- 1Department of Clinical Laboratory, Affiliated Yantai Yuhuangding Hospital of Qingdao University, Yantai, China
- 2Department of Cardiology, Affiliated Yantai Yuhuangding Hospital of Qingdao University, Yantai, China
- 3Medical Information Center, Peking University People’s Hospital, Beijing, China
- 4Department of Cardiology, Peking University People’s Hospital, Beijing, China
- 5Biochip Laboratory, Affiliated Yantai Yuhuangding Hospital of Qingdao University, Yantai, China
- 6Gennlife (Beijing) Technology Co., Ltd., Beijing, China
This study aimed to examine the performance of the dual antiplatelet therapy (DAPT) score in two retrospective cohorts of post-percutaneous coronary intervention (PCI) patients and to explore whether incorporating additional biomarkers could further improve the predictive power of the DAPT score. In a retrospective derivation cohort of 4,798 PCI patients, the validity of DAPT score for stratifying ischemic/bleeding risks was explored. Then, the association between the baseline status of 54 laboratory test biomarkers and ischemic/bleeding events was revealed while adjusting for the DAPT score. Combinations of individual laboratory test biomarkers that were significantly associated with ischemic/bleeding events were explored to identify the ones that improved discrimination of ischemic and bleeding events when incorporated into DAPT score. Finally, the impact of the combination of biomarkers with DAPT score was validated in an independent retrospective validation cohort of 1,916 PCI patients. Patients with a high DAPT score (DAPT score ≥ 2) had significantly higher risk of ischemic events and significantly lower risk of bleeding than patients with a low DAPT score (DAPT score < 2). Moreover, the addition of aspartate aminotransferase (AST) and red cell distribution width CV (RDW-CV) into the DAPT score further improved discrimination of ischemia and bleeding. Furthermore, the incremental predictive value of AST + RDW-CV maintained with measurements was updated at post-baseline time points. DAPT score successfully stratified the risks of ischemia/bleeding post PCI in the current cohorts. Incorporation of AST + RDW-CV into the DAPT score further improved prediction for both ischemic and bleeding events.
Introduction
Percutaneous coronary intervention (PCI) is a non-surgical treatment procedure used for improving myocardial perfusion of the heart in patients with coronary artery disease (CAD) (1, 2). Commonly, the dual antiplatelet therapy (DAPT) is used to prevent further thrombotic complications in post-PCI patients (3, 4). However, the optimal duration of the clinical use of DAPT remains controversial as prolonged DAPT treatment is closely associated with an increased risk of bleeding (5). Thus, personalization of DAPT duration based on the prediction of a patient’s ischemic and bleeding risks is necessary (6).
DAPT score is a system that is used to assess ischemic and bleeding risks simultaneously based on an individual patient’s clinical characteristics (7). Higher score quartiles are associated with higher rates of myocardial infarction (MI) or stent thrombosis (ST) and lower rates of moderate or severe bleeding (8). It has been shown that patients with high DAPT scores (DAPT score ≥ 2) benefit from prolonged DAPT due to reduced ischemic risks and an insignificant increase in bleeding risks, while patients with low DAPT scores (DAPT score < 2) suffer from prolonged DAPT due to increased bleeding risks, without a significant reduction in MI or ST risks (9, 10). However, DAPT has not been extensively validated using more complex real-world data, since it has been developed based on clinical trial data (11). Fortunately, soluble biomarkers such as Roche Troponin T and Siemens Troponin I Ultra have revolutionized the management of acute coronary syndromes (12). These biomarkers may play a role in guiding the choice of antiplatelet agent and the optimal DAPT duration (13). A previous study has shown that platelet function testing can be used as a biomarker for the efficacy of antiplatelet drugs in DAPT (14). Although biomarkers may be useful to identify patients who are at high risk for such events, the evaluation between routinely assayed biomarkers and ischemic/bleeding risks is seriously inadequate.
In this study, based on a retrospective derivation cohort, the DAPT score was validated for stratifying ischemic and bleeding risks. Then, the association between the baseline status of 54 laboratory test biomarkers and ischemic/bleeding events was investigated while adjusting for the DAPT score. Combinations of individual laboratory test biomarkers that significantly associated with ischemic or bleeding events were explored to identify the ones that improved discrimination of ischemic and bleeding events when incorporated into the DAPT score. Finally, the prediction results were verified in an independent retrospective validation cohort. We examined the performance of DAPT score in two retrospective “real-world” all-comers cohorts, and explored whether incorporating additional biomarkers could further improve its predictive power.
Materials and Methods
Patients
Between June 2008 and July 2017, consecutive post-PCI patients selected from the institute’s electronic medical records (EMR) system at Yantai Yuhuangding Hospital were enrolled in the derivation cohort. Meanwhile, between October 2008 and December 2018, consecutive post-PCI patients selected from the institute’s EMR system at the Peking University People’s Hospital were enrolled in the validation cohort. Based on 2011 ACCF/AHA/SCAI guideline for PCI, all patients were recommend to receive 12 months of DAPT after surgery (15). Therefore, all enrolled PCI patients were treated uniformly for 12 months with DAPT. Patients were excluded by the following criteria: (1) missing procedure information or no stenting; (2) missing any of the DAPT score variables, including LVEF, smoking status, stent type, prior PCI, or MI information. All patients provided written informed consent for their clinical information to be used in retrospective studies. The study protocol was approved by the Ethics Committee of the Yantai Yuhuangding Hospital (Yantai, China).
Ischemic and Bleeding Endpoints Definition
Based on the diagnosis records in the EMR system, the primary ischemic endpoint was defined as a composite of MI or ST-segment. Meanwhile, the primary bleeding endpoint was defined as the hemoglobin levels that were less than the lower limit of laboratory test records in the EMR system.
Sustained Predictive Value of Candidate Biomarkers in Post-baseline Measurements
Unlike most DAPT score variables that are likely invariable, many of the laboratory test biomarkers may reflect the temporary condition of patients and are susceptible to changes after baseline (16). Thus, the predictive value of genuine predictors for ischemic and bleeding events should remain when measurements are updated at post-baseline time points. Thus, based on the biomarkers obtained above, the last measurements of candidate biomarkers were collected before a series of time points after baseline (every 6 months until 108 months post PCI), followed by the biomarker status update according to patients not yet experiencing an event or not censored. The added predictive values of candidate biomarkers were examined at each time point in the overall cohort.
