- 1Department of Cardiac Surgery, Fujian Branch of Shanghai Children's Medical Center, Fuzhou, China
- 2Fujian Children's Hospital, Fuzhou, China
- 3Fujian Maternity and Child Health Hospital, Affiliated Hospital of Fujian Medical University, Fuzhou, China
- 4Fujian Key Laboratory of Women and Children's Critical Diseases Research, Fujian Maternity and Child Health Hospital, Fuzhou, China
Background: This study aimed to evaluate the effects of pulmonary surfactant (PS) combined with high-frequency oscillatory ventilation (HFOV) or conventional mechanical ventilation (CMV) in infants with acute respiratory distress syndrome (ARDS) after congenital cardiac surgery.
Methods: A total of 61 infants with ARDS were eligible and were randomised to the CMV + PS group (n = 30) or the HFOV + PS group (n = 31) between January 2020 and December 2020. The primary outcomes were the changes in arterial blood gas parameters. The duration of mechanical ventilation, length of hospitalisation and the incidence of complications were considered secondary outcomes.
Results: A total of 61 infants completed the study. In the HFOV + PS group, the blood gas analysis results were significantly improved (P < 0.05), while the duration of mechanical ventilation and length of hospitalisation were shorter than the CMV + PS group (P < 0.05). However, the incidence of complications was not different between the two groups (P > 0.05).
Conclusions: Compared with the CMV + PS group, the HFOV + PS group showed significantly improved ABG variables and had a shortened length of hospitalisation and mechanical ventilation in infants with ARDS after cardiac surgery.
Clinical Trial Registration: Chinese Clinical Trial Registry; Number: ChiCTR2000039457.
Introduction
The continuous improvement of cardiopulmonary bypass technology has increased the safety of surgical correction of congenital heart disease (CHD). However, acute lung injury and acute respiratory distress syndrome (ARDS) often occur after cardiopulmonary bypass (CPB). It is reported that up to 20% of cardiac surgery patients will develop ARDS during the perioperative period, and the mortality rate is as high as 80% (1, 2). The systemic inflammatory response and lung ischaemia-reperfusion injury caused by CPB during cardiac surgery are related to ARDS (3, 4). The cornerstone of ALI/ARDS management is lung-protective mechanical ventilation with low tidal volumes (5). Among them, high-frequency oscillatory ventilation (HFOV) applies tidal volume less than or equal to the anatomical dead space for rapid gas exchange has been proven to have a protective effect on the lungs (6, 7). In addition, the application of exogenous pulmonary surfactant (PS) replacement therapy in neonatal respiratory distress syndrome (NRDS) has been popularised, and the curative effect has been determined. However, the effect of paediatric ARDS needs to be studied further (1, 8). HFOV + PS inhalation therapy has demonstrated good effects in the treatment of various respiratory diseases in newborns and infants, such as NRDS, congenital diaphragmatic hernia and meconium aspiration syndrome (9, 10). However, the research of HFOV + PS for treating secondary ARDS after cardiac surgery in infants remains limited. The purpose of this study is to evaluate the efficacy and safety of HFOV + PS vs. conventional mechanical ventilation (CMV) + PS in the treatment of ARDS after cardiac surgery.
Materials and Methods
Population and Study Criteria
This investigation was a single-centre, prospective randomised controlled study conducted in the cardiac intensive care unit (CICU) from January 2020 to December 2020 at a provincial hospital in China. The trial was approved by the ethics committee of Fujian Maternity and Child Health Hospital (NO. 2020YJ181) and adhered to the tenets of the Declaration of Helsinki (as revised in 2013). In addition, informed parental written consent from all subjects was obtained.
The inclusion criteria were as follows: 1. Infants with ARDS undergoing repair of atrial septal defect (ASD) or ventricular septal defect (VSD) in our CICU. 2. The patient's anatomical treatment was satisfactory, cardiac function recovered well-after the operation, and haemodynamics were stable. The exclusion criteria included haemodynamically significant residual lesions, pulmonary venous obstruction and parents' decision not to participate.
Allocation
After documenting parental consent, all infants were assigned to either HFOV + PS or CMV + PS using a table of computer-generated random numbers and sealed opaque envelopes.
