The Utility of Advanced Cardiovascular Imaging in Cancer Patients—When, Why, How, and the Latest Developments
- 1Nepean Clinical School, University of Sydney, University of Sydney, Sydney, NSW, Australia
- 2Cardiology Department, Nepean Hospital, Sydney, NSW, Australia
- 3Cardiology Department, Blacktown Hospital, Sydney, NSW, Australia
Cardio-oncology encompasses the risk stratification, prognostication, identification and management of cancer therapeutics related cardiac dysfunction (CTRCD). Cardiovascular imaging (CVI) plays a significant role in each of these scenarios and has broadened from predominantly quantifying left ventricular function (specifically ejection fraction) to the identification of earlier bio-signatures of CTRCD. Recent data also demonstrate the impact of chemotherapy on the right ventricle, left atrium and pericardium and highlight a possible role for CVI in the identification of CTRCD through tissue characterization and assessment of these cardiac chambers. This review aims to provide a contemporary perspective on the role of multi-modal advanced cardiac imaging in cardio-oncology.
Introduction
Heart disease and cancer are the two major causes of morbidity and mortality, accounting for over 70% of medically related deaths globally (1). The mortality risk due to cardiovascular complications is nearly four times higher in cancer patients compared to the general population (2). It is highest within the first year after cancer diagnosis and remains persistently elevated in cancer survivors even after the completion of treatment (2). The lifetime risk of cancer therapeutics related cardiac dysfunction (CTRCD) from cancer treatment can be increased up to 15-fold (3). Furthermore, the presence of cancer is independently associated with structural, functional, and tissue characteristic changes (4). Hence, cardiac risk stratification, identification of CTRCD due to cancer therapy and predicting cardiac recovery are important management goals in cancer patients. We define CTRCD for the purposes of this review as the direct effect of cancer treatment on the heart structure, function, and acceleration of coronary artery disease.
Cardiac imaging plays an important role in the diagnosis, management, and prognostication in patients with CTRCD (Supplementary Table 1). LVEF has been the most validated and commonly utilized parameter for the assessment of LV systolic function in CTRCD. However, the traditional approach of using left ventricular ejection fraction (LVEF) as a measure of CTRCD is now believed to be inadequate as changes in LVEF are a late manifestation of CTRCD. A desire to intervene earlier has led to a renewed focus on identifying early biosignatures of CTRCD which predate changes LVEF. This review aims to provide a contemporary perspective on the role of multi-modal cardiac imaging in the diagnosis and management of CTRCD.
Echocardiography
Baseline Cardiovascular Risk Stratification, Identification of CTRCD and Predicting Recovery
Echocardiography is the primary imaging modality of choice for baseline cardiac function assessment by American and European society consensus statements (5–9). The latest guidelines by the European Society of Medical Oncology (ESMO) have given a IA recommendation that all patients undergoing anticancer therapy associated with LV dysfunction should have a baseline LVEF assessment (5). Patients with impaired LVEF at baseline are at highest risk of cardiotoxicity from anticancer therapy.
While echocardiography is a good first line investigation due to its accessibility, low cost, and lack of any radiation, feasibility of high-quality echocardiographic imaging may also be limited by patient body habitus, radiation therapy, or recent surgery (e.g., mastectomy). In these situations, cardiac magnetic resonance imaging (CMR) or contrast echocardiography can overcome some of these imaging limitations and provide a more accurate assessment of LVEF (10).
Left Ventricle
Widely utilized standard 2D echocardiographic methods of LVEF assessment are based on geometrical assumptions which limits accuracy and reproducibility. Three-dimensional (3D) echocardiography overcomes these limitations and is currently recommended for the assessment of LV systolic function (7, 11). Recent developments which allow for semi-automated assessment of 3D LVEF in a clinical setting, have reduced temporal variability in LVEF measurements by improving intra- and interobserver variability and test-retest variability, which is important in CRTCD where serial evaluation of LV systolic function is needed. Despite these advances, the utility of LVEF in CTRCD is still limited since changes of <10 percentage points between examinations do not necessarily represent an actual change in systolic function (11). Furthermore, changes in LVEF are a late manifestation of CTRCD, hence LVEF has a low sensitivity for detecting early subclinical changes in LV function.
Speckle tracking echocardiography, which has been validated against sonomicrometry and tagged magnetic resonance imaging, has provided accurate angle-independent measurements of myocardial strain. Efforts to standardize myocardial deformation imaging has reduced the variability in this measure compared to other conventional echocardiographic measures of LV systolic function making it ideal for CTRCD (12). Global longitudinal strain (GLS) has been shown to detect LV dysfunction earlier than LVEF in patients receiving cancer therapy and has the potential to guide therapy (13). GLS has better inter and intra observer reproducibility than 2D LVEF by biplane method of disks emphasizing recent society statements that encourage the use of GLS and 3D LVEF in baseline echocardiographic assessments of cancer patients (see Figures 1A–C) (7, 14). GLS and 3D LVEF have the lowest temporal variability with respect to the detection of CRTCD. In a group of hematological cancer patients undergoing anthracycline therapy with normal LVEF, those with a baseline GLS <-17.5%, were associated with a six-times higher increase in cardiac death or symptomatic heart failure (15).
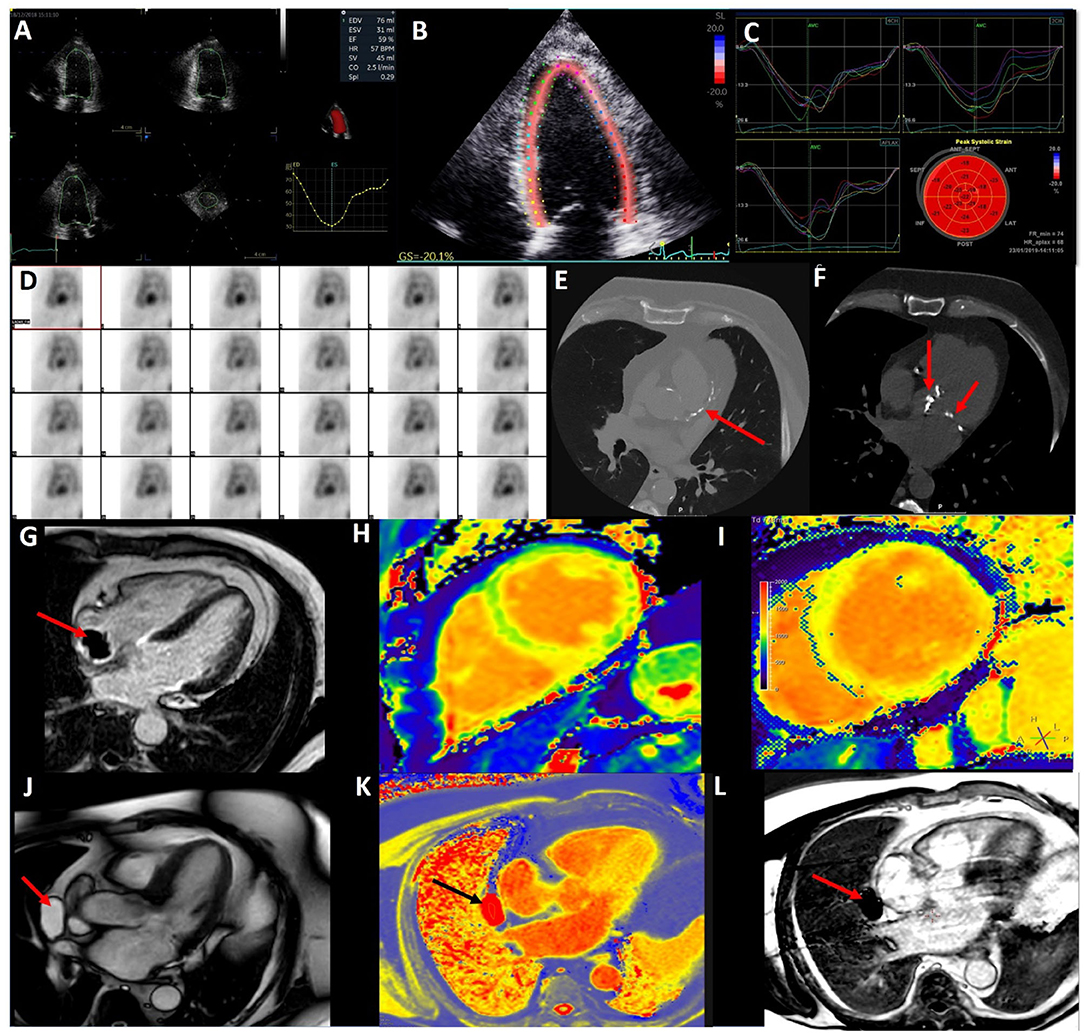
