- 1Department of Soil, Plant and Food Sciences, University of Bari Aldo Moro, Bari, Italy
- 2Department of Civil, Environmental, Land, Construction and Chemistry Engineering, Polytechnic of Bari, Bari, Italy
- 3Department of Environment, Landscape and Urban Quality, Puglia Region, Bari, Italy
- 4Department of Biosciences, Biotechnology and Environment, University of Bari Aldo Moro, Bari, Italy
This study addresses the imperative need for adaptation strategies to climate impacts in coastal areas, particularly vulnerable to climate change effects. Under the Interreg project “AdriaClim,” our research aims to provide a comprehensive account of predicted climate impacts and corresponding adaptation measures for three key sectors for the coastal economy of the Puglia region (Southern Italy): coastal erosion, aquaculture, and tourism. Analyzing regional climate projections, predicted climatic anomalies for Apulia have been delineated. Together with a comprehensive description of the investigated sectors, the expected sectoral climate impacts have been identified. The respective adaptation measures for each sector have been evaluated in terms of coherence with the studied context and effectiveness, among those proposed in the literature. In aquaculture, climate change may influence ecosystem structure, functionality, production cycles, infrastructure, and organism physiology. Integrated multitrophic aquaculture (IMTA) emerges as a solution to enhance the resilience of Apulian aquatic production systems to climate change. For coastal tourism, the vulnerability to various climate impacts is emphasized. These impacts can directly influence tourist flows or indirectly alter the attractiveness of the Apulian Adriatic coast. Given the sector's strong ties to anthropic, natural, and cultural elements, a comprehensive adaptive approach proves most effective. Concerning coastal erosion, results underscore the prevalence of numerous stretches of eroding low coastline, with future projections indicating scenarios of widespread retreat along the southern Adriatic coast. Consequently, protective, and coastal management measures become imperative. The analyses and the obtained results serves as a valuable knowledge framework essential for formulating a climate change adaptation plan for the Apulian Adriatic coast, aligning with the regional authorities' orientations.
1 Introduction
Ecosystems and human communities have already experienced the early consequences of climate change (Johnson and Haynes, 2023; Mirón et al., 2023; Neale et al., 2023). Human activities, primarily attributable to the emission of greenhouse gases, have caused global warming, resulting in a sensible increase in the Earth's surface temperature on a global scale (IPCC, 2023). Human-caused climate change has manifested in the alteration of numerous weather and climate extremes worldwide. Consequently, this has engendered pervasive adverse consequences on aspects such as food and water security, human health, and the socio-economic fabric, giving rise to concomitant losses and damages (IPCC, 2023).
Coastal areas are particularly vulnerable to the effects of climate change (Hinkel and Klein, 2009; Torresan et al., 2012; IPCC, 2023). For numerous coastal countries and communities, coastal zones represent a paramount region, given the critical infrastructure, the increasing urbanization processes, the significant economic activity, and amenities it accommodates (IPCC, 2007, 2014; Nicholls et al., 2016). This heightens the exposure of these areas to the effects of climate change (Neumann et al., 2015; Vousdoukas et al., 2018). The global temperature increase has resulted in the melting of glaciers and polar ice caps, leading to rising sea levels that pose a threat to lower-lying coastal areas (Vousdoukas et al., 2020; Wang et al., 2024). Sea level rise caused an increase in erosion and coastal flooding events, causing land loss and modifications to the coastal landscape (Hinkel and Klein, 2009; Torresan et al., 2012). Consequently, these changes have diverse adverse effects on coastal regions, including the destruction of vital ecosystems (Brown et al., 2013). Global warming is altering oceanic circulation patterns, causing significant variations in the overall number and distribution of marine species. The rise in atmospheric CO2 concentrations is responsible for an increase in ocean acidity, while changes in migratory routes can have a cascading impact on fishing, raising concerns about potential food insecurity (Wang et al., 2024).
Furthermore, climate change effects manifest in an increase in the frequency and intensity of extreme weather events impacting coastal areas (Trenberth et al., 2015; Laino and Iglesias, 2023). These events result in health issues, irreversible property damage, and biodiversity loss (Seneviratne et al., 2012). Extreme weather events are typically characterized by windstorms, storm surges, heavy rainfall, or prolonged periods of drought (Laino and Iglesias, 2023). Temperature and precipitation variations can reduce water availability, contribute to eutrophication, and promote wildfires, algal blooms, and fish die-offs (Laino and Iglesias, 2023). In this context, adaptive actions are becoming increasingly urgent to avert the irreversible consequences of climate change and leverage development opportunities (Abbass et al., 2022; IPCC, 2023). It is crucial to integrate scientific knowledge with proactive adaptation and mitigation measures to promote coastal resilience and ensure sustainable coastal development amidst ongoing climate changes (Brown et al., 2013; Laino and Iglesias, 2023; Wang et al., 2024).
Numerous adaptation options exist, but their proper implementation hinges on the capacity and effectiveness of governance and decision-making processes, as well as advancements in scientific research on the subject (IPCC, 2023). The adoption of climate adaptation strategies for coastal areas constitutes a sound approach to mitigate or prevent social, economic, and environmental losses attributable to climate change. This involves leveraging, whenever feasible, development opportunities arising from these strategies.
The evolution of global climate policies reveals a significant shift toward recognizing of adaptation as a crucial strategy for addressing the negative effects of climate change. Initially overshadowed by the mitigation efforts in the mid-1980s to the early years after the Rio Agreements (UNFCCC) in 1992, adaptation gained prominence due to uncertainties in mitigation effectiveness and rising greenhouse gas concentrations. This shift is evident in the Intergovernmental Panel on Climate Change (IPCC) reports, with minimal attention to adaptation in the first report in 1992 (IPCC, 1992) to an entire dedicated volume in 2001 (IPCC, 2001) and a focus on limits and the need for further research in the 2023 report (IPCC, 2023). The Paris Agreement in 2015 officially acknowledged adaptation policies as essential alongside mitigation strategies (Lesnikowski et al., 2017).
At the European level, climate adaptation took its first steps with the 2013 EU Climate Change Adaptation Strategy, which was later updated in 2021 as the “New EU Climate Change Adaptation Strategy” (European Commission, 2020). This new strategy acknowledges the crucial role of each Member State in implementing adaptation proposals through the development of strategies, action plans, and monitoring and evaluation schemes. Local adaptation is especially crucial, a focus that the EU seeks to bolster through projects and initiatives, including those carried out under the Covenant of Mayors. This initiative, established in 2015, supports regional and local entities committed to developing planning tools for climate change adaptation and mitigation (EU, 2015).
In 2015, Italy adopted its own National Strategy for Adaptation to Climate Change (SNACC), driven by the Ministry for Environment, Land, and Sea Protection (MATTM). The SNACC is the first document setting out the vision and general objectives to be achieved to initiate an adaptation pathway in Italy. It consolidates existing knowledge regarding sector-specific impacts (environmental, social, and economic) using key concepts. Furthermore, it offers a range of proposals for adaptation actions across various sectors, accompanied by criteria for evaluating priority actions. SNACC serves as an informative document, while the primary tool for executing the National Strategy is the National Climate Change Adaptation Plan - PNACC (MASE, 2023). The PNACC houses a shared database of data, information, and analytical methodologies essential for regional and local administrators to identify sector-specific impacts and related adaptation actions. Effectively adapting to climate impacts necessitates intricate planning and a robust governance system to guide stakeholders in setting specific objectives and implementing adaptation measures.
At the local level, the Regional council with DGR n. 1187 of 08/08/2023 took note of the “Guidelines for the drafting of the Regional Strategy for adaptation to climate change— SRACC” and the “Regional Guidelines for the drafting of Action Plans for Sustainable Energy and the Climate (PAESC)” and approved the “Plan for adaptation to climate change for the Adriatic coastal areas of Puglia” resulting from the AdriaClim project. These documents represent a valid and organic analysis of the Apulian knowledge on climate matters, with a significant and transversal strategic value for the broader areas of application at a regional level. These studies also envisage the main dangers linked to climate change for the Apulian context, proposing a set of adaptation actions, classified with reference to the territorial area analyzed. The adopted Plan is expected to serve as a useful guideline for the drafting of Local Action Plans for Sustainable Energy and Climate (SECAPs), which are a local planning tool under municipal jurisdiction within the European process of the Covenant of Mayors for Climate and Energy. For each municipality in Puglia, a detailed climate analysis has been defined in order to provide a preliminary climate scenario analysis, as "pre-processed" data for the assessment of climate risk and vulnerability. Furthermore, Apulia Region, in 2022, financed the definition of SECAPs through the provision of vouchers with an economic commitment of approximately 1 million euros.
Research on coastal adaptation solutions is poor and fragmented (Laino and Iglesias, 2023). One possible explanation lies in the intricate nature of coastal adaptation solutions that typically demand a multidisciplinary approach and extensive collaboration among researchers, policymakers, and stakeholders (i.e., Mani-Peres et al., 2016; Fatorić et al., 2017; IRGC, 2020; Bruno et al., 2021). Research efforts aimed at developing viable adaptation solutions in coastal regions also necessitate substantial financial investments and logistical support (Laino and Iglesias, 2023).
This paper presents some of the research activities conducted in the EU research project “Climate Change Information, Monitoring, and Management Tools for Adaptation Strategies in Adriatic Coastal Areas” (known as AdriaClim). The primary objective of the AdriaClim project is to collect valuable information for planning and implementing adaptation measures for marine and coastal areas along the Adriatic Sea in both Italy and Croatia. Within this project, an investigation was conducted into three pivotal sectors along the Apulian Adriatic coast: aquaculture, tourism, and coastal erosion. A comprehensive analysis was carried out, utilizing accessible data for each sector along with regional climate projections, to identify sector-specific risks associated with climate change. The formulation of adept adaptation measures for the identified impacts was executed by referencing data present in both scientific and gray literature. The proposed measures were conceptualized with both a sectoral and cross-sectoral perspective, tailored to the distinctive context of Apulia.
This paper presents some of the research activities conducted in the EU research project “Climate Change Information, Monitoring, and Management Tools for Adaptation Strategies in Adriatic Coastal Areas” (known as AdriaClim). The primary objective of the AdriaClim project is to monitor climate change, collecting valuable information for the purpose of planning and implementing measures aimed at enhancing the adaptability of the marine and coastal areas along the Adriatic Sea in both Italy and Croatia. Within this project, three key sectors of the Apulian Adriatic coast, namely aquaculture, tourism, and coastal erosion, have been thoroughly investigated. All available data concerning the three fields were analyzed create a framework that was as comprehensive and representative as possible of the economic, social, and territorial realities under examination, in addition to regional climate projections. Potential risks associated with climate change have been identified, and adaptation measures have been developed to address the anticipated challenges and harness potential development opportunities. These measures have been designed with a sector-specific and cross-sectoral perspective tailored to the unique context of Apulia. On the other hand, such a complex context requires a significant variety of adaptation measures, which can also be adopted individually in simpler cases.
2 Study area
The regional territory of Apulia region, situated in the easternmost part of the Italian peninsula, is characterized by highly varied topography as illustrated in Figure 1A. In northern Apulia, there is a large alluvial plain, known as Tavoliere, bordered to the west by the Monti Dauni and to the northeast by the Gargano promontory. In central Apulia, there is an extensive hilly region oriented toward NW-SE, referred to as the Murge. To the NE, the Murge slopes toward the Adriatic Sea, delimiting a narrow and flat coastal strip, known as the “Costa centrale Adriatica”. On the other hand, the Arco Ionico is identified with a karst territory where the Murge degrades toward the Ionian Sea, crossed by numerous eroding grooves known as “Gravine”. The Valle dellOfanto marks the border between the northern and central portions of the region. The southern part of Apulia, called Salento is occupied by a large plain and a modest hilly system to the south. It exhibits significant territorial discontinuity and a prominent coastline (about 1035 km long inclusive of the Tremiti Islands), along the Adriatic and the Ionian Seas (Figure 1B).
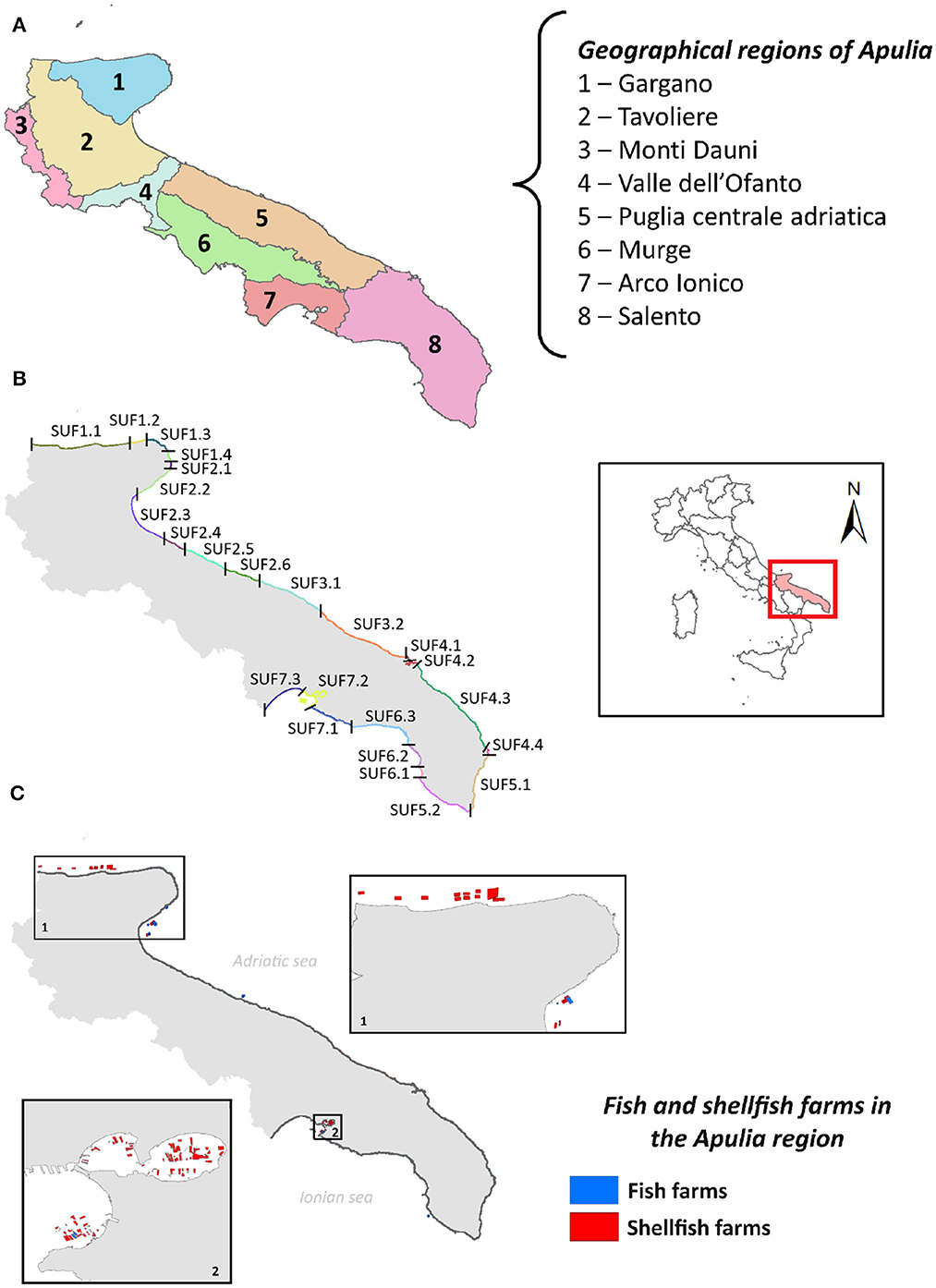
Figure 1. Geographical regions of Apulia (A), Apulian coastal physiographic sub-units (B), and map of fish and shellfish farms occurring in Apulian region (C).
Gargano, Monti Dauni, and the hinterland of the Arco Ionico and Murge regions showcase the primary forest formations of the Apulia region. These formations are characterized by deciduous oaks and reforestation cores. Along the Apulian coast, one can find numerous forest-protected areas, coastal reforestations, Mediterranean thickets, and scrublands (Elia et al., 2019). The central areas of the region are primarily dominated by the agricultural landscape component and pastures.
Due to its peculiar geographical position and topography, Apulia presents highly varied climatic conditions, both within its different regional geographical areas and in relation to the broader Mediterranean macroclimate that exerts influence over it. Hence, the assessment of future climate projections at the local level becomes essential to better define climate risks and to formulate appropriate strategies and adaptation actions for the examined sectors. The Adriatic side suffers markedly from the continental climate determined by the mountainous complexes of the northeastern sector and the vast plains of Eastern Europe progressively attenuated to the south by the influence of the eastern Mediterranean. The north-western part is influenced by the mountain climate of the nearby Apennines contrasted to the south by the Ionian Sea and the central Mediterranean. Consequently, Apulia exhibits a mosaic of climatic conditions (Macchia et al., 2000) and, along with the broader Mediterranean region, it is acknowledged as an area particularly exposed to the effects of climate change (Agency, 2012; IPCC, 2014, 2023).
Along the Apulia coastal region, aquaculture and coastal tourism are some of the most developed economic sectors. Apulia plays a prominent role in the context of Italian shellfish farming with a production approximately of 5000 tons in 2020 (MASAF, 2021) and most plants located along the northernmost Adriatic Apulian coast and in the transitional water system Mar Piccolo, within the northernmost part of the Gulf of Taranto (Figure 1C).
