- 1Heart and Lung Center, Helsinki University Hospital and University of Helsinki, Helsinki, Finland
- 2Department of Radiology, Helsinki University Hospital (HUS) Medical Imaging Center and Helsinki University Hospital and University of Helsinki, Helsinki, Finland
- 3Department of Internal Medicine, Päijät-Häme Central Hospital, Lahti, Finland
- 4Department of Pharmacology, Faculty of Medicine, University of Helsinki, Helsinki, Finland
- 5Pediatric Cardiac Surgery, Children's Hospital, Helsinki University Hospital and University of Helsinki, Helsinki, Finland
- 6Department of Public Health, University of Helsinki, Helsinki, Finland
- 7Department of Anesthesiology and Intensive Care, Helsinki University Hospital and University of Helsinki, Helsinki, Finland
Background: Cardio-regenerative cell therapies offer additional biologic support to coronary artery bypass surgery (CABG) and are aimed at functionally repairing the myocardium that suffers from or is damaged by ischemia. This non-randomized open-label study assessed the safety and feasibility of epicardial transplantation of atrial appendage micrografts (AAMs) in patients undergoing CABG surgery.
Methods: Twelve consecutive patients destined for CABG surgery were included in the study. Six patients received AAMs during their operation and six patients were CABG-operated without AAMs transplantation. Data from 30 elective CABG patients was collected for a center- and time-matched control group. The AAMs were processed during the operation from a biopsy collected from the right atrial appendage. They were delivered epicardially onto the infarct scar site identified in preoperative late gadolinium enhancement cardiac magnetic resonance imaging (CMRI). The primary outcome measures at the 6-month follow-up were (i) patient safety in terms of hemodynamic and cardiac function over time and (ii) feasibility of therapy administration in a clinical setting. Secondary outcome measures were left ventricular wall thickness, change in myocardial scar tissue volume, changes in left ventricular ejection fraction, plasma concentrations of N-terminal pro-B-type natriuretic peptide levels, NYHA class, number of days in hospital and changes in the quality of life.
Results: Epicardial transplantation of AAMs was safe and feasible to be performed during CABG surgery. CMRI demonstrated an increase in viable cardiac tissue at the infarct site in patients receiving AAMs treatment.
Conclusions and Relevance: Transplantation of AAMs shows good clinical applicability as performed during cardiac surgery, shows initial therapeutic effect on the myocardium and has the potential to serve as a delivery platform for cardiac gene therapies.
Trial Registration: ClinicalTrials.gov, identifier: NCT02672163.
Introduction
Coronary artery bypass graft (CABG) surgery reinstates myocardial blood flow downstream of an occluded coronary artery. Although CABG provides the patient with symptomatic benefit, it unfortunately does not restore the cells lost to infarction (1, 2). Additional therapies, including regenerative cell transplantation, have therefore been investigated for decades, but none have so far been adopted for clinical use (3–5). Furthermore, many regenerative therapies are associated with lengthy processing times and significant costs (6).
The current consensus for a CABG-supportive cardio-regenerative therapy is that the treatment should consist of a mixture of cardiac cell types and their extracellular matrix in a synergistic composition (7–9). To this end, we have demonstrated the preclinical efficacy and initial clinical feasibility (10) of autologous cardiac microtissue, atrial appendage micrografts (AAMs), and have shown that epicardial AAMs therapy activates cardiogenic and cardioprotective pathways (11). Based on these encouraging results, we initiated an open-label, non-randomized clinical study (12) to evaluate the safety and feasibility of AAMs transplantation in conjunction with CABG surgery. We utilized late gadolinium enhancement cardiac magnetic resonance imaging (CMRI) before and 6 months after treatment to gain detailed insight into the AAMs' efficacy to induce myocardial repair.
Materials and Methods
Ethics and Patient Selection
The study protocol was evaluated and approved by the Surgical Ethics Committee of the Hospital District of Helsinki and Uusimaa (number 180/13/03/02/13). The study is registered in the ClinicalTrials.gov database with the identification number NCT02672163. For the interventional open label non-randomized study, a total of 12 patients scheduled for elective CABG surgery from the Helsinki University Hospital (Helsinki, Finland) were recruited in chronological order. An additional 30 patients served as site- and time-matched reference controls. Similar to our earlier clinical cell therapy trial which assessed the effects of bone marrow mononuclear cell transplantation (13, 14), patients of either gender were evaluated for participation if they had ischemic heart failure and were scheduled for elective CABG. The criteria for inclusion and exclusion of the patients are presented in Table 1. Each patient was given both oral and written information about the trial, and the patient's signed informed consent was required for participation. After recruitment and drug optimization, patients waited 4–12 weeks for the elective operation. During this time, the ejection fraction (EF) changed in the AAMs group and control group.
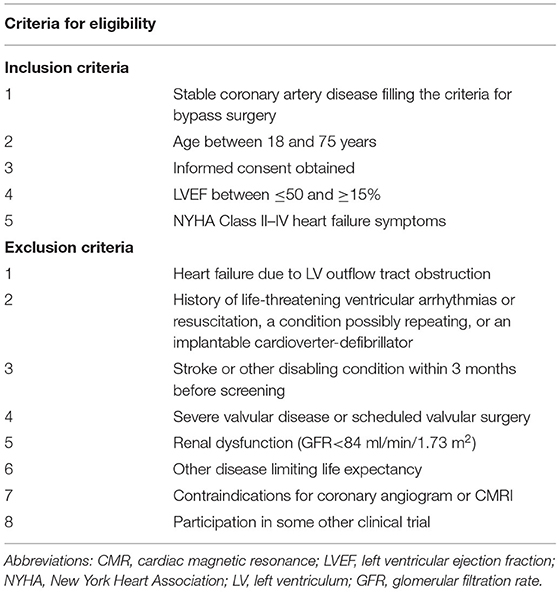
Table 1. Criteria for patient selection—inclusion and exclusion criteria for patients enrolled in the study.
The first six patients were recruited to the AAMs group and they received the AAMs transplant during CABG surgery. The next six patients formed the Control group I. Their surgeries were performed according to the normal hospital protocol and without AAMs transplantation. These two groups underwent the same follow-up protocol. To determine the safety of the procedure, the following 30 patients who met the study's inclusion and exclusion criteria, and were scheduled for elective CABG operation, formed Control group II. These patients were treated according to normal hospital protocol, without the AAMs transplant or any additional imaging, examination or blood tests as were required for the prior two groups.
Echocardiography (echo), New York Heart Association (NYHA) class, basic laboratory tests, and blood N-terminal pro-B-type natriuretic peptide (NT-pro-BNP) concentrations were evaluated at baseline and 3-month follow-up for the patients in the AAMs group and Control group I. CMRI was performed and quality of life (QoL), measured using the health-related questionnaire instrument 15D (15), was evaluated twice per patient: preoperatively and at the 6-month follow-up. The study outline is shown in Figure 1A.
