- 1Xiyuan Hospital, China Academy of Chinese Medical Sciences, Beijing, China
- 2Graduate School, China Academy of Chinese Medical Sciences, Beijing, China
- 3National Clinical Research Center for Chinese Medicine Cardiology, Beijing, China
Purpose: Salvia miltiorrhiza Bge. (Danshen, DS) and Ligusticum chuanxiong Hort. (Chuanxiong, CX) have been widely used in traditional Chinese medicine to prevent and treat myocardial ischemia and renal insufficiency, and their extracts (Guanxinning injection, GXN) have been reported to exhibit antioxidant, anti-inflammatory, and anti-ischemia-reperfusion injury properties. It is well-established that ischemic postconditioning (IPOC) can protect against myocardial ischemia-reperfusion (I/R) injury in rats with chronic renal failure (CRF). However, little is known on whether GXN combined with IPOC may affect myocardial I/R injury in CRF rats. We sought to observe the effect of GXN combined with IPOC on myocardial I/R injury in CRF rats by quantifying changes in the expression of proteins related to mitochondrial dynamics.
Materials and Methods: In a survey, 90 Wistar rats were randomly divided into 6 groups (15 rats per group): CRF group, I/R group, comorbid group (CRF + I/R), IPOC group, IPOC + GXN group and the sham group. Changes in blood myocardial injury markers, urea, and creatinine were analyzed. Heart tissues were harvested for histomorphometry and western blotting when rats were sacrificed. Myocardial infarction area was measured by Evans blue and Triphenyltetrazolium chloride solution staining. The expressions of mitochondrial fission relative proteins (DRP1 and FIS1) and mitochondrial fusion relative proteins (OPA1 and MFN1) were detected by western blotting.
Results: IPOC could significantly decrease myocardial injury markers and myocardial area of necrosis (AN)/area at risk (AAR) of the comorbid model rats. Further results showed that GXN combined with IPOC could significantly reduce CK-MB levels and myocardial AN/AAR in comorbid model rats compared with the IPOC group. Meanwhile, both IPOC and IPOC + GXN significantly reduced DRP1 levels and increased the MFN1 and OPA1 protein levels in the comorbid model rats. However, compared with the IPOC group, MFN1 and OPA1 protein levels increased significantly in the IPOC + GXN group.
Conclusion: Extracts of DS and CX combined with IPOC exert a protective effect against myocardial I/R injury in rats with CRF, mediated by increased expression of mitochondrial fusion proteins (MFN1 and OPA1).
Introduction
The global burden of chronic kidney disease (CKD) has increased in recent years, with approximately 10% of adults worldwide suffering from some form of CKD, leading to 1.2 million deaths and 28 million lives lost each year. Consistently, the prevalence of chronic renal failure (CRF) has also escalated (1). Despite substantial breakthroughs in revascularization and medicinal treatment, acute myocardial infarction (AMI), and subsequent progression to heart failure (HF) are associated with an increased risk of death owing to ischemia-reperfusion (I/R) injury (2). It is widely acknowledged that cardiovascular diseases such as AMI and CKD exacerbate each other, accounting for the high mortality rates (3). A study demonstrated that compared to patients with normal renal function, cardiovascular mortality was twice as high in patients with stage 3 CKD and three times higher in stage 4 disease (4). Besides, CKD patients reportedly account for 16.5% of AMI survivors (5); current evidence suggests that CKD is linked to poor long-term outcomes in patients with AMI, even after revascularization intervention based on standardized western medicine (6). These findings emphasize the need to explore novel approaches to improve the prognosis of AMI patients with CKD and reduce death or disability rates.
Ischemic postconditioning (IPOC) was first proposed in 2003 by Zhao et al., who found that repeated transient ischemia 60 min before ligation of the descending coronary artery could significantly reduce reperfusion injury in dogs before restoration of continuous coronary blood perfusion (7). In addition to animal studies, IPOC has been shown to reduce myocardial injury in patients following percutaneous coronary intervention (PCI) or coronary artery bypass grafting in clinical trials (8, 9). An increasing body of evidence suggests that IPOC can protect against myocardial I/R injury, reduce myocardial infarction area and improve cardiac function in chronic renal failure (CRF) rats (10). In a previous study, we established that IPOC could reduce the myocardial infarct area caused by I/R and protect cardiac function. Interestingly, the application of Huoxue Chinese medicine potentiated the protective effect of IPOC against myocardial injury caused by I/R (11). Moreover, a clinical trial study showed that Huoxue Chinese medicine could reduce the primary endpoint events (death, non-fatal myocardial infarction, ischemia-driven revascularization) and secondary endpoint events (readmission for acute coronary syndrome, stroke, HF) for patients with acute coronary syndrome after PCI (12). The subgroup analysis substantiated that standardized treatment with Huoxue Chinese medicine combined with western medicine could improve cardiovascular prognosis and renal function of patients with acute coronary syndrome complicated with renal insufficiency after PCI (13). Accordingly, further studies are warranted to assess whether Huoxue Chinese medicine combined with IPOC can alleviate myocardial I/R injury in the presence of comorbidities such as CKD.
Salvia miltiorrhiza Bge. (Danshen, DS) and Ligusticum chuanxiong Hort. (Chuanxiong, CX) are commonly Huoxue Chinese medicine during clinical practice. The extracts of DS and CX, which are well-established to protect against myocardial I/R injury, were selected as the research drugs (14). The extracts of DS and CX are compound traditional Chinese medicine (TCM) injections with many functions, including dilating coronary arteries, promoting coronary collateral circulation, accelerating ischemic myocardial repair, protecting ischemic hypoxic myocardium, and treating angina pectoris. It is approved by China’s State Food and Drug Administration (SFDA) for clinical use in patients with coronary artery disease under the trade name Guanxinning (GXN).
Mitochondria are key organelles that participate in the pathophysiology of CKD complicated with AMI (15). Mitochondria are well-recognized to maintain a state of kinetic balance in physiological states, namely continuous division, and fusion. However, during cell injury such as I/R injury, this balance is dysregulated, leading to cell apoptosis or necrosis (16). Indeed, to protect cardiac myocytes, the equilibrium between mitochondrial division and fusion must be maintained. Current evidence suggests that dynamin-related protein-1 (DRP1) and mitochondrial fission protein 1 (FIS1), an adaptor protein anchored in the outer membrane, are involved in mitochondrial division. Mitochondrial fusion is controlled by the outer membrane’s mitofusin (MFN) and the inner membrane’s ocular optic atrophy 1 (OPA1). Therefore, this study sought to observe the effect of the GXN combined with IPOC on protecting against myocardial I/R injury in CRF rats and exploring the underlying mitochondrial dynamics-related mechanisms.
Materials and Methods
Chemicals and Reagents
Adenine containing a purity 99% was obtained from Amresco (Texas, America). The kits for determination of creatine kinase-MB (CK-MB) and cardiac troponin T (cTnT) were provided by BioSino (Beijing, China). The kits of serum creatinine (CREA) and urea nitrogen (UREA) were produced by Roche Diagnostics GmbH (Mannheim, Germany). Evans blue and triphenyltetrazolium chloride solution (TTC) were bought from Sigma-Aldrich (St. Louis, MO, United States). Tissue mitochondria isolation kit was bought from Beyotime Biotechnology Co., Ltd. (Shanghai, China). Protein concentration was measured using a bicinchoninic acid (BCA) protein assay kit (AR1189, Boster, Wuhan, China). Antibodies against FIS1 (1:500), OPA1 (1:1000), MFN1 (1:1000), and COX IV (1:1000) were purchased from Abcam (Cambridge, United Kingdom). Antibody against DRP1 (1:1000) was purchased from Cell Signaling Technology (Beverly, MA, United States). The GXN was purchased from Yabao Pharmaceutical Group Co. Ltd. (Yuncheng, China, SFDA approval number: Z14020782).
Experimental Animals
In a survey, 90 Wistar rats (male, n = 45 and female, n = 45) aged 6 weeks weighing approximately 160–180 g were purchased by SiPeiFu Biotechnology Co., Ltd. (SCXK2011-0004, Beijing, China). Successful model rats were randomly divided into 6 groups, 15 rats each group: CRF group (CRF model was established), I/R group (myocardial I/R injury was performed), comorbid model group (CRF + I/R, myocardial I/R injury was performed in CRF rat), IPOC group (comorbid model rat was treated with IPOC), IPOC + GXN group (GXN was injected into the iliac vein in rats of the IPOC group after 10 min of ischemia). In addition, a sham operation group was set. The care and use of animals in the experiment were in accordance with the requirements of Beijing experimental animal management regulations and animal ethics regulations (Figure 1).