Tourism represents one of the most significant economic sectors in the Apulia region. In 2022, it contributed between 8.3% to 8.8% of the regional GDP (Pugliapromozione, 2023). Coastal tourism plays a key role in the regional tourist framework, with coastal destinations recording the highest numbers of arrivals and tourist stay. Among these, the Adriatic coastal resorts stand out due to their greater tourist significance. The Apulian Adriatic coastline extends approximately 725 km, encompassing the Tremiti Islands. The coastal morphology is highly diverse: roughly 29% consists of cliffs, 28% comprises sandy beaches, 26% is rocky coast, and approximately 9% features anthropogenic structures (Figure 2). This coastline spans 46 municipalities where approximately 31% of the regional population resides, resulting in a densely populated area. The combination of natural coastal areas and historic urban complexes contributes to defining the “Apulia Adriatic coast” as a destination, making the area particularly appealing to tourists.
3 Materials and methods
3.1 Data sources
Since the analysis of climate change impacts on coastal areas at regional scale requires both a detailed analysis of the changing patterns of hydro-climatic variables and the assessment of site-specific metrics in the aquaculture sector, coastal tourism and coastal erosion, climate data has been analyzed, along with all data relevant to representing the current situation in the three sectors under analysis.
The rainfall and temperature data, used to calculate climate indicators, are obtained from the gridded dataset “Dynamical Downscaling with COSMO-CLM of historical (1979/2005) and future climate (2006/2100) data under scenario RCP4.5 and RCP8.5 8km over Italy” produced by the Euro-Mediterranean Center on Climate Change (CMCC).
This dataset provides daily precipitation and temperature data on a regular grid with 8x8km resolution over Italy (Bucchignani et al., 2016; Zollo et al., 2016), generated with the regional climate model COSMO-CLM (Rockel et al., 2008) which in turn is driven by the global climate model CMCC-CM (Scoccimarro et al., 2011). The data available in the dataset covers the “historical” time frame from 1979 to 2005, and the “future” from 2006 to 2100 for the RCP8.5 and RCP4.5 scenarios. In this study, future climate analysis was conducted for the period 2021-2050.
Sea surface water temperature (SST) and sea surface height (SSH) projections have been used to forecast the climate change impact to the examined sectors.
Several models for predicting the rise in mean sea level have been developed in recent years (IPCC, 2014, 2019; Horton et al., 2018; Nicholls et al., 2018; Jevrejeva et al., 2019). All models agree in predicting a global rise in mean sea level (IPCC, 2023), and with specific reference to the Mediterranean Sea, the Adriatic Sea appears to be a hot-spot due, also, to the numerous low-lying areas that characterize the region (Marcos et al., 2016; Reimann et al., 2018).
The sea level analysis uses the global forecasts provided by Jackson and Jevrejeva (2016). SSH projections up to 2050 were estimated by using a probabilistic approach that combines the main factors contributing to SLR: long-term alteration of ocean density change, globally averaged steric sea level change, dynamic sea level change, surface mass balance of ice from glaciers and polar ice sheets, surface mass balance and ice sheet dynamics of Greenland and Antarctica, land-water storage and glacial isostatic adjustment (Jackson and Jevrejeva, 2016).
The climatic analysis of marine areas, for the historical period, uses the marine analyses produced by the European service CMEMS (Copernicus Marine Environment Monitoring Service) for the Mediterranean Sea (Simoncelli et al., 2019). These analyses are obtained through the integration of numerical models and the assimilation of cross-platform observations and represent the state of the art for the characterization of the meteorological conditions of the sea.
The expected climate anomalies in temperature have been extrapolated from climate simulation data for the period 1981-2100 obtained through the NEMO oceanic model (Madec et al., 2023) applied to the Mediterranean Sea (7 km resolution) and forced with atmospheric and hydrological data of the CMCC-CM climate model at 80 km horizontal resolution (Scoccimarro et al., 2011; Lovato et al., 2013; Galli et al., 2017; Reale et al., 2022).
Information related to Apulian aquaculture sector is not collected and systematized but scattered and often do not allow a complete reconstruction of trends in terms of productivity, revenues, number of plants as well as operators employed in the sector. Data used in this study was derived from systematic a review of gray and peer reviewed literature (i.e., Bronzi et al., 2011; Andaloro et al., 2020; Marino et al., 2020) (i.e., Parisi et al., 2014; FAO, 2015; Petrosillo et al., 2023) as well as official information provided in the National Strategic Plan for Aquaculture (MASAF, 2021).
The data collected by the Regional Tourism Agency “Pugliapromozione” has been utilized to delineate the primary characteristics of the tourism sector in the Apulia region, leading to an assessment of Adriatic coastal tourism. Specifically, data related to tourist arrivals and stay, monitored by the SPOT system (Sistema Puglia per lOsservatorio Turistico) from 1998 to 2022, have been gathered.
The “arrivals”, indicating the number of customers, whether Italian or foreign, hosted in accommodation facilities (hotels or complementary), provide insights into the popularity and tourism capacity of a given tourist destination. The “overnight stays,” representing the number of nights spent by customers in accommodation facilities, allow for an assessment of the tourist resources and markets upon which the destination relies, signifying the overall tourism appeal. In order to provide a more detailed analysis of Apulian's tourism sector, reports produced by the association of regional chambers of commerce (Unioncamere) has been considered.
The evaluation of the current status of the coastal zone, which includes determining the current coastline and its morphology, was performed using a very high-resolution (0.2 m) image dataset obtained in 2017. The analysis of coastal evolution involved overlaying the shorelines extracted from the IGM 1:25,000 cartography from 1960, along with three sets of images acquired in various years: 1992, 2005, and 2017.
The impact analysis utilized probabilistic projections of sandy shoreline dynamics by Vousdoukas et al. (2020). These projections combined factors such as projected sea level rise (SLR) under the RCP4.5 and RCP8.5 scenarios (Jackson and Jevrejeva, 2016), variations in active beach profile slope, trends in coastal environmental changes, and future changes in meteorological drivers like storm surges and waves.
3.2 Methods
Specific climate indicators, are calculated to highlight the historical climate characteristic of the Apulia climate starting from rainfall data and temperature values (MASE, 2023). All climate indicators, calculated for land and marine areas, are described in Table 1.
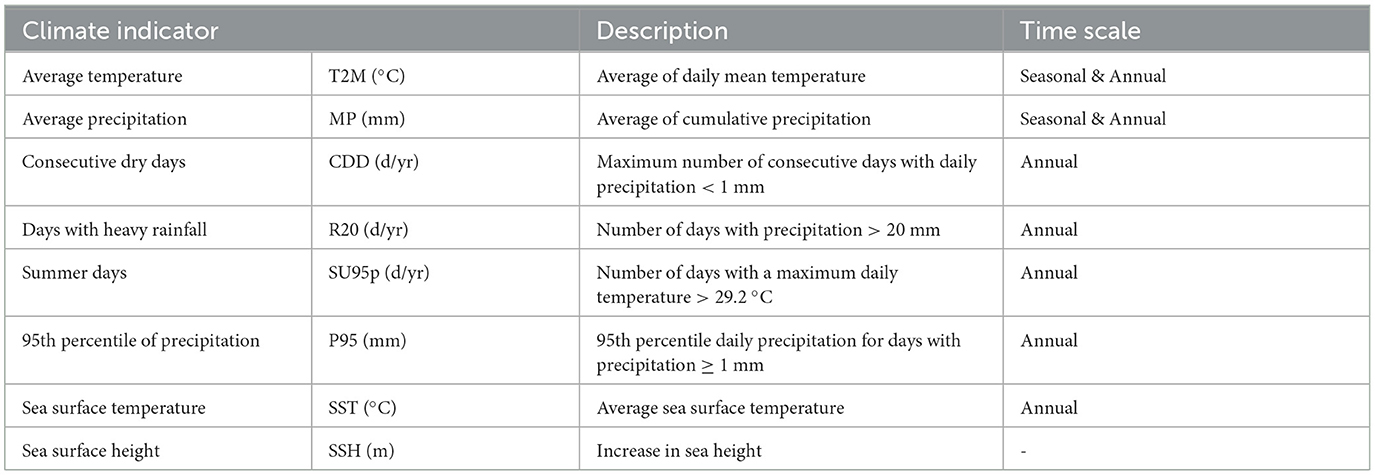
Table 1. Climate indicators adopted in the current and future climate analysis of the land and marine areas of the Apulia region.
Land and marine areas are assessed separately, with consideration for two distinct timeframes: the historical period and the future period.
Two different RCP (Representative Concentration Pathways) emission scenarios are here considered for the analysis of the future climate (IPCC, 2014):
• RCP8.5 “No Mitigation”—considers the growth of emissions at current rates. This scenario assumes, by 2100, atmospheric CO2 concentrations will triple or quadruple (840–1120 ppm) from pre-industrial levels (280 ppm);
• RCP4.5 “Moderate mitigation”—assumes the implementation of some initiatives to control emissions. It is regarded as a stabilization scenario: by 2070, CO2 emissions fall below current levels and atmospheric concentrations stabilize at about twice the pre-industrial levels by the end of the century.
Climate anomalies have been calculated by comparing current indicator values with those derived from future projections in both scenarios considered (MASE, 2023).
Methodology applied to allow the analysis context of the Aquaculture sector at national and regional levels consisted in a data mining carried out consulting specific and official websites such as https://www.politicheagricole.it or https://www.sid.mit.gov.it/documenti-piano and https://www.regione.puglia.it/. Contextually, gray and scientific literature were obtained by entering specific keywords or combinations of them such as aquaculture, Apulian, Italian, mariculture sector, sustainable development, climate changes, adaptation. Moreover, data related to Apulian fish and shellfish farms were elaborated through a GIS tool to provide an overview of aquaculture plants occurring in the region in the framework of the Italian Maritime Space Management Plan.
With the aim of investigating the dimensions and growth dynamics of the tourism sector in Apulia, a descriptive statistical analysis of regional tourism data was conducted. The data were processed to extract information regarding the monthly distribution of tourist arrivals and stays, as well as the nationality of tourists. In the absence of specific data pertaining to coastal tourism, the magnitude of tourist flows in Adriatic locations was assessed by collecting and analyzing arrival and stay data solely from municipalities along the Adriatic coast. This process facilitated the identification of key coastal tourist destinations.
Beach mapping was conducted to assess their changes, including sandy or pebbly sand beaches, as well as those along the rocky coastline, both high and low, with sandy or gravel beaches at their base. Following the beach mapping, various dimensional parameters, including surface area, length, and width, were estimated. Beach width is a crucial factor in assessing evolutionary phenomena, in conjunction with shoreline position changes.
A polygon overlay analysis was employed to identify areas where shoreline deviations exceeded 10 meters, using a threshold value of 30 meters for the period between 1960 and 1992, taking into account geolocation accuracy and map precision. The polygons meeting these criteria were utilized to extract the most recent coastline segments for further analysis. The categorization of segments as advancing or retreating was based on observed changes in these areas. Coastal morphology also influenced the selection process, with rocky coastlines and cliffs considered stable, and only the previously mapped beaches were included in the analysis of coastal evolution.
4 Results
4.1 Current framework
4.1.1 Present climate in land and marine areas
Using the daily temperature and rainfall data, the first 6 climate indicators described in Table 1 are estimated. Figure 3 shows the spatial distribution of the six climate indicators calculated for the analysis of the Apulia climate in the historical period 1979-2005.
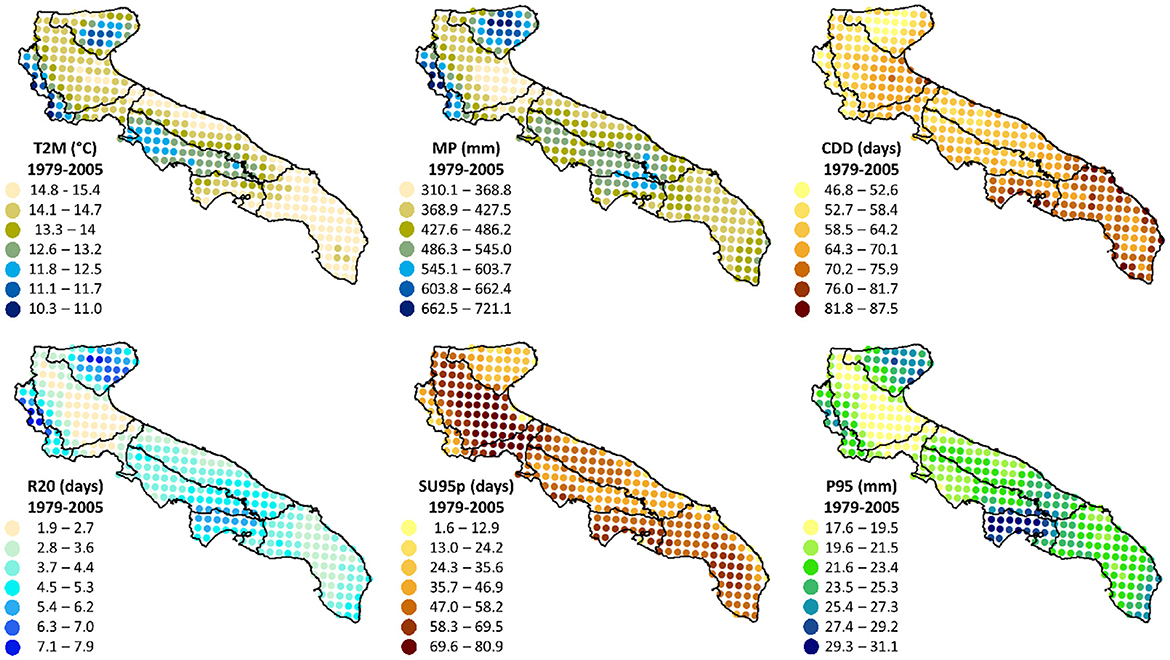
Figure 3. Annual average distribution in Apulia of the climatic indicators analyzed for the historical period 1979–2005.
As shown in Figure 3, during the historical period the average annual temperatures (T2M) vary between 10.3°C and 15.4°C, with the highest values observed in the Tavoliere, Puglia centrale Adriatica, and Salento. The average annual cumulative precipitation (MP) is between 310.1 mm/year and 721.1 mm/year, with Monti Dauni, Gargano, and Murge being the wetter areas, while Tavoliere, Valle dell'Ofanto, and Salento were drier regions. The annual average value for consecutive dry days (CDD) varies between 46.8 and 87.5 days. Tavoliere, Arco Ionico, and Salento being particularly exposed to extended dry periods. The number of days with heavy rainfall (R20), an index of extreme weather events, ranges from 1.8 days/year to 7.9 days/year. Gargano and Monti Dauni have higher frequencies of intense rainfall, followed by the central southern areas of the region (Arco Ionico). Tavoliere is less exposed to such events. The average annual number of summer days (SU95p) varies from less than 15 days/year to more than 80 days/year with the highest values in the Tavoliere. The 95th percentile of precipitation (P95) ranges between 17.6 mm and 31.1 mm, with higher values occurring in the Arco Ionico and limited areas of the Gargano.
The seasonal average temperature and precipitation values, for the historical period 1979–2005, are shown in Figure 4. The lowest average temperature values are recorded in all seasons across the main mountain area of the region including Monti Dauni, Gargano, and Murge. In contrast, Tavoliere, Salento and Puglia Centrale Adriatica recorded higher average temperatures. The highest average precipitation values are recorded during the winter season (December-February), followed by spring (March-May) and autumn (September-November), especially in areas such as Monti Dauni, Gargano, and Murge. The summer season (June-August) is characterized by low precipitation values (MP) throughout the region.
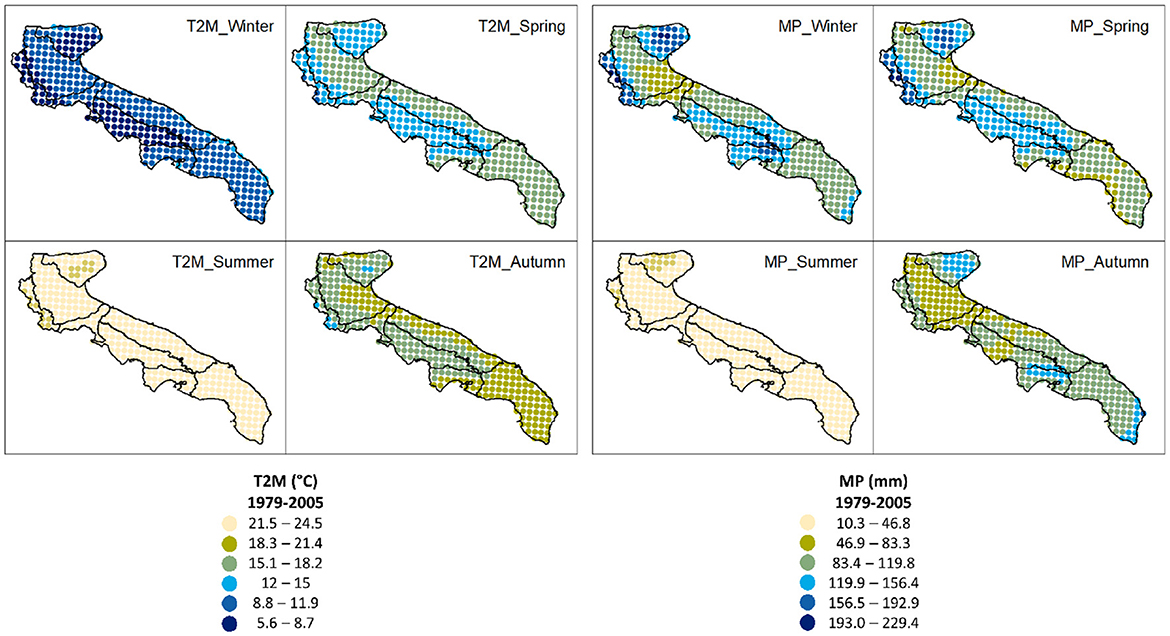
Figure 4. Distribution of the average seasonal values of average temperature (T2M) and average precipitation (MP), for the historical period 1979–2005.