Statistical Analysis
Wilcoxon rank-sum test for continuous variables and Fisher exact test for categorical variables were used for the baseline characteristics comparison between the derivation cohort and the validation cohort, respectively. Cumulative incidences of ischemic and bleeding events were estimated by the Kaplan-Meier (KM) method, followed by the log-rank test. Continuous measurement of each laboratory test biomarker was transformed into categorical score based on the upper limit of the reference range (ULN) and lower limit of the reference range (LLN). Specifically, the measurement score > ULN, ≤ ULN and ≥ LLN, and < LLN was represented as 1, 0, and –1, respectively (Supplementary Table 1). Using the adjusted DAPT score as a continuous variable, the association between an individual laboratory test biomarker and ischemic/bleeding event was examined independently by Cox proportional hazards regression. Biomarkers that showed significant association with either event were selected for further analysis, and the biomarkers that were associated with both ischemic and bleeding events in the same direction [both hazard ratio (HR) > 1 or < 1] were not considered for incorporation into the DAPT score. Then, all single candidate biomarkers, as well as possible combinations between one ischemia- and one bleeding-associated biomarker were exhaustively tested for incorporation into the DAPT score. C statistic of the DAPT score (17) was used to determine whether there was an improvement in the current new model. The C index values, confidence interval (CI) values, as well as the significance levels of these two C index values for the DAPT and DAPT + labtest models were calculated using compareC package in R (18), respectively. The net reclassification improvement (NRI) and integrated discrimination improvement (IDI) were calculated to assess whether added biomarkers improved the performance of DAPT score (19). The survIDINRI in R (20) was used to calculate the value of IDI and NRI at the 30th month as time point (bootstrap: 1,000 times). To correct for multiple hypothesis testing, the Benjamini-Hochberg method was applied to revise P-values resulted from candidate biomarker selection and combination screening. P < 0.05 was considered as statistically significant. All data were analyzed using R (version: 3.5.1) software.
Results
Baseline Characteristics
A total of 6,714 post-PCI patients (4,798 in the derivation cohort and 1,916 in the validation cohort) with complete data regarding DAPT score variables were included in the current study (Figure 1). The baseline information showed that the mean age of the overall cohort was 62.7 ± 10.8 years old. Among cardiovascular risk factors, hypertension was the most prevalent (63.4%), followed by smoking (32.8%) and diabetes (32.6%). The presence of a history of cardiovascular disease (prior MI 0.6%, prior stroke 7.3%) or cardiovascular intervention (prior PCI 1.5%, prior coronary artery bypass grafting 0.1%) was relatively rare. Patients predominantly received drug-eluting dents (99.9%) and clopidogrel as the P2Y12 inhibitor at discharge (98.3%) (Table 1). There were 881 ischemic events (18.4%) and 520 bleeding events (10.8%) during median 22.1 (10.3–37.7) months follow-up period among the 4,798 patients in derivation cohort. In validation cohort, there were 433 ischemic events (22.6%) and 251 bleeding events (13.1%) during median 30.9 (14.2–50.1) months follow-up period among the 1,916 patients.
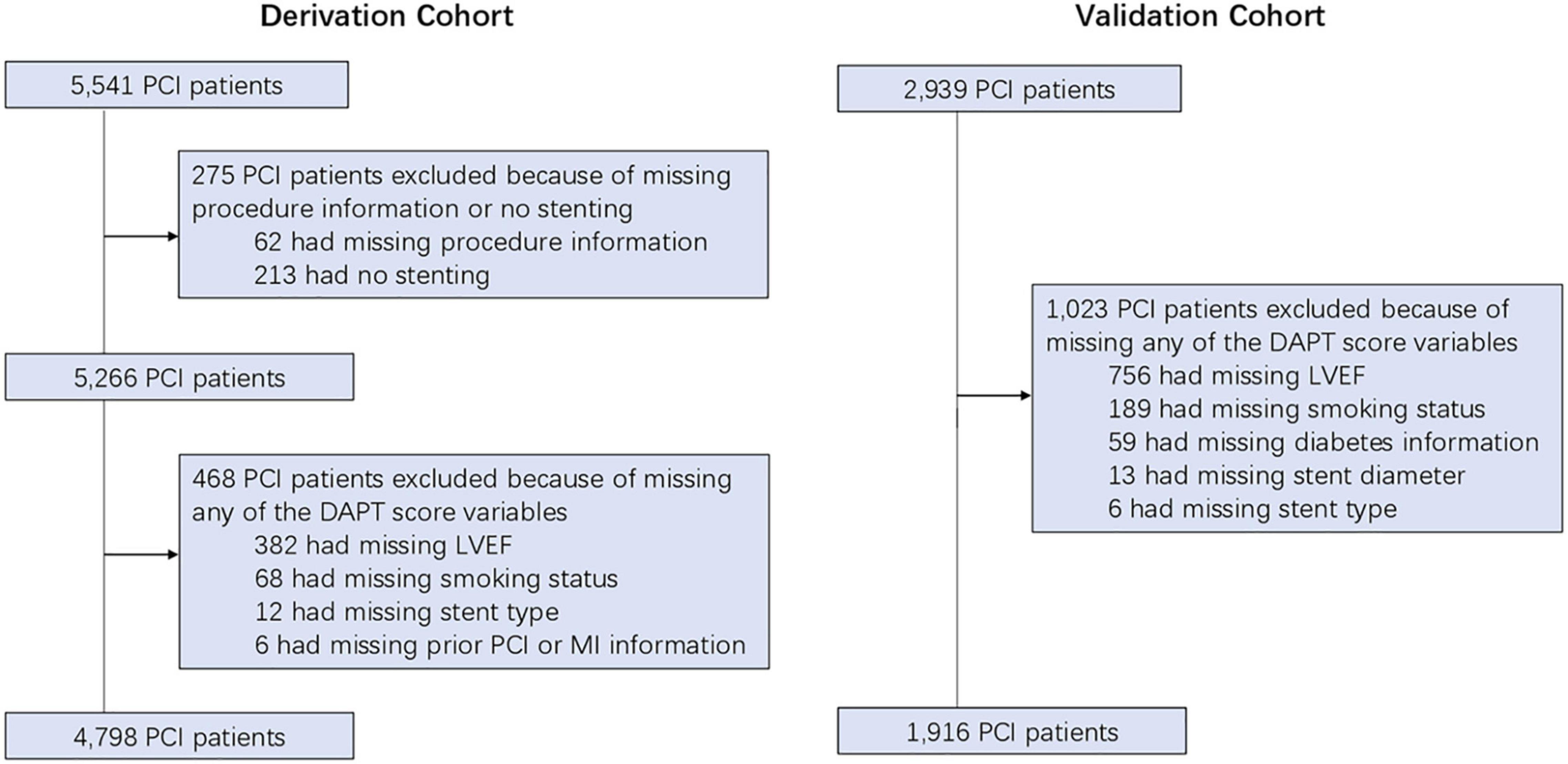
Figure 1. The study flow-chart. PCI, percutaneous coronary intervention; DAPT, dual antiplatelet therapy; LVEF, left ventricular ejection fraction; MI, myocardial infarction.
Ischemic/Bleeding Risks Stratification
The DAPT score for the derivation cohort (-2 to 5) and the validation cohort (-2 to 6) was calculated. The results showed that the median of DAPT score was 1 in both cohorts (Figure 2). In addition, the distribution among the high DAPT group (DAPT score ≥ 2) and the low DAPT group (DAPT score < 2) were similar in both cohorts (P = 0.54). A significantly higher risk of ischemic events (derivation cohort: HR = 1.60; 95% CI:1.40–1.83; P < 0.001; validation cohort: HR = 2.45; 95% CI:2.05–2.92; P < 0.001) and significantly lower risk of bleeding (derivation cohort: HR = 0.61; 95% CI:0.51–0.74; P < 0.001; validation cohort: HR = 0.76; 95% CI:0.61–0.95; P = 0.016) was found in the high DAPT score group that those in the low DAPT score group (Figure 3).
