- 1Key Laboratory of Chinese Internal Medicine of Ministry of Education and Beijing, Dongzhimen Hospital Affiliated to Beijing University of Chinese Medicine, Beijing, China
- 2Department of Encephalopathy, Dongzhimen Hospital Affiliated to Beijing University of Chinese Medicine, Beijing, China
Background/Aims: To explore the imaging changes and related risk factors of heart failure (HF) patients with cognitive impairment (CI).
Methods: A literature search was systematically carried out in PubMed, Web of Science, Embase, and Cochrane Library. In this systematic review, important relevant information was extracted according to the inclusion and exclusion criteria. The methodological quality was assessed by three scales according to the different study types.
Results: Finally, 66 studies were included, involving 33,579 patients. In the imaging changes, the severity of medial temporal lobe atrophy (MTA) and the decrease of gray Matter (GM) volume were closely related to the cognitive decline. The reduction of cerebral blood flow (CBF) may be correlated with CI. However, the change of white matter (WM) volume was possibly independent of CI in HF patients. Specific risk factors were analyzed, and the data indicated that the increased levels of B-type natriuretic peptide (BNP)/N-terminal pro-B-type natriuretic peptide (NT-proBNP), and the comorbidities of HF, including atrial fibrillation (AF), diabetes mellitus (DM) and anemia were definitely correlated with CI in patients with HF, respectively. Certain studies had also obtained independent correlation results. Body mass index (BMI), depression and sleep disorder exhibited a tendency to be associated with CI. Low ejection fraction (EF) value (<30%) was inclined to be associated with the decline in cognitive function. However, no significant differences were noted between heart failure with preserved ejection fraction (HFpEF) and heart failure with reduced ejection fraction (HFrEF) in cognitive scores.
Conclusion: BNP/NT-proBNP and the comorbidities of HF including AF, DM and anemia were inextricably correlated with CI in patients with HF, respectively. These parameters were independent factors. The severity of MTA, GM volume, BMI index, depression, sleep disorder, and low EF value (<30%) have a disposition to associated with CI. The reduction in the CBF volume may be related to CI, whereas the WM volume may not be associated with CI in HF patients. The present systematic review provides an important basis for the prevention and treatment of CI following HF.
Introduction
Heart failure (HF), which is the end-stage manifestation of cardiovascular disease, is the leading cause of death and morbidity and its incidence increases rapidly along with age. HF not only damages the structure or function of the heart itself but also affects the physiological functions of multiple organs. Among them, cognitive impairment (CI) following HF is very common in clinical practice, and its prevalence rate is estimated to 31–85% according to previous investigations (1, 2). CI is associated with an array of poor outcomes in HF patients. Firstly, the presence of CI is strongly correlated with an increased risk of death and recurrent hospitalizations in the HF populations. Muradet al. (3) revealed in a longitudinal study that CI increased the risk of mortality in HF patients (HR 1.33, 95% CI 1.02–1.73). This was confirmed in subsequent studies (4, 5). Secondly, cognitive function is an important factor affecting the quality of life in HF patients. Elderly patients with HF may have worse self-care behaviors due to the development of CI, such as inability to receive their medications on time, dietary restrictions, and lifestyle changes, which will damage patient ability to self-care, and finally reduce the quality of life (6–8). Thirdly, the decline in cognitive function may also affect the clinical symptoms of HF. Memory loss, deterioration of quality of life, and poor executive function are more likely to increase the risk of adverse events (9). Therefore, the symptoms of HF and the deterioration of cognitive function promote and influence each other.
HF patients with CI demonstrated cognitive disorders in attention, orientation, episodic memory, visuospatial function, executive function, and other aspects. In the early stage of HF, patients may be more likely to show a decline in executive function, attention, and visuospatial ability, while the memory function may be slightly impaired (10). With the progression of the disease, the cognitive functions of patients with HF will decline to varying degrees and may develop into Alzheimer's disease (AD) or vascular dementia (VD) in the later stage (11).
Therefore, CI has negative effects on the prognosis of HF patients. Although its etiology and pathological mechanism are not completely clear, it is undeniable that CI following HF is caused due to the interaction of multiple factors. The decrease in the cerebral blood flow (CBF) or the changes in brain structure may be the pathological basis of CI in HF patients (12). In addition, decreased ejection fraction (EF), and comorbidities such as depression, atrial fibrillation (AF), and diabetes mellitus (DM) are also risk factors affecting cognitive function in HF patients (13). However, to the best of our knowledge, no systematic summary has been performed on brain imaging and on the effect of multiple related factors of CI following HF. The present article reviews the imaging changes and risk factors of CI in patients with HF so as to provide the basis for subsequent prevention and treatment.
Methods
Search Strategy
The search was applied to four databases including PubMed, Embase, Web of Science and Cochrane Library (the publishing time ranged from the inception through April 21, 2021). The search strategy used the following general terms as the mesh or the free terms: The search strategy used the search terms “heart failure,” “cognitive impairment,” “brain imaging,” “risk factors,” “cerebral blood flow,” “hippocampus,” “gray matters,” “temporal lobe,” “white matter,” “ejection fraction,” “body mass index,” “electrolyte,” “depressed,” “atrial fibrillation,” “diabetes,” “anemia,” “sleep disorders,” “BNP,” and their common synonyms. The specific retrieval strategy is described in the Supplementary Material 1.
Inclusion and Exclusion Criteria
The inclusion criteria of the studies were as follows: (1) Participants: Patients with clinically diagnosed HF were included, regardless of their race or nationality, with or without the control group; (2) Method of research: Cohort studies, case-control studies, cross-sectional studies, and intervention studies were included; (3) Detection of indices: Imaging on CBF, hippocampus, temporal lobe, gray matter, and white matter; the risk factors included EF, BMI index, and electrolyte, BNP, and NT-proBNP levels; the comorbid diseases included depression, DM, AF, anemia, and sleep disorders; (4) Statistical methods: Correlation analyses were performed between detection indices and cognitive function in HF patients.
It should be noted that certain studies were included, although no correlation analysis was performed in the present systematic review. These studies researched the differences in cognitive function among different types of HF patients, and the types were distinguished by EF. Therefore, these studies can be regarded as the effects of EF on the cognitive function of HF patients.
The exclusion criteria were as follows: (1) non-clinical studies; (2) articles with incomplete information; (3) reviews, meta-analyses, and corresponding/conference abstracts.
Data Extraction
The data were extracted independently by two evaluators. In case of a dispute, this was resolved following discussion by both parties or decided by the third evaluator. The data extracted included the first author's name, publication time, number of cases, patient characteristics (age, gender, type of HF, and cardiac function grade), imaging detection methods, cognitive function detect methods, and main results. The lack of information was supplemented by contacting the author by telephone or email.
Quality Assessment
The agency for Healthcare Research and Quality (AHRQ) was used to assess the quality of cross-sectional studies (14). It included 11 items, such as defining the source of information, listing inclusion and exclusion criteria, and indicating the period used for identifying patients, which answered with “yes,” “no,” and “not clear.” The answer to “yes” scored 1 point, whereas the answer to “no or not clear” 0 points. A score of 0–3 points was indicative of low quality, of 4–7 points of medium quality, and of 8–11 points of high quality (15). The Newcastle–Ottawa scale (NOS) was used to assess the quality of cohort and case-control studies (16). The scale compared eight items from three aspects of study population selection, comparability between groups, and measurement of exposure factors. Each study scored 0–9 points. A score of 0–4 points was indicative of low quality, of 5–6 points of medium quality, and of 7–9 points of high quality. The Methodological Index for Non-randomized Studies (MINORS) was used to conduct the quality evaluation for the self-control studies (17). It includes 8 items, such as clear goals, continuous patient inclusion, prospective data collection, and unbiased assessment of the study endpoint. Each item received a score from 0–2 points, and the total score was 24 points. 0–8 points were indicative of low quality, 9–16 points of medium quality, and 17–24 points of high quality.
Summary Analysis
A qualitative synthesis was adopted for this systematic review.
Results
Literature Search Results
A total of 2,471 records were identified from four electronic databases, and 637 duplicate articles were removed. Following evaluation of titles and abstracts, 1,365 articles were excluded. A total of 403 articles were excluded of the 469 remaining articles, following investigation of the full text. Finally, 66 studies (18–83) were included in the analysis, involving a total of 33,579 patients. The flow chart (Figure 1) indicates the search process and study selection.
Study Characteristics
The study and patient characteristics of the included studies are shown in Tables 1–12. A total of eight studies (18–25) described the relationship between CBF and cognitive function in HF patients, whereas three studies (26–28) described the relationship between MTA and cognitive function in HF patients, and two studies (29, 30) described the relationship between GM changes and cognitive function in HF patients. Moreover, four studies (26–28, 31) described the relationship between WM changes and cognitive function in HF patients, whereas 16 studies (32–47) described the relationship between EF and cognitive function in HF patients, and seven studies (24, 37, 40, 48–51) described the relationship between BMI and cognitive function in HF patients. A total of five studies (39, 52–55) described the relationship between electrolyte levels and cognitive function in HF patients, whereas six studies (41, 46, 57, 81–83) described the relationship between BNP/NT-proBNP levels and cognitive function in HF patients, and 15 studies (23, 42, 44, 48, 56–66) described the relationship between depression and cognitive function in HF patients. In addition, five studies (21, 67–70) described the relationship between AF and cognitive function in HF patients, six studies (49, 55, 61, 69, 71, 72) assessed the relationship between DM and cognitive function in HF patients, six studies (40, 55, 59, 63, 71, 73) evaluated the relationship between anemia and cognitive function in HF patients, and eight studies (32, 74–80) described the relationship between sleep disorder and cognitive function in HF patients.
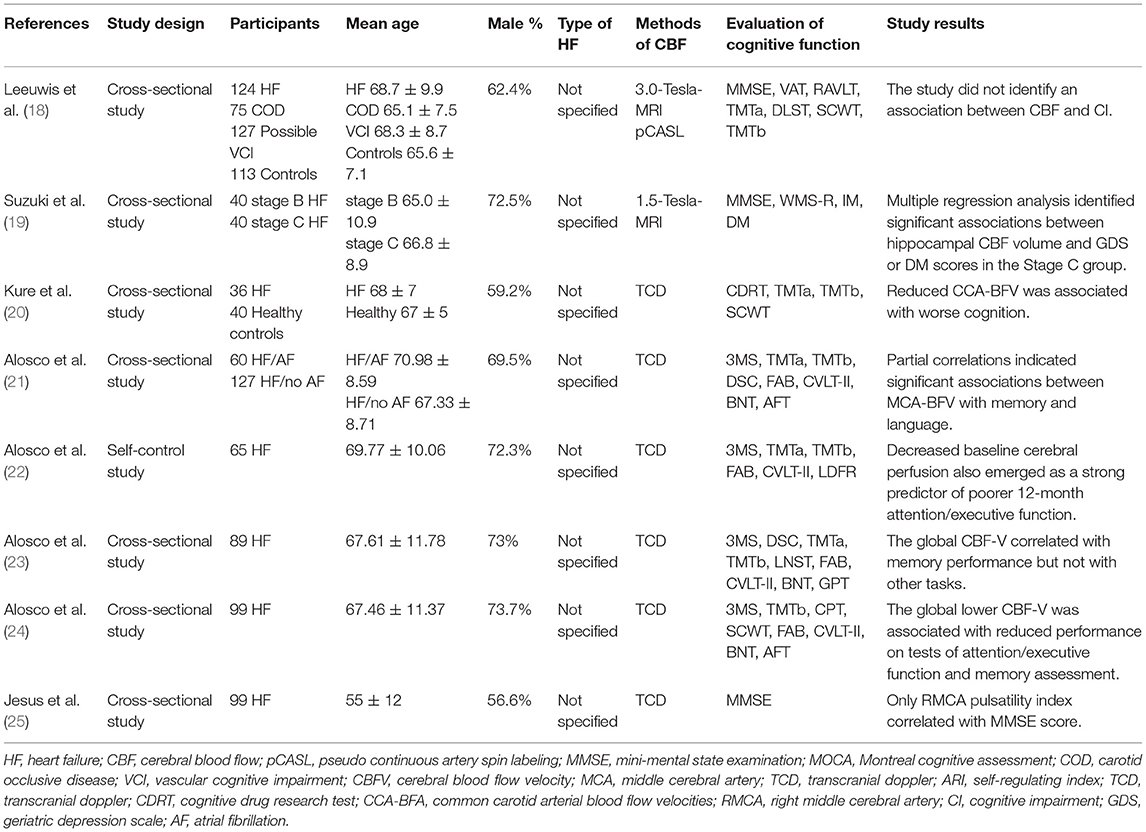
Table 1. The association between the changes noted in the CBF volume and the cognitive function in patients with HF.
Quality Assessment of Included Studies
This systematic review included 66 relevant clinical studies, including 55 cross-sectional studies, three cohort studies, five case-control studies, and three self-control studies.