Table 2 shows the average, maximum, and minimum annual values of the estimated climate indicators as well as the seasonal values of average temperature and average precipitation for the historical period.
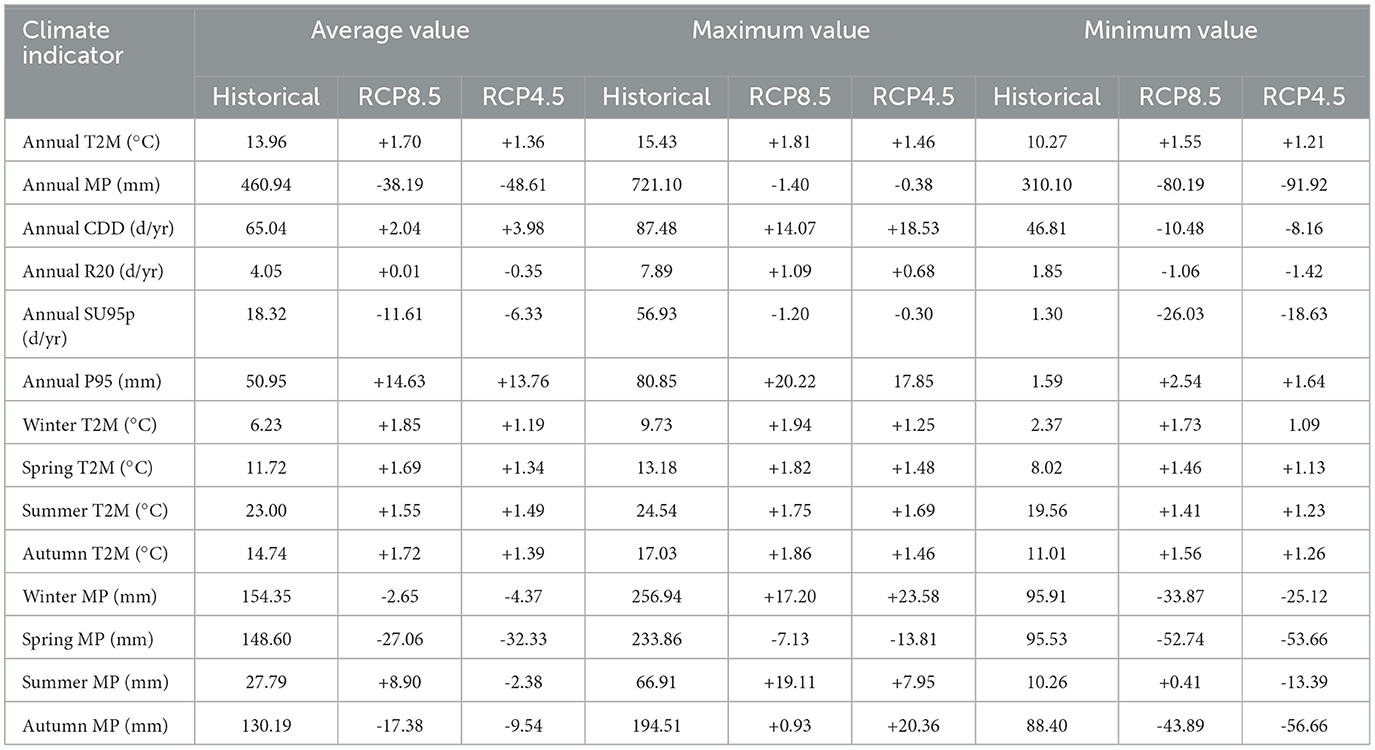
Table 2. Average, maximum, and minimum values of the climate anomalies expected for each indicator calculated for the historical period 1979–2005 and the future period 2021–2050 for RCP8.5 and RCP4.5 scenarios.
4.1.2 Aquaculture analysis
Aquaculture is a very important economic sector of food production that has been experiencing rapid growth in recent years, both globally and in the European Union. Italian aquaculture is an important sector of the national agribusiness and has grown to be a highly diversified activity because of the high environmental diversity occurring in Italy. In 2017, around 150000 tons of fresh fish, mollusks and crustaceans worth more than half a billion euro were produced in Italy. Aquaculture products account for 44% of the total volume of the national fishery sector and over 35% of its value, and during 2020 the national production contributed to the 11% of the entire EU volume (ISPRA, 2023). Aquaculture in Italy is very diverse in terms of species, rearing environments and production techniques. More than 30 species of fish, mollusks, crustaceans, and algae are bred in around 750 production facilities, located in coastal marine areas, transitional water systems and fresh waters. Most of the production, however, involves five main species: rainbow trout, in fresh waters, sea bass, sea bream, mussels and clams, in brackish and marine waters (ISPRA, 2020). Regionally, Veneto and Emilia-Romagna top the list in terms of the number of plants and production. Friuli-Venezia Giulia, Apulia and Sardinia together host 69.3% of aquaculture facilities in their territories and account for 74.3% of national production. A total of 32 farms dedicated to fish farming operate along the Apulian coast, including 23 in the province of Foggia, 1 in the province of Bari, 3 in the province of Brindisi, 4 in the province of Taranto, and 1 in the province of Lecce (Figure 1C). With regard to active offshore plants, most are located in the provinces of Foggia (4) and Taranto (3), with only one offshore plant for the provinces of BAT and Lecce respectively (Petrosillo et al., 2023). The main fish species farmed in Apulia are sea bass and gilthead seabream, as well as a small production of meager and, exceptionally, American meager.
Shellfish farming in Apulia is carried out in Taranto and along the Gargano coast. In the Gargano there are a total of 36 farms, 27 of which are located along the northern coastal area of the Gargano, including the Varano lagoon, and 9 in the waters of the Gulf of Manfredonia. Of the 36 farms, 23 practice breeding in salty waters (64%) (14 along the northern side of the Gargano and 9 in the waters of the Gulf of Manfredonia), while the remaining 13 farms practice breeding in the brackish waters of the Varano lagoon. The species most farmed offshore the North Gargano, in the Varano lagoon and the Gulf of Manfredonia along the South Adriatic coasts, are Mytilus galloprovincialis, Crassostrea gigas and Ruditapes philippinarum. The mussel production characterizes the farming in the Gulf of Taranto in the Northern Ionian Sea.
4.1.3 Coastal tourism analysis
From the analysis of available data on tourist presence, it is observed that the distinctive features of Apulias tourism include:
• Steady growth in tourist arrivals and stays:
Between 1998 and 2019, the number of tourist arrivals and stays in Apulia experienced steady growth (Figure 5A), except during the two years affected by the COVID-19 pandemic (2020–2021). During the summer of 2022, tourist arrivals and stays reached and even exceeded the values recorded in 2019. It's worth noting that the growth of Apulia's tourism sector has undoubtedly benefited from the effects of tourism planning in Apulia. Apulia was, indeed, the first region in Italy to implement a Regional Tourism Development Plan called “Puglia365”.
• High seasonality of tourism demand:
The monthly trend of tourist arrivals and presences in Apulia, monitored by the SPOT system (Sistema Puglia per l'Osservatorio Turistico), shows higher values during the summer months (Figure 5B). Seasonality has always characterized Apulia's tourist demand, with the summer season recording the highest number of total arrivals and stays. This aspect significantly influences the regional tourism offer.
• Local and national tourism market:
The Apulian tourist market has consistently attracted predominantly local and national tourists (Figure 5C). In 2019, international tourist arrivals and stays accounted for 28% of the total arrivals in Apulia, a figure that decreased to 18.2% in 2021. International tourists in Apulia primarily originate from Europe. At the provincial level, the foreign tourism specialization index (the ratio between the weight of foreign tourist stays in the province and the weight of foreign stays in Italy, both expressed in %) remains consistently below 1 in every Apulian province, indicating a low specialization in international tourism (Unioncamere-Puglia, 2022).
• Good differentiation of regional tourism supply:
The official regional tourism website, “Viaggiareinpuglia.it”, lists a variety of attractions in Apulia, both natural and man-made, classified by themes such as Arts and Culture, Tradition and Spirituality, Nature, Sports, Wellness, and Wine ad Food, with the Sea theme being the most popular. These regional tourism themes significantly contribute to the diversification of tourism offerings.
• High values of tourism pressure in coastal destinations:
Data on municipal tourism trends indicate a greater presence of tourists in coastal localities, with higher tourist pressure indices (number of presences per resident) compared to inland areas. The Adriatic coast plays a pivotal role in the Apulian tourism market. In 2021, approximately 60% of the total regional tourist arrivals and stays were recorded in Adriatic locations (Figure 5D). The tourist importance of the Adriatic coast is linked to its rich tourist offerings. In terms of infrastructure, the presence of the main regional civil ports and airports along the Adriatic coast is worth mentioning. In terms of attractions, the Adriatic itinerary offers numerous natural resources such as the Gargano National Park and coastal lagoons, the Tremiti Islands, Torre Guaceto, and the Cesine. Anthropic attractions are represented by numerous coastal villages and towns and the rich historical and artistic heritage they preserve. Lastly, in terms of hospitality and services, the available data highlight the highest number of accommodations and tourist facilities along the Adriatic resorts, some also internationally renowned.
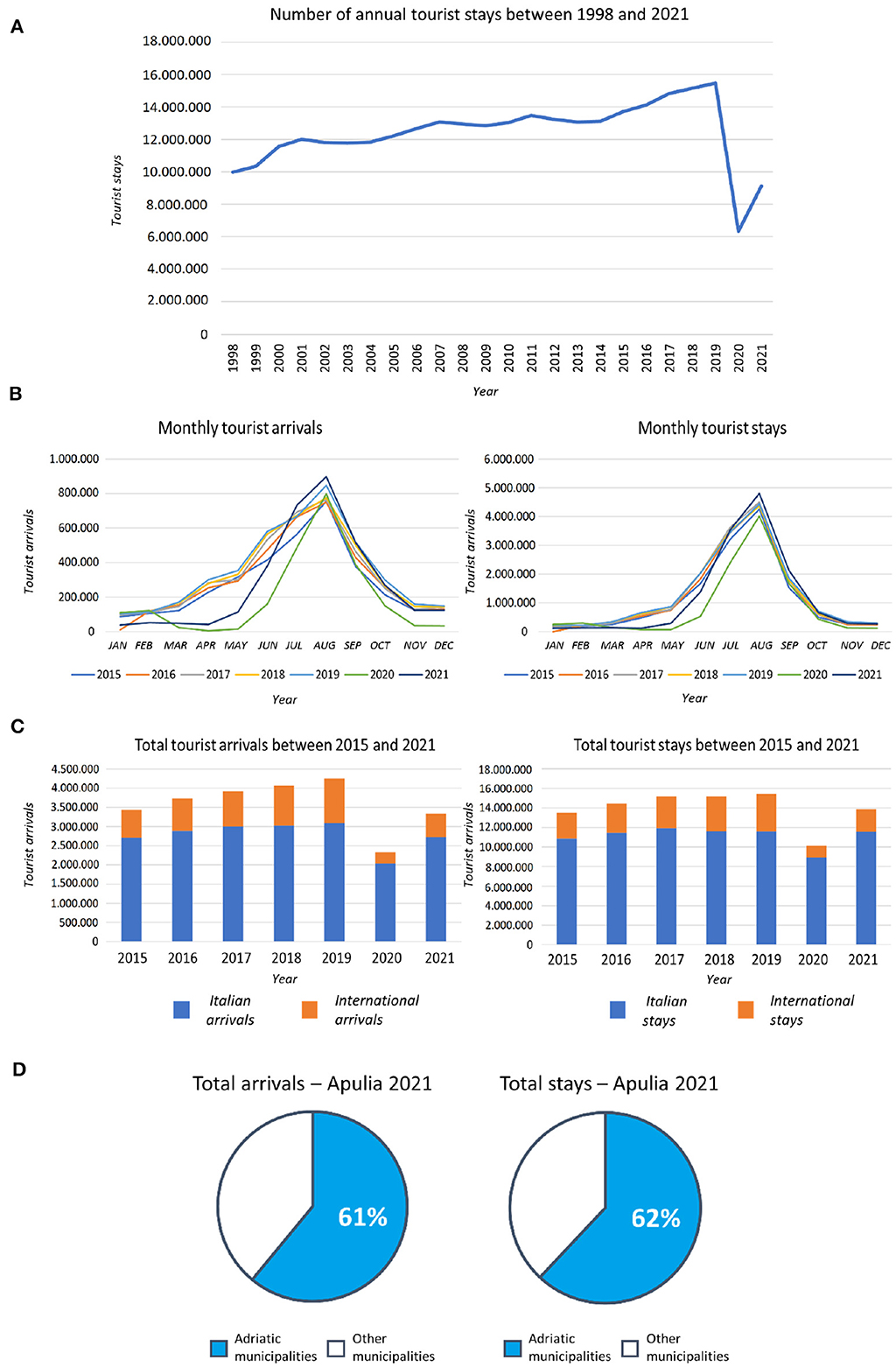
Figure 5. Apulian tourism trend analysis with Number of annual tourist stays between 1998 and 2019 (A), Monthly tourist arrivals and stays (B), Total Italian and international tourist arrivals and stays between 2015 and 2021 (C), Total tourist arrivals and stays of the Adriatic municipalities (D).
4.1.4 Coastline evolution analysis
The geometric attributes of the mapped beaches, along with their corresponding coastal Physiographic Sub-Units (SUFs), are detailed in Table 3. The beach mapping reveals that, along the Apulian Adriatic coastline, many of the beaches, especially in the coastal regions encompassing UF3 and UF4, have very narrow widths. The analysis of coastline evolution reveals significant issues with beach retreat over the examined time intervals. The aggregated findings for the Apulian Adriatic coast, as shown in Figure 6, indicate that coastal erosion is a long-standing phenomenon. Specifically, during the period from 1960 to 1992, 103 kilometers of sandy coastline (equivalent to 40% of the sandy coastline) experienced retreat due to a reduction in sediment supply to the coastal region.
From 1992 to 2005, erosive phenomena affected only 23% of the sandy coastline, equivalent to 60 kilometers of coastline. However, there was a notable increase in erosive phenomena in the most recent timeframe, from 2005 to 2017, when the lengths of eroded sandy coastline nearly doubled, reaching 97 kilometers, or approximately 38% of the total sandy coastline.
When examining the trends in various physiographic units, it is essential to highlight that UF1, UF3, and UF4 stand out as the most critical areas in terms of the percentage of eroded sandy coastline. However, it is crucial to note that the entire Adriatic side of the regional coastline is facing significant critical issues. Furthermore, UF1 and UF3 have experienced a substantial worsening in conditions during the most recent period, indicating an exacerbation of erosion in these regions.
The analysis of advancing and retreating areas reveals that an overall negative balance in different physiographic units, with some UFs experiencing substantial loss of sediments on the emerged beach. SUF1.1 and SUF3.2 exhibit the most critical situations in terms of lost areas.
A sharp and sudden worsening of the sandy shorelines became apparent between the years 2008 and 2010, as noted by Bruno et al. (2020). While the situation appeared relatively stable in 2008 compared to 2005, erosive phenomena had already significantly manifested in some SUFs. This erosive trend can be attributed to various factors, including changes in mean sea level and wave energy during the examined period (Bruno et al., 2014, 2019).
The increase in erosive phenomena is not solely due to the expansion of the phenomenon to adjacent areas already experiencing retreat (e.g., Foce Fortore, Foce Ofanto, Lecce, Cesine). Instead, new crisis situations have emerged along the coastline (e.g., Rodi Garganico, Torre Canne, Torre Guaceto) (Figure 7). Many of these new sections have exhibited a significant erosive tendency, leading to shoreline retreats of more than 30 meters. Moreover, it is important to note that the presence of coastal protection structures has, in many cases, mitigated the evolution of the trend by preventing shoreline retreat and irreversible changes to the coastline.
4.2 Future projections
Following the analysis of projected climate anomalies in the Adriatic region, potential impacts on the examined sectors have been identified using the findings from relevant scientific and gray literature.
4.2.1 Future climate trend in land and marine areas
Using future climate projection data generated by the CMCC model, expected climatic variations, referred to as climatic anomalies, have been estimated. This quantification was done by subtracting the historical average value for each calculated indicator from the anticipated average future value, with separate considerations for the RCP8.5 and RCP4.5 scenarios.
Figures 8A, B show the regional distribution of the expected climatic anomalies in the future period 2021-2050, for scenario RCP8.5 and RCP4.5 respectively. The expected climatic anomalies show an increase in average annual temperatures (T2M) across the Apulia region, with an average increase of 1.7°C in the RCP8.5 scenario and an average increase of 1.36°C in the RCP4.5 scenario. Similarly, an increase in summer days (SU95p) is projected throughout the region, reaching a maximum of 20 days/year in the RCP8.5 scenario and almost 18 days/year in the RCP4.5 scenario.