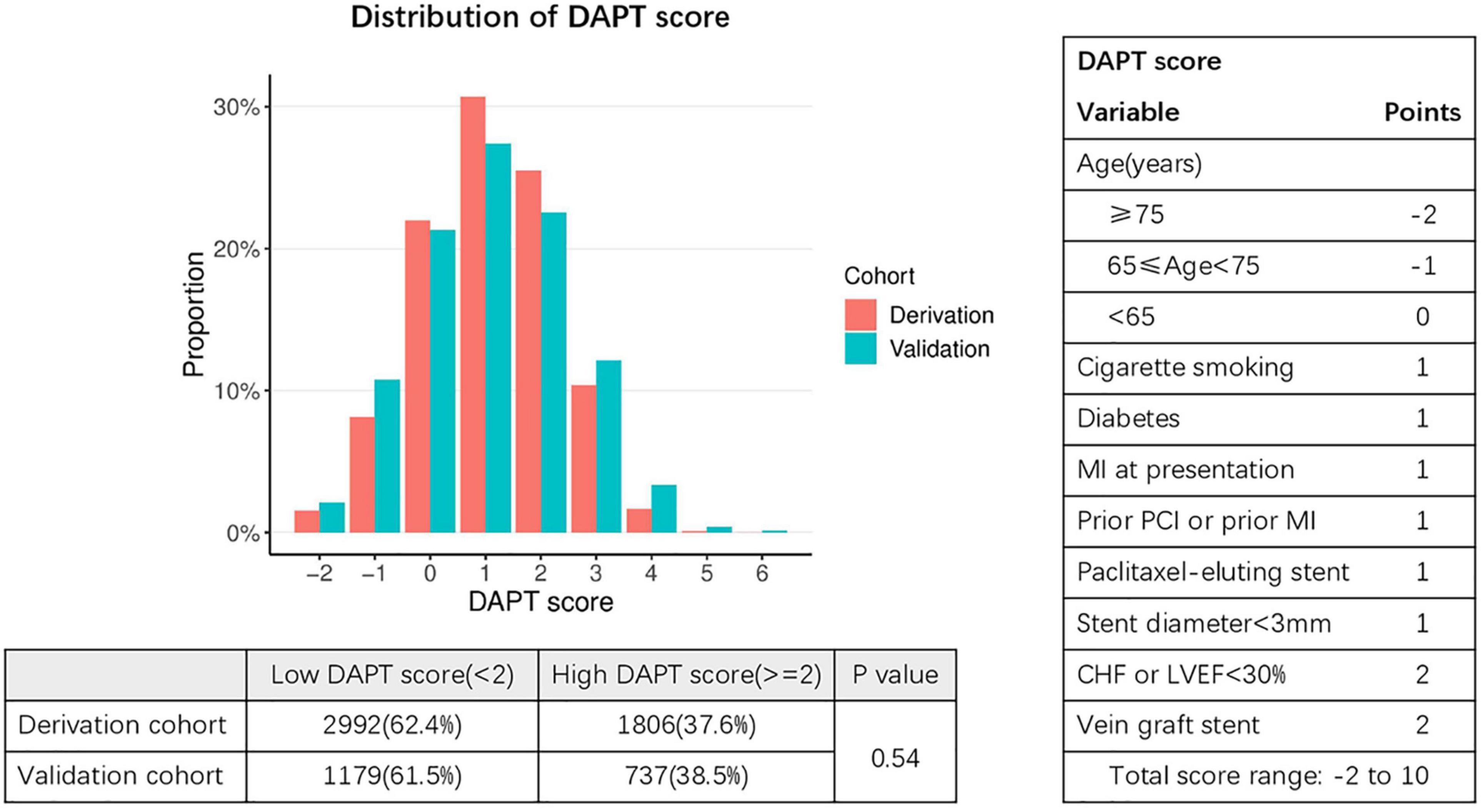
Figure 2. Distribution of DAPT scores in the derivation and validation cohorts. Fisher exact test was used to compare distribution of patients with low DAPT score (< 2) and high DAPT score (≥ 2) in the derivation and validation cohorts. DAPT, dual antiplatelet therapy; MI, myocardial infarction; PCI, percutaneous coronary intervention; CHF, congestive heart failure; LVEF, left ventricular ejection fraction. The X-axis represents DAPT score, while the Y-axis represents proportion.
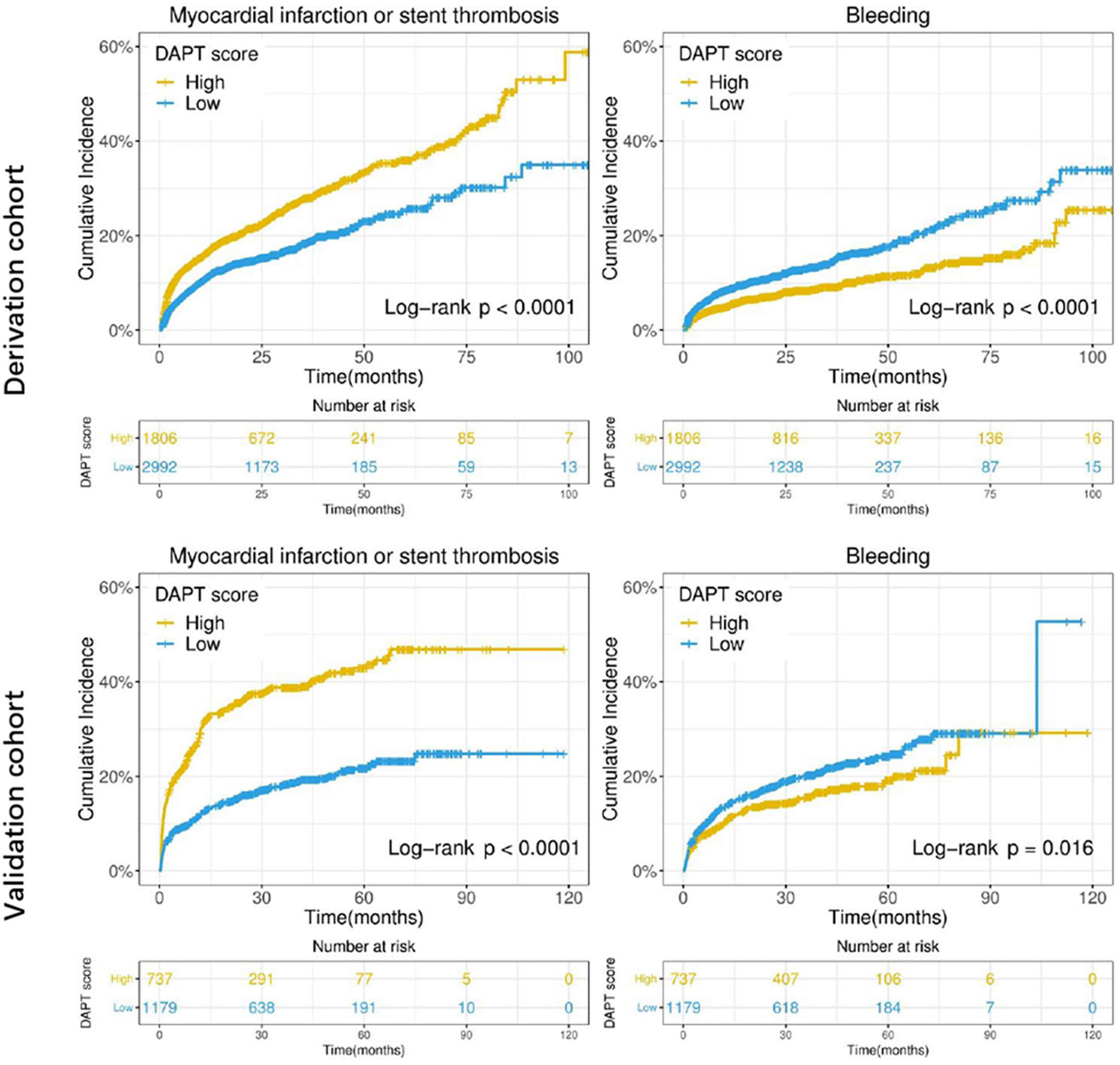
Figure 3. Cumulative incidence of ischemic (myocardial infarction or stent thrombosis) and bleeding events stratified by high (≥ 2) vs. low (< 2) DAPT score in the derivation and validation cohorts. The X-axis represents time (month), while the Y-axis represents cumulative cadences. P < 0.05 was considered to indicate statistically significant differences.
