- Department of Cardiology, Leiden University Medical Center, Leiden, Netherlands
Aims: Major adverse event (MAE) rates during left ventricular assist device (LVAD) therapy in advanced heart failure (HF) patients are high, and impair quality of life and survival. Prediction and risk stratification of MAEs in order to improve patient selection and thereby outcome during LVAD therapy is therefore warranted. Circulating natriuretic peptides (NPs) are strong predictors of MAEs and mortality in chronic HF patients. However, whether NPs can identify patients who are at risk of MAEs and mortality or tend toward myocardial recovery after LVAD implantation is unclear. The aim of this systematic review is to analyze the prognostic value of circulating NP levels before LVAD implantation for all-cause mortality, MAEs and myocardial recovery after LVAD implantation.
Methods and Results: Electronic databases were searched for studies analyzing circulating NP in adults with advanced HF before LVAD implantation in relation to mortality, MAEs, or myocardial recovery after LVAD implantation. Twenty-four studies published between 2008 and 2021 were included. Follow-up duration ranged from 48 hours to 5 years. Study sample size ranged from 14 to 15,138 patients. Natriuretic peptide levels were not predictive of all-cause mortality. However, NPs were predictive of right ventricular failure (RVF) and MAEs such as ventricular arrhythmias, moderate or severe aortic regurgitation, and all-cause rehospitalization. No relation between NPs and myocardial recovery was found.
Conclusion: This systematic review found that NP levels before LVAD implantation are not predictive of all-cause mortality after LVAD implantation. Thus, NP levels may be of limited value in patient selection for LVAD therapy. However, NPs help in risk stratification of MAEs and may be used to identify patients who are at risk for RVF, ventricular arrhythmias, moderate or severe aortic regurgitation, and all-cause rehospitalization after LVAD implantation.
Introduction
The prognosis of advanced heart failure (HF) is poor, with annual mortality rates over 50%, and limited treatment options (1). Cardiac transplantation is the most effective treatment, although its availability is limited due to insufficient number of donor organs and strict eligibility criteria. Left ventricular assist devices (LVADs) are an alternative treatment option through mechanical unloading of the failing left ventricle (LV). To date, LVAD therapy is increasingly used as destination therapy in patients not eligible for transplantation. Patient selection and timing of LVAD implantation is guided by the profiles of the Interagency Registry for Mechanically Assisted Circulatory Support (INTERMACS) classifying patients with advanced heart failure (2).
The most recent INTERMACS report has shown a 1-year survival rate of 79–80% in patients receiving continuous flow (CF)-LVAD therapy (3, 4). Major adverse event (MAE) and rehospitalization rates are high, and impair quality of life and survival (4, 5). These MAEs include neurologic event (defined as stroke or transient ischemic attack), gastrointestinal bleeding, major infection, and right heart failure (RVF) occurring 13–20, 20–25, 40–43, and 29–38% at 1 year after CF-LVAD implantation, respectively (4). All-cause rehospitalization rates were 21–23% (4). Device explantation for LV myocardial recovery is rare with 3.1% at 3 years, and <5% at 5 years follow-up (3, 6). It would be beneficial to identify patients prone to myocardial recovery, and consider adjustments of their pharmacological treatment (7). To identify patients at risk of MAEs and early mortality would be of great importance. Treatment options for MAEs are limited and often ineffective, having corresponding high mortality rates. Thus, prediction and risk stratification of MAEs before LVAD implantation is warranted in order to improve patient selection, and thereby outcome of LVAD therapy. Measurement of circulating biomarkers such as natriuretic peptides (NPs) may help in risk stratification.
Three subtypes of NP are known; atrial natriuretic peptide (ANP), B-type natriuretic peptide (BNP), and C-type natriuretic peptide (CNP) (8, 9). Natriuretic peptides mainly reflect the hemodynamic burden of the failing heart, and are regulated by volume overload and neuro-hormonal stimulation. Prehormone pro-BNP is released by cardiomyocytes in reaction to mechanical stretch and myocardial ischemia. Upon secretion into the circulation it is cleaved in biologically active BNP and its inactive remnant N-terminal proBNP (NT-proBNP) (Figure 1) (8–12). Levels of NP are influenced by various factors including age, gender, comorbidities, renal function, pulmonary disease, and obesity (13–15). Heart failure medication, including beta-blockers, diuretics, and inotropes affect NP levels, reflecting the improvement in hemodynamic state induced by these therapies. The novel HF drug sacubitril/valsartan influences BNP and NT-proBNP levels differently, in particular during the first 8–10 weeks after initiation. Whereas, the use of sacubitril/valsartan, a neprilysin inhibitor, may increase the circulating levels of BNP, it does not affect the circulating levels of NT-proBNP since the latter is not a substrate of neprilysin inhibition. Nonetheless, both BNP and NT-proBNP have prognostic value during treatment with sacubitril/valsartan (8, 16). Finally, it has been demonstrated that a large percentage of measured circulating BNP or NT-proBNP is in fact their prehormone proBNP. Therefore, BNP, NT-proBNP, and proBNP measurements from different assays are not reliably comparable due to their differences in cross-reactivity (17, 18).
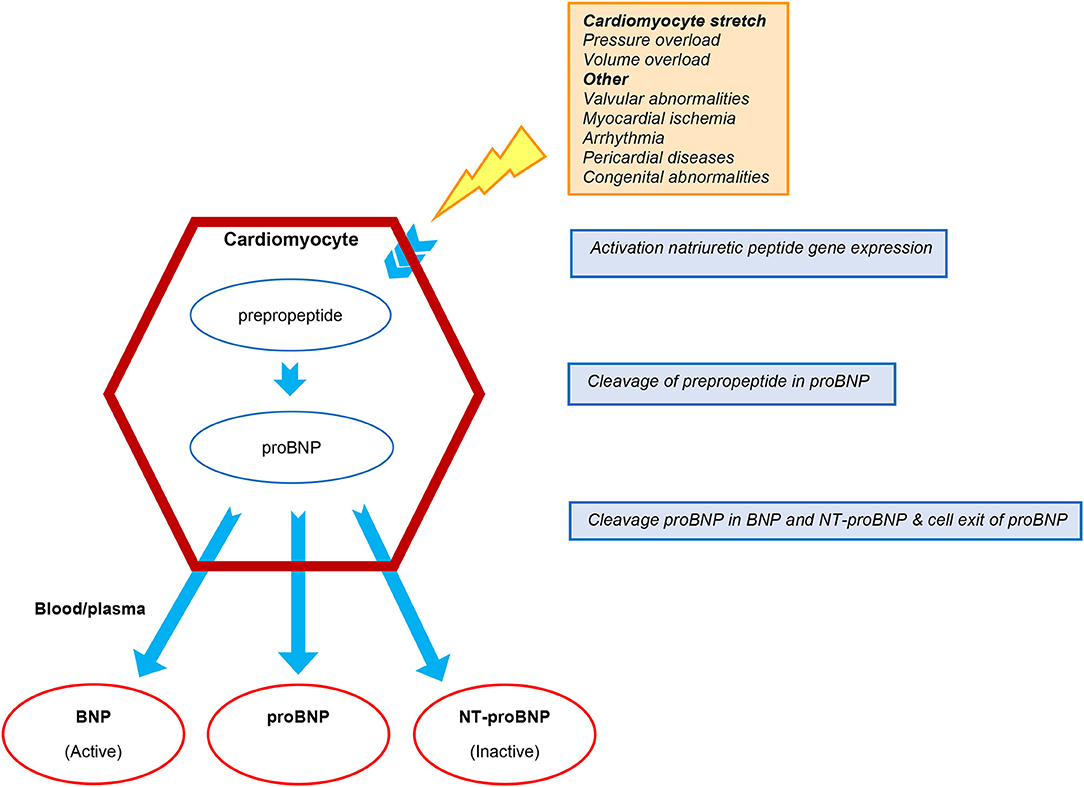
Figure 1. Production and cleavage of proBNP. BNP, B-type natriuretic peptide; NT-proBNP, N-terminal pro-BNP.
