- 1National Key Discipline, Department of Nutrition and Food Hygiene, School of Public Health, Harbin Medical University, Harbin, China
- 2Department of Pharmacology, College of Pharmacy, Harbin Medical University, Harbin, China
- 3Department of Cardiology, The First Affiliated Hospital of Harbin Medical University, Harbin, China
Background: Acrylamide is a common environmental volatile organic compound that humans are frequently exposed to in their daily lives. However, whether exposure to acrylamide is associated with long-term survival in patients with hyperglycemia remains largely unknown.
Methods and Results: A total of 3,601 hyperglycemic people were recruited in this study, including 1,247 people with diabetes and 2,354 people with pre-diabetes, who enrolled in the National Health and Nutrition Examination survey (2003–2004, 2005–2006, and 2013–2014). The acrylamide exposure was measured by the serum hemoglobin adduct of acrylamide (HbAA) and glycidamide (HbGA), and the ratio of HbAA and HbGA (HbAA/HbGA) was calculated, which were all categorized into quintiles. The National Death Index was used to identify the participants' death information until 2015. Cox proportional hazards (CPHs) regression models were performed to examine the survival relationship between these biomarkers and mortality. During the 28,652 person-year follow-up, 268 deaths due to the cardiovascular disease (CVD) were documented. After adjustment for multiple confounders, compared with participants in the lowest quintile of HbAA/HbGA, the participants in the highest quintile were more likely to die due to CVD (hazard ratio [HR] = 1.61, 95% CI: 1.09–2.39) and all-cause (HR = 1.59, 95% CI: 1.25–2.01). Moreover, subgroup analysis showed that the highest quintile of HbAA/HbGA in the people with diabetes or pre-diabetes was related to mortalities risk of CVD (HRdiabetes = 1.92, 95% CI: 1.11–3.31; HRpre−diabetes = 1.78, 95% CI: 1.01–3.14) and all-cause mortality (HRdiabetes = 1.81, 95% CI: 1.27–2.58; HRpre−diabetes = 1.59, 95% CI: 1.14–2.20). Additionally, no significant association between the levels of HbAA or HbGA and CVD mortality was observed among people with diabetes or pre-diabetes.
Conclusion: Higher levels of HbAA/HbGA are associated with greater mortalities of CVD and all-cause among hyperglycemic people.
Introduction
Acrylamide is an environmentally volatile organic compound, and human beings are frequently exposed to it in their everyday life (1). Acrylamide can be found in many aspects of daily life, such as daily cosmetics, textiles and plastic, cigarettes, water purification, and dietary intakes including various heated foods, beverages, potato crisps, and coffee (2–4). In addition to the neurotoxicity and carcinogenic toxicity of high concentrations of acrylamide, a few in vivo and in vitro studies have found that long-term exposure to low concentrations of acrylamide could induce hepatic oxidative stress and disturb lipid metabolism in rats (5, 6), and could also stimulate the inflammatory response and cell proliferation in HepG2 cells (7). Moreover, recent population-based studies found that serum biomarkers of acrylamide exposure were associated with hyperglycemia and dyslipidemia, prompting the conclusion that daily exposure to acrylamide probably influences the daily glucose control among people with hyperglycemia (8, 9). In addition, an association between acrylamide exposure and a higher risk of cardiovascular disease (CVD) was demonstrated in a population-based study (10). However, it is still largely unknown whether exposure to acrylamide is associated with long-term survival among people with hyperglycemia.
Evidence from animal studies has demonstrated that rats exposed to acrylamide could disrupt glucose homeostasis, induce β-cell dysfunction, and reduce the area of the aortic vessel and acetylcholine to endothelial-dependent arterial diastolic reactions (11, 12). Moreover, in vitro experiments revealed that chronic exposure to acrylamide could induce accelerated endothelial aging, even at low concentrations (13). These mechanisms are highly overlapping with the effect of hyperglycemia on the development of CVD. Based on this evidence, we, therefore, hypothesize that exposure to acrylamide is associated with an increased risk of CVD mortality among people with hyperglycemia. To examine this hypothesis, we examined the association of serum biomarkers of acrylamide with mortalities of CVD and all-cause in the participants with hyperglycemia using the national representative sample from the National Health and Nutrition Examination Survey (NHANES).
Materials and Methods
Study Population
The NHANES is a stratified, multilevel study using a national population sample in the United States, and its detailed information has been provided elsewhere (14). The present study included 3,601 hyperglycemic participants, consisting of 1,247 diabetes and 2,354 pre-diabetes, who were over 18 years old and measured the serum biomarkers of acrylamide. The participants who had missing information on serum acrylamide metabolites, mortality of all-cause, and CVD, and questionnaire were excluded. The NHANES program was approved by the National Health Statistics Research Ethics Committee, and all informed consent was provided by participants.
Definition of Diabetes and Pre-diabetes
According to the American Diabetes Association, diabetes was defined as conforming to at least one of the following criteria: fasting blood glucose (FBG) > 7.0 mmol/L; 2-h postprandial blood glucose > 11.1 mmol/L; HbA1c > 6.5%; or a random plasma glucose > 11.1 mmol/L. Pre-diabetes was determined as meeting at least one of the criteria created by: FBG > 5.6 and <7.0 mmol/L; 2-h postprandial blood glucose > 7.8 and <11.1 mmol/L; or HbA1c > 5.7% and <6.5%.