Respiratory Management
PS
The PS (porcine surfactant, Curosurf®, Chiesi Pharmaceutical SpA, Parma, Italy) was used for intratracheal injection. The infants in both groups were given 100 mg/kg in one dose within 6 h after ARDS diagnosis. Before application, the airway secretions were aspirated, and circulation was maintained stable to correct the acid-base disorder. After the PS injection, pressurise and ventilate rapidly for 1 min, then connect to a ventilator to maintain HFOV/CMV ventilation.
Mechanical Ventilation: HFOV + PS Group
A high-frequency oscillating ventilator (SLE 5,000, SLE UK, Croyden, United Kingdom) was used for mechanical ventilation before PS application. The ventilator's initial parameters were as follows: A frequency of 8–12 Hz and an inspiratory expiratory ratio of 1:1 were used in each case; The mean airway pressure (MAP) is initially set at 10–15 cmH2O and then gradually increased with steps of 1 cmH2O every 2–3 min until the oxygenation no longer improved; Then, the MAP was decreased by 1–2 cmH2O every 2–3 min until TcSaO2 decreased, and then added 1–2 cmH2O on the basis of this MAP. The amplitude was initially set at 30–40 cm H2O. After 1 h of mechanical ventilation, the ideal lung inflation was examined by chest radiography, and the right diaphragm was generally kept at the level of the ninth rib. The parameter adjustment is determined according to the results of the dynamic monitoring of blood gas analysis. After continuous ventilation for at least 10 min, the PS was injected through the tracheal tube. The changes in oxygen saturation (SaO2), heart rate, respiration and blood pressure were closely monitored during the treatment. If the infant suffered from apnoea, SaO2 or the heart rate dropped, the injection was suspended and oxygen was rapidly pressurised until the stable state was restored. After PS was applied, mechanical ventilation was continued, and the ventilator parameters were readjusted according to the SaO2 results, blood gas analysis and chest radiograph. Infants were extubated when they achieved the following criteria: haemodynamic stability, MAP <8 cm H2O, FiO2 < 40% and weaned sedation. The infants were transferred to conventional ventilation before extubation. After weaning, nasal continuous positive pressure ventilation (NCPAP) was administered through a nasal interface.
CMV + PS Group
The Stephanie ventilator (Fritz Stephan GmbH, Gackenbach, Germany) was used for CMV. The initial parameters: FiO2: 30–50%, PIP: 18–25 cm H2O, PEEP: 4–6 cm H2O, I/E: 1–1.5:1, RR: 30–40 times/min. According to the results of SaO2, arterial blood gas (ABG) analysis and chest radiograph, the parameters of the ventilator were adjusted, and continuous positive pressure ventilation (NCPAP) was given through nasal congestion after weaning.
Definitions and Data Collection
Outcome Measures
The ventilatory settings, ABG analysis, oxygenation index (OI), duration of mechanical ventilation and complications of ventilation were recorded during the study period. The primary outcome was the changes of ABG parameters, and the secondary outcome was the duration of mechanical ventilation and length of hospitalisation. The diagnosis of ARDS was established according to the medical history, clinical manifestations and chest radiograph results which defined in 2015 by the Paediatric Acute Lung Injury Consensus Conference Group (PALCCG) (1) and met the following conditions: (1) hypoxaemia with OI ≥ 4 or oxygenation saturation index (OSI) ≥ 5; (2) new radiological lung infiltrates; (3) occurred within 7 days of a known clinical insult and (4) could not be explained by cardiac failure or fluid overload. (5) other patients with chronic lung/heart diseases, such as cyanotic heart disease, chronic lung disease and left ventricular dysfunction, to be included as long as they fulfilled the criteria above. The acute deterioration in oxygenation and pulmonary infiltrates could not be explained by their pre-existing diseases. (6) excluded patients with perinatal related lung disease. For infants younger than 44 weeks included in the study, we referred to the Montreux definition of neonatal ARDS (11). The diagnosis of ventilator-associated pneumonia (VAP) was based on the criteria established by the Centres for Disease Control and Prevention, aided by chest radiographs, positive sputum cultures, transtracheal fluid, bronchial washings and clinical findings (12). A VAP diagnosis was considered when it was identified after 48 h of mechanical ventilation. We analysed the demographics and clinical characteristics, surgical and CPB techniques, radiology and laboratory test results, ABG analysis, short-term outcomes, such as VAP, sepsis, length of mechanical ventilation, length of ICU stay and in-hospital mortality. ABG analysis was evaluated 30 min after starting HFOV or administering PS using a blood gas analyser (ABLTM 700 radiometer, Copenhagen, Denmark) and repeated every 4–6 h or more often, as needed. We elected to use OI [(FiO2 × MAP × 100) ÷ PaO2] to define the severity of PARDS. The severity of ARDS was defined as follows: mild 4 ≤ OI <8, moderate 8 ≤ OI <16, severe OI ≥ 16 (1).