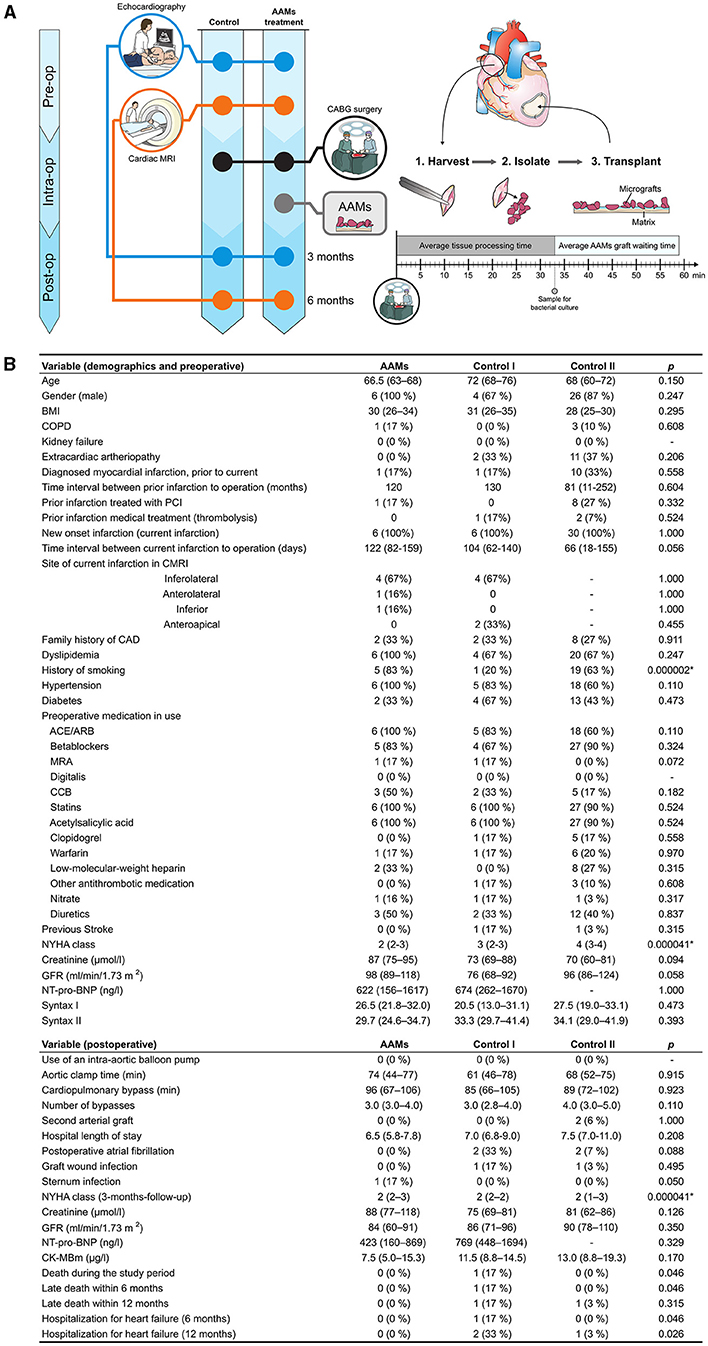
Figure 1. Study outline and demographics—study outline and demographics with pre-operative and post-operative data. (A) All patients underwent pre-operative (Pre-op) echocardiography and CMRI. The Study group received AAMs therapy during coronary artery bypass (CABG) surgery, while the Control group I (Control I) only underwent CABG surgery. AAMs were harvested from the right atrial appendage, mechanically isolated, and transplanted intraoperatively (Intra-op). Patients in both groups were examined 3-months post-operatively (Post-op) by echocardiography and 6 months after the operation using CMRI. (B) Demographics, pre- and post-operative data of the AAMs group (AAMs) and both control groups (Control I and Control II). 1ACE, angiotensin-converting enzyme inhibitor; ARB, angiotensin receptor blocker; BMI, body mass index; CAD, coronary artery disease; CCB, calcium channel blockers; CK-MB, creatine kinase myocardial band; CMRI, cardiac magnetic resonance imaging; COPD, chronic obstructive pulmonary disease; GFR, glomerular filtration rate; MRA, mineralocorticoid receptor antagonists; NT-Pro-BNP, N-terminal pro-B-type natriuretic peptide; NYHA, New York Heart Association; PCI, percutaneous coronary intervention. Results are presented as median (IQR), group comparisons were performed with the Kruskal-Wallis test. Mann Whitney U-test was used for the variables in only Study and Control group I results, or Chi Square for ordinal variables. Significant results after correction (Bonferroni) for multiple comparison are marked by an asterisk (*), with the results of post-hoc between-group analyses found in the text.
Micrograft Isolation
During CABG surgery, AAMs were harvested from the right atrial appendage. A small piece of atrial appendage was removed at the site of insertion for a venous cannula for the heart-and-lung machine. The size and quality of the atrial appendage differ between patients according to age, gender, and comorbidities. To standardize the obtainable size of atrial appendage tissue, a minimum length of 5 × 10 mm and weight from 600 to 800 mg was required for each sample.
The harvested tissue was processed on-site in the operating room using a cell therapy tissue homogenizer (Rigenera-system, HBW s.r.l., Turin, Italy). Cell isolation was performed by a nurse who had received training in a cell-culture laboratory and in our previous studies by using a large-animal model (manuscript in preparation, Nummi et al.). Isolation was performed under strict adherence to sterility. We have previously described the preparation of the AAM transplant in detail (10, 12). As quantified earlier (10), the cell yield from this procedure is 9.76 × 106 ± 0.53 × 106 cells/g of tissue. Cell viability was 90.6% (10).
The isolated AAMs were applied in cardioplegia suspension to an extracellular matrix sheet (Cormatrix® ECMTM Technology, Cormatrix Cardiovascular Inc., Atlanta, GA, USA). Fibrin sealant (TisseelTM, Baxter Healthcare Corp. Westlake Village, CA, USA), routinely used in surgery as tissue glue, was added to the cell suspension to secure the AAMs to the matrix.
Therapy Administration
A standard CABG operation was performed under cardiopulmonary bypass and mild hypothermia, where patients were under cardiac arrest and receiving cardioplegia protection. After completion of the bypass anastomoses, the AAMs transplant was placed over the infarct scar area as determined preoperatively from each individual patient's pre-CABG CMRI images. The sheet with AAMs was placed on top of the damaged myocardium so that the micrografts were facing the epicardium. The matrix sheet attached well to the myocardial surface, and was further secured by three to four simple sutures with non-absorbable monofilament polypropylene string. The therapy application procedure was carefully photographed during each surgery, and the treatment administration site was meticulously detailed in patient documents for further CMRI and echo analyses.
Tests for Transplant Sterility
To verify sterility, samples for microbial cultures were taken from each AAM-transplant.
Clinical Cardiac Magnetic Resonance Imaging
CMRI was performed with a 1.5 T Avanto fit scanner and phase array cardiac coil (Siemens, Erlangen, Germany). Images were electrocardiography-gated and taken during breath-holding. left ventricle (LV) structure and function were imaged by a standardized CMRI protocol. TrueFISP cine series were obtained at the vertical and horizontal long axis for scouts to line up short-axis images. The stack of short-axis images was obtained from the mitral valve plane through the apex. To detect the myocardial scar, late gadolinium enhancement (LGE) was imaged with a 2D-segmented inversion recovery gradient echo sequence 12–20 min after Dotarem® injection (279.3 mg/ml; dose 0.2 mmol/kg). LGE images were obtained for the same views and slice/gap thickness as cine imaging.