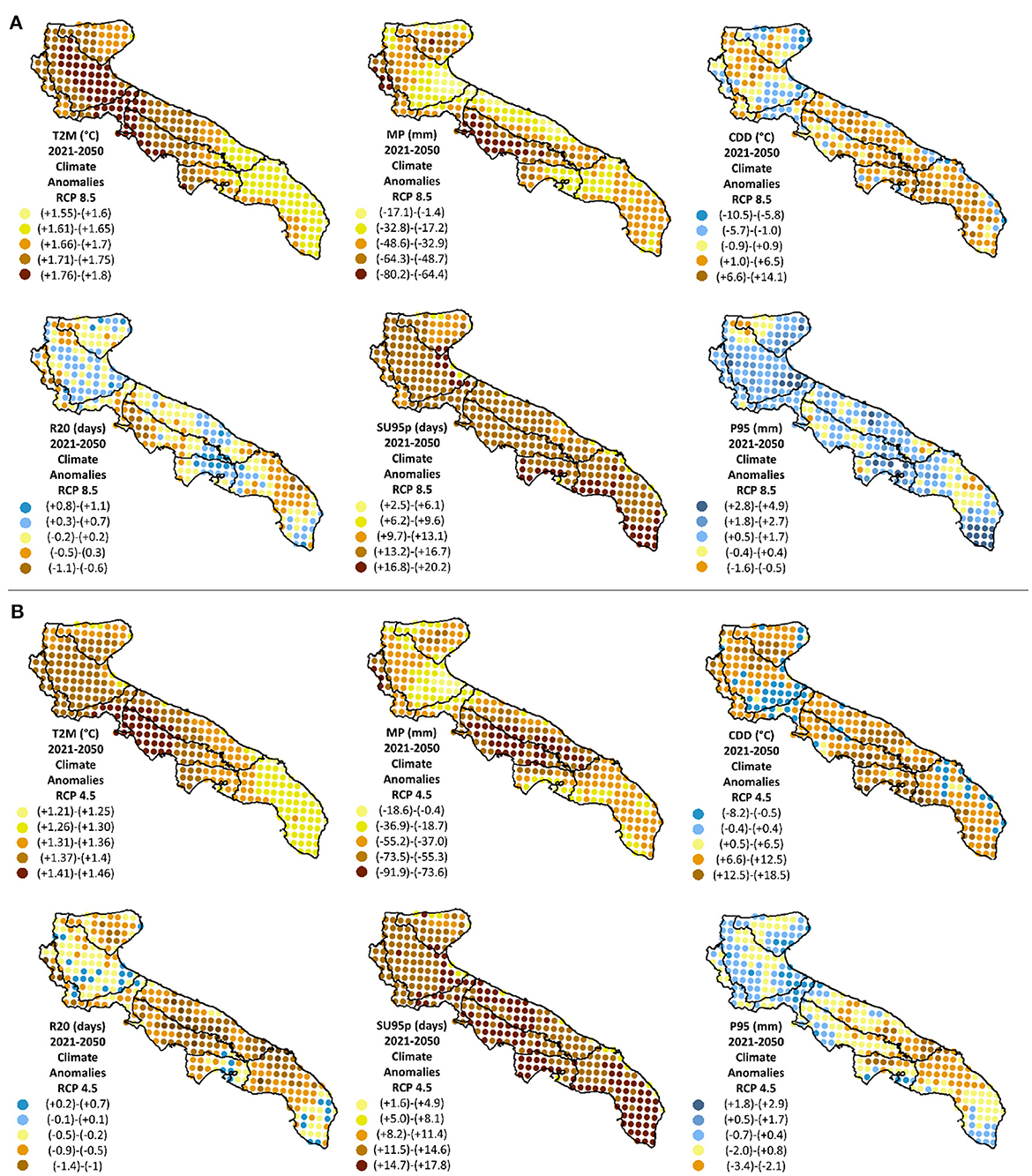
Figure 8. Regional distribution of expected climatic anomalies in the future period (2021–2050) compared to the historical period for the RCP8.5 scenario (A) and RCP4.5 scenario (B).
Regarding precipitation, both future scenarios show a reduction in average annual precipitation (MP), with a maximum reduction of -80.2 mm in the RCP8.5 scenario and -91.9 mm in the RCP4.5 scenario. Puglia Centrale Adriatica and Murge regions are expected to experience the most significant decline in rainfall. Heavy rainfall days (R20) exhibit a slight increase in the RCP8.5 scenario and a slight decrease in the RCP4.5 scenario.
The 95th percentile of precipitation (P95) shows variable increases, with higher increments in the RCP8.5 scenario (maximum +4.9 mm) compared to the RCP4.5 scenario (maximum +2.9 mm). In the RCP8.5 scenario, most of the region is projected to experience an increase in P95, while in the RCP4.5 scenario, a decline in P95 is anticipated, particularly in the central southern Adriatic areas of the region.
The maximum number of consecutive dry days (CDD) is expected to increase in both future scenarios, with a maximum increase of 14 consecutive dry days in the RCP8.5 scenario and 18 days in the RCP4.5 scenario. This suggests that a significant portion of the regional territory may face extended periods without rainfall, aligning with numerous climate projections for the Mediterranean (Giannakopoulos et al., 2009; Cramer et al., 2018). For a visual representation of these seasonal anomalies, please refer to Figure 9, which illustrate the spatial distribution of T2M and MP anomalies for the RCP8.5 and RCP4.5 scenarios.
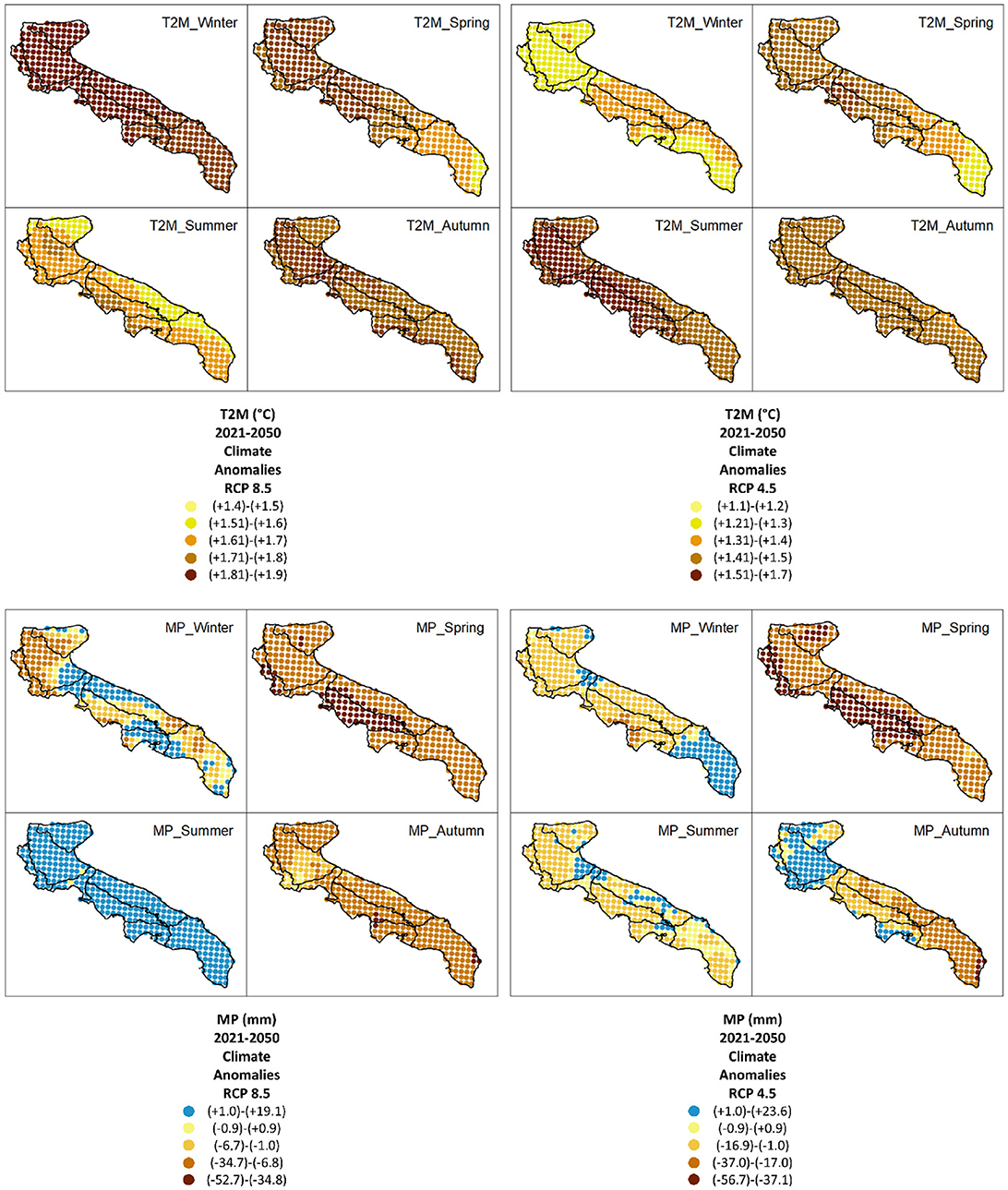
Figure 9. Distribution of the climatic anomalies of the average seasonal temperature (T2M) and average seasonal precipitation (MP) in Apulia, calculated on the future period 2021-2050 compared to the historical period 1979–2005 for the climate scenarios RCP8.5 and RCP4.5.
In both future emission scenarios, temperatures (T2M) are expected to rise in all seasons. Under the RCP8.5 scenario, a temperature rise ranging from +1.4°C to +1.9°C is forecasted, while the RCP4.5 scenario indicates an increase in temperature between +1.1°C and +1.7°C.
In both scenarios, the expected rise of temperatures leads to the progressive mitigation of cold seasons and the progressive heating of summer. The probability of higher and more frequent heat peaks increases (Simolo et al., 2014). The seasonal average precipitation anomalies show a general decrease, more pronounced in the RCP4.5 scenario. The spring season is expected to suffer the greatest drop in rainfall. The summer season shows an increase in rainfall in the RCP8.5 scenario and a decrease in the RCP4.5 scenario.
For a complete assessment of the expected anomalies for each analyzed climate indicator for the period 2021–2050, compared to the historical period 1979–2005, refer to Table 2. The results of climate projections align with earlier studies on the impact of climate change in the Mediterranean region. These studies have consistently identified the Mediterranean, including Apulia, as a particularly vulnerable area to the effects of global climate change (Giorgi and Lionello, 2008; Barkhordarian et al., 2012; Jacobeit et al., 2014; Todaro et al., 2022).
A mean sea level rise of 0.19 m and 0.25 m in the South Adriatic Sea is expected for the RCP4.5 and RCP8.5 scenario, respectively. In the RCP8.5 scenario, SST is expected to rise above 2°C in the time period 2036–2065, compared to the average value of the reference period 1981–2010 (18-20°C). The increase in temperature will be almost constant throughout the year keeping the seasonality of each area unchanged.
4.2.2 Impacts for aquaculture
Physical factors such as water temperature, currents, sunlight, nutrient availability, have a direct effect on the growth of aquaculture species, especially for autotrophic species and filter feeders as they depend on their surroundings for energy supply. Aquaculture production is therefore affected by a combination of external climatic variables, including droughts, floods, global warming, ocean acidification, rainfall variation, salinity and sea-level rise (De Silva and Soto, 2009), making it highly sensitive to climate change. Changes in thermal regimes, rainfall and their effects on dissolved oxygen concentration and salinity can have direct and unfavorable effects on the reproduction, growth and survival of farmed species. Similarly, for species where breeding depends on the availability of wild-collected seed and juveniles, changes in the habitats on which adults depend may reduce the availability of seed material, with indirect effects on production cycles.
Extreme weather conditions, which are becoming more intense and more frequent, cause major effects such as, for example, rising sea levels that can impact aquaculture infrastructures in coastal areas. Other changes due to climate change, which may affect livestock farming, include the increase in sea and atmospheric temperatures due to global warming, leading to a decrease in dissolved oxygen concentration due to lower oxygen solubility in warmer waters, and the rise in atmospheric CO2, which causes ocean acidification (Greenwood, 2010). This condition of increased vulnerability of aquaculture production is already a dramatic situation in Apulia as well (Giordano et al., 2019). The Apulian shellfish industry is suffering from the heatwaves that are now occurring more frequently and especially in the summer period, i.e. just when production is ready for the market. These sudden changes in temperature cause major losses of produce and, consequently, revenue, thus jeopardizing the survival of the entire production sector. Since such sudden temperature increases cannot be prevented, the solution must be sought in a system to reduce product losses. By using satellite tracking technology, in-situ monitoring, and numerical modeling, and integrating the results of the forecasts into an “early warning” information system, shellfish farmers can be warned on heat waves early enough to give them the chance to secure production, for example, by moving them to another body of water.
In general, the main climate variations that will affect the Mediterranean region with possible implications for aquaculture are:
• increase in water temperature (expected to be about + 2°C by the end of the century);
• salinity increase (0.5 ppt by the end of the century, more in the Adriatic);
• acidification of the sea (0.3-0.4 pH units by the end of the century);
• sea level rise (30-60 cm by the end of the century);
• increased frequency of extreme weather-climate events (heat waves, drought episodes, heavy rainfall, floods, coastal storms);
• reduction in average annual precipitation and river flows (and associated reduction in primary and secondary productivity along coastal areas near the mouths of major rivers);
• stress on water resources.
In addition, climate variations could lead to major biophysical effects of change on species (Franco et al., 2003; Sims et al., 2004; Orr et al., 2005; Battaglene et al., 2008; Gazeau et al., 2010; Range et al., 2011; Vaquer-Sunyer and Duarte, 2011; Hinder et al., 2012; Jennings et al., 2016; Bayne, 2017; Catalán et al., 2019; Neubauer and Andersen, 2019; Reid et al., 2019), particularly for:
• exceeding the thermal tolerance of bred species;
• hypoxic water conditions (stratification);
• phenological changes of species (early maturation, early reproduction, early appearance of larval forms etc.);
• calcification problems of organisms (mollusk shell and developmental problems);
• development of harmful organisms (algal blooms, jellyfish and other species invasions);
• increased risk of disease spread and increased virulence of pathogens;
• introduction of new pathogens;
• spread of invasive species;
• coastline retreat and increased erosion (flooding/seawater etc.);
• reduction in water quality and availability (especially in the South and the islands).
According to the relevant literature and those above cited there is evidence of potential impacts of climate change on aquaculture (De Silva et al., 2013):
• reduced growth and survival of species;
• occurrences of widespread die-offs;
• increased susceptibility to disease;
• new diseases;
• health risk from algal biotoxins;
• alteration of the migratory phases and reproductive cycle of fish species;
• reduction of natural and seed recruitment for the start of production cycles;
• infrastructure damage and loss of raised lots;
• reduction of suitable sites for breeding;
• reduced productivity.
For marine fish farming, particularly when located in offshore areas, the greatest impact will be driven by the increased frequency of extreme marine weather events. Although the current knowledge about the impacts of climate change on aquaculture activities allows certain predictions and assessments to be made (e.g., effects on the physiology of bred species, availability of suitable breeding sites, risks to animal and public health), implementing knowledge about the vulnerability of aquaculture to climate change is a priority.
4.2.3 Impacts for coastal tourism
The impacts of climate change on tourism can be classified into two main categories: i) direct impacts that cause changes in the trend of tourist flows typical of a tourist destination; ii) indirect impacts that induce changes in the attractiveness of the tourist destination, thus influencing its tourist arrivals and stays.
The direct impacts of climate change on tourism significantly affect the annual and seasonal numbers of tourist arrivals and stays (Koenig and Abegg, 1997). Climate plays a crucial role in tourists' travel choices, either enhancing or compromising their overall experience. Many recreational activities are influenced by climate conditions, and beach tourism, which is a prominent component of the Apulian tourist offerings, is particularly vulnerable.
Projections indicate that temperatures in Apulia are expected to increase by 2050, especially during the summer, with maximum increases of up to +2°C (Figure 9). Furthermore, the number of summer days is expected to increase by a maximum of +20 days per year in the RCP8.5 scenario and a maximum of +18 days per year in the RCP4.5 scenario. These rising temperatures may lead to a decline in tourist numbers in Apulia, as it becomes less comfortable for tourists to stay in holiday areas and limiting also the feasibility of many activities, directly impacting the reasons for their trips (Hooyberghs et al., 2017). Conversely, milder temperatures in winter, spring, and autumn could benefit tourism (Scheraga and Grambsch, 1998), contributing to seasonal adjustments by better distributing tourist flows throughout the year.
The increase in temperatures will not only affect Apulia but also other competitive tourist destinations (Koenig and Abegg, 1997), both nationally and internationally. This can have a negative or positive impact on regional tourist demand, depending on the effects that climate change will have on other tourist destinations. Generally, at the European level, an improvement in summer climatic conditions is expected in the countries of central and northern Europe (Scott et al., 2012), with an advantage for tourism, while for the Mediterranean countries, the increase in summer temperatures will impact negatively on the sector (Hooyberghs et al., 2017). It's important to note that not only high temperatures, but also extreme, more intense, and frequent climatic events, can affect tourist demand (Bigano et al., 2005).
Apulian tourism, being seasonal and heavily reliant on the “sea” product, is particularly susceptible to the direct effects of climate change. However, due to the lack of long time series data, it is currently challenging to assess the impact of climate change on the number of tourist arrivals and stays recorded in Apulia. Therefore, ongoing monitoring of tourist flows remains essential.
Indirect impacts of climate change on tourism lead to changes in various aspects that characterize a tourist destination (Scott and Lemieux, 2010; MASE, 2023). These aspects include natural and artificial tourist attractions, accommodation and service facilities, and transport networks. Based on the unique characteristics of tourism in the region, the geographical features, and climate predictions, six primary indirect impacts have been identified, each affecting the appeal and competitiveness of the “Apulian Adriatic Coast” destination to varying degrees:
1. Increase in forest fires in tourist coastal areas: Due to climate change, the duration of the fire season is expected to lengthen (Giannakopoulos et al., 2009), rendering forest systems more vulnerable to fires, especially in the Mediterranean region (García-Ruiz et al., 2011). The growing risk of forest fires is a significant concern for tourism (Otrachshenko and Nunes, 2022) due to the physical damage to forest attractions, tourist accommodations, and potential risks to peoples lives (Shaposhnikov et al., 2014). In Apulia, the urban-forest interface areas in Apulia are particularly exposed to fire risks due to a higher likelihood of ignition (Elia et al., 2014). There are numerous fire-prone areas along the Apulian Adriatic coast, such as coastal forests and Mediterranean spots near tourist areas (Elia et al., 2019), where inadequate forest management practices contribute to the risk of fires (Elia et al., 2014).