Figure 1. Multi-modal imaging in cardio-oncology. (A) 3D echocardiography to accurately calculate left ventricular volumes and ejection fraction. (B) 2D Speckle Tracking Echocardiography of the left ventricle (4 chamber view) for Global Longitudinal Strain. (C) 2D Left Ventricular Global Longitudinal Strain curves from 4, 2, and 3 chamber views. (D) Nuclear medicine—Multi-gated blood pool imaging to determine LVEF. (E) CT coronary angiogram demonstrating coronary artery calcium in the left anterior descending artery in a lymphoma survivor. (F) CT coronary angiogram demonstrating radiotherapy related aortic and mitral valve calcification in the same patient. (G) Cardiac Magnetic Resonance (CMR) late gadolinium enhancement with long T1 inversion time demonstrating a thrombus (red arrow) on the end of a Hickman's line in a cancer patient. (H) Normal CMR T1 map (green is normal myocardium). (I) T1 map showed elevated T1 times of the left ventricle in myocarditis. (J) CMR 3Ch cine demonstrating a pericardial mass (red arrow). (K) CMR T1 map highlight the pericardial mass is fill with fluid (black arrow). (L) CMR LGE with long T1 inversion time demonstrating mass (red arrow) is avascular with no enhancement.
The latest guidelines define CTRCD as a LVEF drop of ≥10% to a value below the lower limit of normal (<50%) (5, 16). Risk stratification is key to determining surveillance strategy, to ensure patients undergo optimal cancer therapy whilst minimizing the risk of CTRCD. High risk treatment factors are simultaneous doxorubicin and trastuzumab, high-dose doxorubicin (≥400 mg/m2 or equivalent), ≥30 Gy of radiotherapy to the chest involving the heart and tyrosine kinase inhibitors following doxorubicin chemotherapy (9). High risk patients are those with underlying cardiovascular disease, numerous cardiac risk factors, age ≥65 years, impaired LV function and previous cardiotoxic therapy (9). Those that are high risk should have imaging surveillance every two cycles or every cycle above 240 mg/m2 of doxorubicin or equivalent (9). In regards to frequency of serial echocardiograms, it is dependent on the type and dose of anticancer agent as well as symptoms (5).
The value of imaging surveillance and risk stratification was highlighted in patients treated with anthracyclines and HER2 inhibitors, with a decline in GLS reported as early as 3 months after the initiation of trastuzumab in the adjuvant setting (13, 17–19). GLS has a good prognostic ability for detecting CTRCD and the latest guidance stipulate a 12% relative decrease or a ≥5% absolute decrease in GLS with normal LVEF should trigger the treating physician to consider cardioprotective therapy and a repeat LVEF and strain measurement in 3 months if asymptomatic (Figure 2) (5). Cardioprotective therapy consists of angiotensin converting enzyme inhibitors (ACEi), beta blockers (BB) and dexrazoxane (5). Recently the Strain Surveillance of Chemotherapy for Improving Cardiovascular Outcomes (SUCCOR) study published its 1-year data (20). Though the SUCCOR primary outcome of change in LVEF was not significantly different between a GLS and 2D LVEF treatment strategy (p = 0.05), patients in the GLS arm had higher cardioprotective therapy rates and fewer developed CTRCD (6 vs. 14%; p = 0.02) (20).
CTRCD recovery is dependent on early detection. Those who start treatment with a cardioprotective agent(s) within 2 months of diagnosis have a better chance of LV function recovery (21). Additionally, those that start CTRCD treatment with a lower LVEF may have a lower chance of recovery in LVEF (22). In an anthracycline and trastuzumab breast cancer cohort, patients who had reversible CTRCD had higher nadir GLS compared to those with irreversible CTRCD (−17 vs. −11.7%, respectively) (23). This emphasizes the importance of cardiac imaging and the need for improved and better imaging parameters in CTRCD. A future potential echocardiographic parament is 3D LV strain. It includes no through-plane motion of speckles and the ability to track speckles in 3D space. This permits the calculation of circumferential, radial, and longitudinal strain in one measurement. Though an exciting prospect, more studies are needed in this area.
Left Ventricular Diastolic Function
Baseline LV diastolic function is not predictive of CTRCD and there is limited evidence on it predicting CTRCD (24). The largest prospective study to date demonstrated a worsening in diastolic function from baseline is associated with a 1.4% decrease in LVEF from baseline with a 2.2 times increased risk of developing CTRCD (24).
Right Ventricle
Though still limited by small studies, quantitative assessment of the RV size and function is recommended as RV dysfunction can occur during cancer treatment (7). In addition to standard parameters, 2D RV strain is recommended in the latest joint society guidelines (25). The data on RV strain in CTRCD suggests RV dysfunction frequently occurs with LV dysfunction and pertains a reduced recovery in LV function, though further studies are needed (26). Additionally, other studies suggest 2D and 3D RV strain deterioration occurs prior to LV parameters (27, 28).
Pumonary Hypertension
Tyrosine Kinase Inhibitors (TKI) (e.g., dasatinib) are associated with pulmonary hypertension, though the incidence is difficult to estimate because of lack of screening data due to asymptomatic study participants and overall small study sizes. A position statement suggests 3–6 monthly ECHOs in asymptomatic patients on anticancer agents that can cause pulmonary hypertension such as TKIs (16). Dasatinib induced pulmonary hypertension is often reversible, but not to normal baseline pulmonary pressures highlighting the importance of identifying a better method to find at risk patients earlier (29).
Stress Echocardiography
The role of stress echocardiography is potentially useful in risk stratifying patients undergoing cancer therapies associated with ischemia. This includes antimetabolites (5-FU), VEGF inhibitors and TKIs (30). The advantage of exercise or pharmacological stress echocardiography is no radiation, high feasibility, and low cost. The role of stress echocardiography beyond ischemia testing to identify CTRCD using parameters such as diastolic dysfunction is inconclusive (31, 32).
Left Atrium
Aspects of the left atrium that have been investigated include baseline left atrial volume index and left atrial longitudinal strain (33, 34). Bergamini et al. found that a dilated left atrium with normal LV function on baseline echocardiography prior to adjuvant or neoadjuvant traztuzumab is associated with the development of CTRCD in patient receiving trastuzumab (33). Park et al. found peak atrial longitudinal strain decline at the end of chemotherapy could predict CTRCD with better sensitivity and specificity than LV GLS (34).