All images were analyzed using a dedicated workstation (Medis® suite 3.2.28.0. Medis Medical Imaging Systems, Leiden, the Netherlands). LV function and volumetry were assessed from cine images with Qmass Analyse Program analysis software (Medis) and global longitudinal strain with Qstrain (Medis). Full width with half maximum (FWHM) technique and signal threshold vs. reference mean (STRM) threshold of 5 standard deviations (SD) above the mean signal intensity (SI) was used to assess myocardial and infarcted area mass. The thickness of infarction was measured from the thinnest point exactly at the same position from both images.
End-Point Measures
The primary outcome measures were patient safety in terms of hemodynamic and cardiac function and feasibility of the therapy administration in a clinical setting. Hemodynamics were evaluated during each patient's operation and stay at the intensive care unit (ICU) given requirement for vasoactive medication and success of weaning from cardiopulmonary bypass and respirator. Post-operative hemodynamic criteria for assessing safety were cardiac index, hemoglobin, central venous oxygenation (SvO2), serum potassium level, blood glucose and lactate levels, and arterial pH. Cardiac function was evaluated during the operation by transesophageal echo and during the patient's stay at the ICU by transthoracic echo as well as constant telemetric monitoring of rhythm. Criteria for peri- and post-operative myocardial infarctions were: new regional wall motion abnormality confirmed by echo, ck-mb >50 μg/L, ischemic changes in ECG (LBBB or Q-waves), ventricular arrhythmia, or angiographically documented new graft or new native coronary artery occlusion. Feasibility was evaluated by the success in completing the delivery of the AAMs transplant to the myocardium, waiting times in minutes for the AAMs transplant and the success in closing the right atrial appendage by purse-string suture without additional sutures or patching.
The secondary outcome measures were change in LV infarct area thickness, movement and diastolic function assessed by CMRI, change in the amount of myocardial scar tissue as assessed by CMRI, local changes in systolic, and diastolic measures as estimated by echo, changes in LV ejection fraction (LVEF), NT-pro-BNP level, NYHA class, hospitalization or the days in hospital, and QoL.
Statistics
Results are given as median with interquartile ranges (IQR), or n (%) of group for ordinal and nominal values. Comparisons between groups were performed with the Mann Whitney U-test, or between three groups with the Kruskal-Wallis test. Ordinal variables were tested with the Chi Square test. Multiple comparisons were corrected with the Bonferroni method, significant findings were further tested groupwise using the Mann Whitney U-test or Chi Square test, as applicable. Quality of life data are presented as mean (SD), and were analyzed with the independent samples t-test (two-sided). The level of significance (α) was 0.05. Analyses were performed with the IBM SPSS Statistics 25 program (IBM Corp., Armonk, NY).
Results
Patient demographics are presented in Figure 1B. The median NYHA class differed between the AAMs group and Control group II [median NYHA 2 (range 2–3) in the AAMs group and median 4 (range 2–4) in the Control group II; p < 0.0001]. Control group I had NYHA median 3 (range 2–3). A history of smoking was more common in the AAMs group (n = 5, 83%) than in the control groups (Control I, n = 1, 20%; Control II, n = 19, 63%; p < 0.0001). Otherwise, there were no significant differences between the groups.
All atrial appendages were closed without difficulties with a single purse-string suture. The AAMs transplant was easily prepared in the operating room during CABG surgery. The median time for preparing the AAMs transplant was 33 min (range 22–43 min), and the median waiting time for the transplant was 26 min (range 7–54 min). In every operation, the AAMs transplant was ready to be placed before the anastomosis was done. In the AAMs group there were 4 inferolateral, 1 anterolateral, and 1 inferior and in Control I group 4 inferolateral and 2 anteroapical wall infarcts. All transplants were secured with three to four sutures without difficulties. Bacterial cultures taken from each AAMs transplant were negative for bacterial growth, demonstrating the sterility of the procedure.
Physiological data from the ICU at admission and 24 h after are shown in Table 2. Despite a significant difference in 24 h lactate concentrations between the three groups, all lactate values were within normal range (0.8–1.1 mmol/L). Echo measurements and ECG findings demonstrated no significant differences between the study groups (Table 3).
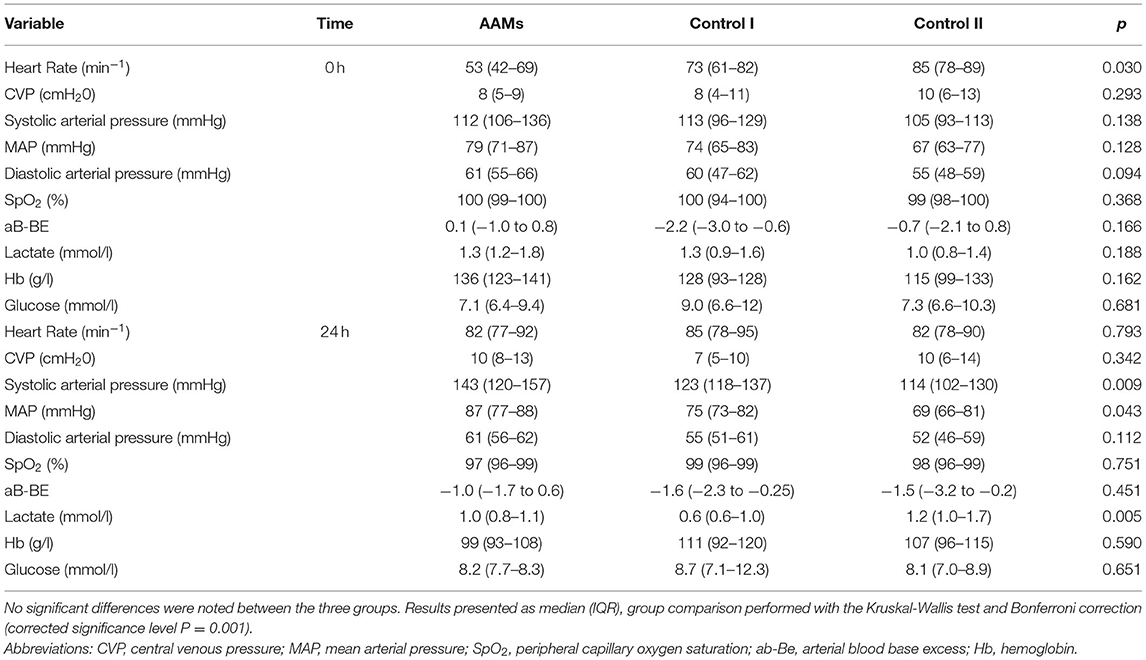
Table 2. Physiological data from ICU—physiological data from the first 24 h of the ICU, comparing the study group to the two control groups.