In the American College of Cardiology/American Heart Association guideline, BNP and NT-proBNP have a class IA recommendation to establish disease severity and prognosis of patients with chronic HF (2). Hutfless et al. showed that preoperative BNP levels are strong predictors of postoperative need for intra-aortic balloon pump, longer postoperative hospital stay, and higher 1-year mortality in patients undergoing open heart surgery (19). Furthermore, the prognostic value of NP levels related to all-cause mortality, adverse events, and rehospitalization in chronic HF patients has been well-established (20–24).
Whether preoperative NP levels can improve patient selection for LVAD therapy by identifying patients who are at risk for early all-cause mortality, right ventricular failure (RVF), or MAEs, and can identify patients who tend toward myocardial recovery after LVAD implantation, is not yet systematically evaluated. In this review, we sought to systematically evaluate the prognostic value of circulating NP levels in advanced HF patients before LVAD implantation for all-cause mortality, RVF, MAEs including rehospitalization, and myocardial recovery after successful LVAD implantation.
Methods
This systematic review is written in accordance with the Preferred Reporting Items for Systematic Reviews and Meta-analyses (PRISMA) guideline (Supplementary Material 1). Since individual patient data are not included, institutional review board approval was not required.
Literature Search and Selection
Seven electronic databases were searched: MEDLINE, Web of Science Core Collection, Cochrane Reviews, Cochrane Trials, PubMed, Factiva, and Embase. The following (MeSH) terms were used: “left ventricular assist device,” “ventricular assist device,” “mechanical circulatory support,” “biomarkers,” “natriuretic peptide,” “B-type natriuretic peptide,” “brain natriuretic peptide,” “pro B-type natriuretic peptide,” “pro brain natriuretic peptide,” “NT-pro B-type natriuretic peptide,” “N-terminal pro B-type natriuretic peptide,” and “N-terminal pro brain natriuretic peptide.” The search was restricted to human studies published in English up to January 1st, 2021. Study selection criteria were predefined as described in Table 1.
The authors of this manuscript screened the titles and abstracts of all studies retrieved from the literature search. Potentially relevant studies, or studies whose relevance could not be ascertained based on the abstract, were screened full text. A single assessor screened each article full text for inclusion. Corresponding authors were contacted to obtain full data not covered in the publication.
Data Collection
Extracted data included details of the patient population, etiology of HF, type of VAD, device strategy, timing of blood sampling for NPs measurement, type of NPs, cut-off points of NPs (when available), type of statistical analysis, adjusted variables for multivariate analysis, and duration of follow-up. The outcomes all-cause mortality, RVF, MAEs (including all-cause planned and unplanned rehospitalization) and myocardial recovery, and their describing definitions were extracted. Major adverse events were defined according to the “2020 Updated definitions of adverse events for trials and registries of mechanical circulatory support” (25). In the current review, the following MAEs were included: ventricular arrhythmia (VA), aortic regurgitation (AR), “combined adverse events” (including episode of VA, HF, chest pain, bleeding, infection, thrombosis, pump-related problems, biliary disfunction, elective procedures), complicated postoperative stay, and all-cause rehospitalization.
Study Quality
The Newcastle–Ottawa Scale (NOS) for observational cohorts was used to assess the quality of all included studies (26). The NOS score was then converted into Agency for Healthcare Research and Quality standards (AHRQ); good, fair, and poor (27).
Results
Literature Search
The literature search and selection process is presented in the PRISMA flow diagram in Figure 2. The literature search retrieved 1,321 citations from the seven electronic databases. After duplicates were removed, 769 citations went through title and abstract screening, of which 351 articles were screened full text. A total of 745 citations were excluded; the majority did not meet the inclusion criteria regarding advanced HF, reporting any kind of endpoint, receiving LVAD therapy, or measurement of circulating NPs prior to device implantation. Eventually, 24 articles passed full text screening and were included in this review.
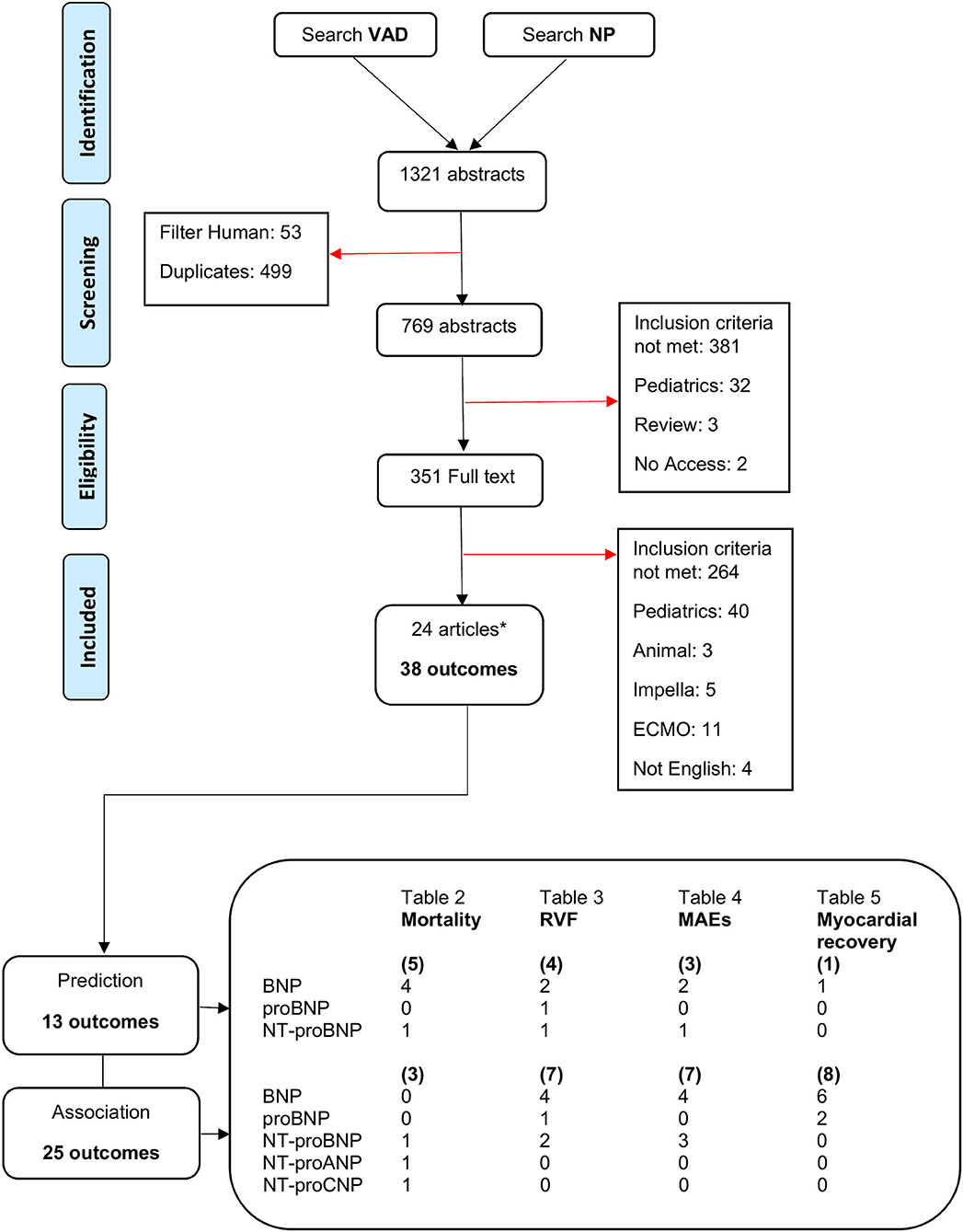
Figure 2. PRISMA flow diagram for literature search and study selection process. *Several articles contain multiple circulating NP or outcomes. ANP, atrial natriuretic peptide; BNP, B-type natriuretic peptide; CNP, C-type natriuretic peptide; ECMO, extracorporeal membrane oxygenation; MAEs, major adverse events; NP, natriuretic peptide; NT-proBNP, N-terminal pro-BNP; RVF, right ventricular failure; VAD, ventricular assist device.