Statistical Analysis
Based on the improvement of ABG in the pre-experiment, assuming the difference between the two independent populations was 15%, α = 0.05 and β = 0.2, the number of participants needed was 27 in each group. Assuming a 10% attrition rate, the total sample size was 60 (30 per group).
Data were analysed using SPSS software version 25.0 for Windows (IBM SPSS Inc., Chicago, IL, USA). Independent continuous variables are presented as the mean ± standard deviation and were analysed by t-tests. Counts and percentages describe the enumeration data. Means were compared using Student's t-test, and Fisher's exact test was used for categorical data. The Mann–Whitney U-test was applied for nonnormally distributed data. A two-sided P-value of <0.05 was regarded as statistically significant.
Results
Baseline Data
A total of 67 infants who underwent an ASD or VSD repair were screened between January 2020 and December 2020, of which four did not meet inclusion criteria, two parents of infants declined to participate. Ultimately, 61 infants were enrolled and finished the trial (31 in HFOV + PS group and 30 in CMV + PS group) (Figure 1). There were no significant differences between the two groups regarding their main clinical characteristics, including gender, GA, weight, the severity of ARDS or surgical data (Table 1). These results indicated that the two patient groups were homogeneous and comparable.
Primary Outcomes: ABG Analysis Results
There was no significant difference between the two groups of children at the beginning of treatment in arterial partial pressure of oxygen (PaO2), arterial partial pressure of carbon dioxide (PaCO2), PaO2/FiO2 and OI (P > 0.05). After 6, 12, 24, and 48 h of mechanical ventilation treatment, the blood gas analysis results were significantly improved and the difference was significant (P < 0.05) (Figure 2).
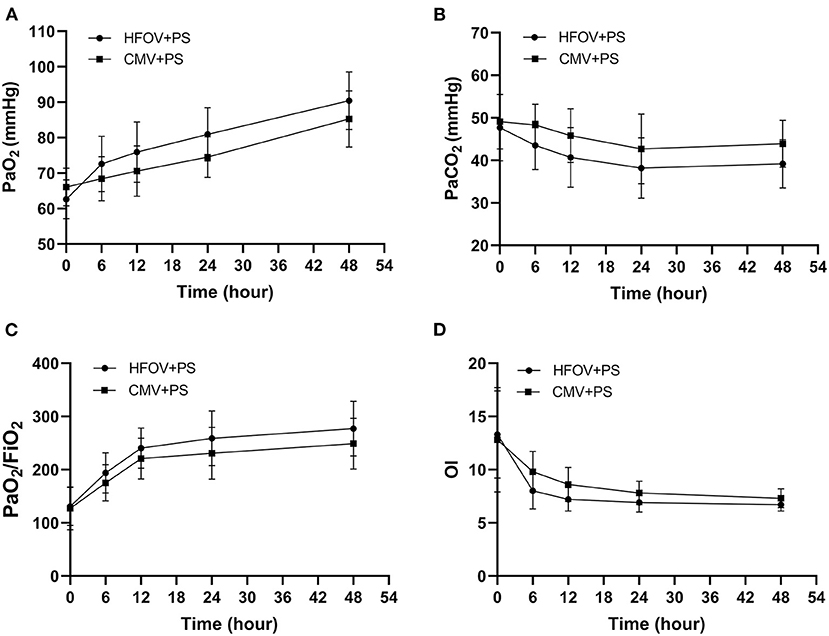
Figure 2. Arterial blood gas analysis results during the study period (compare the values at the beginning of the treatment and at 6, 12, 24, and 48 h, respectively). (A): Evaluation of PaO2 in the two groups. The PaO2 in the HFOV + PS group was significantly higher than that in the CMV + PS group during the study period (P < 0.05). (B): Evaluation of PaCO2 in the two groups during the study period. The PaCO2 in the HFOV + PS group was significantly lower than that in the CMV + PS group during the study period (P < 0.05). (C): Evaluation of PaO2/FiO2 ratio in the two groups during the study period. The PaO2/FiO2 ratio in the HFOV + PS group was significantly lower than that in the CMV + PS group during the study period (P < 0.05). (D): Evaluation of OI in the two groups during the study period. The OI in the HFOV + PS group was significantly lower than that in the CMV + PS group during the study period (P < 0.05).
