Pollination and natural pest control are beneficial to coffee farming, have positive impacts on other farming activities within the landscape, and may improve general well-being of people. Abundance and diversity of beneficial species is increased by offering diverse habitats through crop diversification, introducing climate buffering elements, or preserving natural habitats. Other land uses within the region profit strongly from the landscape benefits mentioned, but will also restrict if full potential can be realized (Fischer et al., 2006; Tscharntke et al., 2011).
Whether or not, and to what extent coffee farms can deliver positive services to the surrounding landscape depends on how farms and other land uses are scattered across the landscape, and on a smaller scale the nature of their structural elements (Tscharntke et al., 2012). Whether the distribution of land use and structural elements within the landscape is clustered, forms blocks or linear elements, or is randomly or homogeneously distributed influences which landscape service(s) can be delivered, see Figure 3.
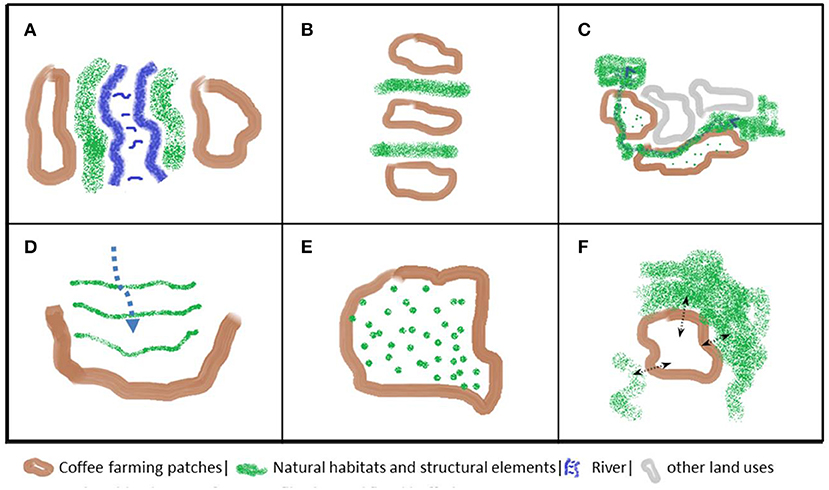
Figure 3. Spatial patterns influencing landscape service delivery. (A) Green riverside elements for water filtering and flood buffering. (B) Forest blocks as separation between coffee patches, slowing down wind speeds and disease spread (e.g. coffee rust). (C) Joining natural in-field elements for enhanced habitat connectivity and creation of natural corridors. (D) Orthogonal arrangement of blocked structural elements to slow down wind and/or water erosion, as well as to protect from strong winds. (E) Integrating natural elements on-farm for highest diversity and landscape permeability. (F) Maintaining natural habitats in neighboring areas to allow positive spill-over effects (pollination, natural pest control). Own elaboration, based on Goldman et al. (2007).
Blocked patches of natural habitats like forests or groups of trees and shrubs as structural elements can reduce wind speed, thus providing protection from windstorms, as well as slowing down the spread of fungal spores and reducing the spread of coffee-berry borer (Avelino et al., 2012).
Linear elements enhance landscape connectivity (Fischer et al., 2006; Tscharntke et al., 2012). When placed parallel to river flows, they can mitigate flooding and also serve as water filtering elements (Goldman et al., 2007). Clustered forests or structural elements close to and within a plantation can serve as natural habitats for biological control agents such as birds and ants (Verburg et al., 2019). However, depending on species of pollinators and trees within the patches, these elements might also lure pollinators or host pests (Verburg et al., 2019).
Mixing natural and structural elements within the coffee farms enhances habitat permeability and on-farm biodiversity, contributing to pollination and pest control (Tscharntke et al., 2015). Furthermore, depending on species chosen and stand density, ground temperature and throughfall rates of light and rain can be altered (Verburg et al., 2019). The created microclimate influences susceptibility to some pests and diseases, slowing down the development rate of coffee-berry borer larvae, but also potentially increasing likelihood of fungal infection if microclimates become too humid and air flow is overly reduced (Escobar-Ramírez et al., 2019; Verburg et al., 2019).
Global Benefits
Contribution to biodiversity conservation is achieved by offering new natural habitats and preserving existing ones, as well as by enhancing on- and off-farm habitat connectivity and land-use diversity, with spatial and functional diversity present (Jha et al., 2011; Tscharntke et al., 2015). This is of particular importance as coffee is farmed in the tropics, where a large share of global biodiversity is found that otherwise might be lost (Pimm et al., 2014). Biodiversity not only holds an intrinsic value but can also be highly beneficial to mankind as native crops and animals may be the basis for new breeds, or varieties, or pharmaceutical ingredients for example (MEA, 2005).
Climate mitigation is achieved by on- and off-farm carbon sequestration, reducing the carbon footprint of coffee farming, as well as preserving natural carbon sinks (Jha et al., 2011; Rahn et al., 2014). Corresponding functional groups include soil characteristics, climate buffer and adjustment, natural habitats and structural elements as well as system functioning.
Climate cooling can be achieved when forested areas and vegetal cover are provided and preserved, lowering surface temperature by increasing transpiration and redistributing solar energy (Ellison et al., 2017). Functional groups especially contributing to this service are natural habitats and structural habitats, as well as climate buffer and adjustment.