Study Characteristics
The included studies were published between 2008 and 2020, and were from countries in Europe, the United States of America, and Japan. Twenty-three of the included studies were retrospective cohort studies. The 24 studies were fairly heterogenous reporting on multiple subtypes of NP, and various and multiple outcomes. This resulted in a total of 38 outcomes in all studies, where predictive relations were studied in 13 and associative relations in 25 (Figure 2). Follow-up duration ranged from 48 hours up to 5 years after LVAD implantation. Study sample sizes ranged from 14 to 15,138 patients. Natriuretic peptides were extracted from various materials (blood or plasma), measured with different assays, and presented in diverse measuring units. The upper cut off levels for normal NP levels varied from one study to the other. It should be pointed out that NP levels, unless log transformed, are non-normal distributed. Nevertheless, several studies included in this review chose to report NP levels non-log transformed. Descriptive statistic, mean ± standard deviation (SD), was used for log transformed NP measurements. Median and (interquartile) range was used for non-log transformed NP measurements. Groups were compared using various analyses depending on how continuous variables were expressed and how many groups were compared. Univariate and multivariate Cox regression was used for survival analyses expressed in hazard ratio. Univariate and multivariate logistic regression was used to estimate the strength of the effect of NPs expressed in odds ratio. The quality assessed by the NOS was “good” in all studies (Supplementary Material 2).
Study Results
The 24 studies assessing the relation between NP levels before LVAD implantation and all-cause mortality, RVF, MAEs, and myocardial recovery after implantation are summarized in Tables 2–5.
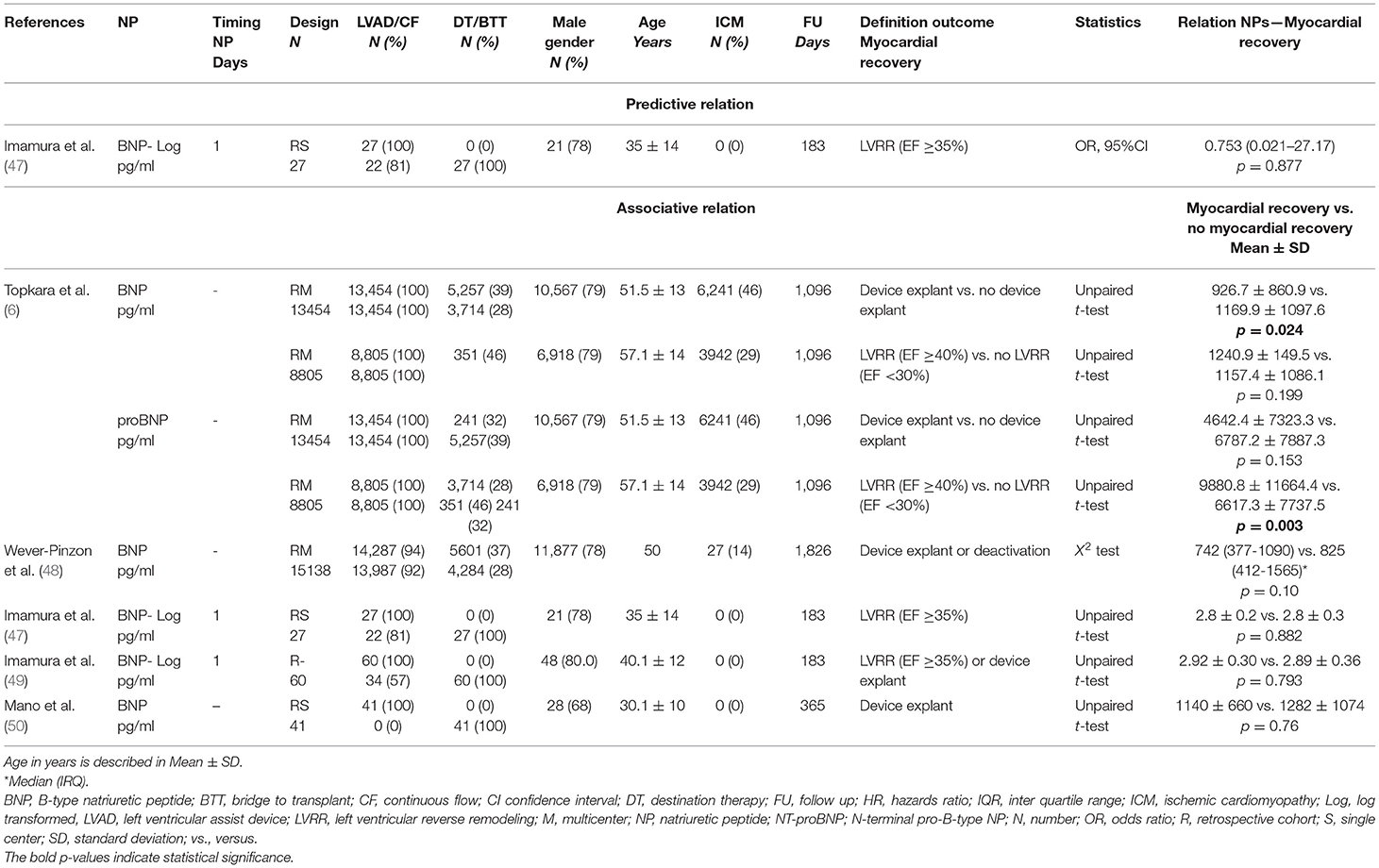
Table 5. Baseline characteristics and outcomes of included studies for left ventricular (LV) myocardial recovery.
All-Cause Mortality
Five studies analyzed the predictive value of NPs for all-cause mortality, of which 4 studies included BNP and 1 study included NT-proBNP (Table 2). None of the studies found BNP levels before LVAD implantation predictive of all-cause mortality (28–31). In contrast, NT-proBNP levels before LVAD implantation were predictive of 30-days all-cause mortality (32). The study by Cabiati et al. looked at an associative relation and found that NT-proBNP was associated with 4-weeks all-cause mortality, while NT-proANP and NT-proCNP were not (33).
Right Ventricular Failure
Four studies assessed the predictive value of NPs for RVF (two studies BNP, one study proBNP, and one study NT-proBNP) (Table 3) (34–37). All studies had at least the outcome “need for right ventricular assist device (RVAD).” Study sample size was 54–79, with the exception of the study by Loghmanpour et al. with a large study population of N = 10,909 (34–37). The two studies analyzing BNP demonstrated that BNP levels before LVAD implantation were predictive of the need of RVAD postoperatively up to 14 days (34, 35). In the study of Shiga et al. it was demonstrated that BNP levels ≥1,200 pg/ml were not predictive of RVF, while in the study by Kato et al. BNP levels ≥1,232 ng/ml were an independent predictor of RVF after 2–14 days (34, 35). In a Bayesian prediction model, proBNP levels had high predictive value for RVF in 2–14 days after LVAD implantation (36). NT-proBNP levels before LVAD implantation were not predictive of RVF within 48 hours post-operatively (37). Importantly, this study by Potapov et al. used a cut-off value of NT-proBNP >10,000 pg/ml (37). Of the seven studies analyzing an associative relation, four analyzed BNP, one analyzed proBNP, and two analyzed NT-proBNP (34, 35, 37–41). Shiga et al. found that BNP levels were associated with “need for RVAD” within the postoperative period (34). Both Hennig et al. and Potapov et al. found that NT-proBNP was associated with RVF within 48 hours postoperatively (37, 41). The remaining studies did not find an association between NP levels and RVF, although in some studies results were close to statistical significance (35, 38–40).