Long-Term Cardiac Complications in Cancer Survivors
Long-term cardiac surveillance is recommended post completion of chemotherapy (9). In low to medium risk patients, a 12 months post final treatment echocardiogram is recommended with 5-yearly reviews if asymptomatic (9). In high risk groups 6 and 12 months post final cycle review followed by annually for 2–3 years is initially recommended (9). In patients with symptomatic CTRCD annual review with echocardiography is recommended (7). Female survivors of childbearing age who have had cardiotoxic therapy/chest radiotherapy should have a cardiology consultation prior to pregnancy with echocardiographic surveillance performed in the first trimester (35). It is important to note, 10–20 years post anthracyclines therapy nearly 50% of patients will show evidence of CTRCD and experience reduced quality of life and a mortality similar to dilated cardiomyopathy patients (36–38). Reassuringly, modern anthracycline regimes appear to have a lower impact on LVEF (39).
A collection of echocardiographic parameters rather than solely LVEF maybe important in identifying at risk patients, as the prevalence of abnormal GLS and diastolic function is higher in a cancer survivor cohort (40). This is supported by another long-term childhood cancer survivor cohort, which demonstrated significantly impaired RV function (41).
Cardiac Magnetic Resonance
Baseline Cardiovascular Risk Stratification
CMR is the gold standard for measuring left and right ventricular volume and function (42, 43). However, its major limitation is it is high cost and lack of availability relative to echocardiography (44).
The main indication in the current cardio-oncology guidelines for CMR is when there is suboptimal image acquisition and it is preferred over nuclear imaging (6, 7, 16). A key strength of CMR is in the assessment of cardiac masses and inflammatory conditions such as myocarditis, pericarditis, and myopericarditis. This is due to its advantages of multi-planar image acquisition, high spatial resolution, a large field of view, and tissue characterization (45). Tumors and thrombi can be easily differentiated with difference CMR sequence. Tumors tend to be hyperintensity on T2-weighed turbo spin echo, contrast first pass perfusion, and late gadolinium enhancement (LGE), whereas thrombi are hyperintensity with short T1 and hypointensity with long T1 times (see Figure 1G) (46).
Using the forementioned CMR sequences, CMR has a high accuracy for discriminating between benign and malignant lesions, with a good interobserver agreement (47). Figures 1J–L show a pericardial cyst, identified by different CMR techniques. The differentials for the cyst are a pericardial diverticulum and mediastinal mass.
The role of CMRs in cardio-oncology from current patients to survivors is likely to increase given its high reproducibility and lack of ionizing radiation. This may be enhanced with the potential development of a limited 10 min CMR examination focusing on volumes and function (48). This would invariable lower the cost and increase the availability of CMR.
Identification of CTRCD and Predicting Recovery
Though CMR main role in baseline assessment is limited, its versatility makes it an important imaging modality in the identification of CTRCD.
Cardiac Dysfunction
The definition of CTRCD is based on a LVEF decline of >10%, reiterating the importance of accurate and reproducible imaging. CMR is superior to 2D echocardiography in identifying LV dysfunction as its volumes are not based on geometric assumptions and less prone to suboptimal imaging (49). Furthermore, it has superior reproducibility compared to echocardiography (43).
CMR can also measure myocardial strain. The data on CMR myocardial strain correlates with a decline in LVEF, however its prognostic ability has not been assessed to the extent it has been in echocardiography (50–53).
Late Gadolinium Enhancement
The composition of the extracellular matrix is altered in myocardial fibrosis. This structural change allow gadolinium to accumulate in areas of replacement fibrosis. On T1-weighted sequences regions of gadolinium accumulation appear hyperintense (bright) in contrast to healthy myocardium, which appear dark. The data on the utility of LGE in CRTCD is conflicting. The majority of short term (<6 months) studies have not reported any LGE (51, 53, 54). Longer term follow up studies document LGE incidence of 5–19% (55, 56). The largest and most recent study to date on LGE and anthracyclines +/- trastuzumab identified a LGE incidence of 10% with an alternative cause for LGE identified in nearly all cases, calling into question the value of LGE in identifying CRTCD secondary to anthracyclines and/or trastuzumab (57).
Tissue Characterization
The utility of tissue characterization has becoming increasingly popular over the last decade with the advent of validated software enabling the quantification of T1, T2 mapping, and extracellular volume (ECV) fraction estimation. T1 mapping allows us to detect a range of diffuse pathologies including myocardial fibrosis, myocarditis, cardiac amyloidosis, key aspects of cardio-oncology as well as other pathologies such as storage disorders. T1 measures the longitudinal time to equilibrium post a radiofrequency pulse. The commonest technique to acquire a T1 map is the modified Look-Locker pulse sequence (MOLLI) or “shortened” version known as the shMOLLI (58). Though derived T1 values, the main advantage of these techniques are reduced acquisition time and the shMOLLI in particularly has shorter breath-holds. Native T1 mapping refers to the acquisition of a T1 map without contrast (Figures 1H,I). It is important to note T1 values vary from scanner to scanner and tesla strength (59). The literature on T1 mapping identifying CTRCD are conflicting and limited with the focus being on survivors. The three human studies to date show differing changes in T1 value shortly post anthracycline exposure, with two showing increases in T1 values whilst the other identified a decrease in T1 at 48 h post anthracycline as predictive of CTRCD (54, 60, 61).
ECV is an additive tool in the assessment of myocardial fibrosis assessing interstitial fibrosis. ECV requires a pre- and post-contrast T1 map as well as the subject's hematocrit to calculate the ECV fraction. Normal values are between 20 and 26%, with it being slightly higher in women and similar between 1.5T and 3T scanners (59, 62, 63). There are only two human studies to date assessing ECV changes during chemotherapy with one showing a significant temporal change in those developing CTRCD whilst the other showed no significant changes in ECV fraction (54, 61). Furthermore, the temporal variability of T1 mapping and ECV was comparable to those with no CTRCD (61).
T2 measures the transverse time to equilibrium post a radiofrequency pulse. T2 mapping is used in the identification of myocardial oedema, which can occur in myocarditis to infarction to cardiomyopathy. Its use along with T1 mapping is supported in myocardial inflammation recommendations (64). The data on T2 is limited to two human studies with conflicting results (54, 61).
The data on T1 time and ECV in cancer survivors are heterogenous. Increases in T1 and ECV correlated to cumulative dose, reduced exercise capacity and myocardial wall thinning in cancer survivors whom received anthracycline and were at least 7 years in remission (65). Additionally, the ECV fraction is further increased in those with reduced LVEF (66). However, other studies have found no significant increase in native T1 and ECV in childhood cancer survivors (67, 68). The role of tissue characterization in CTRCD requires further studies given the limited and conflicting results to date.
Myocarditis
Though immune checkpoint inhibitor (ICI) related myocarditis is rare, its frequency will increase with increased ICI usage. CMR is a valuable non-invasive diagnostic tool in the assessment of myocarditis with the Lake Louise criteria and the addition of T1 and T2 maps (Figure 1I) (64). Its role has been supported in the workup of ICI myocarditis (69). The largest study to date, a registry of 136 ICI myocarditis patients showed abnormal T1 values were associated with more symptoms, lower cardiac function (70). Furthermore, higher T1 values had independent prognostic value for the subsequent development of major adverse cardiac events (70). Elevated T1 times were commoner than elevated T2 times at 78 and 43% of patients, respectively, however all patients met the modified Lake Louise criteria (70).
Computer Tomography
Baseline Cardiovascular Risk Stratification
The calcium artery calcium (CAC) score can be determined on non-gated non-contrast CT chest scans performed for staging and assessment of the primary malignancy, this CAC should be reported as per guidelines (71). The concept of higher CAC score correlates with higher acute coronary events has been reaffirmed in the lung cancer screening and breast cancer populations (72, 73). A recent study using an automated CAC algorithm in >14,000 breast cancer patients has demonstrated a CAC score >400 is associated with a five times higher risk of cardiovascular death compared to a zero CAC (74). Though the role of CT is limited in cardio-oncology guidelines, CT coronary angiography may serve as an alternative imaging modality to stress echocardiography in a baseline assessment, though may be limited by its higher cost and radiation exposure.
