- 1Arizona Respiratory Neurophysiology Laboratory, Department of Physiology, University of Arizona, Tucson, AZ, United States
- 2Department of Integrative Physiology, University of Colorado Boulder, Boulder, CO, United States
- 3Department of Epidemiology and Biostatistics, Mel and Enid Zuckerman College of Public Health, University of Arizona, Tucson, AZ, United States
- 4College of Medicine, University of Arizona, Tucson, AZ, United States
- 5Division of Cardiology, Sarver Heart Center, College of Medicine, University of Arizona, Tucson, AZ, United States
- 6Division of Pulmonary, Allergy, Critical Care and Sleep Medicine, Banner – University Medical Center, Tucson, AZ, United States
- 7Sleep Disorders Center, Banner – University Medical Center, Tucson, AZ, United States
Background: Cardiovascular disease is a major global health concern and prevalence is high in adults with obstructive sleep apnea (OSA). Lowering blood pressure (BP) can greatly reduce cardiovascular disease risk and physical activity is routinely prescribed to achieve this goal. Unfortunately, many adults with OSA suffer from fatigue, daytime sleepiness, and exercise intolerance—due to poor sleep quality and nocturnal hypoxemia—and have difficulty initiating and maintaining an exercise program. High-resistance inspiratory muscle strength training (IMST) is a simple, time-efficient breathing exercise consistently reported to reduce BP in small, selective groups of both healthy and at-risk adults. Herein we present the study protocol for a randomized clinical trial to determine the long-term efficacy of IMST performed regularly for 24 weeks in middle-aged and older adults with OSA. The primary outcome is casual systolic BP. Secondary outcomes are 24-h systolic BP and circulating plasma norepinephrine concentration. Other outcomes include vascular endothelial function (endothelial-dependent and -independent dilation), aortic stiffness, casual and 24-h diastolic BP, and the influence of circulating factors on endothelial cell nitric oxide and reactive oxygen species production. Overall, this trial will establish efficacy of high-resistance IMST for lowering BP and improving cardiovascular health in middle-aged and older adults with OSA.
Methods: This is a single-site, double-blind, randomized clinical trial. A minimum of 92 and maximum of 122 male and female adults aged 50–80 years with OSA and above-normal BP will be enrolled. After completion of baseline assessments, subjects will be randomized in a 1:1 ratio to participate in either high-resistance or sham (low-resistance) control IMST, performed at home, 5 min/day, 5 days/week, for 24 weeks. Repeat assessments will be taken after the 24-week intervention, and after 4 and 12 weeks of free living.
Discussion: This study is designed to assess the effects of 24 weeks of IMST on BP and vascular function. The results will characterize the extent to which IMST can reduce BP when performed over longer periods (i.e., 6 months) than have been assessed previously. Additionally, this study will help to determine underlying mechanisms driving IMST-induced BP reductions that have been reported previously.
Clinical Trial Registration: This trial is registered with ClinicalTrials.gov (Registration Number: NCT04932447; Date of registration June 21, 2021).
Introduction
Background and Rationale
Cardiovascular disease (CVD) is the leading cause of death in the US and worldwide (1, 2). Obstructive sleep apnea (OSA) is a chronic sleep-related breathing disorder, characterized by repeated partial (hypopnea) or complete (apnea) collapse of the pharyngeal airway, with higher-than-average rates of CVD (3). OSA prevalence has risen sharply over recent decades (4), with current estimates indicating one billion adults (ages 30–69 years) are affected worldwide and prevalence rates in the US nearing 48% (5).
From a physiological perspective, airflow limitation and obstructions often result in chronic intermittent hypoxemia, arousal from sleep, and bursts of sympathetic activity that provoke surges in blood pressure (BP) and heart rate (6–9). In turn, chronic intermittent hypoxemia promotes reactive oxygen species (ROS) overproduction and contributes to chronic systemic inflammation (10, 11). The health implications are especially profound for older adults (65+ years of age) regardless of OSA status, as over 80% of all cardiovascular deaths occur in this age group (12). Age-related stiffening of the large elastic arteries (i.e., aortic and carotid) (13–16) and vascular endothelial dysfunction compound the problem.
The primary therapy for OSA is continuous positive airway pressure (CPAP), which delivers a steady stream of pressurized air via a mask that stents the airway, preserving airflow and oxygenation. CPAP is highly efficacious among adherent users (≥4 h/night, 5 nights a week) as it normalizes sleep architecture (17, 18), reduces sympathetic nervous system activity (19, 20) and lowers systolic BP (SBP) (2–6 mmHg) (21, 22). Despite enhanced usability, CPAP adherence rates remain low (30–60%) (23), with ~50% of patients discontinuing use within the first year (24). Low adherence rates limit the therapeutic impact of CPAP for ongoing CVD and/or CVD development (25–27).
Regular aerobic exercise has important physiologic and clinical benefits in adults with above-normal BP and/or OSA, including reductions in BP (28), OSA severity (29), and risk for adverse cardiovascular events (30, 31). Unfortunately, the salient features of OSA, including obesity (BMI >30 kg/m2), excessive daytime sleepiness (32), and lethargy (33–37), render many adults with this disorder unwilling or unable to exercise, or cause them to discontinue exercise before achieving health gains (38, 39). Time availability is a major barrier to exercise adherence (39, 40) and the need to establish the efficacy of novel forms of exercise that are time-efficient, well-tolerated, and effective has never been greater (41, 42).
Among the exercise modalities that offer improved adherence, shorter duration of effort [relative to current guidelines (43)], and BP-lowering effects, is a respiratory training protocol known as inspiratory muscle strength training, or IMST (42). IMST is performed on a portable hand-held device (Figure 1) and entails repeated inspiratory efforts against a resistance. IMST is distinct from more traditional forms of aerobic exercise in that it is performed (a) in stationary sitting or standing, (b) at slower breathing rates (~10–12 breaths/min), and (c) requires large inspiratory (i.e., negative) pressures (44, 45) that exceed those generated in tidal breathing (46), deep breathing (45), or high-intensity aerobic exercise (i.e., 95% VO2max) (47, 48). Another key distinction is a very abbreviated training format which, for high resistance IMST, comprises just 30 breaths (5 min/day), or a total training time of ~30 min per week.
Over the short-term (i.e., 6 weeks), high-resistance IMST has yielded improvements in SBP, sympathetic nervous system activity, and endothelial function in small groups of healthy and at-risk populations (45, 49–52), including middle aged and older (MA/O) adults with OSA. However, the results for OSA adults are preliminary and require confirmation in a larger group of patients. Furthermore, guidelines issued by the American College of Cardiology and the American Heart Association (ACC/AHA) indicate that non-pharmacological therapies designed to reduce BP should be assessed for efficacy within 3–6 months of treatment initiation (28), and the efficacy of high-resistance IMST administered over the longer-term has yet to be assessed. By extension, it is not known how long IMST-induced BP reductions persist after training cessation. Lastly, in view of evidence that high-resistance IMST improves endothelial function in otherwise healthy MA/O adults with above-normal BP (52), the potential for IMST to induce similar benefits in MA/O adults with OSA warrants assessment.
The CHART (Cardiovascular Health, Apnea, and Respiratory Training) Study is a Phase II clinical trial that seeks to establish the benefits of 24 weeks IMST on: (1) casual (resting) and 24-h (ambulatory) SBP, and (2) to determine if the reductions in SBP are sustained following cessation of IMST. In addition, we seek to understand the underlying mechanisms that drive IMST-related BP reductions. Specifically, we will assess the effects of IMST on: (1) markers of sympathetic nervous system activity and other potential vasoconstrictor factors, arterial stiffness, nitric-oxide (NO)-mediated endothelial function (endothelial-dependent dilation), smooth muscle sensitivity to NO (endothelial-independent dilation), and systemic oxidative stress and inflammation; and (2) the influence of changes in circulating factors on the release of NO and ROS from human umbilical vein endothelial cells ex vivo, using a novel cell-culture model. To achieve these objectives, we will assess the effects of high- vs. low-resistance IMST performed at home 5 min/day, 5 days/week, for 24 weeks in adults 50–80 years of age with OSA (apnea-hypopnea index ≥15) and above-normal SBP (≥120 mmHg). Further, we will reassess these measures after 4 and 12 weeks of free-living following cessation of the 24-week training intervention.
Methods
Study Design
The CHART Study is a Phase II randomized, double-blind, sham-controlled, mechanistic clinical trial comparing high- and low-resistance IMST in 122 MA/O adults (50–80 years) with OSA. The study is a repeated measures design with assessments at four time points: baseline, post intervention, and at follow-up 4- and 12-weeks post-intervention (Figure 2).