Major Adverse Events
A total of five studies assessed the predictive or associative relation between NPs and MAEs. The following MAEs were identified in these studies: VA, AR, “combined adverse events” (including episode of VA, HF, chest pain, bleeding, infection, thrombosis, pump-related problems, biliary disfunction, elective procedures), complicated postoperative stay, and all-cause rehospitalization. Three studies analyzed whether NPs were predictive of MAEs, of which two studies assessed BNP and one study assessed NT-proBNP (Table 4). All studies found that BNP and NT-proBNP levels before LVAD implantation were predictive of MAEs (42–44). Hellman et al. demonstrated that BNP was an independent predictor for VA within 15 days post-operative (43). In a large study by Truby et al. BNP >500 ng/l was predictive of the development of moderate or severe AR (42). NT-proBNP measured at “hospital admission” before LVAD implantation was an independent predictor for rehospitalization due to cardiac, bleeding, infection, thrombosis, pump related, biliary, or “elective” events (44). Of the studies reporting on associative relations, three studies analyzed BNP levels and two studies analyzed NT-proBNP levels (42, 44–46). In these studies, BNP levels before LVAD implantation were associated with MAEs between 2 weeks up to 2 years (42, 43, 45). In a sub-analysis, Hegarova et al. demonstrated that although BNP was associated with adverse events up to 1.5 years after initial discharge, it was not associated with subsequent rehospitalizations (45). The two studies analyzing NT-proBNP levels found that it was not associated with complicated post-operative stay. However, it was associated with less rehospitalization for combined adverse events (44, 46).
Myocardial Recovery
Only one study assessed the predictive value of NP for myocardial recovery (Table 5). This study found that BNP levels before LVAD implantation were not predictive of LV recovery after 6 months (47). Five studies reported on associative relations between NP and myocardial recovery. All studies analyzed BNP levels, whereas Topkara et al. additionally investigated proBNP levels. Besides the large study by Topkara et al. none of the included studies found an association between BNP and LV recovery (6, 47–50).
Discussion
To the best of our knowledge, this is the first systematic review assessing the prognostic value of circulating NP levels in advanced HF patients receiving LVAD therapy. The main findings are as follows:
1. B-type natriuretic peptide is not predictive of all-cause mortality at a follow-up of 3 months or longer. Evidence regarding NT-proBNP is insufficient to draw a reliable conclusion.
2. B-type natriuretic peptide is predictive of RVF in the postoperative period after the first 48 hours. In contrast, NT-proBNP seems associated with RVF within 48 hours after LVAD implantation.
3. B-type natriuretic peptide and NT-proBNP levels appear to be predictive of various MAEs, and related to rehospitalization up to 1.5 years after LVAD implantation.
4. B-type natriuretic peptide is not predictive of, and most likely not associated with, myocardial recovery.
All-Cause Mortality
None of the studies found that BNP levels before LVAD implantation are predictive of all-cause mortality up to 2 years after implantation. In contrast, Topilsky et al. demonstrated that preoperative NT-proBNP levels are predictive of 1-month mortality after LVAD implantation (32). The study by Papathanasiou et al. analyzing BNP, had a similar study sample size and baseline characteristics compared to the study by Topilsky et al., but did not report a significant predictive relation (28). The differences in type of NPs and length of follow-up may have contributed to this contradictory finding. The follow-up duration may be an important factor, as both studies analyzing 1-month mortality found a significant relation (32, 33). This may suggest that NPs are related to early postoperative mortality, but lose their prognostic value for all-cause mortality at longer follow-up. Of note, both studies analyzed NT-proBNP, and to date no studies are available analyzing BNP levels in relation to 1-month mortality after LVAD implantation. Whether BNP and NT-proBNP have different prognostic power regarding all-cause mortality after LVAD implantation needs to be investigated in future prospective studies.
In the studies included in this review, BNP levels are not predictive of all-cause mortality after LVAD implantation. This is an interesting finding, since NPs (including BNP and NT-proBNP) are strong predictors of all-cause mortality in HF patients (2, 20–22). In addition, BNP levels are independent predictors of mortality in advanced HF patients receiving cardiac resynchronization defibrillator (CRT-D) therapy (51, 52). It may well be that NPs are not so much a predictor of mortality risk after LVAD implantation, but rather a reflection of disease severity. Furthermore, the change in prognostic value of NPs may be caused by several mechanisms related to the LVAD itself. The device unloads the LV, thereby reducing pressure and stretch of cardiomyocytes. Reduced myocardial stretch may lead to lower NP levels. Decreased NP levels are related to a lower mortality risk (53). In parallel with the improvements in hemodynamics and prognosis provided by the LVAD, NT-proBNP levels decrease after LVAD implantation (27, 49). However, they remain abnormal and elevated compared to the levels in chronic HF patients, suggesting that key pathological changes on cellular myocardial level remain, despite LVAD support (49). This may partly be explained by the fact that the flow mechanisms of the devices, including lack of pulsatility and high rotation speed of the LVAD disc or propeller, influence several physiological processes connected to NPs, like neurohormonal changes and sympathetic and renin-angiotensin-aldosterone activity (27, 49, 51). These processes may result in altered NP release. Therefore, the prognostic value of NP levels before LVAD implantation may be changed by the therapy itself. Nonetheless, several studies have shown that NP measurements and their fluctuations during LVAD therapy are strongly related to adverse outcome including mortality (28, 29, 45). These findings may suggest, that NP levels before implantation and during LVAD support may not have similar predictive value for all-cause mortality, as the hemodynamic support provided by the LVAD may change NP levels and the accompanied mortality risk.
Finally, another explanation for the lack of predictive value of NPs regarding all-cause mortality could be the erratic course of LVAD therapy. Major adverse event rates are high and correspond to high mortality rates. The studies included in this review that found no relation between NPs and all-cause mortality had a follow-up duration of 90, 180, and 730 days after LVAD implantation. Competing risk analyses should have been performed to account for the effect of MAEs on mortality. However, none of the studies included in this review provided these analyses. This statistic error could explain why in the included studies NPs are not predictive for mortality, but appear to have predictive value for various MAEs.
Right Ventricular Failure
Four studies investigated whether NP levels were predictive of RVF after LVAD implantation. Due to the large study by Loghmanpour et al. the sample size of the studies (BNP, proBNP) reporting a positive predictive value for RVF was 11.049 patients, whereas the total sample size of the studies (NT-proBNP) which reported no predictive value was 54 patients (34–37). All studies analyzing BNP and proBNP were predictive, whereas the study by Potapov et al. analyzing NT-proBNP was not. It should be noted that this is only one study with a small study population (37). Nevertheless, this finding may be linked to the type of NPs that was investigated. In addition, this could be explained by the follow-up duration. Potapov et al. investigated RVF within 48 hours postoperatively, whereas all other studies analyzed RVF after the first 48 hours post LVAD implantation (34–37). Furthermore, it should be noted that in all studies, the definition of RVF included “need for RVAD.” Since the decision to use an RVAD after LVAD implantation may vary based on clinical practice, this may change the definition of outcome and thereby the prognostic value of NPs for prediction of RVF.
It is well-known that RVF after LVAD implantation severely impairs prognosis. In the INTERMACS registry, RVF represented the specific cause of death in 4% of all patients (4). The interaction between the LVAD, the right ventricle (RV), and NP system is complex. Preoperative elevated NP levels, inflammatory markers and cytokines may represent a worse hemodynamic status and therefore a higher susceptibility to RVF after LVAD implantation (41). At the same time, it has been suggested that elevations in neurohumoral markers and cytokines may directly influence RV function, contributing to the development of RVF (41).
One study included in our review analyzed late RVF after LVAD implantation, and found no relation of BNP levels before LVAD implantation to late RVF (mean follow-up of 3.4 years) (38). This may be explained by the fact that development of RVF during long-term support is most likely multi-factorial. Different from the LV, the RV does not exhibit significant reverse structural remodeling despite reduced RV afterload during LVAD support (54–56). Kato et al. demonstrated that the CF-LVAD impairs the physiological contractility of cardiomyocytes by the non-pulsatile mode of LV unloading, which over time could lead to decreased RV compliance and contractility (40, 57). Furthermore, interventricular septum displacement caused by suction of the CF-LVAD may result in RV dysynchrony and also reduced cooptation of the tricuspid valve. In long-term LVAD therapy, this may gradually increase tricuspid regurgitation and subsequent increase RV preload. Over time these factors could contribute to the development of late RVF (38). Future studies should address preoperative circulating NP levels in relation to these different factors, in order to better predict RVF during long-term LVAD support.
