- 1Administration of Pharmaceutical Services, Main Hospital, King Fahad Medical City, Riyadh, Saudi Arabia
- 2Pharmacology and Biostatistics/Comparative Medicine, Alfaisal University College of Medicine, King Faisal Specialist Hospital and Research Centre, Riyadh, Saudi Arabia
- 3Clinical Pharmacy Department, King Saud Medical City, Riyadh, Saudi Arabia
- 4Department of Clinical Pharmacy, Almoosa Specialist Hospital, Al-Ahasa, Saudi Arabia
- 5Department of Neurology, King Fahad Medical City, Riyadh, Saudi Arabia
- 6Pharmacy College, Shaqra University, Riyadh, Saudi Arabia
- 7Department of Clinical Pharmacy Service, Prince Mohammed Bin Abdulaziz Hospital, Riyadh, Saudi Arabia
- 8Pharmacy College, Princess Nourah Bint Abdulrahman University, Riyadh, Saudi Arabia
- 9Department of Clinical Pharmacy, College of Pharmacy, Prince Sattam Bin Abdulaziz University, Al-Kharj, Saudi Arabia
- 10Research Center, King Fahad Medical City, Riyadh, Saudi Arabia
- 11Medicine Department, King Fahad Medical City, Riyadh, Saudi Arabia
- 12Department of Pediatric Dentistry, King Fahad Medical City, Riyadh, Saudi Arabia
- 13College of Medicine, King Saud Bin Abdulaziz University for Health Sciences, Riyadh, Saudi Arabia
- 14Department of Pharmacy Service, Prince Mutib Bin Abdulaziz Hospital, Sakaka, Saudi Arabia
- 15Department of Pediatric Dentistry, College of Dentistry, King Abdulaziz University, Jeddah, Saudi Arabia
- 16Department of Pharmacy, Alahsa Hospital, Al-Ahsa, Saudi Arabia
- 17Department of Pharmacy Practice, College of Clinical Pharmacy, King Faisal University, Al-Ahsa, Saudi Arabia
- 18Department of Pharmacy Practice and Science, College of Pharmacy, University of Arizona, Tuscon, AZ, United States
Introduction: Thrombotic complications of coronavirus disease 2019 (COVID-19) have received considerable attention. Although numerous conflicting findings have compared escalated thromboprophylaxis doses with a standard dose to prevent thrombosis, there is a paucity of literature comparing clinical outcomes in three different anticoagulation dosing regimens. Thus, we investigated the effectiveness and safety profiles of standard, intermediate, and high-anti-coagulation dosing strategies in COVID-19 critically ill patients.
Methodology: This retrospective multicenter cohort study of intensive care unit (ICU) patients from the period of April 2020 to August 2021 in four Saudi Arabian centers. Inclusion criteria were age ≥ 18 years, diagnosis with severe or critical COVID-19 infection, and receiving prophylactic anticoagulant dose within 24–48 h of ICU admission. The primary endpoint was a composite of thrombotic events, with mortality rate and minor or major bleeding serving as secondary endpoints. We applied survival analyses with a matching weights procedure to control for confounding variables in the three arms.
Results: A total of 811 patient records were reviewed, with 551 (standard-dose = 192, intermediate-dose = 180, and high-dose = 179) included in the analysis. After using weights matching, we found that the standard-dose group was not associated with an increase in the composite thrombotic events endpoint when compared to the intermediate-dose group {19.8 vs. 25%; adjusted hazard ratio (aHR) =1.46, [95% confidence of interval (CI), 0.94–2.26]} or when compared to high-dose group [19.8 vs. 24%; aHR = 1.22 (95% CI, 0.88–1.72)]. Also, there were no statistically significant differences in overall in-hospital mortality between the standard-dose and the intermediate-dose group [51 vs. 53.4%; aHR = 1.4 (95% CI, 0.88–2.33)] or standard-dose and high-dose group [51 vs. 61.1%; aHR = 1.3 (95% CI, 0.83–2.20)]. Moreover, the risk of major bleeding was comparable in all three groups [standard vs. intermediate: 4.8 vs. 2.8%; aHR = 0.8 (95% CI, 0.23–2.74); standard vs. high: 4.8 vs. 9%; aHR = 2.1 (95% CI, 0.79–5.80)]. However, intermediate-dose and high-dose were both associated with an increase in minor bleeding incidence with aHR = 2.9 (95% CI, 1.26–6.80) and aHR = 3.9 (95% CI, 1.73–8.76), respectively.
