- Faculty of Computer and Information System, Islamic University of Madinah, Madinah, Saudi Arabia
Electricity is still a highly demanding resource in developing countries, especifically in rural areas. Local communities in developing countries are now focusing on generating their own electricity using solar and trading the energy which is in access to the supplier. Energy trading at the peer-to-peer level is a novel paradigm. It allows customers to contribute energy to the grid and power generation and supply companies. In this paper, we investigate the energy trading enabling technologies, their applications, challenges, and benefits to the local communities from various aspects. We analyse how blockchain and IoT technologies can help energy trading at the customer level from recent research proposals. We also present our analysis of recent research on the implementation of energy trading technologies and future directions.
1. Introduction
Many developing countries are unable to meet the increasing demand for electricity, especifically rural areas suffer from the shortage of electricity (Shrestha et al., 2019). According to the world bank survey, Bank (2019) related to access to electricity worldwide suggests that around 22.5% of rural and 3% of the urban populations around the world still do not have access to electricity. Considering the lack of electricity from official suppliers and the availability of renewable sources of energy such as solar systems, the concept of peer-to-peer energy trading has emerged in the last 3–4 years. People are not only employing solar energy systems to fulfill their needs but also trading electricity for access to the local grid or electricity suppliers. These renewable energy sources (RES), such as solar panels and wind turbines, are environment-friendly and economical.
The concept of distributed energy resource (DER) network has played the role of the catalyst in peer-to-peer energy trading. DER consists of a network of energy generation sources distribution, storage, and load management. These DER systems are not further matured and are known as peer-to-peer distributed energy trading (P2P DET), its components include renewable energy producer (prosumer), consumer, and distributed energy management systems. According to International Renewable Energy Agency (IREA), P2P energy trading is bringing innovation in this area and has a bright future (Irena, 2020c). The acceptability of P2P DET has improved due to the advanced technologies such as Blockchain and Internet of Things (IoT). This is to support each stage of the process (Mengelkamp et al., 2018). The P2P DET allows energy exchange by peers in a distributed manner to either other peers or the energy supplier companies. This exchange can also help rural areas of developing countries where there is no electricity from the central suppliers. The farmers in the village can produce their electricity using either solar panels or wind turbines to not only fulfill their needs but also trade with the nearby regions.
The advancement in technologies has enabled the realization of P2P ET. First, the improvement was made in existing technologies such as utility sale batteries behind the meters, flexibility in existing plants, and the emergence of renewable mini-grids, and super grids. Then, blockchain, IoT technologies with artificial intelligence were emerged. Inherited characteristics of blockchain technology such as distributed ledger, transparency, smart contracts, and integrity, and consistency of transactions are a perfect match for enabling P2P energy trading, especially for distributed renewable energy resources management and its trading. Therefore, blockchain for P2P energy trading has become a cutting-edge research area for the research community in the last few years. IoT is the concept of interconnecting various things that can sense physical objects and communicate data through the interconnection for analysis and interpretation. Recently, various IoT-based smart energy platform has been proposed to solve various aspects related to renewable energy such as energy efficiency enhancement, its management, and trading. IoT technology can also be used to connect various things and services in a microgrid through information gateway architecture. Artificial Intelligence and various algorithms introduce learning and intelligence in distributing energy trading process.
There are a handful of surveys in the recent few years on some specific aspects of P2P energy trading, such as Von Wirth et al. (2018) and Andoni et al. (2019), reviewing proposals related to P2P energy trading in smart grids. Andoni et al. (2019) discussed challenges associated with P2P energy trading and proposed architecture and Von Wirth et al. (2018) briefly reviewed the energy trading models and required framework. However, in this survey, we covered all important aspects related to P2P energy trading, including associated challenges, enabling technologies, especifically blockchain and IoT including in-depth analysis, comparison of methods used, proposed architectures, its benefits, and applications in detail. Figure 1 shows the structure of our survey in this study including our classification of P2P ET enabling technologies, their applications, and challenges.
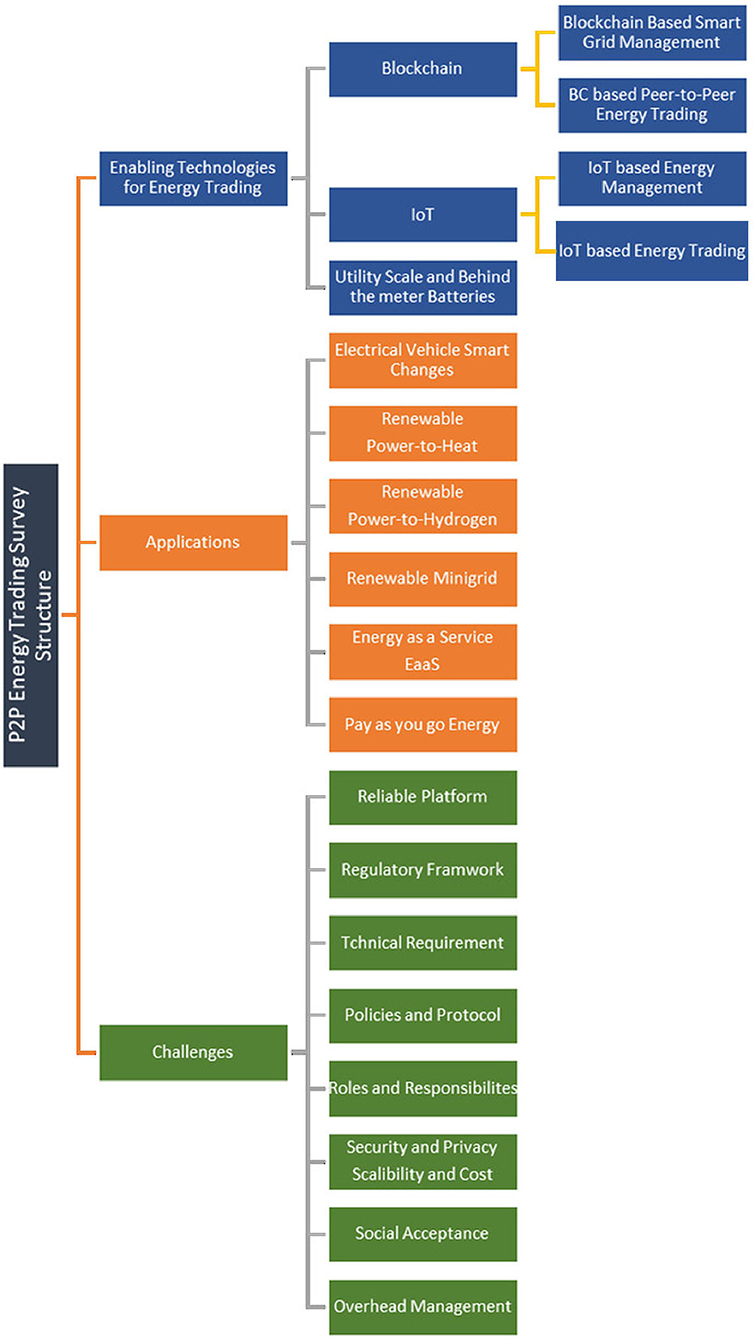
Figure 1. Our study and classification of peer-to-peer Energy Trading technologies applications and challenges.
The rest of the study is organized as follows. Section 2 presents the key challenges associated with energy trading. Section 3 presents energy trading's current and future applications. Section 4 discusses in detail the enabling technologies for P2P distributed energy trading for local communities. Section 5 summarizes and presents our analysis of this study. Finally, we conclude our work in this paper including future directions.
2. Challenges in energy trading
There are a number of challenges associated with the implementation of peer-to-peer energy trading at the customer level. Also, many factors are important for the successful deployment of such distributed P2P ET systems in developing countries. In this section, we highlight major factors that can lead to the successful development of such a system and then discuss the major challenges associated with the implementation of such a system (Andoni et al., 2019; Irena, 2020c).
2.1. Reliable platforms
A reliable platform is the most important requirement for the successful deployment of any P2P system. The platform should manage various layers involved. For example, at the physical layer, the producer of the electricity produces data at the peer level related to the amount of energy they are producing in access for trading. Second, the consumers who are in need of energy will send their energy requirements to the platform. Thus, a reliable platform is required to manage the data from both producers and consumers of the electricity in real-time with a high degree of accuracy and availability. The platform should also maintain logs of trading. The reliability of the platform will depend on two important parameters of availability and accuracy of the platform. Few such platforms have been proposed in the literature in recent years which is reviewed in the next section.
2.2. Regulatory framework
A regularity framework is another major challenge and requirement for successful P2P energy trading at the customer level. The framework should define regulations favorable for all stakeholders at both local neighborhood and regional levels. The rights and obligations of all parties involved in energy trading should be well defined without any bias. Alongside these general regulations and trading, parties could also trade based on an agreed contract. The European Commission introduced such regulations in 2018, which can be taken as an example for developing countries.
2.3. Attaining technical requirements
To achieve effective results of implementation of P2P energy trading, the stakeholders must work together to fulfill technical requirements at the hardware, software, and communication protocol level. At the hardware level, we must define how the distribution network will be organized in either micro, mini or smart grids. The process of billing done using either existing electric meters or smart meters will be required for this purpose. At the software level, technology will be adapted to enable trading such as blockchain or IoT. It is important to know which algorithms could be applied for load balancing, billing, and maintaining the log of the transaction at an affordable cost.
