Tinkering with social touch technology
- 1Human Media Interaction Group, Faculty of Electrical Engineering, Mathematics and Computer Science (EEMCS), University of Twente, Enschede, Netherlands
- 2Robotics and Mechatronics Group, Faculty of Electrical Engineering, Mathematics and Computer Science (EEMCS), University of Twente, Enschede, Netherlands
- 3Netherlands Organization for Applied Scientific Research TNO, Soesterberg, Netherlands
Social touch technology, haptic technology to mediate social touch interactions, potentially contributes to reducing negative effects of skin hunger and social isolation. This field is developing and while there are a number of prototypes, few became products and less persisted in the market today. Viable social touch technology is essential for research on social touch and it has an unexplored market potential. Making prototypes and evaluating them is the approach of generating knowledge in Research through Design (RtD). In RtD, researchers investigate the speculative future, probing on what the world could and should be, leaving the exact method of designing prototypes open. One possible method is tinkering, characterized by a playful and creative exploration. Tinkering environments, however, need a careful design of toolkits and setting. In this study, we report on the toolkit and setup we used for a tinkering-based teaching unit on social touch technology, held within an introductory course of an Interaction Technology master program, and describe the resulting prototypes. With a qualitative analysis of the results, we consider the teaching unit as a success, w.r.t. the diversity of the concepts developed. Tinkering is well-known as a playful method for education in Science, Technology, Engineering, and Maths, aiming at school children and high school students. It is not yet established as a design method in itself, and not considered as element of an academic skill set. Here, we argue that tinkering is a valuable design method in the context of social touch technology, and that it has a place in the design approaches within an academic setting. In a further step, we also want to include experts from other domains in the design process, such as psychologists or fashion designers. For that end, we suggest expanding a current toolkit for wearable technology with concepts from the teaching unit, more scaffolding tools, a variety of tactile actuators, and a software tool that allow for (re)configuring designs rather than programing them.
1. Introduction
1.1. Social touch in social touch technology
Social touch plays a key role in close social relationships and fulfills an important role in the regulation of physical and emotional wellbeing (Field, 2010). However, distance and social isolation create barriers for social touch, and, overall, a decline in social touch over the past two decades is signaled (Jewitt et al., 2021). Negative effects of touch deprivation can be partially mitigated by mediating social touch through technology, known as social touch technology or STT (van Erp and Toet, 2015). However, while there existed already a range of prototype applications (Huisman, 2017), only few concepts made it to commercially available products, where even less persisted. Two factors may play a role here. One is that the potential for innovative future applications and products is huge and not yet explored and understood. In this line, Jewitt et al. (2021) presents a call to action to designers, developers, and researchers to rethink and reimagine social touch through a deeper engagement with the social and sensory aspects of touch. The other factor that may contribute to the low persistence of products on the market may be found in low acceptance. Technology Acceptance Models (TAM) indicate that perceived usefulness and perceived ease of use are main reasons for the intention to use a product (Davis, 1989). Accordingly, these have to be addressed in the design of products for the market. Both factors will be addressed and discussed in this study.
Above observations motivated us to develop an innovative approach based on tinkering to design STT that increases the chances of end-user acceptance and market success. We report the development of the tinkering approach and its first results, and provide a toolkit with tinkering components and a set of “scaffolding” cards ready to be used in educational and other settings.
From the technological point of view, there are solutions such as (almost) real-time connection over internet, and small electronic building blocks allowing for wearability. Actuators for social touch, however, are still restricted; vibration motors are the most simple and accessible realization, but are noisy and give a restricted tactile sensation, that is different from a human touch in many ways. Other technologies such as servo motors, shape memory alloys, and pneumatic or hydraulic actuators can stimulate different sensory receptors, but come also with different drawbacks (e.g., they are bulky and noisy) that make them not yet suitable (for wearables) for mediated social touch. However, a number of initiatives and research lines are busy with the development of textile actuators or new developments in wearable pneumatic actuators that will be available in the near future (Maziz et al., 2017).
From an application point of view, STT is still coming short in the replication of a human touch. In Huisman (2017), the author states “Mediated social touch is less sensory rich than actual social touch, not just in terms of cutaneous and kinesthetic feedback, but also in terms of feedback from other modalities which may not necessarily be present in mediated social touch.”
At this point, we want to take the perspective that (a) human-to-human touch might be an inspiration for applications of STT, but technical applications of social touch may supersede human possibilities (imagine a full body hug with ten arms) and (b) that achievement of the positive effect on social needs is more important than a replication of a human touch. This leaves space for a wide range of out-of-the-box concepts.
1.2. Research through Design and tinkering
For novel applications, creative ideas are relevant, and, beyond these, also knowledge on how to address new characteristics introduced by social touch over a distance, such as inherent asymmetry in comparison to a direct human-to-human touch, the non-reciprocity (in a physical touch, the toucher also feels the touch s/he gives), ambiguous attribution (who is actually the initiator of a touch if there is, e.g., computation involved) (Huisman, 2017), complex agency (e.g., is the vest touching me or the person who gave me the vest?), or intrusiveness (a touch might be unexpected and not fitting a situation). In the context of STT, Research through Design (RtD) could be a suitable approach to gain this knowledge. “RtD asks researchers to investigate the speculative future, probing on what the world could and should be” (Zimmerman and Forlizzi, 2014). It builds on generating knowledge by introducing prototypes into the world, and reflect, measure, discuss, and analyze the effect, sometimes the coming-into-being, of these artifacts (Stappers and Giaccardi, 2017). According to Gaver (2012), making is part of the paradigms of RtD, i.e., most of us agree that the practice of making is a route to discovery, and that the synthetic nature of design allows for richer and more situated understandings than those produced through more analytic means. While in this, approach design is driving part of research, RtD does not define how to generate and design new prototypes. It is method agnostic (Stappers and Giaccardi, 2017), in the sense that the method of designing prototypes can be filled in various ways.
In this study, we suggest that tinkering is a suitable design approach for out-of-the box prototypes in the context of STT. Tinkering is characterized by a playful way of working, stimulating creativity, where goals are self chosen, evaluated, and re-defined in an iterative process. In tinkering, the design space is explored in a hands-on manner, inviting to get into a conversation with the material (Schön, 1992), experience its possibilities and restrictions, and getting inspiration from these. Tinkering is a process having trial-and-error at its core (Martinez and Stager, 2013), where trial-and-error should not be understood as randomness, but more as a way to explore and experience material properties. Depending on the background of the tinkerer, this exploration can be very systematic (as we have seen in tinkerers with an art school education), but are always guided by curiosity and background (including professional background). Following this process, tinkerers are open to serendipity, observation of the unexpected. There is no expectation that others following the same process would produce the same or even a similar final artifact (Zimmerman and Forlizzi, 2014). Especially in the context of haptics, experiencing the material is also a key factor in the exploration. Insights that need to be experienced are, for example, a stroke by a series of vibration motors is very different from a stroke by a hand, feeling one's own heart beat via a vibration motor is awkward, and the noise of a (vibration) motor can spoil the touch experience, but a vibration motor still can generate a pleasant feeling of massage on a shoulder. There is an endless list on the qualities of touch experiences, and contexts in which they are perceived differently, that need a hands-on approach for evaluation. The hands-on approach shows limitations of the material, but at the same time also shows new possibilities and stimulates creativity.
Most tinkering approaches in teaching aim children in primary schools or high school students, as in-school activities as well as out-school activities, and the approach is especially popular for Science, Technology, Engineering, and Mathematics (STEM) education (Vossoughi and Bevan, 2014). However, as the authors elaborated in Quinn and Bell (2013) and Mader and Dertien (2016), tinkering can also be a valuable contribution in academic teaching. At our university, we are applying tinkering-based teaching approaches in several courses of our bachelor program on Creative Technology as well as in a master program on Interaction Technology. In both, it has demonstrated its value for creating innovative concepts and prototypes.
1.3. Tinkering with social touch technology
We expect that the need for innovative applications of STT makes tinkering a promising approach that stimulates the creation of prototypes with novel concepts. Especially for touch, it is important to create tangible prototypes; it is inherent to this sensory modality. User evaluation of these prototypes drive insights in the RtD approach (Zimmerman and Forlizzi, 2014), and has the potential to increase the body of knowledge on STT, and further on also to improve the maturity of concepts.
According to these expectations, we come to the following research question:
Is tinkering a design approach that can foster the finding of novel applications in STT?
In this study, we will answer this research question based on the results of a tinkering-based teaching unit on STT in an Interaction Technology master program.
Tinkering activities need the design of a setup, i.e., material, scaffolding, etc. We will describe the components of such a setup in general, and specifically for the teaching unit considered. The analysis of the students' results will provide an answer to our research question.
Students of Interaction Technology have technical knowledge beyond what a “general public” would have, which allowed them to manage the technical challenges of the tinkering task. However, we expect it to be valuable if multidisciplinary teams, with no specific technical background, could also tinker with touch technology. Therefore, as a step further, we would like to enable experts of other domains to explore the possibilities of STT, such as psychologists, fashion and interaction designers, or physiotherapists. They can bring in different perspectives of user needs and, accordingly, contribute to a better perception of usefulness and technology acceptance. To this end, we suggest further steps in the design of a toolbox for STT, building on an existing toolbox for tinkering with wearable technology.
1.4. Outline of this study
In Section 2, we report on a tinkering-based teaching unit on STT in an Interaction Technology master program. It builds on a generic framework for tinkering sessions, that is elaborated conceptually, and on the design of its key elements. Concept, material, and results of this teaching unit for two cohorts of students will be presented. We will discuss how the results of the students answer our research question. Section 3 is about the additional design steps needed for the toolkit to make it accessible for non-technologist. We describe material developed earlier for tinkering with wearable technology, and suggest extensions allowing broader groups of stakeholders to tinkering with STT. A discussion is contained in Section 4, and a conclusion can be found in Section 5.
2. Tinkering for social mediated touch
In this section, we report on a tinkering-based teaching unit on social touch technology, and the evaluation of the results from that teaching unit.