Conclusion: Among COVID-19 patients admitted to the ICU, the three dosing regimens did not significantly affect the composite of thrombotic events and mortality. Compared with the standard-dose regimen, intermediate and high-dosing thromboprophylaxis were associated with a higher risk of minor but not major bleeding. Thus, these data recommend a standard dose as the preferred regimen.
Introduction
In addition to pulmonary manifestations of coronavirus 2019 (COVID-19) (1, 2), there is increasing concern about COVID-19-related extra-pulmonary complications, including thrombotic complications (3). Following emerging data, investigators paid more attention to the hypercoagulability state seen in COVID-19 cases that can result in the development of microthrombi in pulmonary microvasculature, deep vein thrombosis (VTE), and pulmonary embolisms (PE) (4). Initial studies from the early days of COVID-19 found a trend of thrombotic events in COVID-19 patients which prompted a flood of research in this area (5, 6). The incidence of thrombotic events was broadly inconsistent, with reports claiming that up to 69% of COVID-19 patients in a specific population were affected, despite the use of thromboprophylaxis (5–8). Compared to non-COVID-19 patients, previous studies showed COVID-19 patients have a greater risk of venous thromboembolism (VTE) 11.7 vs. 4.8% (9). According to meta-analyses, the incidence of VTE in intensive care unit (ICU)-admitted patients is higher than in those admitted to the general ward, with rates of 31 and 7%, respectively (10, 11). Such coagulation dysfunction has been associated with poor prognosis and negative outcomes, with 40% dying in those who developed VTE in the ICU (12, 13). Given high VTE occurrence reports and its unfavorable prognosis post-COVID-19 infection (14), some experts, agencies, and scientific committees advocated for increased thromboprophylaxis doses to be considered despite the absence of randomized evidence (15–18).
A comprehensive understanding of COVID-19 pathogenesis is still unclear. However, with available data related to the pathology of venous thromboembolism, severe acute respiratory syndrome coronavirus-2 (SARS-CoV-2) has an affinity binding to angiotensin-converting-enzyme (ACE) 2 receptor, which exists in different tissues including, but not limited to, arterial and venous endothelial cells (19). As per Virchow's triad, a possible higher risk of developing thromboembolic events is expected due to vascular injury. Another possible explanation of VTE is that the inflammatory reaction caused by viral, bacterial and fungal infection results in the activation of host defense systems. This eventually contributes to the up-regulation of coagulation factors and thrombin formation pathways (20, 21). In addition to the risk factors of VTE that critically ill patients carry as a result of venous stasis (22–25), other factors that increase the risk of hypercoagulability may exist. The pro-coagulant profile, particularly D-dimer, is speculated to be a predictor of VTE development with sensitivity and specificity of 85 and 88.5%, respectively (12, 26, 27).
The anticipated benefit of increasing the anticoagulant dose is still debated. A meta-analysis of 23 retrospective observational studies found a favorable mortality reduction with the escalated dose of prophylactic anticoagulant when compared to the standard dose (28). However, high quality evidence of meta-analyses and randomized controlled trials (RCTs) investigating the clinical outcomes of various prophylactic regimens in critically ill patients have revealed conflicting results (29–33). Generally, previous studies were notably limited by poor study designs (6, 34), diversity of study populations (34–37), small sample sizes (6, 36, 38), variations in treatment settings (ICU vs. non-ICU) (39), heterogeneity of thromboprophylaxis dosing regimen definition (40), and unadjusted pooled crude estimates (34). Furthermore, numerous studies compared the effectiveness and safety of just two thromboprophylaxis regimens. However, one open question about comparing three different regimens of thromboprophylaxis simultaneously in critically ill COVID-19 patients is whether they are comparable in terms of effectiveness and coagulopathy. Obviously, limiting comparisons to two thromboprophylaxis regimens (standard and intermediate/high) may cast doubt on whether observed associations of thrombotic events or bleeding are causal or simply artifacts of more complex interrelationships between the disease itself, outcome, interventional dose, and covariates. Thus, our study aimed to assess how three different thromboprophylaxis dose regimens affect the rate of thrombotic events in critically ill COVID-19 patients.