Benefits Under an Ecosystem-Service Perspective
Provisioning services provided include the diverse products and by-products offered by the crops planted, foremost coffee, but also fruits and timber. In most cases, within the landscape, only the farmers themselves will profit directly, but others may experience indirect benefits, for example, when being employed for pruning or harvesting. Fuel energy and basic resources are obtained, and food security is strengthened. Improved water quality is achieved by reducing water pollution, including erosion control, as well as water filtering. Amongst others, contribution to human well-being is made by providing clean drinking water.
Regulating services are especially pronounced: Coffee plants themselves, as well as climate-buffering elements on-farm, contribute to improved regional climate and buffer extreme weather events, also on a larger scale. Together with water buffers and natural habitats on-farm, they can reduce floods and regulate water flows. Natural habitats and structural elements can contribute to pollination and biological pest control within the landscape, as well as hindering spread of pests and diseases. Regarding human well-being, different regulating services contribute to different elements of security, helping to maintain a clean and safe environment for the local people and lower vulnerability to ecological stresses and shocks, including climate change. Influence on health and disease is more difficult to attribute or disaggregate: although natural medicine may be gathered in natural habitats, those habitats in turn may also serve as sources of diseases affecting the local populations.
Cultural services are also delivered: In many regions, especially in South and Central America, coffee farming is part of the local cultural heritage and strengthens the sense of belonging and social wellbeing. Natural habitats preserved through the adoption of CS-practices may have recreational and aesthetic value to people and can serve as an educational space on ecosystem functioning. Moreover, parts of the farms or aligned natural habitats may serve as recreational areas or induce (nature) tourism.
Discussion—Toward Climate-Smart Coffee Landscapes
Adopting CS-practices in coffee farming systems provides benefits that reach beyond the farm scale. Instead, the benefits help progress toward multifunctional landscapes and landscape and global sustainability, especially when coordination across the landscape takes place. In the tropics, and especially Latin America, coffee-farming landscapes face vast distortions due to climate change. By more widely introducing CS-practices in coffee farming, local actors can capitalize on the benefits provided to (i) promote especially those CS-practices that offer local benefits most needed in the local context, (ii) connect to international donors and value-chain actors, that have an interest in contributing to preserve the globally-valued landscape services these coffee systems provide (or which could be improved through the adoption of CS-practices), and (iii) coordinate and support coffee farmers to maximize effectiveness of their individual climate-adaptation processes. Within this context, our collection and classification of existing climate-smart practices in coffee farming can help to select most appropriate practices for local adaptation needs. Then, our review and the linking of functional groups to benefits further allows for efficient communications with policy makers and “best-fit” selection of CS-practices to be introduced in the coffee-farming systems.
However, there are three additional considerations: firstly, regarding possible conflicts that may arise; secondly, the need to coordinate action at the landscape scale; and thirdly, financing needs and lead time for introducing the appropriate practices.
Tensions can exist within coffee landscapes as it is traded globally but impacts landscapes locally. Coffee is a global commodity crop that shapes local culture and landscapes, trading local ecosystem services for financial returns, as Schröter et al. (2018) have shown. With coffee being traded on a buyer-driven market (Grabs and Ponte, 2019), external dynamics shape coffee landscapes, causing potential tensions within the landscape regarding land-use scarcity and pathways of future farm management. The benefits found at landscape scale, facilitated by the integration of CS-coffee practices on farm, show that opportunities exist to reconcile export-orientated farming with local well-being. Thereby potential land-use tensions within the landscape can be alleviated.
However, some CS-coffee practices themselves may lead to tensions within the region, especially irrigation: although beneficial for the individual farmer, irrigation may compromise water availability for other users within the landscape. When precipitation patterns change, this will also negatively affect groundwater recharge, thus if farmers purely rely on introducing irrigation systems to increase their climate-smartness, groundwater levels will sink. Therefore, coordination at landscape scale will be needed, bearing in mind that landscape services depend on landscape wide implementation and spatial patterns.
Thus, landscape-wide coordination is not only needed “to enhance field-level benefits of [sic] climate-smart practices, […] secure ecosystem functions, and […] enhance the effectiveness of climate mitigation efforts” (Scherr et al., 2012; p. 5) but is also of great importance to mitigate potential conflicts between different land users and with civil society throughout the landscape.
Climate-smart coffee landscapes can act as a possible entry point for sustainable landscape governance and climate adaptation. To avoid the risks that commodity-centric landscape governance may pose (c.f. Bastos Lima and Persson, 2020) landscape managers should combine perspectives from inside and outside the coffee commodity crop sector, carefully balancing power relations. The multi-stakeholder platform proposed by Scherr et al. (2012) might be an adequate agora to facilitate this coordination. In addition, livelihood opportunities outside the coffee sector will have to be identified, as some coffee farmers will face transformative adaptation where local capacity or climate change intensity make incremental or systemic adaptation insufficient. Here, too, a wider approach to developing multi-stakeholder platforms that integrates coffee and non-coffee actors will be needed for a smoother transition to alternative sectors within the landscape, connecting social systems and offering alternative livelihood opportunities.