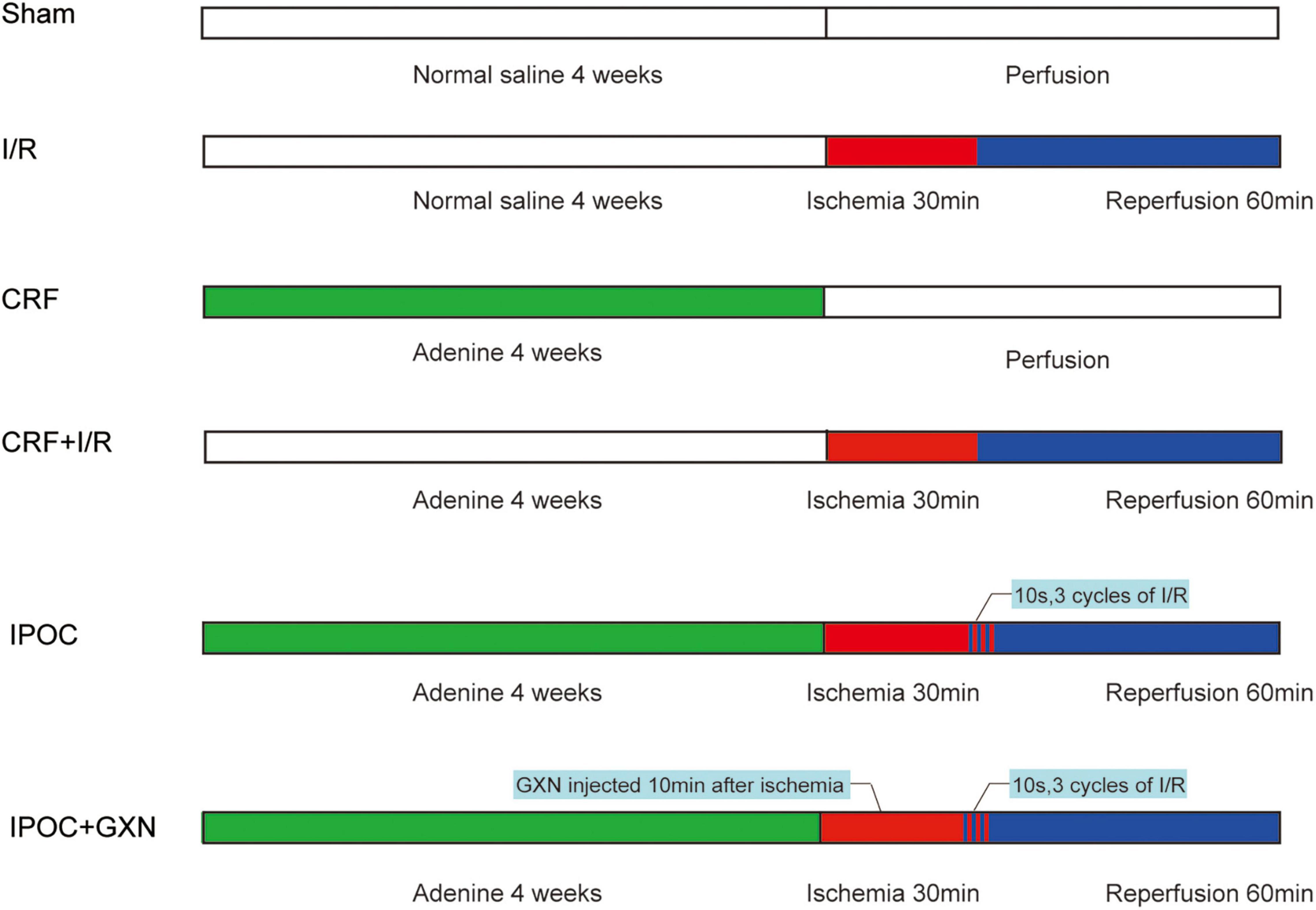
Figure 1. Experimental protocol. The rats were given adenine daily for 4 weeks to establish the CRF model. Then the rats underwent a 30 min ischemia procedure, followed by 60 min of reperfusion. After 30 min of ischemia, the rats were immediately given 3 cycles of reperfusion and ischemia (reperfusion: the suture line was relaxed for 10 s, ischemia: the suture line was tightened for 10 s). The GXN is injected into the iliac vein 10 min after ischemia. The sham-operated group was given an equal amount of normal saline and underwent a procedure without ischemia, but it was time-matched. Sham, sham-operated group; I/R, ischemia/reperfusion injury group (no CRF); CRF, chronic renal failure group (no I/R injury); CRF + I/R, comorbid model group; IPOC, comorbid model rat was treated with ischemic postconditioning; IPOC + GXN, the Guanxinning injection was injected in rats of the IPOC group after 10 min of ischemia.
Chronic Renal Failure Rat Model
The rats were given 200 mg/kg adenine daily for 4 weeks after adaptive feeding for 1 week to establish the CRF model. Rats in the sham group were given a normal diet for 4 weeks, ad libitum intake of normal feed, and oral gavage with an equal amount of normal saline.
Myocardial Ischemia-Reperfusion Injury Model in Chronic Renal Failure Rat
Chronic renal failure rats were anesthetized with 1% sodium pentobarbital (50 mg/kg) by intraperitoneal injection and fixed in the supine position on the operating table. The electrocardiogram lead II was recorded. The neck and left chest skin were shaved preoperative and sterilized. The neck skin was cut open, the trachea was exposed, and the animal ventilator (tidal volume was 10 mL/kg weight, the respiratory frequency was 60 times/min) was connected to the endotracheal tube. A thoracotomy incision was made between the third and fourth rib was operated along the left edge sternum. The pericardium was cut open, and the heart was exposed. The left anterior descending coronary artery was occluded between the lower margin of the left auricle and the left margin of the pulmonary conus, and a silicone hose of diameter 1.5 mm was placed at the ligated position. Occlusion was performed for 30 min and reperfusion for 60 min. In the sham group, the left anterior descending coronary artery was not occluded.
The rats were given 200 mg/kg adenine daily for 4 weeks after adaptive feeding for 1 week to establish the CRF model. The rats in the sham operation group were given a normal diet for 4 weeks, ad libitum intake of normal feed, and oral gavage with an equal amount of normal saline.
Ischemic Postconditioning Operation
After occlusion of the left anterior descending coronary artery for 30 min, the rat was immediately given 3 cycles of reperfusion and ischemia (reperfusion: the suture line was relaxed for 10 s, ischemia: the suture line was tightened for 10 s). The suture was completely released, and reperfusion was conducted for 60 min.
Drug Intervention
Guanxinning injection is composed of extracts of DS and CX and is manufactured according to Chinese Materia Medica standards, whose important ingredients include Danshensu, Salvianolic acid B, Protocatechuic aldehyde, Rosmarinic acid, Senkyunolide I, Salvianolic acid A, and so on (Figure 2; 17, 18). The GXN is injected into the iliac vein 10 min after ischemia.
