- 1Laboratory of Cardiology, Department of Cardiology, GIGA Institute, CHU Sart Tilman, University of Liège, Liège, Belgium
- 2Center for Proteomics and Metabolomics, Leiden University Medical Center, Leiden, Netherlands
- 3Department of Pathology, Centre Hospitalier Universitaire (CHU) University Hospital, Liège University, Liège, Belgium
- 4Laboratory of Experimental Pathology, Groupe Interdisciplinaire de Géno-protéomique Appliquée (GIGA) Institute, Liege University, Liège, Belgium
- 5Gruppo Villa Maria Care and Research, Maria Cecilia Hospital, Cotignola, Italy
- 6Anthea Hospital, Bari, Italy
Aims: Palmitic acid (PA) and oleic acid (OA) are two main dietary fatty acids. Dietary intake of PA has been associated with cardiovascular disease risk, and the effect of OA remains uncertain. Our study aimed to assess the effect of a short-term intake of lard, as source of PA and OA, on aorta and aortic valve.
Methods and Results: Rabbits were fed with two lard-enriched diets, containing either elevated levels of PA or of both PA and OA as compared to chow diet. After 16 weeks of each diet, calcification was observed in the aortic intima and in the aortic valve. The extent of calcification did not differ between the two diets. In contrast, rabbits fed chow diet did not develop any calcification. In blood, PA enrichment resulted in decreased lymphocyte and monocyte counts and increased levels of hemoglobin and haematocrit. Levels of the calcification inhibitor fetuin-A were also diminished, whereas creatinine levels were raised. Of note, none of the diets changed cholesterol levels in LDL or HDL. Comprehensive quantitative lipidomics analysis identified diet-related changes in plasma lipids. Dietary PA enrichment led to a drop of polyunsaturated fatty acids (PUFA), in particular of linoleic acid in cholesteryl esters, triglycerides and diacylglycerols (DAG). Ratios of PA to 18-carbon PUFA in DAG were positively correlated with the extent of aortic valve calcification, and inversely with monocyte counts. PA content in blood correlated with aorta calcification.
Conclusions: Regular dietary PA intake induces vascular and valvular calcification independently of traditional risk factors. Our findings raise awareness about PA-rich food consumption and its potential deleterious effect on cardiovascular health.
Introduction
It is well-established that the risk of cardiovascular disease (CVD) is influenced by nutrition habits (1, 2). Dietary fatty acids, comprising saturated fatty acids (SFAs), monounsaturated fatty acids (MUFAs) and polyunsaturated fatty acids (PUFAs) are known to differentially affect CV health. It has been long considered that SFAs could increase the risk of CVD through their ability to raise low density lipoprotein cholesterol (LDL-C), whereas PUFAs could play a protective role by lowering total and LDL-C (3). Recently, it has been found that dietary SFAs do not necessarily increase the risk of CVD, which could be explained by various effects on LDL profiles (e.g., small dense LDL, large buoyant LDL, very low density lipoproteins, LDL oxidation) with distinct atherogenic effects (4), smaller size LDL particles being more likely to penetrate in vessel walls and contributing to atherosclerosis. It has now become clear that the food source and type of SFA are key in determining the risk of CVD (5, 6). Data from the Rotterdam Study revealed that a high risk of coronary artery disease (CAD) was associated with intake of palmitic acid (16:0) (PA), but not of SFA with other chain lengths (7). PA is the major SFA in palm oil, palm kernel oil and lard, the most widely consumed vegetable oils and animal fat, respectively. PA could be responsible for harmful cardiovascular effects by acting as a pro-inflammatory stimulus (8–11) and/or through direct effect on cardiovascular structures. An in vitro study indicates that PA promotes vascular calcification through direct effect on vascular smooth muscle cells (12, 13). The health impact of MUFAs is a matter of controversy (14). Oleic acid (18:1) (OA) is the most abundant MUFA, with a high content in olive oil. In the Multi-Ethnic study of Atherosclerosis, high plasma levels of OA correlated with coronary artery calcification, carotid plaque and aortic valve calcification. Most importantly, elevated OA levels were associated with CV events and all-cause mortality independently of typical risk factors (15). However, dietary OA hardly correlates with plasma levels of OA (16), and the impact of dietary OA on CV risk remains uncertain. PA and OA are two major daily food components. Yet, to date, there are no experimental studies that investigated whether regular PA or OA intake causes vascular and/or valvular calcification.
Our study aimed to assess the effect of a regular intake of a PA- and OA-rich food on rabbit aorta and aortic valve structures. Plasma lipids were then analyzed by comprehensive quantitative lipid analysis in order to identify diet-related changes in lipid profiles.
Materials and Methods
Ethic Statement and Animal Model
All rabbit experiments conform to the European Union guidelines for the care and use of laboratory animals and were conducted with protocols approved by the Animal Ethical Committee of the University of Liège (protocol number 1951). Twenty eight-week-old male New Zealand White rabbits (body weight 1.85 ± 0.17 kg) were purchased from Charles River (Charles River, France). Animals were kept in a temperature- and humidity-controlled environment in an air-conditioned room with standard rabbit chow and tap water ad libitum. After 2 weeks of acclimation, rabbits were randomly assigned in three different groups. They received the following diets for 16 weeks. The control group was composed of seven rabbits that received standard rabbit chow. The 0.175% group (0.175%) was composed of seven rabbits that received standard rabbit chow supplemented with 1.75 g of lard/kg. The 5% group (5%) was composed of six rabbits that received standard rabbit chow supplemented with 50 g of lard/kg. Lard-supplemented chows were produced by Safe company (Safe, France). Lard contained 23.8% PA and 41.2% OA. Body weight was measured on a weekly basis. Blood draws and echocardiography were performed at baseline, mid-protocol and end-protocol. Aortic valve and left ventricular functions were assessed by echocardiography using a neonatal 12S probe and a VividTM E95 system (GE Healthcare). Anesthesia was induced prior to any procedure (except body weight measurements) by intramuscular injections of droperidol (0.625 mg/kg), xylazine (5 mg/kg) and ketamine (35 mg/kg) in hind legs. Euthanasia was conducted in anesthetized animals by intracardiac injection of pentobarbital (200 mg/kg).
Histological Analyses of Rabbit Heart
Hearts were harvested, weighed and fixed in 4% paraformaldehyde–PBS solution for 24 h before dehydration into 70% ethanol solution overnight at room temperature. One heart from a rabbit of the chow diet group was lost during the procedure. They were then embedded in paraffin wax and sectioned at 7 μm. Tissue structure was studied on hematoxylin-eosin stained sections. Tissue calcification was assessed on alizarin red stained sections (Supplementary Figure 1). Quantification of aorta and aortic valve calcified areas was conducted on alizarin stained heart sections using QuPath, an open-source software designed for histopathology slide analysis (17). We used the random forest method. This algorithm is a robust supervised machine learning method for pixel classification. Classification was based on calcified and non-calcified areas of available sections from rabbit aorta showing macrocalcifications that were detectable by computed tomography.
Blood Processing
At each blood draw, differential white blood cell counts, red blood cell indices, platelet count and mean platelet volume were measured in 3.8% citrated-anticoagulated whole blood on a Cell-Dyn 3700 hematocytometer (Abbott Laboratories). EDTA and 3.8% citrated plasma aliquots were prepared through two successive centrifugation steps at 1,700 g at room temperature and stored at −80°C. Serum aliquots were prepared via a 30-min rest to allow blood clotting followed by one centrifugation step at 1,700 g at room temperature. They were stored at −80°C until further analysis.