To this end, we introduce the basic concepts of tinkering, and the relevant aspects that need to be designed for tinkering activities. We further describe how these concepts were instantiated in a teaching unit. The tinkering-based teaching unit on remote social touch was part of an introductory course “Foundations of Interaction Technology”. In total, 34 teams, each consisting of two students, followed a 2-week haptics challenge. This course was given in the academic year 2020/2021 two times, where more students participated in the first round. The introductory course is part of the master program “Interaction Technology”. Students participating come from different bachelor programs, such a Creative Technology (an ICT-based design program), Computer Science, Industrial Design, Mechanical Engineering, Electrical Engineering, Artificial Intelligence, and also from Psychology and other bachelor programs.
The abstract schema of our research approach is illustrated in Figure 1 and will be detailed later.
In our context, we understand tinkering as both a method and a mindset that can be applied in design and teaching, stimulating creativity, stressing ownership, and motivation.
Resnick and Rosenbaum (2013) give the following definition: The tinkering approach is characterized by a playful, experimental, iterative style of engagement, in which makers are continually reassessing their goals, exploring new paths, and imagining new possibilities.
Adding onto this definition (Mader and Dertien, 2016), tinkerers follow a process in which problems and challenges are self-defined and re-defined during the process. Depending on the background, the process may initially be seemingly undirected, or start with a systematic exploration of the material (as we have observed, e.g., for people with an education in art). Tinkering is driven by curiosity and playfulness. It is characterized by an iterative way of experimenting and prototyping, where observation and reflection lead to defining a new challenge. Failing is embraced as a vehicle for insight and progress (Ryoo and Kekelis, 2018). Many people in our culture have early tinkering experiences with playing Lego™, where often no goal was set in the beginning, and constructions were explored by experimenting.
The concept of tinkering as used was initially formulated by the Do It Yourself (DIY) and Maker Movement, and tinkering is often mentioned as an approach used in Maker Spaces. In the literature, the distinction between making and tinkering is often not sharp (Vossoughi and Bevan, 2014) and concepts gets mixed. A useful definition is provided by Martinez and Stager (2013):
• Making is about the active role construction plays in learning. The maker has a product in mind when working with tools and materials.
• Tinkering is a mindset, a playful way to approach and solve problems through direct experience, experimentation, and discovery.
Many making and tinkering activities aim school children, out-of-school or in-school activities (Martinez and Stager, 2013; Petrich et al., 2013; Vossoughi and Bevan, 2014). According to Vossoughi and Bevan (2014), its invitational potential may be a part of what is driving so much interest in making as an important innovation in teaching and learning practice, particularly after the predominance of text-based, test-driven, teacher-centered STEM instruction. Tinkering-based STEM activities are designed for different target groups, ranging from children in primary schools to high school students (Martinez and Stager, 2013; Petrich et al., 2013; Resnick and Rosenbaum, 2013; Vossoughi and Bevan, 2014). Learning goals of tinkering activities are broad, where often a range of process-related capabilities and higher order thinking skills are considered as relevant (Exploratorium, 2017).
In teaching, at our university, we have been applying tinkering approaches in several bachelor and master courses for a number of years. We argue (Quinn and Bell, 2013; Mader and Dertien, 2016) that tinkering can also contribute to teaching in an academic setting, as elements of scientific activities, such as observation, reflection, hypothesis forming, and experimentation, are also activities within a tinkering process. In addition to these generic tasks in science, learning goals in our programs also include design skills, i.e., that students can come up with novel concepts, realize them in working prototypes, and evaluate their concepts.
The main point is that even if the participants self-direct their process, a carefully chosen setting is relevant for the success of the approach. We identified a number of key ingredients (Resnick and Rosenbaum, 2013; Mader and Dertien, 2016) that constitute a setting for enabling participants to tinker, that we call a “tinkering playground”.
Since the tinkering playground should contain everything regarding (or designed for) a tinkering activity, we also refer to the tinkering playground as the “whole”: the combination of seed, toolbox, discovery (goal), and facilitation—bound to a location in time and space. In the following, we describe the elements of a playground, and how we implemented these for the haptics challenge.
2.1. Space, time, and basic equipment
In Resnick and Rosenbaum (2013), the authors state that “designing contexts for tinkerability is as important as designing kits for tinkerability.” Tinkering takes place in a certain environment. Ideally, it should be a stimulating place, inviting to be creative, providing the space necessary. This includes the space for experiments, and also the space for interaction with at least the facilitator, or other tinkerers. It is bound by time, has a start moment, and a defined end.
Due to COVID-19 regulations, the haptics teaching unit was given online, and the students worked from home, i.e., most students in a team also worked in remote places. For regular education activities, our university provides a stimulating learning and prototyping environment in the DesignLab, that includes open space available, with tables, whiteboards, basic materials like paper and post-its, a mechanical and an electrical workshop, sewing machines, 3D-printers, and laser cutters, inspired by FabLab or MakerSpace concepts.
2.2. Toolbox
In general, a toolbox contains different sorts of building blocks. In the first place, these may be physical building blocks (paper, clay, and electronics), but also can be virtual (software and algorithms) or concepts (social media and serious games). Moreover, a toolbox always also contains what a person brings in, skills and knowledge or templates gained by experience. The toolbox, therefore, does not only contain what is provided but also the background of a tinkerer, and is therefore also a very personal and growing set. Mature tinkerers can extend their toolset independently, and finally, take the whole world as a source. Higher level knowledge can certainly be part of a (personal) toolbox of a tinkerer, and can range from, e.g., knowledge in the electrical domain like resistance of wires to existing examples or insights on the context in the domain of interest, as well as scientific results in an academic context.
For a specific tinkering activity, a toolbox tailored for the aims of the activity is required. The choice for a good toolbox is not only about an adequate selection of material. Rather, some material needs to be designed to be accessible in a tinkering workshop for a specific target group, taking the area of interest into account. In Resnick and Rosenbaum (2013), identified immediate feedback, fluid experimentation, and open exploration as key principles guiding the design of toolboxes (kits). Fluid experimentation, according to the authors, includes the ease of getting started and connecting objects.
For ease of connection, we consider affordance of the toolbox objects as of one of the crucial criteria. In short, affordance describes the property of an object to self-explain its usage (Norman, 2002): a button invites to push it, Lego™ does not need much explanation how to stick blocks together. In a tinkering session, it should be easy for the participants to find out how to use the building blocks. A card set with explanations for each toolbox element can be one useful option here.
For the ease of getting started, a toolbox needs to be introduced. Scaffolding exercises, like small step-by-step instructions, can help participants to start using some of the tools or techniques. For example, needle and thread, hot-melt and cardboard, soldering iron and connecting wires, wiring up sensors to an Arduino board, and starting up and using a certain programing environment.
In general, the elements of a toolbox have to be designed for the target users (learners or designers) in a way, that they can focus on a general theme of the tinkering playground, and do not need to spend a major part of the time on getting the supporting material to work. Participants without programing experience cannot be expected to learn to program an Arduino as part of a tinkering session. A dedicated graphical interface allowing to select, position, and connect components to a configuration that then generates Arduino code would be an example for such a design effort to take. As another example, one of the authors designed through a number of editions of a tinkering workshop on inflatables, a paper-cup construction with a powerful copter motor and fan as the easy-to-use core technology for inflation (Neidlinger and Dertien, 2015; Dertien and Neidlinger, 2016).
For the haptics challenge, the tinkering part addressed a conceptual level and a material level. Accordingly, the toolbox also contained these two levels. The conceptual part of the toolbox was covered with an introductory overview presentation on haptics and social touch. These included the sensory system, the role and relevance of touch, the effect of touch in different contexts, examples for touch technology, and more. For the material part of the toolbox, students received a list of hardware components to buy (usually, we provide boxes of material, due to remote teaching in this year, students needed to get the components themselves). For sensing touch, two sensors were chosen, a bend sensor (60 mm) and touch sensor (capacitative sensors can be constructed using aluminum foil and wires). Vibration motors (3 × vibration DC motor module) as actuators were added to the toolbox. For the control, an Arduino nano v3.0 microcontroller was selected. In addition, cables, a breadboard and prototyping wires, resistors (4.7kΩ, 3 × 1MΩ), and tape were required. Additionally, students could make use of whatever material they had available, especially a laptop or PC with internet connection, textile, stuffed animals, garment, or other. Concerning software, the programing environments of Processing and Arduino including a WebSocket library for the internet connection (standard available in Processing) had to be downloaded and installed. Instructions for these components, actions, program fragments for the respective sensors, and references to websites with more detailed explanations and instruction how to use the material were given. The students were composed in teams, such that in each team, knowledge on Arduino programing and prototyping was present. Specific design of the toolbox components was not required here.
2.3. Seed
A tinkering process needs a starting point, a “prompt” or “trigger”. This point should be a stimulant to enter a self-directed process. There are various possibilities for a seed: beginners, not experienced in tinkering, may need a small example project to perform, with the idea that they understand the possibilities of the toolbox and can proceed with an own idea. An alternative for a seed is to give a first goal to achieve, which is less defined than an example project. There, the process and specific building blocks needed to get there, are still open. For more experienced tinkerers, simply a new material or interesting new piece of technology could work as a seed. Also, on a conceptual level, a theme might also be seed to start a process. The seed needs some introduction, e.g., a step-by-step group exercise, or an easy to follow description how to set up an example project.
For the haptics challenge, on the conceptual level, the seed is basically the theme of the challenge, remote social touch. On the material level, an example project of a remote handshake was provided as a seed, building on the toolbox given. This example project consisted of building instructions and code.
2.4. Process
The overall process includes introduction of the topic and mindset, the setting, the toolbox, and possibly also the seed. Times for feedback or breaks may be included, or starting moments for new iterations. The timing of the process is also a relevant design step for a tinkering session. Altogether, the start-up exercises should take only a small fragment of the overall time available. The core part of a tinkering process begins with the seed. The process is driven by curiosity, creativity, and serendipity as guiding principles. It needs observation and reflection to learn from experiments and early discoveries, to extend and improve for the next discovery. It is ideally an iterative process with a series of discoveries and starting over again. The process depends very much on the maturity and experience of the tinkerer, and it also forms the core of the tinkering mindset. Many of tinkering activities for children span only over a block for a few hours, but, especially in an academic teaching context, can also be spread over a longer period.
The teaching unit on haptics took 2 weeks overall. Students had approximately 26 h available for the teaching unit, of which 8 h were scheduled and 18 h for their individual tinkering process.