The results of using AHRQ to evaluate the risk of bias and method quality of 55 cross-sectional studies are shown in Supplementary Material 2 (Supplementary Table 1). A total of seven studies (18, 30, 37, 40, 41, 68, 82) were included with high quality, and the remaining 48 studies were with medium quality. Specifically, all the included cross-sectional studies contained clear data sources, the inclusion and exclusion criteria, and the evaluations for quality assurance purposes. No subjective factors were included that could affect each study. Only one study (67) described a possible strategy to compensate for lost data. A total of five studies (30, 63, 67, 70, 82) followed up the patients and explained the follow-up results. A total of four studies (25, 29, 32, 47) did not present a plausible explanation of how to evaluate and control confounding factors. In addition, a total of 26 studies indicated the period of identifying patients, whereas 17 studies indicated that the included subjects were continuous. A total of 23 studies explained the reasons for exclusion of patients in the analysis, and 17 studies summarized the response rate and the integrity of data collection.
The results of using NOS to evaluate the risk of bias and the method quality of five case-control studies are shown in Supplementary Material 2 (Supplementary Table 2). The mean score of NOS in the included case-control studies was 5.8. One study (27) was of high quality, and 4 studies (32, 44, 61, 66) exhibited medium quality. Specifically, all studies indicated that the diagnosis of the disease was adequate, and included the definition of control, and the determination and method of exposure. Only the most important confounding factors were controlled in the feasibility analysis. Only two studies (27, 61) collected representative cases in succession. No study indicated that the non-response rates of the study group and the control group were the same. In terms of control selection, two studies (27, 44) selected community control and three studies (32, 61, 66) hospital control, respectively.
The results of using NOS to evaluate the risk of bias and the quality of the methods of three cohort studies are shown in Supplementary Material 2 (Supplementary Table 3). The mean score of NOS in the included cohort studies was 7.3. A total of two studies (34, 35) exhibited high quality, and one study (80) was of medium quality. The exposure cohorts of all studies were well-represented, whereas the non-exposure cohorts and the exposure cohorts were from the same community. The determination of exposure and the evaluation of outcome events were reliable, and only the most important confounding factors were controlled in the feasibility analysis. In addition, two studies (34, 35) reported on optimal adequacy and completeness of the follow-up period.
The results of using MINORS to evaluate the risk of bias and the quality of the methods of three self-control studies are shown in Supplementary Material 2 (Supplementary Table 4). A total of two studies (22, 83) exhibited high quality, and one study (50) exhibited medium quality. All three studies (22, 50, 83) indicated the clarity of research purpose, the representativeness of endpoint indicators, sufficient follow-up time, and the low loss of follow-up rate. However, neither study exhibited prospective data collection, unbiased assessment of endpoint indicators, prospective calculation of the study size, and setting of a control group. In addition, only two studies (22, 83) indicated the consistency of the included patients.
Outcome Analysis
Association of Brain Imaging and Cognitive Function in Patients With HF
Association of CBF Changes and Cognitive Function in Patients With HF
A total of eight studies reported the association between cognitive function and the changes of CBF in patients with HF. The details of the studies are shown in Table 1. Firstly, two studies (18, 20) revealed that the CBF volumes of the HF patients were significantly reduced compared with those of the control group. Secondly, seven studies (19–25) demonstrated that the decrease in CBF volume or cerebral blood flow velocity (CBF-V) was significantly associated with the impairment of multiple cognitive domains in HF patients. A longitudinal study (22) indicated that the reduced CBF volume at baseline in HF patients was a powerful predictor of poor attention/executive function following 12 months of intervention. A total of two studies demonstrated that the reduced CBF-V volumes of the common carotid arterial (CCA) (20) or the right middle cerebral artery (RMCA) (25) were significantly correlated with the decline of cognitive function in HF patients. A previous study indicated that the blood flow of the hippocampal tissues of patients with HF symptoms in stage C was significantly decreased compared with that of patients with structural heart disease without HF symptoms in stage B (19). In addition, it was positively correlated with the score of delayed memory. The changes noted in the CBF volume were examined in three studies (21, 23, 24), which elucidated that the combination of CBF decline with AF, depression, or BMI may result in higher number of adverse effects on attention, executive function, memory, and other cognitive areas of HF patients. However, one study (18) indicated that the CBF value was lower in the HF group, whereas no significant correlation was noted between CBF and CI compared with the control group.
Effects of MTA on Cognitive Function in Patients With HF
A total of three studies reported on the association between cognitive function and MTA in patients with HF. The details of the studies are shown in Table 2. Firstly, it was shown by two studies that MTA was more pronounced in HF patients than healthy controls (26, 27). One of these studies (27) pointed out that the degree of atrophy of the right temporal lobe was more obvious. Secondly, three studies revealed the correlation between MTA and cognitive decline in HF patients. Specifically, a previous study (26) suggested that MTA was significantly associated with the severity of overall CI and deficits in cognitive areas, such as attention and verbal memory in HF patients. Another study (28) demonstrated a similar conclusion, in which MTA was associated with memory impairment and executive dysfunction in HF patients. Furthermore, the association was found only in left MTA in a case-control study (27).
Effects of the Changes Noted in GM and DM on the Cognitive Function of Patients With HF
A total of two studies reported on the association between cognitive function and the changes of GM in patients with HF. The details of these studies are shown in Table 3. Both studies (29, 30) indicated the correlation between decreased total GM and cognitive decline in HF patients. Furthermore, it was pointed out (29) that this correlation may be more evident on attention/executive function.
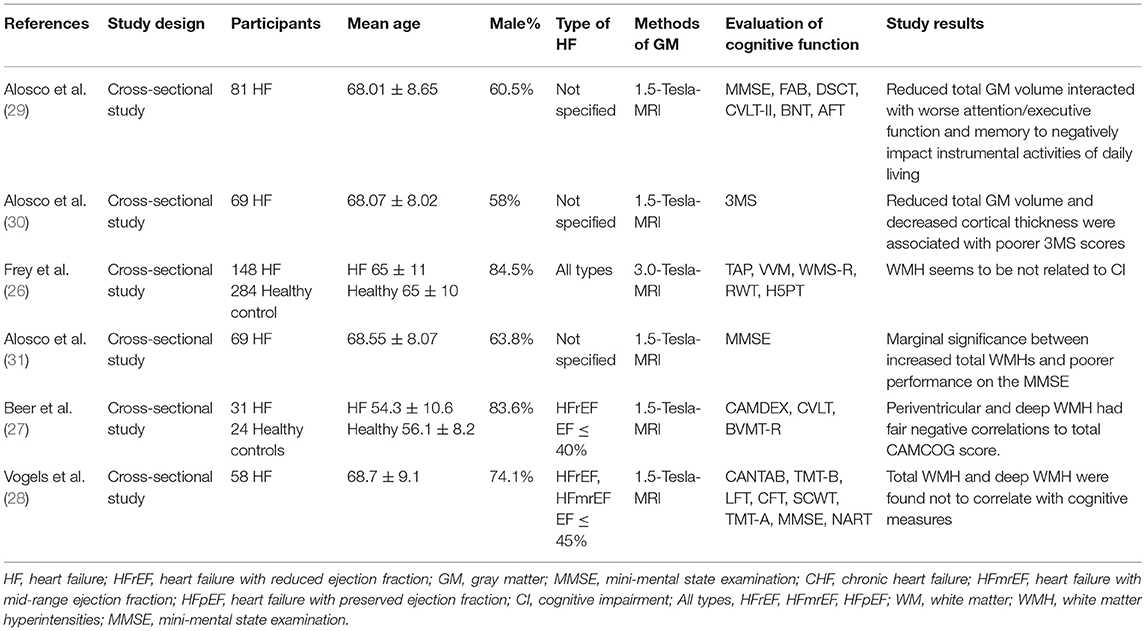
Table 3. The association between changes noted in the GM and WM with cognitive function in patients with HF.
A total of four studies reported on the association between cognitive function and the changes of WM in patients with HF. The details of the studies are shown in Table 3. A total of two studies reported the moderate (27) or marginal (31) correlation between white matter hyperintensities (WMH) and cognitive function score in HF patients. However, two other studies (26, 28) revealed that WMH was not related to cognitive function scores.
Effects of EF on Cognitive Function in Patients With HF
The correlation between EF and cognitive function in patients with HF was reported in nine studies, as well as in seven studies with differences in cognitive function among different subtypes of HF. The details of the studies are shown in Table 4.
Previous studies (four in total) that examined the association between EF and cognitive function (40, 41, 44, 46) suggested no significant correlation between these two parameters. However, a set of five different studies exhibited different results. These data indicated that EF was significantly correlated with cognition in HF, notably when it was <30%, which was noted in the total cognitive ability (45, 47), attention (38), memory (43), and subjective cognition (42), respectively.
A total of seven (32–37, 39) studies investigated the differences in cognitive function among patients with different types of HF. Among them, two large prospective cohort studies (34, 35) (n = 4,864/6,495) indicated no significant differences in the decline of cognitive function scores in heart failure with reduced ejection fraction (HFrEF) and heart failure with preserved ejection fraction (HFpEF) patients following 5 or 15 years of follow-up, respectively. Similar results were obtained from the other two observational studies (33, 36). However, three studies had reached opposite conclusions, and two studies (32, 37) pointed out that patients with HFpEF exhibited lower cognitive function scores at baseline compared with those of patients with HFrEF and control subjects. Finally, it was also shown (39) that HFrEF exhibited lower MOCA scores relative to HFpEF.
Effects of Specific Detection Indices on Cognitive Function in Patients With HF
Effects of BMI on Cognitive Function in Patients With HF
A total of seven studies reported the association between BMI and cognitive function in patients with HF. The details of studies are shown in Table 5. A total of two studies (37, 49) reported that the increase in BMI was significantly correlated with the decrease in the cognitive function of HF patients. BMI was associated with multiple cognitive domains in HF patients including attention, language, and executive function (24, 50, 51). Furthermore, for every 1 unit decrease in BMI, the improvement of the executive function in patients with HF was 1.4% (50). However, another two studies (40, 48) exhibited the opposite conclusions, and it was shown that negative correlations were present between BMI and cognitive function in HF patients.
Effects of Electrolyte Levels on Cognitive Function in HF Patients
A total of five studies reported on the correlation between electrolyte levels and cognitive function in patients with HF. The details of the studies are shown in Table 6. A large secondary analysis study (55) (n = 1,511) indicated that serum sodium (<135 mEq/l) and potassium (<3.6 mEq/l) levels exhibited a significant negative correlation with the degree of CI in patients with HF. An additional study (39) suggested that hypokalemia significantly increased CI, whereas no correlation was found. In addition, high sodium levels may also exhibit adverse effects on cognitive function in patients with HF, and a pertinent correlation was noted in a study (54) following completion of a dietary questionnaire. In contrast to these findings, a previous study (53) demonstrated that in patients with HF who ingested excessive sodium and insufficient potassium, the increased Na/K ratio was significantly associated with CI. However, in another study (52), a different conclusion was reached, and it was suggested that by measuring urinary sodium excretion in HF patients, sodium consumption exceeded the recommended amounts, and was unrelated to cognitive function.
Effects of BNP/NT-proBNP Levels on Cognitive Function in HF Patients
A total of six studies reported on the association between cognitive function and BNP/NT-proBNP levels in patients with HF. The details of these studies are shown in Table 7. A large cross-sectional study (82) discovered (n = 951) that BNP was an indicator of severity in HF patients, and exhibited a significant correlation with the MMSE score. Similar results have been shown by other studies (46, 47). Another study (57) indicated a correlation between Log BNP and ACE-R (Addenbrooke's cognitive examination-revised) fluency subtest in HF patients. In addition, NT-proBNP was independently associated with cognitive function in patients with HF (81). Notably, van Vliet et al. (83) described that the combination of high NT-proBNP levels and low systemic BP could predict the decline in cognitive function in HF patients following 5 years of follow up and adjustment for the relevant confounding factors.
Effects of Comorbidities on Cognitive Function in Patients With HF
Effects of Depression on Cognitive Function in Patients With HF
A total of 15 studies reported on the association between cognitive function and depression in patients with HF. The details of these studies are shown in Table 8. A total of 11 studies demonstrated a correlation between the aforementioned parameters. A total of six studies (56, 57, 59, 61, 63, 66) reported that depression was a significant risk factor for cognitive decline in HF patients. Furthermore, the association was mainly driven by non-somatic symptoms of depression (60). Depression can affect multiple cognitive fields in HF patients. A total of three studies (23, 62, 64) indicated that depression was related to the impairment of memory function, attention, executive ability, language ability, and other cognitive fields in HF patients. In addition, the combined effects of depression and physical weakness increased the risk of CI in HF patients (58). However, four studies had found opposite results. A total of three cross-sectional studies (42, 48, 65) suggested that no significant correlation was present between depressive symptoms and/or the severity of depression and cognitive function in HF patients. Similar results were obtained from another case-control study (44).
Effects of AF on Cognitive Function in Patients With HF
A total of five studies reported on the association between cognitive function and AF in patients with HF. The details of the studies are shown in Table 9. A large cross-sectional study (69) (n = 881) suggested that permanent atrial fibrillation (permAF) was an independent risk factor for CI in HF patients. The same conclusion was reported in another cohort study (70) (n = 331). A total of two cross-sectional studies (21, 68) further showed that HF patients with AF exhibited worse cognitive function and memory ability compared with patients without AF. In addition, in terms of cognitive function, HF and AF interacted with each other. In a large prospective cross-sectional study (67) (n = 1,244) with 1-year follow-up, it was shown that HF was independently associated with CI at baseline in AF patients, but not with cognitive function after follow-up.