Incorporation of Laboratory Test Biomarkers Into Dual Antiplatelet Therapy Score
Based on data availability in more than 70% of samples in the derivation cohort, a total of 54 laboratory test biomarkers were included for ischemic and bleeding events analysis (Supplementary Table 1). While adjusting for the DAPT score as a continuous variable, a total of 21 and 24 biomarkers were revealed to be significantly associated with ischemic events and bleeding events, respectively (Supplementary Table 2). After removing 14 unqualified biomarkers that were associated with both ischemic and bleeding events in the same direction (both HR > 1 or both HR < 1), a total of 7 ischemia-associated biomarkers and 10 bleeding-associated biomarkers were used as candidates for incremental predictive value investigation (Table 2). No single biomarker exhibited significant improvement in discrimination between ischemic and bleeding events when incorporated into DAPT score alone. Notably, among all possible combinations between 1 ischemia-associated biomarker and 1 bleeding-associated biomarker, the combination of aspartate aminotransferase (AST) and red cell distribution width CV (RDW-CV) showed significant improvement in discrimination between ischemia and bleeding (both P < 0.05) (Table 3). Similarly, high DAPT + labtest (AST + RDW-CV) score group showed higher risk of ischemic events (derivation cohort: P < 0.001; validation cohort: P < 0.0001) and lower risk of bleeding (derivation cohort: P = 0.0046; validation cohort: P = 0.042) than low DAPT + labtest score group (Figure 4).
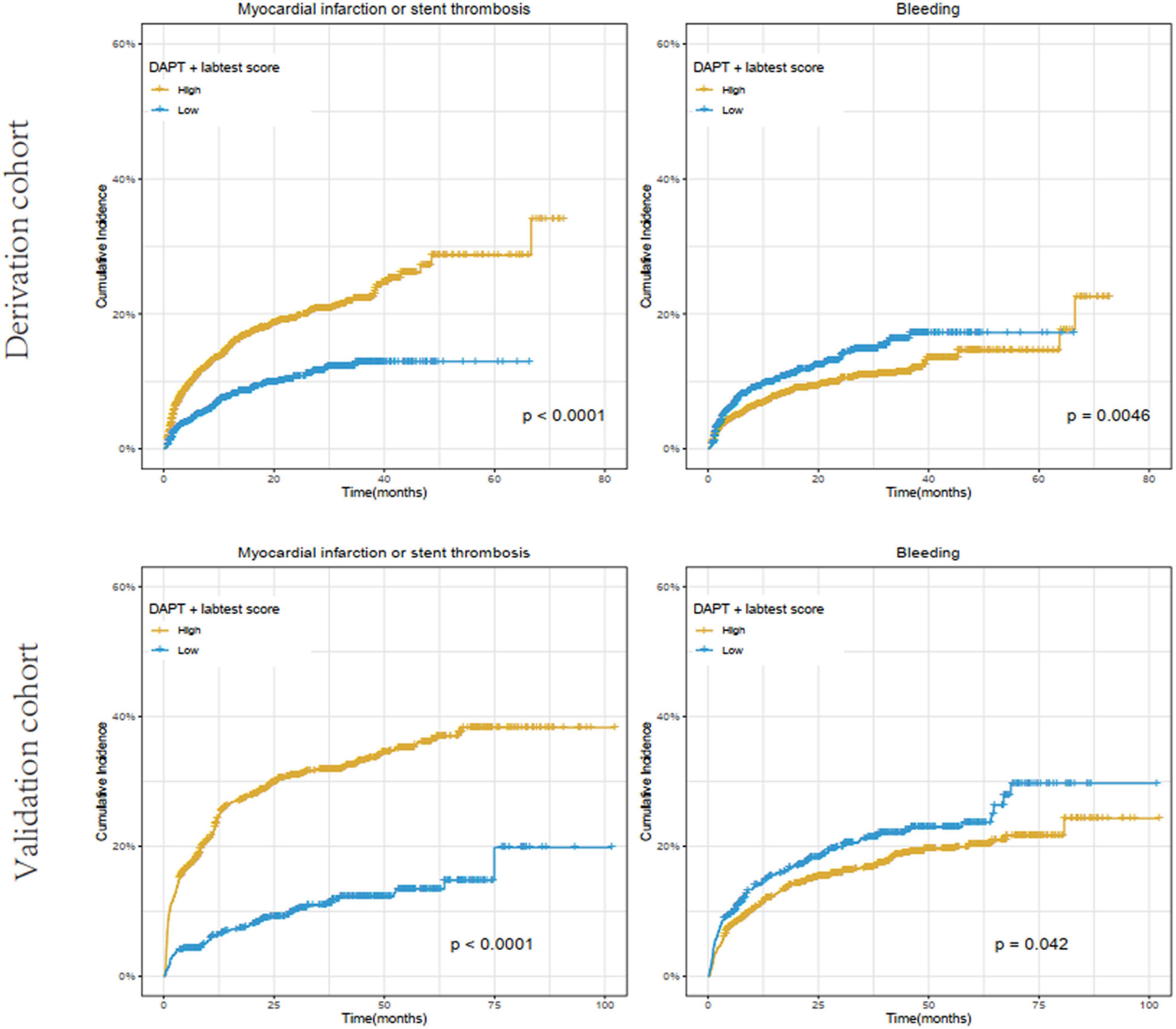
Figure 4. Cumulative incidence of ischemic (myocardial infarction or stent thrombosis) and bleeding events stratified by high (≥ 2) vs. low (< 2) DAPT + labtest (AST + RDW-CV) score in the derivation and validation cohorts. The X-axis represents time (month), while the Y-axis represents cumulative cadences. P < 0.05 was considered to indicate statistically significant differences.