Measurements of Serum Biomarkers of Acrylamide
The N-terminal hemoglobin adducts of acrylamide (HbAA) and hemoglobin glycidamide (HbGA) in 300 μl of human whole blood or erythrocytes were quantified using high-performance liquid chromatography/tandem mass spectrometry (HPLC/MS/MS) based on a modified Edman reaction (15). The well-established procedure was briefly illustrated as follows: using pentafluorophenyl isothiocyanate as an Edman reagent, two adducts were isolated from the protein chain by modified Edman reaction (16). After the modified EDMAN degradation reaction, liquid–liquid extraction was performed, and the results were analyzed based on HPLC/MS/MS. The calibrator, reagent blank, and quality control materials were pretreated in the same way as the samples. Each sample was performed with two independent repeated measurements. The limits of the detection of HBbaA and HbGA were 3 and 4 pmol/g, respectively.
Main Exposure and Main Outcome
The main exposures in our study were HbAA and HbGA levels in whole blood and the ratio of HbAA and HbGA (HbAA/HbGA). In addition, the outcome was the status of mortality according to the National Death Index (NDI) until the year 2015. The NDI is a reliable death identification resource. The disease-specific death was defined as International Classification of Diseases (ICD)-10 and the death due to CVD was determined as ICD-10 codes (I00-I09, I11, I13, I20-I51, and I60-I69). In summary, a total of 268 deaths (132 in diabetes and 136 in pre-diabetes) due to CVD and 709 deaths (332 in diabetes and 377 in pre-diabetes) due to all-cause were documented during the 28,652 person-year follow-up.
Covariates Assessment
The covariates in our study included age (years), sex (women/men), race/ethnicity (Mexican American/non-Hispanic white/non-Hispanic black/other), smoking (yes or no), drinking status (yes or no), education levels (less than high school education and high school and above), annual household income (more than $100,000/$45,000–$75,000/$20, 000-$45,000/ < $20,000), occupation, body mass index (BMI, kg/m2), energy (kcal), exercise (yes or no), mean blood pressure (mmHg), whether to use insulin hypoglycemic tablets (yes or no), whether to take medication for hypertension (yes or no), and whether to take medication for cholesterol (yes or no).
Statistical Analyses
Demographic and anthropometric characteristics were presented using the mean and standard deviation (SD) for the continuous variables and the number and percentage for categorical variables. The general linear model adjusted for age and sex was used to compare the differences in the biomarkers of acrylamide by different baseline characteristics. All statistical analyses were conducted by R 4.0.2 software, and all tests were two-sided. The value of p < 0.05 was considered statistically significant.
Cox Proportional Hazards (CPH) Models
Cox proportional hazards (CPHs) models were conducted to calculate hazard ratios (HRs) and 95% CI for all-cause and CVD mortalities. The time scale in the Cox model used for the follow-up time was obtained by person-months from the date of the interview to their death, or the end of 2015, which was used for the time scale this Cox model. The HbAA, HbGA, and HbAA/HbGA were respectively categorized into quintiles, and the lowest quintile is regarded as the reference group. The confounders in the CPH model included age, sex, race, smoking status, drinking status, exercise, energy, education level, occupation, annual household income, BMI, mean blood pressure, whether to take medication for diabetes, whether to take medication for hypertension, and whether to take medication for cholesterol.
Results
Participant Characteristics
The differences in the whole blood HbAA, HbGA, and HbAA/HbGA levels according to the baseline characteristics are presented in Table 1. The results of the normality test showed that HbAA, HbGA, and HbAA/HbGA levels were non-normally distributed (Supplementary Figure 1). The participants with higher HbAA levels were younger, men, non-Hispanic blacks, with a lower use rate of medication for glucose and blood pressure, and higher use of medication for cholesterol, and higher smoking rate, drinking, and energy intake (all the p < 0.05). Similarly, the participants with higher HbGA levels were younger, Mexican American, with a lower use rate of medication for blood pressure and higher use of medication for cholesterol, and higher rates of smoking, drinking, and energy intake (all the p < 0.05). Additionally, the participants with obesity, diabetes, or hypertension had lower levels of HbAA, and the participants with hypertension had lower levels of HbGA, respectively (all the p < 0.05). Meanwhile, the participants with higher HbAA/HbGA levels were men, non-Hispanic blacks, with lower medication use for glucose and cholesterol, higher medication use for blood pressure, and higher rates of smoking, drinking, and energy intake. The ratio of HbAA/HbGA was significantly lower in obese, diabetes, and dyslipidemia participants (p < 0.05).