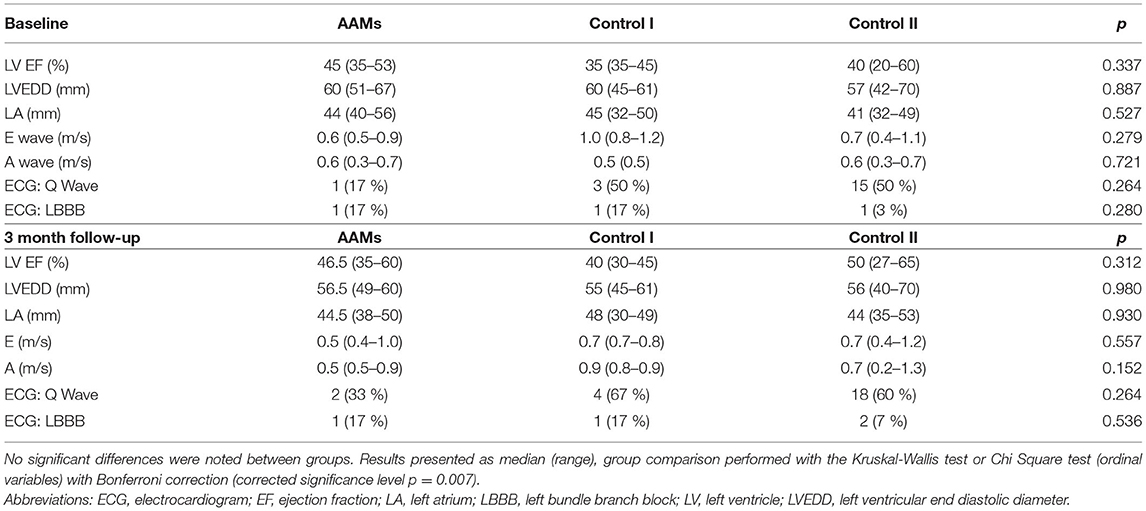
Table 3. Cardiac ultrasound and electrocardiogram data—cardiac ultrasound and electrocardiogram data from the preoperative baseline to the 3-month check-up visit, with comparisons of the study group vs. both control groups.
Postoperative complications are presented in Figure 1B. One patient from the AAMs group was diagnosed with a sternum-wound infection postoperatively. The patient recovered from the infection with antibiotics and no further operative procedures were required. There were no sternum-wound infections in the control groups. Venous graft wound infections in legs were diagnosed from two patients in Control group I and one patient in Control group II. There were no strokes or myocardial infarctions during the study period in any of the three groups.
Discharge times from the hospital were similar between the groups. Readmission to hospital due to heart failure occurred more often in Control group I than in the AAMs group (n = 0, 0%; Control I n = 2, 33%; and Control II n = 1, 3%, p = 0.02). There were no deaths in the AAMs group during the study period of 6 months or the extended follow up time of 1 year. One patient from Control group I died of systolic heart failure during the study period (p = 0.046) and was lost to follow up.
Pre- and postoperative NT-pro-BNP-levels showed no statistical significance between the groups, even though IQR and median values are higher in the Control group I. Delta values demonstrated decreasing trend in AAMs group [ΔNT-pro-BNP in AAMs group −200 ng/l (range −838 to 713 ng/l) and Control group I 83 ng/l (range −160 to 424 ng/l), p = 0.177].
CMRI (Figure 2) was performed on patients from the AAMs group and Control group I preoperatively and at 6-months follow-up. Infarct scar was detected in all patients' preoperative CMRI analyses. Change of viable myocardial thickness at the infarcted area demonstrated a significant increase in the AAMs group [1.0 mm (range 0.2–1.3 mm)] and reduction in the Control group I [−1.4 mm (range −1.7 to 0.0 mm), p = 0.009]. The mainly preferred method of evaluation is the STRM of 5SD which gives a better approximation of the extent of heart infarct as compared to FWHM (16, 17). However, because many studies often use the FWHM, these results are also presented in Figure 2. Representative CMRI pictures from Control I group and AAMs group at both pre- and postoperative time points are shown in Figure 3.
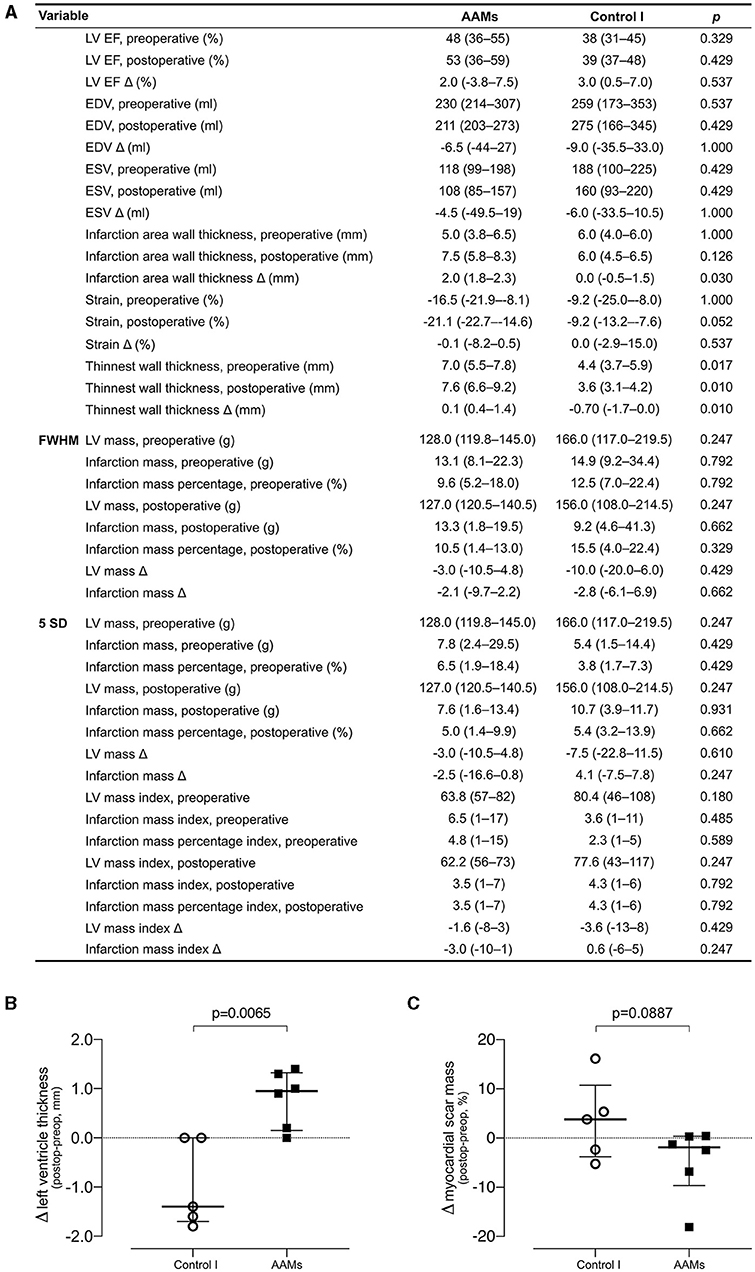
Figure 2. Cardiac magnetic resonance imaging—evaluation of function and structure by cardiac magnetic resonance imaging. (A) Comparison of the AAMs group patients with the Control group I patients. For all variables, a preoperative and post-operative value is presented, followed by the absolute change (Δ) of these values. Results presented as median and IQR, group comparison performed with the Mann Whitney U-test (Bonferroni adjusted P of 0.002). (B) Single parameter comparison of post-operative-preoperative change in viable left ventricle thickness at infarct scar site. (C) Single parameter comparison of post-operative-preoperative change in myocardial scar mass. 1EDV, end diastolic volume; EF, ejection fraction; ESV, end systolic volume; LV, left ventricle; FWHM, full width with half maximum; SD, standard deviation.