2.4. Policies and protocols
Conducive policies are required for effective utilization of renewable energy production and its trading at the local grid or smart grid. Policies should also define a road map for the existing grid systems to enable P2P energy trading without any conflict with the existing authorities. Policies to encourage P2P energy trading initiatives should be implemeneted in developing countries at both government, private companies, and producer levels. Protocols are required for effective coordination between various stakeholders in the system including communication protocols between producer and consumer levels.
2.5. Roles and responsibilities
The roles and responsibilities should be well defined for all stakeholders involved in the energy trading system including producers, consumers of energy, platform providers, and market operators.
2.6. Security and privacy
For the successful implementation of a P2P energy trading system, it is important to ensure the desired level of security and privacy. It not only requires security at the general abstract level of the entire system but also focuses on security at devices, platforms, and payment methods used by the stakeholders during the P2P energy trading. It is necessary to ensure secure access to the trading system by all entities and protecting the privacy and confidentiality of the significant information of various stakeholders and transactions in the system. To ensure the availability of the trading system for authentic users, It's essential to protect it against denial of service attacks. We must also take measures to ensure secure payment between the trading peers and the ability to deal with any conflict related to the payments. Then, we must store energy trading transactions in a way that they are temper proof and traceable.
2.7. Scalability and cost
Another key challenge is the scalability of such energy trading systems and the rates of energy being traded. Currently, different scenarios of energy trading systems are proposed in terms of the number of prosumer and consumers from small scale to local and city-wide electricity grids. It is also important to see the impact of scalability on the overall cost and rates of energy units traded in the system.
2.8. Social acceptance
Social acceptance of energy trading between the common prosumer and consumer by the community is also important (Von Wirth et al., 2018).
2.9. Overhead management
One core challenge is the management of various overheads in the energy trading system, such as cryptocurrency system integration with the payment system. The variation in the rates of cryptocurrency is not consistent, which also poses a challenge for the stakeholders.
3. Energy trading applications
Renewable energy trading has brought about various innovative ideas and existing power section operations and business management. According to the IRENA report (Irena, 2019d), the landscape of renewable power has the potential for innovations in various dimensions, and it can be used by both end users and the power industry around the world. Now we discuss P2P renewable energy trading in the existing and future applications.
3.1. Electric vehicle smart charging
Recently, we observe some commercial energy organizations applying renewable energy trading for electrical vehicle (EV) smart charging. For example, Smart Energy International company from the Netherlands (Nhede, 2018) has enabled energy trading between EV owners and the local energy market. They enable the integration of the EV charging platform with the energy trading tool Exergy (Dincer and Rosen, 2021). The concept of the vehicle to grid (V2G) has emerged which has introduced bi-directional energy trading. It provides a big opportunity for peers to trade energy for access to the smart grid. However, due to the expected large number of users in the future, it is important to investigate such trading infrastructure scalability and security for reliable energy trading between EV owners and power distribution companies (Javaid and Sikdar, 2021). In an article (Greencar Congress, 2018), authors review an initial project between eMotorWreaks and Lo3 Energy that will connect the EV charging platform with the Lo3 energy marketplace to enable energy trading. They also proposed the implementation architecture as shown in Figure 2.
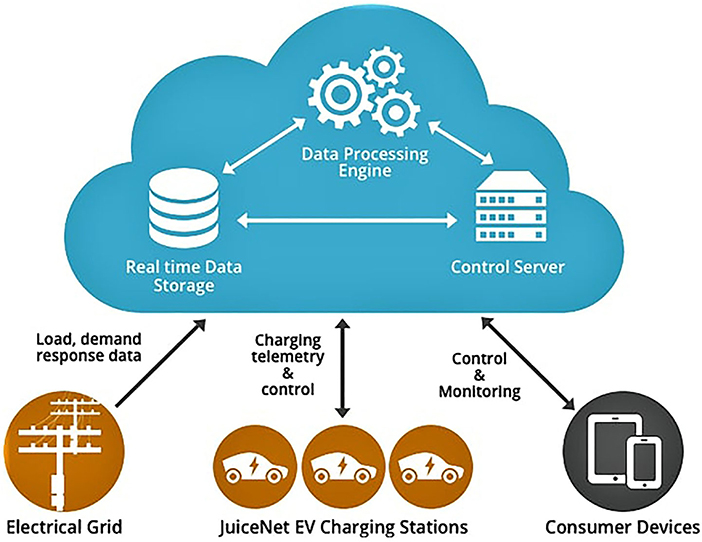
Figure 2. An integration architecture of energy trading between local energy grids and EV charging stations (Greencar Congress, 2018).
3.2. Renewable power-to-heat
There are many countries where average temperatures are very low most part of the year. These colder countries spend more electricity for providing constant heating at home, government, and private organizations than general electricity consumption. Therefore, the concept of renewable energy trading can also be used as a power to heat. In Bloess et al. (2018), authors have studied the integration of renewable energy trading for power-to-heat including its various aspects such as modeling approaches and enabling technologies. The findings of their study indicate that power-to-heat using renewable technologies can be cost-effective as compared to traditional energy sources. In another example (Energiewende, 2014), a Germany-based power company has investigated the role of power-to-heat in renewable energy integration. They investigate the potential commercial use of power-to-heat and make future recommendations. They also propose the residential power-to-heat options shown in Figure 3.
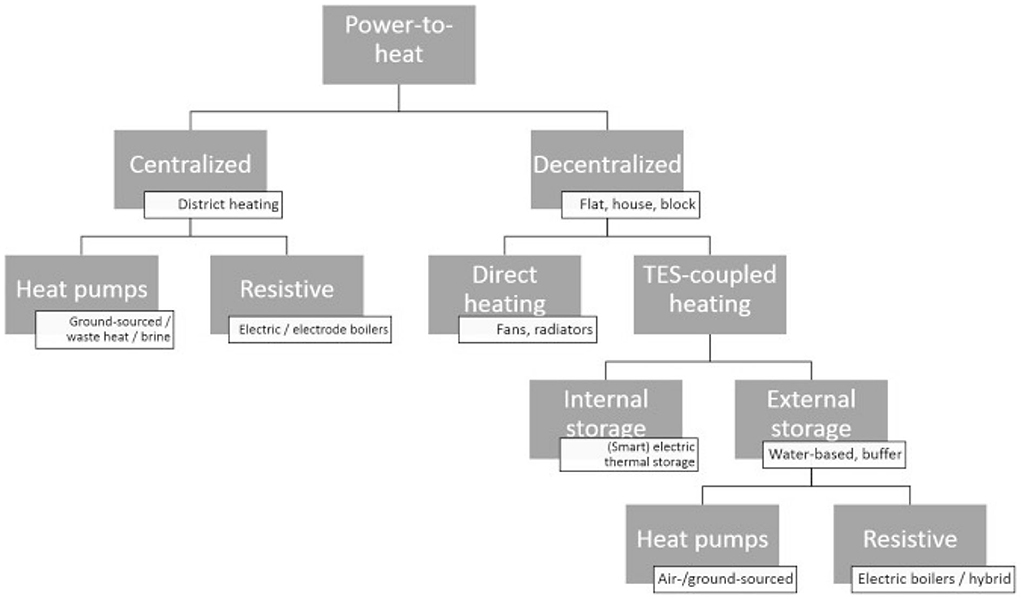
Figure 3. Residential power-to-heat options (Energiewende, 2014).
3.3. Renewable power-to-hydrogen
Experts predict that hydrogen will be the future energy resource for the majority of users. Therefore, they suggest producing hydrogen from renewable sources. In a recent meeting of IRENA in 2020, they discussed the prospects of producing hydrogen from renewable energy sources. They have also studied the prospect of using hydrogen as a clean energy source for a better environment (Irena, 2019a) as shown in Figure 4. The Oxford institute for energy studies has recently analyzed the role of hydrogen in the future energy transition (Lambert, 2021).
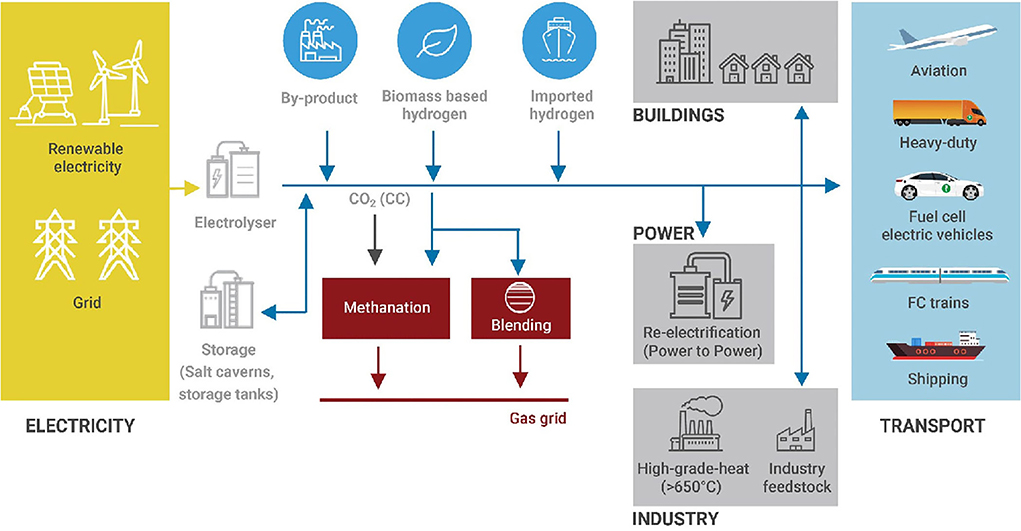
Figure 4. Renewable power-to-hydrogen architecture (Irena, 2019a).