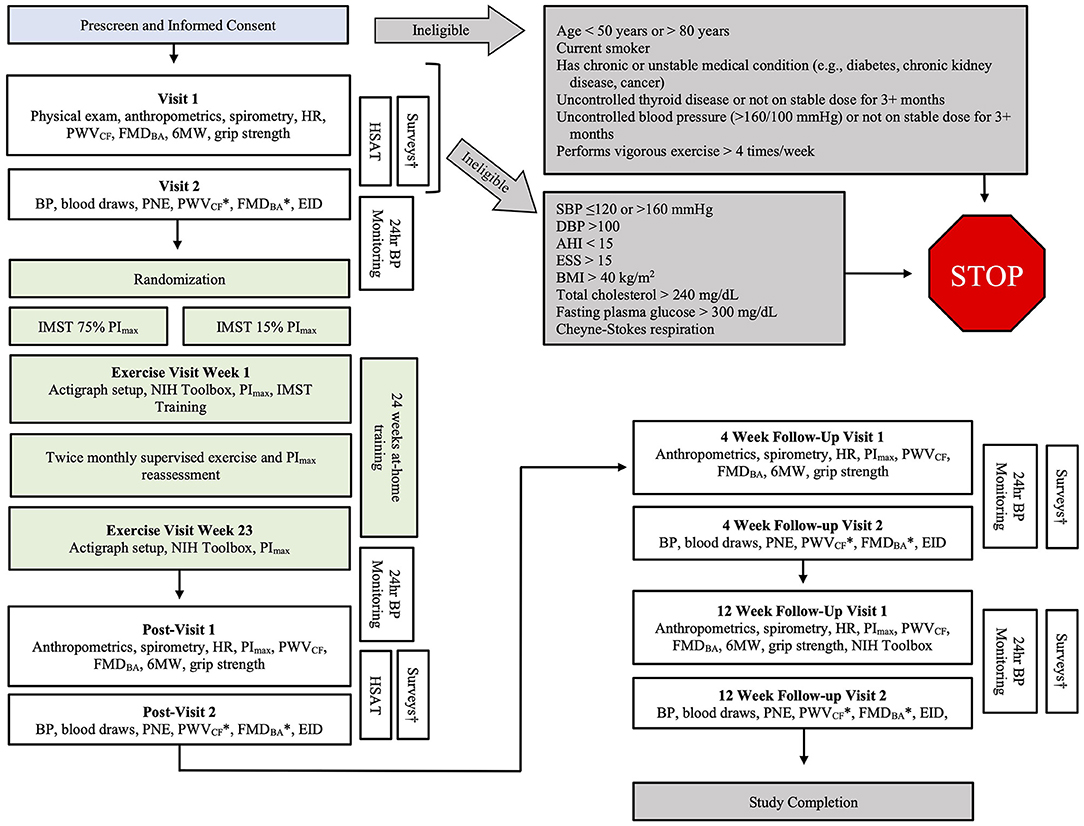
Figure 2. Detailed overview of the CHART study protocol. 6MW, six-minute walk; AHI, apnea hypopnea index; BMI, body mass index; BP, blood pressure; CHAMPS, Community Healthy Activities Model Program for Seniors physical activity questionnaire; ESS, Epworth Sleepiness Scale; FMDBA, brachial artery flow-mediated dilation; HR, heart rate; IMST, inspiratory muscle strength training; PImax, maximal inspiratory pressure; PNE, plasma norepinephrine; PSQI, Pittsburgh Sleep Quality Index; PWVCF, carotid-femoral pulse wave velocity; SBP, systolic blood pressure; *Indicates PWVCF and FMDBA will be performed with saline and Vitamin C infusion. †The following surveys will be completed at home: CHAMPS, ESS, PSQI.
Study Population
Men and women 50–80 years of age with OSA and above-normal BP from all ethnic backgrounds will be eligible to participate. Subjects will be screened to determine whether they meet all the inclusion criteria and have none of the exclusion criteria (Table 1).
Outcomes
Primary Outcome
Casual Systolic Blood Pressure
Casual SBP will be assessed in accordance with ACC/AHA guidelines (28) between 7 and 10 a.m. and after overnight fasting. Subjects will be seated quietly with their back supported, feet flat on the floor, and arms supported on a table at heart level with the palms facing up. SBP will be measured with an automated oscillometric sphygmomanometer (SunTech CT40, SunTech Medical), validated according to standards set by the British Hypertension Society, International Protocol and Association for the Advancement of Medical Instrumentation (53), and calibrated regularly by the manufacturer. Measurements will be performed in triplicate over the brachial artery of the non-dominant arm after at least 5 min of quiet rest and with 1 min of recovery between measures. SBP will be defined as the average of the three pressures, and will be recorded at baseline, weekly throughout the intervention, after the 24-week training period, and at 4- and 12-weeks post-intervention.
Secondary Outcomes
Twenty-Four-Hour Ambulatory Systolic Blood Pressure
Twenty-four-hour SBP will be assessed with 24-h ambulatory non-invasive BP monitoring. Given the propensity for sleep disturbance/arousal reaction to contribute to elevations of BP, assessments will be continuous, beat-to-beat over a 24-h period with the and European Association of Hypertension validated cuffless monitor (SOMNOtouch, SOMNOmedics Germany) (54). Participants will be given a diary to record any physical or psychological events that occur during the 24-h period that may influence BP. SBP will be averaged over the entire 24-h period at baseline, after the 24-week training period, and at 4- and 12-weeks post-intervention.
Daytime and Nighttime Ambulatory Systolic Blood Pressure
Ambulatory SBP data will be broken into daytime (16 h) and nighttime (8 h) segments according to subjects' individual sleep-wake cycles. Mean SBP (mmHg) will be calculated for daytime and nighttime periods to evaluate circadian SBP profile at baseline, after the 24-week training period, and at 4- and 12-weeks post-intervention.
Circulating Plasma Norepinephrine
Concentration of circulating PNE (pg/mL) will be determined from venous blood samples acquired following 20 min of supine rest in a quiet, temperature-controlled room. PNE will be quantified via high-performance liquid chromatography (55) from blood collected at baseline, after the 24-week training period, and at 4- and 12-weeks post-intervention.
Other Outcomes
Endothelial Dependent Dilation
EDD will be assessed via brachial artery flow-mediated dilation (FMDBA) using high-resolution ultrasonography (Canon Xario 200G) (52, 56), the gold-standard in vivo measure of conduit artery vascular endothelial function, that is primarily mediated by NO. FMDBA will be assessed by measuring brachial artery diameter and blood velocity at baseline and for 3 min following reactive hyperemia induced by 5 min of forearm blood flow occlusion with a cuff placed on the upper forearm and inflated at least 50 mmHg above SBP.
Data will be expressed as both percent and absolute (mm) change in arterial diameter from baseline (pre-cuff inflation diameter). Shear area-under-the-curve up to peak diameter will be calculated, and if group- or timepoint-differences exist, FMDBA will be adjusted accordingly. Brachial artery diameter and blood velocity will be analyzed offline with commercially available software (Brachial Analyzer, Medical Imaging Applications LLC, Coralville, IA, USA). Peak arterial diameter will be compared to baseline diameter to calculate absolute change (mm) and percent change in diameter in response to the forearm hyperemic stimulus at baseline, after the 24-week training period, and at 4- and 12-weeks post-intervention.
To determine tonic oxidative stress-mediated suppression of endothelial function, FMDBA will be repeated after intravenous infusion of supratherapeutic concentrations of the potent ROS-scavenging antioxidant, Vitamin C, and isovolumic saline infusion, as described previously (57, 58). A bolus of 0.06 g Vitamin C/kg fat-free mass dissolved in 100 mL of saline will be infused by IV at a rate of 5 mL/min for 20 min, followed immediately by a drip infusion of 0.02 g/kg fat-free mass dissolved in 30 mL saline infusion at a rate of 0.5 mL/min. FMDBA will be performed after the bolus dose, during the drip infusion. FMDBA with infusions will be performed at baseline, after the 24-week training period, and at 4- and 12-weeks post-intervention.
Endothelial Independent Dilation
EID will be assessed in response to sublingual nitroglycerin to assess smooth muscle sensitivity to NO. EID will be determined by measuring brachial artery dilation for 8 min after sublingual nitroglycerin administration (0.4 mg) using high-resolution ultrasonography, as described above. Absolute change and percent change in arterial diameter will be used for analysis. EID will be performed at baseline, after the 24-week training period, and at 4- and 12-weeks post-intervention.