2. Increase of alien and invasive species in land and sea tourist coastal areas:
Climate change can facilitate the spread of invasive species (alien species), both terrestrial and marine, to new regions (Katsanevakis et al., 2014). These invasions have various effects on tourism (Vilà et al., 2010). While there may be some advantages, such as the creation of new habitats with species of interest to tourists (Liquete et al., 2013), negative impacts include the loss of native species and habitats (IPCC, 2023), aesthetic issues like the proliferation of algae and jellyfish on beaches (Tsirintanis et al., 2022), and health concerns such as injuries, allergies, and the transmission of diseases (Edelson et al., 2023). Apulia's coast is home to numerous alien marine (Gravili et al., 2010) and terrestrial species, although further research is required to gain a better understanding of the consequences of these invasions on tourism (Arabadzhyan et al., 2021).
3. Thermal stress and health hazards to tourists and practitioners:
Rising temperatures and more frequent heat waves pose increased health risks to vulnerable residents, tourists, and workers (IPCC, 2023). In Apulia, an increase in average maximum temperatures and a longer summer season are expected (Figure 8). In coastal regions, the combination of rising day and night temperatures and high relative humidity levels (MASE, 2023) can create challenging conditions for both locals and tourists (Giannakopoulos et al., 2011). Densely populated urban areas (e.g. Bari in Apulia) are already experiencing “heat islands” which can contribute to local temperature increases (Martinelli et al., 2020), leading to heightened health risks for vulnerable individuals (Di Rienzi et al., 2006; Noro and Lazzarin, 2015). High temperatures deter tourism and can influence tourist behavior (Gössling et al., 2006), movement patterns, activities (Gómez-Martín et al., 2014; Cavallaro et al., 2017), and accommodation preferences (Gómez-Martín et al., 2014), affecting both the supply and demand of a tourist destination.
4. Decreasing domestic water for the tourism industry and increasing energy consumption:
Climatic projections for Apulia and Southern Italy indicate a progressive decrease in average annual rainfall and longer dry periods (De Luca et al., 2022). Water scarcity can seriously damage the competitiveness of a tourist destination, especially in coastal tourism during the summer, which requires substantial water for sanitation, catering, and recreational activities. Water scarcity can make water-based recreational activities infeasible and leave the accommodation and service facilities without this essential resource (Arabadzhyan et al., 2021). Climate change also influences energy consumption levels, either enhancing or worsening the thermal comfort of a region, resulting in increased or decreased energy usage (Giannakopoulos et al., 2011). Rising summer temperatures may lead to higher energy consumption for cooling accommodations (Giannakopoulos et al., 2009). In Southern Europe, this can exceed the reduction in winter heating needs, resulting in higher annual energy costs (Pilli-Sihvola et al., 2010; Zachariadis, 2010). The increase in energy consumption due to climate change, combined with an increase in tourist arrivals and stays, especially in highly seasonal tourism, can strain the energy distribution system. This is particularly significant for densely populated tourist resorts with outdated energy infrastructure that is vulnerable to high temperatures.
5. Damage from extreme weather events to accommodation, service, transportation, and cultural and agricultural heritage infrastructure and facilities:
The frequency and intensity of extreme weather events are expected to increase due to climate change, especially in the Mediterranean area (Trenberth et al., 2015). Apulia has historically been exposed to extreme climatic events, including heavy rains, tornadoes, and storms (Gianfreda et al., 2001; De Martini et al., 2003; Mastronuzzi et al., 2007). Future climate projections until 2050 indicate slight growth rates for indicators R20 and P95 (Figure 8), suggesting that the frequency of extreme events is not expected to undergo significant changes compared to today; at most, they may tend to increase. The Adriatic coastal areas of Apulia, characterized by intense urban and tourist development, are vulnerable to storms or extreme climatic events, which can result in significant damage and severe economic consequences (Martinotti et al., 2017). On an individual structure and infrastructure level, climate change, including rising temperatures and extreme climatic events, can accelerate deterioration, leading to increased damages, maintenance, and renovation costs for architectural and cultural heritage assets (Orr et al., 2021; Sesana et al., 2021).
6. Coastal erosion and reduced beach availability:
Connected to climate change, rising sea levels, and coastline recession (Vousdoukas et al., 2020) contribute to further beach erosion, impacting seaside and coastal tourism (Rizzetto, 2020). Reduced beach availability diminishes the attractiveness of coastal tourist destinations (Delle Rose, 2015), indirectly affecting tourist movement. Future projections indicate a high risk of erosion along the Apulian Adriatic coast. Predicted retreats, considering the already limited widths of average Adriatic beaches, could result in the disappearance of some existing beaches. For more information on coastal erosion in Apulia, please refer to Section 4.2.4.
4.2.4 Impacts for coastal erosion
The analysis focused on the expected impacts for coastal areas, specifically concerning the intensification of coastal erosion processes.
To analyze the effects of climate change along the Apulian Adriatic coast, the median values of long-term displacements (P50) were extrapolated along the examined area from the projections of sandy shoreline dynamics by Vousdoukas et al. (2020).
Despite some limitations in the global study, such as not accounting for the limited space for coastal retreat and discrepancies in classifying coastal morphology, the study provides clear indications of a serious risk of coastline retreat in both scenarios (Figure 10). Moreover, these findings align perfectly with the results from the analysis of recent beach evolution (see Figure 7), which has revealed alarming rates of erosion and these rates are expected to escalate in the coming decades due to the projected rise in mean sea level. This could lead to further degradation of the already narrow Adriatic sandy strip (Table 3).
Unlike the predictive models for mean sea level, which unanimously assert that there will be a significant increase in global mean sea level during the 21st century, the robustness and reliability of the predicted change in wave climate is still a subject of debate (Morim et al., 2019). In general, there is a greater degree of consensus on the expected change in mean annual values, while the trend in extreme events has greater uncertainty (Lobeto et al., 2021).
On a global scale, the results of wave climate projections from 91 different sources showed a general agreement on a decreasing trend for annual mean values of significant wave heights in the Mediterranean Sea, while with regard to extreme wave height values, no general consensus was found (Morim et al., 2018).
The analysis of projected wave climate change in the RCP8.5 scenario in the Mediterranean Sea showed a decreasing trend in annual averages and annual maximums of significant wave heights and mean periods in most of the Mediterranean basin (De Leo et al., 2021).
5 Discussion
The implementation of adaptation measures is essential to address the inevitable consequences of climate change in the short and medium term. Adaptation implies anticipating the adverse impacts of climate change and adopting appropriate measures to prevent or minimize damages, as well as to exploit emerging opportunities. Exploiting the sectorial impacts analysis conducted for the three key sectors under investigation, the research for the corresponding adaptation actions has been outlined. Adaptation measures have been chosen from the literature, taking into consideration their suitability to the local context and the specific characteristics of the analyzed sectors. Greater priority has been given to research experiences, and policies on climate adaptation developed within the European, Italian, and Apulian context.
5.1 Adaptation actions for aquaculture
Adaptation strategies will need to include actions that seek to increase scientific knowledge on the phenology of farmed species and their adaptive and resilience capacities to different pressures related to climate change.
There is need of experimental tests, the development of forecasting models and specific indicators; on the possibility of selecting species/strains tolerant to climate change-induced conditions; and to develop methods (risk analysis) for analyzing the vulnerability of the various production systems in the country. It will be important to devise more streamlined and manageable plant structures, with materials endowed with greater elasticity and capacity to withstand extreme weather events for which weather warning and criticality systems are important.
Additionally, monitoring systems also need to be implemented by means of the use of satellite data and data collection systems for the physical-chemical and biological characteristics of water bodies.
Finally, vocational training for the aquaculture sector is of particular importance to attract young people and enable fishermen to retrain, helping to create jobs in coastal regions and islands that are traditionally more dependent on fishing activities. One of the possible climate change adaptation strategies for the aquaculture sector is Integrated Multi-Trophic Aquaculture (IMTA) (Sreejariya et al., 2011; Chung et al., 2013; Geere, 2014; Clements and Chopin, 2017). IMTA is a farming system that combines commonly farmed species like fish and crustaceans with other organisms like algae and invertebrates (e.g., filter feeders or detritivores) in a single integrated farm (Troell et al., 2009; Chopin, 2011; Chopin et al., 2012) (Figure 11). This approach aims to recycle waste products from one species into inputs for another, promoting environmental sustainability, economic viability, and social acceptability (Barrington et al., 2009). IMTA systems are used worldwide, including in countries like Canada, Chile, China, Japan, the United States, and various European nations, with recent interest also in Bangladesh (Chopin, 2011; Sarker et al., 2014; Kibria, 2016).
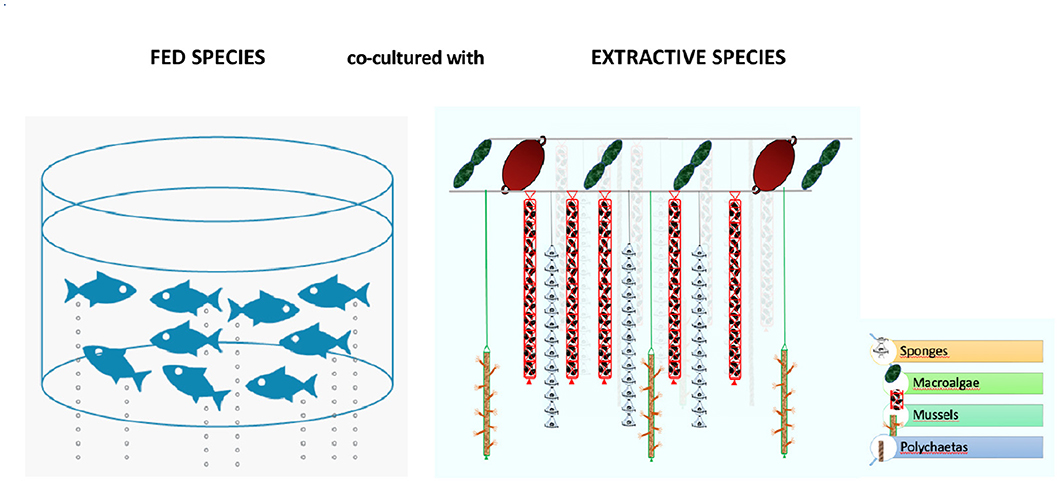
Figure 11. Schematic conceptual diagram of an IMTA system developed for REMEDIA Life project (modified from Giangrande et al., 2020).
IMTA has gained popularity as a strategy to address nutrient pollution caused by intensive farming, especially in Asia (Neori et al., 2004). IMTA configurations can vary significantly, with different species combinations. Generally, in these systems, the algal component is used to reduce the nitrogen load produced by fish feces and the decomposition processes of unused feed; the bivalves, to reduce the rise in the phytoplanktonic component triggered by the increase in nutrients. However, numerous variations to this basic scheme have been trialed, with the use different categories of extractive species, both edible (e.g., mollusks, macroalgae) able also to contribute to CO2 sequestration and the subtraction of nitrogen and phosphorous (nutrients), and non-edible (e.g., porifera, polychaetas, ascidians, holothuroids, sea urchins etc.) able to mitigate the excessive trophism of water resulting from fish farms and mitigate the possible presence of algal biotoxins or pathogens (Chopin, 2011; Giangrande et al., 2020; Papageorgiou et al., 2023). In addition, the trend toward diversification of farmed species and relocation of production facilities to the open sea has long been established, mainly with the aim of making mariculture more competitive and environmentally friendly, but recently also for responding to the new challenges that climate change requires (Abellan and Basurco, 1999; Ahmed and Glaser, 2016). IMTA creates a virtuous system in the mariculture sector, generating multiple benefits (Figure 12) such as reducing impacts from fish farming and achieving balanced systems, performance improvement, production diversification including additional by product exploitable biomass, greater social acceptance, new job opportunities and mitigation of climate change effects. Thus, IMTA is considered an adaptation strategy of the ecosystem approach to climate change (IFAD, 2014), and coastal IMTA in open waters could play an important role in strengthening the resilience coastal systems to climate change (Ahmed and Glaser, 2016).
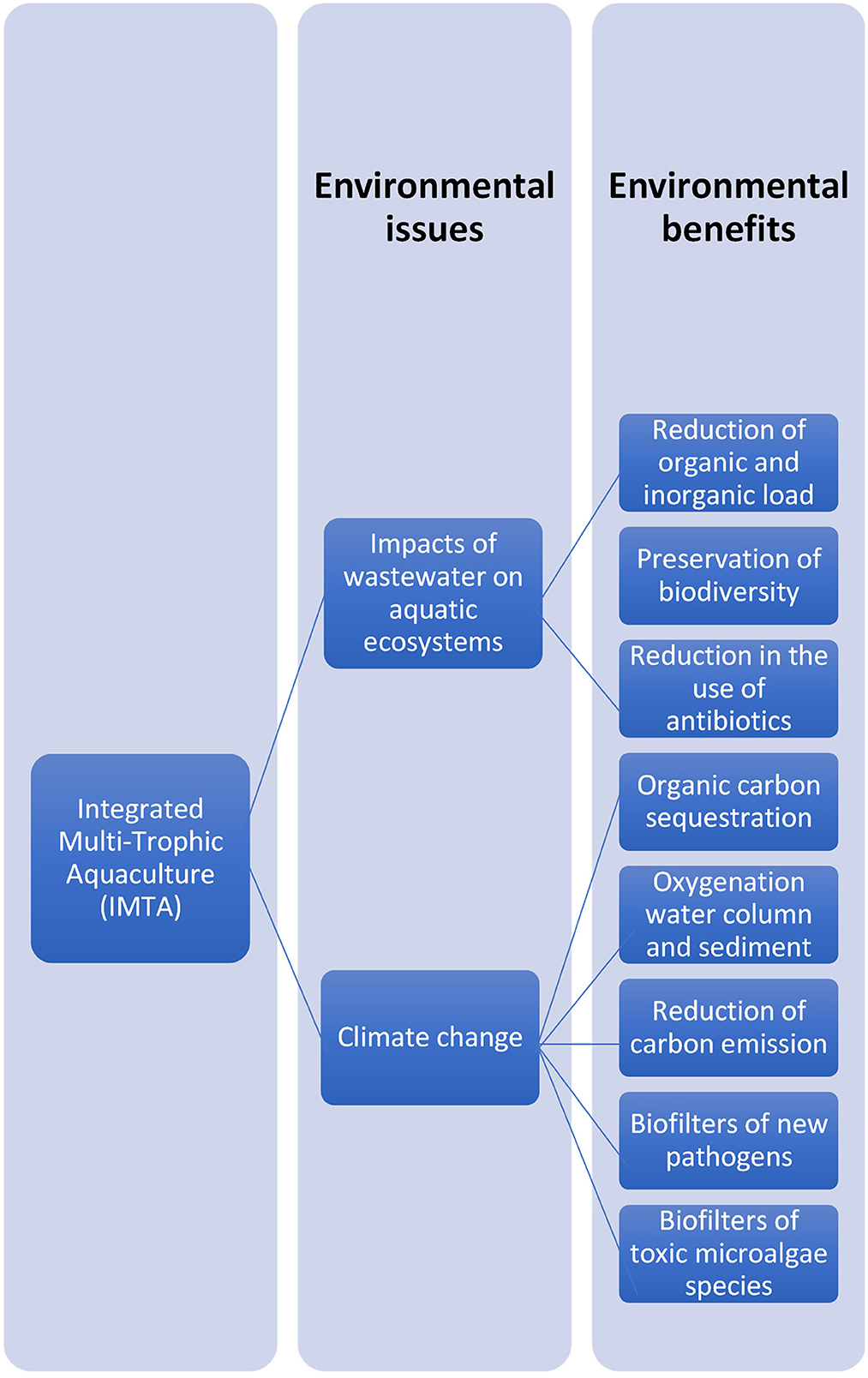
Figure 12. Main environmental benefits from the development of IMTA practices in open sea, which contribute to the response to climate change effects.
The implementation of IMTA systems in confined coastal areas, for example, can increase the availability of O2 produced by photosynthesis by reared macroalgae. In addition, IMTA can reduce O2 consumption due to the decomposition of organic matter by microorganisms through the bioremediation achieved by reared filter-feeders (Sreejariya et al., 2011). In IMTA systems, the dissolved oxygen (O2) produced by the photosynthetic activity of algae has multiple benefits. It enhances microbial activity also within the sediment, facilitating the efficient recycling of nutrients from the decomposition of dead organisms, as demonstrated by Björk et al. (2008). Furthermore, IMTA plays a crucial role in reducing carbon dioxide (CO2) emissions: algae utilize CO2 for photosynthesis, and integrated mussel farming contributes to carbon sequestration since they build their shells (Mcleod et al., 2011; Wolff and Beaumont, 2011; Duarte et al., 2013; Siikamäki et al., 2013).
Algae and all filter-feeding organisms reared in IMTA systems can help keep the water column in good condition by absorbing pollutants, sediments and toxic substances (Chung et al., 2013).
The outbreaks spread is a clear example of spatial distribution influenced by global warming, resulting in significant mortality events and reduced production of, for example, marine mollusks on Mediterranean coasts (Villalba et al., 2004, 2005). According to recent estimates, despite the differential distribution of bacteria and toxic microalgae species along the Mediterranean coasts, pathogen outbreaks are expected to occur rapidly, particularly due to thermophilic species (Galli et al., 2017). The integrated farming in IMTA systems of filter-feeding species, both edible and non-edible, that are naturally effective biofilters, can help alleviate the risk of disease outbreaks both for farmed species and humans.