Effects of DM on Cognitive Function in Patients With HF
A total of six studies reported on the association between cognitive function and diabetes mellitus (DM) in patients with HF. The details of the studies are shown in Table 10. A total of two studies (55, 69) indicated that diabetes was independently associated with CI in patients with HF following adjustment for potential confounding factors, while three studies (49, 71, 72) demonstrated that DM was positively correlated with cognitive dysfunction. Another large longitudinal study (61) (n = 702) further suggested that DM was particularly related to an increased risk of 10-year progression to vascular dementia in HF patients.
Effects of Anemia on Cognitive Function in Patients With HF
A total of six studies reported on the association between cognitive function and anemia in patients with HF. The details of the studies are shown in Table 11. Multiple studies (40, 55, 59, 63, 71, 73) elucidated that the severity of anemia was negatively associated with cognitive status in patients with HF. Furthermore, three studies (40, 55, 63) indicated that the correlation existed independently. Compared with non-anemic HF patients, the combined anemia increased the risk of CI (73).
Effects of Sleep Disorder on Cognitive Function in Patients With HF
A total of eight studies reported on the association between cognitive function and sleep disorders in patients with HF. The details of the studies are shown in Table 12. The effects of sleep on cognitive function in HF patients are contradictory. However, the majority of the large-sample studies have deduced that sleep will affect cognitive function. Daytime sleepiness is closely related to CI in HF patients, which would lead to the decline of memory, executive function (77), and attention (78). HF patients were accompanied by excessive daytime sleepiness (EDS) and were more likely to exhibit problems of failure to adhere to medication, which may lead to a decline in attention function (80). Furthermore, insomnia was associated with the decline of overall cognitive function in HF patients (79). Another cross-sectional study (76) further revealed that cognitive function in HF patients of different ages was related to different types of sleep disorders. Specifically, cognitive function was associated with daytime sleepiness in adults and poor nighttime sleep quality in older adults. However, this study (75) did not indicate a correlation between daytime sleepiness and cognitive function in HF patients. In addition, sleep-related breathing disorders may also affect cognitive function in HF patients. A significant negative correlation was noted between cognitive function score and oxygen saturation index (ODI) (32) in HF patients compared with the apnea-hypopnea index (AHI) (74).
Discussion
CI is a common concurrent disease of HF, with a high incidence in the clinic. It exerts negative effects on the prognosis of HF patients. Although the etiology and pathological mechanism of this disease are not completely clear, it is undeniable that this is due to the interaction and common effects of multiple factors. An increasing number of studies have explored the discovery of the risk factors and imaging changes of CI in patients with HF. The risk factors affecting cognitive function in HF patients include decreased EF and electrolyte levels, notably sodium and potassium ions, or proportion imbalance, high BMI, increased BNP, and co-morbidities such as AF, DM, anemia, depression, and sleep disorders. The data indicated that these risk factors exhibited negative effects on cognitive function by different mechanisms of action and that they could affect the prognosis of HF patients.
The Effects of Imaging Changes on Cognitive Function in Patients With HF
HF is caused by cardiac filling and/or systolic dysfunction as a result of various factors, which leads to a decrease in cardiac output and cannot meet the needs of tissue metabolism. Brain damage caused by reduced cardiac output may be an important cause of CI in patients with HF and lead to related brain imaging changes. The related imaging changes mainly include the CBF, medial temporal lobe, WM, GM, and hippocampus. At present, the main findings reported on the changes of the hippocampus in HF patients are that the volumes of the hippocampus and parahippocampal gyrus are smaller (84–86), whereas only one study (86) reported on the correlation between cognitive function and hippocampal changes. Therefore, the present study only systematically analyzed the impact of the other three imaging changes on the cognitive function of HF patients.
In patients with HF, low cardiac output or impaired automatic regulation of vascular tension may lead to decreased CBF and hypoxia in the brain tissue (87, 88). The brain is very sensitive to ischemia and hypoxia, which may lead to neuron loss, glial cell proliferation, and activation of oxidative stress (20, 89). These often occur in the prefrontal lobe, temporal lobe, hippocampus, amygdala, and other parts, which may result in the decline of cognitive function (90).
In the current systematic review, seven studies (19–25) demonstrated the correlation between the decline of CBF and CI in HF patients with the exception of one study (18). The possible reasons for the negative result are as follows: Firstly, the present study did not control the confounding factors, such as hypertension and diabetes. The proportion of these HF patients was higher than those complicated with these diseases in the control group. Moreover, the study included all types of HF patients and did not analyze the correlation between cognitive function and CBF of different types of HF. It is important to note that the present study used large ROI (frontal, parietal, temporal, and occipital brain lobes), which may mask the subtle regional associations with cognitive function. Moreover, previous studies (21, 23, 24) have found that the combination of CBF decline with AF, depression, and BMI may further damage the cognitive function of HF patients, possibly due to the fact that AF, BMI, and depression can also aggravate the CI of HF patients or affect cerebral hemodynamics. Furthermore, the different measures of CBF used in the included studies affected the correlation results. A total of two studies (18, 19) used MRI to detect the CBF of participants, while other studies (20–25) used TCD to detect the blood flow velocity of cerebral vessels to represent the changes of CBF. In contrast to these observations, the use of MRI can objectively reflect the changes of CBF in HF patients, since TCD is more likely to be affected by the examiner's technique. In conclusion, the decrease in CBF volume may be associated with CI in HF patients.
The temporal lobe is located below the lateral fissure of the brain and the main functions are related to hearing, language, and memory. The medial temporal lobe mainly includes the hippocampus and its surrounding areas, which play an important role in the generation and storage of memory. The decrease of EF in HF patients leads to cerebral ischemia and hypoxia, which may lead to MTA. A previous study (91) has shown that compared with the healthy control group, the MTA of HF patients was significantly increased. MTA may lead to a series of memory problems. Several studies (92, 93) suggested that MTA was related to CI caused by various factors, including Alzheimer's and cerebrovascular diseases. Furthermore, the degree of MTA could be used as a clinical marker for the transformation from mild CI to dementia (94).
In the present system review, three studies (26–28) had reached similar conclusions and demonstrated a correlation between MTA and cognitive decline in patients with HF. It is interesting to note that CI in patients with HF was only associated with left MTA, but not with right MTA as demonstrated by a previous study (27). The possible explanation for this finding was that the observed differences may be false and that they could be attributed to methodological factors, small sample size (n = 55) as well as the relatively young age of the included HF patients.
GM and WM are important components of the central nervous system. GM is mainly composed of neuronal cell bodies, which is the part of the central nervous system that deeply processes a variety of information, such as sensation and movement. WM is mainly composed of projections surrounded by myelin sheath in neurons, which mainly play a role in the transmission of information. Brain hypoxia/ischemia in HF patients caused due to long-term decline of cardiac output may lead to chronic damage in brain tissues, such as GM and WM (95, 96). In HFpEF patients, diastolic dysfunction may lead to systemic hypoperfusion as a result of the reduction of left ventricular flow, resulting in the development of WM lesions (97). In HF patients, loss of GM may occur to a higher extent in the bilateral parahippocampal gyrus, bilateral cingulate gyrus, left superior temporal gyrus, and middle gyrus (98). Total WM injury is increased with the duration of HF (99). These may have negative impacts on cognitive function in HF patients.
A total of two studies (29, 30) have shown the correlation between the reduction of GM volume and the decline of cognitive function in HF patients. However, since the sample size used was small and no control group was present, the universality of the conclusion is limited. Therefore, the changes in GM may be associated with CI in HF patients. However, large-scale prospective studies are required to confirm this hypothesis.
At present, no consistent conclusion has been proposed regarding the relationship between WM changes and cognitive function in HF patients. A total of two studies (26, 28) suggested that WMH was not associated with cognitive function scores in HF patients, while two studies (27, 31) demonstrated that WMH may be mildly or moderately related to cognitive function. The possible causes may be related to a variety of different factors. It was shown that WMH (26) was influenced by several factors, including HF patients with hypertension, DM, AF, and various other diseases, whereas it included higher number of male participants (84%). However, one of these studies included non-demented HF patients, with relatively normal cognitive function, which may also be the reason for the negative results (28). Cerebral WM is affected by several factors, and the complexity of clinical HF patients may also account for the opposite conclusion. Therefore, the changes of WM may not be related to CI in HF patients, which must be confirmed by large-scale prospective studies.
Effect of LVEF on Cognitive Function in Patients With HF
In terms of the common classification, HF can be classified into the three following groups according to the percentage of EF: HFrEF (EF < 40%), HF with mid-range EF (HFmrEF: 40 ≤ EF < 49%), and HFpEF (EF ≥ 50%). HFmrEF can progress into either HFrEF or HFpEF (100). The decline of LVEF (HFdEF) in HFpEF patients during disease progression is a special type of HF, with grave outcomes (101). HFrEF and HFpEF are the most common types and account for approximately the same proportion in HF populations (102). Certain differences have been noted between HFrEF and HFpEF in the development and progression of the HF disease subtypes. HFrEF is mainly manifested as decreased left ventricular systolic function, and HFpEF is mainly manifested as severe left ventricular diastolic function and increased operative diastolic elastance (ED)/effective arterial elastance (EA), which is more pronounced in women (103). The left ventricular diastolic dysfunction of HFpEF is associated with diffuse myocardial fibrosis (104).
EF is a key factor affecting the progression and prognosis of patients with HF, and it may also affect the cognitive function of patients. Previous studies that investigated EF and CI in HF patients did not reach consistent conclusions, and some of these findings were contradictory. The possible reason for these findings was the average EF level in enrolled HF patients. A total of three studies (38, 43, 47) demonstrated that low EF exhibited adverse effects on certain cognitive domains of HF patients, notably when EF was <30%. The remaining six studies (40–42, 44–46) did not analyze the correlation between EF and cognitive score according to the different range of EF, which may affect the conclusions. The average EF of the study (41) participants was 43.4 ± 15.8%, including HF patients with diastolic dysfunction, which may also affect cognitive function. Therefore, when EF decreases significantly (<30%) in patients with HF, cognitive function may be impaired. When EF > 30%, the effect of EF on cognitive function may not be particularly clear.
Current studies have also yielded inconsistent conclusions regarding the differences in cognitive function among patients with different types of HF. This may be due to the different EF values defined in the EF classification, which were 40% (39), 45% (34, 36), and 50% (35), respectively. The criteria of the three studies (32, 33, 37) were unclear. The inconsistent classification of patients with HF may have a greater impact on the research results. The EF standard of HFrEF was shown to be lower and the damage to cardiovascular and cerebral perfusion was more serious as demonstrated in a previous study (39). In addition, HFpEF patients are often accompanied by diastolic dysfunction, which also damages cognitive function. Therefore, when the defined EF is high, the cognitive scores of HFrEF and HFpEF may be similar.
The possible mechanism is related to the damage of the brain structure and the accumulation of pathological products. The decrease in EF levels may lead to cerebral hypoperfusion, whereas the decrease of EF in patients with HF was significantly correlated with the decrease of GM density in the frontal lobe as well as in the hippocampus and precuneus, which also indicated the existence of cognitive-related brain structure damage (105). In addition, the levels of Neuron Specific Enolase (NSE) are significantly increased in HFrEF patients during the acute decompensated phase, demonstrating an increased rate of SCI (106). It has been proposed that low EF is associated with increased CSF t-tau and p-tau levels in normal cognitive subjects, suggesting that lower EF may lead to neurodegeneration (107). All of these may exacerbate the impairment of cognitive function.
Effect of Specific Detection Indices on Cognitive Function in Patients With HF
BMI refers to the square of weight/height (kg/m2) and is an important international index to measure the degree of obesity. The World Health Organization (WHO) states that normal BMI is between 18.5 and 24.9 kg/m2, whereas a BMI value between 25 and 29.9 kg/m2 corresponds to overweight subjects, and a BMI ≥ 30 kg/m2 is used to characterize obese adults (108).
A total of four studies (24, 37, 49, 50) indicated that BMI was significantly and positively correlated with cognitive function in HF patients. Several possible mechanisms have been proposed for the influence of BMI on the cognition function of HF. Firstly, BMI may affect the brain structure of HF patients. A previous study (109) suggested that a high-fat diet could increase oxidative stress in rats, resulting to damage in the hippocampal neurons. In addition, the increase in BMI interacted with cerebral perfusion, resulting in the decrease of WM and GM (108). A higher BMI value in HF can increase the risk of complications, such as hypertension and T2DM, resulting in poor health, and it may aggravate cognitive dysfunction by affecting endothelial, microvascular, nervous system, and other functions (49). Moreover, BMI and obesity are associated with the changes in the levels of circulating biomarkers, such as brain-derived neurotrophic factor (BDNF), amyloid-beta, and leptin, which can reduce cognitive function in multiple domains (110), notably in attention and executive function (24).
A previous study (51) demonstrated that this association may only apply to male patients. The possible reason was that the obese female patients included in that study were 5.7 years younger than the obese male patients and 8.2 years younger than the non-obese women, and age was a confounding factor affecting the evaluation of the correlation index. Concomitantly, men with more ischemic factors and bad habits would also affect the assessment of the results obtained. A total of two studies (40, 48) reached the opposite conclusion. The lower EF, the more the anxiety and the depression of the participants. Malnutrition or sarcopenia in patients with end-stage HF may be another factor that could explain these findings.