Lastly, practices such as shade-tree planting will require a certain lead time or need financial capital to be implemented. Payment measures like carbon credits (e.g., Gold Standard5), recognizing the benefits of carbon storage and carbon mitigation may finance some of the actions, but additionally financial instruments will need to be established. Similarly, payments for ecosystem services may finance transition as improved ecosystem deliverance has been highlighted here. Already existing practices like in Costa Rica for landscape coordination and transition payments (introduction of NAMA coffee6) may serve as examples here.
Conclusion
Our research has shown the complexity of interactions in the socio-ecological system of coffee farming. It becomes clear that though climate change threatens to distort the socio-ecological system of coffee farming, the introduction of CS-practices and climate-smart landscapes can safeguard local livelihoods.
We propose a classification of the seven previously-listed functional groups for CS-practices in coffee farming, highlighting functional traits these practices address within the system of coffee farming. These groups help to understand underlying mechanisms and allow targeting specific functional traits at the farm and landscape level to advance toward developing and harnessing landscape multifunctionality. Additionally, if implemented in a coordinated way throughout the landscape, the adoption offers landscape and global benefits that include (non-exhaustively) improved water quality, biodiversity conservation and habitat connectivity, as well as stabilized regional climate patterns.
Our considerations regarding transition toward climate-smart landscapes can help to mitigate potential conflicts and trade-offs within landscapes. Having outlined how local action and adoption of CS-practices help to sustain landscape services, different land-users can better understand and negotiate which landscape services they value particularly or want to prioritize and how to allocate burdens and rewards for conservation or sustainable actions. This can include for example transfer payments such as payments for ecosystem services directed to benefit local coffee farmers.
Trade-offs from especially threatening possible adaptation scenario can be avoided if opportunities for in-field climate adaptation are realized: Farmers performing altitudinal migration in cooler climates threaten native forests that remain there. Likewise, if they abandon coffee farming the existing agroforestry systems of coffee farms will be degraded as they are generally replaced by annual farming systems. Instead, financial and knowledge support from external actors can enable farmers to also implement those CS-practices that may be more costly or require specialized knowledge, but at the same time preserve the valuable landscape services needed within the landscape.
Policy makers in coffee farming regions now need to facilitate participative processes on prioritization of vital landscape services (c.f. Brandt et al., 2017 as an example). Based on these, locally-relevant functional groups of CS-practices in coffee farming can be identified. For the adoption of the corresponding functional groups, interactions are needed between researchers, proposing innovative financial instruments, policy-makers and cooperatives, disseminating knowledge and offering support, and practitioners, actively being involved in a process of co-creation.
Further research needs to design and support processes for actual realization of such landscapes, where the implementation of appropriate CS-coffee practices is linked to landscape-wide processes of integrated landscape management, seizing on the particular opportunities of the coffee farming sector. In this respect, financing options may especially be studied further. As a first step, one may think of a climate-smart coffee label, which may be oriented in the example of NAMA café. Whilst long debated, landscape certification is still scarcely found in practice (Flinzberger et al., 2020) but further on could provide a useful mechanism to finance shaping climate-smart landscapes (c.f. Ghazoul et al., 2009; Williams-Guillén and Otterstrom, 2014; Tscharntke et al., 2015; Deans et al., 2018; Harvey et al., 2021). Social science is needed to facilitate developing inclusive multi-stakeholder platforms that enable climate services (Findlater et al., 2021) to become on-ground climate action, integrating the concept of climate-smart (coffee) landscapes,. Also, using insights from social sciences and studying cultural and social landscape services can reveal interconnectedness and further beneficial effects of climate-smart coffee landscapes on psychological well-being and sense of belonging, amongst others. Moreover, although applying the concept of climate-smart landscapes may increases sustainability within farming landscapes, the reduction of trade-offs and climate impacts along the value chain shall not be forgotten, as here great carbon-reduction potentials persist (Kilian et al., 2013). In coffee, the focus will then need to be laid especially on implementing more sustainable ways of processing (Wainaina et al., 2020), which will also need to be considered in climate-smart landscapes for landscape-related commodity crops other than coffee.
Our review demonstrated how adopting climate-smart practices in coffee-farming systems provides local, landscape and global benefits. Benefits include improved water quality, biodiversity conservation and habitat connectivity, as well as stabilized regional climate patterns. These benefits show that opportunities exist to reconcile export-orientated farming with local ecosystem services. When landscape wide coordination is assured for, the multiple interests in coffee landscapes can be met and conflicts avoided. Local farmers, policy-makers and global donors must unite to improve uptake of climate-smart coffee practices in a coordinated way, to thereby augment and safeguard coffee-farming's socio-ecological system along with associated local, landscape and global benefits.
Author Contributions
Initial idea was developed by CB. Material preparation, data collection, and analysis were performed by PS. The first draft of the manuscript was written by PS, and CB commented on previous versions of the manuscript. All authors contributed to the study conception and design, read, and approved the final manuscript.
Conflict of Interest
The authors declare that the research was conducted in the absence of any commercial or financial relationships that could be construed as a potential conflict of interest.
Publisher's Note
All claims expressed in this article are solely those of the authors and do not necessarily represent those of their affiliated organizations, or those of the publisher, the editors and the reviewers. Any product that may be evaluated in this article, or claim that may be made by its manufacturer, is not guaranteed or endorsed by the publisher.