3.4. Renewable mini grids
Renewable mini-grid get the supply of electricity from the main grid to their charging battery and then either utilize or trade the energy to other consumers. According to the study by IRENA (Irena, 2016), the renewable mini-grid can help to provide power supply in unserved areas as shown in Figure 5. These mini-grids could rely on solar, wind, or other sources based on existing environmental conditions. In Zebra et al.'s (2021) study, authors have studied the renewable energy system using mini-grids for off-grid electrification in developing countries. They also investigated the use of mini-grids in rural areas.
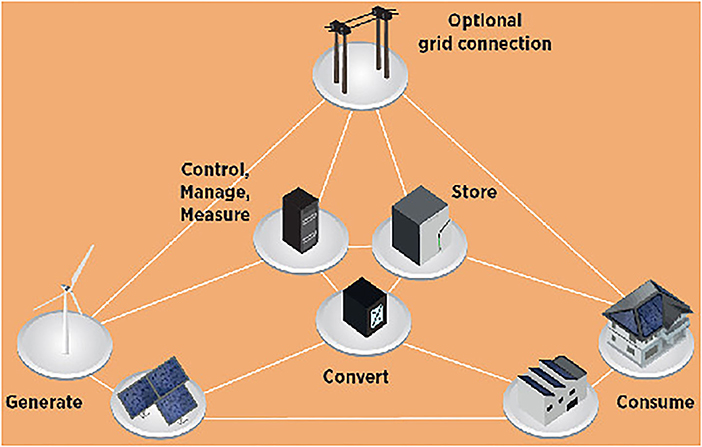
Figure 5. A design of renewable mini grid (Irena, 2016).
3.5. New business model and market design for power systems
There are various new business models related to energy distribution and management have been proposed since the emergence of the P2P energy trading concept. For example, energy as a service (EaaS) allows more than just supplying electricity to the consumers including technology, analytics, and personalized services. According to the study by IRENA (Irena, 2020b), EaaS unlocks demand-side flexibility and can enable performance-based control of various energy-related services. Pay-as-you-go energy is another example of a new business model which allows the user to pay as they use the energy, either electricity or gas. The smart pay-as-you-go energy model has already been implemented in the UK by various energy companies such as SSE Smart Pay As You Go (SSE Energy Services, 2022). The smart meter at home allows user to not only view their usage but also can manage it according to their needs.
3.6. Improved system operations
Renewable energy and its trading have brought about new techniques to improve the overall operations of energy distribution and management. For example, the advanced forecasting algorithms to predict the renewable energy (solar & wind) output based on analyzing weather-related data. The IRENA report (Irena, 2020a) has suggested that accurate forecasting of the output of solar and wind energy can provide stability to the power distribution system. In another example, virtual power lines storage system at both supply and demand sides allows large-scale integration of renewable energy without grid congestion (Irena, 2020d). Similarly, to reduce congestion in power lines, dynamic line ratings are now used. Ngoko et al. (2018) have studied the benefits of dynamic line ratings on intermittent renewable energy.
4. Enabling technologies for energy trading
Peer-to-peer renewable energy trading has created an online marketplace that is expected to grow aggressively in the next 5–10 years. Technologies play an important role in the realization of P2P trading goals. First, we observed a great improvement in the existing battery technologies such as utility-scale batteries and behind-the-meter batteries. Second, the emergence of new technologies, such as blockchain and IoT with artificial intelligence, has served as major enabling technologies for P2P renewable energy trading in recent years. We presented a comparative analysis of the suitability, existing proposals, and real project implementation of these enabling technologies for P2P energy trading.
4.1. Utility scale and behind the meter batteries
Utility scale and behind-the-meter batteries are stationary batteries designed to last for many years. These batteries connect with the power generation transmission network and have the capacity to store several megawatt for hours. A behind-the-meter battery sits on the customer premises for smooth energy flow. Utility-scale batteries allow storage of excess renewable energy to increase the capacity of the grid and get the maximum advantage of variable renewable energy produced by either solar or wind systems. According to IRENA (Irena, 2019c), utility-scale battery storage systems increase the flexibility of the energy grid system as shown in Figure 6. The study predicted that utility-scale batteries allow greater input of variable renewable energy into the grid for reliable and cheaper energy trading. Furthermore, these storage systems of utility-scale batteries offer various other services as illustrated in Figure 6 (Irena, 2019c).
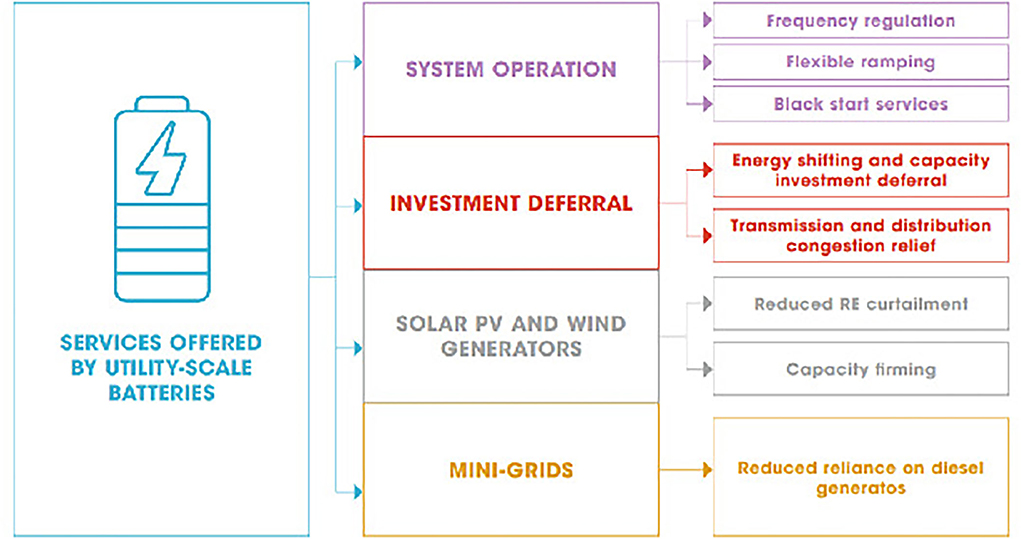
Figure 6. Services offer by utility scale batteries (Irena, 2019c).
Unlike utility-scale batteries that allow storage at the front of the meter such as at grid distribution or transmission network, the storage of behind-the-meter batteries allows consumers to manage their energy demand and usage for cost-effectiveness and management. According to the study (Irena, 2019b), behind the meter batteries, small-scale storage support local renewable energy generation by increasing self-consumption and its revenue. In addition, these storage systems allow consumers of electricity to decrease self-consumption, backup power, saving on electricity bills, and several other services related to system operations and mini-grid as illustrated in Figure 7 (Irena, 2019b).
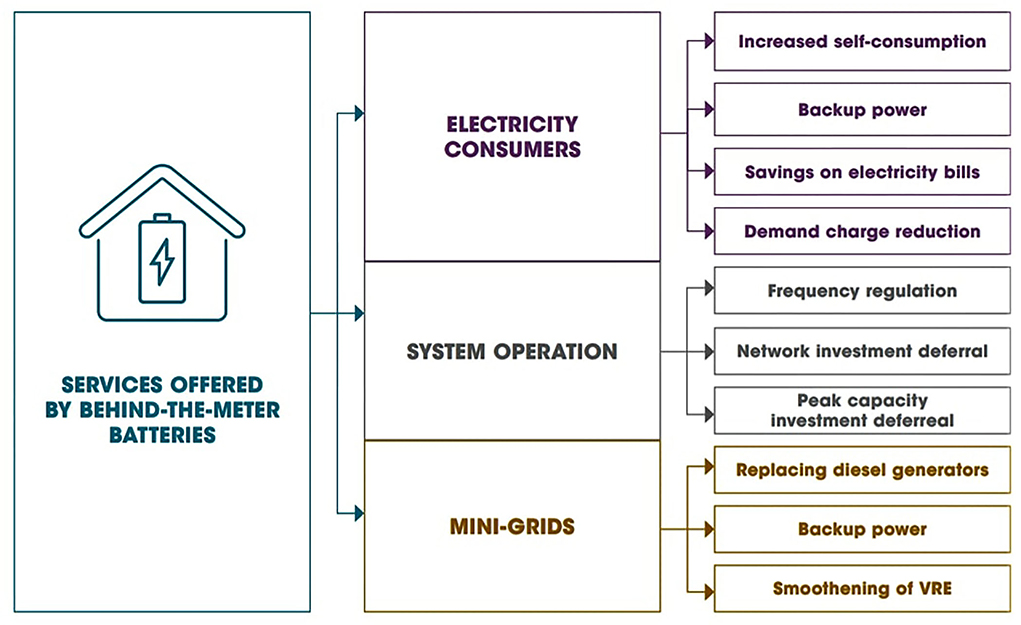
Figure 7. Services offer by behind the meter batteries (Irena, 2019b).
4.2. Blockchain as energy trading enabling technology
As the name suggests, the blockchain is the block connected in a chain, where a block can be seen as a storage of data and its related transactions. Technically speaking, it is a distributed ledger technology that replaces the third-party concept in centralized security systems. That means, a secure transaction among various parties can be achieved without the intervention of a central authority. Thus, it enables a secure transaction between nodes in the network and records each transaction. It utilizes the consensus algorithm and smart contract concepts for agreement between stakeholders in the network.