Electrolyte disturbances often occur during the development and treatment of HF, notably with regard to the imbalance of sodium and potassium ions. Excessive intake of sodium leads to the retention of sodium and water, which can aggravate HF. Moreover, since the majority of the patients with HF receive diuretic therapy for a long time period, an imbalance in sodium and potassium can be caused. For example, administration of a loop diuretic for a long period of time can lead to low levels of serum sodium and potassium, while administration of an aldosterone antagonist can increase the content of potassium due to the mechanism of potassium-sparing. The imbalance of sodium and potassium intake is associated with major cardiovascular events and mortality (111).
A total of four studies demonstrated that the high (54) or low (39, 55) sodium and potassium ions as well as the imbalance of sodium/potassium ratio (53) were associated with cognitive decline in HF patients. Several mechanisms may account for these results. High or low blood sodium may affect cognitive function. An in vivo study (112) demonstrated that the extracellular glutamate concentration was increased in the hippocampus of rats with chronic hyponatremia, and the sustained low extracellular sodium ions concentration reduced further the glutamate uptake by primary astrocytes, resulting in damage to the long-term enhancement mechanism (LTP). Hyponatremia could also directly affect the distribution of the mitochondria, reducing the content of ATP in neurons. The long-term increase in blood sodium levels will lead to dehydration of brain cells, reduced volume, and decreased antioxidant capacity of the hippocampus (113, 114). Furthermore, blood potassium levels are considered another cause of CI, and insufficient potassium intake may lead to the accumulation of Alzheimer's disease-related pathological products, such as the Aβ protein (115).
However, the levels of urinary sodium were not associated with CI (52). Administration of mixed diuretics could be the main factor affecting patients with severe CI (unable to complete two urine collections, 47% of all participants) although correlation analysis was not performed.
BNP and NT-proBNP are the most promising biomarkers in the clinic, and are mainly used for evaluating cardiac function and the presence of myocardial injury (116). BNP is released from ventricular muscles in response to the increase of ventricular wall stress (117). BNP and NT-proBNP have equally powerful and independent predictive effects on all-cause death and rehospitalization of HF patients (OR 1.46 vs. 1.45) (118). In addition, the increase of BNP and NT-proBNP levels predicts the accelerated decline of cognitive function in the elderly (119). Multiple studies (46, 47, 57, 81–83) have demonstrated that this association may be more significant in patients with HF.
The mechanism of BNP and NT-proBNP affecting cognitive function in patients with HF is unclear. Several possible reasons may account for these findings. BNP reflects left ventricular systolic and diastolic function and is associated with the incidence of additional heart diseases, such as myocardial infarction, AF, valvular disease, and renal function (120). Therefore, BNP may reflect the comprehensive effects of these processes on cognitive function. Higher concentration levels of NT-proBNP were significantly associated with a smaller brain GM volume, which may lead to the decline in cognitive function (121).
Effects of Comorbidities on Cognitive Function in Patients With HF
HF patients are often accompanied by a variety of other diseases, such as AF, anemia, diabetes, depression, and sleep disorders. These diseases may also affect cognitive function in HF patients.
Depression is very common in HF patients. A previous study indicated that 47.13% of HF patients exhibited depressive symptoms (122). Depression causes more physical symptoms, and increases the hospitalization, mortality, and financial burden of the patients (123, 124).
Previous studies on depression and cognitive dysfunction of HF patients have not reached consistent conclusions, and contradictory results have also been reported. The correlation between cognitive function and depression in HF patients was discovered in 73% of the studies. Furthermore, a previous study (60) indicated that the association between cognitive function and depression in HF patients was mainly driven by non-somatic symptoms of depression. The somatic subscale (three items) exhibited lower number of items compared with that of the non-somatic subscale of PHQ-9 (six items), which may also lead to the correlation between somatic symptoms and cognition.
The underlying mechanism of depression leading to cognitive decline in HF patients is not completely clear. In the present study, several possible reasons may be attributed for these results. It has been shown by various in vivo studies that cytokines, such as IL-1 β, IL-6, CX3CR1, and hypocretin-1 are involved in the pathological processes of depression affecting cognition, which may be mediated through direct and indirect effects on long-term enhancement, neurogenesis, and synaptic plasticity (125–127). In contrast to these observations, the occurrence and development of depression may affect cognitive function by leading to deep subcortical WMH, thinning of the hippocampal cortex and abnormal functional connection of the cognitive network (128–130). In addition, depressive symptoms can cause CI, such as attention and executive function in HF patients by exacerbating hypoperfusion, overstimulating the sympathetic nervous system, and reducing mental space, and mental flexibility (131).
However, this association was not found in 27% of studies, and the possible reasons may be multifaceted. In the present study, 55% of HF patients were obese, and the confounding factor was not excluded in the correlation analysis (48). In a previous study (42), the included patients were younger, and 60% of the patients exhibited HF caused by dilated cardiomyopathy, whereas only 1.8% were patients with CI, which may lead to selection bias. The same bias was reported by a previous study (65), which suggested that patients with CI accounted for only 10% of the total sample. However, although MHI was applied to depression assessment (44), the assessment tool was not sensitive enough to capture the extent of the negative mood state, whereas patients with severe CI may lack emotional insight and processing. In summary, these conflicting findings are due to different samples (age of patients, sample size) and to the different instruments used to assess cognitive function, as well as due to the different scales used to evaluate depressive symptoms. Therefore, depression may aggravate CI in patients with HF.
AF is the most common arrhythmia disorder noted in clinical practice, and it is also a cardiovascular disease complication, which is frequently followed by HF. The prevalence of AF in HF patients was 10.6% (132). AF was significantly correlated with cognitive dysfunction in HF patients. A total of five studies (21, 67–70) demonstrated that AF was significantly associated with CI in patients with HF. The mechanism of cognitive decline following AF may be related to cerebral hemodynamic changes caused by irregular rhythm and brain injury caused by microemboli. Arrhythmia and rapid ventricular rate may lead to abnormal changes of CBF volume in AF patients. Saglietto et al. (133) suggested that the higher ventricular rate was associated with a gradual increase in hypoperfusion and hypertensive events by simulating the relationship between ventricular rate and cerebral hemodynamic changes in AF patients. The mechanism of its occurrence may be attributed to irregular ventricular rates in AF patients triggering a higher variability of the distal cerebral hemodynamic variables, which leads to severe cerebral hemodynamic events of reduced blood flow or excessive pressure (134). Moreover, the alteration of microcirculation hemodynamic (pressure and flow rate) patterns during AF may be involved in the occurrence and development of cerebral hypoperfusion (135). Hemodynamic disorders in patients with AF may lead to CI due to chronic ischemia, whereas in the case of brain injury, asymptomatic cerebral infarction may also be a key factor affecting the cognitive function of AF patients. AF was found to be a risk factor for all cortical microinfarcts, and exhibited adverse effects on cognitive function (136). Moreover, Galenko et al. (137) indicated that the expression levels of the biological markers of brain injury, such as astrocyte-specific glial acidic fibrillary protein (GFAP) and microtubule-associated Tau protein were significantly higher in patients with AF than those noted in normal subjects. These studies indicated that brain injury was common in patients with AF. Microthromboembolism derived from the left atrial fibrosis, complex plaque in the aortic arch, or mobile plaques may be important causes of SCI in patients with non-valvular AF (138). These brain injuries contribute to the impairment of cognitive function in AF patients. In addition, the decline of brain autoregulation and connectivity of brain network regions in patients with AF may also lead to the decline of cognitive function. Junejo et al. (139) demonstrated by using specific experiments that patients with AF exhibited impaired neurovascular coupling responses to visual stimulation, while the automatic regulation function of the brain was weakened. Silva et al. (140) demonstrated that the patients with AF exhibited decreased connectivity in regions of the default mode network (DMN) including the frontal lobe (left superior frontal gyrus and left medial frontal gyrus), bilateral precuneus, and left angular gyrus.
However, this study (70) did not yield an association between HF and cognitive function in patients with AF following 1 year of follow-up, which may be due to the short follow-up time period as well as the absence of collection and analysis of the changes in the conditions of HF patients during this period.
DM is the most common endocrine disorder and is also considered to be a common complication of HF. Epidemiological studies have shown that the prevalence of DM ranges between 10 and 47% in the HF cohort, including HFrEF and HFpEF (141). DM is closely related to diastolic dysfunction, decreased quality of life, and increased mortality in HF patients (142, 143). In addition, DM is a significant risk factor for cognitive decline in patients with HF, which has been confirmed by several studies (49, 55, 61, 69, 71, 72). The mechanism of action may be associated with several factors. Hyperglycemia possibly leads to hippocampal tissue damage. An animal study (144) suggested that overexpression of Dipeptidyl peptidase-4 (DPP4) in diabetic rats may reduce peroxisome proliferator-activated receptors γ-Coactivator 1α expression, leading to mitochondrial dysfunction. Hyperglycemia may also increase the levels of inflammatory cytokines, such as interferon-γ (IFN-γ) and interleukin-6 in the hippocampus, resulting in the apoptosis of hippocampal neurons (145). Abnormal expression of vascular endothelial growth factor (VEGF) and insulin resistance in DM patients may also account for the damage of WM as well as the decreased volume of CBF in the hippocampus, occipital lobe, and default model network related areas (146–149). In addition, pathological cerebrovascular neovascularization, increased blood-brain barrier (BBB) permeability, cerebrovascular pericyte dysfunction, and synaptic protein injury may be responsible for the ability of hyperglycemia to affect cognition (150–152).
Anemia is present in more than 50% of HF patients and affects the quality of life and the prognosis of these patients (153, 154). According to WHO, anemia is defined as hemoglobin concentration (Hb) < 130 g/l for adult men, < 120 g/l for adult women, and < 110 g/l for pregnant women. The main causes of anemia in CHF patients are kidney damage, anemia of chronic disease, and iron deficiency. Other factors, including hypothyroidism, deficiency of vitamin B12 and folate are also affecting the development of this disease (155). In the present study, six studies (40, 55, 59, 63, 71, 73) demonstrated that anemia was a risk factor for cognitive deterioration in patients with HF. The possible biological mechanism may be due to brain injury caused by decreased hemoglobin. It has been shown by certain studies that low hemoglobin can cause chronic hypoxia of brain cells, mitochondrial dysfunction, and oxidative stress, resulting in decreased CBF and hippocampal volume, reduced amyloid deposition as well asatrophy of the frontal and temporal cortex (156–161). Anemia is usually caused by iron deficiency or vitamin B12 and folic acid insufficiency. Iron deficiency may induce the decline in cognition, notably in learning and memory function, by decreasing the expression and function of insulin-like growth factors I and II (IGF-I/II) and brain-derived neurotrophic factor (BDNF) in specific areas of the brain (162). The deficiency of vitamin B12 and/or folate can elevate homocysteine levels, which can affect cognitive function by inducing WM damage, brain atrophy, and neurofibrillary tangles (163).
The incidence rate of sleep disorders is high in patients with HF. In a cross-sectional study of 267 HF patients, 61.8% of participants exhibited poor sleep quality (Pittsburgh Sleep Quality Index ≥5) and 22.5% demonstrated excessive daytime sleepiness (Epworth Sleepiness Scale > 10) (75). Poor sleep quality, excessive daytime sleepiness, sleep-disordered breathing (SDB), and insomnia may aggravate cognitive dysfunction in this population.
Among them, daytime sleepiness and SDB have demonstrated significant effects on the cognitive function of HF patients (76–78, 80). It is interesting to note that this study (76) notably emphasized on the significant correlation between cognition and daytime sleepiness in adults with HF (≤ 65). The reason is unclear, and may be related to the fact that adults are more sensitive to body input and sleep drive than the elderly. However, a different study (75) reached the opposite conclusion, possibly due to using self-reported measures of sleep quality and daytime dysfunction, and presenting no objective sleep measure, which may have affected the results.
SDB is a special type of sleep disorder, mainly manifested as abnormal changes in breathing during sleep. It is characterized by repetitive nocturnal apnea and/or hypopnea, followed by a recovery phase with hyperpnea, which leads to circulation patterns of intermittent hypoxia-reoxygenation, arousal, and sympathetic activation (164). A previous study (32) revealed the correlation between cognitive function scores and oxygen desaturation index in HF patients. However, different conclusions have also been reported, possibly due to the use of a portable SDB monitoring device, which may not fully detect the sleep architecture, and the small sample size (n = 28) and can also affect the correlation results (74).
The mechanism of sleep disorder affecting cognitive function in HF patients may be based on the dysregulation of the hypothalamic-pituitary-adrenal axis (HPA), which is accompanied by high levels of cortisol and pro-inflammatory factors as well as central cholinergic dysfunction leading to neurotoxicity effects on the hippocampus (165). Alperin et al. (166) has also found that the cortical and subcortical volumes of the bilateral hippocampus and the parietal lobule were significantly reduced in sleep-deprived subjects. Furthermore, sleep disorders may reduce the cerebral flow in the left and right frontal lobes, impair memory consolidation and restore brain function during the night, as well as lead to cognitive decline (167, 168). In addition, daytime sleepiness may be more easily affected by pathological changes related to Alzheimer's disease, such as β-protein deposition in the brain (169). SDB may affect cognitive function due to intermittent cerebral hypoxia, changes in cerebral hemodynamics, and oxygen saturation. These conditions may lead to cognition-related brain GM loss, WM neurons, and neuronal damage (170, 171).