Several porifera species have been shown to be effective bioremediators of different categories of microbiological pollutants, organic and inorganic compounds (e.g., Aguilo-Arce et al., 2023). Sponges are predominantly marine benthic invertebrates that are effective filter feeders, capable of removing organic particles (dissolved and particulate organic matter, heterotrophic bacteria, heterotrophic eukaryotes, phytoplankton) from sea water. Picoplankton (< 2 microns) and, in particular, bacterioplankton are considered to be the major source of carbon for sponges and can potentially entirely supply their diet, especially in coastal waters with high organic content (Reiswig, 1975; Pile et al., 1996). A field experiment aimed to evaluate the demosponge Hymeniacidon perlevis microbiological accumulation consequence on the filtration of Mytilus galloprovincialis, showed important application potential in the field of environmental mitigation in shellfish farms. Indeed, the presence of the sponge demonstrated a positive effect on M. galloprovincialis, reducing its accumulation of all considered bacterial categories (vibrios, coliforms and enterococci) (Longo et al., 2016). The results obtained in the near-shore polyculture pilot plant of M. galloprovincialis and H. perlevis encourage the implementation of polyculture systems for mussels and sponges, which would lead to an improvement in the microbiological characteristics of farmed mussels and to a mitigation of microbiological pressures in mollusk farming areas (Longo et al., 2016).
IMTA experiences in productive mariculture plants in the Mediterranean area, including the Apulia region, have been carried out recently in different research projects demonstrating its potential to mitigate the adverse effects of mariculture activities including nutrient pollution and disease outbreaks (Gifford et al., 2007; Longo et al., 2010, 2016; Giangrande et al., 2020; Chatzivasileiou et al., 2022; Papageorgiou et al., 2023; Stabili et al., 2023). The development and spread of IMTA practices along the Apulian coasts could represent an environmental challenge for adaptation and mitigation to climate change in the marine ecosystems of this area.
5.2 Adaptation actions for coastal tourism
Following the identification of anticipated climate change impacts on Apulia's Adriatic coastal tourism, adaptation strategies have been identified. These actions, tailored to each specific impact, have been carefully evaluated and selected based on scientific, regulatory, and project documents ensuring to select those that exhibited the greatest effectiveness in i) reaping benefits from the expected impacts by stimulating tourism development; ii) preventing the occurrence of the impacts themselves by acting on the triggering conditions; iii) effectively managing the expected impacts to contain their negative effects.
To manage the direct impacts of climate change on coastal tourism and prevent a decline in tourism flows, effective adaptation strategies aim to achieve seasonal adjustments and diversify tourist offerings (MIBACT, 2017). Seasonal adjustment allows for capitalizing on the progressive increase in temperatures during periods of lower tourist activity, such as the winter season, thereby promoting year-round tourism growth. Diversification of tourist offerings encourages the creation of new industry segments that can better adapt to changing climatic conditions. Although Apulia Region is actively implementing both strategies through its regional strategic tourism plan (Puglia365), further efforts are still required to reduce the strong dependence of Apulian Adriatic tourism on the “sea” product and the summer season. Tourists could also benefit from specific measures, such as purchasing insurance to manage climate-related risks (Olya et al., 2019; MASE, 2023), or participating in climate change awareness campaigns that may also involve professionals and tour operators (MASE, 2023).
In managing the indirect impacts of climate change on coastal tourism, various approaches can be adopted depending on the specific impact under consideration. Effective adaptation strategies for addressing tourism's indirect impacts should involve effective coordination with adaptation initiatives in other tourism-related sectors. For example, in managing the increased risk of forest fires in coastal tourist areas, climate change adaptation should encompass both specific actions for the forestry sector as well as customized adaptation measures for tourism. Supplementary Table S1, presented in the Supplementary material, provides a comprehensive overview of the main adaptation actions to be implemented within the tourism sector by tourists, operators, and local tourism administrators to address all selected indirect impacts. This Table includes a description of their objectives and sources. Priority has been given to adaptation initiatives that are already being implemented at the regional level, albeit for different purposes.
5.3 Adaptation actions for coastal erosion
Given the potential impacts of climate change in the region, it is of utmost importance to develop appropriate strategies to address these challenges. A correct approach to coastal management should consider the confluence of both global and local factors, which combine to create a situation of beach erosion and flooding in already vulnerable coastal areas in the coming years.
Analyses of the evolutionary dynamics on the Apulian regional coast have revealed numerous beaches undergoing retreat. Given the scale of these phenomena and their localized nature, addressing all erosive tendencies may not be realistically feasible, especially considering the importance of preserving the natural coastal environment that characterizes the region (Cantasano et al., 2023). To protect the coastal area and preserve its landscape, naturalistic, and environmental value, soft-type structural interventions such as nourishment (preferably protected) and, locally, the seasonal movement of sediments in the active beach should be prioritized. Nature-Based Solutions (NBS) could also be employed to mitigate marine erosion and flooding (Morris et al., 2018; Chausson et al., 2020). These interventions not only benefit the public use of the coastal area but also drive tourism development, revitalizing areas facing coastal degradation that may otherwise experience a decline in tourist flows.
The emphasis should be on implementing interventions aimed at regulating and guiding the coast's evolutionary processes, sometimes requiring a “strategy of retreat”. In areas where urbanization has not yet affected the beaches, construction should only be permitted where erosion is absent or less severe. Alternatively, construction could take place while maintaining a substantial non-buildable buffer zone. The buildable stretch closest to the sea should be allocated for lower-cost interventions that allow for restructuring or conversion.
Other important interventions include restoring or stabilizing river flows into the sea and halting mining activities in riverbeds, which can reduce sediment supply. It is crucial to pay special attention to managing river mouth areas, as they are vital to the coastal system. Construction of maritime works (piers, breakwaters, etc.) in these areas, which might obstruct sediment transport and disrupt adjacent beach nourishment, should be avoided. Another significant adaptation intervention involves recreating or preserving existing dunes (Fernández-Montblanc et al., 2020). However, it is essential to acknowledge that erosion of beaches, caused by the net decrease in naturally supplied sediments and extensive coastal and hinterland development, will not cease completely with either rigid or soft protection interventions. All coastal protection measures require post-construction maintenance, especially recharge interventions, which necessitate cyclically foreseen compensation for lost volumes over time.
6 Conclusion
Climate Change and its effects necessitate the adoption of adaptation strategies at various levels, aimed at managing future climate risks and seizing opportunities. Adaptation becomes imperative, especially in regions like Apulia, which may face significant impacts from climate change in the future.
The analysis conducted in this study, focusing on the sectors of aquaculture, coastal tourism, and coastal erosion along the Apulian Adriatic coast, offers an approach for analyzing and evaluating the adaptation measures currently in place and those that can be applied to manage the anticipated impacts of climate change for the three studied sectors.
Climate change-induced alterations in ecosystems, production cycles, infrastructure, and the physiology of organisms produced or harvested from nature will have profound consequences for Apulian aquaculture. The rising water temperatures represent a primary climatic threat that the sector will confront. The choice of species for farming can be both a vulnerability and an opportunity. Anticipated increases in summer maximum temperatures in Apulia may lead to potential decreases in summer tourist arrivals throughout the region, favoring the development of a more seasonally adjusted tourism industry. Considering the characteristics of the Adriatic coastal territory and other tourism-related sectors, numerous indirect impacts on the sector have been identified.
Analyses of the evolving dynamics along the regional coast of Apulia have highlighted the presence of numerous stretches of eroding coastline. Future projections indicate scenarios of widespread coastline retreat, resulting in a progressive reduction in beach depth, which is already characterized by limited amplitudes. These changes could have possible consequences for the economic, environmental, and social aspects of the coastal regions.
The observed adaptation initiatives in the three sectors appear to be primarily sector-specific, designed to address current impacts or short-term risks, and often motivated by planning rather than implementation. In some cases, adaptation actions for certain risks have not been identified, but alternative solutions have been proposed to achieve objectives that remain consistent with adaptation goals.
Apulian aquaculture, for instance, could potentially benefit from the introduction of new farming systems such as “Integrated Multi-Trophic Aquaculture” (IMTA), which would enable sustainable production diversification and enhance the resilience of farming systems to climate change.
The significance of intersectoral adaptation is evident, particularly in coastal tourism, where coordinated adaptation actions and initiatives not only benefit the tourism sector but also have positive spillover effects on related sectors. For example, effective management of beaches and coastal dunes can enhance tourism while mitigating the adverse effects of coastal erosion on beaches, benefiting numerous other sectors.
In this paper, it is recognized that governance capacity and decision-making processes are fundamental in implementing an adaptation plan. Monitoring and evaluation are crucial steps to track progress and identify areas where further action may be necessary. Moreover, integrating adaptation and mitigation into planning can promote climate-resilient development, particularly in locations with persistent development gaps and limited resources. This study supports the development of a regional climate adaptation plan for Apulia and underscores the significance of multi-scale, intersectoral, and environmentally friendly adaptation approaches that involve all social strata.
Data availability statement
The original contributions presented in the study are included in the article/Supplementary material, further inquiries can be directed to the corresponding author.
Author contributions
GP: Conceptualization, Data curation, Formal analysis, Investigation, Methodology, Resources, Software, Validation, Visualization, Writing – original draft, Writing – review & editing. MB: Conceptualization, Data curation, Formal analysis, Investigation, Methodology, Resources, Software, Visualization, Writing – original draft, Writing – review & editing. PC: Investigation, Writing – original draft, Writing – review & editing. RC: Investigation, Writing – original draft, Writing – review & editing. MC: Investigation, Writing – original draft, Writing – review & editing. GD'O: Investigation, Methodology, Writing – original draft, Writing – review & editing. AF: Formal analysis, Investigation, Resources, Software, Visualization, Writing – original draft, Writing – review & editing. UF: Investigation, Methodology, Supervision, Writing – original draft, Writing – review & editing. CL: Investigation, Writing – original draft, Writing – review & editing. FL: Investigation, Writing – original draft, Writing – review & editing. SS: Investigation, Writing – original draft, Writing – review & editing. FG: Conceptualization, Funding acquisition, Investigation, Methodology, Project administration, Supervision, Validation, Writing – original draft, Writing – review & editing.
Funding
The author(s) declare that financial support was received for the research, authorship, and/or publication of this article. This work was funded by the Project Climate adaptation and mitigation plan in the eligible area of the Puglia Region—AdriaClim between University of Bari Aldo Moro and Department of Environment, Urban Quality and Landscape of Regione Puglia, within the framework of the AdriaClim project—Interreg V-A Program Italy—Croatia CBC 2014-2020.
Acknowledgments
The research activities of this article originated during the study carried out within the Project “Climate adaptation and mitigation plan in the eligible area of the Puglia Region—AdriaClim”, within the framework of the AdriaClim project—Interreg V-A Program Italy—Croatia CBC 2014–2020.
Conflict of interest
The authors declare that the research was conducted in the absence of any commercial or financial relationships that could be construed as a potential conflict of interest.
The author(s) declared that they were an editorial board member of Frontiers, at the time of submission. This had no impact on the peer review process and the final decision.
Publisher's note
All claims expressed in this article are solely those of the authors and do not necessarily represent those of their affiliated organizations, or those of the publisher, the editors and the reviewers. Any product that may be evaluated in this article, or claim that may be made by its manufacturer, is not guaranteed or endorsed by the publisher.
Supplementary material
The Supplementary Material for this article can be found online at: https://www.frontiersin.org/articles/10.3389/fclim.2024.1378253/full#supplementary-material
References
Abbass, K., Qasim, M. Z., Song, H., Murshed, M., Mahmood, H., and Younis, I. (2022). A review of the global climate change impacts, adaptation, and sustainable mitigation measures. Environ. Sci. Pollut. Res. 29:42539–42559. doi: 10.1007/s11356-022-19718-6
Abellan, E., and Basurco, B. (1999). Marine Finfish Species Diversification: Current Situation and Prospects in Mediterranean Aquaculture. Zaragoza: CIHEAM-IAMZ.
Agency, E. E. (2012). Climate Change, Impacts and Vulnerability in Europe 2012 an Indicator-Based Report. Luxembourg: European Environment Agency (EEA).
Aguilo-Arce, J., Ferriol, P., Trani, R., Puthod, P., Pierri, C., and Longo, C. (2023). Sponges as emerging by-product of integrated multitrophic aquaculture (IMTA). J. Mar. Sci. Eng. 11:80. doi: 10.3390/jmse11010080
Ahmed, N., and Glaser, M. (2016). Can “ntegrated multi-trophic aquaculture (IMTA)” adapt to climate change in coastal Bangladesh? Ocean & Coastal Managem. 132 120–131. doi: 10.1016/j.ocecoaman.2016.08.017
Andaloro, F., Antonini, C., Battaglia, P., Donadelli, V., Giovanardi, O., Marino, G., et al. (2020). Annuario 2020. Rome: ISPRA.
Arabadzhyan, A., Figini, P., García, C., González, M. M., Lam-González, Y. E., and León, C. J. (2021). Climate change, coastal tourism, and impact chains-a literature review. Curr. Issues Tour. 24, 2233–2268. doi: 10.1080/13683500.2020.1825351
Barkhordarian, A., Bhend, J., and von Storch, H. (2012). Consistency of observed near surface temperature trends with climate change projections over the mediterranean region. Climate Dynam. 38, 1695–1702. doi: 10.1007/s00382-011-1060-y
Barrington, K., Chopin, T., and Robinson, S. (2009). “Integrated multi-trophic aquaculture (IMTA) in marine temperate waters,” in Integrated Mariculture: a Global Review. FAO Fisheries and Aquaculture Technical Paper. No. 529, ed D. Soto (Rome: FAO), 7–46.
Battaglene, S. C., Hobday, A., Carter, C., Lyne, V., and Nowak, B. (2008). Scoping Study into Adaptation of the Tasmanian Salmonid Aquaculture Industry to Potential Impacts of Climate Change. Tasmania: University of Tasmania.
Bigano, A., Goria, A., Hamilton, J. M., and Tol, R. S. J. (2005). “The effect of climate change and extreme weather events on tourism,” in FEEM Working Paper No. 30.05, CMCC Research Paper No. 01 (Gland: IUCN). Available online at: https://ssrn.com/abstract=673453
Björk, M., Short, F., Mcleod, E., and Beer, S. (2008). Managing Seagrasses for Resilience to Climate Change.
Bronzi, P., Rambaldi, E., Cardillo, A., Dell-Aquila, M., Di Dato, P., and Cataudella, S. (2011). “The state of the Italian aquaculture,” in The State of the Italian Marine Fisheries and Aquaculture, eds. S. Cataudella, and M. Spagnolo (Rome: MiPAAF), 239–271.
Brown, S., Nicholls, R. J., Woodroffe, C. D., Hanson, S., Hinkel, J., Kebede, A. S., et al. (2013). Sea-Level Rise Impacts and Responses: A Global Perspective. Dordrecht: Springer Netherlands, 117–149.
Bruno, M., Molfetta, M., and Petrillo, A. (2014). The influence of interannual variability of mean sea level in the adriatic sea on extreme values. J. Coastal Res. 70, 241–246. doi: 10.2112/SI70-041.1
Bruno, M. F., Molfetta, M. G., Pratola, L., Mossa, M., Nutricato, R., Morea, A., et al. (2019). A combined approach of field data and earth observation for coastal risk assessment. Sensors 19:1399. doi: 10.3390/s19061399
Bruno, M. F., Motta Zanin, G., Barbanente, A., and Damiani, L. (2021). Understanding the cognitive components of coastal risk assessment. J. Mar. Sci. Eng. 9:780. doi: 10.3390/jmse9070780
Bruno, M. F., Saponieri, A., Molfetta, M. G., and Damiani, L. (2020). The dpsir approach for coastal risk assessment under climate change at regional scale: the case of apulian coast (Italy). J. Mar. Sci. Eng. 8:531. doi: 10.3390/jmse8070531
Bucchignani, E., Montesarchio, M., Zollo, A. L., and Mercogliano, P. (2016). High-resolution climate simulations with COSMO-CLM over Italy: performance evaluation and climate projections for the 21st century. Int. J. Climatol. 36, 735–756. doi: 10.1002/joc.4379
Cantasano, N., Boccalaro, F., and Ietto, F. (2023). Assessing of detached breakwaters and beach nourishment environmental impacts in Italy: a review. Environ. Monit. Assess. 195:127. doi: 10.1007/s10661-022-10666-9
Catalán, I. A., Auch, D., Kamermans, P., Morales-Nin, B., Angelopoulos, N. V., Reglero, P., et al. (2019). Critically examining the knowledge base required to mechanistically project climate impacts: a case study of Europe's fish and shellfish. Fish Fisher. 20, 501–517. doi: 10.1111/faf.12359
Cavallaro, F., Ciari, F., Nocera, S., Prettenthaler, F., and Scuttari, A. (2017). The impacts of climate change on tourist mobility in mountain areas. J. Sustain. Tour. 25, 1063–1083. doi: 10.1080/09669582.2016.1253092
Chatzivasileiou, D., Dimitriou, P. D., Theodorou, J., Kalantzi, I., Magiopoulos, I., Papageorgiou, N., et al. (2022). An IMTA in Greece: co-culture of fish, bivalves, and holothurians. J. Mar. Sci. Eng. 10:776. doi: 10.3390/jmse10060776
Chausson, A., Turner, B., Seddon, D., Chabaneix, N., Girardin, C. A., Kapos, V., et al. (2020). Mapping the effectiveness of nature-based solutions for climate change adaptation. Glob. Chang. Biol. 26, 6134–6155. doi: 10.1111/gcb.15310
Chopin, T. (2011). Progression of the integrated multi-trophic aquaculture (IMTA) concept and upscaling of IMTA systems towards commercialization. Aquacult. Eur. 36, 5–12.