Arterial Stiffness
Arterial stiffness will be assessed via carotid-femoral pulse wave velocity (PWVCF) using a transcutaneous tonometer (SPT-301 ADInstruments, Colorado Springs, CO) placed sequentially in position at the carotid and femoral arteries, with simultaneous electrocardiograph (ECG) recording (59). PWVCF will be calculated as mean distance/transit time. Transit time will be determined as the time between the ECG R-wave and carotid pulse subtracted from the time between the ECG R-wave and femoral pulse. Thirty consecutive pulse waves from each arterial site will be analyzed and averaged. PWVCF will be calculated from difference in carotid-sternal and femoral-sternal distance (m) divided by difference in transit time (s). PWVCF will be assessed at baseline, after the 24-week training period, and at 4- and 12-weeks post-intervention.
Influence of Circulating Factors on Nitric Oxide and Reactive Oxygen Species Production
Using blood drawn from experimental groups we will determine if IMST improves endothelial function via changes in circulating factors that increase endothelial cell NO production and/or reduce endothelial ROS production in cultured human umbilical vein endothelial cells (HUVECs) (American Type Culture Collection). We will expose HUVECs ex vivo, to media conditioned with serum drawn from participants in each group as described previously (52). After 2–4 passages, HUVECs will be plated in 96-well culture plates and incubated for 24 h at 37°C and 5% CO2 in basal media supplemented with 10% subject serum obtain before and after 24 weeks of low-resistance or high-resistance IMST. After incubation, cells will be co-incubated with fluorescent probes DAR-4M AM to detect NO production, and CellROX Deep Red to detect ROS production. Cells imaged with DAR-4M AM will be imaged 5 min after the addition of 200 μmol/L of acetylcholine to the cell culture media to stimulate NO production. Analysis will be done with Image J (National Institutes of Health). Data will be expressed as a fold change in NO/ROS production relative to the baseline timepoint.
Casual Diastolic Blood Pressure
Casual (resting) measures of DBP will be measured according to ACC/AHA guidelines (28). Measures will be made in triplicate over the brachial artery of the non-dominant arm after 5 min of quiet rest, with 1 min of recovery between each measure. Subjects will be seated quietly with their back supported, feet flat on the floor, and arm at heart level. DBP will be measured with an automated oscillometric sphygmomanometer (SunTech CT40, SunTech Medical), validated according to British Hypertension Society, International Protocol, and Association of Medical Instrumentation standards. Mean DBP will be calculated as the average of the three pressures, and will be recorded at baseline, weekly throughout the intervention, post intervention, and at 4- and 12-weeks post-intervention.
Twenty-Four-Hour Ambulatory Diastolic Blood Pressure
Twenty-four-hour DBP will be assessed with 24-h ambulatory non-invasive BP monitoring, as described above. Ambulatory DBP will be averaged over the entire 24-h period at baseline, post intervention, and at follow-up 4- and 12-weeks after cessation of the intervention.
Daytime and Nighttime Ambulatory Diastolic Blood Pressure
Ambulatory DBP data will be broken into daytime (16 h) and nighttime (8 h) segments according to subjects' individual sleep-wake cycles. Mean DBP (mmHg) will be calculated for the daytime and nighttime periods to evaluate circadian DBP profile at baseline, after the 24-week training period, and at 4- and 12-weeks post-intervention.
Six-Minute Walk Distance
A six-minute walk test will be performed in a 30-m hallway with cones to mark the ends of the track. The subject will be instructed to walk as quickly as they can, without running, for 6 min. They will start at the first cone and walk down the hallway, rounding the second cone, and returning up the hallway toward the first cone. They will continue to walk up and down the hallway, completing as many laps as possible in 6 min. Subjects will be told how much time is remaining every minute of the test, and with 30 and 10 s remaining, and encouraged to walk as quickly as possible each time. Distance covered in 6 min will be recorded at baseline, after the 24-week training period, and at 4- and 12-weeks post-intervention.
NIH Toolbox—Cognitive Domain
Subjects will complete the 7 cognitive domain assessments from the NIH Toolbox for the Assessment of Neurological and Behavioral Function (60). These tests include: (1) flanker inhibitory control and attention test; (2) dimensional change card sort test; (3) list sorting working memory test; (4) picture sequence memory test; (5) oral reading recognition test; (6) picture vocabulary test; and (7) pattern comparison processing speed test. All tests will be performed on an iPad (Apple Inc, Cupertino, CA, USA) with an investigator presenting task instructions and monitoring compliance, as recommended by the American Academy of Neurology (60). Scores will be recorded at baseline after the 24-week training period, and at 12-weeks post-intervention.
Maximal Inspiratory Pressure
Maximal inspiratory pressure (PImax) will be assessed by having subjects generate 3 maximal inspiratory efforts against near-infinite resistance measured at the mouth with a POWERbreathe™ KH2 trainer (POWERbreathe™ KH2, POWERbreathe International Ltd., Warwickshire, ENG). The KH2 includes computer software (Breathe-Link Medic, POWERbreathe International Ltd., Warwickshire, ENG) that gives live feedback and can assess PImax and peak inspiratory flow. If there is >10% difference between efforts, subjects will perform up to 3 more efforts to ensure PImax is achieved. The average of the 3 greatest pressures will be used for analysis, and will be assessed at the first exercise session, after the 24-week training period, and at 4- and 12-weeks post-intervention.
Resting Heart Rate
Resting heart rate will be measured simultaneously with blood pressure using the same automated oscillometric sphygmomanometer as is used for blood pressure assessments (SunTech CT40, SunTech Medical). As with blood pressure, heart rate will be defined as the average of the three readings at baseline, and will be performed at baseline, after the 24-week training period, and at 4- and 12-weeks post-intervention.
Home Sleep Apnea Testing
Severity of obstructive sleep apnea (i.e., AHI) will be assessed with a Nox T3s Home Sleep Apnea Testing diagnostic device (Nox T3s, Nox Medical, Reykjavik, IS), a Type III polygraph with actigraphy. Subjects will be instructed on the use and fitting of the device. They will be instructed to turn on the device immediately prior to going to bed and to turn it off upon waking. Subjects with an AHI < 15 will be excluded from the study. Outcomes include total sleep time, apneas/hour, hypopneas/hour, average O2 saturation (SpO2), minimum SpO2, duration of SpO2 < 90%, and duration of SpO2 < 85%. Home sleep apnea testing will be performed at baseline and after the 24-week training period, but not at 4- and 12-weeks post-intervention.
Grip Strength
Maximal grip strength will be obtained with a Jamar hydraulic grip strength dynamometer (Performance Health, LLC, Akron, OH USA). Handle position of the dynamometer will be standardized at position II (61). The subject will be seated with the shoulder in neutral position and the elbow supported and flexed to 90° with the forearm and wrist in neutral position. The subject will squeeze the dynamometer handle as hard as possible for 3 s and maximal force will be recorded in kg. The subject will perform three trials with each hand, and 15 s rest will be allowed between trials. Peak force of each hand will be used for analysis. Grip strength will be performed at baseline, after the 24-week training period, and at 4- and 12-weeks post-intervention.
Muscle Sympathetic Nerve Activity
Muscle sympathetic nerve activity (MSNA) will be recorded from the common peroneal nerve via tungsten microelectrode (200 μm: 25–40 mm, impedance: 5 MX) (FHC, Bowdoin, ME) inserted percutaneously immediately posterior to the fibular head. Due to the technically difficult and time-consuming nature of MSNA, it will be assessed in a subset of subjects in the CHART study (n = 10/group). Subjects selected to complete MSNA testing will do so at a visit separate from Visit 1 and Visit 2 at baseline, after the 24-week intervention, and at the 12-week follow-up. Subjects will rest in a chair reclined ~45° with the right knee and foot supported by positioning pillows (VersaForm, Performance Health, Warrenville, IL). Microelectrode placement will be confirmed via electrical stimulation (0.02 mA, 1 Hz) as described previously (62, 63). A second microelectrode will be inserted just below the skin surface ~1.0 cm from the first to serve as a reference electrode. Electrode position in sympathetic fascicles will be confirmed by pulse synchronous bursts of activity, elicitation of afferent nerve activity by mild muscle stretch, and absence of response to light stroking of the skin or with startle response to loud noises (62). The recorded signal will be amplified (gain 2 × 104), bandpass filtered (500–2.0 kHz) using a pre-amplifier (NeuroAmp Ex; ADInstruments, Colorado Springs, CO), and signals will be full-wave rectified (100 ms moving window) and sampled at 10 kHz. The resulting signal will be monitored using a computer-based data acquisition and analysis system (LabChart 8.0 software, ADInstruments, Colorado Springs, CO) and loudspeaker throughout the experiment. MSNA will be recorded for 10 min, and bursts that exceed a predetermined threshold will be marked and counted. Outcomes will include bursts/minute, bursts/100 heart beats, and mean burst area/minute.