However, the present review contains several limitations. Firstly, the qualitative synthesis rather than the quantitative method was used due to the varied risk factors and the inconsistency of cognitive function evaluation methods. Therefore, specific conclusions could not be drawn with this meta-analysis. Secondly, the majority of the included studies were cross-sectional studies. Therefore, they could not reflect the causal relationship. In the future, large prospective studies are required to verify the relationship between these risk factors and CI in patients with HF.
Conclusion
A total of 66 literature studies were included to review the imaging changes of the brain and multiple risk factors in patients with CI after HF. The imaging changes included CBF, medial temporal lobe volume, GM volume and WM volume. The risk factors included EF, BNP/NT-proBNP, BMI index, electrolyte disturbance, combined with AF, DM, anemia, depression, sleep disorders and other diseases. Following comprehensive analysis, the following conclusions were obtained: BNP/NT-proBNP and co-morbidities of HF including AF, DM and anemia were inevitably correlated with CI in patients with HF and certain studies obtained independent correlation results. The severity of MTA, GM volume, BMI index, depression, sleep disorder and low EF value (<30%) were inclined to be associated with CI. The reduction in the volume of CBF may be related to CI, whereas the change of the WM volume was likely to be independent of CI in HF patients. The imaging changes and related risk factors summarized in the present study provide a basis for the early warning and prevention of CI following HF, and the clinical attention and improvement of these risk factors in HF patients may alleviate the cognitive decline. In the future, large prospective studies are required to verify the relationship between these risk factors and CI in patients with HF. And we will find the causes of CI in HF patients through study the cerebral perfusion pressure, synaptic function and reactive oxygen species metabolites.
Data Availability Statement
The original contributions presented in the study are included in the article/Supplementary Material, further inquiries can be directed to the corresponding author/s.
Author Contributions
YJ and LW: theme and design of the research. ZL, SC, and YT: verification of data. TL and YL: statistical analysis. YJ: writing of the manuscript. MZ and YX: critical revision of the manuscript for intellectual content and obtaining funding. All authors have approved the final manuscript for submission.
Funding
This study was supported by the National Natural Science Foundation Project of China (no. 81973787) and Beijing Tongzhou District Science and Technology Project (no. KJ2019CX017).
Conflict of Interest
The authors declare that the research was conducted in the absence of any commercial or financial relationships that could be construed as a potential conflict of interest.
Publisher's Note
All claims expressed in this article are solely those of the authors and do not necessarily represent those of their affiliated organizations, or those of the publisher, the editors and the reviewers. Any product that may be evaluated in this article, or claim that may be made by its manufacturer, is not guaranteed or endorsed by the publisher.
Supplementary Material
The Supplementary Material for this article can be found online at: https://www.frontiersin.org/articles/10.3389/fcvm.2021.838680/full#supplementary-material
Abbreviations
HF, heart failure; CI, cognitive impairment; HFrEF, heart failure with reduced ejection fraction; HFmrEF, heart failure with mid-range ejection fraction; HFpEF, heart failure with preserved ejection fraction; CBF, cerebral blood flow; AF, atrial fibrillation; MTA, medial temporal lobe atrophy; GM, gray matter; WM, white matter; WMH, white matter hyperintensities; EF, ejection fraction; LVEF, left ventricular ejection fraction; BMI, body mass index; BNP, B-type natriuretic peptide; NT-proBNP, N-terminal pro-B-type natriuretic peptide; DM, diabetes mellitus; T2DM, type 2 diabetes mellitus.
References
1. Yohannes A, Chen W, Moga A, Leroi I, Connolly M. Cognitive impairment in chronic obstructive pulmonary disease and chronic heart failure: a systematic review and meta-analysis of observational studies. J Am Med Direct Assoc. (2017) 18:451.e1–11. doi: 10.1016/j.jamda.2017.01.014
2. Taylor J. Cognitive impairment predicts worse outcome in heart failure. Eur Heart J. (2015) 36:1945.
3. Murad K, Goff D, Morgan T, Burke G, Bartz T, Kizer J, et al. Burden of comorbidities and functional and cognitive impairments in elderly patients at the initial diagnosis of heart failure and their impact on total mortality: the cardiovascular health study. JACC Heart Failure. (2015) 3:542–50. doi: 10.1016/j.jchf.2015.03.004
4. Lan H, Hawkins L, Kashner M, Perez E, Firek C, Silvet H. Cognitive impairment predicts mortality in outpatient veterans with heart failure. Heart Lung J Crit Care. (2018) 47:546–52. doi: 10.1016/j.hrtlng.2018.06.008
5. Sokoreli I, Pauws S, Steyerberg E, de Vries G, Riistama J, Tesanovic A, et al. Prognostic value of psychosocial factors for first and recurrent hospitalizations and mortality in heart failure patients: insights from the OPERA-HF study. Eur J Heart Fail. (2018) 20:689–96. doi: 10.1002/ejhf.1112
6. Uchmanowicz I, Jankowska-Polańska B, Mazur G, Sivarajan Froelicher E. Cognitive deficits and self-care behaviors in elderly adults with heart failure. Clin Interv Aging. (2017) 12:1565–72. doi: 10.2147/CIA.S140309
7. Gelow J, Mudd J, Chien C, Lee C. Usefulness of cognitive dysfunction in heart failure to predict cardiovascular risk at 180 days. Am J Cardiol. (2015) 115:778–82. doi: 10.1016/j.amjcard.2014.12.040
8. Huynh Q, Negishi K, De Pasquale C, Hare J, Leung D, Stanton T, et al. Cognitive domains and postdischarge outcomes in hospitalized patients with heart failure. Circul Heart Failure. (2019) 12:e006086. doi: 10.1161/CIRCHEARTFAILURE.119.006086
9. Kim J, Hwang S, Heo S, Shin M, Kim S. Predicted relationships between cognitive function, depressive symptoms, self-care adequacy, and health-related quality of life and major events among patients with heart failure. Eur J Cardiovasc Nurs J Working Group Cardiovasc Nurs Eur Soc Cardiol. (2019) 18:418–26. doi: 10.1177/1474515119840877
10. Nordlund A, Berggren J, Holmström A, Fu M, Wallin A. Frequent mild cognitive deficits in several functional domains in elderly patients with heart failure without known cognitive disorders. J Card Fail. (2015) 21:702–7. doi: 10.1016/j.cardfail.2015.04.006
11. Adelborg K, Horváth-Puhó E, Ording A, Pedersen L, Sørensen H, Henderson V. Heart failure and risk of dementia: a Danish nationwide population-based cohort study. Eur J Heart Fail. (2017) 19:253–60. doi: 10.1002/ejhf.631
12. Cannon J, McMurray J, Quinn T. 'Hearts and minds': association, causation and implication of cognitive impairment in heart failure. Alzheimer's Res Ther. (2015) 7:22. doi: 10.1186/s13195-015-0106-5
13. Alagiakrishnan K, Mah D, Ahmed A, Ezekowitz J. Cognitive decline in heart failure. Heart Fail Rev. (2016) 21:661–73. doi: 10.1007/s10741-016-9568-1
14. Rostom A, Dube C, Cranney A. Summary, evidence report/technology assessment No 104 (Prepared by the University of Ottawa evidence-based Practice center, under contract, No. 290-02-0021), AHRQ publication No 04-E)29-1. (2004).
15. Hu J, Dong Y, Chen X, Liu Y, Ma D, Liu X, et al. Prevalence of suicide attempts among Chinese adolescents: a meta-analysis of cross-sectional studies. Compr Psychiatry. (2015) 61:78–89. doi: 10.1016/j.comppsych.2015.05.001
16. Wells GA, Shea BJ, O'Connell D, Peterson J, Tugwell P. The Newcastle–Ottawa Scale (NOS) for Assessing the Quality of Non-Randomized Studies in Meta-Analysis. (2000).
17. Slim K, Nini E, Forestier D, Kwiatkowski F, Panis Y, Chipponi J. Methodological index for non-randomized studies (minors): development and validation of a new instrument. ANZ J Surg. (2003) 73:712–6. doi: 10.1046/j.1445-2197.2003.02748.x
18. Leeuwis A, Hooghiemstra A, Bron E, Kuipers S, Oudeman E, Kalay T, et al. Cerebral blood flow and cognitive functioning in patients with disorders along the heart-brain axis: cerebral blood flow and the heart-brain axis. Alzheimer's Dement. (2020) 6:e12034. doi: 10.1002/trc2.12034
19. Suzuki H, Matsumoto Y, Ota H, Sugimura K, Takahashi J, Ito K, et al. Hippocampal blood flow abnormality associated with depressive symptoms and cognitive impairment in patients with chronic heart failure. Circul J Off J Jap Circul Soc. (2016) 80:1773–80. doi: 10.1253/circj.CJ-16-0367
20. Kure C, Rosenfeldt F, Scholey A, Pipingas A, Kaye D, Bergin P, et al. Relationships among cognitive function and cerebral blood flow, oxidative stress, and inflammation in older heart failure patients. J Card Fail. (2016) 22:548–59. doi: 10.1016/j.cardfail.2016.03.006
21. Alosco M, Spitznagel M, Sweet L, Josephson R, Hughes J, Gunstad J. Atrial fibrillation exacerbates cognitive dysfunction and cerebral perfusion in heart failure. Pacing Clin Electrophysiol. (2015) 38:178–86. doi: 10.1111/pace.12543
22. Alosco M, Spitznagel M, Cohen R, Raz N, Sweet L, Josephson R, et al. Decreased physical activity predicts cognitive dysfunction and reduced cerebral blood flow in heart failure. J Neurol Sci. (2014) 339:169–75. doi: 10.1016/j.jns.2014.02.008
23. Alosco M, Spitznagel M, Raz N, Cohen R, Sweet L, Garcia S, et al. The interactive effects of cerebral perfusion and depression on cognitive function in older adults with heart failure. Psychosom Med. (2013) 75:632–9. doi: 10.1097/PSY.0b013e31829f91da
24. Alosco M, Spitznagel M, Raz N, Cohen R, Sweet L, Colbert L, et al. Obesity interacts with cerebral hypoperfusion to exacerbate cognitive impairment in older adults with heart failure. Cerebrovasc Dis Extra. (2012) 2:88–98. doi: 10.1159/000343222
25. Jesus P, Vieira-de-Melo R, Reis F, Viana L, Lacerda A, Dias J, et al. Cognitive dysfunction in congestive heart failure: transcranial Doppler evidence of microembolic etiology. Arquivos de neuro-psiquiatria. (2006) 64:207–10. doi: 10.1590/S0004-282X2006000200007
26. Frey A, Sell R, Homola G, Malsch C, Kraft P, Gunreben I, et al. Cognitive deficits and related brain lesions in patients with chronic heart failure. JACC Heart Failure. (2018) 6:583–92. doi: 10.1016/j.jchf.2018.03.010