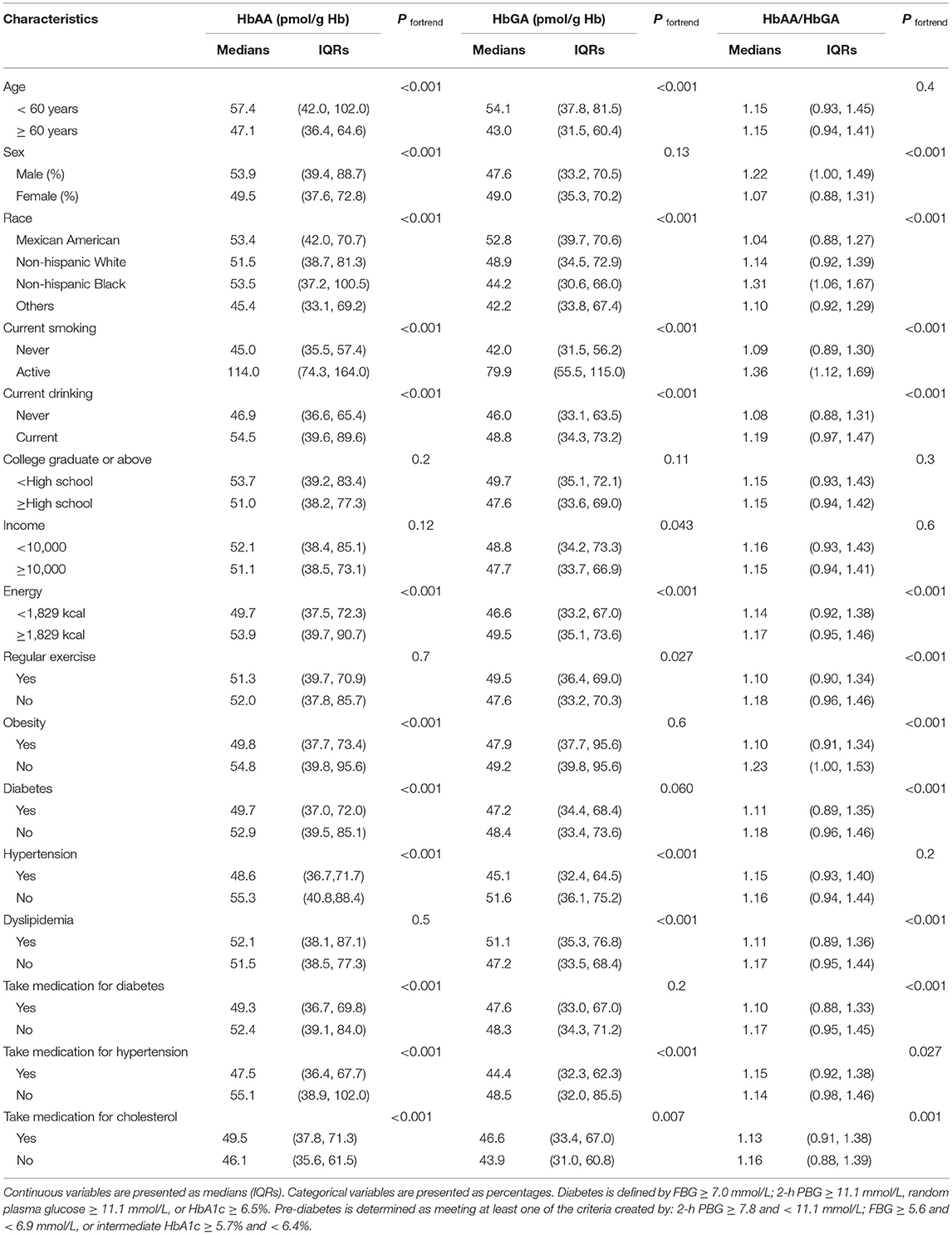
Table 1. The hemoglobin biomarkers of hemoglobin adduct of acrylamide (HbAA), hemoglobin adduct of glycidamide (HbGA), and HbAA/HbGA are grouped by various characteristics of the total hyperglycemia population.
Acrylamide Exposure and Mortality
The relationships of HbAA, HbGA, and HbAA/HbGA in whole blood with CVD and all-cause mortalities among people with hyperglycemia are presented in Table 2. Compared with those in the lowest quintile of HbAA/HbGA, the participants in the highest quintile were more prone to die due to CVD and all-cause, with the HRs (95% CI) being 1.61 (1.09–2.39) and 1.59 (1.25–2.01), respectively, and sex was not a significant effect modifier of the above association (peffectmodificationwithsex > 0.05). Meanwhile, compared with the participants in the lowest quintile of HbAA, the participants in the highest quintile of HbAA had a greater risk of CVD mortality (HR: 1.84, 95% CI: 1.00–3.37) and all-cause mortality (HR: 2.21, 95% CI: 1.46–3.05). Moreover, no significant association between quintiles of HbGA with CVD mortality was observed.