Secondary Outcomes: Treatment Efficacy and Complications
During the application of HFOV + PS treatment, the heart rate decreased slightly (134 ± 17 vs. 143 ± 20 beats/min). In contrast, the mean arterial pressure (79 ± 14 vs. 83 ± 10 mmHg) and inotropic score (17.0 ± 5.5 vs. 16.0 ± 6.3) remained stable during this period, and the difference was significant. The length of hospital stays between the two groups of infants and the CICU length of stay in the HFOV + PS group were significantly shorter than in the CMV + PS group (P < 0.05). The duration of mechanical ventilation in the HFOV + PS group was also considerably shorter than that in the CMV + PS group (P = 0.03). There was no significant difference between the two groups in pneumothorax, VAP, sepsis, pulmonary haemorrhage and in-hospital mortality (P > 0.05) (Table 2).
Discussion
ARDS is a serious complication after cardiac surgery in children. The main manifestations are an increase in extensive alveolar permeability and refractory hypoxaemia. Hypoxia and acidosis damage pulmonary vascular endothelial cells and alveolar epithelial cells. Increased pulmonary microvascular permeability leads to alveolar and pulmonary interstitial oedema, damages alveolar type II cells and decreases endogenous PS production or release and activity. It also increases alveolar surface tension and decreases lung compliance leading to impaired lung function and decreased oxygenation levels (13, 14). Hypoxia and acidosis will affect haemodynamics in the short term, aggravate the degree of low cardiac output and even cause multiple organ failure. Mechanical ventilation is the essential treatment. However, studies have found that CMV can also cause and aggravate lung damage, mainly due to high airway pressure, high lung volume and repeated opening and closing of collapsed alveoli, which can trigger the release of inflammatory factors and secondary multiple organ dysfunction (15). Therefore, the lung-protective ventilation strategy has become the key to mechanical ventilation. The two most important aspects are limiting the excessive expansion of alveoli and applying relatively high PEEP to avoid the repeated closure of end-expiratory alveoli, thus reducing lung injury and promoting disease recovery. HFOV generates biphasic pressure changes with a tidal volume less than or equal to the anatomic dead space, low periodic pressure changes and hyperphysiological respiratory frequency oscillations. It then achieves an alveolar ventilation mode of effective gas exchange, which can uniformly expand the alveoli in a short time and improve gas exchange and lung compliance. HFOV is active in inhalation and exhalation during ventilation, effectively improving oxygenation and carbon dioxide emission without increasing barotrauma. Its hypoventilation strategy can prevent lung injury during mechanical ventilation and improve the survival rate (16, 17). Poddutoor et al. (18) conducted a study on 675 neonates receiving CMV. They found that 97 cases were switched to HFOV treatment after CMV treatment failed. In addition, the pulmonary oxygenation function and ABGs were significantly improved after 2 h. Our study also showed that the ABG results in the HFOV + PS group were significantly improved after treatment. Although the ABG results in the CMV + PS group were also improved, the effect was not as apparent as that in the HFOV + PS group. In addition, the average length of hospital stays and on-boarding time of infants in the HFOV + PS group were significantly shorter than those in the CMV + PS group. Also, the length of hospitalisation and the duration of mechanical ventilation in the HFOV + PS group were considerably shorter than those in the CMV + PS group. This was because, compared with CMV, the high-speed flow of gas produced by HFOV could quickly diffuse the PS to the surface of the alveoli, reduce its surface tension, further expand the alveoli, maintain their stability, improve the alveolar ventilation and ventilation function and further relieve the symptoms of hypoxia and acidosis.