Blockchain as an enabling technology has evolved (Bhutta et al., 2021) in the recent 5 years. Blockchain technology has been applied (Syed et al., 2019) and tested in various walks of life, for example, in healthcare (Wang et al., 2018; Zarour et al., 2020; Khatri et al., 2021); business (Mukkamala et al., 2018; Conway and Garimella, 2020; Garcia-Garcia et al., 2020; Syed et al., 2020); finance (Guerar et al., 2020; Zhang et al., 2021); supply chain (Saberi et al., 2019; Chang and Chen, 2020; Valle and Oliver, 2020); security apps (Vishwakarma and Das, 2020; Bai and Liu, 2021; Mendki, 2021); payments systems (Pouraghily and Wolf, 2019; Zouina and Outtai, 2019; Li et al., 2020a); electrical vehicle industry (Li et al., 2021; Xu et al., 2021); and property registration (Ali et al., 2020b).
Now, we will review the current and future applications scenarios of blockchain in energy management and trading.
4.2.1. Blockchain-based smart grid management
Blockchain was first considered for decentralized management of energy systems (Cioara et al., 2020), and various smart grid management using blockchain have been proposed. Cioara et al. (2020) proposed novel features of blockchain-based smart grid administration including using smart contracts, metering of energy data, and secure registration of stakeholders in the energy system. Similarly, Pop et al. (2018) have investigated the use of blockchain for decentralized management of energy demand and response in the smart energy grid as shown in Figure 8. They suggested storing information on a distributed ledger, collecting information on energy presumption from IoT meters, defining the flexibility of energy presumption using a smart contract, and ruling to balance between demand and supply of energy at the energy grid level.
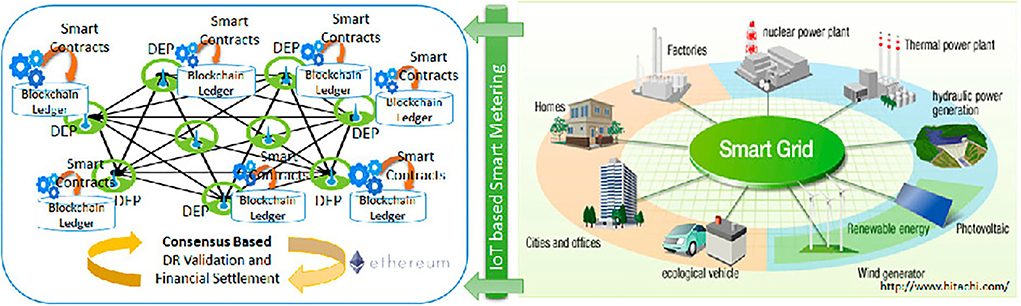
Figure 8. Blockchian based decentralized management of energy grid (Pop et al., 2018).
Samy et al. (2021) explored the provision of security for the smart grids using blockchain-based systems. They first surveyed the use of blockchain for smart grids in general and then analyzed the ways blockchain can secure various processes in energy management in a grid. They proposed a way to deal with fake data injection attacks in the energy system. In another example (Badra and Borghol, 2021), the author presented how blockchain can be used to preserve the privacy of users in the smart energy grid and also proposed how other attacks such as data injection, data forgery, and replay attack can be detected using this technology. Recently, the proof of authority of a blockchain consensus algorithm applicability is examined for smart grid (Chikezie et al., 2021). They also proposed how blockchain can be used for P2P ET. A new energy trading scheme for the virtual power plants is proposed in Seven et al. (2020). They first highlighted the challenges related to P2P energy trading in a virtual power plant and then implemented a smart contract and tested it in a virtual power plan framework. They validated their proposed architecture using Ethereum virtual machine. Alladi et al. (2019) have presented a comprehensive review of blockchain in smart grids and its various use cases including its commercial implementation. They also highlighted the challenges for blockchain-based smart grids including scalability issues, development and infrastructure cost, and legal and regularity support.
4.2.2. Blockchain based peer-to-peer energy trading
Inherent features of blockchain allow P2P ET. For example, Niloy et al. (2021) have proposed a Blockchain-based P2P ET system. It facilitates the local consumer in two major ways: (1) it allows consumers to trade the energy generated from renewable sources to the grid and (2) the integration with a smart microgrid allows it to remain functional in the case of isolation from the official national grid. The proposed smart contract design consists of rules on transaction settlement and asset exchange schema to abide by the rules of transparent blockchain transactions between stakeholders.
Thakur and Breslin (2020) have claimed to develop a method to perform a cost analysis of blockchain-based peer-to-peer energy trading systems. The method estimates the trade-off between the cost of a blockchain network and the profit from energy trading. The main factor contributing to the overall cost is the cost of mining the blockchain and defines the workflow of each miner of the blockchain network.
Lee et al. (2019) have proposed software architecture for blockchain-based P2P trading systems between neighbors. The proposed software architecture consists of two smart contracts. The first is built on Ethereum for trading price determination and the second finds prosumers and consumers based on estimated price. To provide proof of concept they build a private Ethereum network using Raspberry Pi devices. Figure 9 shows the core functionality of the proposed architecture for blockchain-based P2P energy trading.
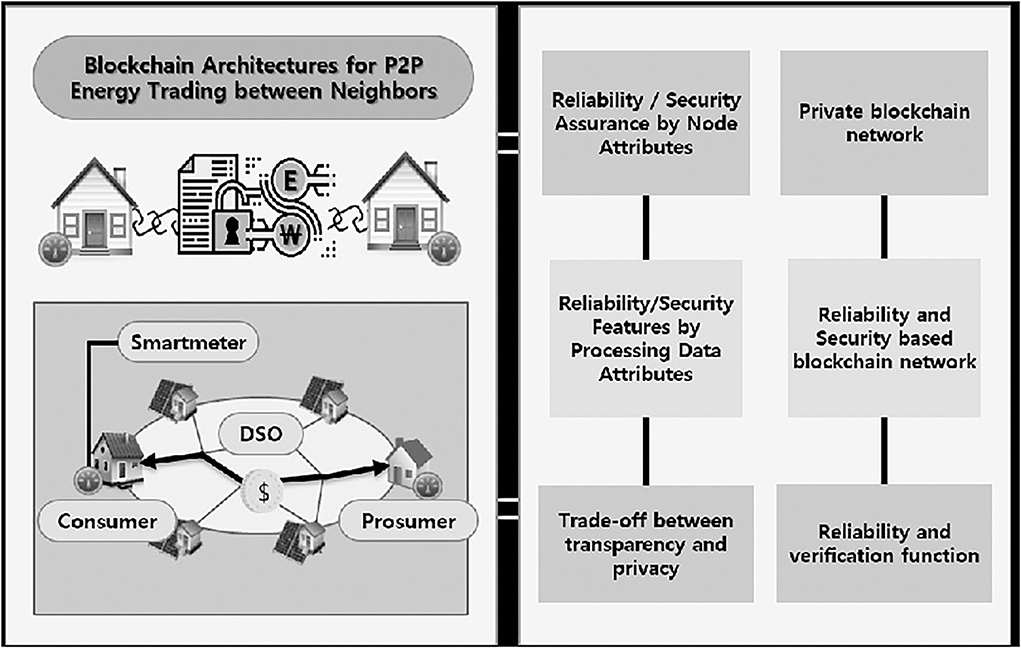
Figure 9. Blockchain-based architecture for P2P energy trading between neighbors (Lee et al., 2019).
Pee et al. (2019) proposed a smart blockchain-based energy trading platform. The idea is to use the smart contract and the concept of tokens for payment to allow peers to freely trade their energy access to their needs. The system generated ERC20 tokens as defined in smart contracts. Payment is done using the generated token and energy is transferred using an energy storage system.
4.3. IoT as energy trading enabling technology
Internet of things refers to any physical object of the real world that has the capability of sensing, processing/communicating wirelessly. We can also describe IoT as an internet-based information architecture that enables the communication of services, data, and goods through IoT devices connected to the network. IoT networks are generally large and produce big data which is then analyzed by applying various artificial intelligence techniques for improving process.
Along with blockchain, IoT is the evolutionary technology of this era. IoT technology has already been implemented in various fields including healthcare (Haghi et al., 2020; Asokan et al., 2021; Bhuiyan et al., 2021; Mehmood et al., 2021; Sharma et al., 2022); smart cities (Zafeiriou, 2020; Hajam and Sofi, 2021; Kaushik and Bagga, 2021; Nadeem et al., 2021); security (Siddiqui et al., 2019, 2020); business (Aagaard et al., 2019; Ali et al., 2019, 2020a; Fattouch et al., 2020; Torres et al., 2020); and agriculture (Azfar et al., 2018; Kassim, 2020; Syed et al., 2020) etc.
This study briefly reviewed the recent use of IoT for energy trading and highlighted the future directions.
4.3.1. IoT-based energy management and trading
Internet of things has recently been proposed for both energy management and trading. We now review the recent proposals for IoT for both energy management and energy trading. For example, Verma and Prakash (2019) have studied the challenges and issues in IoT-based intelligent energy management. The challenges included the availability of IoT sensors and scalability (i.e., dealing with a huge number of IoT devices). It highlighted the issues of security of IoT devices, addressing, and vulnerability of losing data. Nguyen et al. (2020) first studied how an energy service provider and ISP interact and then proposed energy trading and time scheduling for low-power IoT networks. The idea was to maximize the network throughput along with making it energy efficient. Ku et al. (2017) proposed an IoT-based smart energy management platform for micro grids. The main idea is to provide energy efficiency and its trading using IoT in supply transfer systems utilizing the information about energy. Figure 10 shows the proposed architecture of the IoT energy information gateway. The figures show how they collect the information about the requirement and the use of energy through the IoT gateway and then utilize this information to enhance the efficacy of utilized energy and sharing/trading of energy that is in access to the grid.