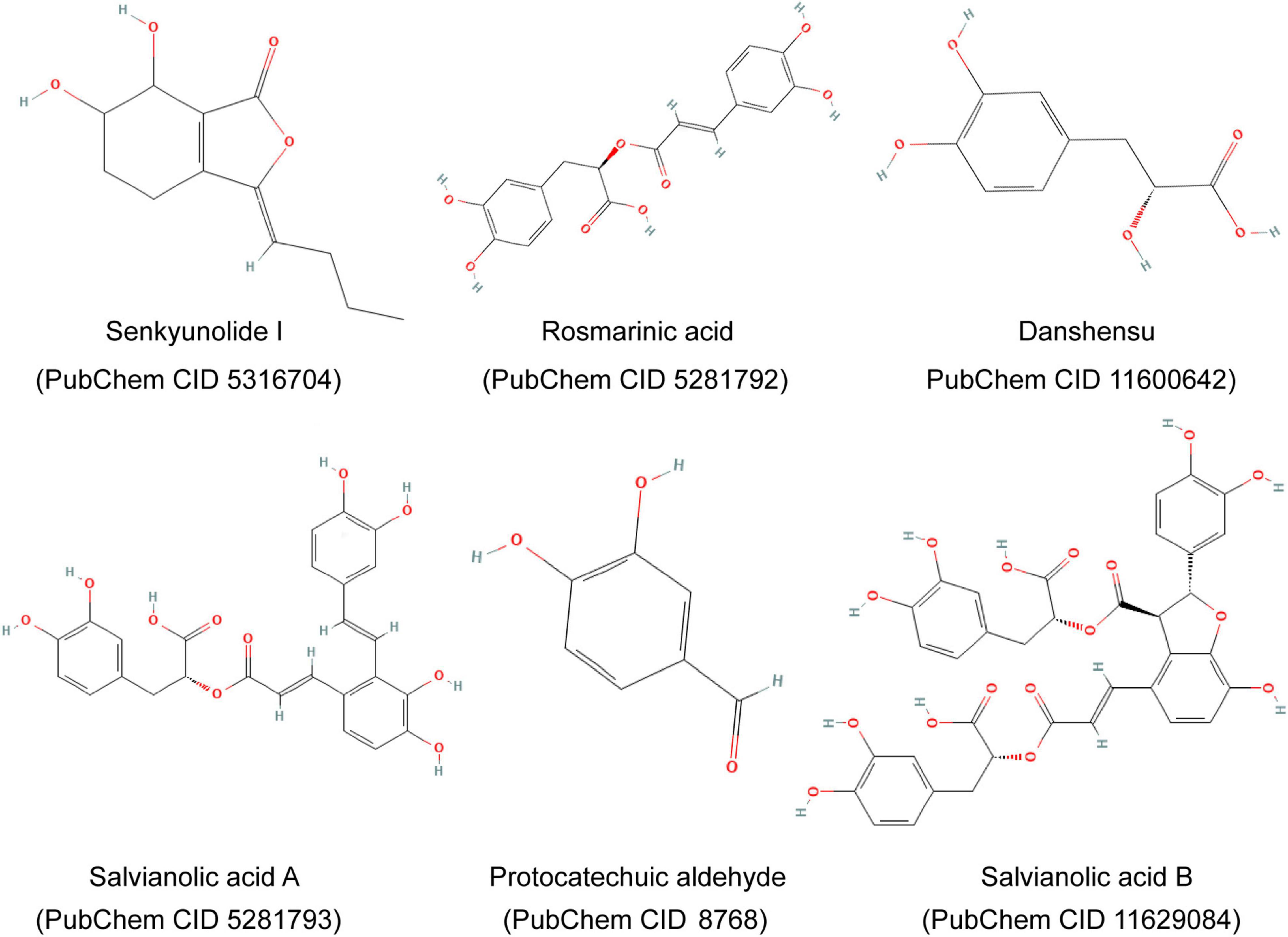
Figure 2. Major components and chemical structures of extracts of Salvia miltiorrhiza Bge. and Ligusticum chuanxiong Hort. (Guanxinning injection).
Myocardial Injury Markers and Renal Function Indexes
Two mL blood samples were collected from the abdominal aorta of rats, and serum was separated. The cTnT levels were determined by an automatic microplate reader (STAT FAX 2100, Awareness Technology, Inc., Palm City, FL, United States). CK-MB, creatinine, and urea were measured by an automatic biochemical analyzer (Hitachi 7160, Tokyo, Japan).
Myocardial Infarction Area and Pathological Observation
The infarction area was assessed by Evans blue and TTC staining. The left anterior descending coronary artery in myocardial I/R rat models was occluded in situ, then 0.5% Evans blue (2–3 mL) was injected into the heart via the aorta. Subsequently, the heart was quickly removed and washed with physiological saline. The heart below the level of ligation was cut into five sections, which were immersed in 2% TTC phosphate buffer, incubated at 37°C for 30 min, and then fixed with 10% formaldehyde for 1 day. After the above treatment, the myocardial tissue was classified as normal myocardium (in blue), ischemic myocardium (in pale red), and infarcted myocardium (in pale white). The percentage of the area at risk (AAR, ischemic area, and infarcted area) in the left ventricular (LV) area (AAR/LV) and the percentage of the area of necrosis (AN) in the AAR (AN/AAR) were calculated by Image-Pro Plus 6.0 (Media Cybernetics, Inc., Rockville, MD, United States).
The Morphology and Ultrastructure of the Myocardium
After fixation with 10% formalin solution, the ischemic myocardial tissue was routinely dehydrated and paraffin-embedded. Five microns-thick sections of the ischemic area were obtained (the slices are perpendicular to the long axis of the ventricle). The slices were stained with Harris alum hematoxylin staining solution and the eosin staining solution. Muscle fibers and stromal edema in ischemic myocardial tissue were observed by Olympus optical microscope (BH-2, Tokyo, Japan).
The ischemic myocardial tissue was fixed by 2.5% glutaric dialdehyde and stained by uranyl acetate and lead hydrochloride. Ultrastructural changes of mitochondria, myofibrils, and cell nuclei in cardiomyocytes were observed by Hitachi H-7650 transmission electron microscopy.
Mitochondrial Protein Extraction and Western Blotting Detection
The ventricular part below the ligation in the rat myocardium was examined, washed repeatedly with phosphate buffer saline (PBS), and then crushed in mitochondria isolation buffer (MIB; 210 mM mannitol, 70 mM sucrose, 5 mM Tris-HCl, 1 mM EDTA, pH 7.4). Centrifugation was conducted at 600 × g for 10 min at 4°C, and the supernatant was retained. The process was repeated three times. Then the supernatant was centrifuged at 12,000 × g for 10 min at 4°C to precipitate the mitochondria. According to the weight of myocardial mitochondria, lysates (9 mM urea, 2% CHAPS, 65 mM DTT, 1 mM PMSF, 0.5% IPG buffer) of about 4 times the volume were added and suspended. The protein sample solution was quantified at a wavelength of 562 nm, following the instructions of the BCA protein assay kit.
Western blotting was used to determine changes in the expression levels of mitochondrial fission-related proteins DRP1 and FIS1 and mitochondrial fusion-related proteins OPA1 and MFN1. Briefly, after quantification, protein samples (5 μg) were separated by SDS-PAGE electrophoresis, then transferred to PVDF, sealed with 5% skim milk, and incubated with the primary antibody at 4°C overnight, followed by incubation with secondary antibodies at room temperature for 1 h. Images were then acquired using the chemiluminescent darkroom development technique. Quantification was done by densitometry of Western Blot bands using Image J software (National Institutes of Health: Bethesda, MA, United States).
Statistical Analysis
All data were expressed as mean ± standard deviation, and SPSS software (Version 16.0, IBM Corp., Armonk, NY, United States) was used for statistical analysis. One-way analysis of variance was used to assess differences between groups. A Homogeneity of variance test was also performed, and data sets with homogeneity of variance were then submitted to the least-square deconvolution method for pairwise comparison between groups. A P-value < 0.05 was statistically significant.
Results
Myocardial Injury and Renal Function Markers
Compared with the sham group, the myocardial injury markers, cTnT and CK-MB, were significantly increased in the I/R group rats (cTnT: 25.69 ± 3.83 U/L vs. 44.73 ± 9.16 U/L, P = 0.0000; CK-MB: 280.13 ± 79.98 pg/mL vs. 727.16 ± 162.68 pg/mL, P = 0.0000), but there was no significant difference between the sham and CRF groups (cTnT: 28.45 ± 7.87 U/L, P = 0.4591; CK-MB: 316.81 ± 88.15 pg/mL, P = 0.5900). Compared with the I/R group, the myocardial injury markers in the CRF + I/R group were significantly increased (cTnT: 51.76 ± 10.2 U/L, P = 0.0382; CK-MB: 860.75 ± 172.3 pg/mL, P = 0.0362). Compared with the CRF + I/R group, IPOC significantly reduced the expression of myocardial injury markers in the comorbid group rats (cTnT: 36.15 ± 9.90 U/L, P = 0.0000; CK-MB: 564.20 ± 172.34 pg/mL, P = 0.0000). Importantly, IPOC combined with GXN significantly reduced the expression of myocardial injury markers in the comorbid group rats (cTnT: 32.24 ± 6.96 U/L, P = 0.0000; CK-MB: 426.18 ± 108.21 pg/mL, P = 0.0000). The expression of CK-MB in the IPOC + GXN group was significantly lower than that in the IPOC group (P = 0.0312). Moreover, creatinine and urea levels in the IPOC and IPOC + CM groups were lower than that in the CRF + I/R group, but the difference was not statistically significant (Figure 3 and Supplementary Table 1).