Long-Term Cardiac Complications in Cancer Survivors
Coronary Artery Disease
Complications of radiotherapy include accelerated atherosclerosis (75). Effects are often identified in the medium to long term. There is a linear radiation dose to risk of CAD relationship, with the excess relative risk of CAD per mean heart Gray dose being 7% (76). Whether radiotherapy leads to an increase visible CAC on CT is contested (77, 78). Figure 1E illustrates extensive CAC in a lymphoma survivor. In regard to acute coronary syndrome, the main risk factor is the volume of LV receiving 5Gy and for each Gy the cumulative incidence increase of acute coronary syndrome is 17% (78). Despite advances in radiotherapy technology and techniques to minimize cardiac complications, close surveillance of radiotherapy therapy patients is warranted 5–10 years post radiotherapy (79).
Valvular Disease
Radiotherapy can impact the valvular apparatus causing thickening, fibrosis, and significant valvular heart disease (75). The risk of valvular disease from radiotherapy is 34 times higher and occurs in the second decade post treatment (80). The role of CT has been elevated with ability to assess of aortic valve calcium scoring (81). Figure 1F illustrates an example of aortic and mitral valve calcification post radiotherapy in a lymphoma survivor. Radiotherapy patients experience mediastinal damage, such as mediastinal fibrosis and porcelain aorta, which can make cardiac surgery more complicated.
Pericardial Disease
Acute and chronic pericarditis are side effects often associated with mediastinal radiotherapy, though can also occur with chemotherapy (75). Their incidence is related to the cumulative radiation dose. The incidence of radiation induced pericarditis has significantly decreased to 2.5% due to advances in radiotherapy techniques and shielding methods (82). In chronic pericarditis calcification of the pericardium may occur. CT is the ideal modality for assessing pericardial calcification and thickening due to its excellent spatial resolution (83).
Nuclear Imaging
Multi-gated blood pool imaging (MUGA) was the first imaging modality to be used in cardio-oncology (84). Its primary role historically has been in the measurement of the LVEF (see Figure 1D). MUGA's LVEF measurement was reported superior to 2D echocardiography in 1980's, however, is inferior to CMR (85, 86). Its limitation is the relatively high radiation exposure (5–10 mSV) and the inability to assess other cardiac parameters, has led to a decline in its usage (7). However, there is a role for 18F fluorodeoxyglucose (18FDG) positron emission tomography (PET)-CT scans particularly in the diagnosis and staging of cancer patients. Inflammation is associated with both cancer and cardiovascular disease. 18FDG-PET can identify cardiac inflammation within atherosclerotic plaque as well as myocardial tissue and valves (87, 88). Retrospective data in patients who have received doxorubicin and a subsequent increase in LV 18FDG uptake, are associated with a decline in LVEF (89). This increased uptake suggests there may be a myocardial inflammation component to CTRCD and warrants further investigation despite its high cost and lower availability. With the increasing use of ICIs myocardial inflammation will become an increasing problem. The limited evidence to date indicates 18FDG-PET does not identify ICI related atherosclerosis, however further investigation is warranted, potentially with more specific tracers for vascular inflammation (87, 90).
Future Directions
All cardiac imaging modalities have a promising role in the future of cardio-oncology. Areas of particular interest that may rise to prominence, are advanced echocardiographic assessment of cardiac structures in addition to the left ventricle in conjunction with the use of 3D volumes and myocardial deformation indices over standard 2D echocardiography as described earlier. Similarly, the utility of diffusion tensor CMR can increase due to its ability to assess myocardial microstructure, such as, cardiomyocyte and sheetlet-level in vivo (91). As CT technology and techniques evolve, CT may potentially play a more significant role in tissue characterization such as ECV as well as chamber volume assessments should radiation doses reduce sufficiently (92, 93). Despite nuclear imaging's higher radiation dose, it will continue to play an important role in certain subsets of cancer patients where it can be utilized to monitor patients' baseline cancer stage and progression. Incorporating more cardiac specific parameters such as LV 18FDG uptake may identify new CTRCD markers at no inconvenience to patients. Lastly, contrast-enhanced ultrasound (CEU) molecular imaging could become a novel imaging modality in cardio-oncology. CEU can track temporal and spatial changes in tissues and the vasculature, which is important, as anthracyclines can damage the myocardial vascular bed by labeling molecular markers with specific antibodies (94, 95). Though this is currently limited to research, the transition to clinical use warrants monitoring.
Conclusion
The field of cardio-oncology has evolved rapidly over the last couple of decades. Cardiac imaging has an integral role in this specialty and advances in imaging techniques allow clinicians to identify at risk patients earlier. Each imaging modality has its pros and cons with no sole technique superior in all domains of cardiac imaging. Thus, the key to a successful cardio-oncology patient journey involves a multi-modal approach so that patients can hopefully complete their optimal cancer therapy with minimal disruption to their cardiac health.
Author Contributions
All authors have contributed to the planning, writing, and reviewing of the manuscript.
Funding
KN was supported by a Fellowship (Award Reference No. 101868) from the National Heart Foundation of Australia. CY was supported by the National Health Medical Research Council (Australia) and National Breast Cancer Foundation (Australia) postgraduate scholarship (Award Reference Numbers: APP2002728 and DS-21-02).
Conflict of Interest
The authors declare that the research was conducted in the absence of any commercial or financial relationships that could be construed as a potential conflict of interest.
Publisher's Note
All claims expressed in this article are solely those of the authors and do not necessarily represent those of their affiliated organizations, or those of the publisher, the editors and the reviewers. Any product that may be evaluated in this article, or claim that may be made by its manufacturer, is not guaranteed or endorsed by the publisher.