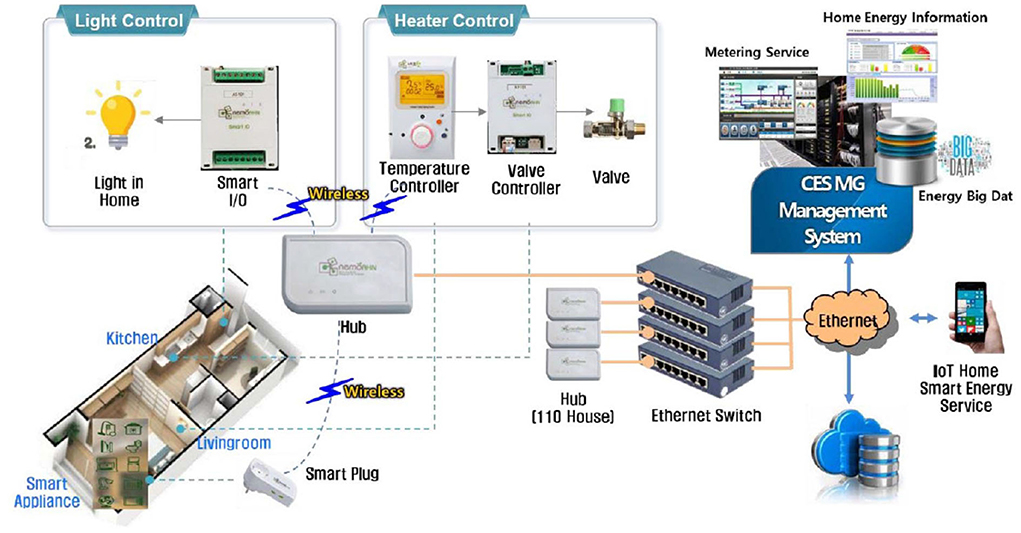
Figure 10. Proposed architecture of IoT energy management gateway (Ku et al., 2017).
Salvi et al. (2020) proposed the use renewable energy through solar panels and presented an IoT-based prototype for energy trading. Figure 11 shows the proposed IoT-based energy trading using a renewable energy source. It can be seen from the diagram that the proposed system utilizes solar panels on the roof of the houses which are connected with two types of battery systems: (a) primary battery and (b) secondary battery. These batteries are connected to IoT power nodes which work as a gateway device. The primary battery is used to provide energy to the electronic devices at home. In contrast, the secondary battery stores the energy in access from the solar system and trade this energy to other peers connected with them. They also proposed an app that can be used by homeowners for both monitoring and trading electricity Salvi et al. (2020). They also highlighted the requirements analysis and initial implementation of the proposed prototype.
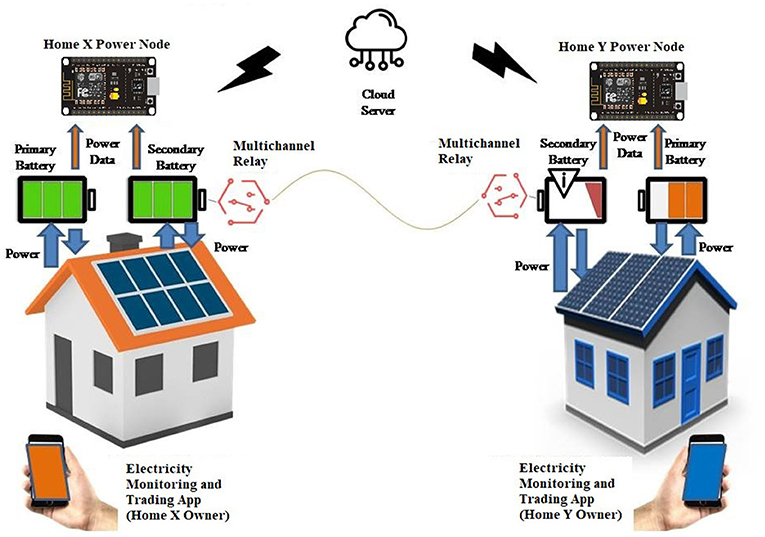
Figure 11. Proposed architecture of IoT-based energy trading using solar system (Salvi et al., 2020).
Nguyen et al. (2021) proposed energy trading and time scheduling for heterogeneous wireless powered backscattered IoT networks. They proposed an economic framework to gain the highest throughput in IoT service and energy efficiency in energy service by using the Stackelberg game. Figure 12 shows the proposed model. The authors claimed that their findings suggest that improving both energy trading and time allocation of IoT networks improves the overall profit as compared to the usual transmission method.
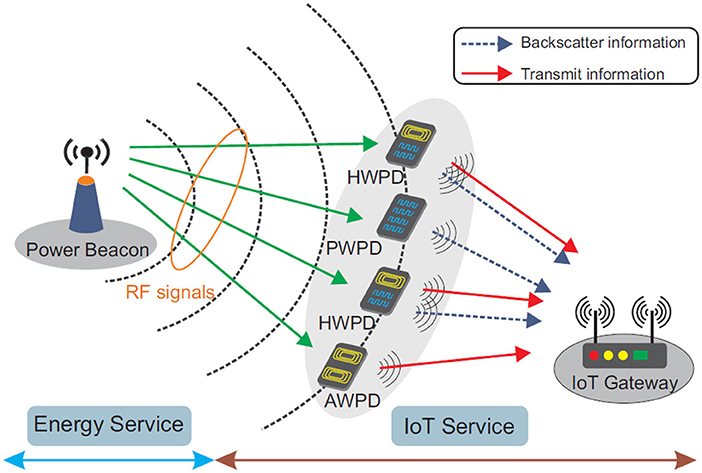
Figure 12. Proposed model for energy trading for heterogeneous wireless power IoT networks (Nguyen et al., 2021).
4.3.2. IoT and blockchain-based energy trading
There are some proposals in the literature where the researchers suggest utilizing a combination of blockchain and IoT technologies for energy trading. For example, Li et al. (2020b) considered energy trading in industrial IoT and first highlighted the weak security of various proposed energy trading models against attacks such as cheating attacks. Then they developed a consortium blockchain between stakeholders for providing secure and record energy trading transactions. The authors implemented a prototype by a local Ethereum test network and analyzed the security of Fenechain. The proposed energy trading blockchain is shown in Figure 13. Similar proposals for using blockchain for Industrial IoT are proposed in Lin et al. (2017) Hou et al. (2019), and Zhu et al. (2022).
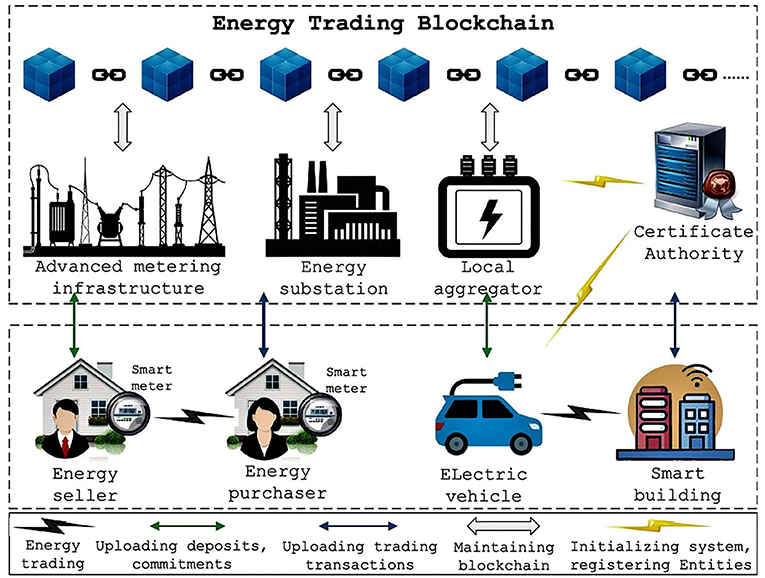
Figure 13. Proposed model of energy trading blockcahin for IoT (Li et al., 2020b).
In another example, Sanju et al. (2018) have performed a comparison of blockchain platforms for IoT based on energy. They argued that there are various blockchain platforms to provide security for IoT solutions in various fields. However, there are no comparative studies available to determine the best blockchain implementation for IoT solutions. Therefore, [83] presented an energy-based comparison of blockchain platforms including Ethereum and Hyperledger fabric platforms.
Baig et al. (2020) proposed IoT and blockchain-based P2P energy trading platform. In the proposed model, the peers can participate in trading energy independently. The proposed platform is shown in Figure 14 that includes all basic features, such as energy metering, its transfer, and secure and transparent payment transfer using the Ethereum blockchain.
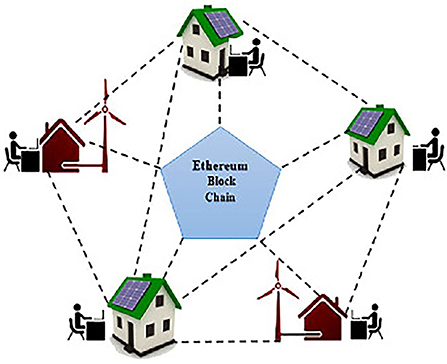
Figure 14. Architecture of proposed blockchain and IoT based P2P energy trading platform (Baig et al., 2020).
Researchers recently have suggested using the game theory approach for peer-to-peer energy trading. For example, Malik et al. (2020) have proposed a collation-based game theory approach to increase the overall profit of trading. They also simulated several trading scenarios and analyzed their operational performance. In another example, Yap et al. (2020) have proposed a motivational game theory-based P2P ET framework as a case study for Malaysia. Similarly, An et al. (2020) have proposed a design for distributed energy trading for energy internet using game theory. A genetic algorithm was also considered by some researchers for distributed energy trading, for example, Hyacinth et al. (2021) have proposed a genetic algorithm for improving the stability of voltage in the hybrid energy system. Similarly, Teive et al. (2010) have suggested using genetic algorithms for energy trading risk management.