Sample Size Calculation and Power
A minimum of 92 and maximum of 122 participants will be enrolled and randomized in this study (n = 46–61 per group). As OSA prevalence is 2-fold greater in males than females over the age of 50 (64–66), we expect to enroll males at a 2:1 ratio to females. However, we will not restrict enrollment based on sex.
In our preliminary study (49), the effect sizes (ESs) for SBP, PNE, and 24-h SBP were 2.50, 1.25, and 0.63. The within individual standard deviation (SD) was set to be the larger of the SDs for the two groups, although differences in SDs across groups were not substantial. If 45 participants per arm complete the main study, we will have over 80% power for any outcome with an ES > 0.63. Sex-specific comparisons of mean change scores between IMST groups can detect an ES > 1.19, provided at least 30 females complete the study (i.e., 75% of 1/3 of the initial sample size of 122), with at least 15 in each arm. This calculation includes a Bonferroni correction of the significance level—that is, the sex-specific comparisons are conducted at the 2.5% significance level.
Treatment of Subjects
Study Location and Timeline
All baseline, training, post-assessment and follow up measures will be conducted at the University of Arizona's Clinical and Translational Research Services (CATS) facility. The enrollment period for an individual participant will be ~42 weeks. The timeline for the entire study (i.e., recruitment of first subject to study completion for final subject) will be ~54 months.
Subjects who have been screened will have 6 months from the prescreening date to commence the study. If after 6 months they have not attended the first baseline session, they will be required to undergo prescreening assessments again prior to proceeding to baseline assessments.
Two baseline sessions, spaced at least 24 h apart, will be completed within 14 days. Participants will commence the intervention within 14 days of the second baseline assessment and perform their prescribed exercise at home, 5 days/week for 24 weeks. Post-intervention assessments will be completed within a 14-day period, at least 24 h apart and in week 25 ± 1 week. Follow-up assessments will be performed within a 14-day period, at least 24 h apart at 4 ± 1 weeks and 12 ± 1 weeks after the last post-intervention assessment (Table 2).
Baseline Assessments
Eligible participants who are enrolled into the study will undergo the Outcome Assessments and Internal Control Assessments, found in Table 3, within a period of 14 days. Subjects will be asked to maintain their behavior throughout the study, and we will account for unreported changes with the Internal Control Assessments. Protocols for these assessments can be found in the Supplementary Material.
Intervention
Following baseline assessments, participants will begin the training intervention. All training will be performed on the POWERbreathe™ K3 trainer (POWERbreathe™ K3, POWERbreathe International Ltd., Warwickshire, ENG), a handheld pressure-threshold device that can: (1) be pre-programmed to provide the appropriate resistance to inspiration, and (2) record data from all training sessions. Each participant will be provided their own device on which to perform their prescribed exercise at home, 5 days/week for 24 weeks, and will receive verbal and written instruction on the training protocol and K3 operation from the unblinded Research Technician.
The Research Technician will supervise the subject's first training session to ensure proper technique and comprehension. In training weeks 1–3, participants will perform 5 training sessions per week; one training session will be supervised by the Research Technician in the CATS facility, and 4 training sessions will be performed unsupervised at home. In training weeks 4–24, participants will perform training sessions unsupervised at home returning to the CATS facility for supervised training sessions twice monthly. At each visit, the unblinded Research Technician will determine the participant's casual BP, collect sleep and activity logs, transfer saved training data from the K3 device, and record any changes in medications, diet, exercise, and/or health status since the previous visit. The participant will complete 3 PImax efforts to establish the following weeks' training level. The new resistance will be set on the K3 using the Manual function to ensure that the target resistance remains the same until the next in-person visit. The participant then will perform one supervised training session at this new training level. Subjects will be contacted weekly via phone or email to encourage participants and aid in retention and adherence.
Subjects assigned to high-resistance IMST will train against a resistance set at 75% of their PImax. Subjects randomly assigned to low-resistance IMST will train against a resistance set at 15% of PImax. Subjects in both groups will perform 5 sets of 6 breaths, with 1 min rest between sets, for a total of 30 breaths per day (49). All exercise sessions will be recorded in the device memory card and participants will be required to complete a weekly training log.
Post Assessments
To determine the chronic effects of IMST and avoid confounding by acute effects of the intervention, two post-intervention assessments will be completed within a 14-day period, at least 24 h apart beginning in week 25 ± 1 week. Participants will continue to perform daily training (except for the days they will be performing post-intervention assessments) until the post-intervention assessments are completed. Post-assessment measurements will encompass all measures obtained at baseline and will be made under the same experimental conditions, at the same time of day, in the same order and with the same techniques as for baseline testing (Table 2).
Follow-Up Evaluations
Following completion of the post-assessments, participants will return the K3 training device and begin a period of free-living. During this period, subjects will not complete any interventions and will be asked to refrain from making any significant lifestyle changes. Subjects will be contacted weekly via phone or email. Primary, secondary, and other outcomes measures will be reevaluated in all participants during follow-up testing after 4 and 12 weeks of free-living. All measurements will be made under the same experimental conditions, in the same order and with the same techniques as for baseline testing, with the exception of home sleep apnea testing which will not be performed at follow-up visits (Table 2).
Procedures
Recruitment, Eligibility, and Consent
Recruitment will be via newspaper and newsletter advertisement to the general population, University of Arizona (UA) press releases, Facebook, and public presentations to local community groups in the Tucson/Oro Valley/Green Valley region. Once a potential study participant has been identified, they will be directed to the study website where they can complete a questionnaire to determine their eligibility.
Interested candidates will contact the Study Research Professional via phone or email (contact information supplied with recruitment materials) and will be asked to complete a general questionnaire online through the Research Electronic Data Capture (REDCap) system to determine eligibility. Information regarding participants' eligibility (whether eligible, ineligible, or withdrawn questionnaires), will be entered into the electronic Screening Log. Questionnaires that do not meet inclusion criteria will be discarded and the candidate will be informed of their ineligibility. Eligible questionnaires will be sent to study physicians for review. Candidates that meet eligibility will be contacted for a study overview session.
Following the study overview, informed consent will be obtained only by members of the research team who have been observed and approved by the Principal Investigator and authorized by the University of Arizona Institutional Review Board (IRB). Written informed consent will be obtained either online via REDCap or in person from each subject before the start of any study-related procedures and will be reviewed and signed and dated by the participant and the Study Research Professional in accordance with the Declaration of Helsinki. Ethical Approval for this study has been obtained from the University of Arizona IRB (Protocol #1200000220). We will recruit eligible participants who are able to provide informed consent and who are proficient or independent speakers of English or for whom English is their first language. As such, there is no provision or plan for obtaining consent from speakers of a language other than English.
Screening Assessment
Participants who have provided informed consent, will undergo screening assessments in Visit 1 to determine whether they meet the enrollment criteria. Screening evaluations will be performed in sequence as follows:
• BP measurement: SBP ≥120 mmHg and <160 mmHg, DBP <100 mmHg after 5 min rest in seated position.
• Physical examination: assessments of the following parameters (jugular venous pressure, cervical lymph nodes, carotid pulse, normal breath sounds, regular heart rhythm, no abdominal swelling/tenderness, no swelling or discoloration of extremities, normal walking/gait, normal arm and leg strength, no rashes).
• Spirometry in accordance with guidelines from the American Thoracic Society (67): normal (FEV1.0) ≥80%.
• Height and weight measurement: BMI ≤ 40 kg/m2.
• Home sleep apnea test: Apnea Hypopnea Index ≥ 15 events/hour sleep (completed at home after Visit 1 and analyzed before Visit 2).
• Screening blood chemistries: Total cholesterol <240 mg/dL; Fasting plasma glucose <300 mg/dL.
• Epworth Sleepiness Scale score <15 (survey completed at home after Visit 1 but before Visit 2).
Randomization, Blinding, and Treatment Allocation
Participants will be randomized after completing baseline assessments. Assignment to the training program will be by an unblinded Senior Research Professional. The study statistician will use a computer-generated randomization list for the study. Block randomization with block sizes of 2, 4, and 6 will be used to randomize participants into two groups (high- vs. low-resistance IMST) in a 1:1 ratio. Randomization will be stratified by sex (M/F) and OSA disease severity (moderate/severe). The randomization list will be stored in a .CSV file with the randomized group assignment hidden and only revealed by the Senior Research Professional at the time of randomization. Assignment to groups will be sequentially within strata.