Biomarker Measurements
Measurements of fetuin-A and IL-6 were performed in serum by enzyme-linked immunosorbent assays according to the manufacturer's recommendations (fetuin-A: MyBiosource, IL-6: Cusabio). Inactive dp-ucMGP level was measured in EDTA plasma through an automated chemiluminescence sandwich immunoassay on the IDS-iSYS Multi-Discipline Automated System (ImmunoDiagnosticSystems). Measurements of calcium, phosphorus, iron, transferrin, creatinine, total cholesterol, triglycerides, LDL-c, HDL-c, ApoA and ApoB were performed in serum by the use of the AU480 Chemistry Analyzer (Beckman Coulter).
Lipidomics
Lipidomics analysis was carried out on the commercial LipidyzerTM platform, according to the manufacturer's instructions with some modifications (Sciex) (18, 19). The Lipidyzer platform is a targeted, quantitative lipidomics platforms, monitoring more than 1.000 lipid species from 13 lipid classes (CE, cholesterol ester; CER, ceramides; DAG, diacylglycerides; DCER, dihydroceramides; FFA, free fatty acids; HCER, hexosylceramides; LPC, lysophosphatidylcholine; LPE, lysophosphatidylethanolamine; PC, phosphatidylcholine; PE, phosphatidylethanolamine; SM, sphingomyelin; and TAG, triacyltriglycerides). The platform is a flow-injection based approach based on differential mobility separation of lipid classes with subsequent MRM scanning of individual lipid species on a QTrap 5500 mass spectrometer equipped with SelexION technology (Sciex). Quantification is based on isotope dilution, incorporating 54 isotopically labeled standards. Each lipid species is corrected by the closest deuterated IS within its lipid class, in terms of carbon and double bond number of the fatty acid side chain. Subsequently, the obtained area ratio is multiplied by the concentration of the respective IS and corrected for volume and weight; as a consequence, this quantitation can be considered accurate within a specified quantitative bias. For a detailed technical description of the method and its quantitative nature, please refer to Alarcon-Barrera et al. (19), Contrepois et al. (20), and Cao et al. (21). Briefly, lipid analysis was performed in 3.8% citrated plasma after methyl-tert-butyl ether (MTBE) extraction in flow-injection mode, separating lipid classes by differential mobility spectroscopy (22) followed by tandem mass spectrometry of lipid species with a QTrap 5500 operated in multiple reaction monitoring mode. Lipid species were identified and quantified on the basis of characteristic mass spectrometric transitions. Commercial Lipidyzer software automatically calculated lipid species concentrations. All samples were analyzed in a randomized fashion. Control plasma samples, blanks and pooled study samples were assessed daily as quality controls. To 25 μL of rabbit plasma was added 25 μL of Lipidyzer internal standard mix. Six hundred microliters MTBE and 150 μL methanol was added and the samples were vortex and stored at room temperature for 30 min. Subsequently, samples were centrifuged at 16.100 × g for 5 min. Seven hundred and fifty microliters of the organic extract was transferred to a fresh 2 mL Eppendorf tube. Lipid extraction was repeated adding 300 μL MTBE and 100 μL methanol to the original sample. Samples were vortexed and centrifuged for 15 min at 16.100 × g. Three hundred and fifty microliters was transferred and organic extracts combined. For phase separation, 300 μL of water was added to the combined organic extracts and samples were spun for 5 min. at 16.100 × g. Seven hundred microliters of the organic extract was subsequently transferred to a glass vial and dried under a gentle stream of nitrogen. The dried organic extract was re-dissolved in 250 μL Lipidyzer running buffer [10 mM ammonium acetate in (50:50 [v/v]) methanol:dichloromethane] and transferred to a glass micro-vial insert. Samples were analyzed on the Lipidyzer platform.
Statistical Analysis
Readouts of the Lipidyzer™ platform were lipid class concentration (nmol/g), lipid species concentration (nmol/g), specific fatty acid concentration by lipid class (nmol/g) and the corresponding compositional data (%) of all previously mentioned items. The entire lipidomic datasets are shown in Supplementary Table 1. Missing data were imputed with 10% of the lowest data measured. Between-diet comparison was performed by ANOVA and post-hoc Dunnett's test with log2 transformed data. Normality of variables was tested by Shapiro-Wilk test. Over time changes in blood cells and circulating biomarkers were assessed by either paired t-test or Wilcoxon signed-rank test when appropriate. Correlations between calcification, lipid data and circulating biomarkers were analyzed by either Pearson or Spearman method and results were shown with corresponding correlation coefficient (Pearson r or Spearman ρ). Data are presented as median (interquartile range [p25–p75]). All tests were performed 2-sided and P < 0.05 was considered significant. All statistical analyses were performed using SAS 9.4 (SAS Institute, Cary NC).
Results
Regular Intake of PA Causes Mild Calcification of Aortic Valve and Aorta
Rabbits were fed with a standard chow diet or diets enriched with 0.175% and 5% lard diet groups. Diet compositions are shown in Table 1. Proteins and nitrogen free extracts did not differ between the three diets. The 0.175% lard diet contained PA levels similar to average consumption by children aged 3 to 14 years (CCAF 2013 survey, France). In this diet, PA accounted for most SFA. It contained twice less OA compared to chow diet. PA/PUFA, SFA/PUFA and MUFA/PUFA ratios were increased by about 2-fold, while the ratio of OA/PUFA was slightly lower than in chow diet. The 5% lard diet contained higher levels of both PA and OA, which resulted in elevated ratios of PA (7.4-fold) and OA (3-fold) to PUFA as compared to chow diet.
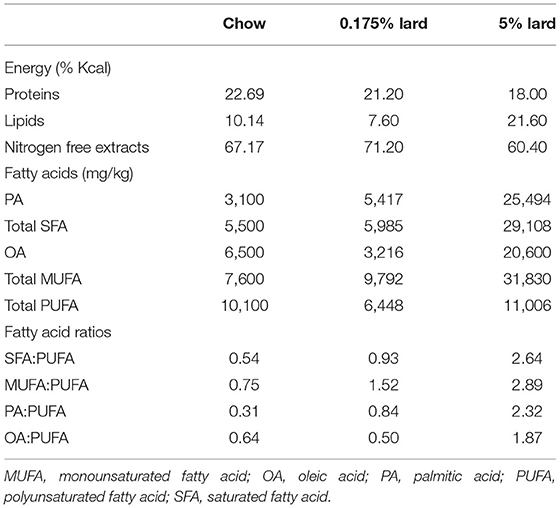
Table 1. Composition of chow, 0.175% and 5% lard diets in terms of energy (%Kcal) and fatty acid content.
After 16 weeks of diets, body and heart weights remained unchanged between groups. Echocardiographic examination did not reveal any vascular or valvular functional alteration. We then performed histological analyses of heart sections. Hematoxylin-eosin staining of heart sections from rabbits fed with lard-containing diets did not show any vascular or valvular structural modification as compared to control rabbits (Figures 1A,B). However, alizarin red staining of serial sections revealed calcium deposits in arterial and valvular tissues in both 0.175% and 5% lard groups (Figures 1A,B and Supplementary Figure 1). Whole tissue of large arteries and aortic valve appeared reddish by optical microscopy. We observed microcalcifications in the aortic media as well as calcification lining the aortic intima and the ventricularis side of the aortic valve. There were no calcium deposits in the control group. Computational quantification of alizarin red-stained calcium deposits indicated variable percentages of calcified area in aorta and aortic valves among rabbits fed with lard diets, which did not differ between the 0.175% and the 5% lard diet groups (Figures 1A,B). Thus, these results indicate that a slight elevation of dietary PA triggers a calcification process in aorta and aortic valve. Higher levels of both PA and OA in diets does not increase this process further.