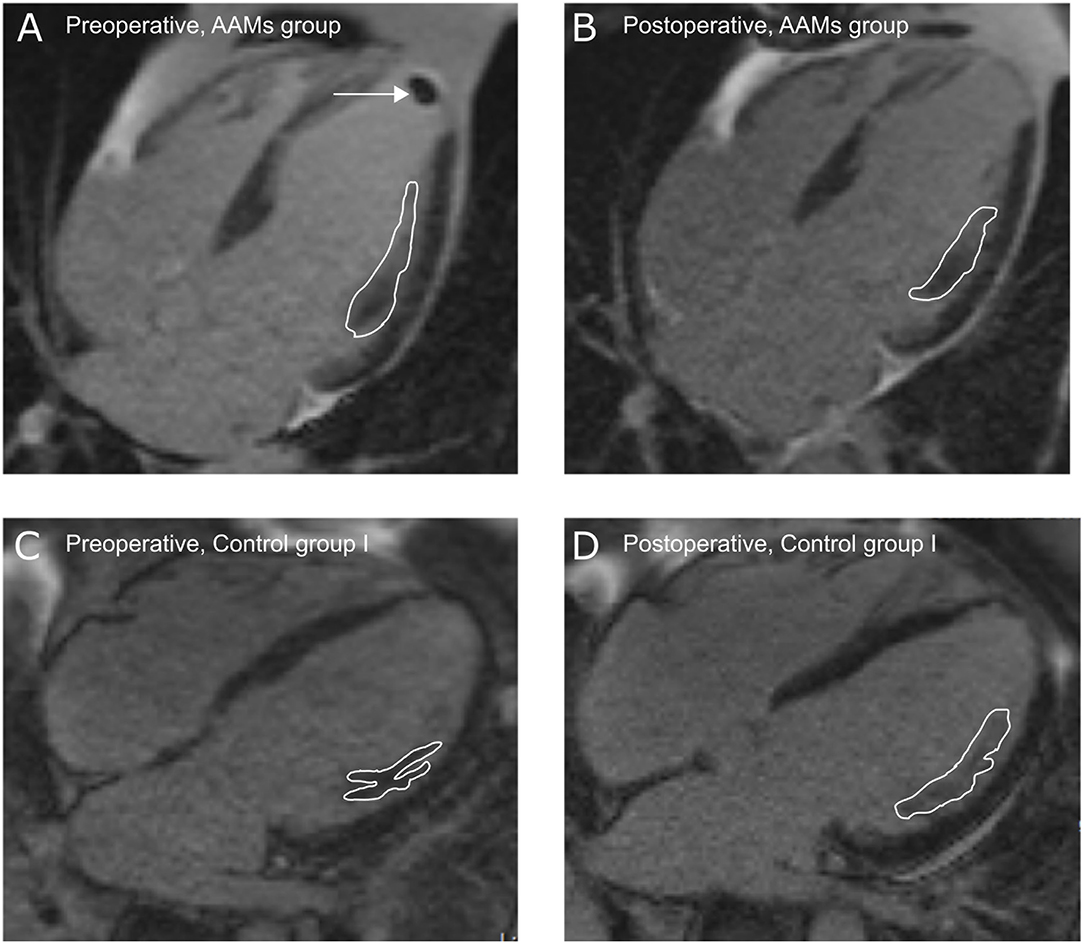
Figure 3. Infarct site in cardiac magnetic resonance imaging—representative pictures from cardiac magnetic resonance imaging illustrating infarct size and site (white lines) at pre- (A,C) and post-operative (B,D) time-points. (A,B) AAMs group and (C,D) Control group I. Arrow shows an apical thrombus that was removed during the coronary artery bypass surgery.
The health-related QoL of the AAMs and Control group I patients did not differ significantly at baseline or at 6 months. Similarly, neither group's QoL differed significantly from that of the reference population, that is, age-matched samples of the general population. The individual mean 15D scores for both groups are presented in Table 4. Figure 4 is a graphical presentation of all parameters at pre- and postoperative time points as compared to the general population reference. At the 6-month follow-up, the AAMs group 15D Index score mean (SD) of 0.8634 (0.07685) was slightly better than the Control group I 15D Index score mean (SD) of 0.8207 (0.15693). The mean (95% CI) difference was 0.04267 (−0.11628 to 0.20162); independent t-test T(10) = 0.598, p = 0.006. According to the data of Sintonen (15), the Finnish age-matched adult general population (n = 562) has a mean (SD) 15D HRQoL index score of 0.9210 (0.07611). Compared to the reference population, the AAMs group (n = 6) had a mean (95% CI) difference of −0.06 (−0.27 to 0.16); independent t-test T(5) = −0.69, P = 0.519. The Control group I's (n = 5) mean (95% CI) 15D index score differed −0.10 (−0.54 to 0.34); independent t-test T(5) = −0.59, p = 0.580.
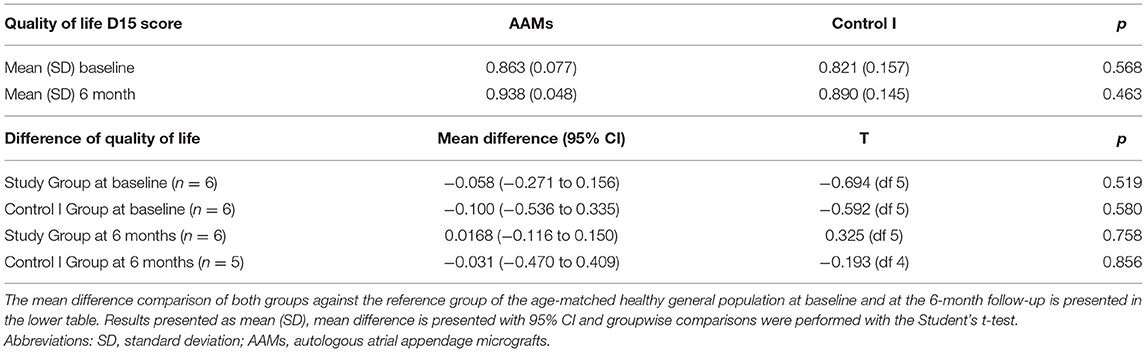
Table 4. Quality of life comparison—quality of life of the study and Control I group at baseline and at the 6-month follow up, with groupwise comparison.