5. Summary and analysis
We have summarized our review of P2P energy trading technologies from the recent research conducted mainly in the recent 5 years in Table 1. Based on our recent review of the literature, we have divided the P2P energy trading technologies from the recent literature into four main categories: blockchain-based, IoT-based, blockchain and IoT-based, and utility-scale batteries. The first category of blockchain-based energy trading is further divided into smart grid management and P2P energy trading, and Table 1 shows the research work focus on each of the categories. It suggests that blockchain technology is generally considered a transparent way to manage smart grid transactions. In terms of P2P trading, the blockchain is used both as a means of developing consensus among energy trading parties and performing transparent transactions. Similarly, IoT has also been proposed by various researchers as a technology that can enable P2P energy trading utilizing inherited features of the technology. Following review, we have classified IoT-based solutions into two categories. The first one is energy trading in industrial IoT and the second is general IoT networks, and Table 1 shows the relevant studies in these two categories. We also notice that there is some proposal that utilizes both blockchain and IoT technologies to take advantage of key features of both emerging technologies. Table 1 also highlights the energy trading implementation platforms used by various researchers including Ethereum, Hyperledger Fabric, and smart/micro/mini-grid. The bottom of Table 1 indicates the papers discussing the applications of P2P energy trading. We have categorized it into six categories including EV smart charging, renewable power-to-heat, renewable power-to-hydrogen, renewable microgrid, EaaS, and pays-as-you-go energy.
Figure 15 shows the distribution of four major methods used in recent research on P2P energy trading technologies. The consensus and smart contracts are integral parts of blockchain technology, which is aggressively proposed by researchers for P2P energy trading in the power grid, load balancing, and scheduling of energy in micro-grid. The consensus algorithm enables the parties involved in the transaction to agree on a certain value, rule, or parameter. After consensus, all parties sign a smart contract to carry out the energy trading. We observe that the distributed algorithms are the second method employed by the researcher. Distributed algorithms are generally used in grid computing and process control applications. These algorithms generally have high throughput, so effective for P2P energy trading, but sometimes it is difficult to coordinate multiple processes in parallel. Game theory is the third methodology employed by some researchers for P2P energy trading as it is a good method to gather and structure knowledge from stakeholders. Genetic algorithms are also suggested by a few researchers as a method for P2P energy trading as it is faster but can give an inaccurate solution sometimes.
6. Conclusion and future work
We considered the importance of growing energy demand and the new concept of customers trading the energy in surplus with other peers of the national grid. In this paper, we performed a systematic review of recent research work conducted in the recent 5 years on P2P energy trading including the aspects of its challenges, applications, and enabling technologies. We observed that most of the researcher has mainly proposed to use the blockchain and IoT technology for P2P energy trading. But, we have categorized enabling technology into three categories after including the utility-scale behind the meter batteries which are key to storing and managing the energy produced from renewable sources in order to enable its trading. We also highlighted the implementation platform employed by the researchers to test their proposal related to P2P energy trading. In the future, we will develop blockchain-based energy trading prototype applications, as inherent features of blockchain, such as transparency, security, and smart contract, are highly suitable for P2P energy trading. We are planning to perform a case study in a rural area of KSA to first present the feasibility of using renewable energy sources and then motivate them to participate in energy trading at the peer level. In addition, we are looking forward to developing blockchain-based prototype application for testing its performance analysis for energy trading.
Author contributions
AN: conceptualization, investigation, methodology, writing review and editing, and funding acquisition.
Funding
This research was supported by the Deanship of Scientific Research, Islamic University of Madinah, Madinah (KSA), under Tammayuz Program Grant No. 1442/505.
Conflict of interest
The author declares that the research was conducted in the absence of any commercial or financial relationships that could be construed as a potential conflict of interest.
Publisher's note
All claims expressed in this article are solely those of the authors and do not necessarily represent those of their affiliated organizations, or those of the publisher, the editors and the reviewers. Any product that may be evaluated in this article, or claim that may be made by its manufacturer, is not guaranteed or endorsed by the publisher.
References
Aagaard, A., Presser, M., and Andersen, T. (2019). “Applying iot as a leverage for business model innovation and digital transformation,” in 2019 Global IoT Summit (GIoTS) (Aarhus: IEEE).
Ali, J. A., Nasir, Q., and Dweiri, F. T. (2019). “Business continuity management framework of internet of things (IoT),” in 2019 Advances in Science and Engineering Technology International Conferences (ASET) (Dubai: IEEE).
Ali, M. A. M., Basahr, A., Rabbani, M. R., and Abdulla, Y. (2020a). “Transforming business decision making with internet of things (IoT) and machine learning (ML),” in 2020 International Conference on Decision Aid Sciences and Application (DASA) (Sakheer: IEEE).
Ali, T., Nadeem, A., Alzahrani, A., and Jan, S. (2020b). “A transparent and trusted property registration system on permissioned blockchain,” in 2019 International Conference on Advances in the Emerging Computing Technologies (AECT) (Al Madinah Al: IEEE).
Alladi, T., Chamola, V., Rodrigues, J. J. P. C., and Kozlov, S. A. (2019). Blockchain in smart grids: a review on different use cases. Sensors 19, 4862. doi: 10.3390/s19224862
An, E., Sun, Q., Hu, J., and Wang, R. (2020). “A differential game approach to distributed energy trading for energy internet,” in 2020 IEEE 4th Conference on Energy Internet and Energy System Integration (EI2) (Wuhan), 3608–3613.
Andoni, M., Robu, V., Flynn, D., Abram, S., Geach, D., Jenkins, D., et al. (2019). Blockchain technology in the energy sector: a systematic review of challenges and opportunities. Renew. Sustain. Energy Rev. 100, 143–174. doi: 10.1016/j.rser.2018.10.014
Asokan, A., Priyanka, B., Thenmozhi, S., and Sumathi, K. (2021). “IoT based healthcare system for emergency medical services,” in 2021 5th International Conference on Electronics, Communication and Aerospace Technology (ICECA) (Coimbatore: IEEE).
Azfar, S., Nadeem, A., Alkhodre, A., Ahsan, K., Mehmood, N., Alghmdi, T., et al. (2018). Monitoring, detection and control techniques of agriculture pests and diseases using wireless sensor network: a review. Int. J. Adv. Comput. Sci. Appl. 9, 260. doi: 10.14569/IJACSA.2018.091260
Badra, M., and Borghol, R. (2021). “Privacy-preserving and efficient aggregation for smart grid based on blockchain,” in 2021 11th IFIP International Conference on New Technologies, Mobility and Security (NTMS) (Paris: IEEE).
Bai, L., and Liu, L. (2021). “Research on software defined network security model based on blockchain,” in 2021 6th International Conference on Intelligent Computing and Signal Processing (ICSP) (Xi'an: IEEE).
Baig, M. J. A., Iqbal, M. T., Jamil, M., and Khan, J. (2020). “IoT and blockchain based peer to peer energy trading pilot platform,” in 2020 11th IEEE Annual Information Technology, Electronics and Mobile Communication Conference (IEMCON) (Vancouver, BC: IEEE).
Bank, W. (2019). Access to Electricity (% of Population). World Bank Global Electrification Database Portal. Available online at: https://data.worldbank.org/indicator/EG.ELC.ACCS.ZS
Bhuiyan, M. N., Rahman, M. M., Billah, M. M., and Saha, D. (2021). Internet of things (IoT): A review of its enabling technologies in healthcare applications, standards protocols, security, and market opportunities. IEEE Internet Things J. 8, 10474–10498. doi: 10.1109/JIOT.2021.3062630
Bhutta, M. N. M., Khwaja, A. A., Nadeem, A., Ahmad, H. F., Khan, M. K., Hanif, M. A., et al. (2021). A survey on blockchain technology: Evolution, architecture and security. IEEE Access 9, 61048–61073. doi: 10.1109/ACCESS.2021.3072849
Bloess, A., Schill, W.-P., and Zerrahn, A. (2018). Power-to-heat for renewable energy integration: a review of technologies, modeling approaches, and flexibility potentials. Appl. Energy 212, 1611–1626. doi: 10.1016/j.apenergy.2017.12.073
Chang, S. E., and Chen, Y. (2020). When blockchain meets supply chain: a systematic literature review on current development and potential applications. IEEE Access 8, 62478–62494. doi: 10.1109/ACCESS.2020.2983601
Chikezie, U., Karacolak, T., and Prado, J. C. D. (2021). “Examining the applicability of blockchain to the smart grid using proof-of-authority consensus,” in 2021 IEEE 9th International Conference on Smart Energy Grid Engineering (SEGE) (Oshawa, ON: IEEE).
Cioara, T., Pop, C., Zanc, R., Anghel, I., Antal, M., and Salomie, I. (2020). “Smart grid management using blockchain: future scenarios and challenges,” in 2020 19th RoEduNet Conference: Networking in Education and Research (RoEduNet) (Bucharest: IEEE).
Conway, D., and Garimella, K. (2020). Enhancing trust in business ecosystems with blockchain technology. IEEE Eng. Manag. Rev. 48, 24–30. doi: 10.1109/EMR.2020.2970387
Dincer, I., and Rosen, M. A. (2021). “Chapter 5-applications of exergy in industry,” in Exergy, 3rd Edn, eds I. Dincer and M. A. Rosen (Elsevier), 91–100.