Major Adverse Events
In the INTERMACS registry, the most frequently reported MAEs after LVAD implantation are infection, neurologic events, RVF, device malfunction including pump thrombosis, and multiple system organ failure (4). However, apart from RVF, there were no studies available that assessed the relation between NPs and these specific MAEs. The studies included in this review assessed the relation between NP levels before LVAD implantation and MAEs including VA, AR, “combined adverse events,” complicated postoperative stay, and all-cause rehospitalization. All studies that were included found that NP (BNP and NT-proBNP) levels before LVAD implantation are predictive of diverse MAEs occurring in the postoperative period within 15 days up to 2 years follow-up, and rehospitalizations within 1.5 year after LVAD implantation (42–44).
Ventricular Arrhythmia
Hellman et al. demonstrated that high BNP levels before LVAD implantation are a powerful predictor for VA up to 15 days (43). Several mechanisms may explain this finding. BNP levels reflect ventricular stretch and hypertrophy, which over time results in tissue fibrosis and other changes of the myocardium that may be a substrate for VA (58). The LVAD unloads the failing heart, but cannot initiate reverse remodeling within 15 days. Thus, the substrate for VA remains, as does the prognostic value of BNP before LVAD implantation. Another possible explanation may be that high BNP levels are associated with elevated levels of cytokines and catecholamines, resulting in prolongation of the action potential and enhanced calcium entry, causing QTc prolongation and promoting arrhythmogenesis, eventually triggering VA (43, 59).
Aortic Regurgitation
During CF-LVAD therapy, up to 15% of the patients may develop moderate to severe AR, with significant impact on morbidity and mortality (42). The study by Truby et al. identified BNP levels >500 ng/L as a predictor in a univariate analysis of a cox proportional hazard model for the development of moderate or severe AR after 2 years of LVAD therapy. However, BNP levels were not taken into account in the multivariate analysis of AR development (42). Factors like body mass index, sex, and destination therapy strategy appear to be stronger predictors of development of moderate or severe AR than BNP levels (42). Nevertheless, this study points out that BNP identifies patients who are vulnerable for adverse events. In patients with AR, NPs are predictive of the development of HF and mortality (60). However, no studies are available that assess the prognostic value of NPs in relation to AR in (advanced) HF patients. Therefore, more studies are needed to get mechanistic insights into the relation between NPs and AR in HF patients receiving LVAD therapy.
Rehospitalization and Combined Adverse Events
Two studies analyzed rehospitalization after initial discharge, of which Hasin et al. found higher NT-proBNP before LVAD implantation predictive for, and associated with, less rehospitalization for any MAE (44). Hegarova et al. demonstrated that higher BNP levels were associated with less or no combined adverse events that required outpatient care or rehospitalization. In a sub-analysis, the authors found that BNP levels were not able to differentiate between combined adverse events that required rehospitalization and those that did not (45). Interestingly, both studies demonstrated that higher NP levels before LVAD implantation were related to less combined adverse events (44, 45). This finding may be related to the kind of MAE. Hasin et al. found that cardiac events (30.4%) including VA, HF, and chest pain, and bleeding events (29.6%) were the main reasons for rehospitalization, whereas Hegarova et al. found that pump thrombosis (29%) and decompensated HF (26%) were the most frequent adverse events (44, 45). These findings suggest that NP levels before LVAD implantation within a certain range may be predictive for rehospitalization of specific MAEs.
Myocardial Recovery
Among the articles considered in this systematic review, five studies investigated the relation of NPs with myocardial recovery. Imamura et al. demonstrated that BNP before LVAD implantation was not predictive for LV ejection fraction recovery (47). The study by Topkara et al. demonstrated that NP levels (BNP, proBNP) were associated with myocardial recovery, while the other studies did not (6, 47–50). The total sample size in the four studies that found no association was just slightly larger than the sample size of the one study that did, mainly due to the large studies by Topkara et al. (6) and Wever-Pinzon et al. (48). Both authors extracted their data from the INTERMACS registry, had comparable inclusion criteria, baseline characteristics, outcome and median follow-up. Nevertheless, they found conflicting results. This may be related to the fact that Wever-Pinzon et al. additionally included patients implanted with pulsatile-flow LVADs, and a relatively high number of INTERMACS level 1 patients, who were in critical cardiogenic shock at time of device implantation (48). The higher number of INTERMACS level 1 patients may explain the higher levels of BNP found within the recovery group, and may diminish the associative relation between lower levels of BNP and myocardial recovery. Taken together, these reports are indicative for the fact that NPs may not be a specific marker for cardiac recovery, but rather reflect the general physical condition and the severity of HF in advanced HF patients receiving LVAD therapy.
Limitations
Although we systematically assessed the evidence for NP and its role as prognostic biomarker in advanced HF patients who receive LVAD therapy, our study is not devoid of its own limitations. According to the NOS score, most studies included in our review were of good quality. However, a number of these studies had a small patient population and therefore low statistical power. The heterogenous nature of the data in terms of timing of NP measurements, subtypes of NP, follow-up time, statistical analyses, and end-points pre-empted us from performing a meta-analysis and derive definitive conclusions. In addition, in a number of studies included in this review, the predictive value of NPs in LVAD patients was not the main hypothesis. We were not able to asses all end-points because of limited literature, and several end-points had heterogenous and subjective definitions, such as “need for RVAD” for the definition of RVF. Although it is generally accepted that NP levels are influenced by gender, age, BMI, comorbidities, kidney disease, and HF medication, most studies did not take all variables into account. In addition, some bias was created as the manuscripts from the same authors, and several studies analyzing multiple subtypes of NP or end-points, were included.
Future Perspective
Given the high incidence of MAEs after LVAD implantation, optimization of patient selection is crucial in order to improve outcome after LVAD implantation. Circulating NP levels may have some power predicting MAEs, RVF and rehospitalization during LVAD therapy. However, new, more promising, circulating biomarkers have been identified for prognostication of MAEs and mortality in HF patients (15, 61–64). Multi-biomarker panels seem to improve the prognostic power of these biomarkers. Emdin et al. compared a multi-biomarker panel [NT-proBNP, soluble suppression of tumorigenicity-2 (sST2), high-sensitive troponin T (hs-TnT)] with a single biomarker (NT-proBNP). Relative risk for all-cause mortality was higher among patients with elevated levels of all multi-panel biomarkers compared to patients with elevated levels of a single biomarker (NT-proBNP, sST2, hs-TnT; RR 9.5 vs. NT-proBNP; RR 2.3, respectively) (65). Ahmad et al. showed that novel biomarkers, such as galectin-3 (GAL-3), ST2, growth differentiation factor-15 (GDF-15), high sensitive C-reactive protein (hs-CRP), and copeptin, when stratified by baseline NT-proBNP levels in their cohort of advanced HF patients, were more sensitive of maladaptive processes than traditional laboratory markers with established prognostic significance, such as red blood cell distribution width, creatinine, blood urea nitrogen, and sodium, which remained within normal limits (66). These studies show that novel biomarkers and their multi-biomarker panels may reflect disease severity more accurately than currently used metrics (65–67). In addition, these novel biomarkers provide a unique insight into the pathophysiologic changes of HF as they reflect the different maladaptive processes involved e.g., oxidative stress, fibrosis, and inflammation (68). Therefore, novel biomarkers may be considered for screening of patients with advanced HF requiring CF-LVAD therapy, and monitoring of LVAD patients. The present systematic review demonstrates that in order to improve generalizability and interpretation, large prospective studies with predefined outcome, and follow-up duration analyzing preimplantation NPs, multi-biomarker panels and their changes over time are warranted. Validated assays in consecutive patients should be used, and detailed cardiovascular profiles should be created to systematically define pathologies contributing to the levels of NP and other circulating biomarkers.