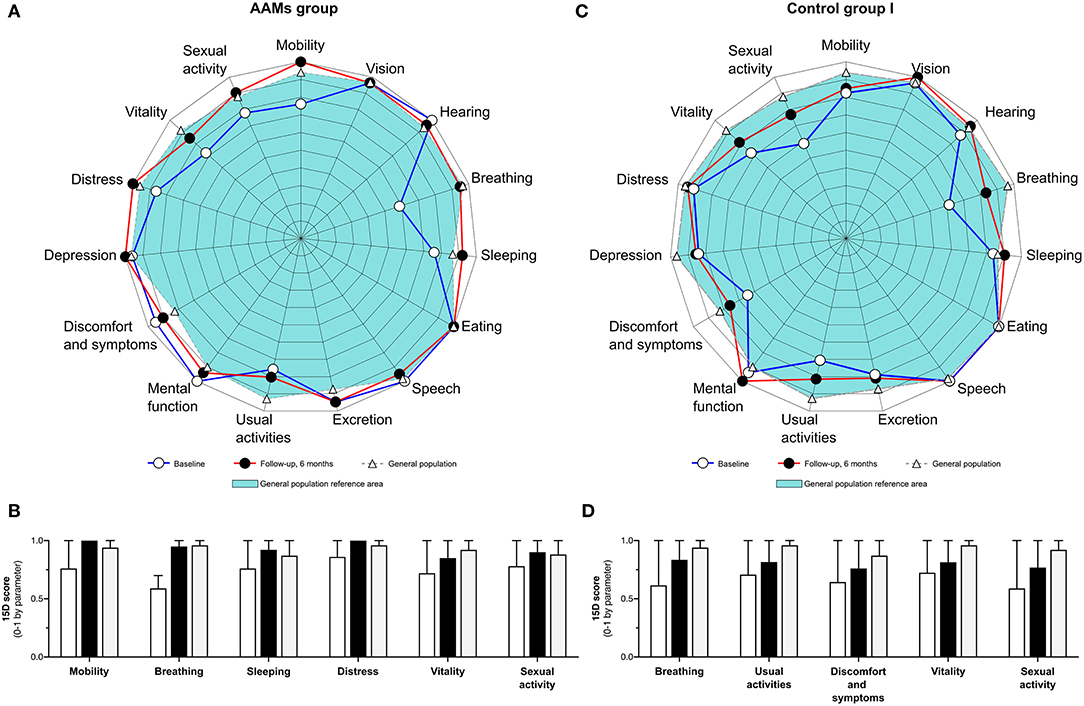
Figure 4. Graphical presentation of quality of life—health-related quality of life QoL in the AAMs group and the Control group I. (A) Graphical presentation of 15D QoL scores preoperatively and at the 6-month follow-up in the AAMs group. (B) Selected parameters of the 15D QoL in the AAMs group. White bars represent preoperative values, black bars represent values at 6-months follow-up and gray bars represent the respective parameter's 15D QoL score in the general population. (C) Graphical presentation of 15D QoL scores preoperatively and at the 6-month follow-up in the Control group I (Control I). (D) Selected parameters of the 15D QoL in the Control I group. White bars represent preoperative values, black bars represent values at 6-months follow-up and gray bars represent the respective parameter's 15D QoL score in the general population.
Discussion
Epicardial transplantation of AAMs was both safe and feasible to be performed during CABG surgery. The AAMs transplant did not increase the risk for any adverse effects or complications during the follow-up time. Preparing the transplant in the operation room was efficient and did not delay the operation more than the time caused by suturing it to the myocardium.
A significant increase in the change of viable myocardial thickness at the infarcted area was found in AAMs group when compared to the Control group I. This encouraging result implies that epicardial delivery of AAMs may also provide benefit as improved local functional capacity of LV. Moreover, with the AAMs transplant the risk for infarct complications such as aneurysms due to the thinning of LV are not as expected, because the infarct area is strengthened. This may also have an impact on the patients' long-term survival.
The quest for the best transplantation route for cell-therapies has been ongoing for decades. Epicardial transplantation with a matrix sheet is considered an attractive choice because of its ability to target the damaged area of myocardium, ensure better cell engraftment, and less damage for the cellular grafts than injections (18–22). The long-term survival of cells after transplantation using this method (23) is, however, in need of further studies.
Cell-based therapies are considered to exert their therapeutic effects via paracrine signaling (24, 25). The soluble factors that are secreted or released by the transplanted cells activate pathways in the host tissue that instigate intrinsic repair processes in the damaged myocardium (4, 26–28). Paracrine factors, such as cytokines, chemokines, and growth factors, drive cascades that eventually improve blood perfusion, tissue repair, and remodeling as well as inhibit hypertrophy and fibrosis (29–31). These signaling factors can also be secreted in the forms of extracellular vesicles or exosomes (32, 33). Exosomes have been proved to increase cell proliferation, reduce infarct size, increase EF (32, 33), and modify inflammation (26–28).
Paracrine activity of the AAMs may also be due to natriuretic peptides, such as atrial natriuretic peptide (ANP) and B-type natriuretic peptide (BNP), produced and secreted from the atrial myocardium (34). Secretion of natriuretic peptides is stimulated by atrial distension, stress, hypoxia, or ischemia, but may also be affected by the mechanical processing of the atrial appendage into micrografts or the epicardial microenvironment. In addition to their systemic effects regulating blood volume and vascular tone, the natriuretic peptides have been demonstrated to have paracrine anti-hypertrophic and anti-fibrotic effects on the myocardium (35). Further investigations into the paracrine factors responsible for the therapeutic effect of AAMs are warranted. Moreover, because angiogenic and cardiopoietic events are likely to occur prior to changes in myocardial viability and thus increases in scarred myocardial wall thickness, targeted investigations to evaluate the mechanisms and contribution of these events in response to AAMs treatment are in need to be addressed in further studies.
Few recent multicenter and randomized trials have shown cell-based therapies to be safe, feasible, and have long-term clinical benefit for patients. CONCERT-HF trial demonstrated a reduction in 1-year cumulative heart failure-related major adverse cardiac events after transendocardial injection of c-kit-positive cardiac cells (36). Moreover, injection of bone marrow mesenchymal cells with or without c-kit positive cardiac cells improved the patients' quality of life compared to placebo (36). In the CHART-1 trial, intramyocardially injected cardiopoietic stem cells were associated with reduced risk of death or cardiovascular hospitalization in a subpopulation of patients with advanced LV enlargement as compared to a sham procedure (37). Despite the lack of evidence in reduction of infarct scar area or improvement in LVEF, these encouraging results indicate that cell-based therapies can have long term effects in preventing progression of HF.
This study is limited by its non-randomized and open-label design as well as the low number of patients. However, for a safety and feasibility study this design can be considered appropriate. Interestingly, already in this limited patient population we identified first indications of efficacy in terms of myocardial structural changes using LGE-CMRI.
We conclude that epicardial transplantation of AAMs is safe and feasible to be performed during CABG surgery in the operating room. These results warrant further evaluation of the AAMs treatment's efficacy in larger randomized controlled trials, with changes in myocardial function and structure by LGE-CMRI as the primary efficacy endpoints. The combination of intraoperative harvesting, isolation, and transplantation of AAM cardiac micrografts shows promise as a safe, straightforward, and clinically feasible therapy during CABG surgery.
Data Availability Statement
The original contributions presented in the study are included in the article/Supplementary Material, further inquiries can be directed to the corresponding author/s.
Ethics Statement
The studies involving human participants were reviewed and approved by Surgical Ethics Committee of the Hospital District of Helsinki and Uusimaa (number 180/13/03/02/13). The patients/participants provided their written informed consent to participate in this study.