Acknowledgments
We thank Juan Francisco Estrada Delgado for his encouraging and valuable comments and specific insights on the day-to-day practices of coffee farming. We thank the reviewers and the guest editor for their detailed and helpful comments. We also acknowledge Vincent Johnson for his editorial review and helpful comments.
Supplementary Material
The Supplementary Material for this article can be found online at: https://www.frontiersin.org/articles/10.3389/fclim.2021.746139/full#supplementary-material
Footnotes
1. ^It has to be noted that what we refer to is actually “climate change mitigation” and “climate change adaptation”, but in line with the general literature we wil use the shortened terminology of “climate mitigation” and “climate adaptation” throughout this paper.
2. ^UNESCO (2011). https://whc.unesco.org/en/list/1121
3. ^UNESCO (1993). https://en.unesco.org/biosphere/lac/triunfo
4. ^UNESCO (2007). https://en.unesco.org/biosphere/lac/apaneca-ilamatepec
5. ^Gold Standard (2014). https://www.goldstandard.org/blog-item/leading-businesses-join-fairtrade-and-gold-standard-fight-against-climate-change
6. ^NAMA café (2020). https://www.namacafe.org
References
Arksey, H., and O'Malley, L. (2005). Scoping studies: Towards a methodological framework. Int. J. Soc. Res. Methodol. 8, 19–32. doi: 10.1080/1364557032000119616
Avelino, J., Romero-Gurdián, A., Cruz-Cuellar, H. F., and Declerck, F. A. J. (2012). Landscape context and scale differentially impact coffee leaf rust, coffee berry borer, and coffee root-knot nematodes. Ecol. Applic. 22, 584–596. doi: 10.1890/11-0869.1
Ball, B. C., Guimaraes, R. M. L., Cloy, J. M., Hargreaves, P. R., Shepherd, T. G., and McKenzie, B. M. (2017). Visual soil evaluation: a summary of some applications and potential developments for agriculture. Soil Tillage Res. 173, 114–124. doi: 10.1016/j.still.2016.07.006
Bastian, O., Grunewald, K., Syrbe, R.-U., Walz, U., and Wende, W. (2014). Landscape services: the concept and its practical relevance. Landsc. Ecol. 29, 1463–1479. doi: 10.1007/s10980-014-0064-5
Bastos Lima, M. G., and Persson, U. M. (2020). Commodity-centric landscape governance as a double-edged sword: the case of soy and the cerrado working group in Brazil. Front. Forests Glob. Change 3:27. doi: 10.3389/ffgc.2020.00027
Baumüller, H. (2018). The little we know: an exploratory literature review on the utility of mobile phone-enabled services for smallholder farmers. J. Int. Dev. 30, 134–154. doi: 10.1002/jid.3314
Brandt, P., Kvakić, M., Butterbach-Bahl, K., and Rufino, M. C. (2017). How to target climate-smart agriculture? Concept and application of the consensus-driven decision support framework “targetCSA”. Agric. Syst. 151, 234–245. doi: 10.1016/j.agsy.2015.12.011
Browning, D. (2019). “How many coffee farms are there in the world?” in: Technical Presentation at the Statistics Commitee. 124th Session of the International Coffee Council, Nairobi, Kenya. Available online at: http://www.ico.org/documents/cy2018-19/Presentations/statistics-item-3-enveritas.pdf (accessed June 18, 2021).
Bunn, C., Läderach, P., Ovalle Rivera, O., and Kirschke, D. (2015). A bitter cup: climate change profile of global production of Arabica and Robusta coffee. Clim. Change 129, 89–101. doi: 10.1007/s10584-014-1306-x
c&c (2015). Climate Change Adaptation in Coffee Production (p. 184). Initiative for coffee & climate (c&c). Available online at: https://toolbox.coffeeandclimate.org/wp-content/uploads/2012/10/cc-step-by-step-guide-for-climate-change-adaptation-in-coffee-production.pdf (accessed November 02, 2019).
Capitani, C., Garedew, W., Mitiku, A., Berecha, G., Hailu, B. T., Heiskanen, J., et al. (2019). Views from two mountains: exploring climate change impacts on traditional farming communities of Eastern Africa highlands through participatory scenarios. Sustain. Sci. 14, 191–203. doi: 10.1007/s11625-018-0622-x
Chandra, A., McNamara, K. E., and Dargusch, P. (2018). Climate-smart agriculture: perspectives and framings. Clim. Policy 18, 526–541. doi: 10.1080/14693062.2017.1316968
CIAT (2017). Climate Smart Coffee in Honduras. 27. Available online at: https://hdl.handle.net/10568/97530 (accessed November 02, 2019).
DaMatta, F. M., Avila, R. T., Cardoso, A. A., Martins, S. C. V., and Ramalho, J. C. (2018). Physiological and agronomic performance of the coffee crop in the context of climate change and global warming: a review. J. Agric. Food Chem. 66, 5264–5274. doi: 10.1021/acs.jafc.7b04537
Deans, H., Ros-Tonen, M. A. F., and Derkyi, M. (2018). Advanced value chain collaboration in ghana's cocoa sector: an entry point for integrated landscape approaches? Environ. Manag. 62, 143–156. doi: 10.1007/s00267-017-0863-y
Descroix, F., and Snoeck, J. (2009). “Environmental factors suitable for coffee cultivation,” in Coffee: Growing, Processing, Sustainable Production. A Guidebook for Growers, Processors, Traders, and Researchers, ed J. N. Wintgens (Weinheim: WILEY-VCH), 168–181.