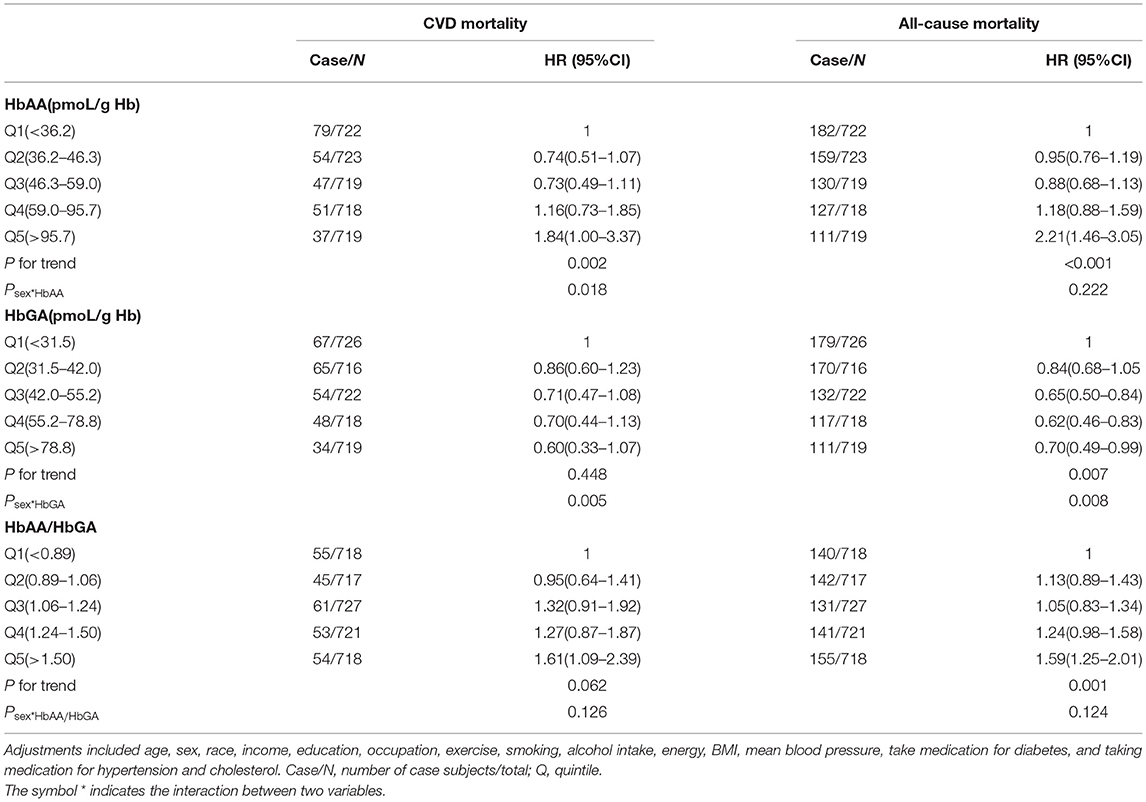
Table 2. Adjusted hazard ratios (HRs) for the associations between HbAA, HbGA, HbAA/HbGA and CVD or all-cause mortality in the total hyperglycemia population.
Further, in line with the results of the total sample, results of subgroup analysis in the people with diabetes and pre-diabetes indicated that compared with the participants in the lowest quintile of HbAA/HbGA, the participants in the highest quintile of HbAA/HbGA had a greater risk of CVD mortality (HR: 1.92, 95% CI: 1.11–3.31 for diabetes and HR: 1.78, 95% CI:1.01–3.14 for pre-diabetes) and all-cause mortality (HR: 1.81, 95% CI:1.27–2.58 for diabetes and HR: 1.59, 95% CI: 1.14–2.20 for pre-diabetes), and sex was not a significant effect modifier of the above association (peffectmodificationwithsex > 0.05) (Tables 3, 4). Meanwhile, no significant association between quintiles of HbAA or HbGA with mortality from CVD was observed among people with diabetes and pre-diabetes, respectively.
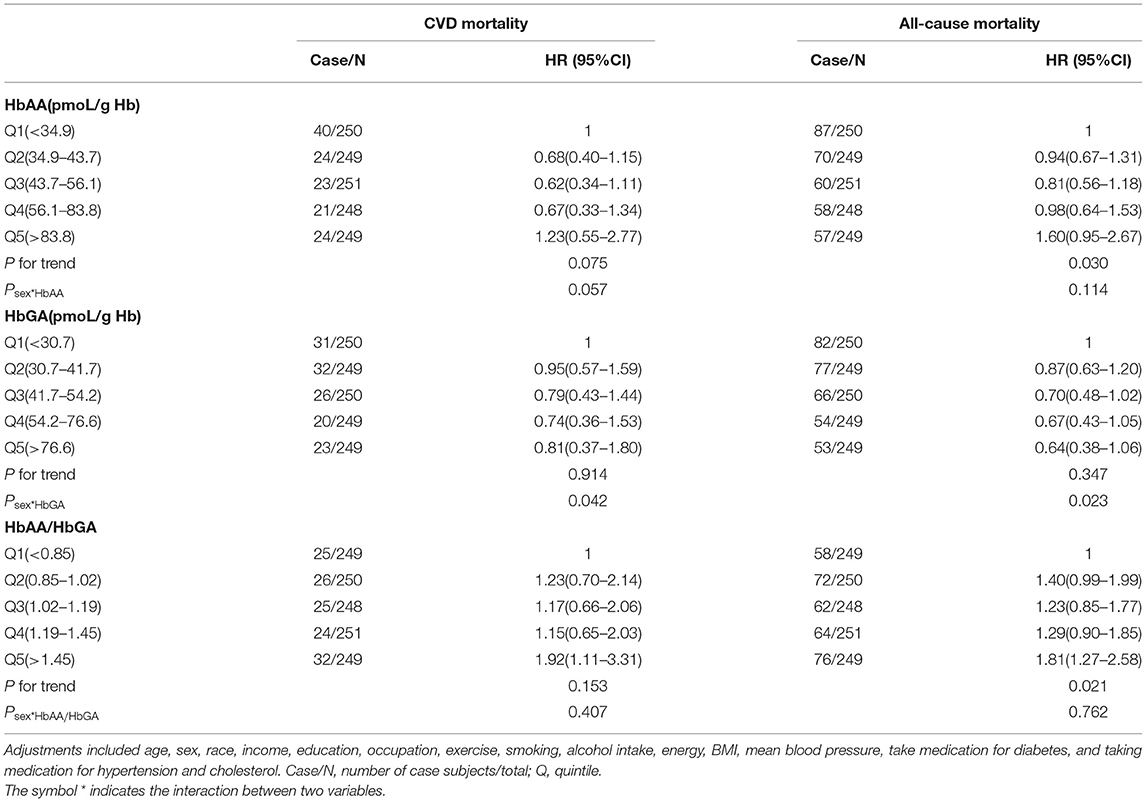
Table 3. Adjusted HRs for the associations between HbAA, HbGA, HbAA/HbGA and CVD or all-cause mortality in diabetes population.