Supplementary Material
The Supplementary Material for this article can be found online at: https://www.frontiersin.org/articles/10.3389/fcvm.2021.728215/full#supplementary-material
References
1. Jones DS, Podolsky SH, Greene JA. The burden of disease and the changing task of medicine. N Engl J Med. (2012) 366:2333–8. doi: 10.1056/NEJMp1113569
2. Sturgeon KM, Deng L, Bluethmann SM, Zhou S, Trifiletti DM, Jiang C, et al. A population-based study of cardiovascular disease mortality risk in US cancer patients. Eur Heart J. (2019) 40:3889–97. doi: 10.1093/eurheartj/ehz766
3. Armenian SH, Gelehrter SK, Chow EJ. Strategies to prevent anthracycline-related congestive heart failure in survivors of childhood cancer. Cardiol Res Pract. (2012) 2012:713294. doi: 10.1155/2012/713294
4. Labib D, Satriano A, Dykstra S, Hansen R, Mikami Y, Guzzardi DG, et al. Effect of active cancer on the cardiac phenotype: a cardiac magnetic resonance imaging-based study of myocardial tissue health and deformation in patients with chemotherapy-naive cancer. J Am Heart Assoc. (2021) 10:e019811. doi: 10.1161/JAHA.120.019811
5. Curigliano G, Lenihan D, Fradley M, Ganatra S, Barac A, Blaes A, et al. Management of cardiac disease in cancer patients throughout oncological treatment: ESMO consensus recommendations. Ann Oncol. (2020) 31:171–90. doi: 10.1016/j.annonc.2019.10.023
6. Armenian SH, Lacchetti C, Barac A, Carver J, Constine LS, Denduluri N, et al. Prevention and monitoring of cardiac dysfunction in survivors of adult cancers: American society of clinical oncology clinical practice guideline. J Clin Oncol. (2017) 35:893–911. doi: 10.1200/JCO.2016.70.5400
7. Plana JC, Galderisi M, Barac A, Ewer MS, Ky B, Scherrer-Crosbie M, et al. Expert consensus for multimodality imaging evaluation of adult patients during and after cancer therapy: a report from the American society of echocardiography and the European association of cardiovascular imaging. J Am Soc Echocardiogr. (2014) 27:911–39. doi: 10.1016/j.echo.2014.07.012
8. Eschenhagen T, Force T, Ewer MS, de Keulenaer GW, Suter TM, Anker SD, et al. Cardiovascular side effects of cancer therapies: a position statement from the heart failure association of the European society of cardiology. Eur J Heart Fail. (2011) 13:1–10. doi: 10.1093/eurjhf/hfq213
9. Celutkiene J, Pudil R, Lopez-Fernandez T, Grapsa J, Nihoyannopoulos P, Bergler-Klein J, et al. Role of cardiovascular imaging in cancer patients receiving cardiotoxic therapies: a position statement on behalf of the Heart Failure Association (HFA), the European Association of Cardiovascular Imaging (EACVI) and the Cardio-Oncology Council of the European Society of Cardiology (ESC). Eur J Heart Fail. (2020) 22:1504–24. doi: 10.1002/ejhf.1957
10. Senior R, Becher H, Monaghan M, Agati L, Zamorano J, Vanoverschelde JL, et al. Clinical practice of contrast echocardiography: recommendation by the European Association of Cardiovascular Imaging (EACVI) (2017). Eur Heart J Cardiovasc Imaging. (2017) 18:1205. doi: 10.1093/ehjci/jex182
11. Thavendiranathan P, Grant AD, Negishi T, Plana JC, Popovic ZB, Marwick TH. Reproducibility of echocardiographic techniques for sequential assessment of left ventricular ejection fraction and volumes: application to patients undergoing cancer chemotherapy. J Am Coll Cardiol. (2013) 61:77–84. doi: 10.1016/j.jacc.2012.09.035
12. Voigt JU, Pedrizzetti G, Lysyansky P, Marwick TH, Houle H, Baumann R, et al. Definitions for a common standard for 2D speckle tracking echocardiography: consensus document of the EACVI/ASE/Industry Task Force to standardize deformation imaging. Eur Heart J Cardiovasc Imaging. (2015) 16:1–11. doi: 10.1093/ehjci/jeu184
13. Negishi K, Negishi T, Hare JL, Haluska BA, Plana JC, Marwick TH. Independent and incremental value of deformation indices for prediction of trastuzumab-induced cardiotoxicity. J Am Soc Echocardiogr. (2013) 26:493–8. doi: 10.1016/j.echo.2013.02.008
14. Lambert J, Lamacie M, Thampinathan B, Altaha MA, Esmaeilzadeh M, Nolan M, et al. Variability in echocardiography and MRI for detection of cancer therapy cardiotoxicity. Heart. (2020) 106:817–23. doi: 10.1136/heartjnl-2019-316297
15. Ali MT, Yucel E, Bouras S, Wang L, Fei HW, Halpern EF, et al. Myocardial strain is associated with adverse clinical cardiac events in patients treated with anthracyclines. J Am Soc Echocardiogr. (2016) 29:522–7.e3. doi: 10.1016/j.echo.2016.02.018
16. Zamorano JL, Lancellotti P, Rodriguez Munoz D, Aboyans V, Asteggiano R, Galderisi M, et al. 2016 ESC Position Paper on cancer treatments and cardiovascular toxicity developed under the auspices of the ESC Committee for Practice Guidelines: The Task Force for cancer treatments and cardiovascular toxicity of the European Society of Cardiology (ESC). Eur Heart J. (2016) 37:2768–801. doi: 10.1093/eurheartj/ehw211
17. Negishi K, Negishi T, Haluska BA, Hare JL, Plana JC, Marwick TH. Use of speckle strain to assess left ventricular responses to cardiotoxic chemotherapy and cardioprotection. Eur Heart J Cardiovasc Imaging. (2014) 15:324–31. doi: 10.1093/ehjci/jet159
18. Fallah-Rad N, Walker JR, Wassef A, Lytwyn M, Bohonis S, Fang T, et al. The utility of cardiac biomarkers, tissue velocity and strain imaging, and cardiac magnetic resonance imaging in predicting early left ventricular dysfunction in patients with human epidermal growth factor receptor II-positive breast cancer treated with adjuvant trastuzumab therapy. J Am Coll Cardiol. (2011) 57:2263–70. doi: 10.1016/j.jacc.2010.11.063
19. Hare JL, Brown JK, Leano R, Jenkins C, Woodward N, Marwick TH. Use of myocardial deformation imaging to detect preclinical myocardial dysfunction before conventional measures in patients undergoing breast cancer treatment with trastuzumab. Am Heart J. (2009) 158:294–301. doi: 10.1016/j.ahj.2009.05.031
20. Thavendiranathan P, Negishi T, Somerset E, Negishi K, Penicka M, Lemieux J, et al. Strain-guided management of potentially cardiotoxic cancer therapy. J Am Coll Cardiol. (2021) 77:392–401. doi: 10.1016/j.jacc.2020.11.020
21. Cardinale D, Colombo A, Lamantia G, Colombo N, Civelli M, De Giacomi G, et al. Anthracycline-induced cardiomyopathy: clinical relevance and response to pharmacologic therapy. J Am Coll Cardiol. (2010) 55:213–20. doi: 10.1016/j.jacc.2009.03.095
22. Cardinale D, Colombo A, Bacchiani G, Tedeschi I, Meroni CA, Veglia F, et al. Early detection of anthracycline cardiotoxicity and improvement with heart failure therapy. Circulation. (2015) 131:1981–8. doi: 10.1161/CIRCULATIONAHA.114.013777
23. Fei HW, Ali MT, Tan TC, Cheng KH, Salama L, Hua L, et al. Left ventricular global longitudinal strain in HER-2 + breast cancer patients treated with anthracyclines and Trastuzumab who develop cardiotoxicity is associated with subsequent recovery of left ventricular ejection fraction. Echocardiography. (2016) 33:519–26. doi: 10.1111/echo.13168