Chopin, T., Cooper, J. A., Reid, G., Cross, S., and Moore, C. (2012). Open-water integrated multi-trophic aquaculture: environmental biomitigation and economic diversification of fed aquaculture by extractive aquaculture. Rev. Aquacult. 4, 209–220. doi: 10.1111/j.1753-5131.2012.01074.x
Chung, I. K., Oak, J. H., Lee, J. A., Shin, J. A., Kim, J. G., and Park, K.-S. (2013). Installing kelp forests/seaweed beds for mitigation and adaptation against global warming: Korean project overview. ICES J. Marine Sci. 70, 1038–1044. doi: 10.1093/icesjms/fss206
Clements, J. C., and Chopin, T. (2017). Ocean acidification and marine aquaculture in north america: potential impacts and mitigation strategies. Rev. Aquacult. 9, 326–341. doi: 10.1111/raq.12140
Cramer, W., Guiot, J., Fader, M., Garrabou, J., Gattuso, J.-P., Iglesias, A., et al. (2018). Climate change and interconnected risks to sustainable development in the mediterranean. Nat. Clim. Chang. 8, 972–980. doi: 10.1038/s41558-018-0299-2
De Leo, F., Besio, G., and Mentaschi, L. (2021). Trends and variability of ocean waves under rcp8. 5 emission scenario in the mediterranean sea. Ocean Dynam. 71, 97–117. doi: 10.1007/s10236-020-01419-8
De Luca, D. L., Apollonio, C., and Petroselli, A. (2022). The benefit of continuous hydrological modelling for drought hazard assessment in small and coastal ungauged basins: a case study in southern italy. Climate 10:34. doi: 10.3390/cli10030034
De Martini, P. M., Burrato, P., Pantosti, D., Maramai, A., Graziani, L., and Abramson, H. (2003). Identification of tsunami deposits and liquefaction features in the Gargano area (Italy): paleoseismological implication. Ann. Geophys. 46. doi: 10.4401/ag-3460
De Silva, S. (2013). “Climate change impacts: challenges for aquaculture,” in Proceedings of the Global Conference on Aquaculture 2010. Farming the Waters for People and Food. Arkansas: FAO/NACA, 75–110.
De Silva, S. S., and Soto, D. (2009). Climate change and aquaculture: potential impacts, adaptation and mitigation. Climate change implications for fisheries and aquaculture: overview of current scientific knowledge. FAO Fisher. Aquacult. Techn. Paper 530, 151–212.
Delle Rose, M. (2015). Medium-term erosion processes of south adriatic beaches (apulia, italy): a challenge for an integrated coastal zone management. J. Earth Sci. Clim. Change 6:2. doi: 10.4172/2157-7617.1000306
Di Rienzi, M., Ciancio, B., Binkin, N., Perra, A., Prato, R., Bella, A., et al. (2006). Fattori di rischio di morte in occasione delle ondate di calore: risultati di uno studio caso-controllo, bari (estate 2005). Bollettino Epidemiologico Nazionale 19, i—ii.
Duarte, C. M., Losada, I. J., Hendriks, I. E., Mazarrasa, I., and Marbà, N. (2013). The role of coastal plant communities for climate change mitigation and adaptation. Nat. Clim. Chang. 3, 961–968. doi: 10.1038/nclimate1970
Edelson, P. J., Harold, R., Ackelsberg, J., Duchin, J. S., Lawrence, S. J., Manabe, Y. C., et al. (2023). Climate change and the epidemiology of infectious diseases in the united states. Clin. Infect. Dis. 76, 950–956. doi: 10.1093/cid/ciac697
Elia, M., Giannico, V., Lafortezza, R., and Sanesi, G. (2019). Modeling fire ignition patterns in mediterranean urban interfaces. Stochastic Environm. Res. Risk Assessm. 33, 169–181. doi: 10.1007/s00477-018-1558-5
Elia, M., Lafortezza, R., Colangelo, G., and Sanesi, G. (2014). A streamlined approach for the spatial allocation of fuel removals in wildland-urban interfaces. Landsc. Ecol. 29, 1771–1784. doi: 10.1007/s10980-014-0070-7
EU (2015). EU Covenant of Mayors for Climate & Energy. Available online at: https://eu-mayors.ec.europa.eu/en/home (accessed 14 November, 2023).
European Commission (2020). Forging a Climate-Resilient Europe - the New EU Strategy on Adaptation to Climate Change COM(2021) 82 Final. Luxembourg: The European Commission.
FAO (2015). Mediterranean Coastal Lagoons: Sustainable Management and Interactions among Aquaculture, Capture Fisheries and the Environment Studies and Reviews. Rome: General Fisheries Commission for the Mediterranean.
Fatorić, S., Morén-Alegret, R., Niven, R. J., and Tan, G. (2017). Living with climate change risks: stakeholders' employment and coastal relocation in mediterranean climate regions of australia and spain. Environm. Syst. Deci. 37, 276–288. doi: 10.1007/s10669-017-9629-6
Fernández-Montblanc, T., Duo, E., and Ciavola, P. (2020). Dune reconstruction and revegetation as a potential measure to decrease coastal erosion and flooding under extreme storm conditions. Ocean & Coastal Managem. 188, 105075. doi: 10.1016/j.ocecoaman.2019.105075
Franco, A., Fiorin, R., Malavasi, S., Franzoi, P., and Torricelli, P. (2003). Spatial and temporal variation in reproductive and somatic investment of zosterisessor ophiocephalus (pisces, gobiidae) in the venice lagoon. Biol Mar Medit 10, 152–158.
Galli, A., Iha, K., Halle, M., El Bilali, H., Grunewald, N., Eaton, D., et al. (2017). Mediterranean countries' food consumption and sourcing patterns: an ecological footprint viewpoint. Sci. Total Environm. 578, 383–391. doi: 10.1016/j.scitotenv.2016.10.191
García-Ruiz, J. M., López-Moreno, J. I., Vicente-Serrano, S. M., Lasanta-Martínez, T., and Beguería, S. (2011). Mediterranean water resources in a global change scenario. Earth-Sci. Rev. 105, 121–139. doi: 10.1016/j.earscirev.2011.01.006
Gazeau, F., Gattuso, J.-P., Dawber, C., Pronker, A., Peene, F., Peene, J., et al. (2010). Effect of ocean acidification on the early life stages of the blue mussel mytilus edulis. Biogeosciences 7,2051–2060. doi: 10.5194/bg-7-2051-2010
Geere, D. (2014). Adaption to Climate-Related Changes in Seagrass Ecosystems in Chwaka Bay (zanzibar). Göteborg: University of Göteborg.
Gianfreda, F., Mastronuzzi, G., and Sansò, P. (2001). Impact of historical tsunamis on a sandy coastal barrier: an example from the northern gargano coast, southern italy. Natural Hazards and Earth System Sciences 1, 213–219. doi: 10.5194/nhess-1-213-2001
Giangrande, A., Pierri, C., Arduini, D., Borghese, J., Licciano, M., Trani, R., et al. (2020). An innovative imta system: Polychaetes, sponges and macroalgae co-cultured in a southern italian in-shore mariculture plant (ionian sea). J. Mar. Sci. Eng. 8:733. doi: 10.3390/jmse8100733
Giannakopoulos, C., Kostopoulou, E., Varotsos, K. V., Tziotziou, K., and Plitharas, A. (2011). An integrated assessment of climate change impacts for greece in the near future. Regional Environm. Change 11, 829–843. doi: 10.1007/s10113-011-0219-8
Giannakopoulos, C., Le Sager, P., Bindi, M., Moriondo, M., Kostopoulou, E., and Goodess, C. (2009). Climatic changes and associated impacts in the mediterranean resulting from a 2 c global warming. Glob. Planet. Change 68, 209–224. doi: 10.1016/j.gloplacha.2009.06.001
Gifford, S., Dunstan, R. H., O'Connor, W., Koller, C. E., and MacFarlane, G. R. (2007). Aquatic zooremediation: deploying animals to remediate contaminated aquatic environments. Trends Biotechnol. 25, 60–65. doi: 10.1016/j.tibtech.2006.12.002
Giordano, L., Portacci, G., and Caroppo, C. (2019). Multidisciplinary tools for sustainable management of an ecosystem service: The case study of mussel farming in the Mar Piccolo of Taranto (Mediterranean, Ionian Sea). Ocean & Coastal Managem. 176, 11–23. doi: 10.1016/j.ocecoaman.2019.04.013
Giorgi, F., and Lionello, P. (2008). Climate change projections for the mediterranean region. Glob. Planet. Change 63, 90–104. doi: 10.1016/j.gloplacha.2007.09.005
Gómez-Martín, M. B., Armesto-López, X. A., and Martínez-Ibarra, E. (2014). The spanish tourist sector facing extreme climate events: a case study of domestic tourism in the heat wave of 2003. Int. J. Biometeorol. 58, 781–797. doi: 10.1007/s00484-013-0659-6
Gössling, S., Bredberg, M., Randow, A., Sandström, E., and Svensson, P. (2006). Tourist perceptions of climate change: A study of international tourists in zanzibar. Curr. Issues Tour. 9, 419–435. doi: 10.2167/cit265.0
Gravili, C., Belmonte, G., Cecere, E., Denitto, F., Giangrande, A., Guidetti, P., et al. (2010). Nonindigenous species along the apulian coast, italy. Chem. Ecol. 26, 121–142. doi: 10.1080/02757541003627654
Greenwood, J. (2010). Evidence that increased nitrogen efflux from wave-influenced marine sediment enhances pelagic phytoplankton production on the inner continental shelf of Western Australia. Marine Freshwat, Res. 61, 625–632. doi: 10.1071/MF09236
Hinder, S. L., Hays, G. C., Edwards, M., Roberts, E. C., Walne, A. W., and Gravenor, M. B. (2012). Changes in marine dinoflagellate and diatom abundance under climate change. Nat. Clim. Chang. 2, 271–275. doi: 10.1038/nclimate1388
Hinkel, J., and Klein, R. J. (2009). Integrating knowledge to assess coastal vulnerability to sea-level rise: the development of the diva tool. Global Environm. Change 19, 384–395. doi: 10.1016/j.gloenvcha.2009.03.002
Hooyberghs, H., Verbeke, S., Lauwaet, D., Costa, H., Floater, G., and De Ridder, K. (2017). Influence of climate change on summer cooling costs and heat stress in urban office buildings. Clim. Change 144, 721–735. doi: 10.1007/s10584-017-2058-1
Horton, B. P., Kopp, R. E., Garner, A. J., Hay, C. C., Khan, N. S., Roy, K., et al. (2018). Mapping sea-level change in time, space, and probability. Annu. Rev. Environ. Resour. 43, 481–521. doi: 10.1146/annurev-environ-102017-025826
IFAD (2014). Guidelines for Integrating Climate Change Adaptation into Fisheries and Aquaculture Projects. Rome: IFAD.
IPCC (2007). “Climate change 2007: impacts, adaptation and vulnerability,” in Contribution of Working Group II to the Fourth Assessment Report of the Intergovernmental Panel on Climate Change, eds. M. L. Parry, O. F. Canziani, J. P. Palutikof, P. J. van der Linden and C. E. Hanson Geneva: IPCC.
IPCC (2014). “Climate change 2014: synthesis report,” in Contribution of Working Groups I, II and III to the Fifth Assessment Report of the Intergovernmental Panel on Climate Change eds. R. K. Pachauri and L. A. Meyer. Geneva: IPCC.
IPCC (2023). “Climate change 2023: synthesis report,” in Contribution of Working Groups I, II and III to the Sixth Assessment Report of the Intergovernmental Panel on Climate Change, H. Lee and J. Romero. Geneva: IPCC.
IRGC (2020). Involving Stakeholders in the Risk Governance Process. Lausanne: Epfl International Risk Governance Center.
ISPRA (2020). L' acquacoltura italiana in numeri: numero di impianti, produzioni e principali specie allevate (2019 e 2020) Elaborazione ISPRA su dati MiPAAF-CREA, EUROSTAT. Available online at: https://indicatoriambientali.isprambiente.it/sys_ind/1059 (accessed 14 November, 2023).
ISPRA (2023). Ambiente in Italia: uno sguardo d'insieme Annuario dei dati ambientali 2022. Rome: ISPRA.
Jackson, L. P., and Jevrejeva, S. (2016). A probabilistic approach to 21st century regional sea-level projections using rcp and high-end scenarios. Glob. Planet. Change 146, 179–189. doi: 10.1016/j.gloplacha.2016.10.006
Jacobeit, J., Hertig, E., Seubert, S., and Lutz, K. (2014). Statistical downscaling for climate change projections in the mediterranean region: methods and results. Regional Environm. Change 14, 1891–1906. doi: 10.1007/s10113-014-0605-0
Jennings, S., Stentiford, G. D., Leocadio, A. M., Jeffery, K. R., Metcalfe, J. D., Katsiadaki, I., et al. (2016). Aquatic food security: insights into challenges and solutions from an analysis of interactions between fisheries, aquaculture, food safety, human health, fish and human welfare, economy and environment. Fish Fisher. 17, 893–938. doi: 10.1111/faf.12152
Jevrejeva, S., Frederikse, T., Kopp, R., Le Cozannet, G., Jackson, L., and Van De Wal, R. (2019). Probabilistic sea level projections at the coast by 2100. Surveys Geophys. 40, 1673–1696. doi: 10.1007/s10712-019-09550-y
Johnson, D. M., and Haynes, K. J. (2023). Spatiotemporal dynamics of forest insect populations under climate change. Curr. Opin. Insect Sci. 101020. doi: 10.1016/j.cois.2023.101020
Katsanevakis, S., Wallentinus, I., Zenetos, A., Leppäkoski, E., Çinar, M., Oztürk, B., et al. (2014). Impacts of invasive alien marine species on ecosystem services and biodiversity: a pan-european review. Aquatic Invasions 9:391–423. doi: 10.3391/ai.2014.9.4.01
Kibria, A. (2016). Studies on Integrated Multi-Trophic Aquaculture (IMTA) Systems in Freshwater Ponds (PhD thesis). Mymensingh: Department of Aquaculture, Bangladesh Agricultural University.
Koenig, U., and Abegg, B. (1997). Impacts of climate change on winter tourism in the Swiss Alps. J. Sustain. Tour. 5, 46–58. doi: 10.1080/09669589708667275
Laino, E., and Iglesias, G. (2023). Scientometric review of climate-change extreme impacts on coastal cities. Ocean & Coastal Management 242:106709. doi: 10.1016/j.ocecoaman.2023.106709
Lesnikowski, A., Ford, J., Biesbroek, R., Berrang-Ford, L., Maillet, M., Araos, M., et al. (2017). What does the paris agreement mean for adaptation? Climate Policy 17, 825–831. doi: 10.1080/14693062.2016.1248889
Liquete, C., Piroddi, C., Drakou, E. G., Gurney, L., Katsanevakis, S., Charef, A., et al. (2013). Current status and future prospects for the assessment of marine and coastal ecosystem services: a systematic review. PLoS ONE 8, e67737. doi: 10.1371/journal.pone.0067737
Lobeto, H., Menendez, M., and Losada, I. J. (2021). Future behavior of wind wave extremes due to climate change. Sci. Rep. 11, 7869. doi: 10.1038/s41598-021-86524-4
Longo, C., Cardone, F., Corriero, G., Licciano, M., Pierri, C., and Stabili, L. (2016). The co-occurrence of the demosponge hymeniacidon perlevis and the edible mussel mytilus galloprovincialis as a new tool for bacterial load mitigation in aquaculture. Environ. Sci. Pollut. Res. 23, 3736–3746. doi: 10.1007/s11356-015-5587-z
Longo, C., Corriero, G., Licciano, M., and Stabili, L. (2010). Bacterial accumulation by the demospongiae hymeniacidon perlevis: A tool for the bioremediation of polluted seawater. Mar. Pollut. Bull. 60, 1182–1187. doi: 10.1016/j.marpolbul.2010.03.035
Lovato, T., Vichi, M., and Oddo, P. (2013). “High-resolution simulations of mediterranean sea physical oceanography under current and scenario climate conditions: model description, assessment and scenario analysis,” in CMCC Research Paper, 207.
Macchia, F., Cavallaro, V., Forte, L., and Terzi, M. (2000). Vegetazione e clima della Puglia. Cahiers Options Méditerranéennes 53, 33–49.
Madec, G., Bell, M., Blaker, A., Bricaud, C., Bruciaferri, D., Castrillo, M., et al. (2023). Nemo Ocean Engine Reference Manual.
Mani-Peres, C., Xavier, L. Y., Santos, C. R., and Turra, A. (2016). Stakeholders perceptions of local environmental changes as a tool for impact assessment in coastal zones. Ocean & Coastal Managem. 119, 135–145. doi: 10.1016/j.ocecoaman.2015.10.005
Marcos, M., Jorda, G., Le Cozannet, G., Moatti, J., and Thiébault, S. (2016). “Sea level rise and its impacts on the mediterranean. The Mediterranean region under climate change. A scientific update,” in IRD Éditions, Marseille, 265–275. doi: 10.4000/books.irdeditions.23454
Marino, G, Petochi, T, and Cardia, F. (2020). Assegnazione di Zone Marine per l'Acquacoltura (AZA). Guida Tecnica. Rome: ISPRA, 214.