27. Beer C, Ebenezer E, Fenner S, Lautenschlager N, Arnolda L, Flicker L, et al. Contributors to cognitive impairment in congestive heart failure: a pilot case-control study. Intern Med J. (2009) 39:600–5. doi: 10.1111/j.1445-5994.2008.01790.x
28. Vogels R, Oosterman J, van Harten B, Gouw A, Schroeder-Tanka J, Scheltens P, et al. Neuroimaging and correlates of cognitive function among patients with heart failure. Dement Geriatr Cogn Disord. (2007) 24:418–23. doi: 10.1159/000109811
29. Alosco M, Brickman A, Spitznagel M, Narkhede A, Griffith E, Cohen R, et al. Reduced gray matter volume is associated with poorer instrumental activities of daily living performance in heart failure. J Cardiovasc Nurs. (2016) 31:31–41. doi: 10.1097/JCN.0000000000000218
30. Alosco M, Brickman A, Spitznagel M, Griffith E, Narkhede A, Raz N, et al. Poorer physical fitness is associated with reduced structural brain integrity in heart failure. J Neurol Sci. (2013) 328:51–7. doi: 10.1016/j.jns.2013.02.015
31. Alosco M, Brickman A, Spitznagel M, Garcia S, Narkhede A, Griffith E, et al. Cerebral perfusion is associated with white matter hyperintensities in older adults with heart failure. Congest Heart Failure. (2013) 19:E29–34. doi: 10.1111/chf.12025
32. D'Elia E, Stamerra C, Vecchi A, Duino V, Grosu A, Abete R, et al. Association between sleep disordered breathing, neurocognitive impairment and diastolic function in acute heart failure patients: an insight after the vulnerable phase of the hospitalization. Intern Emerg Med. (2021) 16:369–77. doi: 10.1007/s11739-020-02397-x
33. Faulkner K, Dickson V, Fletcher J, Katz S, Chang P, Gottesman R, et al. Factors associated with cognitive impairment in heart failure with preserved ejection fraction. J Cardiovasc Nurs. (2020). doi: 10.1097/JCN.0000000000000711
34. Hammond C, Blades N, Chaudhry S, Dodson J, Longstreth W, Heckbert S, et al. Long-term cognitive decline after newly diagnosed heart failure: longitudinal analysis in the CHS (Cardiovascular Health Study). Circul Heart Failure. (2018) 11:e004476. doi: 10.1161/CIRCHEARTFAILURE.117.004476
35. Witt L, Rotter J, Stearns S, Gottesman R, Kucharska-Newton A, Richey Sharrett A, et al. Heart failure and cognitive impairment in the atherosclerosis risk in communities (ARIC) study. J Gen Intern Med. (2018) 33:1721–8. doi: 10.1007/s11606-018-4556-x
36. Warraich H, Kitzman D, Whellan D, Duncan P, Mentz R, Pastva A, et al. Physical function, frailty, cognition, depression, and quality of life in hospitalized adults ≥60 years with acute decompensated heart failure with preserved versus reduced ejection fraction. Circul Heart Failure. (2018) 11:e005254. doi: 10.1161/CIRCHEARTFAILURE.118.005254
37. Adebayo P, Akintunde A, Adebayo A, Asaolu S, Audu M, Ayodele O. Comparison of neuropsychological patterns in nigerians with different heart failure phenotypes. Archiv Clin Neuropsychol. (2017) 32:280–8. doi: 10.1093/arclin/acw113
38. Shin M, An M, Kim S, Shim J, Park J, Kim J. Concomitant diastolic dysfunction further interferes with cognitive performance in moderate to severe systolic heart failure. PLoS ONE. (2017) 12:e0184981. doi: 10.1371/journal.pone.0184981
39. Albabtain M, Brenner M, Nicklas J, Hummel S, McCormick M, Pawlowski J, et al. Hyponatremia, cognitive function, and mobility in an outpatient heart failure population. Med Sci Monit Int Med J Exp Clin Res. (2016) 22:4978–85. doi: 10.12659/MSM.898538
40. Graham S, Ye S, Qian M, Sanford A, Di Tullio M, Sacco R, et al. Cognitive function in ambulatory patients with systolic heart failure: insights from the warfarin versus aspirin in reduced cardiac ejection fraction (WARCEF) trial. PLoS ONE. (2014) 9:e113447. doi: 10.1371/journal.pone.0113447
41. Feola M, Garnero S, Vallauri P, Salvatico L, Vado A, Leto L, et al. Relationship between cognitive function, depression/anxiety and functional parameters in patients admitted for congestive heart failure. Open Cardiovasc Med J. (2013) 7:54–60. doi: 10.2174/1874192401307010054
42. Steinberg G, Lossnitzer N, Schellberg D, Mueller-Tasch T, Krueger C, Haass M, et al. Peak oxygen uptake and left ventricular ejection fraction, but not depressive symptoms, are associated with cognitive impairment in patients with chronic heart failure. Int J Gen Med. (2011) 4:879–87. doi: 10.2147/IJGM.S23841
43. Festa J, Jia X, Cheung K, Marchidann A, Schmidt M, Shapiro P, et al. Association of low ejection fraction with impaired verbal memory in older patients with heart failure. Arch Neurol. (2011) 68:1021–6. doi: 10.1001/archneurol.2011.163
44. Sauvé M, Lewis W, Blankenbiller M, Rickabaugh B, Pressler S. Cognitive impairments in chronic heart failure: a case controlled study. J Card Fail. (2009) 15:1–10. doi: 10.1016/j.cardfail.2008.08.007
45. Hoth K, Poppas A, Moser D, Paul R, Cohen R. Cardiac dysfunction and cognition in older adults with heart failure. Cognit Behav Neurol. (2008) 21:65–72. doi: 10.1097/WNN.0b013e3181799dc8
46. Feola M, Mauro F, Rosso G, Peano M, Agostini M, Aspromonte N, et al. Correlation between cognitive impairment and prognostic parameters in patients with congestive heart failure. Arch Med Res. (2007) 38:234–9. doi: 10.1016/j.arcmed.2006.10.004
47. Zuccalà G, Cattel C, Manes-Gravina E, Di Niro M, Cocchi A, Bernabei R. Left ventricular dysfunction: a clue to cognitive impairment in older patients with heart failure. J Neurol Neurosurg Psychiatry. (1997) 63:509–12. doi: 10.1136/jnnp.63.4.509
48. Ely A, Alio C, Bygrave D, Burke M, Walker E. Relationship between psychological distress and cognitive function differs as a function of obesity status in inpatient heart failure. Front Psychol. (2020) 11:162. doi: 10.3389/fpsyg.2020.00162
49. Alosco M, Spitznagel M, Cohen R, Sweet L, Josephson R, Hughes J, et al. Obesity and cognitive dysfunction in heart failure: the role of hypertension, type 2 diabetes, and physical fitness. Eur J Cardiovasc Nurs. (2015) 14:334–41. doi: 10.1177/1474515114535331
50. Alosco M, Spitznagel M, Cohen R, Sweet L, Josephson R, Hughes J, et al. Decreases in body mass index after cardiac rehabilitation predict improved cognitive function in older adults with heart failure. J Am Geriatr Soc. (2014) 62:2215–6. doi: 10.1111/jgs.13123
51. Hawkins M, Gunstad J, Dolansky M, Redle J, Josephson R, Moore S, et al. Greater body mass index is associated with poorer cognitive functioning in male heart failure patients. J Card Fail. (2014) 20:199–206. doi: 10.1016/j.cardfail.2013.12.014
52. Dolansky M, Schaefer J, Hawkins M, Gunstad J, Basuray A, Redle J, et al. The association between cognitive function and objective adherence to dietary sodium guidelines in patients with heart failure. Patient Prefer Adherence. (2016) 10:233–41. doi: 10.2147/PPA.S95528
53. Hwang S, Kim J. An examination of the association of cognitive functioning, adherence to sodium restriction and Na/K ratios in Korean heart failure patients. J Clin Nurs. (2016) 25:1766–76. doi: 10.1111/jocn.13198
54. Alosco M, Spitznagel M, Raz N, Cohen R, Sweet L, Colbert L, et al. Dietary habits moderate the association between heart failure and cognitive impairment. J Nutr Gerontol Geriatr. (2013) 32:106–21. doi: 10.1080/21551197.2013.781408
55. Zuccalà G, Marzetti E, Cesari M, Lo Monaco M, Antonica L, Cocchi A, et al. Correlates of cognitive impairment among patients with heart failure: results of a multicenter survey. Am J Med. (2005) 118:496–502. doi: 10.1016/j.amjmed.2005.01.030
56. Sargent L, Flattery M, Shah K, Price E, Tirado C, Oliveira T, et al. Influence of physiological and psychological factors on cognitive dysfunction in heart failure patients. Appl Nurs Res. (2020) 56:151375. doi: 10.1016/j.apnr.2020.151375
57. Pierobon A, Granata N, Torlaschi V, Vailati C, Radici A, Maestri R, et al. Psychomotor speed as a predictor of functional status in older chronic heart failure (CHF) patients attending cardiac rehabilitation. PLoS ONE. (2020) 15:e0235570. doi: 10.1371/journal.pone.0235570
58. Lee J, Won M, Son Y. Combined influence of depression and physical frailty on cognitive impairment in patients with heart failure. Int J Environ Res Public Health. (2018) 16:10066. doi: 10.3390/ijerph16010066
59. Taraghi Z, Akbari Kamrani A, Foroughan M, Yazdani J, Mahdavi A, Baghernejad S. Cognitive impairment among elderly patients with chronic heart failure and related factors. Iran J Psychiatry Behav Sci. (2016) 10:e4500. doi: 10.17795/ijpbs-4500
60. Hawkins M, Dolansky M, Schaefer J, Fulcher M, Gunstad J, Redle J, et al. Cognitive function in heart failure is associated with nonsomatic symptoms of depression but not somatic symptoms. J Cardiovasc Nurs. (2015) 30:E9–17. doi: 10.1097/JCN.0000000000000178
61. Hjelm C, Broström A, Dahl A, Johansson B, Fredrikson M, Strömberg A. Factors associated with increased risk for dementia in individuals age 80 years or older with congestive heart failure. J Cardiovasc Nurs. (2014) 29:82–90. doi: 10.1097/JCN.0b013e318275543d
62. Hanon O, Vidal J, de Groote P, Galinier M, Isnard R, Logeart D, et al. Prevalence of memory disorders in ambulatory patients aged ≥70 years with chronic heart failure (from the EFICARE study). Am J Cardiol. (2014) 113:1205–10. doi: 10.1016/j.amjcard.2013.12.032
63. Pulignano G, Del Sindaco D, Di Lenarda A, Tinti M, Tarantini L, Cioffi G, et al. Chronic renal dysfunction and anaemia are associated with cognitive impairment in older patients with heart failure. J Cardiovasc Med. (2014) 15:481–90. doi: 10.2459/JCM.0000000000000005
64. Garcia S, Spitznagel M, Cohen R, Raz N, Sweet L, Colbert L, et al. Depression is associated with cognitive dysfunction in older adults with heart failure. Cardiovasc Psychiatry Neurol. (2011) 2011:368324. doi: 10.1155/2011/368324
65. Akomolafe A, Quarshie A, Jackson P, Thomas J, Deffer O, Oduwole A, et al. The prevalence of cognitive impairment among African-American patients with congestive heart failure. J Natl Med Assoc. (2005) 97:689–94.
66. Trojano L, Antonelli Incalzi R, Acanfora D, Picone C, Mecocci P, Rengo F. Cognitive impairment: a key feature of congestive heart failure in the elderly. J Neurol. (2003) 250:1456–63. doi: 10.1007/s00415-003-0249-3
67. Wang W, Lessard D, Saczynski J, Goldberg R, Go A, Paul T, et al. Incident frailty and cognitive impairment by heart failure status in older patients with atrial fibrillation: the SAGE-AF study. J Geriatr Cardiol. (2020) 17:653–8.