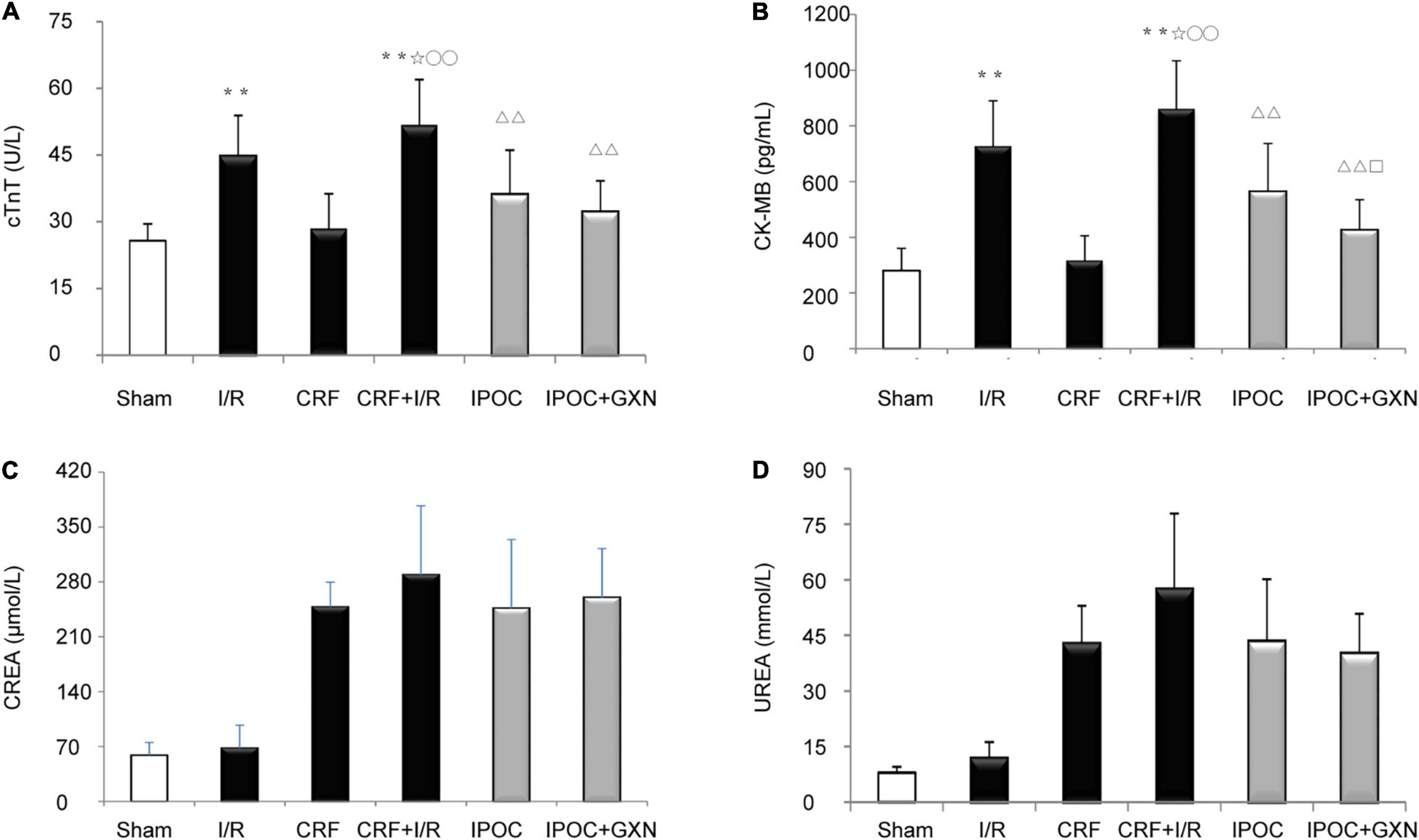
Figure 3. Myocardial injury markers and renal function. (A) cTNT; (B) CK-MB; (C) CREA; (D) UREA. Compared with Sham group, **P < 0.01. Compared with the I/R group, ✩P < 0.05. Compared with CRF group, ○○P < 0.01. Compared with CRF+IR group, ΔΔP < 0.01. Compared with IPOC group, □P < 0.05.
Myocardial Ischemiac and Infarcted Area
The myocardial AN/AAR in the I/R group was significantly higher than that in the sham group (0.30 ± 0.08, P = 0.0000). Compared with the I/R group, the AN/AAR of the myocardium in the CRF + I/R group was significantly increased (0.39 ± 0.07, P = 0.0175). However, compared with the CRF + I/R group, IPOC could significantly reduce the AN/AAR in the comorbidity model rats (0.29 ± 0.07, P = 0.0051). IPOC combined with GXN could also significantly reduce the myocardial AN/AAR of the CRF + I/R group rats (0.17 ± 0.09, P = 0.0000). Finally, compared with the IPOC group, the myocardial AN/AAR in the IPOC + CM group was significantly decreased (P = 0.0038). AAR/LV was not statistically significant between groups (Figure 4 and Supplementary Table 2).
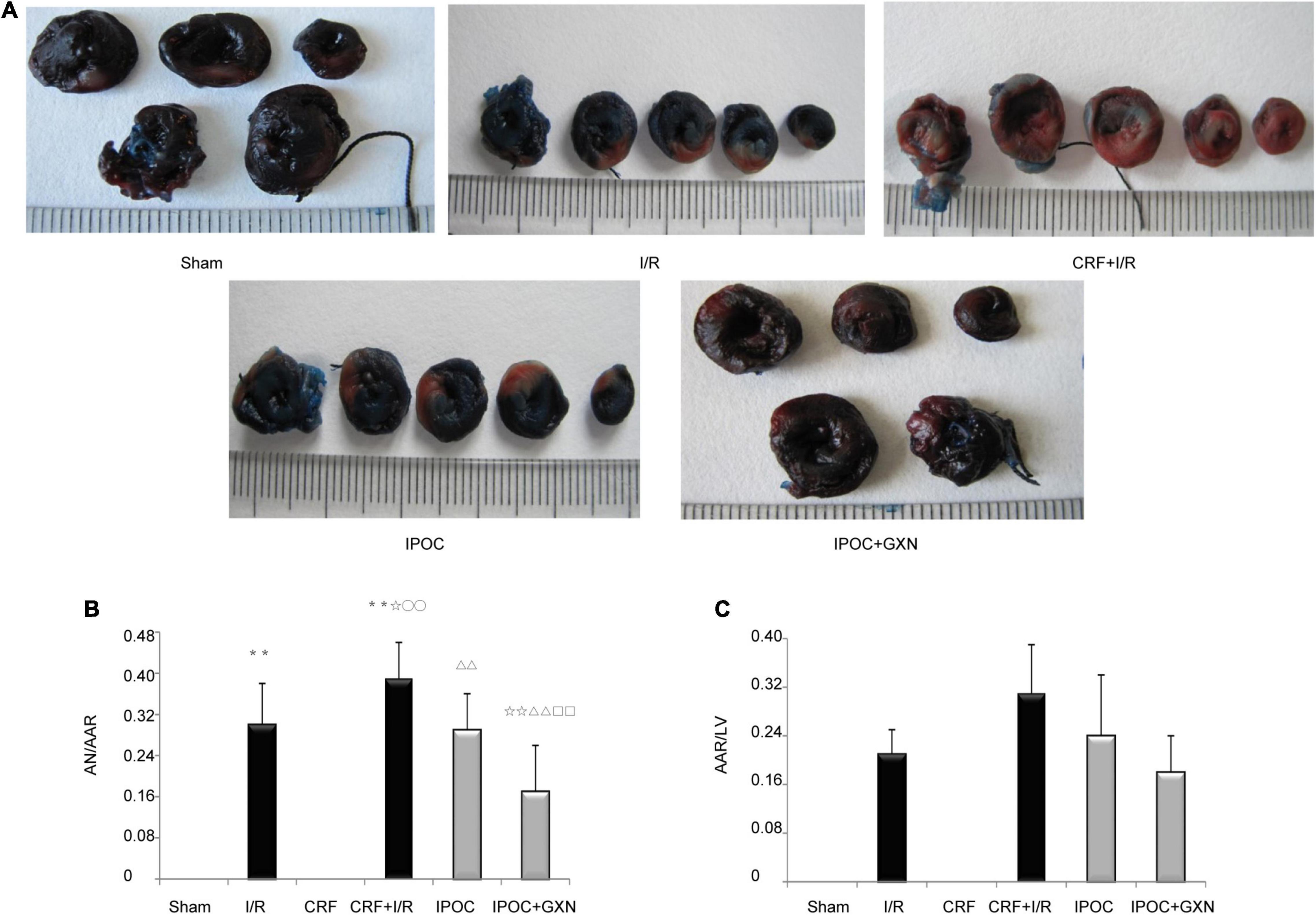
Figure 4. Myocardial ischemia and infarct area. (A) Myocardial infarction area assessed by Evans blue and Triphenyl tetrazolium chloride staining; (B) AN/AAR; (C) AAR/LV. Compared with Sham group, **P < 0.01. Compared with the I/R group, ✩P < 0.05, ✩✩P < 0.01. Compared with CRF group, ○○P < 0.01. Compared with CRF+IR group, ΔΔP < 0.01. Compared with IPOC group, □□P < 0.01.