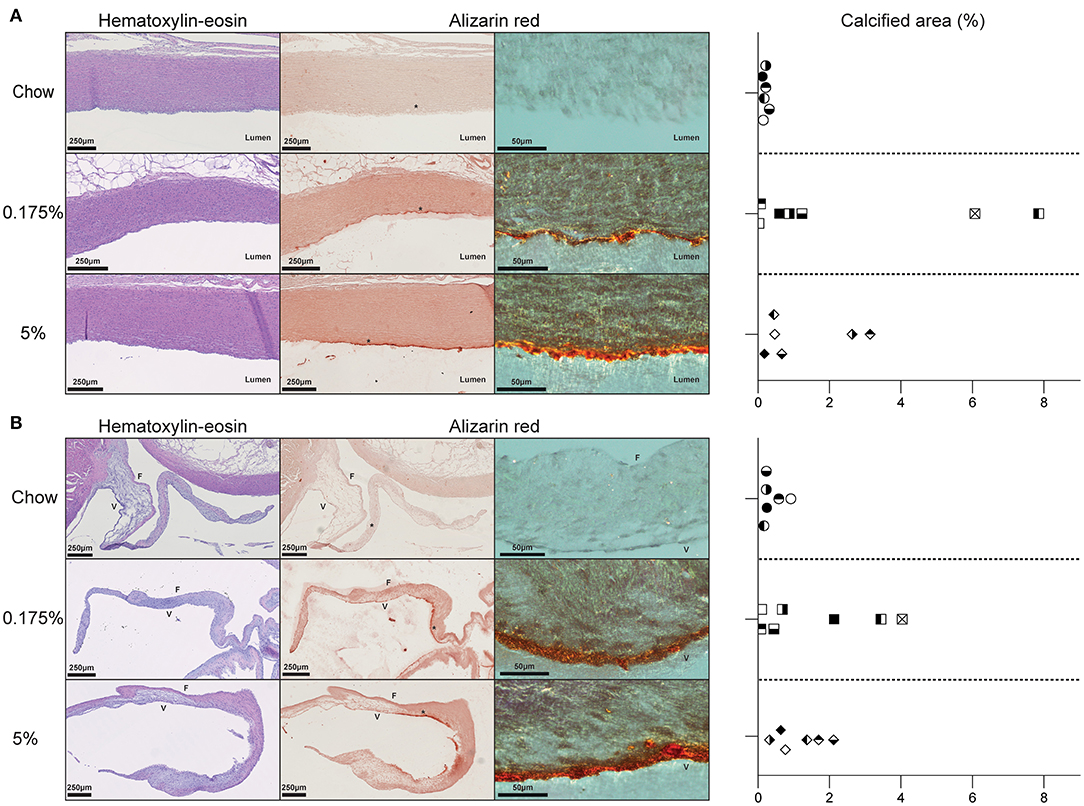
Figure 1. Effect of PA and PA/OA enriched diets on rabbit aorta and aortic valve structures. (A). Representative pictures of aortic wall for chow, 0.175% and 5% lard diet groups stained with hematoxylin-eosin or alizarin red. Graph represents the percentages of calcified areas in aorta in all three rabbit groups. (B). Representative pictures of aortic valve of chow, 0.175% and 5% lard diet groups stained with hematoxylin-eosin, hematoxylin-eosin or alizarin red. Graph represents the percentages of calcified areas in aortic valve in all three rabbit groups. Alizarin red pictures are taken with light microscopy (left panels) and with polarized light microscopy at higher magnification of * zone (right panels). In graphs, one symbol corresponds to one rabbit.
Influence of PA- and PA/OA-Enriched Diets on Blood Cells and Circulating Biomarkers
We investigated whether the 0.175% and 5% lard diet groups provoked changes in blood cells, inflammation, calcification biomarkers, and minerals after 16 weeks (Table 2). The 0.175% lard diet caused a decrease of lymphocyte, monocyte and basophil counts, which led to an increase of platelet-to-lymphocyte ratio, of neutrophil percentage and neutrophil-to-lymphocyte ratio. In the 5% lard group, the monocyte counts similarly decreased and the neutrophil percentage increased, but the lymphocyte counts were not modified. Levels of hemoglobin and haematocrit raised in the two lard diet groups together with a decrease of the calcification inhibitor fetuin-A and phosphorous, and an increase of creatinine levels. In contrast, we did not observe any changes in dephosphorylated-uncarboxylated Matrix Gla-protein (dp-ucMGP), nor significant induction of the inflammatory cytokine, interleukin-6 (IL-6). Hence, blood biomarkers are consistent with the observed mild calcification induced by the two diets. Modifications in circulating blood cells induced by the 0.175% lard diet are compatible with the occurrence of a low-grade inflammatory response.
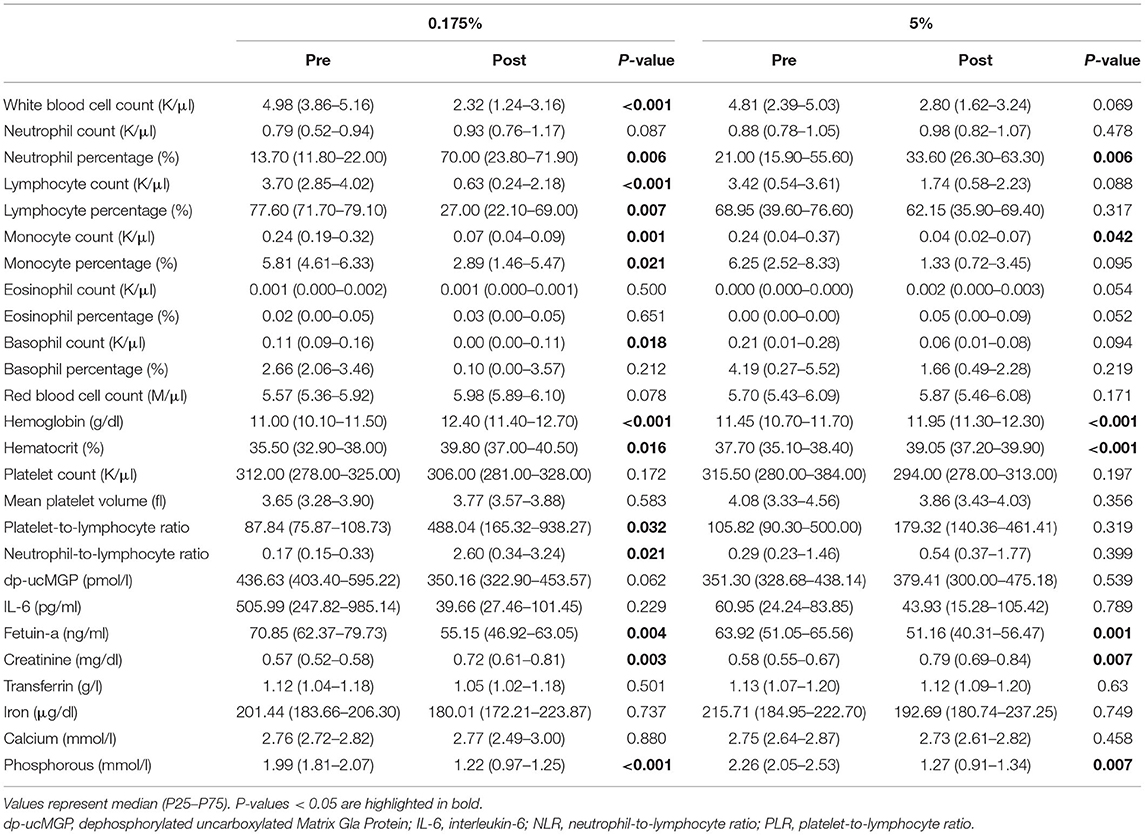
Table 2. Levels of circulating biomarkers measured before (pre) and after (post) 16 weeks of PA or PA/OA-enriched diets.