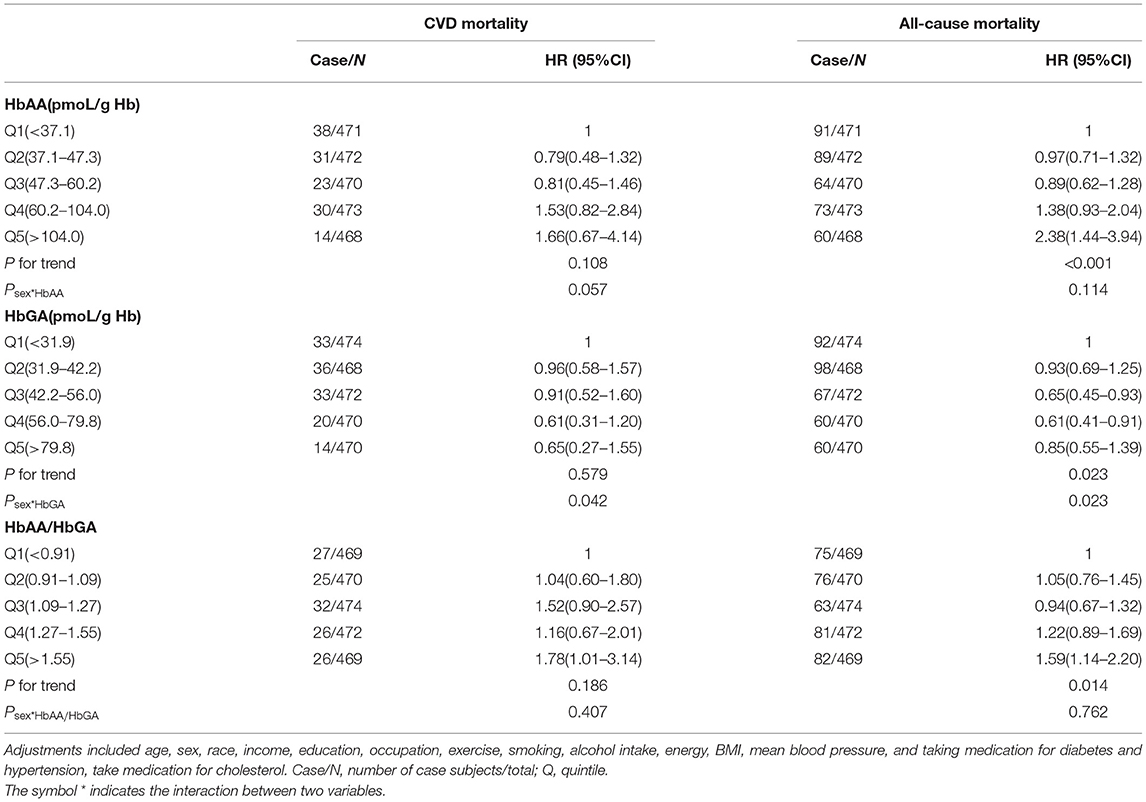
Table 4. Adjusted HRs for the associations between HbAA, HbGA, HbAA/HbGA and CVD or all–cause mortality in pre-diabetes population.
Additionally, the significant modification effects of sex on the relationship between HbAA and CVD mortality in the total sample, and the association of HbGA with mortalities of CVD and all-cause were observed among the total sample, and the samples of pre-diabetes and diabetes, respectively. We therefore examined this above association by sex, which is presented in the Supplementary Materials. In the total sample, the increased HbAA levels were negatively related with CVD mortality in men; whereas it was positively related with CVD mortality in women (Supplementary Table 1). Moreover, no significant relationship between HbGA and CVD mortality was observed in men (Supplementary Table 2); whereas an increase in HbGA was negatively associated with CVD or all-cause mortality in women (Supplementary Table 3). These findings indicated that compared with the ratio of HbAA and HbGA, the individual association of HbAA or HbGA with CVD mortality was more easily influenced by sex.
Discussion
To our knowledge, the present study is the first to examine whether exposure to acrylamide is associated with long-term survival among people with hyperglycemia. In this study, we observed that higher levels of the ratio between HbAA and HbGA were related to greater CVD and all-cause mortalities among people with hyperglycemia, independent of other traditional risk factors of CVD. Moreover, this association was relatively robust, which was consistently observed among people with diabetes and pre-diabetes, respectively.
Currently, only a few cross-sectional studies and one prospective study have examined the association of the biomarkers of acrylamide with blood glucose, lipids, and CVD mortality among the general population (8, 17, 18). However, there are some contradictions among these results. The previous study found that a higher HbAA level was associated with lower fasting insulin and glucose (19), whereas the recent study found that increased urine biomarkers of acrylamide were associated with increased fasting blood glucose, probably through lipid peroxidation (20). Moreover, it has been found that HbGA is positively associated with serum triglycerides and low-density lipoprotein cholesterol, suggesting that a higher level of HbGA is associated with dyslipidemia (17), which contradicts the recent results regarding the association between whole blood HbGA and CVD, reporting that higher levels of HbGA were associated with the lower prevalence of CVD and CVD-mortality (21). The conflicting findings of these studies suggest that both HbAA and HbGA have limitations as markers of acrylamide exposure alone, whereas the present study integrates two acrylamide metabolites, HbAA and HbGA, by using a ratio of the two as biomarkers of acrylamide exposure for a more comprehensive evaluation. Furthermore, these conflicting results warrant a study focusing on people with diabetes or pre-diabetes to elucidate the association between exposure to acrylamide and long-term survival in this specific population.