Randomization will, on average, balance CPAP users (Yes/No) between the two arms within sex and severity strata however, the effect of treatment may differ depending on CPAP use. We will account for the potential effect of CPAP on outcomes, and possible interaction with treatment, by including CPAP and treatment-by-CPAP interaction effects in the mixed effects models. Based our pilot trials, we anticipate ~10–15% CPAP users in our study sample. The power to detect either a CPAP or a treatment-by-CPAP interaction, that is, our ability to tease out CPAP from IMST effects, will depend on the treatment-by-CPAP cell sample sizes and effect sizes.
Outcomes assessors and data analysts will be blinded to training group allocation. As both training programs have the potential to affect outcome measures, neither is considered a “placebo.” Subjects will be blinded to their allocation group.
Study Withdrawal
Participants can withdraw consent at any time and for any reason if they wish to do so without harm of penalty. The researcher also may remove the participant from the study at any time if they believe study procedures have not been followed, for the benefit of the participant, or in the event of an adverse event (i.e., the development of severe side effects that, in the opinion of the supervising physicians, make it unsafe for the subject to continue in the study). Other discontinuation criteria will include cardiovascular or metabolic disease-related events (e.g., hypertensive crisis, myocardial ischemia/angina, myocardial infarction), major surgery, or other serious changes in physical or mental health status. The reason/s for withdrawal will be registered in the electronic Case Report Form.
Adverse Events
An adverse event (AE) is any harmful and unintended reaction to a study assessment or intervention under investigation. Participants will be instructed to report side effects and AEs to members of the study team. All AEs occurring after the informed consent is signed and up until the subject has completed the study will be reported to the study physicians.
A serious adverse event (SAE) is any untoward medical occurrence that results in death, is life-threatening, requires inpatient hospitalization, results in persistent or significant disability/incapacity. In the event an SAE occurs, it will be reported to the University of Arizona IRB and the supervising physician within a maximum of 24 h from the moment the event is identified.
Throughout the study, the Principal Investigator will prepare yearly safety reports to the regulatory authorities and to the IRB at The University of Arizona following the schedule established in the current institutional guidelines.
Statistical Analysis
The same statistical approach will be used to address all hypotheses, as each hypothesis uses data collected on continuous outcomes (e.g., casual and 24-h BP) from a two-arm design with multiple time points. A standard repeated measures (with the number of time points depending on outcome) mixed model analysis of variance will be used to estimate average change from baseline within arms. Models will adjust for stratification on OSA severity, sex, and age. Subject specific random effects will be used to account for the repeated measures on an individual. Inferences will be based on available data using maximum likelihood, which accommodates data missing at random. We propose a within-individual analysis for the study (comparisons between arms will be based on average change from baseline vs. average at endpoint) as the correlations between baseline and 6-week SBP and plasma norepinephrine in our preliminary data were ~0.60 or larger (49). Effect sizes and confidence intervals will be reported in addition to p-values. Unless otherwise mentioned, tests will be two-sided and conducted at the 5% significance level.
Adherence
Successful adherence is defined as a study subject who achieves an adherence rate of at least 70% (i.e., completes at least 74 of the 106 training sessions and attends 10 of 14 in-person training sessions).
Data Management
Data will be collected manually and or with case report forms (CRF) and source documents. CRFs are defined as an electronic document used to record all protocol relevant subject information. CRFs will generated and accessed through REDCap. CRFs are specific to each visit and will be completed for each subject. Research personnel responsible for visits will be required to complete the appropriate report form in an accurate and concise manner. Source documents are raw data documents where clinical observations are initially recorded. This includes laboratory reports, device reports, and physical examination reports. Information will be entered in CRF so that it follows what is included in source documents.
After the completion of a CRF, data will be exported into a master spreadsheet for analysis using a double-entry method. All data included in this sheet will be de-identified. Manually recorded data will be entered into a digital master data file within 1 week of data collection by the investigator who collected the measure. Data that has been acquired digitally (e.g., FMDBA, PWVCF) will be checked for completeness immediately after collection and then stored for later analysis. Data recorded at baseline, post-intervention, and follow-up for each subject will be analyzed individually after that subject completes all follow-up testing sessions to ensure within-subject accuracy.
Data Monitoring
This study will have a Safety Officer who is completely independent of the sponsor. The Safety Officer is a physician-investigator with extensive experience in clinical trials on MA/O adults and adults with hypertension. The Safety Officer will meet with the Study Oversight Team at regular intervals (at least twice yearly), with one in-person meeting and the other via teleconference, to review enrollment progress, safety data, data quality, and the primary efficacy endpoints.
Anticipated Results
Primary Hypothesis
Twenty-four weeks of high-resistance IMST will lower casual SBP in MA/O adults with above-normal BP and OSA by an average of 15 mmHg; some BP reduction will persist after 4, but not 12 weeks of free living. Adherence to IMST will be high (>80% sessions).
Other Hypotheses
Twenty-four weeks of high-resistance IMST will:
1. Lower 24-h, daytime, and nighttime SBP, as well as PNE.
2. Improve large elastic artery stiffness (i.e., PWVCF reduction), NO-mediated EDD capacity, and ex vivo ROS production.
3. Reduce superoxide-related suppression of NO-mediated EDD (as assessed by FMDBA with Vitamin C infusion).
4. Cause no change in EID (assessed as brachial artery dilation to sublingual nitroglycerin) indicating any improvements in FMDBA observed are due to improvements in vascular endothelial function rather than increased vascular smooth muscle sensitivity to NO.
5. Lower casual DBP and 24-h, daytime, and nighttime DBP.
Some effects will persist after 4, but not 12 weeks of free living.
Discussion
Brief-duration, high-resistance IMST is a simple, time-efficient breathing exercise that substantially lowers BP, and associated CVD risk, in a relatively short period of time (i.e., reductions of 6–12 mmHg after 6 weeks of daily training). These reductions have been reported in small groups of MA/O adults with above-normal BP (41), MA/O adults with OSA and above-normal BP (49), and normotensive young adults (50), but have not yet been established in a large-scale clinical trial. It is likely that performance of IMST over a longer time period (i.e., 24 weeks) will result in even greater reductions in BP, however, this has not been systematically assessed. The purpose of this study is to assess the effects of long-term, high-resistance IMST on BP in a large, representative group of adults with OSA. Additionally, we will assess the extent to which IMST-induced adaptations are sustained following training cessation. Finally, we will elucidate the underlying mechanisms that drive IMST-induced BP reductions by assessing the potential for high-resistance IMST to improve endothelial function, arterial stiffness, and vascular oxidative stress.
Adults with OSA are a unique clinical population because the prevalence of hypertension and CVD is higher than for the general population (3, 68). This is attributed to sleep fragmentation and nightly hypoxemic events that increase oxidative stress and sympathetic nervous system activity (69–71), and result in chronic increases in BP. The ability of IMST to reduce BP despite a chronic stimulus to increase BP is encouraging for other populations with above-normal BP. Further, because IMST is performed in a stationary sitting or standing position, it holds promise for many clinical populations that may be unable to perform traditional aerobic exercise and/or rehabilitation strategies (e.g., heart failure patients, mobility-limited older adults).
We do not expect any significant problems with these well-established training procedures that we have successfully employed previously (49, 52). In light of our previously published outcomes with IMST, our focus will be on outcomes for resting (casual) SBP (primary) and 24-h ambulatory SBP (secondary). FMDBA and PWVCF are strong independent predictors of incident CVD in MA/O adults (72, 73). Therefore, each of these vascular outcomes are important clinically as intermediary therapeutic targets of vascular disease. Measures of clinical CVD endpoints could be incorporated into a subsequent larger, more comprehensive, multi-site clinical trial. Although our ability to interrogate mechanisms of action in a definitive manner in all cases is limited, we have: (1) proposed mechanistic assessments based on findings from our foundational preclinical work in healthy young adults and (2) proposed to focus on assessments of NO-mediated vascular EDD because our pilot studies in MA/O adults without OSA support these as primary mechanisms (52). Last, recent prevalence estimates suggest >80% of adults >50 years exhibit age-related increases in aortic stiffness (elevated PWVCF) and/or hypertension (74) that is correlated with OSA severity (14). Thus, we anticipate a majority of subjects will exhibit aortic stiffening at baseline, and will benefit from IMST.