Conclusions
This systematic review demonstrates that BNP levels before LVAD implantation are not predictive of all-cause mortality after LVAD implantation. The implantation of an LVAD appears to alter prognosis and NP levels to such an extent that prognosis for mortality stratified by NP levels before LVAD implantation is not applicable after LVAD implantation. However, NP levels appear to identify advanced HF patients who are at risk for postoperative RVF and MAEs, such VA, AR, and rehospitalization. More studies regarding the timing of NP measurements, using different subtypes of NPs within prospective cohorts with predetermined end-points and follow-up are needed to confirm the prognostic value of NPs in advanced HF patients who will receive LVAD therapy.
Data Availability Statement
The original contributions presented in the study are included in the article/Supplementary Material, further inquiries can be directed to the corresponding author.
Author Contributions
EJ: conceptualization: lead, formal analysis: lead, investigation: equal, methodology: equal, project administration: lead, software: equal, writing–original draft: lead, writing–review & editing: equal. JJ: conceptualization: lead, investigation: equal, methodology: equal, supervision: lead, writing- original draft: equal, writing–review & editing: equal. SB: conceptualization: lead, methodology: equal, supervision: equal, writing–original draft: equal, writing–review & editing: equal. MS: conceptualization: equal, supervision: equal, writing- original draft: equal, writing–review & editing: equal. LT: conceptualization: lead, formal analysis: equal, methodology: equal, supervision: lead, writing- original draft: lead, writing–review & editing: lead. All authors contributed to the article and approved the submitted version.
Conflict of Interest
The authors declare that the research was conducted in the absence of any commercial or financial relationships that could be construed as a potential conflict of interest.
Supplementary Material
The Supplementary Material for this article can be found online at: https://www.frontiersin.org/articles/10.3389/fcvm.2021.699492/full#supplementary-material
References
1. Ahmad T, Patel CB, Milano CA, Rogers JG. when the heart runs out of heartbeats: treatment options for refractory end-stage heart failure. Circulation. (2012) 125:2948–55. doi: 10.1161/CIRCULATIONAHA.112.097337
2. Yancy CW, Jessup M, Bozkurt B, Butler J, Casey DE Jr, Colvin MM, et al. 2017 ACC/AHA/HFSA focused update of the 2013 ACCF/AHA guideline for the management of heart failure: a report of the American college of cardiology/American heart association task force on clinical practice guidelines and the heart failure society of America. J Am Coll Cardiol. (2017) 70:776–803. doi: 10.1016/j.jacc.2017.04.025
3. Teuteberg JJ, Cleveland JC Jr, Cowger J, Higgins RS, Goldstein DJ, Keebler M, et al. The society of thoracic surgeons intermacs 2019 annual report: the changing landscape of devices and indications. Ann Thorac Surg. (2020) 109:649–60. doi: 10.1016/j.athoracsur.2019.12.005
4. Kormos RL, Cowger J, Pagani FD, Teuteberg JJ, Goldstein DJ, Jacobs JP, et al. The society of thoracic surgeons intermacs database annual report: evolving indications, outcomes, and scientific partnerships. Ann Thorac Surg. (2019) 107:341–53. doi: 10.1016/j.athoracsur.2018.11.011
5. Kirklin JK, Pagani FD, Kormos RL, Stevenson LW, Blume ED, Myers SL, et al. Eighth annual INTERMACS report: special focus on framing the impact of adverse events. J Heart Lung Transplant. (2017) 36:1080–6. doi: 10.1016/j.healun.2017.07.005
6. Topkara VK, Garan AR, Fine B, Godier-Furnemont AF, Breskin A, Cagliostro B, et al. Myocardial recovery in patients receiving contemporary left ventricular assist devices: results from the interagency registry for mechanically assisted circulatory support (INTERMACS). Circ Heart Fail. (2016) 9 e003157.doi: 10.1161/CIRCHEARTFAILURE.116.003157
7. Hon JK, Yacoub MH. Bridge to recovery with the use of left ventricular assist device and clenbuterol. Ann Thorac Surg. (2003) 75:S36–41. doi: 10.1016/S0003-4975(03)00460-0
8. Motiwala SR, Januzzi JL. The role of natriuretic peptides as biomarkers for guiding the management of chronic heart failure. Clin Pharmacol Ther. (2013) 93:57–67. doi: 10.1038/clpt.2012.187
9. Levin ER, Gardner DG, Samson WK. Natriuretic peptides. NEJM. (1998) 339:321–8. doi: 10.1056/NEJM199807303390507
10. Kinnunen P, Vuolteenaho O, Ruskoaho H. Mechanisms of atrial and brain natriuretic peptide release from rat ventricular myocardium: effect of stretching. Endocrinology. (1993) 132:1961–70. doi: 10.1210/endo.132.5.8477647
11. Weber M, Hamm C. Role of B-type natriuretic peptide (BNP) and NT-proBNP in clinical routine. Heart. (2006) 92:843–9. doi: 10.1136/hrt.2005.071233
12. Piek A, Du W, de Boer RA, Sillje HHW. Novel heart failure biomarkers: why do we fail to exploit their potential? Crit Rev Clin Lab Sci. (2018) 55:246–63. doi: 10.1080/10408363.2018.1460576
13. Savic-Radojevic A, Pljesa-Ercegovac M, Matic M, Simic D, Radovanovic S, Simic T. Novel biomarkers of heart failure. Adv Clin Chem. (2017) 79:93–152. doi: 10.1016/bs.acc.2016.09.002
14. Madamanchi C, Alhosaini H, Sumida A, Runge MS. Obesity and natriuretic peptides, BNP and NT-proBNP: mechanisms and diagnostic implications for heart failure. Int J Cardiol. (2014) 176:611–7. doi: 10.1016/j.ijcard.2014.08.007
15. Chow SL, Maisel AS, Anand I, Bozkurt B, de Boer RA, Felker GM, et al. Role of biomarkers for the prevention, assessment, and management of heart failure: a scientific statement from the American heart association. Circulation. (2017) 135:e1054–91. doi: 10.1161/CIR.0000000000000490
16. Myhre PL, Vaduganathan M, Claggett B, Packer M, Desai AS, Rouleau JL, et al. B-Type natriuretic peptide during treatment with sacubitril/valsartan: the PARADIGM-HF trial. J Am Coll Cardiol. (2019) 73:1264–72. doi: 10.1016/j.jacc.2019.01.018
17. Saenger AK, Rodriguez-Fraga O, Ler R, Ordonez-Llanos J, Jaffe AS, Goetze JP, et al. Specificity of B-type natriuretic peptide assays: cross-reactivity with different BNP, NT-proBNP, and proBNP peptides. Clin Chem. (2017) 63:351–8. doi: 10.1373/clinchem.2016.263749
18. Lam CS, Burnett JC Jr, Costello-Boerrigter L, Rodeheffer RJ, Redfield MM. Alternate circulating pro-B-type natriuretic peptide and b-type natriuretic peptide forms in the general population. J Am Coll Cardiol. (2007) 49:1193–202. doi: 10.1016/j.jacc.2006.12.024
19. Hutfless R, Kazanegra R, Madani M, Bhalla MA, Tulua-Tata A, Chen A, et al. Utility of B-type natriuretic peptide in predicting postoperative complications and outcomes in patients undergoing heart surgery. J Am Coll Cardiol. (2004) 43:1873–9. doi: 10.1016/j.jacc.2003.12.048
20. Oremus M, Don-Wauchope A, McKelvie R, Santaguida PL, Hill S, Balion C, et al. BNP and NT-proBNP as prognostic markers in persons with chronic stable heart failure. Heart Failure Rev. (2014) 19:471–505. doi: 10.1007/s10741-014-9439-6
21. Doust JA, Pietrzak E, Dobson A, Glasziou PP. How well does B-type natriuretic peptide predict death and cardiac events in patients with heart failure: systematic review. Brit Med J. (2005) 330:625–7. doi: 10.1136/bmj.330.7492.625
22. Huang YT, Tseng YT, Chu TW, Chen J, Lai MY, Tang WR, et al. N-terminal pro b-type natriuretic peptide (NT-pro-BNP)–based score can predict in-hospital mortality in patients with heart failure. Sci Rep. (2016) 6:29590. doi: 10.1038/srep29590
23. Januzzi JL Jr, Maisel AS, Silver M, Xue Y, DeFilippi C. Natriuretic peptide testing for predicting adverse events following heart failure hospitalization. Congest Heart Fail. (2012) 18:S9–13. doi: 10.1111/j.1751-7133.2012.00306.x
24. Savarese G, Musella F, D'Amore C, Vassallo E, Losco T, Gambardella F, et al. Changes of natriuretic peptides predict hospital admissions in patients with chronic heart failure: a meta-analysis. JACC: Heart Failure. (2014) 2:148–58. doi: 10.1016/S0735-1097(14)60737-3
25. Kormos RL, Antonides CFJ, Goldstein DJ, Cowger JA, Starling RC, Kirklin JK, et al. Updated definitions of adverse events for trials and registries of mechanical circulatory support: a consensus statement of the mechanical circulatory support academic research consortium. J Heart Lung Transplant. (2020) 39:735–50. doi: 10.1016/j.healun.2020.03.010
26. Moskalewicz A, Oremus M. No clear choice between newcastle-ottawa scale and appraisal tool for cross-sectional studies to assess methodological quality in cross-sectional studies of health-related quality of life and breast cancer. J Clin Epid. (2020) 120:94–103. doi: 10.1016/j.jclinepi.2019.12.013
27. Berkman ND, Lohr KN, Morgan LC, Richmond E, Kuo TM, Morton S, et al. Reliability Testing of The Ahrq Epc Approach to Grading The Strength of Evidence in Comparative Effectiveness Reviews. Rockville: AHRQ Methods for Effective Health Care (2012).