Energiewende, A. (2014). “The role of power-to-heat in renewable energy integration,” in Agora Energiewende, Smart Energy for Europe Platform (SEFEP) (Berlin).
Fattouch, N., Lahmar, I. B., and Boukadi, K. (2020). “IoT-aware business process: comprehensive survey, discussion and challenges,” in 2020 IEEE 29th International Conference on Enabling Technologies: Infrastructure for Collaborative Enterprises (WETICE) (Bayonne: IEEE).
Garcia-Garcia, J. A., Sanchez-Gomez, N., Lizcano, D., Escalona, M. J., and Wojdynski, T. (2020). Using blockchain to improve collaborative business process management: systematic literature review. IEEE Access 8, 142312–142336. doi: 10.1109/ACCESS.2020.3013911
Greencar Congress. (2018). “eMotorWerks and LO3 Energy partner on local energy trading platform between EVs and renewable microgrid projects,” in eMotor works (Green Car Congress). Available online at: https://www.greencarcongress.com/2018/12/20181210-lo3.html
Guerar, M., Merlo, A., Migliardi, M., Palmieri, F., and Verderame, L. (2020). A fraud-resilient blockchain-based solution for invoice financing. IEEE Trans. Eng. Manag. 67, 1086–1098. doi: 10.1109/TEM.2020.2971865
Haghi, M., Neubert, S., Geissler, A., Fleischer, H., Stoll, N., Stoll, R., et al. (2020). A flexible and pervasive IoT-based healthcare platform for physiological and environmental parameters monitoring. IEEE Internet Things J. 7, 5628–5647. doi: 10.1109/JIOT.2020.2980432
Hajam, S. S., and Sofi, S. A. (2021). IoT-fog architectures in smart city applications: a survey. China Commun. 18, 117–140. doi: 10.23919/JCC.2021.11.009
Hou, W., Guo, L., and Ning, Z. (2019). Local electricity storage for blockchain-based energy trading in industrial internet of things. IEEE Trans. Ind. Inform. 15, 3610–3619. doi: 10.1109/TII.2019.2900401
Hyacinth, L. R., Sheela, A., Prathiba, S., Alwin Joseph, J., Arul Victor, M., Febin Joseph, T., et al. (2021). “Improvement in voltage stability by optimal location and sizing of hybrid energy sources by genetic algorithm,” in 2021 International Conference on Advancements in Electrical, Electronics, Communication, Computing and Automation (ICAECA) (Bayonne: IEEE), 1–7.
Irena (2016). “Innovation outlook: Renewable mini-grids,” in Innovation Outlook: Renewable Mini-Grids (Abu Dhabi: International Renewable Energy Agency).
Irena (2019a). “Hydrogen: a renewable energy perspective,” in Hydrogen: A Renewable Energy Perspective (Abu Dhabi: International Renewable Energy Agency).
Irena (2019b). “Innovation landscape brief: behind-the-meter batteries,” in Innovation Landscape Brief: Behind-the-Meter Batteries (Abu Dhabi: International Renewable Energy Agency).
Irena (2019c). “Innovation landscape brief: utility-scale batteries,” in Innovation Landscape Brief: Utility-Scale Batteries (Abu Dhabi: International Renewable Energy Agency).
Irena (2019d). “Innovation landscape for a renewable-powered future: Solutions to integrate variable renewables,” in Innovation Landscape for a Renewable-Powered Future: Solutions to Integrate Variable Renewables (Abu Dhabi: International Renewable Energy Agency).
Irena (2020a). “Innovation landscape brief: advanced forecasting of variable renewable power generation,” in Innovation landscape brief: Advanced Forecasting of Variable Renewable Power Generation (Abu Dhabi: International Renewable Energy Agency).
Irena (2020b). “Innovation landscape brief: energy as a service,” in Energy as a Service (Abu Dhabi: International Renewable Energy Agency).
Irena (2020c). “Innovation landscape brief: Peer-to-peer electricity trading,” in Peer-To-Peer Electricity Trading Innovation Landscape Brief (Abu Dhabi: International Renewable Energy Agency).
Irena (2020d). “Virtual power lines, international renewable energy agency,” in Virtual power lines, International Renewable Energy Agency (Abu Dhabi: International Renewable Energy Agency).
Javaid, U., and Sikdar, B. (2021). “A lightweight and secure energy trading framework for electric vehicles,” in 2021 International Conference on Sustainable Energy and Future Electric Transportation (SEFET) (Hyderabad: IEEE), 1–6.
Kassim, M. R. M. (2020). “IoT applications in smart agriculture: Issues and challenges,” in 2020 IEEE Conference on Open Systems (ICOS) (Kota Kinabalu: IEEE).
Kaushik, N., and Bagga, T. (2021). “Smart cities using IoT,” in 2021 9th International Conference on Reliability, Infocom Technologies and Optimization (Trends and Future Directions) (ICRITO) (Noida: IEEE).
Khatri, S., Alzahrani, F. A., Ansari, M. T. J., Agrawal, A., Kumar, R., and Khan, R. A. (2021). A systematic analysis on blockchain integration with healthcare domain: scope and challenges. IEEE Access 9, 84666–84687. doi: 10.1109/ACCESS.2021.3087608
Ku, T.-Y., Park, W.-K., and Choi, H. (2017).“IoT energy management platform for microgrid,” in 2017 IEEE 7th International Conference on Power and Energy Systems (ICPES) (Toronto, ON|: IEEE).
Lambert, M. (2021). “The role of hydrogen in the energy transition,” in Oxford Energy Forum (Oxford: Oxford Institute for Energy Studies).
Lee, B. S., Song, J. G., Moon, S. J., Park, I. H., and Jang, J. W. (2019). “Blockchain architectures for p2p energy trading between neighbors,” in 2019 International Conference on Information and Communication Technology Convergence (ICTC) (Jeju: IEEE).
Li, D., Wong, W. E., Chau, M., Pan, S., and Koh, L. S. (2020a). “A survey ofNFC mobile payment: challenges and solutions using blockchain and cryptocurrencies,” in 2020 7th International Conference on Dependable Systems and Their Applications (DSA) (Xi'an: IEEE).
Li, M., Hu, D., Lal, C., Conti, M., and Zhang, Z. (2020b). Blockchain-enabled secure energy trading with verifiable fairness in industrial internet of things. IEEE Trans. Ind. Inform. 16, 6564–6574. doi: 10.1109/TII.2020.2974537
Li, Z., Chen, S., and Zhou, B. (2021). Electric vehicle peer-to-peer energy trading model based on SMES and blockchain. IEEE Trans. Appl. Superconduct. 31, 1–4. doi: 10.1109/TASC.2021.3091074
Lin, C.-C., Deng, D.-J., Liu, W.-Y., and Chen, L. (2017). Peak load shifting in the internet of energy with energy trading among end-users. IEEE Access 5, 1967–1976. doi: 10.1109/ACCESS.2017.2668143
Malik, S., Duffy, M., Thakur, S., Hayes, B., and Breslin, J. G. (2020). “Cooperative game theory based peer to peer energy trading algorithm,” in The 12th Mediterranean Conference on Power Generation, Transmission, Distribution and Energy Conversion (MEDPOWER 2020), Vol. 2020, 135–142.
Mehmood, A., Nadeem, A., Ashraf, M., Siddiqui, M. S., Rizwan, K., and Ahsan, K. (2021). A fall risk assessment mechanism for elderly people through muscle fatigue analysis on data from body area sensor network. IEEE Sens. J. 21, 6679–6690. doi: 10.1109/JSEN.2020.3043285
Mendki, P. (2021). “Securing cloud native applications using blockchain,” in 2021 12th International Conference on Information and Communication Systems (ICICS) (Valencia: IEEE).
Mengelkamp, E., Gärttner, J., Rock, K., Kessler, S., Orsini, L., and Weinhardt, C. (2018). Designing microgrid energy markets: a case study: The brooklyn microgrid. Appl Energy 210, 870–880. doi: 10.1016/j.apenergy.2017.06.054
Mukkamala, R. R., Vatrapu, R., Ray, P. K., Sengupta, G., and Halder, S. (2018). Blockchain for social business: principles and applications. IEEE Eng. Manag. Rev. 46, 94–99. doi: 10.1109/EMR.2018.2881149
Nadeem, A., Rizwan, K., Mehmood, A., Qadeer, N., Noor, F., and AlZahrani, A. (2021). “A smart city application design for efficiently tracking missing person in large gatherings in madinah using emerging IoT technologies,” in 2021 Mohammad Ali Jinnah University International Conference on Computing (MAJICC) (Karachi: IEEE).
Ngoko, B. O., Sugihara, H., and Funaki, T. (2018). Effect of dynamic line ratings on optimal dispatch considering uncertainty costs due to intermittent renewable energy. IFAC-PapersOnLine 51, 185–190. doi: 10.1016/j.ifacol.2018.11.699
Nguyen, N.-T., Nguyen, D. N., Hoang, D. T., Huynh, N. V., Dutkiewicz, E., Nguyen, N.-H., et al. (2021). Time scheduling and energy trading for heterogeneous wireless-powered and backscattering-based IoT networks. IEEE Trans. Wireless Commun. 20, 6835–6851. doi: 10.1109/TWC.2021.3077018
Nguyen, N.-T., Nguyen, D. N., Hoang, D. T., Huynh, N. V., Nguyen, H.-N., Nguyen, Q. T., et al. (2020). “Energy trading and time scheduling for energy-efficient heterogeneous low-power IoT networks,” in GLOBECOM 2020-2020 IEEE Global Communications Conference (Taipei: IEEE).