Eakin, H., Winkels, A., and Sendzimir, J. (2009). Nested vulnerability: exploring cross-scale linkages and vulnerability teleconnections in Mexican and Vietnamese coffee systems. Environ. Sci. Policy 12, 398–412. doi: 10.1016/j.envsci.2008.09.003
Ellison, D., Morris, C. E., Locatelli, B., Sheil, D., Cohen, J., Murdiyarso, D., et al. (2017). Trees, forests and water: cool insights for a hot world. Glob. Environ. Change 43, 51–61. doi: 10.1016/j.gloenvcha.2017.01.002
Escobar-Ramírez, S., Grass, I., Armbrecht, I., and Tscharntke, T. (2019). Biological control of the coffee berry borer: main natural enemies, control success, and landscape influence. Biol. Control 136:103992. doi: 10.1016/j.biocontrol.2019.05.011
FAO (2013). Climate-Smart Agriculture Sourcebook. Food and Agriculture Organization of the United Nations (FAO). Available online at: http://www.fao.org/climate-smart-agriculture-sourcebook/en/ (accessed November 10, 2019).
Findlater, K., Webber, S., Kandlikar, M., and Donner, S. (2021). Climate services promise better decisions but mainly focus on better data. Nat. Clim. Change 11, 731–737. doi: 10.1038/s41558-021-01125-3
Fischer, J., Lindenmayer, D. B., and Manning, A. D. (2006). Biodiversity, ecosystem function, and resilience: ten guiding principles for commodity production landscapes. Front. Ecol. Environ. 4, 80–86. doi: 10.1890/1540-9295(2006)004[0080:BEFART]2.0.CO;2
Flinzberger, L., Zinngrebe, Y., and Plieninger, T. (2020). Labelling in Mediterranean agroforestry landscapes: a Delphi study on relevant sustainability indicators. Sustain. Sci. 15, 1369–1382. doi: 10.1007/s11625-020-00800-2
Freeman, O. E. (2015). “Characterising multifunctionality in climate-smart landscapes.” in Climate-Smart Landscapes. Multifunctionality in Practice, eds P. A. Minang, M. van Noordwijk, O. E. Freeman, C. Mbow, J. Leeuw, and D. Catacutan [Nairobi: World Agroforestry Centre (ICRAF)], 37–50.
Ghazoul, J., Garcia, C., and Kushalappa, C. G. (2009). Landscape labelling: a concept for next-generation payment for ecosystem service schemes. Forest Ecol. Manag. 258, 1889–1895. doi: 10.1016/j.foreco.2009.01.038
GLP (2005). Science Plan and Implementation Strategy (Text No. 53; IGPB Report, p. 64). IGPB Secretariat. Available online at: https://digital.library.unt.edu/ark:/67531/metadc12009/ (accessed June 17, 2021).
Goldman, R. L., Thompson, B. H., and Daily, G. C. (2007). Institutional incentives for managing the landscape: inducing cooperation for the production of ecosystem services. Ecol. Econ. 64, 333–343. doi: 10.1016/j.ecolecon.2007.01.012
Grabs, J., and Ponte, S. (2019). The evolution of power in the global coffee value chain and production network. J. Econ. Geogr. 19, 803–828. doi: 10.1093/jeg/lbz008
Harvey, C. A., Chacon, M., Donatti, C. I., Garen, E., Hannah, L., Andrade, A., et al. (2014). Climate-smart landscapes: opportunities and challenges for integrating adaptation and mitigation in tropical agriculture. Conserv. Lett. 7, 77–90. doi: 10.1111/conl.12066
Harvey, C. A., Pritts, A. A., Zwetsloot, M. J., Jansen, K., Pulleman, M. M., Armbrecht, I., et al. (2021). Transformation of coffee-growing landscapes across Latin America. A review. Agron. Sustain. Dev. 41:62. doi: 10.1007/s13593-021-00712-0
Harvey, C. A., Saborio-Rodríguez, M., Martinez-Rodríguez, M. R., Viguera, B., Chain-Guadarrama, A., Vignola, R., et al. (2018). Climate change impacts and adaptation among smallholder farmers in Central America. Agric. Food Security 7:17. doi: 10.1186/s40066-018-0209-x
Hirons, M., Comberti, C., and Dunford, R. (2016). Valuing cultural ecosystem services. Annu. Rev. Environ. Resour. 41, 545–574. doi: 10.1146/annurev-environ-110615-085831
Hölting, L., Beckmann, M., Volk, M., and Cord, A. F. (2019). Multifunctionality assessments – more than assessing multiple ecosystem functions and services? A quantitative literature review. Ecol. Indic. 103, 226–235. doi: 10.1016/j.ecolind.2019.04.009
IPBES (2019). “Global assessment report on biodiversity and ecosystem services of the Intergovernmental Science-Policy Platform on Biodiversity and Ecosystem Services,” in IPBES Secretariat, eds E. S. Brondizio, J. Settele, S. Díaz, and H. T. Ngo. Availlable online at: https://ipbes.net/global-assessment (accessed June 18, 2020).