In this study, although we observed the positive association of HbAA with CVD and all-cause mortalities in the total sample, this relationship could not be consistently observed among the people with diabetes or pre-diabetes, suggesting that this significant association in the total sample was likely driven by the relatively large sample size. In contrast, the association of higher levels of the ratio of HbAA and HbGA with mortalities of CVD and all-cause could be consistently observed among people with diabetes or pre-diabetes, suggesting that the combination of HbAA and HbGA was more important than focusing on the individual effect of HbAA or HbGA on the development of CVD among people with hyperglycemia. In fact, when acrylamide enters circulation, a proportion of acrylamide reacts with hemoglobin to form HbAA (22), and the other proportion of acrylamide can be metabolized by binding to glutathione (GSH) (23), which further can be oxidized into glycidamide by cytochrome P450 (24), and the epoxidation of hydrolyzate can largely convert glycidamide into non-toxic glycine amide due to the body's self-protective mechanism (25). The ratio of HbAA and HbGA probably reflects the balance of the detoxification metabolism in the body (4), and based on the findings of this study, the balance of metabolic networks for acrylamide may play a more critical role in the development of CVD among people with hyperglycemia.
Moreover, a series of the previous Vivo- and Vitro-experiments elucidated the mechanisms underlying the relationship between exposure to acrylamide and CVD mortality (26–28). In the past few years, there has been some progress in understanding the underlying impact of acrylamide on CVD, which is that acrylamide exposure may lead to oxidative stress and disturbance of physiological function (26, 28). A human study has found that the consumption of acrylamide-containing potato chips could cause significant changes in the levels of oxidative stress and inflammation response, probably increasing the risk of atherosclerosis (27). Moreover, zebrafish research has shown that acrylamide exposure could aggravate oxidation and degradation of low-density lipoprotein with increased production of reactive oxygen species (ROS) and cause dose-dependent fat accumulation in the liver (29). In addition, another animal study indicated exposure to acrylamide could cause a significant decrease in the GSH level (30) and stimulate oxidative stress, which might strike the blood vessels and exacerbate the progression of atherosclerosis (31). Further, vitro experiments also revealed that chronic exposure to acrylamide and glycidamide could induce accelerated endothelial aging, even at low concentrations (13). The results of our study showed that acrylamide exposure was significantly associated with CVD and all-cause mortalities, which could be partially supported by these studies above.
Further, we observed that among the people with diabetes, the participants whose ratio was ≥1.45 had greater CVD mortality compared with control; whereas among the people with pre-diabetes, the participants whose ratio was ≥1.55 had greater CVD mortality compared with control. These findings suggested that the ratio for increasing the risk of CVD mortality is lower in diabetes than in pre-diabetes, which adds to our understanding of how to develop an adequate range for the ratio among people with diabetes in the future. It has been reported that people with diabetes have higher levels of glutathione and cytochrome P450 2E1 than other people (32). Therefore, in people with diabetes, the circulating acrylamide tends to convert to HbGA, probably making the ratio of HbAA and HbGA significantly lower than in other people, and this mechanism supported the findings in this study.
This present study examined the relationship between exposure to acrylamide and the mortalities of CVD and all-cause, demonstrating the importance of the balance of metabolic networks for acrylamide among people with hyperglycemia. This association documented in our study had relatively strong robustness after adjusting for a range of important classical confounders. These findings also provided evidence for the impact of environmental exposure on the improvement of long-term survival for people with diabetes. Diabetes care professionals should be aware of the current findings from this study regarding the deleterious effect of exposure to acrylamide in daily life. This information is of importance in providing individualized treatment strategies in relation to avoiding environmental exposure for patients with diabetes. In addition, we recognized that this study had several limitations. First, we had adjusted a range of potential confounders, whereas the present study was still observational research, and some unmeasured confounding factors cannot be excluded. Second, this study was not able to distinguish between different types of diabetes. More research in the future is needed to examine this association in terms of type 1 and type 2 diabetes to provide more comprehensive evidence. Last, the measurement of exposure to acrylamide was only conducted at baseline. The studies with more frequent measurements may offer a more complicated blueprint of changes in the exposure to acrylamide and long-term survival among people with hyperglycemia.
Conclusion
Exposure to acrylamide, which is indicated by the higher ratio of HbAA and HbGA in the whole blood, is related to higher CVD and all-cause mortalities among people with diabetes and pre-diabetes.
Data Availability Statement
Publicly available datasets were analyzed in this study. This data can be found here: National Health and Nutrition Examination Survey https://www.cdc.gov/nchs/nhanes/index.htm.
Author Contributions
CS and TH planned the work. JX, XS, HJ, and CH carried out the statistical analysis. HW wrote and reported the work. WW and WJ played critical roles in revising the manuscript. All authors made a significant contribution to this article, critically assessed and reviewed the paper, and approved the version to be published.
Funding
This research was supported by the funding from the HMU Marshal Initiative Funding (HMUMIF-21011 to WJ, HMUMIF-21013 to WW) and the National Natural Science Foundation of China (81803227 to TH).