PS replacement therapy can reduce alveolar surface tension, prevent alveolar collapse at the end of expiration, maintain functional residual capacity, stabilise alveolar pressure and reduce fluid leakage from the capillaries to the alveoli. At the same time, it can improve lung compliance and ventilation function and reduce ventilator parameters (19, 20). Studies have shown that early use of PS in children with ARDS can shorten the duration of mechanical ventilation and improve oxygenation (8, 21, 22). In addition, PS combined with mechanical ventilation can significantly increase PaO2/FiO2, decrease OI and significantly improve the oxygenation function of children with ARDS compared with simple mechanical ventilation (23, 24). This study also showed that the duration of mechanical ventilation and oxygenation of the HFOV + PS group were significantly lower than those of the CMV + PS group. The mechanism may be that HFOV can open occluded small airways and alveoli. The high-speed ventilation frequency and unique gas exchange mode accelerate the uniform distribution of the PS on alveolar walls. In addition, the immunomodulatory, biophysical and antibacterial properties of surfactants help to stabilise the alveoli and reduce alveolar-capillary oedema, ultimately improving lung function (24). When a PS is used in combination with HFOV, it can significantly improve breathing and reduce the ventilation duration.
Our study found that when HFOV was used in combination with the PS, the incidence of pulmonary haemorrhage, pneumothorax, VAP, intracranial haemorrhage and mortality in the two groups was not significant. Also, scholars have always been concerned about the safety of HFOV. Studies have found that the incidence of chronic lung disease and mortality after the application of HFOV is slightly superior to that of CMV, and there is no significant difference in the occurrence of complications, such as air leakage and brain injury (25, 26). Current clinical studies have shown that although exogenous PS can improve pulmonary gas exchange in infants with ARDS, its effect on reducing the mortality rate is uncertain (8, 27). Clinical research should be continued to determine which types of ARDS are effective for PS, and the appropriate timing, dosage and method of administration. Therefore, it is necessary to conduct a multicentre study to discuss related issues and provide evidence for the clinical use of exogenous PS in ARDS.
Although studies have demonstrated the effectiveness of surfactant and HFOV in neonates and infants in NRDS or ARDS. However, the application of HFOV combined with surfactant in ARDS during the perioperative period of congenital heart disease is still blank. This is the first study using HFOV+PS in infants with ARDS after congenital cardiac surgery. However, it has several limitations. First, this randomised controlled study was not a double-blind study; there were many subjective indicators in the research's evaluation criteria. Second, this was a single-centre study with a relatively small sample size. Finally, this study was limited to specific patients undergoing congenital cardiac surgery, and other patients might have different results. Therefore, additional, multicentre, prospective, randomised, double-blind, long-term studies with larger samples are needed.
Conclusions
Compared with CMV + PS group, HFOV + PS can significantly improve the ABG variables and shorten the length of hospitalisation and mechanical ventilation in infants with ARDS after cardiac surgery. Meanwhile, it does not increase the incidence of complications.
Data Availability Statement
The original contributions presented in the study are included in the article/Supplementary Material, further inquiries can be directed to the corresponding authors.
Ethics Statement
The studies involving human participants were reviewed and approved by the ethics board of Fujian Medical University approved the study (NO. 2020KY039). Written informed consent to participate in this study was provided by the participants' legal guardian/next of kin.
Author Contributions
QC and Y-RZ conceived the idea. H-LW and Y-QL conducted the analyses. NX and S-TH provided the data. All authors contributed to the article and approved the submitted version.
Conflict of Interest
The authors declare that the research was conducted in the absence of any commercial or financial relationships that could be construed as a potential conflict of interest.
Acknowledgments
We highly acknowledge the participating researchers' contribution: Qi-Liang Zhang, Wang-Sheng Dai, Ze-Wei Lin, Li-Wen Wang, Jing Wang, and Ling-Shan Yu.