24. Upshaw JN, Finkelman B, Hubbard RA, Smith AM, Narayan HK, Arndt L, et al. Comprehensive assessment of changes in left ventricular diastolic function with contemporary breast cancer therapy. JACC Cardiovasc Imaging. (2020) 13:198–210. doi: 10.1016/j.jcmg.2019.07.018
25. Lang RM, Badano LP, Mor-Avi V, Afilalo J, Armstrong A, Ernande L, et al. Recommendations for cardiac chamber quantification by echocardiography in adults: an update from the American Society of Echocardiography and the European Association of Cardiovascular Imaging. J Am Soc Echocardiogr. (2015) 28:1–39.e14. doi: 10.1016/j.echo.2014.10.003
26. Calleja A, Poulin F, Khorolsky C, Shariat M, Bedard PL, Amir E, et al. Right ventricular dysfunction in patients experiencing cardiotoxicity during breast cancer therapy. J Oncol. (2015) 2015:609194. doi: 10.1155/2015/609194
27. Zhao R, Shu F, Zhang C, Song F, Xu Y, Guo Y, et al. Early detection and prediction of anthracycline-induced right ventricular cardiotoxicity by 3-dimensional echocardiography. JACC: CardioOncology. (2020) 2:13–22. doi: 10.1016/j.jaccao.2020.01.007
28. Planek MIC, Manshad A, Hein K, Hemu M, Ballout F, Varandani R, et al. Prediction of doxorubicin cardiotoxicity by early detection of subclinical right ventricular dysfunction. Cardiooncology. (2020) 6:10. doi: 10.1186/s40959-020-00066-8
29. Montani D, Bergot E, Gunther S, Savale L, Bergeron A, Bourdin A, et al. Pulmonary arterial hypertension in patients treated by dasatinib. Circulation. (2012) 125:2128–37. doi: 10.1161/CIRCULATIONAHA.111.079921
30. Herrmann J. Adverse cardiac effects of cancer therapies: cardiotoxicity and arrhythmia. Nat Rev Cardiol. (2020) 17:474–502. doi: 10.1038/s41569-020-0348-1
31. Civelli M, Cardinale D, Martinoni A, Lamantia G, Colombo N, Colombo A, et al. Early reduction in left ventricular contractile reserve detected by dobutamine stress echo predicts high-dose chemotherapy-induced cardiac toxicity. Int J Cardiol. (2006) 111:120–6. doi: 10.1016/j.ijcard.2005.07.029
32. Lanzarini L, Bossi G, Laudisa ML, Klersy C, Arico M. Lack of clinically significant cardiac dysfunction during intermediate dobutamine doses in long-term childhood cancer survivors exposed to anthracyclines. Am Heart J. (2000) 140:315–23. doi: 10.1067/mhj.2000.108237
33. Bergamini C, Dolci G, Rossi A, Torelli F, Ghiselli L, Trevisani L, et al. Left atrial volume in patients with HER2-positive breast cancer: One step further to predict trastuzumab-related cardiotoxicity. Clin Cardiol. (2018) 41:349–53. doi: 10.1002/clc.22872
34. Park H, Kim KH, Kim HY, Cho JY, Yoon HJ, Hong YJ, et al. Left atrial longitudinal strain as a predictor of Cancer therapeutics-related cardiac dysfunction in patients with breast Cancer. Cardiovasc Ultrasound. (2020) 18:28. doi: 10.1186/s12947-020-00210-5
35. Armenian SH, Hudson MM, Mulder RL, Chen MH, Constine LS, Dwyer M, et al. Recommendations for cardiomyopathy surveillance for survivors of childhood cancer: a report from the international late effects of childhood cancer guideline harmonization group. Lancet Oncol. (2015) 16:e123–36. doi: 10.1016/S1470-2045(14)70409-7
36. Fornaro A, Olivotto I, Rigacci L, Ciaccheri M, Tomberli B, Ferrantini C, et al. Comparison of long-term outcome in anthracycline-related versus idiopathic dilated cardiomyopathy: a single centre experience. Eur J Heart Fail. (2018) 20:898–906. doi: 10.1002/ejhf.1049
37. Harrison JM, Davis MA, Barton DL, Janz NK, Pressler SJ, Friese CR. Functional status and quality of life among breast cancer survivors with heart failure: results of the Medicare Health Outcomes Survey. Support Care Cancer. (2017) 25:2463–73. doi: 10.1007/s00520-017-3653-4
38. Pinder MC, Duan Z, Goodwin JS, Hortobagyi GN, Giordano SH. Congestive heart failure in older women treated with adjuvant anthracycline chemotherapy for breast cancer. J Clin Oncol. (2007) 25:3808–15. doi: 10.1200/JCO.2006.10.4976
39. Jeyaprakash P, Sangha S, Ellenberger K, Sivapathan S, Pathan F, Negishi K. Cardiotoxic effect of modern anthracycline dosing on left ventricular ejection fraction: a systematic review and meta-analysis of placebo arms from randomized controlled trials. J Am Heart Assoc. (2021) 10:e018802. doi: 10.1161/JAHA.120.018802
40. Armstrong GT, Joshi VM, Ness KK, Marwick TH, Zhang N, Srivastava D, et al. Comprehensive echocardiographic detection of treatment-related cardiac dysfunction in adult survivors of childhood cancer: results from the St. Jude lifetime cohort study. J Am Coll Cardiol. (2015) 65:2511–22. doi: 10.1016/j.jacc.2015.04.013
41. Christiansen JR, Massey R, Dalen H, Kanellopoulos A, Hamre H, Ruud E, et al. Right ventricular function in long-term adult survivors of childhood lymphoma and acute lymphoblastic leukaemia. Eur Heart J Cardiovasc Imaging. (2016) 17:735–41. doi: 10.1093/ehjci/jew018
42. Grothues F, Moon JC, Bellenger NG, Smith GS, Klein HU, Pennell DJ. Interstudy reproducibility of right ventricular volumes, function, and mass with cardiovascular magnetic resonance. Am Heart J. (2004) 147:218–23. doi: 10.1016/j.ahj.2003.10.005
43. Grothues F, Smith GC, Moon JC, Bellenger NG, Collins P, Klein HU, et al. Comparison of interstudy reproducibility of cardiovascular magnetic resonance with two-dimensional echocardiography in normal subjects and in patients with heart failure or left ventricular hypertrophy. Am J Cardiol. (2002) 90:29–34. doi: 10.1016/S0002-9149(02)02381-0
44. Armstrong AC, Gidding S, Gjesdal O, Wu C, Bluemke DA, Lima JA. LV mass assessed by echocardiography and CMR, cardiovascular outcomes, and medical practice. JACC Cardiovasc Imaging. (2012) 5:837–48. doi: 10.1016/j.jcmg.2012.06.003
45. American College of Cardiology Foundation Task Force on Expert, Consensus D, Hundley WG, Bluemke DA, Finn JP, Flamm SD, Fogel MA. ACCF/ACR/AHA/NASCI/SCMR 2010 expert consensus document on cardiovascular magnetic resonance: a report of the American college of cardiology foundation task force on expert consensus documents. J Am Coll Cardiol. (2010) 55:2614–62. doi: 10.1016/j.jacc.2009.11.011
46. Pazos-Lopez P, Pozo E, Siqueira ME, Garcia-Lunar I, Cham M, Jacobi A, et al. Value of CMR for the differential diagnosis of cardiac masses. JACC Cardiovasc Imaging. (2014) 7:896–905. doi: 10.1016/j.jcmg.2014.05.009
47. Mousavi N, Cheezum MK, Aghayev A, Padera R, Vita T, Steigner M, et al. Assessment of cardiac masses by cardiac magnetic resonance imaging: histological correlation and clinical outcomes. J Am Heart Assoc. (2019) 8:e007829. doi: 10.1161/JAHA.117.007829
48. Friedrich MG. A 10-Minute Cardiovascular Magnetic Resonance Protocol for Cardiac Disease. (2020). Available online at: https://clinicaltrials.gov/ct2/show/study/NCT04464655