• To provide the conceptual basis as toolbox ingredients, two presentations on social touch and haptics were given, as mentioned above.
• The tinkering period started with an introductory lecture on the setting and procedure.
• As preparation to the introductory lecture, students were asked to get a networking example running, to receive help during the lecture in case it would not work.
• During the introduction, students were asked to set up the example project of a remote handshake based on the networking example, using the bend sensor and a vibration motor. In the session, they could get help with the example project.
• After the introduction session, students could tinker in a first iteration with the material and brainstorm on an application.
• In a second session, students pitched their concepts and received feedback from peers and lecturers.
• Afterward, students started with a second iteration, and realized that in a prototype.
• A teaching assistant was available for a technical help session.
• Finally, the results were presented.
2.5. Facilitator
The facilitators have many tasks. They introduce the toolbox and a seed, or help to identify a seed. They are guarding the mindset, stimulate reflection, give feedback, and give support in scaffolding. They are setting the mood, creating an atmosphere where it is not only ok to fail but faults are considered as stepping stones (Ryoo and Kekelis, 2018). They set the threshold low, the ceiling high, and provide wide walls (Resnick et al., 2018), manage the pace, help to get unstuck (Petrich et al., 2013), and keep the flow.
For the teaching unit on haptics, two of the authors provided the introductory presentations on the conceptual level, followed by the introduction to the tinkering-based haptics challenge. One of them was also main responsible for the teaching unit, and was available for questions of the students during the whole period. For the technical support, a teaching assistant was in charge. Chairing the presentations with feedback and peer feedback was shared between members of the research group.
At this point, the abstract methodology shown in Figure 1 can be detailed as shown in Figure 2.
2.6. Discovery
A crucial aspect of a tinkering process is that the tinkerer decides on her or his own goals. The discovery is self-defined; it might be a new product or prototype, where it is sufficient, if it is new for the tinkerer. It might also be a new or better way of doing things, or a new concept. The self-definition of where it goes makes the tinkerer real owner of the result and the path. The ingredients of the toolbox mentioned above can stimulate in the definition of result and path, but it is also the personal background and experience resulting in story telling that forms a goal for the tinkering efforts. A tinkerer might get help with stimulation, reflection, feedback, or getting unstuck when necessary.
The following descriptions of the discoveries in the haptics challenge are the first part of the evaluation as illustrated in Figure 2.
We first give a few specific examples from the students' results, before analyzing the overall results.
The first example is a ball game. The game starts and ends with a fist bump of the two players, realized with a vibration motor, bend-sensor on the hand, and an accelerometer. One player virtually possesses a ball, and can throw it physically, where the accelerometer would measure the throwing speed. Through a visual, the other player can see the ball approaching, and also feel it through increasing intensity as the virtual ball is “approaching”. When the ball has reached his position, he can catch it, also initializing a vibration at the side of the thrower. Figure 3 shows the visualizations that both players can see while playing the game. The setup and prototype can be seen in Figure 4.

Figure 3. Visualization of the ball game for the remote players. Fist bump left; ball approaching in the middle; ball caught on the right.
The next example is about a remote social touch for a family member who has to undergo diagnosis in an MRI scanner. A patient is there lying in a tube and may feel lonely and scared. The social touch is meant to calm and comfort the patient, and realized by a pair of sleeves. The patient can indicate that she wants to receive a touch by bending her fingers. The remote family member feels this wish by a vibration, and then can give either a soft or strong touch by touching capacitative sensors on his sleeve with a fingertip or with the full hand. The patient would receive a stroking touch through three vibration motors on her sleeve with a fixed stroking illusion pattern, vibrating either soft or strong. Figure 5 shows the setup of the project and the prototype realization.
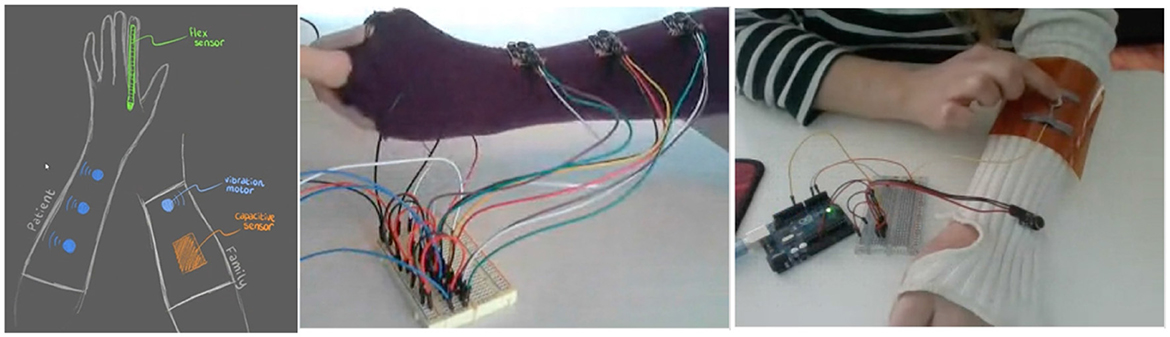
Figure 5. Left sketch of the setup and right prototypes for a comforting touch of a family member during an MRI scan.
In the following example illustrated in Figure 6, the students want to enrich texting on a smartphone by touch and physical elements. For that purpose, they extended existing bunny phone cases with electronics. When pressing the left or right back side of one smartphone, the ears at the other smartphone would move. Also the tail would wiggle and a vibration motor would send a touch.
In the fourth example, the student referred to her childhood. When she was stressed her mother would put her hand on the child's forehead, to comfort her. As the student now lives in a different country, the prototype suggested should realize this gesture on distance. Figure 7 shows the concept. The electronic setup here is very simple, consisting of a bend sensor and vibration motor on each side, integrated in an animal-shaped wash cloth for each side. Technically, the example does not go much beyond the warming up example the students did in the course, but it has a strong story telling part.
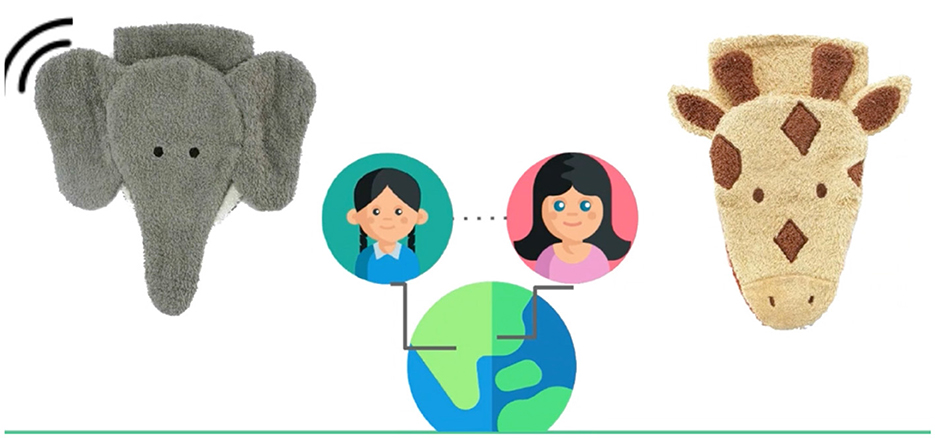
Figure 7. The touch device should allow the mother to give her daughter a comforting touch on the forehead as she has done physically in the past.
The last example is a remote version of the rock-scissors-paper game. As illustrated in Figure 8, the three gestures for a rock, scissors, or paper, respectively, and also for a fist, are detected by a number of bend sensors and capacitative sensors. The winner of a game receives a vibration touch as reward.
For the haptics challenge, the students had, as mentioned above, some general lectures of social touch, and for technology scaffolding, a “hello-world-example” connecting a bend-sensor on one side to a vibration motor on the other side, connected via internet. The categories used to characterize the projects are identified a posteriori, to understand and describe the breadth of projects. The categories were not presented to the students beforehand. The article of Ipakchian Askari et al. (2022) provides an excellent taxonomy of applications in STT. This taxonomy would have been an alternative to analyze the students' projects. Unfortunately, at the time when the characterization was done, the article was not yet published. However, we assume that the main conclusion from the analysis would be similar.
The students' discoveries included a context and interaction for mediated social touch and a prototype. The social contexts students addressed were people in a relationship, grandparents and grandchildren, relatives, (study) friends, or colleagues, children in a hospital and their parents, or a family member in a radio treatment in a hospital.
The social interactions considered were basically of two different categories:
• Exchanging or giving touch as main activity in itself. The touches should represent stroking, caressing, hugging, tickling, tapping on the shoulder, a handshake, or a high-five (28 teams of 34). These touches had the intended meaning to confirm and intensify the relationship, and/or to comfort or de-stress the receiver.
• Augmentation of another shared activity, a ball game, rock-paper-scissors, texting on a smartphone, watching a movie, doing workout, and relaxation movements. These touches had two intended functions, intensify the experience of the activity, and create a feeling of connectedness (7 teams of 34).
The objects they made were:
• Symmetric devices, such as stuffed animals, cushions, squeeze balls, smartphone extensions, or gloves, where symmetry describes that both devices are functionally identical, and a touch can be sent either way.
• Asymmetric devices such as a glove or other touch device connected to a different device, such as a glove, sleeve, facial mask, shoulder wrap or sweater, where asymmetric means that a touch can be sent only one directional, so there is a dedicated sender and receiver of the touch.
2.7. Observations and further evaluation
2.7.1. Story telling
For almost all students, the starting point of their prototype was a story and context (32 out of 34). As the challenges took place during the COVID-19 pandemic, most students took the need for touch due to the contact restriction in this phase as main motivation (24 of 34). Four teams had a combination of other and COVID-19 related contexts, such as in the ball game illustrated in Figure 4 which is also suitable for grandparents who cannot run to play with a grandchild. Four teams had a motivation not connected to the pandemic, such as the example of a family member in an MRI scanner from Figure 5.
2.7.2. Technical level required
Technically, all teams could cope with the technology of the toolbox and the instructions given. In all teams, experience with Arduinos or other microcontrollers was present. All had more or less working prototypes, several with common problems with bad electric connections or poor internet connection. Some prototypes were more elaborate, depending on extra skills of the students concerning sewing, 3D printing or internet technology, and physical computing, such as the examples of the smartphone case in Figure 6 or the ball game in Figure 4. Most used the internet connection provided (31 of 34), and some teams (3 out of 34) used WIFI or MQTT as a data transmission protocol.