This study will assess the efficacy of long-term IMST in adults with OSA. Successful completion of this study will allow us to determine: (1) the extent to which IMST can lower BP over 24 weeks; (2) the degree of adherence to IMST when performed at home, 5 days/week, for 24 weeks; (3) persistence of training effects after cessation of IMST; and (4) the mechanisms driving IMST-induced reductions in BP, including effects on large elastic artery stiffness, oxidative stress-related suppression of NO-mediated EDD, and NO and ROS production. Ultimately, high-resistance IMST may prove to be an invaluable supplement to existing therapies for any population with above-normal blood pressure.
Ethics and Dissemination
Research Ethics Approval
This study has been approved by the University of Arizona Institutional Review Board (Approval Number: 1200000220).
Protocol Amendments
Any modifications to the protocol which may impact the conduct of the study will first be agreed upon by the principal investigators and approved by the University of Arizona IRB prior to implementation. Administrative changes of the protocol are considered minor corrections that have no impact on the way the study is to be conducted. These changes will be agreed upon by the PI and documented.
Consent
The study will be thoroughly explained to each subject over the phone, through an online video messaging platform, or in person, and subjects will have the opportunity to ask questions. Once the Senior Research Professional believes the subject understands the study requirements, they will be directed to an online platform to read and digitally sign the informed consent document. Additionally, there is a consent provision asking subjects if they are willing to allow remaining (unused) blood specimens to be used for future research studies. Subjects will have the option to accept or decline this provision without it affecting their participation status in the clinical trial.
Confidentiality
Identity of subjects will be protected by assigning each a code (i.e., a 3-digit number), and any experimental data collected from these subjects will be recorded under that number. Any identifiable personal information will be kept in a password-protected digital file and/or in a locked cabinet. Only the PI, CO-I, Senior Research Professional, and Research Technician will have access to the information.
Access to Data
The PI, CO-I, and Senior Research Professional will have access to the final trial dataset. Other project team members will be provided de-identified data for their analyses.
Ancillary and Post-trial Care
There are no provisions for ancillary or post-trial care.
Dissemination Policy
Primary outcome papers will be approved by the PI prior to journal submission. Every attempt will be made to release study results to the general public soon after study completion. Interim and final reports may also be presented at various local, regional, and international conferences, with approval from the PI. Eligibility for authorship include (1) substantial contribution to study conception and design AND/OR substantial contributions to acquisition, analysis, or interpretation of data, AND (2) drafting or revising the manuscript, AND (3) final approval of the manuscript. There is no intention to use professional writers.
Ethics Statement
The studies involving human participants were reviewed and approved by University of Arizona Institutional Review Board. The patients/participants provided their written informed consent to participate in this study.
Author Contributions
EFB, DS, and DC conceived of the study and initiated the study design. LR-B oversaw study coordination and implementation. DC provided technical training and oversight. JA and SM provided medical oversight. EJB provided statistical expertise. DT and EFB wrote the initial draft of the manuscript. All authors contributed to the refinement of the study protocol and approved the final manuscript.
Funding
This work was supported by NIA/NIH Grant Number: 1R01AG065346-01A1 (EFB), NIH Training Grant Number: 5T32HL007249-44 (DT), and NIH/NHLBI Grant Number: 1K01HL153326-01 (DHC).
Conflict of Interest
The authors declare that the research was conducted in the absence of any commercial or financial relationships that could be construed as a potential conflict of interest.
Publisher's Note
All claims expressed in this article are solely those of the authors and do not necessarily represent those of their affiliated organizations, or those of the publisher, the editors and the reviewers. Any product that may be evaluated in this article, or claim that may be made by its manufacturer, is not guaranteed or endorsed by the publisher.
Acknowledgments
The authors are grateful for the assistance provided in development of study protocol elements provided by Yanko Limon (RN), Aida Hawatmeh, Shivam Nipanikir, Angel Chavez, and Kaitlin Freeberg.
Supplementary Material
The Supplementary Material for this article can be found online at: https://www.frontiersin.org/articles/10.3389/fcvm.2021.760203/full#supplementary-material
References
1. Murray CJL US Burden of Disease Collaborators. The state of US health, 1990-2010: burden of diseases, injuries, and risk factors. JAMA. (2013) 310:591. doi: 10.1001/jama.2013.13805
2. Forouzanfar MH, Liu P, Roth GA, Ng M, Biryukov S, Marczak L, et al. Global burden of hypertension and systolic blood pressure of at least 110 to 115 mmHg, 1990-2015. JAMA. (2017) 317:165–82. doi: 10.1001/jama.2016.19043
3. Ahmad M, Makati D, Akbar S. Review of and updates on hypertension in obstructive sleep apnea. Int J Hypertens. (2017) 2017:1848375. doi: 10.1155/2017/1848375
4. Peppard PE, Young T, Barnet JH, Palta M, Hagen EW, Hla KM. Increased prevalence of sleep-disordered breathing in adults. Am J Epidemiol. (2013) 177:1006–14. doi: 10.1093/aje/kws342
5. Benjafield AV, Ayas NT, Eastwood PR, Heinzer R, Ip MSM, Morrell MJ, et al. Estimation of the global prevalence and burden of obstructive sleep apnoea: a literature-based analysis. Lancet Respir Med. (2013) 7:687–98. doi: 10.1016/S2213-2600(19)30198-5
6. Kaneko Y, Floras JS, Usui K, Plante J, Tkacova R, Kubo T, et al. Cardiovascular effects of continuous positive airway pressure in patients with heart failure and obstructive sleep apnea. N Engl J Med. (2003) 348:1233–41. doi: 10.1056/NEJMoa022479
7. Konecny T, Kara T, Somers VK. Obstructive sleep apnea and hypertension: an update. Hypertension. (2014) 63:203–9. doi: 10.1161/HYPERTENSIONAHA.113.00613
8. Mansukhani MP, Kara T, Caples SM, Somers VK. Chemoreflexes, sleep apnea, and sympathetic dysregulation. Curr Hypertens Rep. (2014) 16:476. doi: 10.1007/s11906-014-0476-2
9. Cowie MR. Sleep apnea: state of the art. Trends Cardiovasc Med. (2017) 27:280–9. doi: 10.1016/j.tcm.2016.12.005
10. Korcarz CE, Benca R, Barnet JH, Stein JH. Treatment of obstructive sleep apnea in young and middle-aged adults: effects of positive airway pressure and compliance on arterial stiffness, endothelial function, and cardiac hemodynamics. J Am Heart Assoc. (2016) 5:e002930. doi: 10.1161/JAHA.115.002930
11. Mochol J, Gawrys J, Gajecki D, Szahidewicz-Krupska E, Martynowicz H, Doroszko A. Cardiovascular disorders triggered by obstructive sleep apnea — a focus on endothelium and blood components. Int J Mol Sci. (2021) 22:5139. doi: 10.3390/ijms22105139
12. Lakatta EG. Age-associated cardiovascular changes in health: Impact on cardiovascular disease in older persons. Heart Fail Rev. (2002) 7:29–49. doi: 10.1023/A:1013797722156
13. Lakatta EG, Levy D. Arterial and cardiac aging: major shareholders in cardiovascular disease enterprises: part I: aging arteries: a “set up” for vascular disease. Circulation. (2003) 107:139–46. doi: 10.1161/01.CIR.0000048892.83521.58
14. Doonan RJ, Scheffler P, Lalli M, Kimoff RJ, Petridou ET, Daskalopoulos ME, et al. Increased arterial stiffness in obstructive sleep apnea: a systematic review. Hypertens Res. (2011) 34:23–32. doi: 10.1038/hr.2010.200
15. Kylintireas I, Craig S, Nethononda R, Kohler M, Francis J, Choudhury R, et al. Atherosclerosis and arterial stiffness in obstructive sleep apnea—a cardiovascular magnetic resonance study. Atherosclerosis. (2012) 222:483–9. doi: 10.1016/j.atherosclerosis.2012.03.036
16. Fleenor BS. Large elastic artery stiffness with aging: novel translational mechanisms and interventions. Aging Dis. (2013) 4:8.