68. Yang H, Niu W, Zang X, Lin M, Zhao Y. The association between atrial fibrillation and cognitive function in patients with heart failure. Eur J Cardiovasc Nurs. (2017) 16:104–12. doi: 10.1177/1474515116641299
69. Coma M, González-Moneo M, Enjuanes C, Velázquez P, Espargaró D, Pérez B, et al. Effect of permanent atrial fibrillation on cognitive function in patients with chronic heart failure. Am J Cardiol. (2016) 117:233–9. doi: 10.1016/j.amjcard.2015.10.038
70. Pulignano G, Del Sindaco D, Tinti M, Di Lenarda A, Alunni G, Senni M, et al. Atrial fibrillation, cognitive impairment, frailty and disability in older heart failure patients. J Cardiovasc Med. (2016) 17:616–23. doi: 10.2459/JCM.0000000000000366
71. Basile G, Crucitti A, Cucinotta M, Figliomeni P, Lacquaniti A, Catalano A, et al. Impact of diabetes on cognitive impairment and disability in elderly hospitalized patients with heart failure. Geriatr Gerontol Int. (2013) 13:1035–42. doi: 10.1111/ggi.12051
72. Alosco M, Spitznagel M, van Dulmen M, Raz N, Cohen R, Sweet L, et al. The additive effects of type-2 diabetes on cognitive function in older adults with heart failure. Cardiol Res Pract. (2012) 2012:348054. doi: 10.1155/2012/348054
73. Kim E, Son Y. Association between anemia and cognitive impairment among elderly patients with heart failure. Int J Environ Res Public Health. (2019) 16:162933. doi: 10.3390/ijerph16162933
74. Moon C, Melah K, Johnson S, Bratzke L. Sleep-disordered breathing, brain volume, and cognition in older individuals with heart failure. Brain Behav. (2018) 8:e01029. doi: 10.1002/brb3.1029
75. Walter F, Ede D, Hawkins M, Dolansky M, Gunstad J, Josephson R, et al. Sleep quality and daytime sleepiness are not associated with cognition in heart failure. J Psychosom Res. (2018) 113:100–6. doi: 10.1016/j.jpsychores.2018.08.003
76. Byun E, Kim J, Riegel B. Associations of subjective sleep quality and daytime sleepiness with cognitive impairment in adults and elders with heart failure. Behav Sleep Med. (2017) 15:302–17. doi: 10.1080/15402002.2015.1133418
77. Moon C, Yoon J, Bratzke L. The role of heart failure, daytime sleepiness, and disturbed sleep on cognition. West J Nurs Res. (2017) 39:473–91. doi: 10.1177/0193945916675587
78. Moon C, Phelan C, Lauver D, Bratzke L. Is sleep quality related to cognition in individuals with heart failure? Heart Lung. (2015) 44:212–8. doi: 10.1016/j.hrtlng.2015.02.005
79. Hjelm C, Strömberg A, Arestedt K, Broström A. Association between sleep-disordered breathing, sleep-wake pattern, and cognitive impairment among patients with chronic heart failure. Eur J Heart Fail. (2013) 15:496–504. doi: 10.1093/eurjhf/hft014
80. Riegel B, Moelter S, Ratcliffe S, Pressler S, De Geest S, Potashnik S, et al. Excessive daytime sleepiness is associated with poor medication adherence in adults with heart failure. J Card Fail. (2011) 17:340–8. doi: 10.1016/j.cardfail.2010.11.002
81. Dong Y, Teo S, Kang K, Tan M, Ling L, Yeo P, et al. Cognitive impairment in Asian patients with heart failure: prevalence, biomarkers, clinical correlates, and outcomes. Eur J Heart Fail. (2019) 21:688–90. doi: 10.1002/ejhf.1442
82. Leto L, Testa M, Feola M. Correlation between B-type natriuretic peptide and functional/cognitive parameters in discharged congestive heart failure patients. Int J Endocrinol. (2015) 2015:239136. doi: 10.1155/2015/239136
83. van Vliet P, Sabayan B, Wijsman L, Poortvliet R, Mooijaart S, de Ruijter W, et al. NT-proBNP, blood pressure, and cognitive decline in the oldest old: the Leiden 85-plus Study. Neurology. (2014) 83:1192–9. doi: 10.1212/WNL.0000000000000820
84. Meguro T, Meguro Y, Kunieda T. Atrophy of the parahippocampal gyrus is prominent in heart failure patients without dementia. ESC Heart Failure. (2017) 4:632–40. doi: 10.1002/ehf2.12192
85. Woo M, Ogren J, Abouzeid C, Macey P, Sairafian K, Saharan P, et al. Regional hippocampal damage in heart failure. Eur J Heart Fail. (2015) 17:494–500. doi: 10.1002/ejhf.241
86. Frey A, Homola G, Henneges C, Mühlbauer L, Sell R, Kraft P, et al. Temporal changes in total and hippocampal brain volume and cognitive function in patients with chronic heart failure-the COGNITIONMATTERS-HF cohort study. Eur Heart J. (2021) 42:1569–78. doi: 10.1093/eurheartj/ehab003
87. van der Velpen I, Yancy C, Sorond F, Sabayan B. Impaired cardiac function and cognitive brain aging. Can J Cardiol. (2017) 33:1587–96. doi: 10.1016/j.cjca.2017.07.008
88. Toledo C, Andrade D, Díaz H, Inestrosa N, Del Rio R. Neurocognitive disorders in heart failure: novel pathophysiological mechanisms underpinning memory loss and learning impairment. Mol Neurobiol. (2019) 56:8035–51. doi: 10.1007/s12035-019-01655-0
89. Kumar R, Yadav S, Palomares J, Park B, Joshi S, Ogren J, et al. Reduced regional brain cortical thickness in patients with heart failure. PLoS ONE. (2015) 10:e0126595. doi: 10.1371/journal.pone.0126595
90. Roy B, Woo M, Wang D, Fonarow G, Harper R, Kumar R. Reduced regional cerebral blood flow in patients with heart failure. Eur J Heart Fail. (2017) 19:1294–302. doi: 10.1002/ejhf.874
91. Vogels R, van der Flier W, van Harten B, Gouw A, Scheltens P, Schroeder-Tanka J, et al. Brain magnetic resonance imaging abnormalities in patients with heart failure. Eur J Heart Fail. (2007) 9:1003–9. doi: 10.1016/j.ejheart.2007.07.006
92. Arba F, Quinn T, Hankey G, Ali M, Lees K, Inzitari D. Cerebral small vessel disease, medial temporal lobe atrophy and cognitive status in patients with ischaemic stroke and transient ischaemic attack. Eur J Neurol. (2017) 24:276–82. doi: 10.1111/ene.13191
93. Duara R, Loewenstein D, Potter E, Appel J, Greig M, Urs R, et al. Medial temporal lobe atrophy on MRI scans and the diagnosis of Alzheimer disease. Neurology. (2008) 71:1986–92. doi: 10.1212/01.wnl.0000336925.79704.9f
94. Persson K, Barca M, Eldholm R, Cavallin L, Šaltyte Benth J, Selbæk G, et al. Visual evaluation of medial temporal lobe atrophy as a clinical marker of conversion from mild cognitive impairment to dementia and for predicting progression in patients with mild cognitive impairment and mild Alzheimer's disease. Dement Geriatr Cogn Disord. (2017) 44:12–24. doi: 10.1159/000477342
95. Woo M, Palomares J, Macey P, Fonarow G, Harper R, Kumar R. Global and regional brain mean diffusivity changes in patients with heart failure. J Neurosci Res. (2015) 93:678–85. doi: 10.1002/jnr.23525
96. Bhattacharya P, Bao F, Shah M, Ramesh G, Madhavan R, Khan O. Left ventricular dysfunction is associated with cerebral grey matter injury: an in-vivo brain MRI segmentation study. J Neurol Sci. (2012) 321:111–3. doi: 10.1016/j.jns.2012.07.051
97. Shimizu A, Sakurai T, Mitsui T, Miyagi M, Nomoto K, Kokubo M, et al. Left ventricular diastolic dysfunction is associated with cerebral white matter lesions (leukoaraiosis) in elderly patients without ischemic heart disease and stroke. Geriatr Gerontol Int. (2014) 2014:71–6. doi: 10.1111/ggi.12261
98. Almeida O, Garrido G, Etherton-Beer C, Lautenschlager N, Arnolda L, Alfonso H, et al. Brain and mood changes over 2 years in healthy controls and adults with heart failure and ischaemic heart disease. Eur J Heart Fail. (2013) 15:850–8. doi: 10.1093/eurjhf/hft029
99. Stegmann T, Chu M, Witte V, Villringer A, Kumral D, Riedel-Heller S, et al. Heart failure is independently associated with white matter lesions: insights from the population-based LIFE-Adult Study. ESC Heart Failure. (2021) 8:697–704. doi: 10.1002/ehf2.13166
100. Sobue Y, Watanabe E, Lip G, Koshikawa M, Ichikawa T, Kawai M, et al. Thromboembolisms in atrial fibrillation and heart failure patients with a preserved ejection fraction (HFpEF) compared to those with a reduced ejection fraction (HFrEF). Heart Vessels. (2018) 33:403–12. doi: 10.1007/s00380-017-1073-5
101. Park J, Park C, Mebazaa A, Oh I, Park H, Cho H, et al. Characteristics and outcomes of HFpEF with declining ejection fraction. Clin Res Cardiol. (2020) 109:225–34. doi: 10.1007/s00392-019-01505-y
102. Abebe T, Gebreyohannes E, Tefera Y, Abegaz T. Patients with HFpEF and HFrEF have different clinical characteristics but similar prognosis: a retrospective cohort study. BMC Cardiovasc Disord. (2016) 16:232. doi: 10.1186/s12872-016-0418-9
103. Hoshida S, Watanabe T, Shinoda Y, Ikeoka K, Minamisaka T, Fukuoka H, et al. Sex-related differences in left ventricular diastolic function and arterial elastance during admission in patients with heart failure with preserved ejection fraction: The PURSUIT HFpEF study. Clin Cardiol. (2018) 41:1529–36. doi: 10.1002/clc.23073
104. Su M, Lin L, Tseng Y, Chang C, Wu C, Lin J, et al. CMR-verified diffuse myocardial fibrosis is associated with diastolic dysfunction in HFpEF. JACC Cardiovasc Imag. (2014) 7:991–7. doi: 10.1016/j.jcmg.2014.04.022
105. Mueller K, Thiel F, Beutner F, Teren A, Frisch S, Ballarini T, et al. Brain damage with heart failure: cardiac biomarker alterations and gray matter decline. Circ Res. (2020) 126:750–64. doi: 10.1161/CIRCRESAHA.119.315813
106. Ozyuncu N, Gulec S, Kaya C, Goksuluk H, Tan T, Vurgun V, et al. Relation of acute decompensated heart failure to silent cerebral infarcts in patients with reduced left ventricular ejection fraction. Am J Cardiol. (2019) 123:1835–9. doi: 10.1016/j.amjcard.2019.02.055
107. Kresge H, Liu D, Gupta D, Moore E, Osborn K, Acosta L, et al. Lower left ventricular ejection fraction relates to cerebrospinal fluid biomarker evidence of neurodegeneration in older adults. J Alzheimer's Dis. (2020) 74:965–74. doi: 10.3233/JAD-190813
108. Alosco M, Brickman A, Spitznagel M, Narkhede A, Griffith E, Raz N, et al. Higher BMI is associated with reduced brain volume in heart failure. BMC obesity. (2014) 1:4. doi: 10.1186/2052-9538-1-4
109. Alkan I, Altunkaynak B, Gültekin G, Bayçu C. Hippocampal neural cell loss in high-fat diet-induced obese rats-exploring the protein networks, ultrastructure, biochemical and bioinformatical markers. J Chem Neuroanat. (2021) 114:101947. doi: 10.1016/j.jchemneu.2021.101947
110. Alosco M, Spitznagel M, Gunstad J. Obesity as a risk factor for poor neurocognitive outcomes in older adults with heart failure. Heart Fail Rev. (2014) 19:403–11. doi: 10.1007/s10741-013-9399-2
111. O'Donnell M, Mente A, Rangarajan S, McQueen M, Wang X, Liu L, et al. Urinary sodium and potassium excretion, mortality, and cardiovascular events. N Engl J Med. (2014) 371:612–23. doi: 10.1056/NEJMoa1311889
112. Fujisawa H, Sugimura Y, Takagi H, Mizoguchi H, Takeuchi H, Izumida H, et al. Chronic hyponatremia causes neurologic and psychologic impairments. J Am Soc Nephrol. (2016) 27:766–80. doi: 10.1681/ASN.2014121196
113. Arora S. Hypernatremic disorders in the intensive care unit. J Intensive Care Med. (2013) 28:37–45. doi: 10.1177/0885066611403994
114. Liu Y, Chen J, Li Z, Zhao T, Ni M, Li D, et al. High-salt diet enhances hippocampal oxidative stress and cognitive impairment in mice. Neurobiol Learn Mem. (2014) 114:10–5. doi: 10.1016/j.nlm.2014.04.010
115. Cisternas P, Lindsay C, Salazar P, Silva-Alvarez C, Retamales R, Serrano F, et al. The increased potassium intake improves cognitive performance and attenuates histopathological markers in a model of Alzheimer's disease. Biochim Biophys Acta. (2015) 1852:2630–44. doi: 10.1016/j.bbadis.2015.09.009
116. Alawieh H, Chemaly T, Alam S, Khraiche M. Towards point-of-care heart failure diagnostic platforms: BNP and NT-proBNP biosensors. Sensors. (2019) 19:225003. doi: 10.3390/s19225003
117. Okamoto R, Ali Y, Hashizume R, Suzuki N, Ito M. BNP as a major player in the heart-kidney connection. Int J Mol Sci. (2019) 20:143581. doi: 10.3390/ijms20143581
118. Linssen G, Jaarsma T, Hillege H, Voors A, van Veldhuisen D. A comparison of the prognostic value of BNP versus NT-proBNP after hospitalisation for heart failure. Netherlands Heart J. (2018) 26:486–92. doi: 10.1007/s12471-018-1145-x
119. Begic E, Hadzidedic S, Kulaglic A, Ramic-Brkic B, Begic Z, Causevic M. SOMAscan-based proteomic measurements of plasma brain natriuretic peptide are decreased in mild cognitive impairment and in Alzheimer's dementia patients. PLoS ONE. (2019) 14:e0212261. doi: 10.1371/journal.pone.0212261
120. Daniels L, Maisel A. Natriuretic peptides. J Am Coll Cardiol. (2007) 50:2357–68. doi: 10.1016/j.jacc.2007.09.021
121. Ferguson I, Elbejjani M, Sabayan B, Jacobs D, Meirelles O, Sanchez O, et al. N-terminal pro-brain natriuretic peptide and associations with brain magnetic resonance imaging (MRI) features in middle age: the CARDIA brain MRI study. Front Neurol. (2018) 9:307. doi: 10.3389/fneur.2018.00307
122. Yeh H, Shao J. Depression in community-dwelling elderly patients with heart failure. Arch Psychiatr Nurs. (2018) 32:248–55. doi: 10.1016/j.apnu.2017.11.010