PA- and PA/OA-Enriched Diets Modify Plasma Levels of PA, OA, SFA, MUFA, and PUFA
Serum levels of conventional lipid parameters, e.g., total cholesterol, LDL, HDL, non-HDL, ApoB, ApoA-I and triglycerides, were not modified after 16 weeks of lard diets as compared to chow diet (Table 3). In order to assess the impact of the PA- and PA/OA-enriched diets on blood lipids and identify lipid profiles that could be associated with the observed vascular and valvular calcifications, we then performed a detailed quantitative lipidomics analysis on rabbit plasma samples. In total, 719 lipid species in 13 lipid classes were detected in the three rabbit groups (Supplementary Table 1). After 16 weeks of 0.175% and 5% lard diet groups, total levels of lipids within the different classes remained mainly unchanged as compared to control rabbits (Figure 2 and Supplementary Table 2). We only observed a decrease of lysophosphatidylcholine (LPC) concentration in the 5% lard diet as compared to chow diet. Nevertheless, we found striking changes in percentages MUFA and PUFA in total lipids and within several lipid classes (Figure 3A and Supplementary Table 2). The 5% lard diet resulted in elevation of total MUFA, whereas a drop in total PUFA percentages occurred in both 0.175% and 5% lard diet groups (23.93 and 21.44 vs. 28.55%, P = 0.002, P < 0.001). Within lipid classes, PUFA content decreased in cholesteryl esters (CE), diacylglycerols (DAG), triglycerides (TAG), in phospholipids, phosphatidylcholine (PC) and phosphatidylethanolamine (PE), and in free fatty acids (FFA) for the 5% lard group, and in PE and TAG for the 0.175% lard group (Supplementary Table 2). An increase of SFA content was observed in CE and TAG in the 5% lard group, whereas DAG, TAG, FFA, LPC, PE, LPE showed elevated content of MUFA. The 0.175% lard diet caused a rise of MUFA content in DAG.
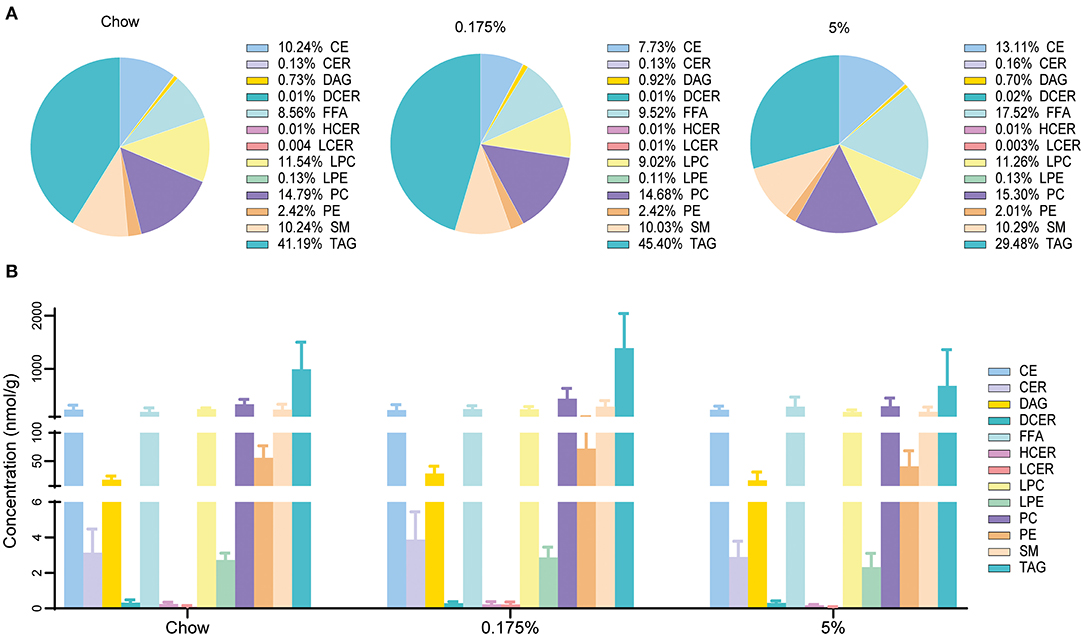
Figure 2. Lipid classes in plasma from rabbits fed chow, 0.175% and 5% lard diet groups. (A). Proportion of lipid classes. (B). Lipid class concentrations. CE, cholesteryl esters; CER, ceramides; DAG, diacylglycerols; DCER, dihydroceramides; FFA, free fatty acids; HCER, hesoxylceramides; LCER, lactosylceramides; LPC, lysophosphatidylcholines; LPE, lysophosphatidylethanolamines; PC, phosphatidylcholines; PE, phosphatidylethanolamines; SM, sphingomyelins; TAG, triacylglycerols.
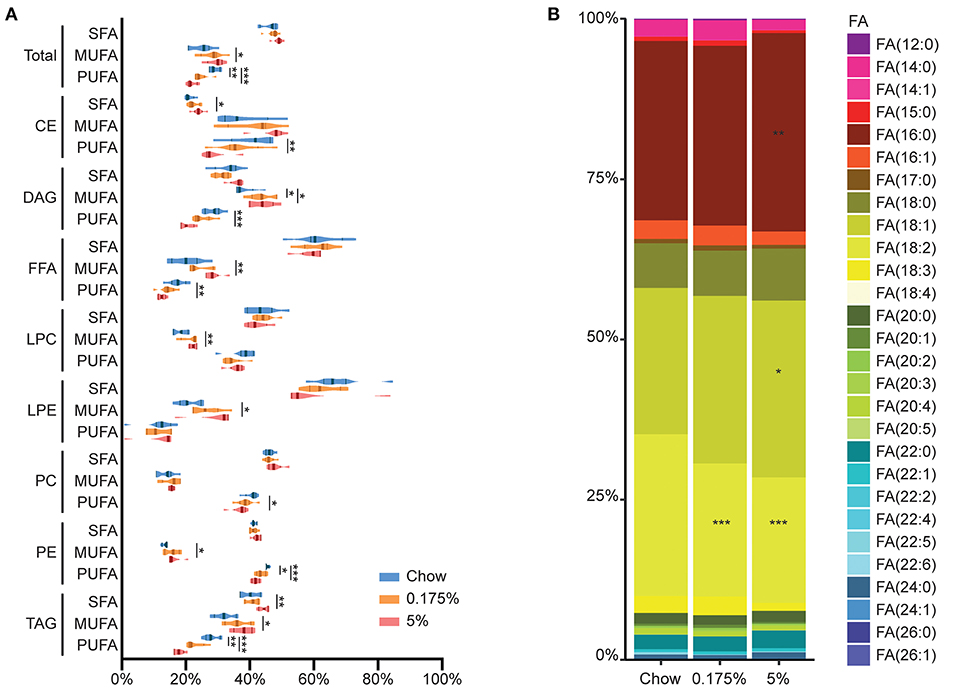
Figure 3. Detailed plasma lipid composition in chow, 0.175% and 5% lard diet groups. (A). Percentages of SFA, MUFA and PUFA in total lipids and within lipid classes. Violin plot shapes represent value distribution within a group, with median shown by thick line and P25-P75 shown by thin lines. (B). Representation of fatty acid percentages in total lipids. *P ≤ 0.05; **P ≤ 0.01; ***P ≤ 0.001 vs. chow.
Regarding the proportions of total fatty acids, we found increased content of total PA and OA in the 5% lard group as compared to chow diet (PA: 30.3 vs. 28.9%, P = 0.005; OA: 27.1 vs. 23.4%, P = 0.018). We also observed decreased linoleic acid content [FA (18:2)] both in the 0.175% and 5% lard groups compared to chow diet (19.6 and 18.9 vs. 24.8%, P < 0.001) (Figure 3B). When studied separately in each class, linoleic acid contents were decreased in CE, DAG and TAG in 5% lard group, and in TAG in the 0.175% lard group (Supplementary Table 2, Supplementary Figure 2).