17. McArdle N, Douglas N. Effect of continuous positive airway pressure on sleep architecture in the sleep apnea–hypopnea syndrome: a randomized controlled trial. Am J Respir Crit Care Med. (2001) 164:1459–63. doi: 10.1164/ajrccm.164.8.2008146
18. Quan SF, Budhiraja R, Kushida CA. Associations between sleep quality, sleep architecture and sleep disordered breathing and memory after continuous positive airway pressure in patients with obstructive sleep apnea in the Apnea Positive Pressure Long-term Efficacy Study (APPLES). Sleep Sci. (2018) 11:231–8. doi: 10.5935/1984-0063.20180037
19. Henderson LA, Fatouleh RH, Lundblad LC, McKenzie DK, Macefield VG. Effects of 12 months continuous positive airway pressure on sympathetic activity related brainstem function and structure in obstructive sleep apnea. Front Neurosci. (2016) 10:90. doi: 10.3389/fnins.2016.00090
20. Lundblad LC, Fatouleh RH, McKenzie DK, Macefield VG, Henderson LA. Brain stem activity changes associated with restored sympathetic drive following CPAP treatment in OSA subjects: a longitudinal investigation. J Neurophysiol. (2015) 114:893–901. doi: 10.1152/jn.00092.2015
21. Drager LF, Brunoni AR, Jenner R, Lorenzi-Filho G, Bensenor IM, Lotufo PA. Effects of CPAP on body weight in patients with obstructive sleep apnoea: a meta-analysis of randomised trials. Thorax. (2015) 70:258–64. doi: 10.1136/thoraxjnl-2014-205361
22. Martínez-García M-A, Capote F, Campos-Rodríguez F, Lloberes P, Díaz de Atauri MJ, Somoza M, et al. Effect of CPAP on blood pressure in patients with obstructive sleep apnea and resistant hypertension: the HIPARCO randomized clinical trial. JAMA. (2013) 310:2407. doi: 10.1001/jama.2013.281250
23. Weaver TE, Grunstein RR. Adherence to continuous positive airway pressure therapy: the challenge to effective treatment. Proc Am Thorac Soc. (2008) 5:173–8. doi: 10.1513/pats.200708-119MG
24. Bartlett D, Wong K, Richards D, Moy E, Espie CA, Cistulli PA, et al. Increasing adherence to obstructive sleep apnea treatment with a group social cognitive therapy treatment intervention: a randomized trial. Sleep. (2013) 36:1647–54. doi: 10.5665/sleep.3118
25. McEvoy RD, Antic NA, Heeley E, Luo Y, Ou Q, Zhang X, et al. CPAP for prevention of cardiovascular events in obstructive sleep apnea. N Engl J Med. (2016) 375:919–31. doi: 10.1056/NEJMoa1606599
26. Lorenzi-Filho G, Almeida FR, Strollo PJ. Treating OSA: current and emerging therapies beyond CPAP. Respirology. (2017) 22:1500–7. doi: 10.1111/resp.13144
27. Tietjens JR, Claman D, Kezirian EJ, De Marco T, Mirzayan A, Sadroonri B, et al. Obstructive sleep apnea in cardiovascular disease: a review of the literature and proposed multidisciplinary clinical management strategy. J Am Heart Assoc. (2013) 8:e010440. doi: 10.1161/JAHA.118.010440
28. Whelton PK, Carey RM, Aronow WS, Casey DE, Collins KJ, Dennison Himmelfarb C, et al. 2017 ACC/AHA/AAPA/ABC/ACPM/AGS/APhA/ASH/ASPC/NMA/PCNA guideline for the prevention, detection, evaluation, and management of high blood pressure in adults. J Am Coll Cardiol. (2018) 71:e127–248. doi: 10.1016/j.jacc.2017.11.006
29. Kline CE, Crowley EP, Ewing GB, Burch JB, Blair SN, Durstine JL, et al. The effect of exercise training on obstructive sleep apnea and sleep quality: a randomized controlled trial. Sleep. (2011) 34:1631–40. doi: 10.5665/sleep.1422
30. Thompson PD, Buchner D, Piña IL, Balady GJ, Williams MA, Marcus BH, et al. Exercise and physical activity in the prevention and treatment of atherosclerotic cardiovascular disease: a statement from the Council on Clinical Cardiology (Subcommittee on Exercise, Rehabilitation, and Prevention) and the Council on Nutrition, Physical Activity, and Metabolism (Subcommittee on Physical Activity). Circulation. (2003) 107:3109–16. doi: 10.1161/01.CIR.0000075572.40158.77
31. Mora S, Cook N, Buring JE, Ridker PM, Lee I-M. Physical activity and reduced risk of cardiovascular events: potential mediating mechanisms. Circulation. (2007) 116:2110–8. doi: 10.1161/CIRCULATIONAHA.107.729939
32. Weaver TE, Mancini C, Maislin G, Cater J, Staley B, Landis JR, et al. Continuous positive airway pressure treatment of sleepy patients with milder obstructive sleep apnea: results of the CPAP Apnea Trial North American Program (CATNAP) randomized clinical trial. Am J Respir Crit Care Med. (2012) 186:677–83. doi: 10.1164/rccm.201202-0200OC
33. Thompson WG, Cook DA, Clark MM, Bardia A, Levine JA. Treatment of obesity. Mayo Clin Proc. (2007) 82:93–102. doi: 10.4065/82.1.93
34. Hargens TA, Guill SG, Zedalis D, Gregg JM, Nickols-Richardson SM, Herbert WG. Attenuated heart rate recovery following exercise testing in overweight young men with untreated obstructive sleep apnea. Sleep. (2008) 31:104–10. doi: 10.1093/sleep/31.1.104
35. Sengul YS, Ozalevli S, Oztura I, Itil O, Baklan B. The effect of exercise on obstructive sleep apnea: a randomized and controlled trial. Sleep Breath. (2011) 15:49–56. doi: 10.1007/s11325-009-0311-1
36. Aihara K, Oga T, Yoshimura C, Hitomi T, Chihara Y, Harada Y, et al. Measurement of dyspnea in patients with obstructive sleep apnea. Sleep Breath. (2013) 17:753–61. doi: 10.1007/s11325-012-0759-2
37. Beitler JR, Awad KM, Bakker JP, Edwards BA, DeYoung P, Djonlagic I, et al. Obstructive sleep apnea is associated with impaired exercise capacity: a cross-sectional study. J Clin Sleep Med. (2014) 10:1199–204. doi: 10.5664/jcsm.4200
38. Caserta MS, Gillett PA. Older women's feelings about exercise and their adherence to an aerobic regimen over time. Gerontologist. (1998) 38:602–9. doi: 10.1093/geront/38.5.602
39. Burton LC, Shapiro S, German PS. Determinants of physical activity initiation and maintenance among community-dwelling older persons. Prev Med. (1999) 29:422–30. doi: 10.1006/pmed.1999.0561
40. Aily JB, Carnaz L, Farche ACS, Takahashi AC de M. Perception of barriers to physical exercise in women population over 60. Mot Rev Educ Física. (2017) 23:e101653. doi: 10.1590/s1980-6574201700020012
41. Craighead DH, Heinbockel TC, Hamilton MN, Bailey EF, MacDonald MJ, Gibala MJ, et al. Time-efficient physical training for enhancing cardiovascular function in midlife and older adults: promise and current research gaps. J Appl Physiol. (2019) 127:1427–40. doi: 10.1152/japplphysiol.00381.2019
42. Craighead DH, Freeberg KA, McCarty NP, Seals DR. Time-efficient, high-resistance inspiratory muscle strength training for cardiovascular aging. Exp Gerontol. (2021) 154:111515. doi: 10.1016/j.exger.2021.111515
43. US Department of Health and Human Services. Physical Activity Guidelines for Americans. 2nd ed. Washington, DC: US Department of Health and Human Services (2018).