IPCC WG III (2014). “Chapter 1—Agriculture, Forestry and Other Land Use (AFOLU),” in Climate Change 2014: Mitigation of Climate Change. Contribution of Working Group III to the Fifth Assessment Report of the Intergovernmental Panel on Climate Change, eds E. Edenhofer, R. Pichs-Madruga, and Y. Sokona (Cambridge University Press), 811–922. Available online at: https://www.ipcc.ch/site/assets/uploads/2018/02/ipcc_wg3_ar5_chapter11.pdf (accessed June 16, 2021).
Jha, S., Bacon, C. M., Philpott, S. M., Ernesto Méndez, V., Läderach, P., and Rice, R. A. (2014). Shade coffee: update on a disappearing refuge for biodiversity. Bioscience 64, 416–428. doi: 10.1093/biosci/biu038
Jha, S., Bacon, C. M., Philpott, S. M., Rice, R. A., Méndez, V. E., and Läderach, P. (2011). “A review of ecosystem services, farmer livelihoods, and value chains in shade coffee agroecosystems,” in Integrating Agriculture, Conservation and Ecotourism: Examples from the Field, Vol. 1, eds W. B. Campbell, and S. Lopez Ortiz (Springer Science+Business Media B.V.), 141–208. doi: 10.1007/978-94-007-1309-3_4
Kilian, B., Rivera, L., Soto, M., and Navichoc, D. (2013). Carbon footprint across the coffee supply chain: the case of costa rican coffee. J. Agric. Sci. Technol. 3, 151–170. Available online at: http://www.davidpublisher.com/index.php/Home/Article/index?id=15477.html
Kumari, R., de Sherbinin, A., Jones, B., Bergmann, J., Clement, V., Ober, K., et al. (2018). Internal Climate Migration in Latin America (No. 3; Policy Note). The World Bank. Available online at: https://documents1.worldbank.org/curated/en/983921522304806221/pdf/124724-BRI-PUBLIC-NEWSERIES-Groundswell-note-PN3.pdf (accessed November 05, 2019).
Läderach, P., Lundy, M., Jarvis, A., Ramirez, J., Portilla, E. P., Schepp, K., et al. (2011). “Predicted impact of climate change on coffee supply chains,” in The Economic, Social and Political Elements of Climate Change, Vol. 323, ed W. L. Filho (Springer), 703–723. doi: 10.1007/978-3-642-14776-0_42
Läderach, P., Ramirez–Villegas, J., Navarro-Racines, C., Zelaya, C., Martinez–Valle, A., and Jarvis, A. (2017). Climate change adaptation of coffee production in space and time. Clim. Change 141, 47–62. doi: 10.1007/s10584-016-1788-9
Lipper, L., Thornton, P., Campbell, B. M., Baedeker, T., Braimoh, A., Bwalya, M., et al. (2014). Climate-smart agriculture for food security. Nat. Clim. Change 4, 1068–1072. doi: 10.1038/nclimate2437
Locatelli, B., Pavageau, C., Pramova, E., and Di Gregorio, M. (2015). Integrating climate change mitigation and adaptation in agriculture and forestry: opportunities and trade-offs. Wiley Interdiscipl. Rev. Clim. Change 6, 585–598. doi: 10.1002/wcc.357
Lynch, C. (2019). The Impacts of Warming Coffee: The Climate Change-Coffee-Migration Nexus in the Northern Triangle of Central America (No. 3008; Independent Study Project (ISP) Collection., p. 32). Available online at: https://digitalcollections.sit.edu/isp_collection/300 (accessed June 17, 2021).
Malik, A. D., Pareskit, S., and Withaningsih, S. (2019). Exploring drivers' interaction influencing the adoption of sustainable coffee production system through a qualitative system dynamics modelling, case study: smallholder coffee plantation in Pangalengan, Bandung, Indonesia. IOP Conf. Ser. Earth Environ. Sci. 306:012007. doi: 10.1088/1755-1315/306/1/012007
Martin, D. A., Osen, K., Grass, I., Hölscher, D., Tscharntke, T., Wurz, A., et al. (2020). Land-use history determines ecosystem services and conservation value in tropical agroforestry. Conserv. Lett. 13:e12740. doi: 10.1111/conl.12740
McCarthy, N. (2014). Climate-Smart Agriculture in Latin America: Drawing on Research to Incorporate Technologies to Adapt to Climate Change (IDB-TN-652; Technical Note, p. 68). Inter-American Development Bank. Available online at: https://publications.iadb.org/en/climate-smart-agriculture-latin-america-drawing-research-incorporate-technologies-adapt-climate (accessed November 02, 2019).
McCarthy, N., Lipper, L., and Branca, G. (2011). Climate Smart Agriculture: Smallholder Adoption and Implications for Climate Change Adaptation and Mitigation [Mitigation of Climate Change in Agriculture (MICCA)]. Food and Agriculture Organization of the United Nations (FAO). Available online at: http://www.fao.org/3/i2575e/i2575e00.pdf (accessed January 25, 2020).