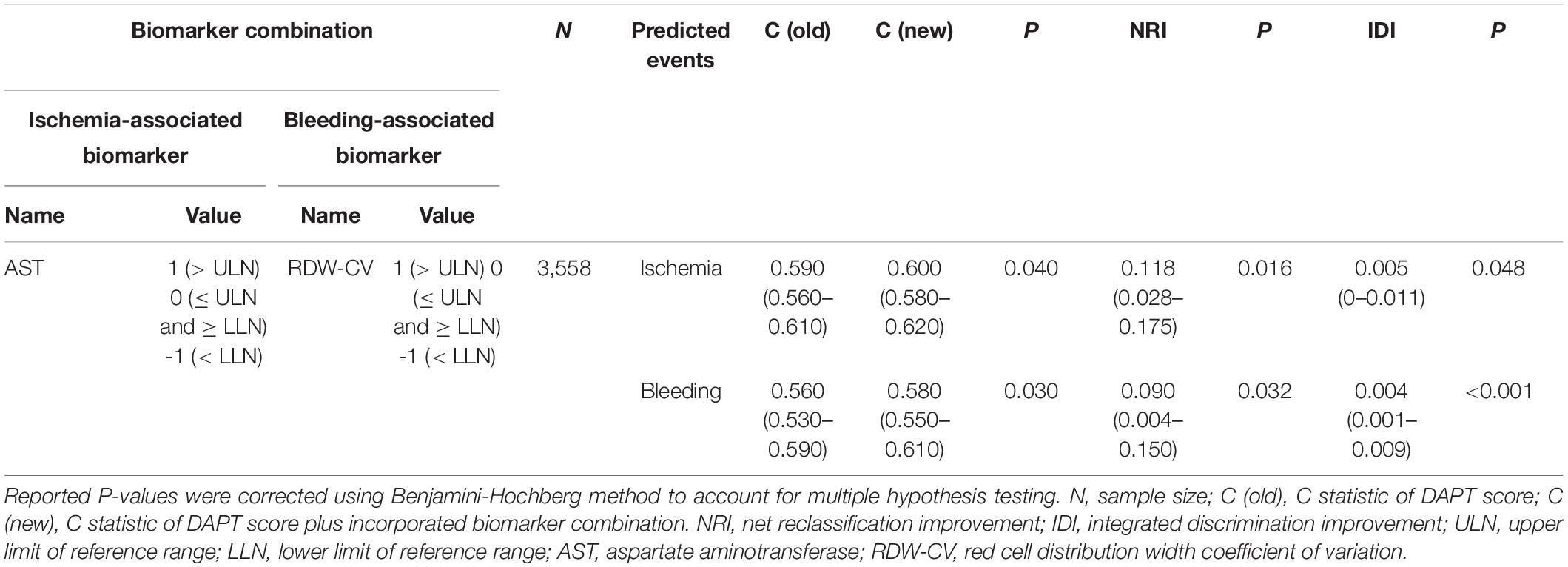
Table 3. The laboratory test biomarker combinations that significantly improved discrimination for both ischemic and bleeding risks when incorporated into DAPT score.
In addition, the incorporation of AST and RDW-CV into DAPT score significantly improved the overall performance (ischemia: C statistic 0.59–0.60, P = 0.04; bleeding: C statistic 0.56–0.58, P = 0.03), as determined by the larger increase in C statistic and the smaller P-value in bleeding prediction. The effect of AST + RDW-CV was also confirmed by NRI (ischemia: 0.118, P = 0.016; bleeding: 0.092, P = 0.032) and IDI (ischemia: 0.005, P = 0.048; bleeding: 0.004, P < 0.001). Meanwhile, AST + RDW-CV also improved discrimination between ischemic and bleeding events (ischemia: C statistic 0.65–0.68, P < 0.001; bleeding: C statistic 0.54–0.56, P = 0.007) in the validation cohort, and the added predictive value was confirmed by NRI (ischemia: 0.28, P < 0.001; bleeding: 0.10, P = 0.022) and IDI (ischemia: 0.03, P < 0.001; bleeding: 0.005, P = 0.002).
Sustained Predictive Value of Aminotransferase + Red Cell Distribution Width CV in the Post-baseline Measurements
To reveal the sustained predictive effect of AST + RDW-CV, the added predictive value of AST + RDW-CV was examined at each time point (every 6 months until 108 months post PCI) in the overall cohort. The results showed that the prediction value of both ischemic and bleeding events was consistently improved at all time points based on C statistic, NRI and IDI analysis (Supplementary Table 3).
Discussion
Although DAPT score can be used to guide DAPT duration for patients undergoing PCI, the impact of ischemia/bleeding risks-related biomarkers on the predictive power of DAPT score is still unclear. In this study, DAPT score successfully stratified post-PCI ischemic and bleeding risks in two independent retrospective derivation and validation cohorts. Moreover, the minimal combination of the laboratory test biomarkers AST plus RDW-CV was revealed to improve prediction for both ischemic and bleeding events when incorporated into DAPT score. Meanwhile, the added predictive value of AST + RDW-CV persisted in the updated measurements taken every 6 months until 108 months post PCI.
Although DAPT can reduce the occurrence of ischemic events after PCI, the bleeding risk is relative high in some circumstances (21). A previous study has shown that the PRECISE-DAPT score after complex PCI was valuable in deciding DAPT duration (22). Alba and Guyatt have shown that the PRECISE-DAPT score can be successfully used to predict moderate bleeding in patients receiving DAPT after coronary stents (23). Another study has shown that the DAPT score can stratify post-PCI ischemic/bleeding events after PCI (24). A meta-analysis has suggested that the DAPT score contributes to either stratifying the risk of ischemia/bleeding or determining the best appropriate DAPT duration in patients with PCI (25). Recent guidelines for DAPT duration showed that patients with ACS should be treated for at least 12 months, unless bleeding exceeded the risk of ischemia (26). It had been suggested that longer DAPT duration could elevate the bleeding events in high-risk patients, but had no effect in the low-risk group, and displayed an obvious ischemic benefit just for low-risk group (27). Hahn et al. showed that 6-month DAPT showed more frequent myocardial infarction and wide non-inferiority margin compared with 12-month or longer DAPT, indicating prolonged DAPT should remain the standard of care in patients with acute coronary syndromes who was not at risk of excessive bleeding (28). In this study, a significant proportion of patients had follow-up information for longer than 30 months post PCI (43% of the overall cohort), which enabled us to evaluate the validity of the DAPT score in revealing the longer term ischemia/bleeding risks. In both, the derivation and validation cohort, the results showed that patients with a high DAPT score had significantly higher risk for ischemic events and significantly lower risk for bleeding compared to patients with a low DAPT score. Thus, we hypothesized that the DAPT score might be used to stratify post-PCI ischemic and bleeding risks.