Next, we calculated the plasma ratios SFA/PUFA, PA/OA, PA/PUFA and OA/PUFA in all three diet groups (Table 4). In total lipids, we observed increased SFA/PUFA ratios in the 0.175% lard group and in the 5% lard group as compared to the chow diet group. PA/PUFA and OA/PUFA ratios were also increased in both 0.175% lard and 5% lard groups. Changes in PA/PUFA ratios were reflected in TAG for the two groups, and in CE, DAG, and FFA for the 5% group. OA/PUFA ratios were increased in DAG, FFA, LPC, PE and TAG for the two groups, and in CE and PC for the 5% group. Of interest, in DAG, we found greatly increased PA/PUFA ratio in the 5% lard group that more particularly concerned PUFA with 18C-long FA chain [1.39 (1.35–1.52) vs. 0.86 (0.78–0.89), P < 0.001]. The ratio of SFA/PUFA with 18C-long FA chain was higher in the 5% lard diet than in chow diet [0.21 (0.17–0.21) vs. 0.12 (0.11–0.14), P < 0.001]. In TAG, a similar increase was observed for SFA/PUFA with 18C-long FA chain [0.24 (0.23–0.26) vs. 0.14 (0.13–0.16), P < 0.001] and PA/PUFA with 18C-long FA chain [2.13 (2.10–2.23) vs. 1.10 (1.06–1.33), P < 0.001].
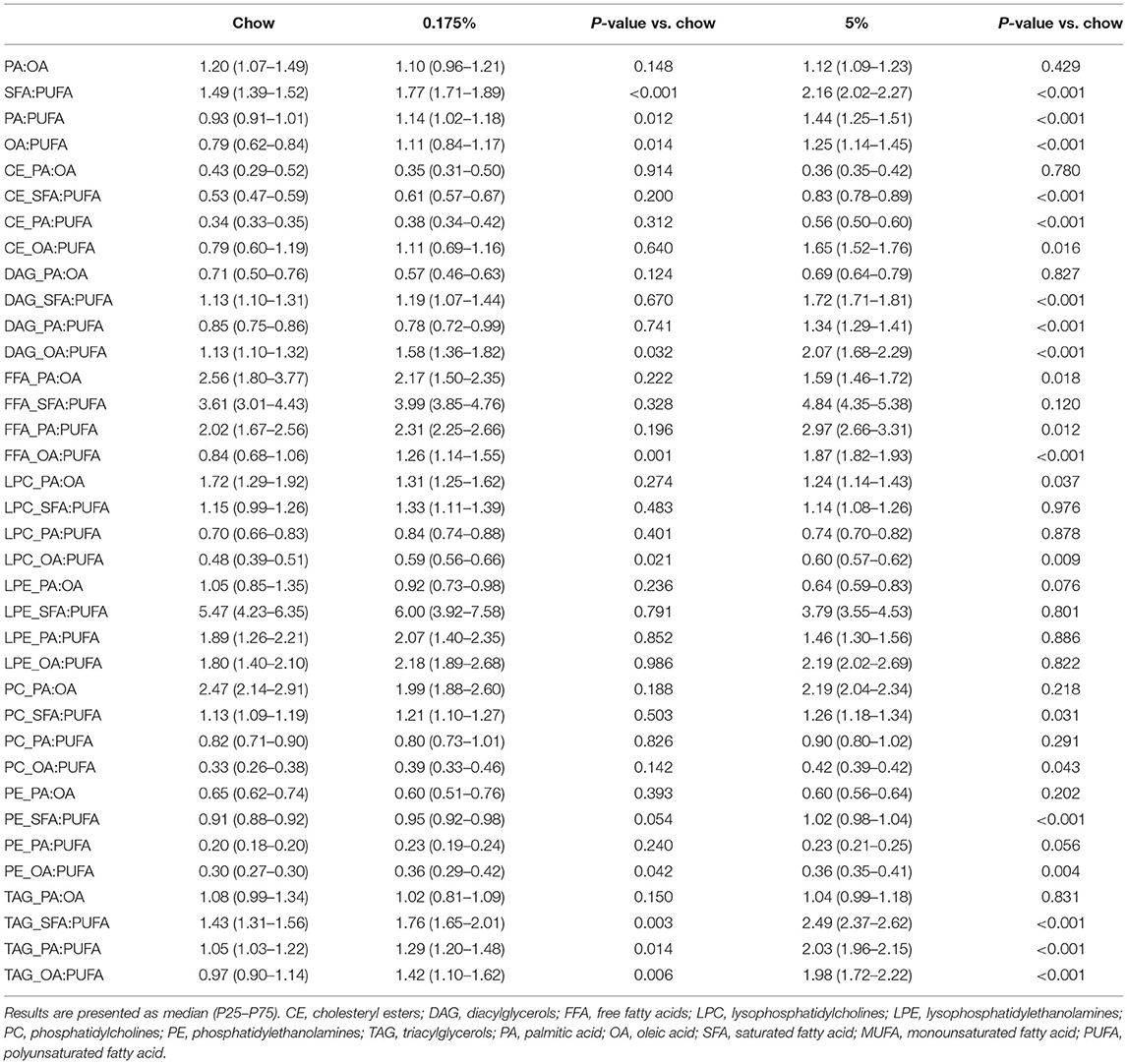
Table 4. Effect of 0.175% lard and 5% lard diets on PA to OA, SFA to PUFA, PA to PUFA and OA to PUFA ratios in total lipids and lipid classes.
Taken together, these data indicate a rise of PA and OA plasma levels upon intake of high levels of these FA. A slight PA enrichment in diet already induces a drop of PUFA, including linoleic acid in major lipid classes. Elevation of plasma SFA and MUFA ratios to PUFA was also observed within several lipid classes in rabbit fed both low and high lard-enriched diets.
Correlations Between Calcification, Lipid Ratios, and Circulating Biomarkers
To get insight into potential mechanisms of diet-induced vascular and valvular calcification, we performed correlation analyses between modified lipids, biomarkers and extent of calcification in aorta and aortic valves. We first searched for correlations between aorta or aortic valve calcification and biomarkers. Among biomarkers that were significantly influenced over time by the diets, we found that the monocyte count was negatively correlated with aortic valve calcification in the 5% lard group (r = −0.89, P = 0.018). We then analyzed the correlations between the extent of calcification and lipids. These analyses revealed several correlations in the 5% lard group. Aortic valve calcification was positively correlated with ratios of PA or SFA to PUFA in DAG and TAG (PA/PUFA in DAG, ρ = 0.83, P = 0.042; SFA/PUFA with 18C-long FA chain in DAG, ρ = 0.89, P = 0.019, and in TAG, ρ = 0.91, P = 0.013; PA/PUFA with 18C-long FA chain FA in DAG, ρ = 0.82, P = 0.047 and in TAG, ρ = 0.84, P = 0.038). Since the drop of monocyte count observed in the 5% lard group correlated with aortic valve calcification, we then wanted to assess the relationship between this biomarker and the identified lipid ratios. Interestingly, negative correlations were observed between monocyte count and PA/PUFA ratio in DAG (r = −0.9, P = 0.014) and PA/PUFA with 18C-long FA chain in DAG (r = −0.87, P = 0.026).
PA content in total lipids and SFA content in CE were positively correlated with aorta calcification (ρ = 0.83, P = 0.042; ρ = 0.89, P = 0.019). Aorta calcification also showed positive correlations with SFA/PUFA ratio in PE (ρ = 0.89, P = 0.019), and OA/PUFA ratio in FFA (ρ = 0.83, P = 0.042). We found that PA content in total lipids positively correlated with the hematocrit (ρ = 0.83, P = 0.042) that was found to be elevated in the 5% lard group.