Concerning the technology, most teams used the components for the toolbox given [i.e., capacitative sensors (16 of 34), bend sensors (24 of 34), vibration motors (34 of 34)], several students used LED lights (7 of 34), and only a few used other technical elements not contained in the toolbox, such as stronger servo motors (1 of 34), piezo speaker (2 of 34), or a resistor network for temperature (1 of 34). Our conclusion here is, that, even if the number of components of the toolbox was very limited, it allowed for a variety of prototypes and concepts. The toolbox, therefore, met the requirement of wide walls allowing for a wide range of different types of projects (Resnick et al., 2018). An explanation here might be, that working on a conceptual level, vibration motors were considered as a placeholder for touch technology that generates different stimuli.
2.7.3. Variety of the results
The example students were given for the technical setup test was a simple handshake (with a bend sensor and vibration motor). In the examples of STT presented in the course, most were related to hugs, and, of course, students had access to publications on social touch, especially the overview article of Huisman (2017). Out of the 34 teams, 9 teams chose for stuffed animals (or similar, like a cushion) and 7 teams had an application including a glove, which was definitely in the range of what was expected. The range of versions of social touch that was addressed, such as stroking, caressing, hugging, tickling, tapping on the shoulder, or high-five, was beyond what was presented in the course for STT, but could have been found in the literature also. A huge variety was in story telling perspective of the projects, ranging from applications for lovers, children and parents, grandparents, relatives, or just friends. Definitely beyond expectation, were applications, where social touch was not used as the primary goal of the exchange, but was added to another form of social interaction to intensify the experience of the activity on one hand, and also create a feeling of connectedness on the other hand. The applications here were diverse, like a ball game, rock-paper-scissors, making music together, texting on a smartphone, watching a movie, doing workout, and relaxation movements, as mentioned above. It is difficult to define a metric for diversity in storytelling. The abstract properties that can be counted still reflect aspects of diversity, but cannot capture the creativity of the concepts.
A few teams also addressed problems on a conceptual level of STT and, e.g., considered different protocols for consensus of receiving touch (7 out of 34), how to initiate and agree on touches over distance, addressing problems of synchronicity, and intrusiveness of remote touch technology. In the case of synchronicity, one problem that was identified was that the sender could not “see” if the receiver was indeed receiving the touch, and, therefore added an option to store a touch, such that the receiver could get the touch later when (s)he would be available. Other students implemented a step of consensus to their protocol, which addresses synchronicity (the receiver is indeed available) and intrusiveness (the receiver is ready to receive a touch). The students did not use definitions from literature of “intrusiveness” and “synchronicity”, but did describe the problems behind.
On top of this, one team added also vibration feedback for reciprocity. The sender could feel when the receiver got the touch. The teams that had primarily another shared activity, which was augmented or intensified by touch, and had all protocols for conditions when to trigger the touch.
Our conclusion based on these results, are two-fold, on one side concerning the teaching perspective, and on the other side concerning the research question of this study.
For the teaching perspective, the question is whether the setup of the course and material led to the intended outcomes. The intended outcomes include a design aspect and an academic (reflection) aspect. On the design part, the main criterium is the diversity of the solutions. Although there were 9 out of 34 prototypes including stuffed animals, and 9 with gloves, the overall diversity is broad, even more than expected, and we can conclude that the choices taken for the setup and toolbox were appropriate. This conclusion follows the statement of Resnick: if we see a great diversity of projects, to us that's a sign of success. Additionally, for the academic part, results from the course were promising. Several teams (7 out of 34), in the discussion of their prototypes were able to identify concepts inherent to touch technology (i.e., synchronicity and intrusiveness) that were not addressed in the course, in a relatively short teaching unit.
At this point, we want to answer the initial research question: Is tinkering a design approach that can foster the finding of novel applications in social touch technology?
The same arguments as above, the diversity of projects, and the generation of project beyond the expected, support a positive answer to our research question.
We also see support for our initial suggestion that tinkering might be a suitable design approach in the context of Research through Design (RtD). The perspective of RtD is that the creation of prototypes is a stepping stone to understanding the conceptual complexity of an application domain. As mentioned above, through designing and realizing a prototype, some of the students were able to identify inherent problems (and possible solutions) of mediated social touch.
3. Accessibility of tinkering for touch technology
The target group of the tinkering challenge in the previous section were university students, where most of them had a knowledge base in technology. As the challenge was set up, experience with sensors, actuators, and microcontrollers was present in each student team. The design effort required to make a toolbox accessible for the target group of tinkerers was very low for the student group, enabling them to create prototypes. Still a few students reported that this caused an imbalance in effort.
In a further step, we would like to open up the approach of tinkering also for different target groups, who probably have more or different knowledge on the conceptual level and less on the technical level. Supporting multidisciplinary design efforts, we would like to include psychologists, fashion and interaction designers, haptologists, physiotherapists, or other stakeholders in a tinkering process on applications in haptics. On a conceptual level, experts from other domains can bring in different expertise and other perspectives. They can contribute to a broader exploration of the design space, and come to richer or more meaningful set of prototypes. Additionally, their reflections also build on different expertise and perspectives. Altogether, knowledge derived by a multidisciplinary team has the chance to be more varied. In this context, we especially consider the hands-on aspects of tinkering as design activity as valuable. Active exploration with direct feedback gives more insight on the possibilities and the restrictions of the (technological) material available. On one hand, this can stimulate a creative process, and on the other hand, it connects concepts invented better to the state-of-the-art technology available.
In this section, we suggest a design of a tinkering playground on the next level, allowing broader groups of tinkerers, and requiring less technical knowledge to come to a working prototype. Following Stappers and Giaccardi (2017), this is a design for design effort. Starting point for our suggestions is a setup and concept for a tinkering playground developed for wearable technology. The toolkit was already designed for a target group of laymen. Experiences with it showed that the concept is suitable for people without technical experience. This setup will serve as basis for a social touch technology tinkering kit, as we consider the latter also in the wearables domain. In the following, we first will describe the ingredients of the wearable technology playground. In the second part, we will suggest extensions of this playground that supports a greater variety of prototypes for touch technology.
3.1. Tinkering with wearable technology
There can be different design goals for a wearable toolbox. For example, for the Lilypad family of components,1 easy integration in garments and textiles was certainly a design goal. There, sensors, controllers, and actuators come mounted on printed circuit boards in round shapes with connection points that can easily be sewed by hand using conductive thread. Using these components supports already a high level of wearability of the garment created. Another example is the Wearable Bits (Jones et al., 2020b), that use felt-mounted sensors and actuators that can be connected using snaps. Integration in or to garments may even be easier there, as no sewing is required and connecting sensors and actuators is simple. How the integration and programing of a controller is solved, is, however, not clear. Another focus is set by Hamdan et al. (2018), where the realization of circuits is supported by embroidery technology. Also here, the textile properties are in the center of attention, more than a toolbox approach. In general, a toolbox for tinkering with wearable technology has to tackle one or more aspects of a multidisciplinary problem space, including fashion, design, electronics, electronics integration, and programing.
In past workshops, we had experienced that tinkering with wearable technology often suffers from a high threshold concerning technology skills. As a consequence, often the workshops did not go much further than a starting exercise of sewing led lights on garments or bags, or following some readymade recipes. It is not uncommon to get stuck with electronic connectivity tasks or programing. Our approach for a wearable technology toolbox emphasizes the exploration of novel concepts. Accordingly, our design focus was the support of easy prototyping. Here, “easy” refers to plug and play technology, where connecting components in a prototype is straightforward, and the programing effort reduced to a minimum. Core of the design is a microcontroller interface that allows to plug in maximal three sensors and three actuators in a uniform way. In addition to the components, tools, and materials themselves, we also provided a set of cards corresponding to these materials, as aid in exploring the toolbox, instruction, planning of a design, and as reference. The wearability aspect was kept simple and solved by a range of materials such as velcro and elastics, allowing to fix a setup on the body.
In November 2019, we organized two workshops with the aim to design novel concepts of wearable technology for a “general” public. This small series of events was organized by the local Media Lab and attracted a diverse audience of students in media/arts, designers, artists, or pensioners interested in arts and crafts. Due to COVID-19, not more editions of this workshop could be performed.
3.1.1. Toolbox
The wearable toolbox consists of sensors, actuators, control, and connectivity. Table 1 contains a list of the components, and Figure 9 shows the toolbox elements with the respective cards. During the workshop, a set of tools and materials/supplies are provided which is also shown on the cards in Figure 10.
The design effort for the toolbox components consisted of the following: Where needed (for example, with the microcontroller and the bend sensor), 3D printed housing was designed to facilitate mounting and protect the electronics for reduced risk of short circuit. The vibration motors come with fragile connection so they have been fortified with a flexible compound (Sugru). The specific microcontoller (Arduino Beetle) is very small, it has the dimensions of a regular USB stick. It has been modified to connect three sensors and three actuators, all using the same 3-wire leads. Resistors and transistors required for the connection of the sensors and actuators were already integrated in the leads. The USB plug of the microcontroller (see Figure 11) can be connected directly into a laptop for programing, or directly into the powerbank for standalone operation, reducing wiring hassle. The leads of sensors and actuators are about 30 cm long and can be extended, such that they can easily be positioned anywhere on the body. The materials that have been provided to make “garments” or, at least, allow the components to be worn have been selected to build low-fi proof of concept designs rather than high-fi fashionable prototypes.
3.1.2. Seed
As process/conversation starters, a series of imaginary designs were provided on paper, consisting of a short description and the specific components (as cards) needed to create them. There is a careful balance to maintain with providing these examples. The example has to be clear, and just interesting enough. It should trigger participants to improve upon rather than to replicate. The starter themes were the following, where the components needed are listed on paper, and the corresponding cards explaining the projects are shown in Figure 12.
• Party: At a party, let your garment blink in the rhythm of the music or your heart (With microphone or heart rate sensor and led strip).
• Posture Coach: Give a sign (vibration or sound) when slouching for too long (With bend sensor and vibration motor or buzzer).
• Tail: When you are happy or frightened, wag your tail or set up spikes (With skin resistance sensor and servo motor).