28. Papathanasiou M, Pizanis N, Tsourelis L, Koch A, Kamler M, Rassaf T, et al. Dynamics and prognostic value of B-type natriuretic peptide in left ventricular assist device recipients. J Thorac Dis. (2019) 11:138–44. doi: 10.21037/jtd.2018.12.43
29. Sato T, Seguchi O, Iwashima Y, Yanase M, Nakajima S, Hieda M, et al. Serum brain natriuretic peptide concentration 60 days after surgery as a predictor of long-term prognosis in patients implanted with a left ventricular assist device. ASAIO J. (2015) 61:373–8. doi: 10.1097/MAT.0000000000000234
30. Yoshioka D, Sakaguchi T, Saito S, Miyagawa S, Nishi H, Yoshikawa Y, et al. Predictor of early mortality for severe heart failure patients with left ventricular assist device implantation: significance of INTERMACS level and renal function. Circ J. (2012) 76:1631–8. doi: 10.1253/circj.CJ-11-1452
31. Shiga T, Kinugawa K, Hatano M, Yao A, Nishimura T, Endo M, et al. Age and preoperative total bilirubin level can stratify prognosis after extracorporeal pulsatile left ventricular assist device implantation. Circ J. (2011) 75:121–8. doi: 10.1253/circj.CJ-10-0770
32. Topilsky Y, Oh JK, Shah DK, Boilson BA, Schirger JA, Kushwaha SS, et al. Echocardiographic predictors of adverse outcomes after continuous left ventricular assist device implantation. JACC: Cardiovasc Imaging. (2011) 4:211–22. doi: 10.1016/j.jcmg.2010.10.012
33. Cabiati M, Caruso R, Caselli C, Frigerio M, Prescimone T, Parodi O, et al. The natriuretic peptide time-course in end-stage heart failure patients supported by left ventricular assist device implant: focus on NT-proCNP. Peptides. (2012) 36:192–8. doi: 10.1016/j.peptides.2012.05.018
34. Shiga T, Kinugawa K, Imamura T, Kato N, Endo M, Inaba T, et al. Combination evaluation of preoperative risk indices predicts requirement of biventricular assist device. Circ J. (2012) 76:2785–91. doi: 10.1253/circj.CJ-12-0231
35. Kato TS, Chokshi A, Singh P, Khawaja T, Iwata S, Homma S, et al. Markers of extracellular matrix turnover and the development of right ventricular failure after ventricular assist device implantation in patients with advanced heart failure. J Heart Lung Transplant. (2012) 31:37–45. doi: 10.1016/j.healun.2011.10.007
36. Loghmanpour NA, Kormos RL, Kanwar MK, Teuteberg JJ, Murali S, Antaki JF. A bayesian model to predict right ventricular failure following left ventricular assist device therapy. JACC Heart Fail. (2016) 4:711–21. doi: 10.1016/j.jchf.2016.04.004
37. Potapov EV, Stepanenko A, Dandel M, Kukucka M, Lehmkuhl HB, Weng Y, et al. Tricuspid incompetence and geometry of the right ventricle as predictors of right ventricular function after implantation of a left ventricular assist device. J Heart Lung Transplant. (2008) 27:1275–81. doi: 10.1016/j.healun.2008.08.012
38. Kapelios CJ, Charitos C, Kaldara E, Malliaras K, Nana E, Pantsios C, et al. Late-onset right ventricular dysfunction after mechanical support by a continuous-flow left ventricular assist device. J Heart Lung Transplant. (2015) 34:1604–10. doi: 10.1016/j.healun.2015.05.024
39. Deswarte G, Kirsch M, Lesault PF, Trochu JN, Damy T. Right ventricular reserve and outcome after continuous-flow left ventricular assist device implantation. J Heart Lung Transplant. (2010) 29:1196–8. doi: 10.1016/j.healun.2010.05.026
40. Pettinari M, Jacobs S, Rega F, Verbelen T, Droogne W, Meyns B. Are right ventricular risk scores useful? Eur J Cardiothorac Surg. (2012) 42:621–6. doi: 10.1093/ejcts/ezs104
41. Hennig F, Stepanenko AV, Lehmkuhl HB, Kukucka M, Dandel M, Krabatsch T, et al. Neurohumoral and inflammatory markers for prediction of right ventricular failure after implantation of a left ventricular assist device. Gen Thorac Cardiovasc Surg. (2011) 59:19–24. doi: 10.1007/s11748-010-0669-9
42. Truby LK, Garan AR, Givens RC, Wayda B, Takeda K, Yuzefpolskaya M, et al. Aortic insufficiency during contemporary left ventricular assist device support: analysis of the INTERMACS registry. JACC Heart Fail. (2018) 6:951–60. doi: 10.1016/j.jchf.2018.07.012
43. Hellman Y, Malik AS, Lin H, Shen C, Wang IW, Wozniak TC, et al. B-type natriuretic peptide levels predict ventricular arrhythmia post left ventricular assist device implantation. Artificial Organs. (2015) 39:1051–5. doi: 10.1111/aor.12486
44. Hasin T, Marmor Y, Kremers W, Topilsky Y, Severson CJ, Schirger JA, et al. Readmissions after implantation of axial flow left ventricular assist device. J Am Coll Cardiol. (2013) 61:153–63. doi: 10.1016/j.jacc.2012.09.041
45. Hegarova M, Kubanek M, Netuka I, Maly J, Dorazilova Z, Gazdic T, et al. Clinical correlates of b-type natriuretic peptide monitoring in outpatients with left ventricular assist device. Biomed Pap Med Fac Univ Palacky Olomouc Czech Repub. (2017) 161:68–74. doi: 10.5507/bp.2017.003
46. Hasin T, Kushwaha SS, Lesnick TG, Kremers W, Boilson BA, Schirger JA, et al. Early trends in N-terminal pro-brain natriuretic peptide values after left ventricular assist device implantation for chronic heart failure. Am J Cardiol. (2014) 114:1257–63. doi: 10.1016/j.amjcard.2014.07.056
47. Imamura T, Kinugawa K, Nitta D, Kinoshita O, Nawata K, Ono M. Preoperative iodine-123 meta-iodobenzylguanidine imaging is a novel predictor of left ventricular reverse remodeling during treatment with a left ventricular assist device. J Artif Organs. (2016) 19:29–36. doi: 10.1007/s10047-015-0857-6
48. Wever-Pinzon O, Drakos SG, McKellar SH, Horne BD, Caine WT, Kfoury AG, et al. Cardiac recovery during long-term left ventricular assist device support. J Am Coll Cardiol. (2016) 68:1540–53. doi: 10.1016/j.jacc.2016.07.743
49. Imamura T, Kinugawa K, Hatano M, Fujino T, Muraoka H, Inaba T, et al. Preoperative beta-blocker treatment is a key for deciding left ventricular assist device implantation strategy as a bridge to recovery. J Artificial Organs. (2014) 17:23–32. doi: 10.1007/s10047-013-0748-7