MEA (2003). Ecosystems and Human Well-Being—A Framework for Assessment. Island Press. Available online at: https://pdf.wri.org/ecosystems_human_wellbeing.pdf (accessed June 05, 2020).
MEA (2005). Ecosystems and Human Well-Being: Synthesis. Island Press. Available online at: http://www.millenniumassessment.org/documents/document.356.aspx.pdf (accessed June 05, 2020).
Moguel, P., and Toledo, V. M. (1999). biodiversity conservation in traditional coffee systems of Mexico. Conserv. Biol. 13, 11–21. doi: 10.1046/j.1523-1739.1999.97153.x
Mosedale, J. R., Abernethy, K. E., Smart, R. E., Wilson, R. J., and Maclean, I. M. D. (2016). Climate change impacts and adaptive strategies: lessons from the grapevine. Glob. Change Biol. 22, 3814–3828. doi: 10.1111/gcb.13406
Mueller, L., Schindler, U., Mirschel, W., Shepherd, T. G., Ball, B. C., Helming, K., et al. (2011). “Assessing the productivity function of soils,” in Sustainable Agriculture, Vol. 2, eds E. Lichtfouse, M. Hamelin, M. Navarrete, and P. Debaeke (Dordrecht: Springer), 743–760. doi: 10.1007/978-94-007-0394-0_33
Muller, R., Berry, D., Avelino, J., and Bieysse, D. (2009). “Coffee diseases,” in Coffee: Growing, Processing, Sustainable Production. A Guidebook for Growers, Processors, Traders, and Researchers, ed J. N. Wintgens (WILEY-VCH), 495–549.
Öborn, I., Kuyah, S., Jonsson, M., Dahlin, A. S., Mwangi, H., and de Leeuw, J. (2015). “Landscape-level constraints and opportunities for sustainable intensification in smallholder systems in the tropics,” in Climate-Smart Landscapes. Multifunctionality in Practice, eds P. A. Minang, M. van Noordwijk, O. E. Freeman, C. Mbow, J. Leeuw, and D. Catacutan [Nairobi: World Agroforestry Centre (ICRAF)], 163–178.
Pendrill, F., Persson, U. M., Godar, J., Kastner, T., Moran, D., Schmidt, S., et al. (2019). Agricultural and forestry trade drives large share of tropical deforestation emissions. Glob. Environ. Change 56, 1–10. doi: 10.1016/j.gloenvcha.2019.03.002
Pham, Y., Reardon-Smith, K., Mushtaq, S., and Cockfield, G. (2019). The impact of climate change and variability on coffee production: a systematic review. Clim. Change 7, 609–630. doi: 10.1007/s10584-019-02538-y
Pimm, S. L., Jenkins, C. N., Abell, R., Brooks, T., Gittleman, J. L., Joppa, L. N., et al. (2014). The biodiversity of species and their rates of extinction, distribution, and protection. Science. 344:1246752. doi: 10.1126/science.1246752
Prager, S., and Pfeifer, C. (2015). Network approaches for understanding rainwater management from a social-ecological systems perspective. Ecol. Soc. 20:13. doi: 10.5751/ES-07950-200413
Rahn, E., Läderach, P., Baca, M., Cressy, C., Schroth, G., Malin, D., et al. (2014). Climate change adaptation, mitigation and livelihood benefits in coffee production: where are the synergies? Mitig. Adapt. Strat. Glob Change 19, 1119–1137. doi: 10.1007/s11027-013-9467-x
Reed, J., van Vianen, J., Deakin, E. L., Barlow, J., and Sunderland, T. (2016). Integrated landscape approaches to managing social and environmental issues in the tropics: learning from the past to guide the future. Glob. Change Biol. 22, 2540–2554. doi: 10.1111/gcb.13284
Rosenzweig, C., and Tubiello, F. N. (2007). Adaptation and mitigation strategies in agriculture: an analysis of potential synergies. Mitig. Adapt. Strat. Glob. Change 12, 855–873. doi: 10.1007/s11027-007-9103-8
Sachs, J., Cordes, K., Rising, J., Toledano, P., and Maennling, N. (2019). Ensuring Economic Viability an Sustainability of Coffee Production. 155. Available online at: http://ccsi.columbia.edu/files/2018/04/Ensuring-Economic-Viability-and-Sustainability-of-Coffee-Production-CCSI-2019.pdf (accessed December 14, 2019).