• Traffic: On your bike, give the direction with a blinking led strip on your back by touch sensors (With led strip and touch sensor).
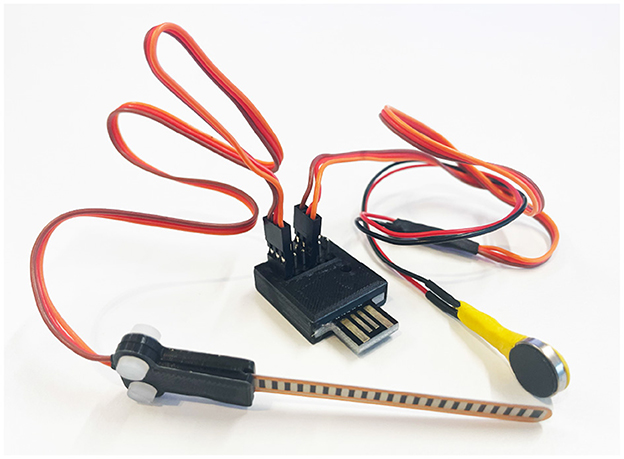
Figure 12. Simple setup for posture-warning device: microcontroller (plugs into a power bank directly), cabling, vibration motor, and bend sensor.
3.1.3. Process
At the start of the workshop, specific instructions were given to the participants aimed at exploring the toolbox and understanding the available materials. In detail, the workshops followed the steps below:
1. A general introduction on wearable technology.
2. Explanation of the sense-think-act paradigm—whereas this paradigm is at the basis of our work, it turned out that it is not self-evident for a general public. The sense-think-act paradigm describes a cyclical process, where the sense-part consists of the collection of all the sensor values by a controller. The think-part refers to how the controller processes the input values. For the application considered here, much of the processing is in a style of if value is above a specified threshold switch on this actuator for this duration. The act-part of the sense-think-act paradigm then includes to the activation or deactivation of the actuators involved.
3. A short introduction to the ingredients of the toolbox;
4. Simple card-sorting exercise (find the part belonging to the card, do you understand what it does?);
5. The assignment for the participants to choose one of the predefined assignments and performing it themselves, if wanted;
6. The assignment to think of a concept and realizing that;
7. Facilitation, stimulation, and answering questions;
8. Configuration of the controller program according to the concept of the participant.
3.1.4. Observations
The card-sorting exercise proved to be sufficient, but also necessary. The provided starter cards with example projects were a good pointer for participants that were immediately stuck. Other participants dived in right away and paid these example designs no heed. This coincides with our observations on different learning styles of (design) students. The two workshops gave indication, that the setup does work. Several participants stuck to the predefined examples, where their result would be the understanding of the concept. Some participants went further and had creative ideas they could realize comparably fast. One example was for cyclists at night, a light on the arm that starts blinking when the arm is raised, realized by a flex sensor and a LED strip. Another example was to prevent falling asleep while watching a film, where the head falling to the side would raise an alarm, realized by an accelerometer on a head-band and a vibration motor. programing was still a bottleneck, where the facilitators had to take over the programing. As the program schema given required more a configuration task than bottom-up programing, the effort was not very high. Still, it would be desirable to have a graphical programing interface that allows the participants themselves to generate code from building blocks that can be selected, connected, and equipped with conditions.
Altogether, we conclude that the workshops showed that participants without a background in electronics can use the components of the wearable toolbox to create prototypes for new, self-defined concepts.
3.2. A toolbox for tinkering with touch technology
As a next step, we suggest the design of a toolbox for tinkering with touch technology. There are not many existing toolboxes that focus on the quality of touch. Some focus on connectivity such as the toolbox kit by hapticlabs.io (Müller, 2020), or focus on a specific technology such as pneumatics by flowIo (Shtarbanov, 2021), or offer textile integration options such as the Swatch-bits (Jones et al., 2020a), but options for users to explore and link the quality of touch to conceptual development is largely lacking.
Based on the positive experience with the wearable toolbox, we would also emphasize here a focus on easy prototyping and integration of electronic components, and also the explanatory material in the form of card sets and example projects, as well as the process. For the multidisciplinary target groups in mind, this setup gives the possibility to create concepts and working prototypes without technical expertise. For the domain of touch technology, however, we consider not only the creation of concepts as relevant, but, as an additional design goal for the toolbox, also the possibility to explore the experience and different qualities of touch. In short, we suggest an extension with the following items, which will be discussed in more detail below:
• Actuators: the most important extension is the option to use more diverse actuators for touch technology, i.e., a cluster or an array of vibration motors and pneumatic elements to explore different directions. Also heating/cooling actuators (Peltier), adjustable ribbons contracted by motors, and shape memory alloy can be considered (but are for now not explored into detail).
• Sensors: more sensors for sensing touch, the intensity of touch, and measuring of touch patterns.
• Control and connectivity: for taking the physical distribution of touch initiation and touch perception into account, controller boards offering wireless (internet) connectivity are necessary. For experimenting and experiencing more complex haptic sensations also specific control devices and hardware setups are relevant.
3.2.1. Actuators
The vibration motor has become a de-facto standard for haptics, at least it is a component many participants, students, and designers recognize from, for example, smartphones or game controllers. Many electronic and software solutions exist to improve the quality and versatility of haptics using these motors. Multiple vibration motors, together with a set of actuation patterns would be desirable. In addition, arrays or grids of vibration motors that allow the generation of haptic illusions could be a meaningful extension. To experiment with multi-channel output, a MIDI compatible miniature driver board (shown in Figure 13) has been developed as potential addition to the toolbox. This board has 14 output channels for vibration motors.
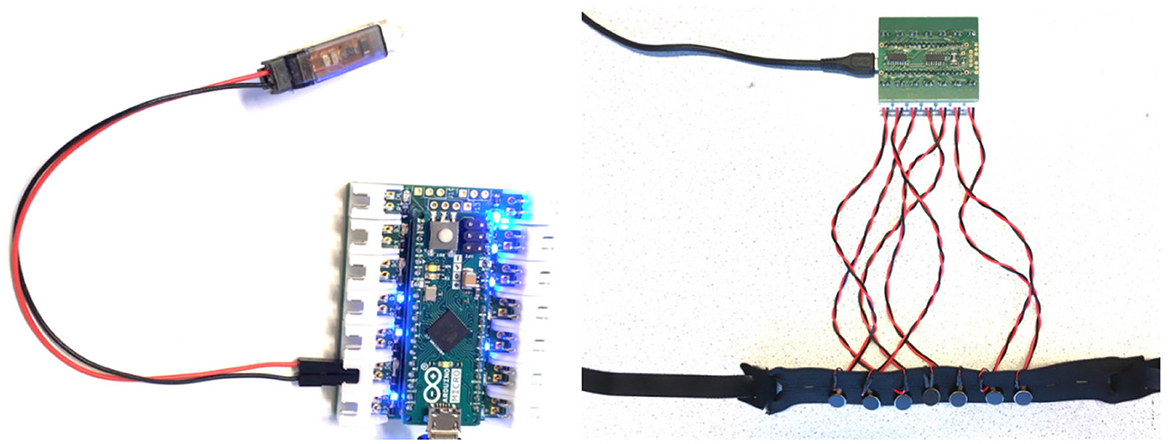
Figure 13. A 14-channel MIDI compatible control board, left controlling a pneumatic valve and right controlling 7 vibration motors.
Although vibration motors are the most mature touch technology, they cover only a fragment of potential touch sensations. It would be desirable to offer other dimensions and qualities of touch to explore. There is a number of different actuation principles that generate different touch experiences, such as stroke or pressure. Motors could be used here that use a contracting mechanism for generating pressure or a squeeze like the Hey bracelet2 contract adjustable ribbons or actuate pneumatic setups.
Inflatables or inflatable wearables have been explored (e.g., Neidlinger and Dertien, 2015), inspired by the soft robotics toolbox (Holland et al., 2014). Also McKibben muscles make use of pneumatics to provide touch through pressure, and can be integrated in textile (Backe et al., 2019). A system making use of pneumatics is typically more complex than a—just—electrically driven system. Where in previous examples, a servo or vibration motor can be connected directly to a powered controller, and for pneumatics, an air reservoir (pump, compressor, or pressurized canister) is needed, combined with air-tight tubing (electrically operated) valves and eventually an actuator, such as an inflatable pocket or bladder. Toy brands such as Lego™and Fischer Technik3 have proven that it is possible to offer a complete functioning pneumatic set at a scale suitable for prototyping of wearables. For experiments with haptic pneumatics, we experimented with a number of valves and pumps used in blood pressure sensors. Also coupling syringes (as manually operated pressure sources) to linear servos have proven to be a versatile (if a bit slow) solution to provide portable, low-power (low-noise) air supply.
To experiment with the design of McKibben actuators, the main ingredients for tinkering we found suitable are:
• Party balloons (1 m long, 5 mm diameter) as bladder
• Braided sleeve for cable protection, 5 mm diameter (stretched)
• Small barbed pneumatic connector pieces (LEGO T-pieces work well too)
• Pneumatic hose (LEGO pneumatic brand works well)
• Miniature cable-ties and heat shrink tube to seal the ends and make mechanical connection possible.
To experiment with inflatable pockets (which can act as pneumatic pressure actuator), we have been using the following ingredients:
• 3D printed casting mold
• EcoFlex 30-50 silicone casting compound
• Pneumatic hose (LEGO Pneumatic brand).
For both type of actuators, the McKibben muscles or inflatable silicone pockets, controlled supply of pressurized air is necessary. To explore pneumatic control, first a simple way of actuation is by using a syringe as pressure source. For controllable actuation, an addition of a linear servo with a stroke in the same order of magnitude works well, although the performance is too slow to generate a haptic “impulse”.
Figure 14 shows the components we eventually selected for tinkering with pneumatic control. As an air supply, either a syringe or a small 3V electric pump (used in conventional blood-pressure measuring devices) can be used. For switching air supply, either a 3-way LEGO valve or SMC070 5V solenoid valves (which can be connected directly to a low-drop power driver, as used on the previously shown board in Figure 13) is used. For air routing, we use the flexible LEGO 4 mm tube with corresponding T-pieces. For sensing air-pressure either a LEGO compatible manometer or a Honeywell NBPDANN150PGUNV with compatible INA122 instrumentation amplifier is offered.