Morphology and Ultrastructure of the Myocardium
Clear muscle fibers with regular myocardial cells and spindle-shaped nuclei were visible in the myocardial tissue of rats in the sham group with no edema or hemorrhage in the stroma. Intercellular edema was observed in myocardial tissue of the CRF group, with clear muscle fibers and myocardial cells of regular morphology. However, in the I/R group, muscle fibers were ruptured and dissolved, with significant intercellular interstitial edema, the myocardial nucleus was constricted, fragmented, or dissolved, and a large number of infiltrated inflammatory cells could be observed. Similarly, significant intercellular interstitial edema was visible in the CRF + I/R group, and the degree of muscle fiber rupture and dissolution was more obvious than in the I/R group. In the IPOC group, there were slightly swollen myocardial fibers and inflammatory cell infiltration of the stroma, which was mild compared to the CRF + I/R group. The arrangement of myocardial fibers in the IPOC + GXN group was regular, intercellular edema was milder than in the IPOC group, and the inflammatory cell infiltration was significantly alleviated (Figure 5A).
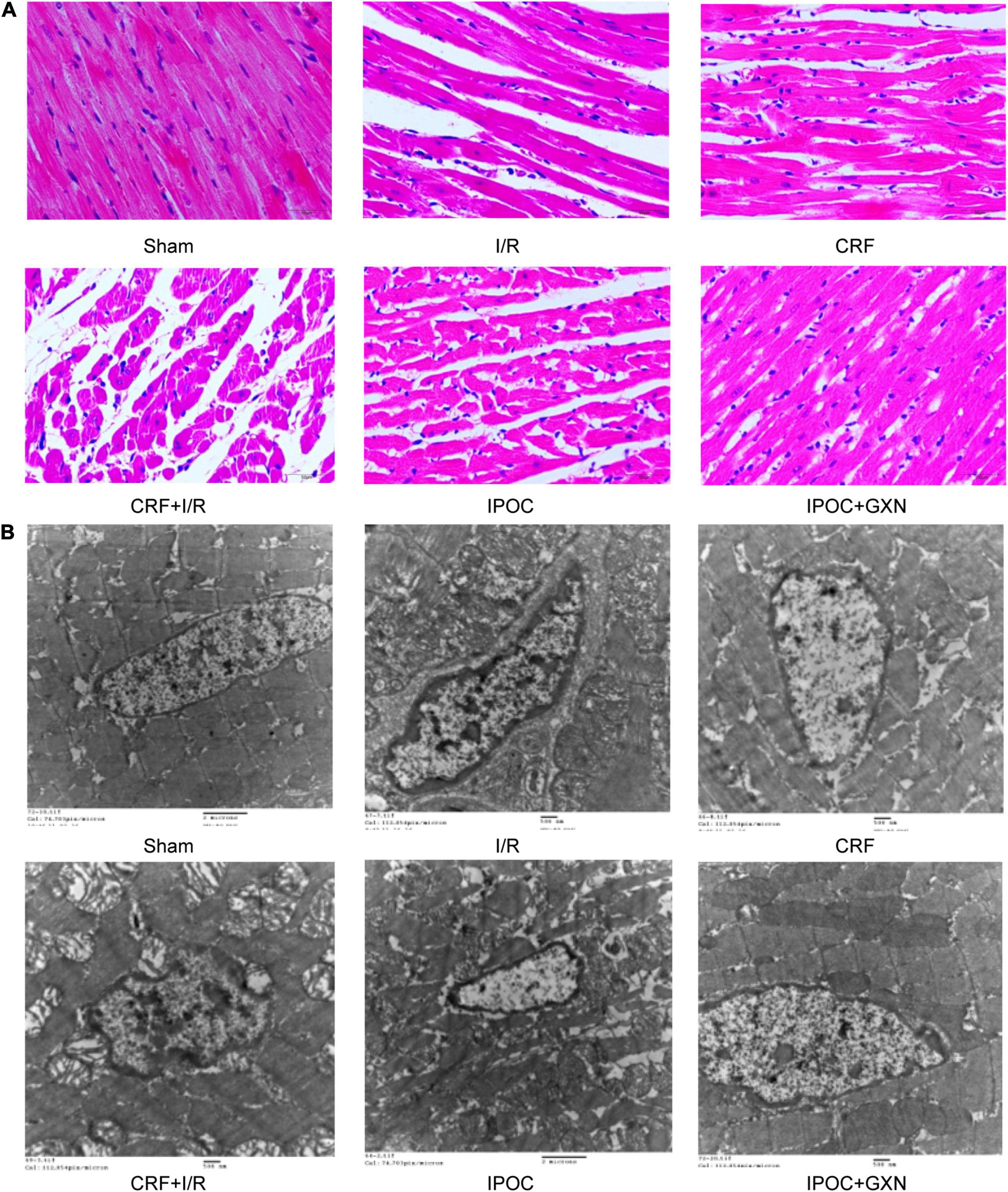
Figure 5. Morphology and ultrastructure of the myocardium. (A) HE staining; (B) Electron microscopical images of mitochondria.
The myocardial structure in the sham and CRF groups was intact. However, in the I/R group, the myocardial structure was significantly damaged, and mitochondria exhibited lattice-shaped inclusion body formation and cristae rupture, the cellular nucleus was deformed, the nuclear membrane was contracted, the nuclear chromatin was condensed with marginal aggregation. Similarly, the myocardial structure was significantly damaged in the CRF + I/R group. However, in the IPOC group, the nucleus of the myocardium was evenly distributed, with mild edema in myocardial cells, slightly swollen mitochondria, reduced number of mitochondrial cristae, and nuclear chromatin condensation. However, no significant karyopyknosis was observed. In the IPOC + GXN group, myofibrils were arranged regularly, with no edema in the cardiomyocytes. The mitochondria exhibited an integral structure with no swelling and vesicular degeneration. Moreover, mitochondrial fusion was observed (Figure 5B).
Mitochondrial Dynamics Protein
Compared with the sham group, the myocardial DRP1 protein levels in the I/R and CRF groups increased significantly (0.47 ± 0.18 vs. 1.11 ± 0.37, P = 0.0045; 0.47 ± 0.18 vs. 1.07 ± 0.46, P = 0.0071). Compared with the I/R groups, the myocardial DRP1 protein levels in the CRF + I/R group increased significantly (1.56 ± 0.50, P = 0.0388). Compared with the CRF + I/R group, both IPOC and IPOC combined with GXN could reduce the DRP1 expression in the comorbid model rats (0.83 ± 0.33, P = 0.0014; 0.49 ± 0.14, P = 0.0003). There was no significant difference in the myocardial FIS1 protein levels (Figures 6A,B).
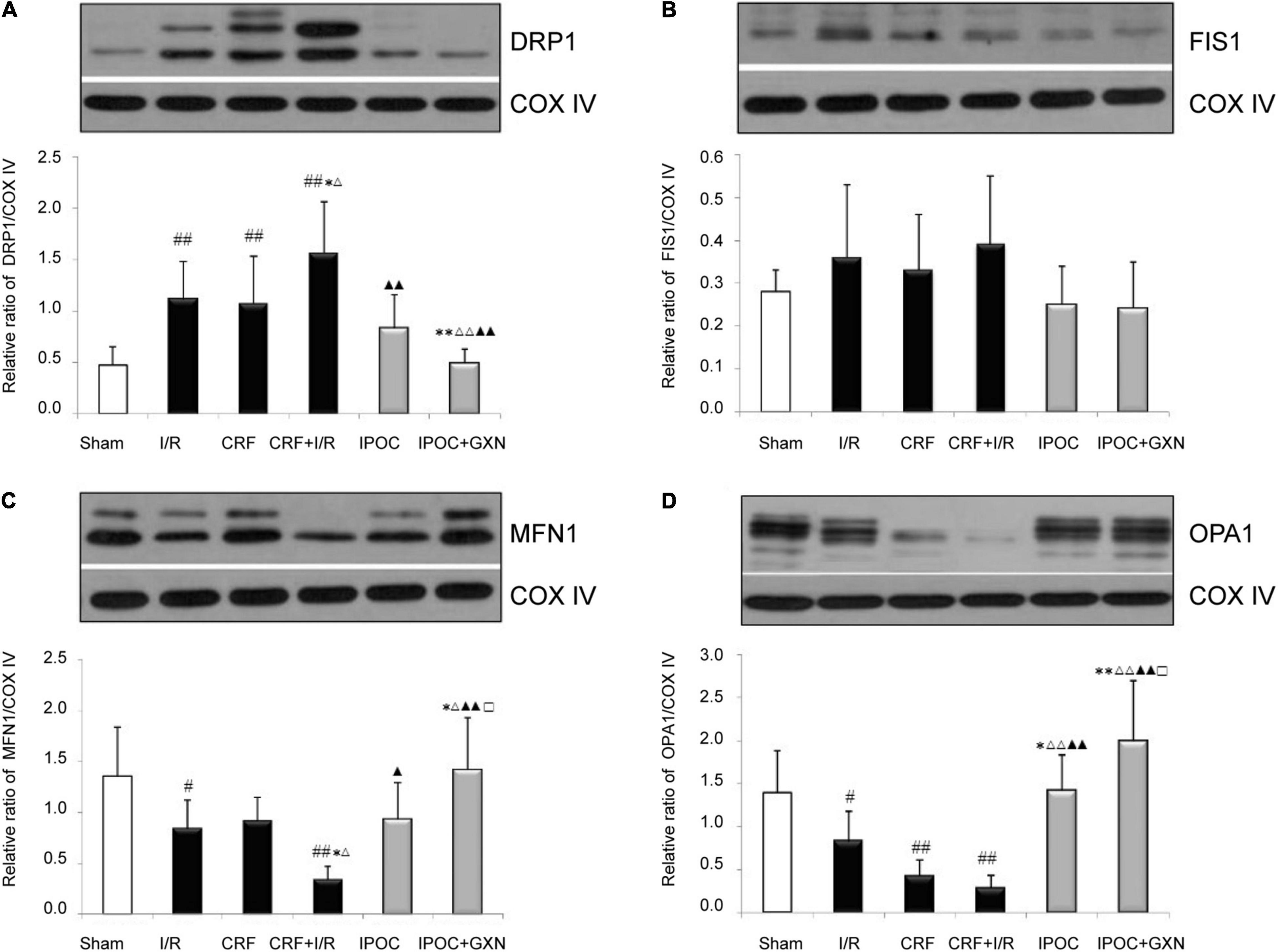
Figure 6. Mitochondrial dynamics protein. (A) DRP1; (B) FIS1; (C) MFN1; (D) OPA1. Compared with SHAM group, #P < 0.05, ##P < 0.01. Compared with the I/R group, *P < 0.05, **P < 0.01. Compared with CRF group, ΔP < 0.05, ΔΔ P < 0.01. Compared with CRF + I/R group, ▲P < 0.05, ▲▲P < 0.01. Compared with IPoC group, □P < 0.05.