AST is an important enzyme in amino acid metabolism in vivo (29). The levels of AST are commonly assayed in the clinic as an important biological marker for health (30). A previous study has shown that compared with patients without ischemic stroke, those with ischemic stroke have significantly higher AST (31). AST is one of the enzymes that metabolize glutamate in peripheral blood. Glutamate is the most abundant excitatory neurotransmitter and under pathological conditions as a potent neurotoxin. During the occurrence of ischemic stroke, a large amount of glutamate is produced in ischemic brain tissue, which aggravates cerebral ischemia injury and causes neurological impairment (32). Elevated AST can accelerate the metabolism of glutamate in peripheral blood, reduce the concentration of glutamate in peripheral blood, and thus promote the transport of glutamate in brain to peripheral blood along the concentration gradient (33). In the current study, the association between baseline status of 54 laboratory test biomarkers and ischemic and bleeding events were systematically examined while adjusting for the DAPT score. The results showed that AST was associated with both ischemia and bleeding risks in opposite direction. Importantly, a previous study has shown that the risk factors for ischemic and bleeding events largely overlap (34). Thus, AST might be valuable for the application of DAPT score in post-PCI. Actually, high AST levels have been shown to be associated with increased incidence of mortality in the STEMI patients after PCI (35, 36). Our results suggested that this might be attributed to increased risks of MI or ST post-PCI. Both, high monocyte percentage and monocyte count have been shown to indicate increased ischemia risk, which can be partially explained by the reported association of high monocyte count with angiographic no-reflow and high thrombus burden (37, 38).
RDW is a measure of the range of variation of red blood cell volume (39). RDW has been associated with high mortality in patients with cardiovascular disease, and has been identified as a predictor for bleeding after PCI (40, 41). A previous study has shown that there is a close relationship between RDW-CV and myocardial reperfusion after PCI in patients with CAD (42). Clinical RDW is an indicator of red blood cell heterogeneity. Increased heterogeneity of red blood cells is accompanied by decreased deformability of red blood cells, which leads to increased viscosity of red blood cells, thus slowing down blood flow velocity in microcirculation and promoting tissue ischemia and thrombosis (43). In addition, in the case of increased heterogeneity of red blood cells, the accumulation of red blood cells in the blood stream will aggravate atherosclerosis, and it is easier to form lipid accumulation and cause vasoconstriction (43, 44). On the other hand, patients with cardiovascular diseases are more likely to be complicated with oxidative stress, inflammation, malnutrition, and other conditions, which may aggravate red blood cells formation disorder, causing more obvious heterogeneity of red blood cells volume (45). These may explain the predictive value of RDW. Our results confirmed this finding by showing that high RDW-CV was associated with increased bleeding risk. Anemia has been linked to increased hemorrhagic complications and mortality following PCI (46). Our data indicated that MCH and MCHC inversely correlated with bleeding incidence.
Optimal biomarkers that improve ischemia/bleeding risks prediction when incorporated into DAPT score may be an innovation for DAPT implementation (47, 48). A previous DAPT study has shown a moderate discrimination for ischemia (C statistic 0.70) and bleeding (C statistic 0.68), since both metrics were calculated based on separate models that contained predictors for both ischemia and bleeding risks (7). As these predictors were not incorporated in the DAPT score, the discrimination power of the DAPT score was likely diminished. In this study, only marginal discrimination between ischemia (C statistic 0.58) and bleeding (C statistic 0.57) was observed with the DAPT score in our derivation cohort. Regarding the laboratory test biomarkers, AST + RDW-CV performed the best among all possible combinations of one ischemia-associated and one bleeding-associated biomarker. More importantly, based on the notion that the updated biomarker status during follow-up before adverse event occurrence should more closely reflect patient condition. The current study investigated whether post-baseline measurements of AST + RDW-CV continued to improve prediction for ischemia and bleeding risks. The prediction for both ischemic and bleeding events was consistently improved using the latest measurements collected at all post-baseline time points, which further consolidated AST + RDW-CV as a robust predictor for ischemia and bleeding risks. However, the current study had several limitations: (i) status of anti-platelet medication during the study period was not identified (for example, some patients might be continue DAPT therapy or aspirin/P2Y12 alone after the 12 months DAPT therapy), which prevented us from comparing benefit and harm due to different DAPT durations in subgroups stratified by DAPT score; (ii) the definition of bleeding endpoint in the current study differed from established bleeding criteria such as GUSTO, TIMI and BARC (49), and (iii) continuous measurements of laboratory test biomarkers were categorized according to the upper and lower limit of the reference range to facilitate model simplicity and ease of clinical adoption, which may have limited the sensitivity of detection of certain biomarkers.
Conclusion
In conclusion, DAPT score might be valuable to stratify ischemic/bleeding risks in post-PCI patients. Moreover, AST + RDW-CV might improve prediction for both ischemic/bleeding events when incorporated into DAPT score.
Data Availability Statement
The original contributions presented in the study are included in the article/Supplementary Material, further inquiries can be directed to the corresponding author/s.
Ethics Statement
The studies involving human participants were reviewed and approved by the Ethics Committee of the Yantai Yuhuangding Hospital. The patients/participants provided their written informed consent to participate in this study.
Author Contributions
JY, WT, CS, LZ, YW, and CC: conception and design of the research. JL, LG, SZ, and JS: acquisition of data. DG, JL, LG, SZ, JS, and YY: analysis, interpretation of data, and statistical analysis. CS, LZ, YW, and CC: drafting the manuscript. JY and WT: revision of manuscript for important intellectual content. All authors read and approved the final manuscript.
Funding
This work was supported by the Key R&D Plan of Shandong Province (Major Scientific and Technological Innovation Project) (Grant No. 2019JZZY011018), the Yantai Science and Technology Plan Project (Grant Nos. 2021MSGY042 and 2019MSGY133), and the Key Research and Development Program of Shandong Province (Grant No. 2019GSF108142).
Conflict of Interest
YY and WT were employed by the company Gennlife (Beijing) Technology Co., Ltd.
The remaining authors declare that the research was conducted in the absence of any commercial or financial relationships that could be construed as a potential conflict of interest.
Publisher’s Note
All claims expressed in this article are solely those of the authors and do not necessarily represent those of their affiliated organizations, or those of the publisher, the editors and the reviewers. Any product that may be evaluated in this article, or claim that may be made by its manufacturer, is not guaranteed or endorsed by the publisher.
Supplementary Material
The Supplementary Material for this article can be found online at: https://www.frontiersin.org/articles/10.3389/fcvm.2022.834975/full#supplementary-material
References
1. Khera AV, Kathiresan S. Genetics of coronary artery disease: discovery, biology and clinical translation. Nat Rev Genet. (2017) 18:331. doi: 10.1038/nrg.2016.160
2. Levine GN, Bates ER, Blankenship JC, Bailey SR, Bittl JA, Cercek B, et al. 2015 ACC/AHA/SCAI focused update on primary percutaneous coronary intervention for patients with ST-elevation myocardial infarction: an update of the 2011 ACCF/AHA/SCAI guideline for percutaneous coronary intervention and the 2013 ACCF/AHA guideline for the management of ST-elevation myocardial infarction. J Am Coll Cardiol. (2016) 67:1235–50.
3. Levine GN, Bates ER, Bittl JA, Brindis RG, Fihn SD, Fleisher LA, et al. 2016 ACC/AHA guideline focused update on duration of dual antiplatelet therapy in patients with coronary artery disease: a report of the American college of cardiology/American heart association task force on clinical practice guidelines. J Am Coll Cardiol. (2016) 68:1082–115.