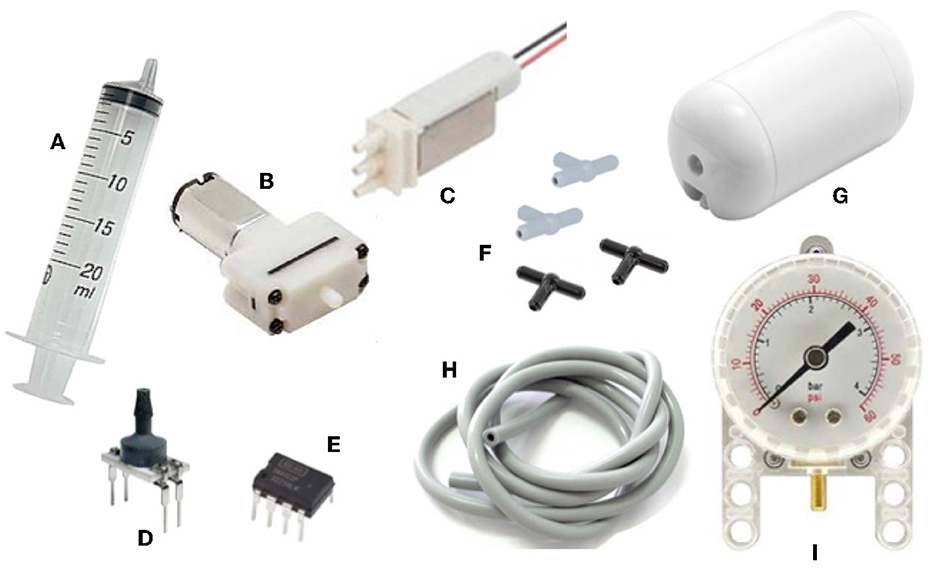
Figure 14. Selection of components for tinkering with pneumatic wearables: syringe (A) and small electric pump (B) for air supply; miniature SMC valve (C), Honeywell air pressure sensor (D), and fitting INA122 instrumentation amplifier (E). For air routing, we use LEGO(R) T-pieces (F), buffer tank (G), hose (H), and pressure gauge (I).
In this way, for all relevant aspects for exploration (supply, routing, measurement, and switching), we offer both a manual option as well as an electronic option.
Although pneumatic bladders or McKibben actuators are more complex to design and integrate than the previously mentioned vibration motors, build, construction, and control have proven to be possible within the bounds of a tinkering session. For example a one-day workshop is typically enough for exploring components, conceptualizing and designing a functional prototype, as demonstrated during a workshop at TEI (Neidlinger and Dertien, 2015).
The other actuation options mentioned, such as twisting yarn actuators using (DC)motors, servo/tendon driven mechanisms, and shape memory alloy-based actuators are still on the wish-list, but prove (for now) to be too much dependent on the integration in a garment as a whole. The actuators we deemed suitable for the tinkering toolbox are self-contained enough to be used, for example, with some simple velcro straps to explore the quality of touch. Eventually, they also allow for integration into the fabric, but it is not a prerequisite to do this from the start.
A special category are actuators that use (or produce) heat. Thermal foil, peltier elements, but also shape memory alloy produce heat, either as desired form of haptics or as a by-product (in the case of shape memory alloy). This requires careful consideration of control, power, and (thermal) protection. For now, these types of actuators have been experimented with, but they are not mature enough to become a building block in the toolbox yet.
3.2.2. Sensors
For the functional aspects of a wearable concept, the previously selected sensors for physiological measurements have proved to be sufficient. The following interesting applications can be conceptualized or prototyped.
• Heart rate (Grove Ear-clip optical heart rate sensor)
• Respiratory rate (Adafruit conductive rubber cord)
• Flexing motion (Spectra Symbol 4.5“ resistive bend sensor)
• Muscle contraction (Sparkfun EMG Muscle Sensor v3 Kit or Myoware)
• Skin resistance (Grove GSR-sensor)
• Displacement (Sharp GP2y0a21yk0f 10-80 cm distance sensor).
However, for many applications, a haptic wearable performs some form of mediation. A touch or gesture by one user is relayed to the wearable of the other, and vice versa. This means that preferably the haptic gesture (i.e., gripping a wrist, stroking an arm, and giving a pat on the back) should be sensed from the user perspective.
At the moment, the following components have been added to the toolbox for this specific category of user interaction, shown in Figure 15.
• Piezo resistive ink-based pressure sensors (such as FlexiForce and ThinForce sensors)
• Single point capacitive touch sensors (integrated boards by Grove, Adafruit, or Sparkfun)
• Multi-point capacitive touch sensors (grove board)
• Spectra Symbol SoftPot linear touch sliders
• Electric contact [Makey Makey (Collective and Shaw, 2012) style].
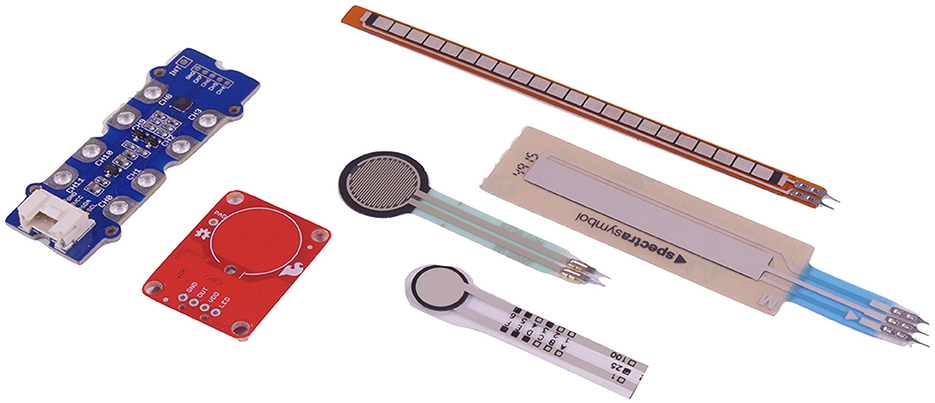
Figure 15. Sensors for sensing haptic gestures: a spectra symbol softpot flexible sliding sensor, two round piezo-resistive ink-based pressure sensors, a capacitive single-point touch sensor, and a Grove compatible 12-channel capacitive sensor board and a resistive bend sensor.
A complex aspect is that these sensors, although they are very lightweight and flexible, still do not allow full, flexible integration into a fabric. Using wires and electric contact allows for spatial layout of contact points in fabric; however, the durability of this (and potential for short circuits) does not make it suitable for prototyping and tinkering.
3.2.3. Connectivity
Since the goal of the toolkit is to facilitate conceptualizing and making working prototypes, connection of components and electronics should take little time, be safe, simple, fault tolerant (without too much in-depth knowledge of electronics), and above all robust enough to continue working in a prototype that is actually worn by a user.
A selection of contemporary toolkits for prototyping of (wearable) electronics has been made focusing on differences in connectivity strategies and also looking at the basic contents and focus. The selection is shown in Table 2 and is shown in Figure 16.
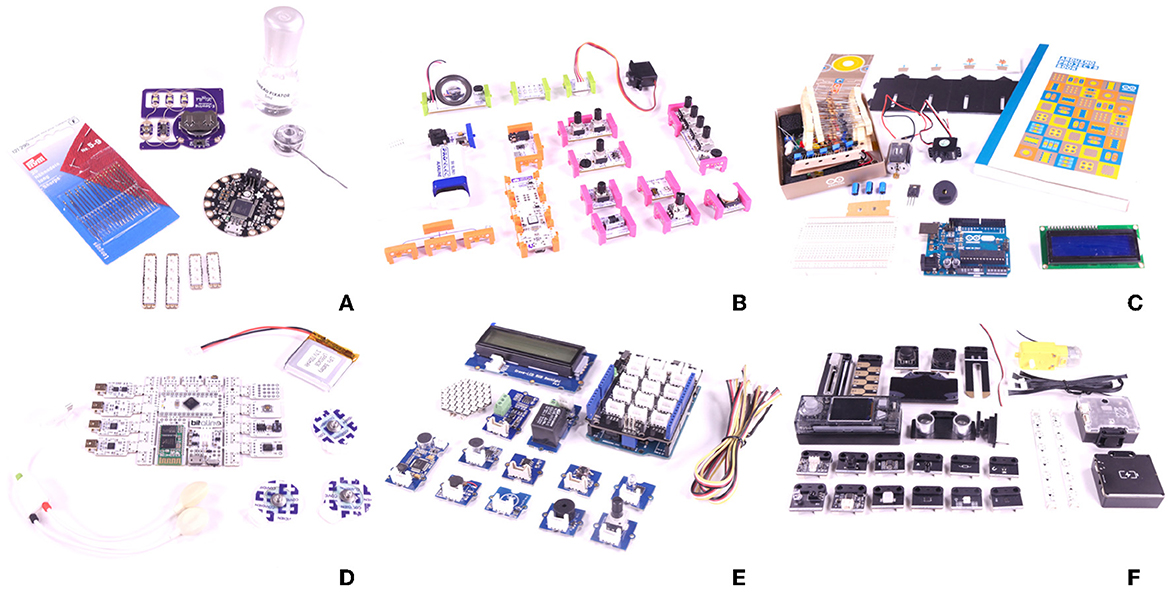
Figure 16. Platforms for prototyping of electronics considered for wearables: (A) Lilypad, (B) LittleBits, (C) Arduino starter kit, (D) Bitalino, (E) Grove, and (F) Makeblock.
Sets using the Arduino Lilypad (shown in Figures 16A) focus on sewable electronics. Components usually have large (3 mm) plated-through holes in a PCB which can be sewn onto cloth using conductive thread. This is an excellent way of experimenting with integration of electronics in clothing, but shifts focus from conceptualizing and prototyping to the more art-and-crafts side of making.