Supplementary Material
The Supplementary Material for this article can be found online at: https://www.frontiersin.org/articles/10.3389/fcvm.2021.675213/full#supplementary-material
References
1. Khemani RG, Smith LS, Zimmerman JJ, Erickson S. Pediatric acute lung injury consensus conference group. Pediatric acute respiratory distress syndrome: definition, incidence, and epidemiology: proceedings from the Pediatric Acute Lung Injury Consensus Conference. Pediatr Crit Care Med. (2015) 16(5 Suppl 1):S23–40. doi: 10.1097/PCC.0000000000000432
2. Stephens RS, Shah AS, Whitman GJ. Lung injury and acute respiratory distress syndrome after cardiac surgery. Ann Thorac Surg. (2013) 95:1122–9. doi: 10.1016/j.athoracsur.2012.10.024
3. Rong LQ, Di Franco A, Gaudino M. Acute respiratory distress syndrome after cardiac surgery. J Thorac Dis. (2016) 8:E1177–86. doi: 10.21037/jtd.2016.10.74
4. Fink MP. Role of reactive oxygen and nitrogen species in acute respiratory distress syndrome. Curr Opin Crit Care. (2002) 8:6–11. doi: 10.1097/00075198-200202000-00002
5. Acute Respiratory Distress Syndrome Network, Brower RG, Matthay MA, Morris A, Schoenfeld D, Thompson BT, et al. Ventilation with lower tidal volumes as compared with traditional tidal volumes for acute lung injury and the acute respiratory distress syndrome. N Engl J Med. (2000) 342:1301–8. doi: 10.1056/NEJM200005043421801
6. Yildizdas D, Yapicioglu H, Bayram I, Yilmaz L, Sertdemir Y. High-frequency oscillatory ventilation for acute respiratory distress syndrome. Indian J Pediatr. (2009) 76:921–7. doi: 10.1007/s12098-009-0151-9
7. Bojan M, Gioanni S, Mauriat P, Pouard P. High-frequency oscillatory ventilation and short-term outcome in neonates and infants undergoing cardiac surgery: a propensity score analysis. Crit Care. (2011) 15:R259. doi: 10.1186/cc10521
8. Willson DF, Thomas NJ, Markovitz BP, Bauman LA, DiCarlo JV, Pon S, et al. Effect of exogenous surfactant (calfactant) in pediatric acute lung injury: a randomized controlled trial. JAMA. (2005) 293:470–6. doi: 10.1001/jama.293.4.470
9. Lin XZ, Lai JD, Lv M, Zhu Y, Wang L, Chen C. Clinical efficacy of high-frequency oscillatory ventilation combined with pulmonary surfactant in treatment of neonatal pulmonary hemorrhage. Zhongguo Dang Dai Er Ke Za Zhi. (2015) 17:345–9. doi: 10.7499/j.issn.1008-8830.2015.04.011
10. Rettwitz-Volk W, Veldman A, Roth B, Vierzig A, Kachel W, Varnholt V, et al. A prospective, randomized, multicenter trial of high-frequency oscillatory ventilation compared with conventional ventilation in preterm infants with respiratory distress syndrome receiving surfactant. J Pediatr. (1998) 132:249–54. doi: 10.1016/S0022-3476(98)70440-8
11. De Luca D, van Kaam AH, Tingay DG, Courtney SE, Danhaive O, Carnielli VP, et al. The montreux definition of neonatal ARDS: biological and clinical background behind the description of a new entity. Lancet Respir Med. (2017) 5:657–66. doi: 10.1016/S2213-2600(17)30214-X
12. Horan TC, Andrus M, Dudeck MA. CDC/NHSN surveillance definition of health care-associated infection and criteria for specific types of infections in the acute care setting. Am J Infect Control. (2008) 36:309–32. doi: 10.1016/j.ajic.2008.03.002
13. Deterding RR. Infants and young children with children's interstitial lung disease. Pediatr Allergy Immunol Pulmonol. (2010) 23:25–31. doi: 10.1089/ped.2010.0011
14. Matthay MA, Zemans RL. The acute respiratory distress syndrome: pathogenesis and treatment. Annu Rev Pathol. (2011) 6:147–63. doi: 10.1146/annurev-pathol-011110-130158
15. Slutsky AS, Ranieri VM. Ventilator-induced lung injury. N Engl J Med. (2013) 369:2126–36. doi: 10.1056/NEJMra1208707