4. Valgimigli M, Bueno H, Byrne RA, Collet JP, Costa F, Jeppsson A, et al. 2017 ESC focused update on dual antiplatelet therapy in coronary artery disease developed in collaboration with EACTS: the task force for dual antiplatelet therapy in coronary artery disease of the European society of cardiology (ESC) and of the European association for cardio-thoracic surgery (EACTS). Eur Heart J. (2018) 39:213–60. doi: 10.1093/eurheartj/ehx419
5. Mauri L, Kereiakes DJ, Yeh RW, Driscoll-Shempp P, Cutlip DE, Steg PG, et al. Twelve or 30 months of dual antiplatelet therapy after drug-eluting stents. N Engl J Med. (2014) 371:2155–66. doi: 10.1056/NEJMoa1409312
6. Cutlip DE. Dual antiplatelet therapy after coronary stenting: rationale for personalized duration of therapy. US Cardiol Rev. (2017) 11:31–6. doi: 10.15420/usc.2017:7:2
7. Yeh RW, Secemsky EA, Kereiakes DJ, Normand SL, Gershlick AH, Cohen DJ, et al. Development and validation of a prediction rule for benefit and harm of dual antiplatelet therapy beyond 1 year after percutaneous coronary intervention. JAMA. (2016) 315:1735–49. doi: 10.1001/jama.2016.3775
8. Gusto Investigators. An international randomized trial comparing four thrombolytic strategies for acute myocardial infarction. N Engl J Med. (1993) 329:673–82. doi: 10.1056/NEJM199309023291001
9. Harada Y, Michel J, Lohaus R, Mayer K, Emmer R, Lahmann AL, et al. Validation of the DAPT score in patients randomized to 6 or 12 months clopidogrel after predominantly second-generation drug-eluting stents. Thromb Haemost. (2017) 117:1989–99. doi: 10.1160/TH17-02-0101
10. Piccolo R, Gargiulo G, Franzone A, Santucci A, Ariotti S, Baldo A, et al. Use of the dual-antiplatelet therapy score to guide treatment duration after percutaneous coronary intervention. Ann Intern Med. (2017) 167:17–25. doi: 10.7326/M16-2389
11. Yeh RW, Kereiakes DJ, Secemsky EA, Steg PG, Mauri L. The DAPT score in Sweden: successful validation, flawed interpretation. J Am Coll Cardiol. (2019) 73:113–4. doi: 10.1016/j.jacc.2018.10.053
12. Luescher TF. Managing Acute Coronary Syndromes: From Novel Biomarkers to Mechanical Support Devices and DAPT in Thrombocytopenia. Oxford: Oxford University Press (2017).
13. Dadu RT, Kleiman NS. Biomarkers for Antiplatelet Therapy. In: Biomarkers in Cardiovascular Disease. Amsterdam: Elsevier (2019). p. 139–48.
14. Lordkipanidzé M, So D, Tanguay JF. Platelet function testing as a biomarker for efficacy of antiplatelet drugs. Biomarker Med. (2016) 10:903–18. doi: 10.2217/bmm-2016-0070
15. Levine GN, Bates ER, Blankenship JC, Bailey SR, Bittl JA, Cercek B, et al. 2011 ACCF/AHA/SCAI guideline for percutaneous coronary intervention. A report of the American college of cardiology foundation/American heart association task force on practice guidelines and the society for cardiovascular angiography and interventions. J Am Coll Cardiol. (2011) 58:e44–122.
16. Bornkamp B, Bermann G. Estimating the treatment effect in a subgroup defined by an early post-baseline biomarker measurement in randomized clinical trials with time-to-event endpoint. Stat Biopharm Res. (2020) 12:19–28. doi: 10.1080/19466315.2019.1575280
17. Kang L, Chen W, Petrick NA, Gallas BD. Comparing two correlated C indices with right-censored survival outcome: a one-shot nonparametric approach. Stat Med. (2015) 34:685–703. doi: 10.1002/sim.6370
18. Kang L, Chen W, Petrick NA, Gallas BD. Comparing two correlated C indices with right-censored survival outcome: a one-shot nonparametric approach. Stat Med. (2015) 34:685–703. doi: 10.1002/sim.6370
19. Pencina MJ, D’Agostino RBSr, D’Agostino RB Jr, Vasan RS. Evaluating the added predictive ability of a new marker: from area under the ROC curve to reclassification and beyond. Stat Med. (2008) 27:157–72. doi: 10.1002/sim.2929
20. Uno H, Cai T. survIDINRI: IDI and NRI for Comparing Competing Risk Prediction Models with Censored Survival Data. R Package Version 1. (2013). Available online at: http://cran.itam.mx/web/packages/survIDINRI/index.html
21. Amin AP, Wang TY, McCoy L, Bach RG, Effron MB, Peterson ED, et al. Impact of bleeding on quality of life in patients on DAPT: insights from TRANSLATE-ACS. J Am Coll Cardiol. (2016) 67:59–65. doi: 10.1016/j.jacc.2015.10.034
22. Costa F, Van Klaveren D, Feres F, Raber L, Pilgrim T, Hong M, et al. P3179 exploring the value of the PRECISE-DAPT score after complex percutaneous coronary intervention to inform dual antiplatelet therapy duration decision-making. Eur Heart J. (2018) 39:ehy563.3179.
23. Alba AC, Guyatt G. In patients receiving DAPT after coronary stents, the PRECISE-DAPT score predicted bleeding moderately well. Ann Intern Med. (2017) 167:JC11–11. doi: 10.7326/ACPJC-2017-167-2-011
24. Ueda P, Bodil Svennblad B, James S, Alfredsson J, Erlinge D, Omerovic E, et al. 1400 external validation of the DAPT score in nationwide real-world data: ischemic and bleeding events following coronary stent implantation. Eur Heart J. (2018) 39:ehy565.1400.
25. Witberg G, Zusman O, Yahav D, Perl L, Vaknin-Assa H, Kornowski R. Meta-analysis of studies examining the external validity of the dual antiplatelet therapy score. Eur Heart J Cardiovasc Pharmacother. (2019) 6:285–91. doi: 10.1093/ehjcvp/pvz075
26. Windecker S, Kolh P, Alfonso F, Collet JP, Cremer J, Falk V, et al. 2014 ESC/EACTS guidelines on myocardial revascularization: the task force on myocardial revascularization of the European society of cardiology (ESC) and the European association for cardio-thoracic surgery (EACTS) developed with the special contribution of the European association of percutaneous cardiovascular interventions (EAPCI). Eur Heart J. (2014) 35:2541–619. doi: 10.1093/eurheartj/ehu278
27. Costa F, van Klaveren D, James S, Heg D, Räber L, Feres F, et al. Derivation and validation of the predicting bleeding complications in patients undergoing stent implantation and subsequent dual antiplatelet therapy (PRECISE-DAPT) score: a pooled analysis of individual-patient datasets from clinical trials. Lancet. (2017) 389:1025–34. doi: 10.1016/S0140-6736(17)30397-5
28. Hahn JY, Song YB, Oh JH, Cho DK, Lee JB, Doh JH, et al. 6-month versus 12-month or longer dual antiplatelet therapy after percutaneous coronary intervention in patients with acute coronary syndrome (SMART-DATE): a randomised, open-label, non-inferiority trial. Lancet. (2018) 391:1274–84. doi: 10.1016/S0140-6736(18)30493-8
29. Zou W, Chen L, Huang X, Wang L, Jiang T, Song J, et al. The prognostic significance of aspartate aminotransferase to neutrophils ratio in HCC patients treated with TACE. J Interv Radiol. (2017) 26:705–11.