In other prototyping systems for embedded electronics, different connectivity designs are used. By far, the easiest to connect are LittleBits shown in Figure 16B: self-guiding magnetic locks automatically “snap” together. Since the connection has to be aligned well, this system is less suitable for wearable use: the system is very sensitive to motion (and works best on a flat surface or build support plate). In most Arduino starter kits (including the “official” starter kit shown in Figure 16C), a standard breadboard and jumper-wires are used. This makes connectivity completely dependent on component and is very cost efficient. A system build on breadboard is typically unsuitable for wearable use, and breadboard wires lack mechanical locking, so they rely on contact mechanics only. Hence, for the prototyping kit described in this study, we chose to find a flexible but uniform wire/cable system.
With systems such as gravity (3-wire, color indexed) and grove (4-wire, orientation lock), shown in Figure 16E, cables are grouped and uniform sockets have been chosen. This approach yields more robust connectivity at the price of having to supply (or manufacture) fixed length cables. Bitalino (Figure 16D) comes with a snap-off board which can be broken up in functional bits to wear the system on the body. For connectivity, a mini-USB (like) cable is used which again has to be obtained in correct lengths. Makeblock MakerX (Figure 16F) uses also a fixed length 4-wire lead, but instead of connecting each device individually to the main control board, the system uses a bus-system so all modules can be daisy-chained. Very robust, small, and flexible, but it comes with a price tag: every module has a small on-board microcontroller to handle bus communication.
As trade-off between complexity, price, and the desire to use uniform connectors, we chose to go for a three-wire link similar to the system proposed by DF-Robot's Gravity. Instead of the form-factor of a large (standard) Arduino or shield, we chose a very small Arduino form factor (the beetle) and augmented this with a row of 3-pin connectors similar to the Gravity system.
For wires, standard extension cables for RC-servos (with Graupner/JR sockets) have been chosen. The wires can be obtained pre-made in different lengths, are very flexible, and cost-effective, and the color coding (signal: orange, VCC: red, and GND: brown) can be explained and used well.
3.2.4. Control
Many controllers exist allowing WIFI or Bluetooth wireless connectivity. Examples are the Adafruit Feather series or ESP32 boards. Although software development and templates/examples for connectivity are vital for prototyping interactive wearables, for the scope of tinkering with haptics and social touch, we put our focus on a different aspect.
To generate haptic patterns and emulate stroking motions (by controlling a sequence of pneumatic muscles contracting, or a series of vibration motors), the control board shown in Figure 13 can be controlled from a PC or laptop as a MIDI compatible musical instrument, facilitating the design of patterns, sequences, and loops and thus allowing for simple experiments with the quality of touch. Any audio scoring software (such as Garageband, Logic, Cubase, Ableton, etc.) can be used as a tool to “compose” haptic patterns.
Patterns can be stored, edited, played, and looped to explore the qualitative aspect. As user to generate patterns, we have been using diverse (musical) input devices such as piano keyboard style (i.e., Korg nanoKey USB MIDI), sampler pad keyboard style (i.e., AKAI MPX series), and a recent (and very suitable device) pressure key style (i.e., ROLI seaboard) shown in Figure 17.
Offering students and designers a ready-to-go setup consisting of the control board (Figure 13), a laptop with audio software (or simple installation instructions) and an input device shifts the focus of the design of haptic wearables for touch from the connectivity and control to experiential design.
4. Discussion
Our initial question was whether tinkering a is design approach that can foster the finding of novel applications in STT. A design challenge based on tinkering with 34 teams of students showed that this is indeed the case. The prototypes designed realized a diverse range of applications, which was the criteria for success. Especially, a category of unexpected results supports this conclusion. These unexpected results did not apply a remote social touch as primary goal, but are part of a shared activity. These shared activities included games such as catching a ball or rock-paper-scissors, making music together, doing workout, relaxation movements, adding touches on a smart phone to text chats, or watching a movie together.
4.1. Stimulating creativity in tinkering
Creativity seems to be stimulated by (at least) the following two sources: the dialogue with the material and story telling, which will be elaborated in the following.
Here, we want to emphasize the difference between “know what” and “know how” (e.g., Cross, 1982). Tinkering, as a hands-on activity, supports “know how”. It is a “dialogue with the material”, that leads to an understanding of the working principles, properties, and limitations of the material, e.g., how components can be combined or need to be combined. To some part, this is a practical skill that includes tacit knowledge, other than explicit criteria, rules, and procedures, and “knowing how” is often in-action (Schön, 1992). In small experiments, the tinkerer gets immediate feedback of effects and experiences generated by the material. Following experiments could be invented to intensify or smoothen the observed experience, set it in a specific context, or combine it with other effects. In this way, the experiences, reflections on them, and associations guide the tinkerer in the realization of prototypes, stimulating and making use of his or her creativity. In contrast to this path, “know what” would just include the knowledge on the components and their functionality. The effect of their combinations would be an imagined experience that possibly does not match with a real experience, and it is questionable to what extent this would stimulate further steps in a design process.
Examining the students' projects, it seems that story telling is also a motor of creativity, stimulated by the toolbox and tinkering playground. With story telling, students defined contexts for which they designed their prototypes. The stories were shaped by personal backgrounds, and, at this point in time, also by the shortcomings due to the lockdown situation. In an iterative tinkering process, by interaction with the material and toolbox, stories, contexts, and prototypes were refined.
The goal of STT products is to create experiences by remote touch that increase wellbeing. On one hand, the currently available technical components come short in the replication of human touch. On the other hand, an experience of touch is not constituted by the sensors and actuators alone. A context or metaphor contributes to a high degree to an experience of touch, like the mother's hand on the forehead, or the ball game from the examples of the students. In this sense, we see the story telling part in the tinkering process as relevant for the creation of experiences. Accordingly, people and experts with different lenses also can contribute different stories and contexts and, accordingly, to prototypes with a wider spectrum of touch experiences.
4.2. Design methods and tinkering
In classical science, observation is one of the first steps of scientific activities, as part of characterization (Brody, 2012). In the world of the artificial, the object of observations is not nature, but artifacts. In Gaver (2012), the author argues “... that an endless string of design examples is precisely at the core of how design research should operate, and that the role of theory should be to annotate those examples rather than replace them.” We would like to add on this statement that there is not only an endless string of prototypes needed but also a diversity in the prototypes of that string.
Accordingly, we expect that other target groups for tinkering with a different background and expertise could increase the diversity of prototypes on STT, and in a next step, increase also the knowledge on STT gained through these. Psychologists, fashion and interaction designers, haptologists, physiotherapists, or artists have different insights about the possibilities and needs for social touch, which could be specific part of their individual tinkering toolboxes. To include these groups, the design step in the technology part of the material is needed to reduce complexity, and make it easily accessible.
Our motivation stemmed from the observation that STT suffers from low acceptance and/or market uptake. We, therefore, explicitly developed the described tinkering approach since it involves stakeholders early in the design process. Comparing the tinkering approach, as suggested in this study, to Participatory Design (PD) (Ehn, 2008), the inclusion of stakeholders in the design process is shared in both design approaches. In general, PD aims at a broader circle of stakeholders than what we have suggested here. However, the main characteristics of stakeholders that we mentioned in this study are that they bring in a different expertise on users' needs, and are not necessarily experts in technology. These characteristics would not exclude stakeholders as considered by PD. The design method in PD, however, is less specific as we see a tinkering approach, and it is more understood as a group process, which we did not emphasize here for tinkering. PD also starts more often with a more specific design question, whereas tinkering most often has a theme, but the design question typically is identified in the process.
Also in Design Thinking (DT) (Johansson-Sköldberg et al., 2013), the starting point is typically a problem to solve, and a team with heterogeneous expertise solves the problem together. Prototypes are also relevant for DT, but are only in one of many different steps in a design process, whereas tinkering is mainly oriented toward prototypes. Definitely, the management aspect in DT is absent in tinkering.
4.3. Technology acceptance
As mentioned above, only few STT products that made it to the market (Huisman, 2017), and of those that did enter the market, the majority does not persist very long. A major reason that STT products do not remain on the market is (probably) that they do not meet expectations of users. The Technology Acceptance Model (TAM) (Davis, 1989) tries to identify reasons that products are not used, one of them is the intention of potential users to really use the product. Main factors influencing the intended usage are perceived usability (PU) and perceived ease of use (PEOU) (Papakostas et al., 2021). The definition of PEOU is the degree to which a person believes that using a particular system would be free from effort (Davis, 1989). This can be directly interpreted also in the context of STT products. The definition of PU is “the degree to which a person believes that using a particular system would enhance their job performance” (Davis, 1989). As the goals of STT are not in the area of job performance, or performing tasks as efficient as possible, more suitable definitions have to be identified here. Instead, STT aims at wellbeing, social connectedness, comforting, sensoric stimulation, etc. (Jewitt et al., 2021). While it seems to be plausible that PU in the context of STT can be interpreted differently, it still needs to be validated to what extent results for PU in the original definition can also be interpreted for goals of STT mentioned. An extension of the TAM models to products of wearable technology (Chang et al., 2016) is also relevant to STT , including a range of other factors such as wearability, connectivity, social norm, and others.
On a different level, the haptics toolbox suggested itself is also a technology product that may find acceptance or not. Considering experts of other domains as users of this technology, also here technology acceptance has to be taken into account, the experts also have a role as learners. The main goals in toolbox design (Resnick et al., 2018) can be mapped directly to TAM elements: “low threshold” describes that the toolbox elements can be used with little effort, which clearly contributes to PEOU. “Wide walls” say that broad range of projects is possible, and “high ceilings” means that the projects allow also for complexity. Both are describing usefulness of a toolbox, and it is up to scaffolding (see also Section 2 for the role of a facilitator), to make this visible to the users to stimulate PU. Moreover, in Liu et al. (2021), it was shown for a learning context, concept maps have positive effect on PU and PEOU. The sense-think-act-paradigm, which is part of the introduction and scaffolding in our context, is an instance of a context map. Playfulness in a learning context was investigated as an external factor for TAM in Papakostas et al. (2022). It was shown to have a positive effect on PU and PEOU. As playfulness is a driving concept of tinkering, we assume that it also here has a positive effect on PU and PEOU. Altogether, we argue that the design criteria for the haptic tinkering toolkit and the tinkering environment described in this study do address the elements of TAM that are relevant for intention to use the haptic tinkering toolkit.
5. Conclusion and recommendations
In our study, we showed that tinkering is a suitable design approach for out-of-the box prototypes in the context of Social Touch Technology. In a teaching unit for a haptics challenge, students of a Interaction Technology master programme produced a wide range of prototypes and concepts on mediated social touch using tinkering. Moreover, it resulted in reflections on relevant concepts like intrusiveness, and how to deal with it.