Conflict of Interest
The authors declare that the research was conducted in the absence of any commercial or financial relationships that could be construed as a potential conflict of interest.
Publisher's Note
All claims expressed in this article are solely those of the authors and do not necessarily represent those of their affiliated organizations, or those of the publisher, the editors and the reviewers. Any product that may be evaluated in this article, or claim that may be made by its manufacturer, is not guaranteed or endorsed by the publisher.
Supplementary Material
The Supplementary Material for this article can be found online at: https://www.frontiersin.org/articles/10.3389/fcvm.2022.930135/full#supplementary-material
References
1. Friedman M. Chemistry, biochemistry, and safety of acrylamide. A review. J Agric Food Chem. (2003) 51:4504–26. doi: 10.1021/jf030204+
2. Lin CY, Lee HL, Chen YC, Lien GW, Lin LY, Wen LL, et al. Positive association between urinary levels of 8-hydroxydeoxyguanosine and the acrylamide metabolite N-acetyl-S-(propionamide)-cysteine in adolescents and young adults. J Hazard Mater. (2013) 261:372–7. doi: 10.1016/j.jhazmat.2013.06.069
4. Koszucka A, Nowak A, Nowak I, Motyl I. Acrylamide in human diet, its metabolism, toxicity, inactivation and the associated European Union legal regulations in food industry. Crit Rev Food Sci Nutr. (2020) 60:1677–92. doi: 10.1080/10408398.2019.1588222
5. Liu Y, Wang R, Zheng K, Xin Y, Jia S, Zhao X. Metabonomics analysis of liver in rats administered with chronic low-dose acrylamide. Xenobiotica. (2020) 50:894–905. doi: 10.1080/00498254.2020.1714791
6. Wang SY, Han D, Pan YL Yu CP, Zhou XR, Xin R, et al. A urinary metabolomic study from subjects after long-term occupational exposure to low concentration acrylamide using UPLC-QTOF/MS. Arch Biochem Biophys. (2020) 681:108279. doi: 10.1016/j.abb.2020.108279
7. Shan X, Li Y, Meng X, Wang P, Jiang P, Feng Q. Curcumin and (-)-epigallocatechin-3-gallate attenuate acrylamide-induced proliferation in HepG2 cells. Food Chem Toxicol. (2014) 66:194–202. doi: 10.1016/j.fct.2014.01.046
8. Lin CY, Lin YC, Kuo HK, Hwang JJ, Lin JL, Chen PC, et al. Association among acrylamide, blood insulin, and insulin resistance in adults. Diabetes Care. (2009) 32:2206–11. doi: 10.2337/dc09-0309
9. Akinlade KS, Kumuyi AS, Rahamon SK, Olaniyi JA. Insulin sensitivity, inflammation, and basal metabolic rate in adults with sickle cell anemia. Int J Appl Basic Med Res. (2018) 8:106–10. doi: 10.4103/ijabmr.IJABMR_96_17
10. Hsu C-N, Hou C-Y, Lu P-C, Chang-Chien G-P, Lin S, Tain Y-L. Association between acrylamide metabolites and cardiovascular risk in children with early stages of chronic kidney disease. Int J Mol Sci. (2020) 21:E5855. doi: 10.3390/ijms21165855
11. Stošić M, Matavulj M, Marković J. Subchronic exposure to acrylamide leads to pancreatic islet remodeling determined by alpha cell expansion and beta cell mass reduction in adult rats. Acta Histochem. (2018) 120:228–35. doi: 10.1016/j.acthis.2018.02.002
12. Yue Z, Chen Y, Song Y, Zhang J, Yang X, Wang J, et al. Effect of acrylamide on glucose homeostasis in female rats and its mechanisms. Food Chem Toxicol. (2020) 135:110894. doi: 10.1016/j.fct.2019.110894
13. Sellier C, Boulanger E, Maladry F, Tessier FJ, Lorenzi R, Nevière R, et al. Acrylamide induces accelerated endothelial aging in a human cell model. Food Chem Toxicol. (2015) 83:140–5. doi: 10.1016/j.fct.2015.05.021
14. Han T, Gao J, Wang L, Li C, Qi L, Sun C, et al. The association of energy and macronutrient intake at dinner versus breakfast with disease-specific and all-cause mortality among people with diabetes: the U.S. National Health and Nutrition Examination Survey, 2003-2014. Diabetes Care. (2020) 43:1442–8. doi: 10.2337/dc19-2289
15. Vesper HW, Sternberg MR, Frame T. Pfeiffer CM. Among 10 sociodemographic and lifestyle variables, smoking is strongly associated with biomarkers of acrylamide exposure in a representative sample of the US Population. J Nutr. (2013) 143:995S−1000S. doi: 10.3945/jn.112.173013
16. Papandrikopoulou A, Ender BE, Gassen HG A. carrier-free 32P-labeled Edman reagent for the detection of proteins in the femtomole range. Biol Chem Hoppe Seyler. (1986) 367:981–8. doi: 10.1515/bchm3.1986.367.2.981
17. Cheang I, Liao S, Zhu X, Lu X, Zhu Q, Yao W, et al. Association of acrylamide hemoglobin biomarkers with serum lipid levels in general US population: NHANES 2013-2016. Ecotoxicol Environ Saf. (2021) 214:112111. doi: 10.1016/j.ecoenv.2021.112111
18. Huang M, Jiao J, Wang J, Chen X, Zhang Y. Associations of hemoglobin biomarker levels of acrylamide and all-cause and cardiovascular disease mortality among U.S. adults: National Health and Nutrition Examination Survey 2003-2006. Environ Pollut. (2018) 238:852–8. doi: 10.1016/j.envpol.2018.03.109
19. Alayash AI. al-Quorain A. Prevalence of diabetes mellitus in individuals heterozygous and homozygous for sickle cell anemia. Clin Physiol Biochem. (1989) 7:87–92.