Sayer, J., Sunderland, T., Ghazoul, J., Pfund, J.-L., Sheil, D., Meijaard, E., et al. (2013). Ten principles for a landscape approach to reconciling agriculture, conservation, and other competing land uses. Proc. Natl. Acad. Sci. U. S. A. 110, 8349–8356. doi: 10.1073/pnas.1210595110
Scherr, S. J., Shames, S., and Friedman, R. (2012). From climate-smart agriculture to climate-smart landscapes. Agric. Food Security 1:12. doi: 10.1186/2048-7010-1-12
Schnabel, F., Melo Virginio Filho, E., Xu, S., Fisk, I. D., Roupsard, O., and Haggar, J. (2018). Shade trees: a determinant to the relative success of organic versus conventional coffee production. Agroforestry Syst. 92, 1535–1549. doi: 10.1007/s10457-017-0100-y
Schröter, M., Koellner, T., Alkemade, R., Arnhold, S., Bagstad, K. J., Erb, K.-H., et al. (2018). Interregional flows of ecosystem services: concepts, typology and four cases. Ecosyst. Serv. 31, 231–241. doi: 10.1016/j.ecoser.2018.02.003
Silva, C. A. (2017). The coffee cultural landscape of Colombia. J. World Heritage Stud. Special Issue 44–50. doi: 10.15068/00148448
Termorshuizen, J. W., and Opdam, P. (2009). Landscape services as a bridge between landscape ecology and sustainable development. Landsc. Ecol. 24, 1037–1052. doi: 10.1007/s10980-008-9314-8
Tscharntke, T., Clough, Y., Bhagwat, S. A., Buchori, D., Faust, H., Hertel, D., et al. (2011). Multifunctional shade-tree management in tropical agroforestry landscapes—a review. J. Appl. Ecol. 48, 619–629. doi: 10.1111/j.1365-2664.2010.01939.x
Tscharntke, T., Klein, A. M., Kruess, A., Steffan-Dewenter, I., and Thies, C. (2005). Landscape perspectives on agricultural intensification and biodiversity and ecosystem service management. Ecol. Lett. 8, 857–874. doi: 10.1111/j.1461-0248.2005.00782.x
Tscharntke, T., Milder, J. C., Schroth, G., Clough, Y., DeClerck, F., Waldron, A., et al. (2015). Conserving biodiversity through certification of tropical agroforestry crops at local and landscape scales. Conserv. Lett. 8, 14–23. doi: 10.1111/conl.12110
Tscharntke, T., Tylianakis, J. M., Rand, T. A., Didham, R. K., Fahrig, L., Batáry, P., et al. (2012). Landscape moderation of biodiversity patterns and processes—eight hypotheses. Biol. Rev. Cambridge Philos. Soc. 87, 661–685. doi: 10.1111/j.1469-185X.2011.00216.x
UNESCO (2011). Biosphere Reserve Information—Ethiopia: Yayu Coffee Forest. Available online at: http://www.unesco.org/new/fileadmin/MULTIMEDIA/HQ/SC/pdf/sc_mab_Ethiopia_Yayu_EN.pdf (accessed February 09, 2020).
van Rikxoort, H., Schroth, G., Läderach, P., and Rodríguez-Sánchez, B. (2014). Carbon footprints and carbon stocks reveal climate-friendly coffee production. Agron. Sustain. Dev. 34, 887–897. doi: 10.1007/s13593-014-0223-8
Verburg, R., Rahn, E., Verweij, P., van Kuijk, M., and Ghazoul, J. (2019). An innovation perspective to climate change adaptation in coffee systems. Environ. Sci. Policy 97, 16–24. doi: 10.1016/j.envsci.2019.03.017
Wainaina, P., Minang, P., and Duguma, L. (2020). Application of the TEEB for Agriculture and Food (TEEBAgriFood) Framework; Case of Cocoa and Coffee Agroforestry Value Chains in Ghana and Ethiopia (p. 103). World Agroforestry Centre (ICRAF), TEEB for Agriculture and Food, UNEP. Available online at: http://teebweb.org/our-work/agrifood/further-analysis/teebagrifood-africa/ghana-ethiopia-agroforestry/ (accessed February 21, 2020).
Williams-Guillén, K., and Otterstrom, S. (2014). “Market-based incentives for the conservation of ecosystem services in agricultural landscapes: examples from coffee cultivation in Latin America,” in Encyclopedia of Agriculture and Food Systems, ed N. K. van Alfen (Amsterdam: Elsevier), 172–185. doi: 10.1016/B978-0-444-52512-3.00011-5
Wintgens, J. N., and Descroix, F. (2009). “Establishing a coffee plantation,” in Coffee: Growing, Processing, Sustainable Production. A Guidebook for Growers, Processors, Traders, and Researchers, ed J. N. Wintgens (Weinheim: WILEY-VCH), 182–249.
Keywords: resilience, climate-smart landscapes, coffee, climate change adaptation, climate change mitigation, socio-ecological system, landscape services
Citation: Schmidt PG and Bunn C (2021) Coordinated Implementation of Climate-Smart Practices in Coffee Farming Increases Benefits at Farm, Landscape and Global Scale. Front. Clim. 3:746139. doi: 10.3389/fclim.2021.746139
Received: 23 July 2021; Accepted: 04 November 2021;
Published: 25 November 2021.
Edited by:
Delia Catacutan, World Agroforestry Centre, IndonesiaReviewed by:
Jeetendra Prakash Aryal, International Maize and Wheat Improvement Center, MexicoSurendra Singh, Central University of Jammu, India
Copyright © 2021 Schmidt and Bunn. This is an open-access article distributed under the terms of the Creative Commons Attribution License (CC BY). The use, distribution or reproduction in other forums is permitted, provided the original author(s) and the copyright owner(s) are credited and that the original publication in this journal is cited, in accordance with accepted academic practice. No use, distribution or reproduction is permitted which does not comply with these terms.
*Correspondence: Paul Günter Schmidt, cC1zY2htaWR0QG1haWxib3gub3Jn