Martinelli, A., Kolokotsa, D.-D., and Fiorito, F. (2020). Urban heat island in Mediterranean coastal cities: The case of Bari (Italy). Climate 8, 79. doi: 10.3390/cli8060079
Martinotti, M. E., Pisano, L., Marchesini, I., Rossi, M., Peruccacci, S., Brunetti, M. T., et al. (2017). Landslides, floods and sinkholes in a karst environment: the 1-6 September 2014 Gargano event, southern Italy. Nat. Hazards Earth Syst. Sci. 17, 467–480. doi: 10.5194/nhess-17-467-2017
Mastronuzzi, G., Pignatelli, C., Sansò, P., and Selleri, G. (2007). Boulder accumulations produced by the 20th of February, 1743 tsunami along the coast of southeastern Salento (Apulia region, Italy). Mar. Geol. 242, 191–205. doi: 10.1016/j.margeo.2006.10.025
Mcleod, E., Chmura, G. L., Bouillon, S., Salm, R., Björk, M., Duarte, C. M., et al. (2011). A blueprint for blue carbon: toward an improved understanding of the role of vegetated coastal habitats in sequestering co2. Front. Ecol. Environ. 9, 552–560. doi: 10.1890/110004
MIBACT (2017). Piano Strategico di Sviluppo del Turismo. Rome: Ministero dei Beni e delle Attività Culturali e del Turismo.
Mirón, I. J., Linares, C., and Díaz, J. (2023). The influence of climate change on food production and food safety. Environ. Res. 216, 114674. doi: 10.1016/j.envres.2022.114674
Morim, J., Hemer, M., Cartwright, N., Strauss, D., and Andutta, F. (2018). On the concordance of 21st century wind-wave climate projections. Glob. Planet. Change 167, 160–171. doi: 10.1016/j.gloplacha.2018.05.005
Morim, J., Hemer, M., Wang, X. L., Cartwright, N., Trenham, C., Semedo, A., et al. (2019). Robustness and uncertainties in global multivariate wind-wave climate projections. Nat. Clim. Chang. 9, 711–718. doi: 10.1038/s41558-019-0542-5
Morris, R. L., Konlechner, T. M., Ghisalberti, M., and Swearer, S. E. (2018). From grey to green: Efficacy of eco-engineering solutions for nature-based coastal defence. Glob. Chang. Biol. 24, 1827–1842. doi: 10.1111/gcb.14063
Neale, P., Williamson, C., Banaszak, A., Häder, D.-P., Hylander, S., Ossola, R., et al. (2023). The response of aquatic ecosystems to the interactive effects of stratospheric ozone depletion, uv radiation, and climate change. Photochem. Photobiol. Sci. 2023, 1–35. doi: 10.1007/s43630-023-00370-z
Neori, A., Chopin, T., Troell, M., Buschmann, A. H., Kraemer, G. P., Halling, C., et al. (2004). Integrated aquaculture: rationale, evolution and state of the art emphasizing seaweed biofiltration in modern mariculture. Aquaculture 231, 361–391. doi: 10.1016/j.aquaculture.2003.11.015
Neubauer, P., and Andersen, K. H. (2019). Thermal performance of fish is explained by an interplay between physiology, behaviour and ecology. Conservat. Physiol. 7:coz025. doi: 10.1093/conphys/coz025
Neumann, B., Vafeidis, A. T., Zimmermann, J., and Nicholls, R. J. (2015). Future coastal population growth and exposure to sea-level rise and coastal flooding-a global assessment. PLoS ONE 10:e0118571. doi: 10.1371/journal.pone.0118571
Nicholls, R. J., Brown, S., Goodwin, P., Wahl, T., Lowe, J., Solan, M., et al. (2018). Stabilization of global temperature at 1.5 c and 2.0 c: implications for coastal areas. Philosoph. Transact. Royal Soc. A: Mathemat. Phys. Eng. Sci. 376:20160448. doi: 10.1098/rsta.2016.0448
Nicholls, R. J., Woodroffe, C., and Burkett, V. (2016). “Coastline degradation as an indicator of global change,” in Climate Change (London: Elsevier), 309–324.
Noro, M., and Lazzarin, R. (2015). Urban heat island in Padua, Italy: Simulation analysis and mitigation strategies. Urban Clim. 14, 187–196. doi: 10.1016/j.uclim.2015.04.004
Olya, H. G., Alipour, H., Peyravi, B., and Dalir, S. (2019). Tourism climate insurance: implications and prospects. Asia Pacific J. Tour. Res. 24, 269–280. doi: 10.1080/10941665.2018.1564338
Orr, J. C., Fabry, V. J., Aumont, O., Bopp, L., Doney, S. C., Feely, R. A., et al. (2005). Anthropogenic ocean acidification over the twenty-first century and its impact on calcifying organisms. Nature 437, 681–686. doi: 10.1038/nature04095
Orr, S. A., Richards, J., and Fatorić, S. (2021). Climate change and cultural heritage: a systematic literature review (2016-2020). Historic Environm.: Policy & Practice 12, 434–477. doi: 10.1080/17567505.2021.1957264
Otrachshenko, V., and Nunes, L. C. (2022). Fire takes no vacation: Impact of fires on tourism. Environm. Dev. Econom. 27, 86–101. doi: 10.1017/S1355770X21000012
Papageorgiou, N., Dimitriou, P. D., Chatzivasileiou, D., Tsapakis, M., and Karakassis, I. (2023). Can IMTA provide added ecosystem value services in the fish farms of Greece? Front. Mar. Sci. 9:1083099. doi: 10.3389/fmars.2022.1083099
Parisi, G., Terova, G., Gasco, L., Piccolo, G., Roncarati, A., Moretti, V. M., et al. (2014). Current status and future perspectives of italian finfish aquaculture. Rev. Fish Biol. Fish 24, 15–73. doi: 10.1007/s11160-013-9317-7
Petrosillo, I., Scardia, A. M. S., Ungaro, N., Specchiulli, A., Fanelli, G., Centoducati, G., et al. (2023). Towards sustainable marine spatial planning of aquaculture. Ecol. Indic. 154:110542. doi: 10.1016/j.ecolind.2023.110542
Pile, A. J., Patterson, M. R., and Witman, J. D. (1996). In situ grazing on plankton. Mar. Ecol. Prog. Ser. 141, 95–102. doi: 10.3354/meps141095
Pilli-Sihvola, K., Aatola, P., Ollikainen, M., and Tuomenvirta, H. (2010). Climate change and electricity consumption–witnessing increasing or decreasing use and costs? Energy Policy 38, 2409–2419. doi: 10.1016/j.enpol.2009.12.033
Pugliapromozione (2023). I principali trend del turismo in Puglia. Bari: Agenzia Regionale del Turismo “Pugliapromozione”.
Range, P., Chícharo, M., Ben-Hamadou, R., Piló, D., Matias, D., Joaquim, S., et al. (2011). Calcification, growth and mortality of juvenile clams ruditapes decussatus under increased pco2 and reduced ph: variable responses to ocean acidification at local scales? J. Exp. Mar. Biol. Ecol. 396, 177–184. doi: 10.1016/j.jembe.2010.10.020
Reale, M., Cossarini, G., Lazzari, P., Lovato, T., Bolzon, G., Masina, S., et al. (2022). Acidification, deoxygenation, and nutrient and biomass declines in a warming mediterranean sea. Biogeosciences 19, 4035–4065. doi: 10.5194/bg-19-4035-2022
Reid, G. K., Gurney-Smith, H. J., Flaherty, M., Garber, A. F., Forster, I., Brewer-Dalton, K., et al. (2019). Climate change and aquaculture: considering adaptation potential. Aquacult. Environm. Interact. 11, 603–624. doi: 10.3354/aei00333
Reimann, L., Vafeidis, A. T., Brown, S., Hinkel, J., and Tol, R. S. (2018). Mediterranean UNESCO World Heritage at risk from coastal flooding and erosion due to sea-level rise. Nat. Commun. 9:4161. doi: 10.1038/s41467-018-06645-9
Reiswig, H. M. (1975). Bacteria as food for temperate-water marine sponges. Can. J. Zool. 53, 582–589. doi: 10.1139/z75-072
Rizzetto, F. (2020). “Effects of climate change on the morphological stability of the Mediterranean coasts: consequences for tourism,” in Climate Change, Hazards and Adaptation Options. Climate Change Management, eds W. Leal Filho, G. Nagy, M. Borga, P. Chávez Muñoz, and A. Magnuszewski (Cham: Springer).
Rockel, B., Will, A., and Hense, A. (2008). The regional climate model COSMO-CLM (CCLM). Meteorologische zeitschrift 17:347. doi: 10.1127/0941-2948/2008/0309
Sarker, P., Pilote, A., Auffret, M., Proulx, É., Villemur, R., Deschamps, M.-H., et al. (2014). Reducing geosmin off-flavor compounds and waste outputs through dietary phosphorus management in rainbow trout aquaculture. Aquacult. Environm. Interact. 6, 105–117. doi: 10.3354/aei00119
Scheraga, J. D., and Grambsch, A. E. (1998). Risks, opportunities, and adaptation to climate change. Climate Res. 11, 85–95. doi: 10.3354/cr011085
Scoccimarro, E., Gualdi, S., Bellucci, A., Sanna, A., Giuseppe Fogli, P., Manzini, E., et al. (2011). Effects of tropical cyclones on ocean heat transport in a high-resolution coupled general circulation model. J. Clim. 24, 4368–4384. doi: 10.1175/2011JCLI4104.1
Scott, D., Gössling, S., and Hall, C. M. (2012). International tourism and climate change. Wiley Interdisciplinary Rev.: Climate Change 3, 213–232. doi: 10.4324/9780203127490
Scott, D., and Lemieux, C. (2010). Weather and climate information for tourism. Procedia Environm. Sci, 1, 146–183. doi: 10.1016/j.proenv.2010.09.011
Seneviratne, S., Nicholls, N., Easterling, D., Goodess, C., Kanae, S., Kossin, J., et al. (2012). “Changes in climate extremes and their impacts on the natural physical environment,” in Managing the Risks of Extreme Events and Disasters to Advance Climate Change Adaptation, eds. C. B. Field, V. Barros, T. F. Stocker, D. Qin, D. J. Dokken, K. L. Ebi (Cambridge: Cambridge University Press), 109–230.
Sesana, E., Gagnon, A. S., Ciantelli, C., Cassar, J., and Hughes, J. J. (2021). Climate change impacts on cultural heritage: a literature review. Wiley Interdisciplinary Rev.: Climate Change 12:e710. doi: 10.1002/wcc.710
Shaposhnikov, D., Revich, B., Bellander, T., Bedada, G. B., Bottai, M., Kharkova, T., et al. (2014). Mortality related to air pollution with the Moscow heat wave and wildfire of 2010. Epidemiology (Cambridge, Mass.) 25:359. doi: 10.1097/EDE.0000000000000090
Siikamäki, J., Sanchirico, J. N., Jardine, S., McLaughlin, D., and Morris, D. (2013). Blue carbon: coastal ecosystems, their carbon storage, and potential for reducing emissions. Environment 55, 14–29. doi: 10.1080/00139157.2013.843981
Simolo, C., Brunetti, M., Maugeri, M., and Nanni, T. (2014). Increasingly warm summers in the euro-mediterranean zone: mean temperatures and extremes. Regional Environm. Change 14, 1825–1832. doi: 10.1007/s10113-012-0373-7
Simoncelli, S., Fratianni, C., Pinardi, N., Grandi, A., Drudi, M., Oddo, P., et al. (2019). Mediterranean Sea Physical Reanalysis (Cmems Med-Physics) (version 1) Ramonville-Saint-Agne: Copernicus Monitoring Environment Marine Service (CMEMS).
Sims, D. W., Wearmouth, V. J., Genner, M. J., Southward, A. J., and Hawkins, S. J. (2004). Low-temperature-driven early spawning migration of a temperate marine fish. J. Anim. Ecol. 73, 333–341. doi: 10.1111/j.0021-8790.2004.00810.x
Sreejariya, P., Gallardo, W., and Dabbadie, L. (2011). The Role of Integrated Multi-Trophic Aquaculture (IMTA) in Climate Change Mitigation and Adaptation. Bangkok: Asian Institute of Technology.
Stabili, L., Giangrande, A., Arduini, D., Borghese, J., Petrocelli, A., Alabiso, G., et al. (2023). Environmental quality improvement of a mariculture plant after its conversion into a multi-trophic system. Sci. Total Environm. 884:163846. doi: 10.1016/j.scitotenv.2023.163846
Todaro, V., D'Oria, M., Secci, D., Zanini, A., and Tanda, M. G. (2022). Climate change over the mediterranean region: Local temperature and precipitation variations at five pilot sites. Water 14:2499. doi: 10.3390/w14162499
Torresan, S., Critto, A., Rizzi, J., Marcomini, A., Mendez, F., Leschka, S., et al. (2012). Assessment of coastal vulnerability to climate change hazards at the regional scale: the case study of the north adriatic sea. Natural Hazards & Earth Syst. Sci. 12:7. doi: 10.5194/nhess-12-2347-2012
Trenberth, K. E., Fasullo, J. T., and Shepherd, T. G. (2015). Attribution of climate extreme events. Nat. Clim. Chang. 5, 725–730. doi: 10.1038/nclimate2657
Troell, M., Joyce, A., Chopin, T., Neori, A., Buschmann, A. H., and Fang, J.-G. (2009). Ecological engineering in aquaculture-potential for integrated multi-trophic aquaculture (IMTA) in marine offshore systems. Aquaculture 297, 1–9. doi: 10.1016/j.aquaculture.2009.09.010
Tsirintanis, K., Azzurro, E., Crocetta, F., Dimiza, M., Froglia, C., Gerovasileiou, V., et al. (2022). Bioinvasion impacts on biodiversity, ecosystem services, and human health in the mediterranean sea. Aquatic Invasions 17, 308–352. doi: 10.3391/ai.2022.17.3.01
Unioncamere-Puglia (2022). Report di Analisi Economico-Territoriale Per Unioncamere Puglia. Bari: Unioncamere Puglia.
Vaquer-Sunyer, R., and Duarte, C. M. (2011). Temperature effects on oxygen thresholds for hypoxia in marine benthic organisms. Glob. Chang. Biol. 17, 1788–1797. doi: 10.1111/j.1365-2486.2010.02343.x
Vilà, M., Basnou, C., Pyšek, P., Josefsson, M., Genovesi, P., Gollasch, S., et al. (2010). How well do we understand the impacts of alien species on ecosystem services? A pan-european, cross-taxa assessment. Front. Ecol. Environm. 8, 135–144. doi: 10.1890/080083
Villalba, A., Casas, S. M., López, C., and Carballal, M. J. (2005). Study of perkinsosis in the carpet shell clam Tapes decussatus in Galicia (NW Spain). II. Temporal pattern of disease dynamics and association with clam mortality. Dis. Aquatic Organ. 65, 257–267. doi: 10.3354/dao065257
Villalba, A., Reece, K. S., Ordás, M. C., Casas, S. M., and Figueras, A. (2004). Perkinsosis in molluscs: a review. Aquat. Living Resour. 17, 411–432. doi: 10.1051/alr:2004050
Vousdoukas, M. I., Mentaschi, L., Voukouvalas, E., Bianchi, A., Dottori, F., and Feyen, L. (2018). Climatic and socioeconomic controls of future coastal flood risk in europe. Nat. Clim. Chang. 8, 776–780. doi: 10.1038/s41558-018-0260-4
Vousdoukas, M. I., Ranasinghe, R., Mentaschi, L., Plomaritis, T. A., Athanasiou, P., Luijendijk, A., et al. (2020). Sandy coastlines under threat of erosion. Nat. Clim. Chang. 10, 260–263. doi: 10.1038/s41558-020-0697-0
Wang, B., Hua, L., Mei, H., Wu, X., Kang, Y., and Zhao, N. (2024). Impact of climate change on the dynamic processes of marine environment and feedback mechanisms: an overview. Arch. Comput. Methods Eng. 132. doi: 10.1007/s11831-024-10072-z
Wolff, J. G., and Beaumont, A. (2011). Shellfish sequestration: The augmented cultivation of molluscs, and the preservation of their shells, as a means of sequestering carbon dioxide. Acessado em 4:10.
Zachariadis, T. (2010). Forecast of electricity consumption in cyprus up to the year 2030: The potential impact of climate change. Energy Policy 38, 744–750. doi: 10.1016/j.enpol.2009.10.019
Keywords: climate impacts, adaptation strategies, coastal erosion, aquaculture, tourism
Citation: Parete G, Bruno MF, Calabrese P, Carlucci R, Chiarulli M, D'Onghia G, Fiore A, Fratino U, Longo C, Longo F, Scorrano S and Gentile F (2024) Climate impacts and adaptation strategies for coastal erosion, aquaculture, and tourism along the Adriatic side of Apulia region. Front. Clim. 6:1378253. doi: 10.3389/fclim.2024.1378253
Received: 29 January 2024; Accepted: 08 April 2024;
Published: 25 April 2024.
Edited by:
Ana Baricevic, Rudjer Boskovic Institute, CroatiaReviewed by:
Mercy J. Borbor-Cordova, ESPOL Polytechnic University, EcuadorManob Das, Mykolas Romeris University, Lithuania
Copyright © 2024 Parete, Bruno, Calabrese, Carlucci, Chiarulli, D'Onghia, Fiore, Fratino, Longo, Longo, Scorrano and Gentile. This is an open-access article distributed under the terms of the Creative Commons Attribution License (CC BY). The use, distribution or reproduction in other forums is permitted, provided the original author(s) and the copyright owner(s) are credited and that the original publication in this journal is cited, in accordance with accepted academic practice. No use, distribution or reproduction is permitted which does not comply with these terms.
*Correspondence: Maria Francesca Bruno, bWFyaWFmcmFuY2VzY2EuYnJ1bm9AcG9saWJhLml0