30. McGill MR. The past and present of serum aminotransferases and the future of liver injury biomarkers. EXCLI J. (2016) 15:817. doi: 10.17179/excli2016-800
31. Zhang X, Qi X, Yoshida EM, Méndez-Sánchez N, Hou F, Deng H, et al. Ischemic stroke in liver cirrhosis: epidemiology, risk factors, and in-hospital outcomes. Eur J Gastroenterol Hepatol. (2018) 30:233–40. doi: 10.1097/MEG.0000000000001011
32. Khanna S, Briggs Z, Rink C. Inducible glutamate oxaloacetate transaminase as a therapeutic target against ischemic stroke. Antioxid Redox Signal. (2015) 22:175–86. doi: 10.1089/ars.2014.6106
33. Chao N, Li ST. Synaptic and extrasynaptic glutamate signaling in ischemic stroke. Curr Med Chem. (2014) 21:2043–64. doi: 10.2174/0929867321666131228204533
34. Matteau A, Yeh RW, Camenzind E, Steg PG, Wijns W, Mills J, et al. Balancing long-term risks of ischemic and bleeding complications after percutaneous coronary intervention with drug-eluting stents. Am J Cardiol. (2015) 116:686–93. doi: 10.1016/j.amjcard.2015.05.036
35. Gao M, Cheng Y, Zheng Y, Zhang W, Wang L, Qin L. Association of serum transaminases with short- and long-term outcomes in patients with ST-elevation myocardial infarction undergoing primary percutaneous coronary intervention. BMC Cardiovasc Disord. (2017) 17:43. doi: 10.1186/s12872-017-0485-6
36. Moon J, Kang W, Oh PC, Seo SY, Lee K, Han SH, et al. Serum transaminase determined in the emergency room predicts outcomes in patients with acute ST-segment elevation myocardial infarction who undergo primary percutaneous coronary intervention. Int J Cardiol. (2014) 177:442–7. doi: 10.1016/j.ijcard.2014.09.002
37. Wang Z, Liu N, Ren L, Lei L, Ye H, Peng J. Association of monocyte count on admission with the angiographic thrombus burden in patients with ST-segment elevation myocardial infarction undergoing primary percutaneous coronary intervention. Arq Bras Cardiol. (2018) 110:333–8. doi: 10.5935/abc.20180034
38. Wang Z, Ren L, Liu N, Lei L, Ye H, Peng J. Association of monocyte count on admission with angiographic no-reflow after primary percutaneous coronary intervention in patients with ST-segment elevation myocardial infarction. Kardiol Pol. (2016) 74:1160–6. doi: 10.5603/KP.a2016.0065
40. Al-Kindi SG, Refaat M, Jayyousi A, Asaad N, Al Suwaidi J, Abi Khalil C. Red cell distribution width is associated with all-cause and cardiovascular mortality in patients with diabetes. Biomed Res Int. (2017) 2017:5843702. doi: 10.1155/2017/5843702
41. Fatemi O, Torguson R, Chen F, Ahmad S, Badr S, Satler LF, et al. Red cell distribution width as a bleeding predictor after percutaneous coronary intervention. Am Heart J. (2013) 166:104–9. doi: 10.1016/j.ahj.2013.04.006
42. Salem HS, El Sheshtawy F, Kassem H, El Amrousy M. The impact of red cell distribution width level on myocardial reperfusion after percutaneous coronary intervention in patients with coronary heart disease. J Am Coll Cardiol. (2018) 16:S48–9. doi: 10.1097/hpc.0000000000000215
43. Jiang P, Song Y, Xu JJ, Ma YL, Tang XF, Yao Y, et al. [Impact of platelet distribution width on the extent and long-term outcome of patients with stable coronary artery disease post percutaneous coronary intervention]. Zhonghua Xin Xue Guan Bing Za Zhi. (2017) 45:862–6. doi: 10.3760/cma.j.issn.0253-3758.2017.10.010
44. Dai C, Chen Z, Qian J, Ge J. Red cell distribution width as a marker of periprocedural myocardial infarction in patients with elective percutaneous coronary intervention. J Cardiovasc Transl Res. (2021) 14:449–56. doi: 10.1007/s12265-020-10073-w
45. Li N, Zhou H, Tang Q. Red blood cell distribution width: a novel predictive indicator for cardiovascular and cerebrovascular diseases. Dis Markers. (2017) 2017:7089493. doi: 10.1155/2017/7089493
46. Voeltz MD, Patel AD, Feit F, Fazel R, Lincoff AM, Manoukian SV. Effect of anemia on hemorrhagic complications and mortality following percutaneous coronary intervention. Am J Cardiol. (2007) 99:1513–7. doi: 10.1016/j.amjcard.2007.01.027
47. Friede K, Voora D. The need for sex-specific precision biomarkers for antiplatelet therapies. Future Med. (2017) 13:419–22. doi: 10.2217/fca-2017-0043
48. Hein R, Verheugt FW, Sibbing D. Risk evaluation tools for prediction and possible guidance of dapt: is scoring a hit in East Asians patients undergoing PCI? Thromb Haemost. (2019) 119:1033–5. doi: 10.1055/s-0039-1692169
Keywords: percutaneous coronary intervention, dual antiplatelet therapy score, aspartate aminotransferase, red cell distribution width CV, biomarker
Citation: Sun C, Zhong L, Wu Y, Cao C, Guo D, Liu J, Gong L, Zhang S, Sun J, Yu Y, Tong W and Yang J (2022) Incorporation of Laboratory Test Biomarkers Into Dual Antiplatelet Therapy Score Improves Prediction of Ischemic and Bleeding Events in Post-percutaneous Coronary Intervention Patients. Front. Cardiovasc. Med. 9:834975. doi: 10.3389/fcvm.2022.834975
Received: 13 January 2022; Accepted: 11 April 2022;
Published: 16 May 2022.
Edited by:
Daniela Trabattoni, Monzino Cardiology Center (IRCCS), ItalyReviewed by:
Mariusz Tomaniak, Medical University of Warsaw, PolandKyeong Ho Yun, Wonkwang University, South Korea
Copyright © 2022 Sun, Zhong, Wu, Cao, Guo, Liu, Gong, Zhang, Sun, Yu, Tong and Yang. This is an open-access article distributed under the terms of the Creative Commons Attribution License (CC BY). The use, distribution or reproduction in other forums is permitted, provided the original author(s) and the copyright owner(s) are credited and that the original publication in this journal is cited, in accordance with accepted academic practice. No use, distribution or reproduction is permitted which does not comply with these terms.
*Correspondence: Weiwei Tong, dG9uZ3dlaXdlaUBnZW5ubGlmZS5jb20=; Jun Yang, eWFuZ2p1bjA5MDdAMTI2LmNvbQ==
†These authors share first authorship