123. Piepenburg S, Ertl G, Angermann C. Depression and heart failure - a dangerous combination. Deutsche medizinische Wochenschrift. (2016) 141:1222–7. doi: 10.1055/s-0042-108681
124. Newhouse A, Jiang W. Heart failure and depression. Heart Fail Clin. (2014) 10:295–304. doi: 10.1016/j.hfc.2013.10.004
125. Tang C, Zhang D, Yang J, Liu Q, Wang Y, Wang W. Overexpression of microRNA-301b accelerates hippocampal microglia activation and cognitive impairment in mice with depressive-like behavior through the NF-κB signaling pathway. Cell Death Dis. (2019) 10:316. doi: 10.1038/s41419-019-1522-4
126. Jin K, Lu J, Yu Z, Shen Z, Li H, Mou T, et al. Linking peripheral IL-6, IL-1β and hypocretin-1 with cognitive impairment from major depression. J Affect Disord. (2020) 277:204–11. doi: 10.1016/j.jad.2020.08.024
127. Liu Y, Zhang T, Meng D, Sun L, Yang G, He Y, et al. Involvement of CX3CL1/CX3CR1 in depression and cognitive impairment induced by chronic unpredictable stress and relevant underlying mechanism. Behav Brain Res. (2020) 381:112371. doi: 10.1016/j.bbr.2019.112371
128. Kim S, Woo S, Kang H, Lim S, Choi S, Myung W, et al. Factors related to prevalence, persistence, and incidence of depressive symptoms in mild cognitive impairment: vascular depression construct. Int J Geriatr Psychiatry. (2016) 31:818–26. doi: 10.1002/gps.4400
129. Donix M, Haussmann R, Helling F, Zweiniger A, Lange J, Werner A, et al. Cognitive impairment and medial temporal lobe structure in young adults with a depressive episode. J Affect Disord. (2018) 237:112–7. doi: 10.1016/j.jad.2018.05.015
130. Albert K, Potter G, Boyd B, Kang H, Taylor W. Brain network functional connectivity and cognitive performance in major depressive disorder. J Psychiatr Res. (2019) 110:51–6. doi: 10.1016/j.jpsychires.2018.11.020
131. Yatawara C, Lim L, Chander R, Zhou J, Kandiah N. Depressive symptoms influence global cognitive impairment indirectly by reducing memory and executive function in patients with mild cognitive impairment. J Neurol Neurosurg Psychiatry. (2016) 87:1375–83. doi: 10.1136/jnnp-2016-314191
132. Ragbaoui Y, Chehbouni C, Hammiri A, Habbal R. Epidemiology of the relationship between atrial fibrillation and heart failure. Pan Afr Med J. (2017) 26:116. doi: 10.11604/pamj.2017.26.116.11470
133. Saglietto A, Scarsoglio S, Ridolfi L, Gaita F, Anselmino M. Higher ventricular rate during atrial fibrillation relates to increased cerebral hypoperfusions and hypertensive events. Sci Rep. (2019) 9:3779. doi: 10.1038/s41598-019-40445-5
134. Anselmino M, Scarsoglio S, Saglietto A, Gaita F, Ridolfi L. Transient cerebral hypoperfusion and hypertensive events during atrial fibrillation: a plausible mechanism for cognitive impairment. Sci Rep. (2016) 6:28635. doi: 10.1038/srep28635
135. Scarsoglio S, Saglietto A, Anselmino M, Gaita F, Ridolfi L. In silico alteration of cerebrovascular haemodynamic patterns due to atrial fibrillation: an investigation. J Royal Soc Interface. (2017) 14:180. doi: 10.1098/rsif.2017.0180
136. Wang Z, van Veluw S, Wong A, Liu W, Shi L, Yang J, et al. Risk factors and cognitive relevance of cortical cerebral microinfarcts in patients with ischemic stroke or transient ischemic attack. Stroke. (2016) 47:2450–5. doi: 10.1161/STROKEAHA.115.012278
137. Galenko O, Jacobs V, Knight S, Bride D, Cutler M, Muhlestein J, et al. Circulating levels of biomarkers of cerebral injury in patients with atrial fibrillation. Am J Cardiol. (2019) 124:1697–700. doi: 10.1016/j.amjcard.2019.08.027
138. Sugioka K, Takagi M, Sakamoto S, Fujita S, Ito A, Iwata S, et al. Predictors of silent brain infarction on magnetic resonance imaging in patients with nonvalvular atrial fibrillation: a transesophageal echocardiographic study. Am Heart J. (2015) 169:783–90. doi: 10.1016/j.ahj.2015.03.016
139. Junejo R, Braz I, Lucas S, van Lieshout J, Phillips A, Lip G, et al. Neurovascular coupling and cerebral autoregulation in atrial fibrillation. J Cerebr Blood Flow Metabol. (2020) 40:1647–57. doi: 10.1177/0271678X19870770
140. Silva D, Avelar W, de Campos B, Lino A, Balthazar M, Figueiredo M, et al. Default mode network disruption in stroke-free patients with atrial fibrillation. Cerebrovasc Dis. (2018) 45:78–84. doi: 10.1159/000486689
141. Dunlay S, Givertz M, Aguilar D, Allen L, Chan M, Desai A, et al. Type 2 diabetes mellitus and heart failure: a scientific statement from the American Heart Association and the Heart Failure Society of America: this statement does not represent an update of the 2017 ACC/AHA/HFSA heart failure guideline update. Circulation. (2019) 140:e294–324. doi: 10.1161/CIR.0000000000000691
142. Yap J, Tay W, Teng T, Anand I, Richards A, Ling L, et al. Association of diabetes mellitus on cardiac remodeling, quality of life, and clinical outcomes in heart failure with reduced and preserved ejection fraction. J Am Heart Assoc. (2019) 8:e013114. doi: 10.1161/JAHA.119.013114
143. Zareini B, Rørth R, Holt A, Mogensen U, Selmer C, Gislason G, et al. Heart failure and the prognostic impact and incidence of new-onset of diabetes mellitus: a nationwide cohort study. Cardiovasc Diabetol. (2019) 18:79. doi: 10.1186/s12933-019-0883-4
144. Sun C, Xiao Y, Li J, Ge B, Chen X, Liu H, et al. Nonenzymatic function of DPP4 in diabetes-associated mitochondrial dysfunction and cognitive impairment. Alzheimer's Dement. (2021) 17:29–40. doi: 10.1002/alz.12437
145. Yun J, Lee D, Jeong H, Kim H, Ye S, Cho C. STAT3 activation in microglia exacerbates hippocampal neuronal apoptosis in diabetic brains. J Cell Physiol. (2021) 236:7058–70. doi: 10.1002/jcp.30373
146. Cui Y, Tang T, Lu C, Cai Y, Lu T, Wang Y, et al. Abnormal cingulum bundle induced by type 2 diabetes mellitus: a diffusion tensor tractography study. Front Aging Neurosci. (2020) 12:594198. doi: 10.3389/fnagi.2020.594198
147. Shao J, Wang J, He Q, Yang Y, Zou Y, Su W, et al. Three-dimensional-arterial spin labeling perfusion correlation with diabetes-associated cognitive dysfunction and vascular endothelial growth factor in type 2 diabetes mellitus rat. World J Diabetes. (2021) 12:499–513. doi: 10.4239/wjd.v12.i4.499
148. Wang Y, Sun L, He G, Gang X, Zhao X, Wang G, et al. Cerebral perfusion alterations in type 2 diabetes mellitus - a systematic review. Front Neuroendocrinol. (2021) 62:100916. doi: 10.1016/j.yfrne.2021.100916
149. Chirinos J, Bhattacharya P, Kumar A, Proto E, Konda P, Segers P, et al. Impact of diabetes mellitus on ventricular structure, arterial stiffness, and pulsatile hemodynamics in heart failure with preserved ejection fraction. J Am Heart Assoc. (2019) 8:e011457. doi: 10.1161/JAHA.118.011457
150. Piatkowska-Chmiel I, Herbet M, Gawronska-Grzywacz M, Ostrowska-Lesko M, Dudka J. The role of molecular and inflammatory indicators in the assessment of cognitive dysfunction in a mouse model of diabetes. Int J Mol Sci. (2021) 22:83878. doi: 10.3390/ijms22083878
151. Liu Y, Zhang H, Wang S, Guo Y, Fang X, Zheng B, et al. Reduced pericyte and tight junction coverage in old diabetic rats are associated with hyperglycemia-induced cerebrovascular pericyte dysfunction. Am J Physiol Heart Circul Physiol. (2021) 320:H549–62. doi: 10.1152/ajpheart.00726.2020
152. Dou Y, Tan Y, Yu T, Ma X, Zhou Y, Zhao Y, et al. MiR-132 down-regulates high glucose-induced β-dystroglycan degradation through Matrix Metalloproteinases-9 up-regulation in primary neurons. J Cell Mol Med. (2021). doi: 10.1111/jcmm.16669
153. Goh V, Tromp J, Teng T, Tay W, Van Der Meer P, Ling L, et al. Prevalence, clinical correlates, and outcomes of anaemia in multi-ethnic Asian patients with heart failure with reduced ejection fraction. ESC Heart Failure. (2018) 5:570–8. doi: 10.1002/ehf2.12279
154. Berry C, Poppe K, Gamble G, Earle N, Ezekowitz J, Squire I, et al. Prognostic significance of anaemia in patients with heart failure with preserved and reduced ejection fraction: results from the MAGGIC individual patient data meta-analysis. QJM. (2016) 109:377–82. doi: 10.1093/qjmed/hcv087
155. Pisaniello A, Wong D, Kajani I, Robinson K, Shakib S. Anaemia in chronic heart failure: more awareness is required. Intern Med J. (2013) 43:999–1004. doi: 10.1111/imj.12195
156. Hare G. Anaemia and the brain. Curr Opin Anaesthesiol. (2004) 17:363–9. doi: 10.1097/00001503-200410000-00003
157. McClorry S, Zavaleta N, Llanos A, Casapía M, Lönnerdal B, Slupsky C. Anemia in infancy is associated with alterations in systemic metabolism and microbial structure and function in a sex-specific manner: an observational study. Am J Clin Nutr. (2018) 108:1238–48. doi: 10.1093/ajcn/nqy249
158. Gottesman R, Sojkova J, Beason-Held L, An Y, Longo D, Ferrucci L, et al. Patterns of regional cerebral blood flow associated with low hemoglobin in the Baltimore Longitudinal Study of Aging. J Gerontol Ser A Biol Sci Med Sci. (2012) 67:963–9. doi: 10.1093/gerona/gls121
159. Beydoun M, Hossain S, MacIver P, Srinivasan D, Beydoun H, Maldonado A, et al. Red cell distribution width, anemia, and brain volumetric outcomes among middle-aged adults. J Alzheimer's Dis. (2021) 81:711–27. doi: 10.3233/JAD-201386
160. Yoshinaga T, Nishimata H, Kajiya Y, Yokoyama S. Combined assessment of serum folate and hemoglobin as biomarkers of brain amyloid β accumulation. PLoS ONE. (2017) 12:e0175854. doi: 10.1371/journal.pone.0175854
161. Park S, Kim H, Lee J, Lee N, Hwang J, Yang J, et al. Decreased hemoglobin levels, cerebral small-vessel disease, and cortical atrophy: among cognitively normal elderly women and men. Int Psychogeriatr. (2016) 28:147–56. doi: 10.1017/S1041610215000733
162. Estrada J, Contreras I, Pliego-Rivero F, Otero G. Molecular mechanisms of cognitive impairment in iron deficiency: alterations in brain-derived neurotrophic factor and insulin-like growth factor expression and function in the central nervous system. Nutr Neurosci. (2014) 17:193–206. doi: 10.1179/1476830513Y.0000000084
163. Smith A, Refsum H. Homocysteine, B vitamins, and cognitive impairment. Annu Rev Nutr. (2016) 36:211–39. doi: 10.1146/annurev-nutr-071715-050947
164. Trupp R, Corwin E. Sleep-disordered breathing, cognitive functioning, and adherence in heart failure: linked through pathology? Prog Cardiovasc Nurs. (2008) 23:32–6. doi: 10.1111/j.1751-7117.2008.08000.x
165. Johar H, Kawan R, Emeny R, Ladwig K. Impaired sleep predicts cognitive decline in old people: findings from the prospective KORA age study. Sleep. (2016) 39:217–26. doi: 10.5665/sleep.5352
166. Alperin N, Wiltshire J, Lee S, Ramos A, Hernandez-Cardenache R, Rundek T, et al. Effect of sleep quality on amnestic mild cognitive impairment vulnerable brain regions in cognitively normal elderly individuals. Sleep. (2019) 42:zsy254. doi: 10.1093/sleep/zsy254
167. Miyata S, Noda A, Ozaki N, Hara Y, Minoshima M, Iwamoto K, et al. Insufficient sleep impairs driving performance and cognitive function. Neurosci Lett. (2010) 469:229–33. doi: 10.1016/j.neulet.2009.12.001
168. Sterniczuk R, Theou O, Rusak B, Rockwood K. Sleep disturbance is associated with incident dementia and mortality. Curr Alzheimer Res. (2013) 10:767–75. doi: 10.2174/15672050113109990134
169. Carvalho D, St Louis E, Knopman D, Boeve B, Lowe V, Roberts R, et al. Association of excessive daytime sleepiness with longitudinal β-amyloid accumulation in elderly persons without dementia. J Am Med Assoc Neurol. (2018) 75:672–80. doi: 10.1001/jamaneurol.2018.0049
170. Sivathamboo S, Perucca P, Velakoulis D, Jones N, Goldin J, Kwan P, et al. Sleep-disordered breathing in epilepsy: epidemiology, mechanisms, and treatment. Sleep. (2018) 41:zsy015. doi: 10.1093/sleep/zsy015
Keywords: heart failure, cognitive impairment, brain imaging, risk factors, systematic review
Citation: Jiang Y, Wang L, Lu Z, Chen S, Teng Y, Li T, Li Y, Xie Y and Zhao M (2022) Brain Imaging Changes and Related Risk Factors of Cognitive Impairment in Patients With Heart Failure. Front. Cardiovasc. Med. 8:838680. doi: 10.3389/fcvm.2021.838680
Received: 18 December 2021; Accepted: 31 December 2021;
Published: 26 January 2022.
Edited by:
Nicola Mumoli, ASST Ovest Milanese, ItalyReviewed by:
Eiichi Watanabe, Fujita Health University, JapanFrancisco Javier Carrasco-Sánchez, Hospital Juan Ramón Jiménez, Spain
Copyright © 2022 Jiang, Wang, Lu, Chen, Teng, Li, Li, Xie and Zhao. This is an open-access article distributed under the terms of the Creative Commons Attribution License (CC BY). The use, distribution or reproduction in other forums is permitted, provided the original author(s) and the copyright owner(s) are credited and that the original publication in this journal is cited, in accordance with accepted academic practice. No use, distribution or reproduction is permitted which does not comply with these terms.
*Correspondence: Mingjing Zhao, bWpneDIwMDRAMTYzLmNvbQ==; Yingzhen Xie, MzE5MUBhbGl5dW4uY29t