Compared with the sham group, the myocardial MFN1 and OPA1 protein levels in the I/R group were significantly decreased (1.36 ± 0.48 vs. 0.84 ± 0.28, P = 0.0285; 1.39 ± 0.49 vs. 0.82 ± 0.36, P = 0.0452). Moreover, the myocardial MFN1 protein level in the CRF + I/R group were significantly lower than in the I/R group (0.34 ± 0.13, P = 0.0356), the OPA1 protein level in the CRF + I/R was also decreased, but the difference fell short of statistical significance (0.28 ± 0.15, P = 0.0610). IPOC could significantly increase the MFN1 and OPA1 protein levels in the comorbid model rats (0.93 ± 0.36, P = 0.0144; 1.41 ± 0.42, P = 0.0003). Compared with the CRF + I/R group, IPOC combined with GXN could also significantly increase myocardial MFN1 and OPA1 protein levels (1.42 ± 0.51, P = 0.0000; 1.99 ± 0.71, P = 0.0000). Finally, the MFN1 and OPA1 protein levels in the IPOC + GXN group were significantly higher than in the IPOC group (P = 0.0387, P = 0.0403) (Figures 6C,D).
Discussion
Despite the growing use and success rate of coronary reperfusion techniques such as PCI, the mortality rate of AMI patients remains high, especially those with CKD (19). Myocardial I/R is widely acknowledged as a complicated process resulting from reperfusion therapy during myocardial infarction, involving metabolic and immunological variables that can potentially obliterate a significant percentage of myocardial rescue obtained by early PCI in AMI patients (20). As a result, numerous techniques to reduce reperfusion damage have been developed, including ischemic postconditioning (21). TCM has been used to treat ailments with herbal mixtures for thousands of years. Given that DS and CX can promote blood circulation and remove blood stasis, they have been used to treat blood stasis symptoms (primarily cardiovascular illnesses) in China and other Asian countries (22). GXN, which consists of extracts from the two plants mentioned above, has been established to exert remarkable cardio-renal protective effects. Danshensu is the main component of DS and reportedly alleviates cardiac I/R injury by inhibiting autophagy and apoptosis via activation of mammalian target of rapamycin (mTOR) signaling pathway (23). Moreover, salvianolic acid A decreases the inflammatory response in CKD and lowers platelet activation and inflammation to prevent myocardial I/R damage (24, 25). The present study found that the myocardial infarction area and myocardial injury indicators were higher in CRF rats, consistent with the literature. The IPOC intervention significantly reduced myocardial damage indicators and infarction area, and the degree of improvement was more substantial when combined with GXN, which may be attributed to enhanced mitochondrial dynamics. Although no changes in CREA and UREA were found in this study, another study revealed that GXN could reduce the elevation of blood UREA and serum cystatin C induced by transverse aortic constriction and alleviate renal pathological injury. Moreover, the metabolic mechanism of GXN in the treatment of HF with CRF by urinary metabolomics analysis showed that the cardiorenal protective mechanism of GXN was mainly related to energy metabolism and oxidative stress (26).
Mitochondrial dynamics refers to the dynamic balance between fusion and division of mitochondria. It is well-established that mitochondrial division increases, whereas fusion decreases during the I/R injury process, leading to a mitochondrial division-fusion imbalance (27). Mitochondrial fusion can reduce the stress response by combining the contents of normal mitochondria with those of partly damaged mitochondria. In contrast, mitochondrial division is required to generate new mitochondria and can be used to separate damaged mitochondria and maintain mitochondrial network quality (28). Mitochondrial fission protein DRP1 located in the cytoplasm is one of the main proteins that mediate mitochondrial division. Interestingly, DRP1 is shifted to the outside of the mitochondrial membrane during mitochondrial division and combines with the potential locus of division in mitochondria, leading to hydrolysis mitochondrial outer membrane mediated by guanosine triphosphate and promoting compression and separation of mitochondria, eventually producing two independent mitochondria (29). It is well-recognized that DRP1-dependent mitochondrial fission plays an essential role in cardiovascular disease (30). In clinically relevant large animal models of AMI, targeted inhibition of DRP1 at the onset of reperfusion was found to induce cardioprotective effects (31). It should be borne in mind that other stimuli are required to recruit DRP1 to mitochondria since it lacks a mitochondrial targeting sequence. FIS1 is one of the protein candidates implicated in the recruitment of DRP1 to the mitochondria, and its structure is similar to mitochondrial import proteins (32). Notwithstanding that FIS1 is required for mitochondrial division, studies have demonstrated that altered FIS1 expression levels do not affect DRP1 recruitment during mitochondrial division (33). Importantly, FIS1 revolves around the outer surface of the mitochondria and is not targeted at the mitochondrial future division site. Moreover, its downregulation can strongly inhibit apoptosis through multiple pathways, with a much greater degree of downregulation than DRP1 (34). Inner mitochondrial membrane (IMM) fusion and outer mitochondrial membrane (OMM) fusion represent two stages of mitochondrial fusion. It has been shown that MFN1 and MFN2 on nearby OMM mediate OMM fusion. OPA1 mediates the fusion of IMM after fusion with the outer membrane, and the mitochondrial matrix fusion generates new mitochondria (35). There is ample evidence substantiating that MFN1 and MFN2 are two MFN isoforms expressed in separate organs. MFN2 is reportedly the most abundant protein in the brain, whereas MFN1 is highly expressed in the heart (36). MFN is involved in the anchoring of apposing mitochondria prior to membrane fusion in mitochondrial fusion. In studies with differently labeled mitochondria, MFN1 tethering between molecules was shown to be more successful than MFN2 tethering in the research (37). In addition, studies have shown that OPA1 cannot promote mitochondrial fusion in MFN1-deficient cells. Similarly, MFN1 cannot promote mitochondrial elongation if OPA1 is ablated. Thus, OPA1 and MFN1 appear to be functionally dependent on each other (38, 39). Besides, substantial evidence suggests that during I/R, OPA1 and MFN protein expression are inhibited, leading to mitochondrial permeability transition pore (MPTP) opening and mitochondrial membrane permeability (MMP) phenomena, inducing apoptosis (40).
Herein, we found that the mitochondrial fission-related protein DRP1 was significantly increased in myocardial I/R injury in CRF rats. DRP1 expression was significantly decreased after treatment with IPOC. Although GXN combined with IPOC yielded a decreasing effect, the difference was not statistically significant. Meanwhile, no significant differences in FIS1 protein expression were found. Moreover, we found no significant correlation between the myocardial function of GXN combined with IPOC intervention in CRF rats after I/R injury and mitochondrial fission-related proteins DRP1 and FIS1. In addition, the myocardial mitochondrial division was significantly increased in CRF rats with I/R injury, the mitochondrial cristae were ruptured, and the expression of the mitochondrial fusion-related proteins OPA1 and MFN1 was significantly decreased. After treatment with GXN combined with IPOC, the expression of OPA1 and MFN1 proteins increased significantly, and myocardial mitochondria fusion with an integral structure was observed under a transmission electron microscope. These results provided compelling evidence that increased OPA1 and MFN1 protein expression was closely related to the protective effect induced by GXN combined with IPOC against myocardial I/R injury in uremic rats.