Our initial motivation for this work was that there are no sufficient products of STT on the market that can mitigate needs of social touch. Increasing the variety of prototypes and concepts is one way to eventually identify the products that are really suitable, and, we showed that tinkering can contribute to the increase of the variety. Another way to identify the useful touch applications is to include experts in the design process who have more insight on the user needs, leading to prototypes that better meet the user needs and have higher acceptance. For this end, we suggest a better design of the tinkering toolkit. The current tinkering toolset is “ready to use” for people with a technical background. Making tinkering with Social Touch Technology accessible for people without technical experience, we recommend the design of a toolkit, with high-level building blocks for sensors and haptic actuators.
Data availability statement
The original contributions presented in the study are included in the article/supplementary material, further inquiries can be directed to the corresponding author.
Ethics statement
Ethical review and approval was not required for the study on human participants in accordance with the local legislation and institutional requirements. The patients/participants provided their written informed consent to participate in this study.
Author contributions
AM, JW, ED, and JE substantial contributions to the conception and design of the work, the acquisition, and analysis or interpretation of data for the work. All authors contributed to the article and approved the submitted version.
Conflict of interest
The authors declare that the research was conducted in the absence of any commercial or financial relationships that could be construed as a potential conflict of interest.
Publisher's note
All claims expressed in this article are solely those of the authors and do not necessarily represent those of their affiliated organizations, or those of the publisher, the editors and the reviewers. Any product that may be evaluated in this article, or claim that may be made by its manufacturer, is not guaranteed or endorsed by the publisher.
Footnotes
References
Backe, C., Guo, L., Jager, E., and N-K, P. (2019). “Towards responding fabrics–textile processing of thin thread-like pneumatic actuators,” in Conference Smart Materials and Surfaces SMS (Lisbon).
Chang, H., Lee, S. C., and Ji, Y. G. (2016). Wearable device adoption model with TAM and TTF. Int. J. Mobile Commun. 14, 518. doi: 10.1504/IJMC.2016.078726
Collective, B. M., and Shaw, D. (2012). “Makey Makey: improvising tangible and nature-based user interfaces,” in Proceedings of the Sixth International Conference on Tangible, Embedded and Embodied Interaction (Ontario: Kingston), 367–370.
Davis, F. (1989). Perceived usefulness, perceived ease of use, and user acceptance of information technology. MIS Q. 13, 319.
Ehn, P. (2008). “Participation in design things,” in Proceedings of the Tenth Anniversary Conference on Participatory Design 2008, PDC '08 (Bloomington: Indiana University), 92–101.
Exploratorium. (2017). Learning Dimensions of Making and Tinkering: A Professional Development Tool for Educators. Available online at: https://www.exploratorium.edu/sites/default/files/files/Learning%20Dimensions%20of%20Making%20and%20Tinkering.pdf
Field, T. (2010). Touch for socioemotional and physical well-being: a review. Dev. Rev. 30, 367–383. doi: 10.1016/j.dr.2011.01.001
Gaver, W. (2012). “What should we expect from research through design?” in Conference on Human Factors in Computing Systems - Proceedings (Austin, TX).
Hamdan, N. A., Voelker, S., and Borchers, J. (2018). Sketch&Stitch: Interactive Embroidery for E-Textiles. New York, NY: Association for Computing Machinery.
Holland, D. P., Park, E. J., Polygerinos, P., Bennett, G. J., and Walsh, C. J. (2014). The soft robotics toolkit: shared resources for research and design. Soft Robot. 1, 224–230. doi: 10.1089/soro.2014.0010
Huisman, G. (2017). Social touch technology: a survey of haptic technology for social touch. IEEE Trans. Hapt. 10, 391–408. doi: 10.1109/TOH.2017.2650221
Ipakchian Askari, S., Haans, A., and IJsselsteijn, W. (2022). Uncovering terra incognita in the AHD design space: a review of affective haptic devices. Front. Comput. Sci. 4, 795772. doi: 10.3389/fcomp.2022.795772
Jewitt, C., Price, S., Steimle, J., Huisman, G., Golmohammadi, L., Pourjafarian, N., et al. (2021). Manifesto for digital social touch in crisis. Front. Comput. Sci. 3, 754050. doi: 10.3389/fcomp.2021.754050
Johansson-Sköldberg, U., Woodilla, J., and Çetinkaya, M. (2013). Design thinking: past, present and possible futures. Creat. Innovat. Manage. 22, 121–146. doi: 10.1111/caim.12023
Jones, L., Nabil, S., and Girouard, A. (2020a). “Swatch-bits: prototyping e-textiles with modular swatches,” in Proceedings of the Fourteenth International Conference on Tangible, Embedded, and Embodied Interaction (Sidney), 893–897.
Jones, L., Nabil, S., McLeod, A., and Girouard, A. (2020b). “Wearable bits: scaffolding creativity with a prototyping toolkit for wearable e-textiles,” in Proceedings of the Fourteenth International Conference on Tangible, Embedded, and Embodied Interaction, TEI '20 (New York, NY: Association for Computing Machinery), 165–177.
Liu, Y., Liu, Y., and Yue, K. (2021). “Investigating the factors that influence technology acceptance of an educational game integrating mixed reality and concept maps,” in 2021 International Conference on Advanced Learning Technologies (ICALT) (Tartu), 409–413.
Mader, A., and Dertien, E. (2016). “Tinkering as method in academic teaching,” in 83: E&PDE 16, ed E. Bohemia (Twente: The Design Society), 240–245.
Martinez, S. L., and Stager, G. (2013). Invent to Learn: Making, Tinkering, and Engineering in the Classroom. Torrance, CA: Constructing Modern Knowledge Press.
Maziz, A., Concas, A., Khaldi, A., Stålhand, J., Persson, N.-K., and Jager, E. W. (2017). Knitting and weaving artificial muscles. Sci. Adv. 3, e1600327. doi: 10.1126/sciadv.1600327
Müller, T. (2020). Designing with haptic feedback (Dissertation). Faculty of Science and Technology, Umeå Institute of Design, Umeå University, Umeå, Sweden. Available online at: http://urn.kb.se/resolve?urn=urn:nbn:se:umu:diva-172622
Neidlinger, K., and Dertien, E. (2015). “Tei 2015 studio interactive inflatables: amplifying human behaviors,” in Proceedings of the Ninth International Conference on Tangible, Embedded, and Embodied Interaction (Stanford, CA: Stanford University), 489–491.
Papakostas, C., Troussas, C., Krouska, A., and Sgouropoulou, C. (2022). Exploring users' behavioral intention to adopt mobile augmented reality in education through an extended technology acceptance model. Int. J. Hum. Comput. Interact. 39, 1294–1302. doi: 10.1080/10447318.2022.2062551
Papakostas, C., Troussas, C., Krouska, A., and Sgouropoulou, C. (2021). Measuring user experience, usability and interactivity of a personalized mobile augmented reality training system. Sensors 21:3888. doi: 10.3390/s21113888
Petrich, M., Wilkinson, K., and Bevan, B. (2013). “Designing for tinkerability,” in It Looks Like Fun, but Are They Learning? eds M. Honey and D. E. Kanter (Taylor & Francis), pp 50–70.
Quinn, h., and Bell, P. (2013). “Designing for tinkerability,” in How Designing, Making, and Playing Relate to the Learning Goals of K-12 Science Education, eds M. Honey and D. E. Kanter (Taylor & Francis), pp 17–33.
Resnick, M The 4 p's of creative learning - passion. Available online at: https://www.youtube.com/watch?v=IAYgBf7S4NQ
Resnick, M., Myers, B., Nakakoji, K., Shneiderman, B., Pausch, R., Selker, T., et al. (2018). Design Principles for Tools to Support Creative Thinking. Carnegie Mellon University. doi: 10.1184/R1/6621917.v1
Resnick, M., and Rosenbaum, E. (2013). “Designing for tinkerability,” in Design, Make, Play: Growing the Next Generation of STEM Innovators, eds M. Honey and D. E. Kanter (Taylor & Francis), pp 163–181.
Ryoo, J., and Kekelis, L. (2018). Reframing failure in making: the value of play, social relationships, and ownership. J. Youth Dev. 13, 49–67. doi: 10.5195/jyd.2018.624
Schön, D. (1992). Designing as reflective conversation with the materials of a design situation. Knowledge Based Syst. 5, 3–14.
Shtarbanov, A. (2021). “FlowIO development platform–the pneumatic “raspberry pi” for soft robotics,” in Extended Abstracts of the 2021 CHI Conference on Human Factors in Computing Systems (Yokohama), 1–6.
Stappers, P., and Giaccardi, E. (2017). Research through Design, 2nd Edn. The Interaction Design Foundation.
van Erp, J. B. F., and Toet, A. (2015). Social touch in human-computer interaction. Front. Digit. Humanit. 2, 2. doi: 10.3389/fdigh.2015.00002
Vossoughi, S., and Bevan, B. (2014). Making and Tinkering: A Review of the Literature. National Research Council Committee on Out of School Time STEM. p. 1–55.
Keywords: tinkering, social touch technology, teaching, Research through Design, toolkit design
Citation: Mader A, Dertien E, Weda J and van Erp J (2023) Tinkering with social touch technology. Front. Comput. Sci. 5:848023. doi: 10.3389/fcomp.2023.848023
Received: 03 January 2022; Accepted: 05 June 2023;
Published: 20 July 2023.
Edited by:
Kostas Karpouzis, Panteion University, GreeceReviewed by:
Insook Choi, University of Salford, United KingdomSteve Boyer, California State University, Long Beach, United States
Antal Haans, Eindhoven University of Technology, Netherlands
Copyright © 2023 Mader, Dertien, Weda and van Erp. This is an open-access article distributed under the terms of the Creative Commons Attribution License (CC BY). The use, distribution or reproduction in other forums is permitted, provided the original author(s) and the copyright owner(s) are credited and that the original publication in this journal is cited, in accordance with accepted academic practice. No use, distribution or reproduction is permitted which does not comply with these terms.
*Correspondence: Angelika Mader, a.h.mader@utwente.nl