50. Mano A, Nakatani T, Oda N, Kato T, Niwaya K, Tagusari O, et al. Which factors predict the recovery of natural heart function after insertion of a left ventricular assist system? J Heart Lung Transplant. (2008) 27:869–74. doi: 10.1016/j.healun.2008.05.007
51. El-Saed A, Voigt A, Shalaby A. Usefulness of brain natriuretic peptide level at implant in predicting mortality in patients with advanced but stable heart failure receiving cardiac resynchronization therapy. Clin Cardiol. (2009) 32:E33–8. doi: 10.1002/clc.20490
52. Shalaby AA, Abraham WT, Fonarow GC, Bersohn MM, Gorcsan J III, Lee LY, et al. Association of BNP and troponin levels with outcome among cardiac resynchronization therapy recipients. Pacing Clin Electrophysiol. (2015) 38:581–90. doi: 10.1111/pace.12610
53. Zile MR, Claggett BL, Prescott MF, McMurray JJ, Packer M, Rouleau JL, et al. Prognostic implications of changes in n-terminal pro-B-type natriuretic peptide in patients with heart failure. J Am Coll Cardiol. (2016) 68:2425–36. doi: 10.1016/j.jacc.2016.09.931
54. Maybaum S, Mancini D, Xydas S, Starling RC, Aaronson K, Pagani FD, et al. Cardiac improvement during mechanical circulatory support–a prospective multicenter study of the LVAD working group. Circulation. (2007) 115:2497–505. doi: 10.1161/CIRCULATIONAHA.106.633180
55. Klotz S, Naka Y, Oz MC, Burkhoff D. Biventricular assist device-induced right ventricular reverse structural and functional remodeling. J Heart Lung Transplant. (2005) 24:1195–201. doi: 10.1016/j.healun.2004.08.005
56. Drakos SG, Wever-Pinzon O, Selzman CH, Gilbert EM, Alharethi R, Reid BB, et al. Magnitude and time course of changes induced by continuous-flow left ventricular assist device unloading in chronic heart failure: insights into cardiac recovery. J Am Coll Cardiol. (2013) 61:1985–94. doi: 10.1016/j.jacc.2013.01.072
57. Kato TS, Chokshi A, Singh P, Khawaja T, Cheema F, Akashi H, et al. Effects of continuous-flow versus pulsatile-flow left ventricular assist devices on myocardial unloading and remodeling. Circulation Heart Fail. (2011) 4:546–53. doi: 10.1161/CIRCHEARTFAILURE.111.962142
58. Tapanainen JM, Lindgren KS, Makikallio TH, Vuolteenaho O, Leppaluoto J, Huikuri HV. natriuretic peptides as predictors of non-sudden and sudden cardiac death after acute myocardial infarction in the beta-blocking era. J Am Coll Cardiol. (2004) 43:757–63. doi: 10.1016/j.jacc.2003.09.048
59. Vrtovec B, Knezevic I, Poglajen G, Sebestjen M, Okrajsek R, Haddad F. Relation of B-type natriuretic peptide level in heart failure to sudden cardiac death in patients with and without QT interval prolongation. Am J Cardiol. (2013) 111:886–90. doi: 10.1016/j.amjcard.2012.11.041
60. Pizarro R, Bazzino OO, Oberti PF, Falconi ML, Arias AM, Krauss JG, et al. Prospective validation of the prognostic usefulness of b-type natriuretic peptide in asymptomatic patients with chronic severe aortic regurgitation. J Am Coll Cardiol. (2011) 58:1705–14. doi: 10.1016/j.jacc.2011.07.016
61. Aimo A, Januzzi JL Jr, Bayes-Genis A, Vergaro G, Sciarrone P, Passino C, et al. Clinical and prognostic significance of sST2 in heart failure: JACC review topic of the week. J Am Coll Cardiol. (2019) 74:2193–203. doi: 10.1016/j.jacc.2019.08.1039
62. Barutaut M, Fournier P, Peacock WF, Evaristi MF, Dambrin C, Caubère C, et al. sST2 adds to the prognostic value of Gal-3 and BNP in chronic heart failure. Acta Cardiol. (2019) 75:739–47. doi: 10.1080/00015385.2019.1669847
63. Bettencourt P, Ferreira-Coimbra J, Rodrigues P, Marques P, Moreira H, Pinto MJ, et al. Towards a multi-marker prognostic strategy in acute heart failure: a role for GDF-15. ESC Heart Fail. (2018) 5:1017–22. doi: 10.1002/ehf2.12301
64. Salah K, Stienen S, Pinto YM, Eurlings LW, Metra M, Bayes-Genis A, et al. Prognosis and NT-proBNP in heart failure patients with preserved versus reduced ejection fraction. Heart. (2019) 105:1182–9. doi: 10.1136/heartjnl-2018-314173
65. Emdin M, Aimo A, Vergaro G, Bayes-Genis A, Lupón J, Latini R, et al. sST2 predicts outcome in chronic heart failure beyond NT-proBNP and high-sensitivity troponin T. J Am Coll Cardiol. (2018) 72:2309–20. doi: 10.1016/j.jacc.2018.08.2165
66. Ahmad T, Fiuzat M, Felker GM, O'Connor C. Novel biomarkers in chronic heart failure. Nat Rev Cardiol. (2012) 9:347–59. doi: 10.1038/nrcardio.2012.37
67. Mathieu K, Ibrahim E-B, Michael B, Martin B, Ibrahim A. Biomarkers in cardiomyopathies and prediction of sudden cardiac death. Curr Pharm Biotechnol. (2017) 18:472–81. doi: 10.2174/1389201018666170623125842
Keywords: left ventricular assist device, circulating biomarkers, natriuretic peptides, adverse events, prognosis
Citation: Janssen E, Jukema JW, Beeres SLMA, Schalij MJ and Tops LF (2021) Prognostic Value of Natriuretic Peptides for All-Cause Mortality, Right Ventricular Failure, Major Adverse Events, and Myocardial Recovery in Advanced Heart Failure Patients Receiving a Left Ventricular Assist Device: A Systematic Review. Front. Cardiovasc. Med. 8:699492. doi: 10.3389/fcvm.2021.699492
Received: 23 April 2021; Accepted: 01 June 2021;
Published: 07 July 2021.
Edited by:
Maria Perticone, University of Magna Graecia, ItalyReviewed by:
Kevin Shah, The University of Utah, United StatesJudith Cuypers, Erasmus Medical Center, Netherlands
Copyright © 2021 Janssen, Jukema, Beeres, Schalij and Tops. This is an open-access article distributed under the terms of the Creative Commons Attribution License (CC BY). The use, distribution or reproduction in other forums is permitted, provided the original author(s) and the copyright owner(s) are credited and that the original publication in this journal is cited, in accordance with accepted academic practice. No use, distribution or reproduction is permitted which does not comply with these terms.
*Correspondence: Laurens F. Tops, bC5mLnRvcHNAbHVtYy5ubA==