Limitations
In this study, we used animals of both sexes to represent the entire population, but we did not evaluate the results for sex differences. The experimental procedures are based on relevant studies, but the short reperfusion time may affect the TTC staining results (41–43). The modeling surgery was done by one skilled researcher, but there is a high difference among the AN/AAR data, which could be related to individual variances. Indeed, this research is still preliminary and exploratory, and it does not explore the upstream signaling pathways of mitochondrial dynamics-related proteins. In the follow-up study, we will pay special attention to these issues and conduct further research.
Conclusion
In this study, based on the observation of gross pathology, myocardial injury markers, and myocardial ultrastructure, we corroborated that Huoxue Chinese medicine combined with IPOC could significantly improve myocardial I/R injury in CRF rats, and the mechanism was associated to mitochondrial fusion-related proteins.
Data Availability Statement
The original contributions presented in the study are included in the article/Supplementary Material, further inquiries can be directed to the corresponding authors.
Ethics Statement
The animal study was reviewed and approved by Ethics Committee of Xiyuan Hospital.
Author Contributions
AW: formal analysis, investigation, data curation, writing—original draft, and visualization. DZ and JL: conceptualization, methodology, and writing—review and editing. HYa: investigation, formal analysis, and data curation. PZ: validation, investigation, and data curation. HYu: resources. XM: supervision and project administration.
Funding
This study was supported by grants from the National Natural Science Foundation of China (Nos. 81303128 and 81173384).
Conflict of Interest
The authors declare that the research was conducted in the absence of any commercial or financial relationships that could be construed as a potential conflict of interest.
Publisher’s Note
All claims expressed in this article are solely those of the authors and do not necessarily represent those of their affiliated organizations, or those of the publisher, the editors and the reviewers. Any product that may be evaluated in this article, or claim that may be made by its manufacturer, is not guaranteed or endorsed by the publisher.
Acknowledgments
We would like to acknowledge the Home for Researchers editorial team (www.home-for-researchers.com) for their language editing service.
Supplementary Material
The Supplementary Material for this article can be found online at: https://www.frontiersin.org/articles/10.3389/fcvm.2022.905254/full#supplementary-material
Abbreviations
AAR, area at risk; AMI, acute myocardial infarction; AN, area of necrosis; CKD, chronic kidney disease; CK-MB, creatine kinase-MB; CREA, Creatinine; CRF, chronic renal failure; cTnT, cardiac troponin T; CX, Ligusticum chuanxiong Hort.; DRP1, dynamin-related protein-1; DS, Salvia miltiorrhiza Bge.; FIS1, mitochondrial fission protein 1; GXN, Guanxinning injection; HF, heart failure; I/R, ischemia-reperfusion; IMM, inner mitochondrial membrane; IPOC, ischemic postconditioning; LV, left ventricular; MFN, mitofusin; MIB, mitochondria isolation buffer; MMP, mitochondrial membrane permeability; MPTP, mitochondrial permeability transition pore; mTOR, mammalian target of rapamycin; OMM, outer mitochondrial membrane; OPA1, ocular optic atrophy 1; PBS, phosphate buffer saline; PCI, percutaneous coronary intervention; SFDA, The State Food and Drug Administration; TTC, triphenyltetrazolium chloride solution; UREA, urea nitrogen.
References
1. Kalantar-Zadeh K, Jafar TH, Nitsch D, Neuen BL, Perkovic V. Chronic kidney disease. Lancet. (2021) 398:786–802. doi: 10.1016/S0140-6736(21)00519-5
2. Bugger H, Pfeil K. Mitochondrial ROS in myocardial ischemia reperfusion and remodeling. Biochim Biophys Acta Mol Basis Dis. (2020) 1866:165768. doi: 10.1016/j.bbadis.2020.165768
3. Jankowski J, Floege J, Fliser D, Bohm M, Marx N. Cardiovascular disease in chronic kidney disease: pathophysiological insights and therapeutic options. Circulation. (2021) 143:1157–72. doi: 10.1161/CIRCULATIONAHA.120.050686
4. Vinnakota S, Scott CG, Rodeheffer RJ, Chen HH. Estimated glomerular filtration rate, activation of cardiac biomarkers and long-term cardiovascular outcomes: a population-based cohort. Mayo Clin Proc. (2019) 94:2189–98. doi: 10.1016/j.mayocp.2019.03.033
5. Yandrapalli S, Christy J, Malik A, Wats K, Harikrishnan P, Aronow W, et al. Impact of acute and chronic kidney disease on heart failure hospitalizations after acute myocardial infarction. Am J Cardiol. (2022) 165:1–11. doi: 10.1016/j.amjcard.2021.10.041
6. Hashimoto Y, Ozaki Y, Kan S, Nakao K, Kimura K, Ako J, et al. Impact of chronic kidney disease on in-hospital and 3-year clinical outcomes in patients with acute myocardial infarction treated by contemporary percutaneous coronary intervention and optimal medical therapy- insights from the J-MINUET study. Circ J. (2021) 85:1710–8. doi: 10.1253/circj.CJ-20-1115
7. Zhao ZQ, Corvera JS, Halkos ME, Kerendi F, Wang NP, Guyton RA, et al. Inhibition of myocardial injury by ischemic postconditioning during reperfusion: comparison with ischemic preconditioning. Am J Physiol Heart Circ Physiol. (2003) 285:H579–88. doi: 10.1152/ajpheart.01064.2002
8. Araszkiewicz A, Grygier M, Pyda M, Rajewska J, Michalak M, Lesiak M, et al. Postconditioning reduces enzymatic infarct size and improves microvascular reperfusion in patients with ST-segment elevation myocardial infarction. Cardiology. (2014) 129:250–7. doi: 10.1159/000367965
9. Safaei N, Sheikhalizadeh MA, Badalzadeh R. Effect of ischemic postconditioning on myocardial protection in patients undergoing coronary artery bypass grafting surgery with cardiopulmonary bypass. J Cardiovasc Thorac Res. (2016) 8:65–71. doi: 10.15171/jcvtr.2016.13
10. Byrne CJ, McCafferty K, Kieswich J, Harwood S, Andrikopoulos P, Raftery M, et al. Ischemic conditioning protects the uremic heart in a rodent model of myocardial infarction. Circulation. (2012) 125:1256–65. doi: 10.1161/CIRCULATIONAHA.111.055392
11. Zhang DW, Zhang L, Liu JG. Effects of xiongshao capsule combined with ischemic postconditioning on monocyte chemoattractant protein-1 and tumor necrosis factor-alpha in rat myocardium with ischemic reperfusion injury. Zhongguo Zhong Xi Yi Jie He Za Zhi. (2010) 30:1279–83.
12. Wang SL, Wang CL, Wang PL, Xu H, Liu HY, Du JP, et al. Combination of Chinese herbal medicines and conventional treatment versus conventional treatment alone in patients with acute coronary syndrome after percutaneous coronary intervention (5C trial): an open-label randomized controlled, multicenter study. Evid Based Complement Alternat Med. (2013) 2013:741518. doi: 10.1155/2013/741518
13. Zhang DW, Wang SL, Wang PL, Du JP, Gao ZY, Wang CL, et al. The efficacy of Chinese herbal medicines on acute coronary syndrome with renal insufficiency after percutaneous coronary intervention. J Ethnopharmacol. (2020) 248:112354. doi: 10.1016/j.jep.2019.112354
14. Zhang DW, Liu JG, Feng JT, Zhang L, Yang XP, Shi DZ, et al. Effects of effective components compatibility of aqueous extracts of Salviae Miltiorrhizae and Rhizoma Chuanxiong on rat myocardial ischemia/reperfusion injury. Zhongguo Wei Zhong Bing Ji Jiu Yi Xue. (2010) 22:109–12.