44. Romer LM, Mcconnell AK, Jones DA. Inspiratory muscle fatigue in trained cyclists: effects of inspiratory muscle training. Med Sci Sports Exerc. (2002) 34:785–92. doi: 10.1097/00005768-200205000-00010
45. Vranish JR, Bailey EF. Daily respiratory training with large intrathoracic pressures, but not large lung volumes, lowers blood pressure in normotensive adults. Respir Physiol Neurobiol. (2015) 216:63–9. doi: 10.1016/j.resp.2015.06.002
46. Walls CE, Laine CM, Kidder IJ, Bailey EF. Human hypoglossal motor unit activities in exercise: respiratory-related genioglossus motoneuron activities in cycling. J Physiol. (2013) 591:3579–90. doi: 10.1113/jphysiol.2013.252452
47. Johnson V, Aaron E, Babcock M, Dempsey J. Respiratory muscle fatigue during exercise: implications for performance. Med Sci Sports Exerc. (1996) 28:1129–37. doi: 10.1097/00005768-199609000-00008
48. Harms CA, Wetter TJ, McClaran SR, Pegelow DF, Nickele GA, Nelson WB, et al. Effects of respiratory muscle work on cardiac output and its distribution during maximal exercise. J Appl Physiol. (1998) 85:609–18. doi: 10.1152/jappl.1998.85.2.609
49. Vranish JR, Bailey EF. Inspiratory muscle training improves sleep and mitigates cardiovascular dysfunction in obstructive sleep apnea. Sleep. (2016) 39:1179–85. doi: 10.5665/sleep.5826
50. DeLucia CM, De Asis RM, Bailey EF. Daily inspiratory muscle training lowers blood pressure and vascular resistance in healthy men and women. Exp Physiol. (2018) 103:201–11. doi: 10.1113/EP086641
51. Ramos-Barrera GE, DeLucia CM, Bailey EF. Inspiratory muscle strength training lowers blood pressure and sympathetic activity in older adults with OSA: a randomized controlled pilot trial. J Appl Physiol. (2020) 129:449–58. doi: 10.1152/japplphysiol.00024.2020
52. Craighead DH, Heinbockel TC, Freeberg KA, Rossman MJ, Jackman RA, Jankowski LR, et al. Time-efficient inspiratory muscle strength training lowers blood pressure and improves endothelial function, NO bioavailability, and oxidative stress in midlife/older adults with above-normal blood pressure. J Am Heart Assoc. (2021) 10:e020980. doi: 10.1161/JAHA.121.020980
53. Polo Friz H, Punzi V, Petri F, Orlandi R, Maggiolini D, Polo Friz M, et al. Simultaneous validation of the SunTech CT40 automated blood pressure measurement device by the 1993 British Hypertension Society protocol and the Association for the Advancement of Medical Instrumentation/International Organization for Standardization 81060-2:2013 standard. Blood Press Monit. (2017) 22:298–301. doi: 10.1097/MBP.0000000000000281
54. Bilo G, Zorzi C, Ochoa Munera JE, Torlasco C, Giuli V, Parati G. Validation of the Somnotouch-NIBP noninvasive continuous blood pressure monitor according to the European Society of Hypertension International Protocol revision 2010. Blood Press Monit. (2015) 20:291–4. doi: 10.1097/MBP.0000000000000124
55. Hjemdal P. Inter-laboratory comparison of plasma catecholamine determinations using several different assays. Acta Physiol Scand Suppl. (1984) 527:43–54.
56. Celermajer DS, Sorensen KE, Gooch VM, Spiegelhalter DJ, Miller OI, Sullivan ID, et al. Non-invasive detection of endothelial dysfunction in children and adults at risk of atherosclerosis. Lancet. (1992) 340:1111–5. doi: 10.1016/0140-6736(92)93147-F
57. Eskurza I, Monahan KD, Robinson JA, Seals DR. Effect of acute and chronic ascorbic acid on flow-mediated dilatation with sedentary and physically active human ageing. J Physiol. (2004) 556:315–24. doi: 10.1113/jphysiol.2003.057042
58. Taddei S, Virdis A, Ghiadoni L, Magagna A, Salvetti A. Vitamin C improves endothelium-dependent vasodilation by restoring nitric oxide activity in essential hypertension. Circulation. (1998) 97:2222–9. doi: 10.1161/01.CIR.97.22.2222
59. Laurent S, Cockcroft J, Van Bortel L, Boutouyrie P, Giannattasio C, Hayoz D, et al. Expert consensus document on arterial stiffness: methodological issues and clinical applications. Eur Heart J. (2006) 27:2588–605. doi: 10.1093/eurheartj/ehl254
60. Weintraub S, Dikmen SS, Heaton RK, Tulsky DS, Zelazo PD, Bauer PJ, et al. Cognition assessment using the NIH Toolbox. Neurology. (2013) 80:S54–64. doi: 10.1212/WNL.0b013e3182872ded
61. Mathiowetz V, Weber K, Volland G, Kashman N. Reliability and validity of grip and pinch strength evaluations. J Hand Surg. (1984) 9:222–6. doi: 10.1016/S0363-5023(84)80146-X
62. Macefield VG, Wallin BG, Vallbo AB. The discharge behaviour of single vasoconstrictor motoneurones in human muscle nerves. J Physiol. (1994) 481:799–809. doi: 10.1113/jphysiol.1994.sp020482
63. DeLucia CM, DeBonis DR, Schwyhart SM, Bailey EF. Acute cardiovascular responses to a single bout of high intensity inspiratory muscle strength training in healthy young adults. J Appl Physiol. (2021) 130:1114–21. doi: 10.1152/japplphysiol.01015.2020
64. Garvey JF, Pengo MF, Drakatos P, Kent BD. Epidemiological aspects of obstructive sleep apnea. J Thorac Dis. (2015) 7:920–9. doi: 10.3978/j.issn.2072-1439.2015.04.52
65. Fatureto-Borges F, Lorenzi-Filho G, Drager L. Effectiveness of continuous positive airway pressure in lowering blood pressure in patients with obstructive sleep apnea: a critical review of the literature. Integr Blood Press Control. (2016) 9:43–7. doi: 10.2147/IBPC.S70402
66. Heinzer R, Vat S, Marques-Vidal P, Marti-Soler H, Andries D, Tobback N, et al. Prevalence of sleep-disordered breathing in the general population: the HypnoLaus study. Lancet Respir Med. (2015) 3:310–8. doi: 10.1016/S2213-2600(15)00043-0
67. Miller M, Hankinson J, Brusasco V, Burgos F, Casaburi R, Coates A, et al. Standardisation of spirometry. Eur Respir J. (2005) 26:319–38. doi: 10.1183/09031936.05.00034805
68. Bundy JD, Mills KT, Chen J, Li C, Greenland P, He J. Estimating the association of the 2017 and 2014 hypertension guidelines with cardiovascular events and deaths in US adults: an analysis of national data. JAMA Cardiol. (2018) 3:572. doi: 10.1001/jamacardio.2018.1240
69. Leuenberger U, Jacob E, Sweer L, Waravdekar N, Zwillich C, Sinoway L. Surges of muscle sympathetic nerve activity during obstructive apnea are linked to hypoxemia. J Appl Physiol. (1995) 79:581–8. doi: 10.1152/jappl.1995.79.2.581
70. Narkiewicz K, Somers VK. Sympathetic nerve activity in obstructive sleep apnoea. Acta Physiol Scand. (2003) 177:385–90. doi: 10.1046/j.1365-201X.2003.01091.x
71. Tamisier R, Pepin JL, Remy J, Baguet JP, Taylor JA, Weiss JW, et al. 14 nights of intermittent hypoxia elevate daytime blood pressure and sympathetic activity in healthy humans. Eur Respir J. (2011) 37:119–28. doi: 10.1183/09031936.00204209
72. Yeboah J, Crouse JR, Hsu F-C, Burke GL, Herrington DM. Brachial flow-mediated dilation predicts incident cardiovascular events in older adults: the cardiovascular health study. Circulation. (2007) 115:2390–7. doi: 10.1161/CIRCULATIONAHA.106.678276
73. Najjar SS, Scuteri A, Shetty V, Wright JG, Muller DC, Fleg JL, et al. Pulse wave velocity is an independent predictor of the longitudinal increase in systolic blood pressure and of incident hypertension in the Baltimore Longitudinal Study of Aging. J Am Coll Cardiol. (2008) 51:1377–83. doi: 10.1016/j.jacc.2007.10.065
Keywords: obstructive sleep apnea, hypertension, inspiratory, exercise, older adults, blood pressure, endothelial, vascular
Citation: Tavoian D, Ramos-Barrera LE, Craighead DH, Seals DR, Bedrick EJ, Alpert JS, Mashaqi S and Bailey EF (2021) Six Months of Inspiratory Muscle Training to Lower Blood Pressure and Improve Endothelial Function in Middle-Aged and Older Adults With Above-Normal Blood Pressure and Obstructive Sleep Apnea: Protocol for the CHART Clinical Trial. Front. Cardiovasc. Med. 8:760203. doi: 10.3389/fcvm.2021.760203
Received: 17 August 2021; Accepted: 18 October 2021;
Published: 24 November 2021.
Edited by:
Giuseppe Maiolino, University Hospital of Padua, ItalyReviewed by:
David Hupin, Karolinska Institutet (KI), SwedenOreste Marrone, National Research Council (CNR), Italy
Copyright © 2021 Tavoian, Ramos-Barrera, Craighead, Seals, Bedrick, Alpert, Mashaqi and Bailey. This is an open-access article distributed under the terms of the Creative Commons Attribution License (CC BY). The use, distribution or reproduction in other forums is permitted, provided the original author(s) and the copyright owner(s) are credited and that the original publication in this journal is cited, in accordance with accepted academic practice. No use, distribution or reproduction is permitted which does not comply with these terms.
*Correspondence: E. Fiona Bailey, ZWJhaWxleUBhcml6b25hLmVkdQ==