16. Singh SN, Malik GK, Prashanth GP, Singh A, Kumar M. High frequency oscillatory ventilation versus synchronized intermittent mandatory ventilation in preterm neonates with hyaline membrane disease: a randomized controlled trial. Indian Pediatr. (2012) 49:405–8. doi: 10.1007/s13312-012-0084-7
17. Marini JJ. Mechanical ventilation: past lessons and the near future. Crit Care. (2013) 17(Suppl. 1):S1. doi: 10.1186/cc11499
18. Poddutoor PK, Chirla DK, Sachane K, Shaik FA, Venkatlakshmi A. Rescue high frequency oscillation in neonates with acute respiratory failure. Indian Pediatr. (2011) 48:467–70. doi: 10.1007/s13312-011-0073-2
19. Walsh BK, Daigle B, DiBlasi RM, Restrepo RD. American association for respiratory care. AARC clinical practice guideline. Surfactant replacement therapy: 2013. Respir Care. (2013) 58:367–75. doi: 10.4187/respcare.02189
20. Ma CC, Ma S. The role of surfactant in respiratory distress syndrome. Open Respir Med J. (2012) 6:44–53. doi: 10.2174/1874306401206010044
21. Amigoni A, Pettenazzo A, Stritoni V, Circelli M. Surfactants in acute respiratory distress syndrome in infants and children: past, present and future. Clin Drug Investig. (2017) 37:729–36. doi: 10.1007/s40261-017-0532-1
22. Yapicioglu H, Yildizdaş D, Bayram I, Sertdemir Y, Yilmaz HL. The use of surfactant in children with acute respiratory distress syndrome: efficacy in terms of oxygenation, ventilation and mortality. Pulm Pharmacol Ther. (2003) 16:327–33. doi: 10.1016/S1094-5539(03)00088-9
23. Möller JC, Schaible T, Roll C, Schiffmann JH, Bindl L, Schrod L, et al. Treatment with bovine surfactant in severe acute respiratory distress syndrome in children: a randomized multicenter study. Intensive Care Med. (2003) 29:437–46. doi: 10.1007/s00134-003-1650-1
24. Rodríguez-Moya VS, Gallo-Borrero CM, Santos-Áreas D, Prince-Martínez IA, Díaz-Casañas E, López-Herce Cid J. Exogenous surfactant and alveolar recruitment in the treatment of the acute respiratory distress syndrome. Clin Respir J. (2017) 11:1032–9. doi: 10.1111/crj.12462
25. Cools F, Offringa M, Askie LM. Elective high frequency oscillatory ventilation versus conventional ventilation for acute pulmonary dysfunction in preterm infants. Cochrane Database Syst Rev. (2015) 3:Cd000104. doi: 10.1002/14651858.CD000104.pub4
26. Gallini F, Maggio L, Cota F, Serrao F, Vento G, Mercuri E, et al. 65 randomized trial of high-frequency oscillatory ventilation versus conventional ventilation: a 3 year follow-up. Pediatr Res. (2010) 68:36. doi: 10.1203/00006450-201011001-00065
Keywords: high-frequency oscillatory ventilation, pulmonary surfactant, acute respiratory distress syndrome, congenital heart surgery, postoperative care
Citation: Zheng Y-R, Lei Y-Q, Liu J-F, Wu H-L, Xu N, Huang S-T, Cao H and Chen Q (2021) Effect of High-Frequency Oscillatory Ventilation Combined With Pulmonary Surfactant in the Treatment of Acute Respiratory Distress Syndrome After Cardiac Surgery: A Prospective Randomised Controlled Trial. Front. Cardiovasc. Med. 8:675213. doi: 10.3389/fcvm.2021.675213
Received: 08 April 2021; Accepted: 28 June 2021;
Published: 22 July 2021.
Edited by:
Massimo Bonacchi, University of Florence, ItalyReviewed by:
Hasan Özkan, Dokuz Eylül University, TurkeyMaruti Haranal, National Heart Institute, Malaysia
Copyright © 2021 Zheng, Lei, Liu, Wu, Xu, Huang, Cao and Chen. This is an open-access article distributed under the terms of the Creative Commons Attribution License (CC BY). The use, distribution or reproduction in other forums is permitted, provided the original author(s) and the copyright owner(s) are credited and that the original publication in this journal is cited, in accordance with accepted academic practice. No use, distribution or reproduction is permitted which does not comply with these terms.
*Correspondence: Qiang Chen, Y2hlbnFpYW5nMjIyOEAxNjMuY29t; Hua Cao, Y2FvaHVhMDc5MUAxNjMuY29t