15. Bigelman E, Cohen L, Aharon-Hananel G, Levy R, Rozenbaum Z, Saada A, et al. Pathological presentation of cardiac mitochondria in a rat model for chronic kidney disease. PLoS One. (2018) 13:e0198196. doi: 10.1371/journal.pone.0198196
16. Bock FJ, Tait SWG. Mitochondria as multifaceted regulators of cell death. Nat Rev Mol Cell Biol. (2020) 21:85–100. doi: 10.1038/s41580-019-0173-8
17. Ruan M, Li Y, Li X, Luo J, Kong L. Qualitative and quantitative analysis of the major constituents in Chinese medicinal preparation Guan-Xin-Ning injection by HPLC-DAD-ESI-MS(n). J Pharm Biomed Anal. (2012) 59:184–9. doi: 10.1016/j.jpba.2011.10.007
18. Xu L, Chang R, Chen M, Li L, Huang Y, Zhang H, et al. Quality evaluation of Guan-Xin-Ning injection based on fingerprint analysis and simultaneous separation and determination of seven bioactive constituents by capillary electrophoresis. Electrophoresis. (2017) 38:3168–76. doi: 10.1002/elps.201700256
19. Edfors R, Lindhagen L, Spaak J, Evans M, Andell P, Baron T, et al. Use of proteomics to identify biomarkers associated with chronic kidney disease and long-term outcomes in patients with myocardial infarction. J Intern Med. (2020) 288:581–92. doi: 10.1111/joim.13116
20. Heusch G. Myocardial ischaemia-reperfusion injury and cardioprotection in perspective. Nat Rev Cardiol. (2020) 17:773–89. doi: 10.1038/s41569-020-0403-y
21. Heusch G, Gersh BJ. The pathophysiology of acute myocardial infarction and strategies of protection beyond reperfusion: a continual challenge. Eur Heart J. (2017) 38:774–84. doi: 10.1093/eurheartj/ehw224
22. Gao ZY, Xu H, Shi DZ, Wen C, Liu BY. Analysis on outcome of 5284 patients with coronary artery disease: the role of integrative medicine. J Ethnopharmacol. (2012) 141:578–83. doi: 10.1016/j.jep.2011.08.071
23. Fan G, Yu J, Asare PF, Wang L, Zhang H, Zhang B, et al. Danshensu alleviates cardiac ischaemia/reperfusion injury by inhibiting autophagy and apoptosis via activation of mTOR signalling. J Cell Mol Med. (2016) 20:1908–19. doi: 10.1111/jcmm.12883
24. Yuan X, Xiang Y, Zhu N, Zhao X, Ye S, Zhong P, et al. Salvianolic acid A protects against myocardial ischemia/reperfusion injury by reducing platelet activation and inflammation. Exp Ther Med. (2017) 14:961–6. doi: 10.3892/etm.2017.4619
25. Zhang HF, Wang YL, Gao C, Gu YT, Huang J, Wang JH, et al. Salvianolic acid A attenuates kidney injury and inflammation by inhibiting NF-kappaB and p38 MAPK signaling pathways in 5/6 nephrectomized rats. Acta Pharmacol Sin. (2018) 39:1855–64. doi: 10.1038/s41401-018-0026-6
26. Wang CL, Li ZH, Liao Q, Zhou J, Wang SX, Xiao CN, et al. Urinary metabolomics analysis to reveal metabolic mechanism of Guanxinning injection on heart failure with renal dysfunction. J Pharm Biomed Anal. (2022) 209:114516. doi: 10.1016/j.jpba.2021.114516
27. Maneechote C, Palee S, Kerdphoo S, Jaiwongkam T, Chattipakorn SC, Chattipakorn N. Balancing mitochondrial dynamics via increasing mitochondrial fusion attenuates infarct size and left ventricular dysfunction in rats with cardiac ischemia/reperfusion injury. Clin Sci (Lond). (2019) 133:497–513. doi: 10.1042/CS20190014
28. Altieri DC. Mitochondrial dynamics and metastasis. Cell Mol Life Sci. (2019) 76:827–35. doi: 10.1007/s00018-018-2961-2
29. Rosdah AA, Smiles WJ, Oakhill JS, Scott JW, Langendorf CG, Delbridge LMD, et al. New perspectives on the role of Drp1 isoforms in regulating mitochondrial pathophysiology. Pharmacol Ther. (2020) 213:107594. doi: 10.1016/j.pharmthera.2020.107594
30. Jin JY, Wei XX, Zhi XL, Wang XH, Meng D. Drp1-dependent mitochondrial fission in cardiovascular disease. Acta Pharmacol Sin. (2021) 42:655–64. doi: 10.1038/s41401-020-00518-y
31. Ong SB, Kwek XY, Katwadi K, Hernandez-Resendiz S, Crespo-Avilan GE, Ismail NI, et al. Targeting mitochondrial fission using Mdivi-1 in a clinically relevant large animal model of acute myocardial infarction: a pilot study. Int J Mol Sci. (2019) 20:3972. doi: 10.3390/ijms20163972
32. Hoppins S, Lackner L, Nunnari J. The machines that divide and fuse mitochondria. Annu Rev Biochem. (2007) 76:751–80. doi: 10.1146/annurev.biochem.76.071905.090048
33. Yu R, Jin SB, Lendahl U, Nister M, Zhao J. Human Fis1 regulates mitochondrial dynamics through inhibition of the fusion machinery. EMBO J. (2019) 38:e99748. doi: 10.15252/embj.201899748
34. Kleele T, Rey T, Winter J, Zaganelli S, Mahecic D, Perreten Lambert H, et al. Distinct fission signatures predict mitochondrial degradation or biogenesis. Nature. (2021) 593:435–9. doi: 10.1038/s41586-021-03510-6
35. Adaniya SM, O-Uchi J, Cypress MW, Kusakari Y, Jhun BS. Posttranslational modifications of mitochondrial fission and fusion proteins in cardiac physiology and pathophysiology. Am J Physiol Cell Physiol. (2019) 316:C583–604. doi: 10.1152/ajpcell.00523.2018
36. Eura Y, Ishihara N, Yokota S, Mihara K. Two mitofusin proteins, mammalian homologues of FZO, with distinct functions are both required for mitochondrial fusion. J Biochem. (2003) 134:333–44. doi: 10.1093/jb/mvg150
37. Ishihara N, Eura Y, Mihara K. Mitofusin 1 and 2 play distinct roles in mitochondrial fusion reactions via GTPase activity. J Cell Sci. (2004) 117:6535–46. doi: 10.1242/jcs.01565
38. Cipolat S, Martins de Brito O, Dal Zilio B, Scorrano L. OPA1 requires mitofusin 1 to promote mitochondrial fusion. Proc Natl Acad Sci U S A. (2004) 101:15927–32. doi: 10.1073/pnas.0407043101
39. Song Z, Ghochani M, McCaffery JM, Frey TG, Chan DC. Mitofusins and OPA1 mediate sequential steps in mitochondrial membrane fusion. Mol Biol Cell. (2009) 20:3525–32. doi: 10.1091/mbc.E09-03-0252
40. Xue RQ, Sun L, Yu XJ, Li DL, Zang WJ. Vagal nerve stimulation improves mitochondrial dynamics via an M3 receptor/CaMKKbeta/AMPK pathway in isoproterenol-induced myocardial ischaemia. J Cell Mol Med. (2017) 21:58–71. doi: 10.1111/jcmm.12938
41. Dong LY, Li S, Zhen YL, Wang YN, Shao X, Luo ZG. Cardioprotection of vitexin on myocardial ischemia/reperfusion injury in rat via regulating inflammatory cytokines and MAPK pathway. Am J Chin Med. (2013) 41:1251–66. doi: 10.1142/S0192415X13500845
42. Gray CB, Suetomi T, Xiang S, Mishra S, Blackwood EA, Glembotski CC, et al. CaMKIIδ subtypes differentially regulate infarct formation following ex vivo myocardial ischemia/reperfusion through NF-κB and TNF-α. J Mol Cell Cardiol. (2017) 103:48–55. doi: 10.1016/j.yjmcc.2017.01.002
Keywords: ischemia-reperfusion injury, chronic renal failure, extracts of Salvia miltiorrhiza Bge. (Danshen) and Ligusticum chuanxiong Hort. (Chuanxiong), Guanxinning injection, mitochondrial dynamics
Citation: Wang A, Zhang D, Liu J, Yan H, Zhang P, Yuan H and Ma X (2022) Guanxinning Injection Combined With Ischemic Postconditioning Attenuate Myocardial Ischemic Reperfusion Injury in Chronic Renal Failure Rats by Modulating Mitochondrial Dynamics. Front. Cardiovasc. Med. 9:905254. doi: 10.3389/fcvm.2022.905254
Received: 26 March 2022; Accepted: 02 May 2022;
Published: 30 May 2022.
Edited by:
Xiaofeng Yang, Temple University, United StatesReviewed by:
Sandro Percario, Federal University of Pará, BrazilFereshteh Golab, Iran University of Medical Sciences, Iran
Copyright © 2022 Wang, Zhang, Liu, Yan, Zhang, Yuan and Ma. This is an open-access article distributed under the terms of the Creative Commons Attribution License (CC BY). The use, distribution or reproduction in other forums is permitted, provided the original author(s) and the copyright owner(s) are credited and that the original publication in this journal is cited, in accordance with accepted academic practice. No use, distribution or reproduction is permitted which does not comply with these terms.
*Correspondence: Dawu Zhang, ZGF3dTQ0MDNAc2luYS5jb20=; Jiangang Liu, bGl1amlhbmdhbmcyMDAyQHNpbmEuY29t