Author Contributions
AN, TN, ML, TP, RS, EK, AH, and AV conceived the study design and coordination and helped to draft the manuscript. ML and EK designed and developed the cell graft. KT and TJ operated the study group patients. JS performed the analysis and helped to draft the manuscript. HS provided the QoL reference data and helped with the analysis. AN and SM drafted the manuscript. All authors contributed to the interpretation of the data, read, and approved the final manuscript.
Funding
This work was supported by AH, MK, AV, and AN by Finnish Government Block Grants for Clinical Research (Grant Nos. TYH Y2016SK013, TYH2016211, TYH2015311, TYH2019266, and Y1016SK017), EK by Finnish Funding Agency for Technology and Innovation (Grant No. 40033/14), and AN by Finnish Society of Angiology.
Conflict of Interest
AN and EK are stakeholders in EpiHeart Ltd. developing medical devices for the operating room. HS is the developer of the 15D.
The remaining authors declare that the research was conducted in the absence of any commercial or financial relationships that could be construed as a potential conflict of interest.
Publisher's Note
All claims expressed in this article are solely those of the authors and do not necessarily represent those of their affiliated organizations, or those of the publisher, the editors and the reviewers. Any product that may be evaluated in this article, or claim that may be made by its manufacturer, is not guaranteed or endorsed by the publisher.
Acknowledgments
Research nurses Liisa Blubaum, Mariitta Salmi, Anna Blubaum, and Merja Autti were gratefully acknowledged and thanked for their professional expertise in the development of the protocol. MSc Sole Lätti was acknowledged for the graphics illustrations. The authors thank Dr. Jennifer Rowland for linguistic editing of the manuscript.
Supplementary Material
The Supplementary Material for this article can be found online at: https://www.frontiersin.org/articles/10.3389/fcvm.2021.726889/full#supplementary-material
References
1. Hillis LD, Smith PK, Anderson JL, Bittl JA, Bridges CR, Byrne JG, et al. ACCF/AHA guideline for coronary artery bypass graft surgery: a report of the American College of Cardiology Foundation/American Heart Association Task Force on Practice Guidelines. Circulation. (2011) 124:652–735. doi: 10.1161/CIR.0b013e31823b5fee
2. Hueb W, Lopes N, Gersh BJ, Soares PR, Ribeiro EE, Pereira AC, et al. Ten-year follow-up survival of the Medicine, Angioplasty, or Surgery Study (MASS II): a randomized controlled clinical trial of 3 therapeutic strategies for multivessel coronary artery disease. Circulation. (2010) 122:949–57. doi: 10.1161/CIRCULATIONAHA.109.911669
3. Fisher SA, Doree C, Mathur A, Martin-Rendon E. Meta-analysis of cell therapy trials for patients with heart failure. Circ Res. (2015) 116:1361–77. doi: 10.1161/CIRCRESAHA.116.304386
4. Nguyen PK, Rhee J, Wu JC. Adult stem cell therapy and heart failure, 2000 to 2016: a systematic review. JAMA Cardiol. (2016) 1:831–41. doi: 10.1001/jamacardio.2016.2225
5. Cheng K, Wu F, Cao F. Intramyocardial autologous cell engraftment in patients with ischaemic heart failure: a meta-analysis of randomised controlled trials. Heart Lung Circ. (2013) 22:887–94. doi: 10.1016/j.hlc.2013.04.112
6. D'Alessandro DA, Michler RE. Current and future status of stem cell therapy in heart failure. Curr Treat Options Cardiovasc Med. (2010) 12:614–27. doi: 10.1007/s11936-010-0099-0
7. Radisic M, Christman KL. Materials science and tissue engineering: repairing the heart. Mayo Clin Proc. (2013) 88:884–98. doi: 10.1016/j.mayocp.2013.05.003
8. Stevens KR, Kreutziger KL, Dupras SK, Korte FS, Regnier M, Muskheli V, et al. Physiological function and transplantation of scaffold-free and vascularized human cardiac muscle tissue. Proc Natl Acad Sci USA. (2009) 106:16568–73. doi: 10.1073/pnas.0908381106
9. Oberwallner B, Brodarac A, Anić P, Šarić T, Wassilew K, Neef K, et al. Human cardiac extracellular matrix supports myocardial lineage commitment of pluripotent stem cells. Eur J Cardiothorac Surg. (2015) 47:416–25. doi: 10.1093/ejcts/ezu163
10. Lampinen M, Nummi A, Nieminen T, Harjula A, Kankuri E. Intraoperative processing and epicardial transplantation of autologous atrial tissue for cardiac repair. J Heart Lung Transplant. (2017) 36:1020–2. doi: 10.1016/j.healun.2017.06.002
11. Xie Y, Lampinen M, Takala J, Sikorski V, Soliymani R, Tarkia M, et al. Epicardial transplantation of atrial appendage micrograft patch salvages myocardium after infarction. J Heart Lung Transplant. (2020) 39:707–18. doi: 10.1016/j.healun.2020.03.023
12. Nummi A, Nieminen T, Pätilä T, Lampinen M, Lehtinen ML, Kivistö S, et al. Epicardial delivery of autologous atrial appendage micrografts during coronary artery bypass surgery-safety and feasibility study. Pilot Feasibility Stud. (2017) 3:74. doi: 10.1186/s40814-017-0217-9
13. Pätilä T, Lehtinen M, Vento A, Schildt J, Sinisalo J, Laine M, et al. Autologous bone marrow mononuclear cell transplantation in ischemic heart failure: a prospective, controlled, randomized, double-blind study of cell transplantation combined with coronary bypass. J Heart Lung Transplant. (2014) 33:567–74. doi: 10.1016/j.healun.2014.02.009
14. Lehtinen M, Pätilä T, Vento A, Kankuri E, Suojaranta-Ylinen R, Pöyhiä R, et al. Prospective, randomized, double-blinded trial of bone marrow cell transplantation combined with coronary surgery - perioperative safety study. Interact Cardiovasc Thorac Surg. (2014) 19:990–6. doi: 10.1093/icvts/ivu265
15. Sintonen H. The 15D instrument of health-related quality of life: properties and applications. Ann Med. (2001) 33:328–36. doi: 10.3109/07853890109002086
16. Vermes E, Childs H, Carbone I, Barckow P, Friedrich MG. Auto-threshold quantification of late gadolinium enhancement in patients with acute heart disease. J Magn Reson Imaging. (2013) 37:382–90. doi: 10.1002/jmri.23814
17. Zhang L, Huttin O, Marie PY, Felblinger J, Beaumont M, Chillou C, et al. Myocardial infarct sizing by late gadolinium-enhanced MRI: comparison of manual, full-width at half-maximum, and n-standard deviation methods. J Magn Reson Imaging. (2016) 44:1206–17. doi: 10.1002/jmri.25285