49. Armstrong GT, Plana JC, Zhang N, Srivastava D, Green DM, Ness KK, et al. Screening adult survivors of childhood cancer for cardiomyopathy: comparison of echocardiography and cardiac magnetic resonance imaging. J Clin Oncol. (2012) 30:2876–84. doi: 10.1200/JCO.2011.40.3584
50. Ong G, Brezden-Masley C, Dhir V, Deva DP, Chan KKW, Chow CM, et al. Myocardial strain imaging by cardiac magnetic resonance for detection of subclinical myocardial dysfunction in breast cancer patients receiving trastuzumab and chemotherapy. Int J Cardiol. (2018) 261:228–33. doi: 10.1016/j.ijcard.2018.03.041
51. Drafts BC, Twomley KM, D'Agostino R Jr., Lawrence J, Avis N, et al. Low to moderate dose anthracycline-based chemotherapy is associated with early noninvasive imaging evidence of subclinical cardiovascular disease. JACC Cardiovasc Imaging. (2013) 6:877–85. doi: 10.1016/j.jcmg.2012.11.017
52. Jordan JH, Sukpraphrute B, Melendez GC, Jolly MP, D'Agostino RB, et al. Early myocardial strain changes during potentially cardiotoxic chemotherapy may occur as a result of reductions in left ventricular end-diastolic volume: the need to interpret left ventricular strain with volumes. Circulation. (2017) 135:2575–7. doi: 10.1161/CIRCULATIONAHA.117.027930
53. Nakano S, Takahashi M, Kimura F, Senoo T, Saeki T, Ueda S, et al. Cardiac magnetic resonance imaging-based myocardial strain study for evaluation of cardiotoxicity in breast cancer patients treated with trastuzumab: A pilot study to evaluate the feasibility of the method. Cardiol J. (2016) 23:270–80. doi: 10.5603/CJ.a2016.0023
54. Muehlberg F, Funk S, Zange L, von Knobelsdorff-Brenkenhoff F, Blaszczyk E, Schulz A, et al. Native myocardial T1 time can predict development of subsequent anthracycline-induced cardiomyopathy. ESC Heart Fail. (2018) 5:620–9. doi: 10.1002/ehf2.12277
55. Neilan TG, Coelho-Filho OR, Pena-Herrera D, Shah RV, Jerosch-Herold M, Francis SA. Left ventricular mass in patients with a cardiomyopathy after treatment with anthracyclines. Am J Cardiol. (2012) 110:1679–86. doi: 10.1016/j.amjcard.2012.07.040
56. Harries I, Biglino G, Baritussio A, De Garate E, Dastidar A, Plana JC, et al. Long term cardiovascular magnetic resonance phenotyping of anthracycline cardiomyopathy. Int J Cardiol. (2019) 292:248–52. doi: 10.1016/j.ijcard.2019.04.026
57. Modi K, Joppa S, Chen KA, Athwal PSS, Okasha O, Velangi PS, et al. Myocardial damage assessed by late gadolinium enhancement on cardiovascular magnetic resonance imaging in cancer patients treated with anthracyclines and/or trastuzumab. Eur Heart J Cardiovasc Imaging. (2021) 22:427–34. doi: 10.1093/ehjci/jeaa279
58. Taylor AJ, Salerno M, Dharmakumar R, Jerosch-Herold M. T1 mapping: basic techniques and clinical applications. JACC Cardiovasc Imaging. (2016) 9:67–81. doi: 10.1016/j.jcmg.2015.11.005
59. Vo HQ, Marwick TH, Negishi K. Pooled summary of native T1 value and extracellular volume with MOLLI variant sequences in normal subjects and patients with cardiovascular disease. Int J Cardiovasc Imaging. (2020) 36:325–36. doi: 10.1007/s10554-019-01717-3
60. Haslbauer JD, Lindner S, Valbuena-Lopez S, Zainal H, Zhou H, D'Angelo T, et al. CMR imaging biosignature of cardiac involvement due to cancer-related treatment by T1 and T2 mapping. Int J Cardiol. (2019) 275:179–86. doi: 10.1016/j.ijcard.2018.10.023
61. Altaha MA, Nolan M, Marwick TH, Somerset E, Houbois C, Amir E, et al. Can quantitative CMR tissue characterization adequately identify cardiotoxicity during chemotherapy?: Impact of temporal and observer variability. JACC Cardiovasc Imaging. (2020) 13:951–62. doi: 10.1016/j.jcmg.2019.10.016
62. Sado DM, Flett AS, Banypersad SM, White SK, Maestrini V, Quarta G, et al. Cardiovascular magnetic resonance measurement of myocardial extracellular volume in health and disease. Heart. (2012) 98:1436–41. doi: 10.1136/heartjnl-2012-302346
63. Gottbrecht M, Kramer CM, Salerno M. Native T1 and extracellular volume measurements by cardiac MRI in healthy adults: a meta-analysis. Radiology. (2019) 290:317–26. doi: 10.1148/radiol.2018180226
64. Ferreira VM, Schulz-Menger J, Holmvang G, Kramer CM, Carbone I, Sechtem U, et al. Cardiovascular magnetic resonance in nonischemic myocardial inflammation: expert recommendations. J Am Coll Cardiol. (2018) 72:3158–76. doi: 10.1016/j.jacc.2018.09.072
65. Tham EB, Haykowsky MJ, Chow K, Spavor M, Kaneko S, Khoo NS, et al. Diffuse myocardial fibrosis by T1-mapping in children with subclinical anthracycline cardiotoxicity: relationship to exercise capacity, cumulative dose and remodeling. J Cardiovasc Magn Reson. (2013) 15:48. doi: 10.1186/1532-429X-15-48
66. Neilan TG, Coelho-Filho OR, Shah RV, Feng JH, Pena-Herrera D, Mandry D. Myocardial extracellular volume by cardiac magnetic resonance imaging in patients treated with anthracycline-based chemotherapy. Am J Cardiol. (2013) 111:717–22. doi: 10.1016/j.amjcard.2012.11.022
67. Tong X, Li VW, Liu AP, So EK, Chan Q, Ho KK, et al. Cardiac magnetic resonance T1 mapping in adolescent and young adult survivors of childhood cancers. Circ Cardiovasc Imaging. (2019) 12:e008453. doi: 10.1161/CIRCIMAGING.118.008453
68. Toro-Salazar OH, Gillan E, O'Loughlin MT, Burke GS, Ferranti J, Stainsby J, et al. Occult cardiotoxicity in childhood cancer survivors exposed to anthracycline therapy. Circ Cardiovasc Imaging. (2013) 6:873–80. doi: 10.1161/CIRCIMAGING.113.000798
69. Bonaca MP, Olenchock BA, Salem JE, Wiviott SD, Ederhy S, Cohen A, et al. Myocarditis in the setting of cancer therapeutics: proposed case definitions for emerging clinical syndromes in cardio-oncology. Circulation. (2019) 140:80–91. doi: 10.1161/CIRCULATIONAHA.118.034497
70. Thavendiranathan P, Zhang L, Zafar A, Drobni ZD, Mahmood SS, Cabral M, et al. Myocardial T1 and T2 mapping by magnetic resonance in patients with immune checkpoint inhibitor-associated myocarditis. J Am Coll Cardiol. (2021) 77:1503–16. doi: 10.1016/S0735-1097(21)04410-7
71. Hecht HS, Cronin P, Blaha MJ, Budoff MJ, Kazerooni EA, Narula J, et al. 2016 SCCT/STR guidelines for coronary artery calcium scoring of noncontrast noncardiac chest CT scans: A report of the society of cardiovascular computed tomography and society of thoracic radiology. J Cardiovasc Comput Tomogr. (2017) 11:74–84. doi: 10.1016/j.jcct.2016.11.003
72. Roos CTG, van den Bogaard VAB, Greuter MJW, Vliegenthart R, Schuit E, Langendijk JA, et al. Is the coronary artery calcium score associated with acute coronary events in breast cancer patients treated with radiotherapy? Radiother Oncol. (2018) 126:170–6. doi: 10.1016/j.radonc.2017.10.009
73. Chiles C, Duan F, Gladish GW, Ravenel JG, Baginski SG, Snyder BS, et al. Association of coronary artery calcification and mortality in the national lung screening trial: a comparison of three scoring methods. Radiology. (2015) 276:82–90. doi: 10.1148/radiol.15142062