Discussion
Our study is the first experimental demonstration that regular dietary PA intake causes aorta and aortic valve calcification (Figure 4). Rabbits fed a diet slightly enriched in PA (0.175% of lard) for a so short period as 16 weeks developed microcalcifications both in aorta and aortic valve. Surprisingly, the extent of calcification was similar to that obtained with a higher PA food content and OA enrichment (5% of lard). This might be related to protective effects of OA (23) that might counteract the additive effects of high PA content on the calcification process.
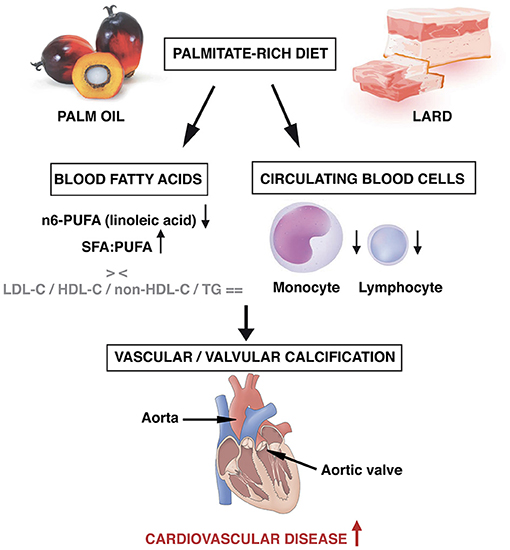
Figure 4. Intake of regular amounts of palmitate, a major dietary saturated fatty acid contained in palm oil and in lard, may promote calcification in aorta and in aortic valve, responsible for an increased burden of cardiovascular disease. In blood, decreased levels of polyunsaturated fatty acids as well as signs of low-grade inflammation may occur, without any changes in traditional cholesterol parameters.
Both diets led to a drop of plasma PUFA within the most abundant lipid classes, in line with reported beneficial effects of PUFA in cardiovascular prevention (24). Of particular interest is the decrease in linoleic acid content in total lipids and in three major lipid classes (CE, TAG, DAG). Indeed, intake of this n-6 PUFA has been associated with a decreased CAD risk (25, 26).
We did not find any modification of traditional cholesterol parameters by the two diets, excluding typical LDL-C or non-HDL-C related effects. Importantly, our findings could, at least partly, explain why a significant part of patients with CVD have normal values of cholesterol parameters (27, 28). Hence, dietary PA intake and subsequent changes in plasma SFA and PUFA might promote CVD independently of traditional risk factors.
In line with this proposition, the Bruneck lipidomic study identified levels of individual species from CE and PE as a molecular signature for CVD risk (3). Also, a recent review of several studies indicated that lipid content in LDL and HDL independent of cholesterol could play a key role as biomarkers of CVD outcomes (29).
Interestingly, we found that the extent of aorta calcification was positively correlated with SFA levels in cholesteryl esters and SFA/PUFA ratio in phosphatidylethanolamines, while SFA and PA to PUFA ratios in DAG and TAG were positively correlated with aortic valve calcification. These data suggest that the high cardiovascular risk that associated with PA could be related to pro-calcifying effects on arteries or aortic valve. Indeed, the extent of intimal vascular calcification that accompanies atherosclerosis progression has been associated with adverse cardiovascular events (30). Likewise, in calcific aortic stenosis, aortic valve calcification predicts disease severity and poor clinical outcomes (31). The study by Torzewski et al. (32) indicates that PA and OA composed the most abundant lysophosphatidic acid species in calcified valve leaflets. Their levels were highly increased in aortic valve leaflets with large calcified nodules, suggesting a possible role for these two fatty acids in aortic valve calcification. Also, in atherosclerosis (33) and in calcific aortic stenosis (34), calcium deposits in tissues at a (sub)micrometer scale (microcalcification) represent early pathological steps. It is therefore tempting to speculate that PA intake might both contribute to disease initiation and to progression.
We found that the 0.175% lard diet induced a drop of lymphocyte and monocyte counts, with concomitant increase of neutrophil percentages and NLR. We did not, however, detect any increase of the inflammatory marker IL-6. This result might indicate that regular PA intake may result in the establishment of a low-grade inflammation, a risk factor for CVD, even at very low LDL-C (35). Several in vitro studies provide evidence for a link between SFA and inflammation, through direct effects on monocytes/macrophages. For instance, long-chain SFA have been shown to induce the expression of pro-inflammatory cytokines in macrophages (36). It has also been reported that SFA promotes the expression of GDF-15 in human macrophages (37), a marker of poor outcome both in CAD (38) and calcific aortic stenosis (39).
The observed drop in monocyte count could be due to higher recruitment of blood monocytes in vascular or valvular tissues and their subsequent differentiation into macrophages, a key event in the initiation of the calcification process (40). The fact that monocyte counts were negatively correlated with both aortic valve calcification and PA/PUFA ratios in DAG might reveal novel mechanistic clues, which warrants further investigations. PA could either promote aortic valve calcification through macrophage activation or differentiation (41) or, indirectly, via a PUFA-lowering effect (42).
We found that PA content in total lipids correlated with aorta calcification. Our observation that lard diets induced a rise of haematocrit that positively correlated with PA content in total lipids would therefore suggest the existence of a link between haematocrit levels and aorta calcification following PA intake. To date, there is no clear evidence for an association between haematocrit and CAD (43–46) although some studies indicate that a rise of hematocrit and subsequent blood viscosity may increase endothelial shear stress and contribute to the initiation and progression of atherosclerosis (47).
Limitations
Our study has some limitations, including low numbers of rabbits, which did not allow us reaching high statistical power. Only male rabbits were included. Also, we did not provide functional demonstration of causal relationship between plasma lipid changes and vascular or valvular calcification. Further investigations are needed to establish the link between PA intake, changes in circulating leukocytes and hematocrit levels and tissue calcification. Also, whether FA, free or in lipids, can serve as sites of calcium precipitation in vascular or valvular tissues remains to be determined. The alizarin red staining enabled us to detect small calcium deposits. Nevertheless, this method did not allow us to visualize crystal shape or structures, which might inform on mineralization mechanisms.
Conclusions
Our study showed that a regular intake of PA-rich lard promotes aorta and aortic valve calcification through plasma lipid modification independent of conventional cholesterol markers. PA is also a main component of palm oil and, as such, it can form a large proportion of total dietary SFA intake. Our study therefore calls for caution when consuming too much of PA-containing food on a regular basis. We propose to consider using both PA and PUFA levels as markers of CV health.
Data Availability Statement
The original contributions presented in the study are included in the article/Supplementary Material, further inquiries can be directed to the corresponding authors.
Ethics Statement
The animal study was reviewed and approved by Animal Ethical Committee of the University of Liège.
Author Contributions
CO, PL, and AN contributed to conception and design of the study. ZJ performed the statistical analysis. ND and CO wrote the manuscript. CD'E, NB, RD, and ND performed the experiments. MG and PD contributed to data interpretation. All authors contributed to manuscript revision, read, and approved the submitted version.
Funding
The project was supported by grants from the Région Wallonne (Projet Complément FEDER BIOMED HUB, convention 1510605) and the Fonds Léon Frédéricq (FLF, Prix de la Province de Liège 2019–2020). Oury C. is Research Director at the National Funds for Scientific Research, Belgium (FRS-FNRS). MG received partial funding from the NOW project 184.034.019.
Conflict of Interest
The authors declare that the research was conducted in the absence of any commercial or financial relationships that could be construed as a potential conflict of interest.
Acknowledgments
The authors thank the GIGA-technological platforms and core facilities (Immunohistochemistry, SPF animal facility and Cell Imaging & Flow Cytometry) for technical support. They also thank MG and the Center for Proteomics and Metabolomics in Leiden University Medical Center for the lipidomic analysis.