Nhede, N. (2018). Partnership Integrates ev Charging and Energy Trading to Enhance Consumer Flexibility. Eindhoven: Smart Energy International.
Niloy, F. A., Nayeem, M. A., Rahman, M. M., and Dowla, M. N. U. (2021). “Blockchain-based peer-to-peer sustainable energy trading in microgrid using smart contracts,” in 2021 2nd International Conference on Robotics, Electrical and Signal Processing Techniques (ICREST) (Dhaka: IEEE), 61–66.
Pee, S. J., Kang, E. S., Song, J. G., and Jang, J. W. (2019). “Blockchain based smart energy trading platform using smart contract,” in 2019 International Conference on Artificial Intelligence in Information and Communication (ICAIIC) (Okinawa: IEEE).
Pop, C., Cioara, T., Antal, M., Anghel, I., Salomie, I., and Bertoncini, M. (2018). Blockchain based decentralized management of demand response programs in smart energy grids. Sensors 18, 162. doi: 10.3390/s18010162
Pouraghily, A., and Wolf, T. (2019). “A lightweight payment verification protocol for blockchain transactions on IoT devices,” in 2019 International Conference on Computing, Networking and Communications (ICNC) (Honolulu, HI: IEEE).
Saberi, S., Kouhizadeh, M., and Sarkis, J. (2019). Blockchains and the supply chain: Findings from a broad study of practitioners. IEEE Eng. Manag. Rev. 47, 95–103. doi: 10.1109/EMR.2019.2928264
Salvi, S., Kumar, S., and Jacob, N. D. (2020). “Saur sikka: an IoT based prototype of basic solar power trading platform for independent distributed nano-grids,” in 2020 Fourth International Conference on I-SMAC (IoT in Social, Mobile, Analytics and Cloud) (I-SMAC) (Palladam: IEEE).
Samy, S., Azab, M., and Rizk, M. (2021). T“owards a secured blockchain-based smart grid,” in 2021 IEEE 11th Annual Computing and Communication Workshop and Conference (CCWC) (Las Vegas, NV: IEEE).
Sanju, S., Sankaran, S., and Achuthan, K. (2018). “Energy comparison of blockchain platforms for internet of things,” in 2018 IEEE International Symposium on Smart Electronic Systems (iSES) (Formerly iNiS) (Hyderabad: IEEE).
Seven, S., Yao, G., Soran, A., Onen, A., and Muyeen, S. M. (2020). Peer-to-peer energy trading in virtual power plant based on blockchain smart contracts. IEEE Access 8, 175713–175726. doi: 10.1109/ACCESS.2020.3026180
Sharma, H. K., Kumar, A., Pant, S., and Ram, M. (2022). “Application of IoT in smart healthcare,” in Artificial Intelligence, Blockchain and IoT for Smart Healthcare (River Publishesr), 47–56.
Shrestha, A., Rana, L. B., Singh, A., Phuyal, S., Ghimire, A., Giri, R., et al. (2019). Assessment of electricity excess in an isolated hybrid energy system: a case study of a dangiwada village in rural nepal. Energy Procedia 160, 76–83. doi: 10.1016/j.egypro.2019.02.121
Siddiqui, M. S., Ali, T., Nadeem, A., and Nawaz, W. S S. (2020). BlockTrack-l: a lightweight blockchain-based provenance message tracking in IoT. Int. J. Adv. Comput. Sci. Appl. 11, 739. doi: 10.1201/9780429288739
Siddiqui, M. S., Rahman, A., and Nadeem, A. (2019). Secure data provenance in iot network using bloom filters. Procedia Comput. Sci. 163, 190–197. doi: 10.1016/j.procs.2019.12.100
SSE Energy Services. (2022). Smart Pay As You Go (Prepayment) meters. Available online at: https://sse.co.uk/smart-meters/pay-as-you-go
Syed, T. A., Alzahrani, A., Jan, S., Siddiqui, M. S., Nadeem, A., and Alghamdi, T. (2019). A comparative analysis of blockchain architecture and its applications: Problems and recommendations. IEEE Access 7, 176838–176869. doi: 10.1109/ACCESS.2019.2957660
Syed, T. A., Siddique, M. S., Nadeem, A., Alzahrani, A., Jan, S., and Khattak, M. A. K. (2020). A novel blockchain-based framework for vehicle life cycle tracking: an end-to-end solution. IEEE Access 8, 111042–111063. doi: 10.1109/ACCESS.2020.3002170
Teive, R., Guder, R., and Sebba, C. (2010). “Risk management in the energy trading activity-an approach by using multi objective genetic algorithm and multi criteria theory,” in 2010 IEEE/PES Transmission and Distribution Conference and Exposition: Latin America (T&D-LA) (São Paulo: IEEE), 504–510.
Thakur, S., and Breslin, J. G. (2020). “Cost analysis of blockchains-based peer to peer energy trade,” in 2020 IEEE International Conference on Environment and Electrical Engineering and 2020 IEEE Industrial and Commercial Power Systems Europe (EEEIC/I&CPS Europe) (Madrid: IEEE), 1–6.
Torres, V., Serral, E., Valderas, P., Pelechano, V., and Grefen, P. (2020). “Modeling of IoT devices in business processes: A systematic mapping study,” in 2020 IEEE 22nd Conference on Business Informatics (CBI) (Antwerp: IEEE).
Valle, F. D., and Oliver, M. (2020). Blockchain enablers for supply chains: How to boost implementation in industry. IEEE Access 8, 209699–209716. doi: 10.1109/ACCESS.2020.3038463
Verma, G., and Prakash, S. (2019). “A study towards current trends, issues and challenges in internet of things (iot) based system for intelligent energy management,” in 2019 4th International Conference on Information Systems and Computer Networks (ISCON) (Mathura: IEEE), 358–365.
Vishwakarma, L., and Das, D. (2020). “BSS: blockchain enabled security system for internet of things applications,” in 2020 IEEE 19th International Symposium on Network Computing and Applications (NCA) (Cambridge, MA: IEEE).
Von Wirth, T., Gislason, L., and Seidl, R. (2018). Distributed energy systems on a neighborhood scale: reviewing drivers of and barriers to social acceptance. Renew. Sustain. Energy Rev. 82, 2618–2628. doi: 10.1016/j.rser.2017.09.086
Wang, S., Wang, J., Wang, X., Qiu, T., Yuan, Y., Ouyang, L., et al. (2018). Blockchain-powered parallel healthcare systems based on the ACP approach. IEEE Trans. Comput. Soc. Syst. 5, 942–950. doi: 10.1109/TCSS.2018.2865526
Xu, S., Chen, X., and He, Y. (2021). EVchain: an anonymous blockchain-based system for charging-connected electric vehicles. Tsinghua Sci. Technol. 26, 845–856. doi: 10.26599/TST.2020.9010043
Yap, Y. H., Jinnie, T.an, W.-S., Ahmad, N. A., Wooi, C.-L., and Wu, Y.-K. (2020). “Motivational game-theory p2p energy trading: a case study in malaysia,” in 2020 2nd International Conference on Smart Power and Internet Energy Systems (SPIES) (Bangkok), 480–485.
Zafeiriou, I. (2020). “IoT and mobility in smart cities,” in 2020 3rd World Symposium on Communication Engineering (WSCE) (Thessaloniki: IEEE).
Zarour, M., Ansari, M. T. J., Alenezi, M., Sarkar, A. K., Faizan, M., Agrawal, A., et al. (2020). Evaluating the impact of blockchain models for secure and trustworthy electronic healthcare records. IEEE Access 8, 157959–157973. doi: 10.1109/ACCESS.2020.3019829
Zebra, E. I. C., van der Windt, H. J., Nhumaio, G., and Faaij, A. P. (2021). A review of hybrid renewable energy systems in mini-grids for off-grid electrification in developing countries. Renew. Sustain. Energy Rev. 144, 111036. doi: 10.1016/j.rser.2021.111036
Zhang, Y., Wang, Z., Deng, J., Gong, Z., Flood, I., and Wang, Y. (2021). Framework for a blockchain-based infrastructure project financing system. IEEE Access 9, 141555–141570. doi: 10.1109/ACCESS.2021.3119589
Zhu, H., Ouahada, K., and Abu-Mahfouz, A. M. (2022). Peer-to-peer energy trading in smart energy communities: a lyapunov-based energy control and trading system. IEEE Access 10, 42916–42932. doi: 10.1109/ACCESS.2022.3167828
Keywords: energy trading, blockchain and IoT, distributed energy management, peer-to-peer energy trading, blockchain technology (BCT)
Citation: Nadeem A (2022) A survey on peer-to-peer energy trading for local communities: Challenges, applications, and enabling technologies. Front. Comput. Sci. 4:1008504. doi: 10.3389/fcomp.2022.1008504
Received: 31 July 2022; Accepted: 05 September 2022;
Published: 27 September 2022.
Edited by:
Qiyue Li, Hefei University of Technology, ChinaReviewed by:
Lexi Xu, China United Network Communications Group, ChinaLei Liu, Xidian University, China
Copyright © 2022 Nadeem. This is an open-access article distributed under the terms of the Creative Commons Attribution License (CC BY). The use, distribution or reproduction in other forums is permitted, provided the original author(s) and the copyright owner(s) are credited and that the original publication in this journal is cited, in accordance with accepted academic practice. No use, distribution or reproduction is permitted which does not comply with these terms.
*Correspondence: Adnan Nadeem, adnan.nadeem@iu.edu.sa