74. Gal R, van Velzen SG, Emaus MJ, van den Bongard DH, Gregorowitsch ML, Blezer EL, et al. The risk of cardiovascular disease in irradiated breast cancer patients: The role of cardiac calcifications and adjuvant treatment. Eur J Cancer. (2020) 138:S6. doi: 10.1016/S0959-8049(20)30543-8
75. Banfill K, Giuliani M, Aznar M, Franks K, McWilliam A, Schmitt M, et al. Cardiac toxicity of thoracic radiotherapy: existing evidence and future directions. J Thorac Oncol. (2021) 16:216–27. doi: 10.1016/j.jtho.2020.11.002
76. van Nimwegen FA, Schaapveld M, Cutter DJ, Janus CP, Krol AD, Hauptmann M, et al. Radiation dose-response relationship for risk of coronary heart disease in survivors of hodgkin lymphoma. J Clin Oncol. (2016) 34:235–43. doi: 10.1200/JCO.2015.63.4444
77. Whitlock MC, Yeboah J, Burke GL, Chen H, Klepin HD, Hundley WG. Cancer and its association with the development of coronary artery calcification: an assessment from the multi-ethnic study of atherosclerosis. J Am Heart Assoc. (2015) 4:2533. doi: 10.1161/JAHA.115.002533
78. van den Bogaard VA, Ta BD, van der Schaaf A, Bouma AB, Middag AM, Bantema-Joppe EJ, et al. Validation and modification of a prediction model for acute cardiac events in patients with breast cancer treated with radiotherapy based on three-dimensional dose distributions to cardiac substructures. J Clin Oncol. (2017) 35:1171–8. doi: 10.1200/JCO.2016.69.8480
79. Lancellotti P, Nkomo VT, Badano LP, Bergler-Klein J, Bogaert J, Davin L, et al. Expert consensus for multi-modality imaging evaluation of cardiovascular complications of radiotherapy in adults: a report from the European association of cardiovascular imaging and the American society of echocardiography. Eur Heart J Cardiovasc Imaging. (2013) 14:721–40. doi: 10.1093/ehjci/jet123
80. Heidenreich PA, Hancock SL, Lee BK, Mariscal CS, Schnittger I. Asymptomatic cardiac disease following mediastinal irradiation. J Am Coll Cardiol. (2003) 42:743–9. doi: 10.1016/S0735-1097(03)00759-9
81. Pawade T, Clavel MA, Tribouilloy C, Dreyfus J, Mathieu T, Tastet L, et al. Computed tomography aortic valve calcium scoring in patients with aortic stenosis. Circ Cardiovasc Imaging. (2018) 11:e007146. doi: 10.1161/CIRCIMAGING.117.007146
82. Carmel RJ, Kaplan HS. Mantle irradiation in Hodgkin's disease. An analysis of technique, tumor eradication, and complications. Cancer. (1976) 37:2813–25. doi: 10.1002/1097-0142(197606)37:6<2813::AID-CNCR2820370637>3.0.CO;2-S
83. Bogaert J, Francone M. Pericardial disease: value of CT and MR imaging. Radiology. (2013) 267:340–56. doi: 10.1148/radiol.13121059
84. Gottdiener JS, Mathisen DJ, Borer JS, Bonow RO, Myers CE, Barr LH, et al. Doxorubicin cardiotoxicity: assessment of late left ventricular dysfunction by radionuclide cineangiography. Ann Intern Med. (1981) 94:430–5. doi: 10.7326/0003-4819-94-4-430
85. Bellenger NG, Burgess MI, Ray SG, Lahiri A, Coats AJ, Cleland JG, et al. Comparison of left ventricular ejection fraction and volumes in heart failure by echocardiography, radionuclide ventriculography and cardiovascular magnetic resonance; are they interchangeable? Eur Heart J. (2000) 21:1387–96. doi: 10.1053/euhj.2000.2011
86. Huang H, Nijjar PS, Misialek JR, Blaes A, Derrico NP, Kazmirczak F, et al. Accuracy of left ventricular ejection fraction by contemporary multiple gated acquisition scanning in patients with cancer: comparison with cardiovascular magnetic resonance. J Cardiovasc Magn Reson. (2017) 19:34. doi: 10.1186/s12968-017-0348-4
87. Tarkin JM, Joshi FR, Rudd JHF. PET imaging of inflammation in atherosclerosis. Nat Rev Cardiol. (2014) 11:443–57. doi: 10.1038/nrcardio.2014.80
88. Osiecki S, Sterliński M, Marciniak-Emmons M, Dziuk M. Feasibility of 18FDG PET in the cardiac inflammation. Int J Cardiovas Imaging. (2021) 37:1097–104. doi: 10.1007/s10554-020-02056-4
89. Sarocchi M, Bauckneht M, Arboscello E, Capitanio S, Marini C, Morbelli S, et al. An increase in myocardial 18-fluorodeoxyglucose uptake is associated with left ventricular ejection fraction decline in Hodgkin lymphoma patients treated with anthracycline. J Transl Med. (2018) 16:295. doi: 10.1186/s12967-018-1670-9
90. Poels K, van Leent Mandy MT, Boutros C, Tissot H, Roy S, Meerwaldt Anu E, et al. Immune checkpoint inhibitor therapy aggravates T cell-driven plaque inflammation in atherosclerosis. JACC: CardioOncol. (2020) 2:599–610. doi: 10.1016/j.jaccao.2020.08.007
91. Khalique Z, Ferreira PF, Scott AD, Nielles-Vallespin S, Firmin DN, Pennell DJ. Diffusion tensor cardiovascular magnetic resonance imaging: a clinical perspective. JACC: Cardiovas Imaging. (2020) 13:1235–55. doi: 10.1016/j.jcmg.2019.07.016
92. Greupner J, Zimmermann E, Grohmann A, Dübel H-P, Althoff T, Borges AC, et al. Head-to-head comparison of left ventricular function assessment with 64-row computed tomography, biplane left cineventriculography, and both 2- and 3-dimensional transthoracic echocardiography: comparison with magnetic resonance imaging as the reference standard. J Am College Cardiol. (2012) 59:1897–907. doi: 10.1016/j.jacc.2012.01.046
93. Lee HJ, Im DJ, Youn JC, Chang S, Suh YJ, Hong YJ, et al. Myocardial extracellular volume fraction with dual-energy equilibrium contrast-enhanced cardiac CT in nonischemic cardiomyopathy: a prospective comparison with cardiac MR imaging. Radiology. (2016) 280:49–57. doi: 10.1148/radiol.2016151289
94. Shentu W, Ozawa K, Nguyen TA, Wu MD, Packwood W, Xie A, et al. Echocardiographic molecular imaging of the effect of anticytokine therapy for atherosclerosis. J Am Soc Echocardiography. (2021) 34:433–42.e3. doi: 10.1016/j.echo.2020.11.012
Keywords: cardio-oncology, cardiotoxicity, cardiac imaging, echocardiography, cardiac magnetic resonance imaging, global longitudinal strain, cardiac computer tomographic imaging, cancer therapeutics related cardiac dysfunction
Citation: Yu C, Pathan F, Tan TC and Negishi K (2021) The Utility of Advanced Cardiovascular Imaging in Cancer Patients—When, Why, How, and the Latest Developments. Front. Cardiovasc. Med. 8:728215. doi: 10.3389/fcvm.2021.728215
Received: 21 June 2021; Accepted: 09 August 2021;
Published: 03 September 2021.
Edited by:
Matteo Cameli, University of Siena, ItalyReviewed by:
Shady Abohashem, Massachusetts General Hospital and Harvard Medical School, United StatesMaria Aurora Morales, National Research Council (CNR), Italy
Copyright © 2021 Yu, Pathan, Tan and Negishi. This is an open-access article distributed under the terms of the Creative Commons Attribution License (CC BY). The use, distribution or reproduction in other forums is permitted, provided the original author(s) and the copyright owner(s) are credited and that the original publication in this journal is cited, in accordance with accepted academic practice. No use, distribution or reproduction is permitted which does not comply with these terms.
*Correspondence: Kazuaki Negishi, kazuaki.negishi@sydney.edu.au
†These authors have contributed equally to this work