Supplementary Material
The Supplementary Material for this article can be found online at: https://www.frontiersin.org/articles/10.3389/fcvm.2021.692184/full#supplementary-material
References
1. Ference BA, Graham I, Tokgozoglu L, Catapano AL. Impact of lipids on cardiovascular health: JACC health promotion series. J Am Coll Cardiol. (2018) 72:1141–56. doi: 10.1016/j.jacc.2018.06.046
2. Afshin A, Sur PJ, Fay KA, Cornaby L, Ferrara G, Salama JS, et al. Health effects of dietary risks in 195 countries, 1990–2017: a systematic analysis for the global burden of disease study 2017. Lancet. (2019) 393:1958–72. doi: 10.1016/S0140-6736(19)30041-8
3. Stegemann C, Pechlaner R, Willeit P, Langley SR, Mangino M, Mayr U, et al. Lipidomics profiling and risk of cardiovascular disease in the prospective population-based bruneck study. Circulation. (2014) 129:1821–31. doi: 10.1161/CIRCULATIONAHA.113.002500
4. DiNicolantonio JJ, O'Keefe JH. Effects of dietary fats on blood lipids: a review of direct comparison trials. Open Hear. (2018) 5:1–5. doi: 10.1136/openhrt-2018-000871
5. Praagman J, Beulens JWJ, Alssema M, Zock PL, Wanders AJ, Sluijs I, et al. The association between dietary saturated fatty acids and ischemic heart disease depends on the type and source of fatty acid in the European prospective investigation into cancer and nutrition-Netherlands cohort. Am J Clin Nutr. (2016) 103:356–65. doi: 10.3945/ajcn.115.122671
6. De Oliveira Otto MC, Mozaffarian D, Kromhout D, Bertoni AG, Sibley CT, Jacobs DR, et al. Erratum: dietary intake of saturated fat by food source and incident cardiovascular disease: the multi-ethnic study of atherosclerosis. Am J Clin Nutr. (2012) 96:397–404. doi: 10.3945/ajcn.112.037770
7. Praagman J, De Jonge EAL, Kiefte-De Jong JC, Beulens JWJ, Sluijs I, Schoufour JD, et al. Dietary saturated fatty acids and coronary heart disease risk in a Dutch middle-aged and elderly population. Arterioscler Thromb Vasc Biol. (2016) 36:2011–8. doi: 10.1161/ATVBAHA.116.307578
8. Jin J, Lu Z, Li Y, Cowart LA, Lopes-Virella MF, Huang Y. Docosahexaenoic acid antagonizes the boosting effect of palmitic acid on lPS inflammatory signaling by inhibiting gene transcription and ceramide synthesis. PLoS ONE. (2018) 13:1–18. doi: 10.1371/journal.pone.0193343
9. Hellmann J, Zhang MJ, Tang Y, Rane M, Bhatnagar A, Spite M. Increased saturated fatty acids in obesity alter resolution of inflammation in part by stimulating prostaglandin production. J Immunol. (2013) 191:1383–92. doi: 10.4049/jimmunol.1203369
10. Wang Y, Qian Y, Fang Q, Zhong P, Li W, Wang L, et al. Saturated palmitic acid induces myocardial inflammatory injuries through direct binding to TLR4 accessory protein MD2. Nat Commun. (2017) 8:13997. doi: 10.1038/ncomms13997
11. Harvey KA, Walker CL, Pavlina TM, Xu Z, Zaloga GP, Siddiqui RA. Long-chain saturated fatty acids induce pro-inflammatory responses and impact endothelial cell growth. Clin Nutr. (2010) 29:492–500. doi: 10.1016/j.clnu.2009.10.008
12. Kageyama A, Matsui H, Ohta M, Sambuichi K, Kawano H, Notsu T, et al. Palmitic acid induces osteoblastic differentiation in vascular smooth muscle cells through ACSL3 and NF-κB, novel targets of eicosapentaenoic acid. PLoS ONE. (2013) 8:e68197. doi: 10.1371/journal.pone.0068197
13. Brodeur MR, Bouvet C, Barrette M, Moreau P. Palmitic acid increases medial calcification by inducing oxidative stress. J Vasc Res. (2013) 50:430–41. doi: 10.1159/000354235
14. Zong G, Li Y, Sampson L, Dougherty LW, Willett WC, Wanders AJ, et al. Monounsaturated fats from plant and animal sources in relation to risk of coronary heart disease among US men and women. Am J Clin Nutr. (2018) 107:445–53. doi: 10.1093/ajcn/nqx004
15. Steffen BT, Duprez D, Szklo M, Guan W, Tsai MY. Circulating oleic acid levels are related to greater risks of cardiovascular events and all cause mortality: the multi-ethnic study of atherosclerosis. J Clin Lipidol. (2018) 12:1404–12. doi: 10.1016/j.jacl.2018.08.004
16. Ma J, Folsom AR, Shahar E, Eckfeldt JH. Plasma fatty acid composition as an indicator of habitual dietary fat intake in middle-aged adults. Am J Clin Nutr. (1995) 62:564–71. doi: 10.1093/ajcn/62.3.564
17. Bankhead P, Loughrey MB, Fernández JA, Dombrowski Y, McArt DG, Dunne PD, et al. QuPath: open source software for digital pathology image analysis. Sci Rep. (2017) 7:1–7. doi: 10.1038/s41598-017-17204-5
18. Ubhi BK. Direct infusion-tandem mass spectrometry (DI-MS/MS) analysis of complex lipids in human plasma and serum using the lipidyzerTM platform. Methods Mol Biol. (2018) 1730:227–36. doi: 10.1007/978-1-4939-7592-1_15
19. Alarcon-Barrera JC, von Hegedus JH, Brouwers H, Steenvoorden E, Ioan-Facsinay A, Mayboroda OA, et al. Lipid metabolism of leukocytes in the unstimulated and activated states. Anal Bioanal Chem. (2020) 412:2353–63. doi: 10.1007/s00216-020-02460-8
20. Contrepois K, Mahmoudi S, Ubhi BK, Papsdorf K, Hornburg D, Brunet A, et al. Cross-platform comparison of untargeted and targeted lipidomics approaches on aging mouse plasma. Sci Rep. (2018) 8:1–9. doi: 10.1038/s41598-018-35807-4
21. Cao Z, Schmitt TC, Varma V, Sloper D, Beger RD, Sun J. Evaluation of the performance of lipidyzer platform and its application in the lipidomics analysis in mouse heart and liver. J Proteome Res. (2020) 19:2742–9. doi: 10.1021/acs.jproteome.9b00289
22. Lintonen TPI, Baker PRS, Suoniemi M, Ubhi BK, Koistinen KM, Duchoslav E, et al. Differential mobility spectrometry-driven shotgun lipidomics. Anal Chem. (2014) 86:9662–9. doi: 10.1021/ac5021744
23. Piccinin E, Cariello M, De Santis S, Ducheix S, Sabbà C, Ntambi JM, et al. Role of oleic acid in the gut-liver axis: from diet to the regulation of its synthesis via stearoyl-CoA desaturase 1 (SCD1). Nutrients. (2019) 11:1–22. doi: 10.3390/nu11102283
24. Bhatt DL, Steg PG, Miller M, Brinton EA, Jacobson TA, Ketchum SB, et al. Cardiovascular risk reduction with icosapent ethyl for hypertriglyceridemia. N Engl J Med. (2019) 380:11–22. doi: 10.1056/NEJMoa1812792
25. Farvid MS, Ding M, Pan A, Sun Q, Chiuve SE, Steffen LM, et al. Dietary linoleic acid and risk of coronary heart disease: a systematic review and meta-analysis of prospective cohort studies. Circulation. (2014) 130:1568–78. doi: 10.1161/CIRCULATIONAHA.114.010236