20. Faure P, Tamisier R, Baguet JP, Favier A, Halimi S, Lévy P, et al. Impairment of serum albumin antioxidant properties in obstructive sleep apnoea syndrome. Eur Respir J. (2008) 31:1046–53. doi: 10.1183/09031936.00062707
21. Zhang Y, Huang M, Zhuang P, Jiao J, Chen X, Wang J, et al. Exposure to acrylamide and the risk of cardiovascular diseases in the National Health and Nutrition Examination Survey 2003-2006. Environ Int. (2018) 117:154–63. doi: 10.1016/j.envint.2018.04.047
22. Duke TJ, Ruestow PS. Marsh GM. The influence of demographic, physical, behavioral, and dietary factors on hemoglobin adduct levels of acrylamide and glycidamide in the general US population. Crit Rev Food Sci Nutr. (2018) 58:700–10. doi: 10.1080/10408398.2016.1215289
23. Zhu Y, Luo Y, Sun G, Wang P, Hu X, Chen F. Role of glutathione on acrylamide inhibition: Transformation products and mechanism. Food Chem. (2020) 326:126982. doi: 10.1016/j.foodchem.2020.126982
24. Sen A, Ozgun O, Arinç E, Arslan S. Diverse action of acrylamide on cytochrome P450 and glutathione S-transferase isozyme activities, mRNA levels and protein levels in human hepatocarcinoma cells. Cell Biol Toxicol. (2012) 28:175–86. doi: 10.1007/s10565-012-9214-1
25. Low MY, Koutsidis G, Parker JK, Elmore JS, Dodson AT, Mottram DS. Effect of citric acid and glycine addition on acrylamide and flavor in a potato model system. J Agric Food Chem. (2006) 54:5976–83. doi: 10.1021/jf060328x
26. Komoike Y, Matsuoka M. In vitro and in vivo studies of oxidative stress responses against acrylamide toxicity in zebrafish. J Hazard Mater. (2019) 365:430–9. doi: 10.1016/j.jhazmat.2018.11.023
27. Wang B, Qiu W, Yang S, Cao L, Zhu C, Ma J, et al. Acrylamide exposure and oxidative DNA damage, lipid peroxidation, and fasting plasma glucose alteration: association and mediation analyses in Chinese urban adults. Diabetes Care. (2020) 43:1479–86. doi: 10.2337/dc19-2603
28. Huang M, Jiao J, Wang J, Xia Z, Zhang Y. Characterization of acrylamide-induced oxidative stress and cardiovascular toxicity in zebrafish embryos. J Hazard Mater. (2018) 347:451–60. doi: 10.1016/j.jhazmat.2018.01.016
29. Kim SM, Baek JM, Lim SM, Kim JY, Kim J, Choi I, et al. Modified lipoproteins by acrylamide showed more atherogenic properties and exposure of acrylamide induces acute hyperlipidemia and fatty liver changes in zebrafish. Cardiovasc Toxicol. (2015) 15:300–8. doi: 10.1007/s12012-014-9294-7
30. Zödl B, Schmid D, Wassler G, Gundacker C, Leibetseder V, Thalhammer T, et al. Intestinal transport and metabolism of acrylamide. Toxicology. (2007) 232:99–108. doi: 10.1016/j.tox.2006.12.014
31. Vogiatzi G, Tousoulis D, Stefanadis C. The role of oxidative stress in atherosclerosis. Hellenic J Cardiol. (2009) 50:402–9.
Keywords: CVD mortality, all-cause mortality, hyperglycemia, NHANES, acrylamide
Citation: Wu H, Sun X, Jiang H, Hu C, Xu J, Sun C, Wei W, Han T and Jiang W (2022) The Association Between Exposure to Acrylamide and Mortalities of Cardiovascular Disease and All-Cause Among People With Hyperglycemia. Front. Cardiovasc. Med. 9:930135. doi: 10.3389/fcvm.2022.930135
Received: 27 April 2022; Accepted: 17 June 2022;
Published: 18 July 2022.
Edited by:
James Philip Hobkirk, Hull York Medical School, United KingdomReviewed by:
Wei Quan, Hunan Agricultural University, ChinaZhou Zhou, Chinese Academy of Medical Sciences and Peking Union Medical College, China
Copyright © 2022 Wu, Sun, Jiang, Hu, Xu, Sun, Wei, Han and Jiang. This is an open-access article distributed under the terms of the Creative Commons Attribution License (CC BY). The use, distribution or reproduction in other forums is permitted, provided the original author(s) and the copyright owner(s) are credited and that the original publication in this journal is cited, in accordance with accepted academic practice. No use, distribution or reproduction is permitted which does not comply with these terms.
*Correspondence: Wei Wei, d2Vpd2VpYnViYmxlMTk5NEAxNjMuY29t; Tianshu Han, c25vd2NhbGVuZGFyQDEyNi5jb20=; Wenbo Jiang, aG11d2VuYm9qaWFuZ0AxNjMuY29t
†These authors have contributed equally to this work