18. Miyagawa S, Saito A, Sakaguchi T, Yoshikawa Y, Yamauchi T, Imanishi Y, et al. Impaired myocardium regeneration with skeletal cell sheets–a preclinical trial for tissue-engineered regeneration therapy. Transplantation. (2010) 90:364–72. doi: 10.1097/TP.0b013e3181e6f201
19. Shimizu T, Yamato M, Isoi Y, Akutsu T, Setomaru T, Abe K, et al. Fabrication of pulsatile cardiac tissue grafts using a novel 3-dimensional cell sheet manipulation technique and temperature-responsive cell culture surfaces. Circ Res. (2002) 90:e40. doi: 10.1161/hh0302.105722
20. Sekine H, Shimizu T, Dobashi I, Matsuura K, Hagiwara N, Takahashi M, et al. Cardiac cell sheet transplantation improves damaged heart function via superior cell survival in comparison with dissociated cell injection. Tissue Eng Part A. (2011) 17:2973–80. doi: 10.1089/ten.tea.2010.0659
21. Fukushima S, Campbell NG, Coppen SR, Yamahara K, Yuen AH, Smolenski RT, et al. Quantitative assessment of initial retention of bone marrow mononuclear cells injected into the coronary arteries. J Heart Lung Transplant. (2011) 30:227–33. doi: 10.1016/j.healun.2010.09.002
22. Hattori F, Chen H, Yamashita H, Tohyama S, Satoh YS, Yuasa S, et al. Nongenetic method for purifying stem cell-derived cardiomyocytes. Nat Methods. (2010) 7:61–6. doi: 10.1038/nmeth.1403
23. Shudo Y, Miyagawa S, Fukushima S, Saito A, Shimizu T, Okano T, et al. Novel regenerative therapy using cell-sheet covered with omentum flap delivers a huge number of cells in a porcine myocardial infarction model. J Thorac Cardiovasc Surg. (2011) 142:1188–96. doi: 10.1016/j.jtcvs.2011.07.002
24. Hodgkinson CP, Bareja A, Gomez JA, Dzau VJ. Emerging concepts in paracrine mechanisms in regenerative cardiovascular medicine and biology. Circ Res. (2016) 118:95–107. doi: 10.1161/CIRCRESAHA.115.305373
25. Plotnikov EY, Silachev DN, Popkov VA, Zorova LD, Pevzner IB, Zorov SD, et al. Intercellular signalling cross-talk: to kill, to heal and to rejuvenate. Heart Lung Circ. (2017) 26:648–59. doi: 10.1016/j.hlc.2016.12.002
26. Gnecchi M, Zhang Z, Ni A, Dzau VJ. Paracrine mechanisms in adult stem cell signaling and therapy. Circ Res. (2008) 103:1204–19. doi: 10.1161/CIRCRESAHA.108.176826
27. Gnecchi M, He H, Liang OD, Melo LG, Morello F, Mu H, et al. Paracrine action accounts for marked protection of ischemic heart by Akt-modified mesenchymal stem cells. Nat Med. (2005) 11:367–8. doi: 10.1038/nm0405-367
28. Stempien-Otero A, Helterline D, Plummer T, Farris S, Prouse A, Polissar N, et al. Mechanisms of bone marrow-derived cell therapy in ischemic cardiomyopathy with left ventricular assist device bridge to transplant. J Am Coll Cardiol. (2015) 65:1424–34. doi: 10.1016/j.jacc.2015.01.042
29. Tang XL, Rokosh G, Sanganalmath SK, Yuan F, Sato H, Mu J, et al. Intracoronary administration of cardiac progenitor cells alleviates left ventricular dysfunction in rats with a 30-day-old infarction. Circulation. (2010) 121:293–305. doi: 10.1161/CIRCULATIONAHA.109.871905
30. Farahmand P, Lai TY, Weisel RD, Fazel S, Yau T, Menasche P, et al. Skeletal myoblasts preserve remote matrix architecture and global function when implanted early or late after coronary ligation into infarcted or remote myocardium. Circulation. (2008) 118:130–7. doi: 10.1161/CIRCULATIONAHA.107.757617
31. Mathieu M, Bartunek J, El Oumeiri B, Touihri K, Hadad I, Thoma P, et al. Cell therapy with autologous bone marrow mononuclear stem cells is associated with superior cardiac recovery compared with use of nonmodified mesenchymal stem cells in a canine model of chronic myocardial infarction. J Thorac Cardiovasc Surg. (2009) 138:646–53. doi: 10.1016/j.jtcvs.2008.12.031
32. Lai RC, Arslan F, Lee MM, Sze NS, Choo A, Chen TS, et al. Exosome secreted by MSC reduces myocardial ischemia/reperfusion injury. Stem Cell Res. (2010) 4:214–22. doi: 10.1016/j.scr.2009.12.003
33. Arslan F, Lai RC, Smeets MB, Akeroyd L, Choo A, Aguor EN, et al. Mesenchymal stem cell-derived exosomes increase ATP levels, decrease oxidative stress and activate PI3K/Akt pathway to enhance myocardial viability and prevent adverse remodeling after myocardial ischemia/reperfusion injury. Stem Cell Res. (2013) 10:301–12. doi: 10.1016/j.scr.2013.01.002
34. Goetze JP, Bruneau BG, Ramos HR, Ogawa T, de Bold MK, de Bold AJ. Cardiac natriuretic peptides. Nat Rev Cardiol. (2020) 17:698–717. doi: 10.1038/s41569-020-0381-0
35. Nishikimi T, Maeda N, Matsuoka H. The role of natriuretic peptides in cardioprotection. Cardiovasc Res. (2006) 69:318–28. doi: 10.1016/j.cardiores.2005.10.001
36. Bolli R, Mitrani RD, Hare JM, Pepine CJ, Perin EC, Willerson JT, et al. A phase II study of autologous mesenchymal stromal cells and c-kit positive cardiac cells, alone or in combination, in patients with ischaemic heart failure: the CCTRN CONCERT-HF trial. Eur J Heart Fail. (2021) 23:661–74. doi: 10.1002/ejhf.2178
Keywords: atrial appendage, autologous micrografts, cell therapy, coronary artery bypass surgery, epicardial cell delivery, ischemic heart failure
Citation: Nummi A, Mulari S, Stewart JA, Kivistö S, Teittinen K, Nieminen T, Lampinen M, Pätilä T, Sintonen H, Juvonen T, Kupari M, Suojaranta R, Kankuri E, Harjula A, Vento A and the AADC consortium (2021) Epicardial Transplantation of Autologous Cardiac Micrografts During Coronary Artery Bypass Surgery. Front. Cardiovasc. Med. 8:726889. doi: 10.3389/fcvm.2021.726889
Received: 19 June 2021; Accepted: 09 August 2021;
Published: 14 September 2021.
Edited by:
Salvatore Pepe, Royal Children's Hospital, AustraliaReviewed by:
Federico Quaini, University of Parma, ItalyTahir Yagdi, EGE University Department of Cardiovascular Surgery, Turkey
Copyright © 2021 Nummi, Mulari, Stewart, Kivistö, Teittinen, Nieminen, Lampinen, Pätilä, Sintonen, Juvonen, Kupari, Suojaranta, Kankuri, Harjula, Vento and the AADC consortium. This is an open-access article distributed under the terms of the Creative Commons Attribution License (CC BY). The use, distribution or reproduction in other forums is permitted, provided the original author(s) and the copyright owner(s) are credited and that the original publication in this journal is cited, in accordance with accepted academic practice. No use, distribution or reproduction is permitted which does not comply with these terms.
*Correspondence: Esko Kankuri, ZXNrby5rYW5rdXJpQGhlbHNpbmtpLmZp
†AADC consortium participant list provided as Supplementary Material