26. Yang WS, Chen YY, Chen PC, Hsu HC, Su TC, Lin HJ, et al. Association between plasma n-6 polyunsaturated fatty acids levels and the risk of cardiovascular disease in a community-based cohort study. Sci Rep. (2019) 9:1–9. doi: 10.1038/s41598-019-55686-7
27. Fernández-Friera L, Fuster V, López-Melgar B, Oliva B, García-Ruiz JM, Mendiguren J, et al. Normal lDL-cholesterol levels are associated with subclinical atherosclerosis in the absence of risk factors. J Am Coll Cardiol. (2017) 70:2979–91. doi: 10.1016/j.jacc.2017.10.024
28. Sachdeva A, Cannon CP, Deedwania PC, LaBresh KA, Smith SC, Dai D, et al. Lipid levels in patients hospitalized with coronary artery disease: an analysis of 136,905 hospitalizations in get with the guidelines. Am Heart J. (2009) 157:111–7.e2. doi: 10.1016/j.ahj.2008.08.010
29. Ding M, Rexrode KM. A review of lipidomics of cardiovascular disease highlights the importance of isolating lipoproteins. Metabolites. (2020) 10:1–13. doi: 10.3390/metabo10040163
30. Mitchell JD, Paisley R, Moon P, Novak E, Villines TC. Coronary artery calcium and long-term risk of death, myocardial infarction, and stroke: the walter reed cohort study. JACC Cardiovasc Imaging. (2018) 11:1799–806. doi: 10.1016/j.jcmg.2017.09.003
31. Clavel MA, Pibarot P, Messika-Zeitoun D, Capoulade R, Malouf J, Aggarval S, et al. Impact of aortic valve calcification, as measured by MDCT, on survival in patients with aortic stenosis: results of an international registry study. J Am Coll Cardiol. (2014) 64:1202–13. doi: 10.1016/j.jacc.2014.05.066
32. Torzewski M, Ravandi A, Yeang C, Edel A, Bhindi R, Kath S, et al. Lipoprotein(a)-associated molecules are prominent components in plasma and valve leaflets in calcific aortic valve stenosis. JACC Basic Transl Sci. (2017) 2:229–40. doi: 10.1016/j.jacbts.2017.02.004
33. Roijers RB, Debernardi N, Cleutjens JPM, Schurgers LJ, Mutsaers PHA, Van Der Vusse GJ. Microcalcifications in early intimal lesions of atherosclerotic human coronary arteries. Am J Pathol. (2011) 178:2879–87. doi: 10.1016/j.ajpath.2011.02.004
34. Lindman BR, Clavel M-A, Mathieu P, Iung B, Lancellotti P, Otto CM, et al. Calcific aortic stenosis. Nat Rev Dis Prim. (2016) 2:16006. doi: 10.1038/nrdp.2016.6
35. Koenig W. Low-grade inflammation modifies cardiovascular risk even at very low lDL-C levels are we aiming for a dual target concept? Circulation. (2018) 138:150–3. doi: 10.1161/CIRCULATIONAHA.118.035107
36. Håversen L, Danielsson KN, Fogelstrand L, Wiklund O. Induction of proinflammatory cytokines by long-chain saturated fatty acids in human macrophages. Atherosclerosis. (2009) 202:382–93. doi: 10.1016/j.atherosclerosis.2008.05.033
37. L'Homme L, Sermikli BP, Staels B, Piette J, Legrand-Poels S, Dombrowicz D. Saturated fatty acids promote GDF15 expression in human macrophages through the PERK/eIF2/CHOP signaling pathway. Nutrients. (2020) 12:3771. doi: 10.3390/nu12123771
38. Wang X, Chen LL, Zhang Q. Increased serum level of growth differentiation factor 15 (GDF-15) is associated with coronary artery disease. Cardiovasc Ther. (2016) 34:138–43. doi: 10.1111/1755-5922.12184
39. Kim JB, Kobayashi Y, Moneghetti KJ, Brenner DA, O'Malley R, Schnittger I, et al. GDF-15 (growth differentiation factor 15) is associated with lack of ventricular recovery and mortality after transcatheter aortic valve replacement. Circ Cardiovasc Interv. (2017) 10:1–9. doi: 10.1161/CIRCINTERVENTIONS.117.005594
40. Li Y, Sun Z, Zhang L, Yan J, Shao C, Jing L, et al. Role of macrophages in the progression and regression of vascular calcification. Front Pharmacol. (2020) 11:661. doi: 10.3389/fphar.2020.00661
41. Davanso MR, Crisma AR, Murata G, Newsholme P, Curi R. Impact of dietary fatty acids on macrophage lipid metabolism, signaling and function. Immunometabolism. (2020) 2:1–41. doi: 10.20900/immunometab20200008
42. Artiach G, Carracedo M, Plunde O, Wheelock CE, Thul S, Sjövall P, et al. Omega-3 polyunsaturated fatty acids decrease aortic valve disease through the resolvin E1 and chemR23 axis. Circulation. (2020) 142:776–789. doi: 10.1161/CIRCULATIONAHA.119.041868
43. Irace C, Ciamei M, Crivaro A, Fiaschi E, Madia A, Cortese C, et al. Hematocrit is associated with carotid atherosclerosis in men but not in women. Coron Artery Dis. (2003) 14:279–84. doi: 10.1097/01.mca.0000071769.74379.49
44. Carallo C, Pujia A, Irace C, De Franceschi MS, Motti C, Gnasso A. Whole blood viscosity and haematocrit are associated with internal carotid atherosclerosis in men. Coron Artery Dis. (1998) 9:113–7. doi: 10.1097/00019501-199802000-00008
45. Gagnon DR, Zhang TJ, Brand FN, Kannel WB. Hematocrit and the risk of cardiovascular disease-the framingham study: a 34-year follow-up. Am Heart J. (1994) 127:674–82. doi: 10.1016/0002-8703(94)90679-3
46. Danesh J, Collins R, Peto R, Lowe GDO. Haematocrit, viscosity, erythrocyte sedimentation rate: meta-analyses of prospective studies of coronary heart disease. Eur Heart J. (2000) 21:515–20. doi: 10.1053/euhj.1999.1699
Keywords: palmitate, nutrition, blood fatty acids, vascular calcification, aortic valve calcification
Citation: Donis N, Jiang Z, D'Emal C, Dulgheru R, Giera M, Blomberg N, Delvenne P, Nchimi A, Lancellotti P and Oury C (2021) Regular Dietary Intake of Palmitate Causes Vascular and Valvular Calcification in a Rabbit Model. Front. Cardiovasc. Med. 8:692184. doi: 10.3389/fcvm.2021.692184
Received: 07 April 2021; Accepted: 24 May 2021;
Published: 23 June 2021.
Edited by:
Vasily Sukhorukov, Research Institute of Human Morphology, RussiaReviewed by:
Sergio Bertazzo, University College London, United KingdomJose Rodríguez-Morató, Mar Institute of Medical Research (IMIM), Spain
Copyright © 2021 Donis, Jiang, D'Emal, Dulgheru, Giera, Blomberg, Delvenne, Nchimi, Lancellotti and Oury. This is an open-access article distributed under the terms of the Creative Commons Attribution License (CC BY). The use, distribution or reproduction in other forums is permitted, provided the original author(s) and the copyright owner(s) are credited and that the original publication in this journal is cited, in accordance with accepted academic practice. No use, distribution or reproduction is permitted which does not comply with these terms.
*Correspondence: Patrizio Lancellotti, cGxhbmNlbGxvdHRpQGNodWxpZWdlLmJl; Cécile Oury, Y2VjaWxlLm91cnlAdWxpZWdlLmJl
†These authors have contributed equally to this work and share last authorship