Methods
Study design and setting
This retrospective analysis of the cohort study was conducted at 4 centers in Saudi Arabia, with patients hospitalized in ICUs of tertiary specialty referral hospitals: King Fahad Medical City (KFMC) in Riyadh, King Saud Medical City Hospital (KSMC) in Riyadh, Prince Mohammed Bin Abdulaziz hospital (PMAH) in Riyadh, and Almoosa Specialized Hospital in Al-Ahsa. Study approval was granted by the Institutional Review Boards at KFMC and PMAH (IRB: 20-666), KSMC (IRB:H1RI-16-Nov20-01), Almoosa hospital (IRB: ARC-20-12-4). Due to the retrospective design, informed consent was waived, as it was considered exempt. Our report adopted the STROBE (Strengthening the Reporting of Observational Studies in Epidemiology) Statement checklist (41).
Participant selection
Lists of critically ill COVID-19 patients admitted to ICU between April 2020 and August 2021 were obtained from the health informatics officers. We used a random-selection technique to screen patients for eligibility. Random selection avoids sampling bias in giving each patient's record an equal chance of selection and coding (42). Inclusion criteria were age ≥18 years, diagnosis of critical SARS-CoV-2 infection by real-time polymerase chain reaction (RT-PCR) from the nasopharyngeal swab and receiving prophylactic anticoagulant within 24–48 h of ICU admission. Patients were excluded if pregnant, diagnosed with VTE or atrial fibrillation during COVID-19 admission, patients with chronic anticoagulants at admission, had a contraindication to anticoagulants including active bleeding, platelet counts <25 × 109/L, and fibrinogen <0.5 g/L, or if they were on VTE-induced medications (oral contraceptives, tamoxifen, etc.,).
Intensities of anticoagulant dose
This study looked at three different anticoagulant prophylactic dosing strategies: standard, intermediate, and high. The “standard dose” was defined as enoxaparin 40 mg subcutaneous (SC) daily, or 30 mg in renal failure patients, heparin 5,000 units SC twice or thrice daily, or fondaparinux 2.5 mg SC daily. The “intermediate dose” included patients treated with enoxaparin 1 mg/kg SC daily or enoxaparin 40 mg SC twice daily, or heparin 7,500 units SC twice or thrice daily. The high dose was enoxaparin 1 mg/kg SC twice daily or heparin infusion. Patients received the prescribed dose of prophylactic regimens within 24–48 h of ICU admission until hospital discharge, developing of thrombotic events, or death.
Data collection
The study data were collected and managed using REDCap (Research Electronic Data Capture) a secure, web-based data capture application (43). Electronic case report forms (eCRFs) were developed, pilot tested, and revised accordingly. Data were manually extracted from electronic health records (EHRs) and entered into the REDCap system in a de-identified manner. A trained team of data managers were recruited to be responsible for delivering a complete, clean, and accurate dataset. Clinical data managers performed various levels of data validation following data collection, known as edit checks, until it was considered “clean” enough to support analysis. During this step, they used categories to define the essential checks which included missing data, simple range checks, logical inconsistencies, cross-form checks, and protocol violations. Edit check specification (data validation procedure) was used to ensure that all data was the same edited consistently throughout the study. Extracted data include demographic characteristics [e.g., age, gender, weight, height, body mass index (BMI)], clinical characteristics [e.g., history of VTE, diabetes, hypertension, cancer, and cerebrovascular and cardiovascular disease, renal failure, renal dialysis, post-surgery, mechanical ventilation, acute respiratory distress syndrome (ARDS), APACHE score, use of a sedative agent or paralytic agents, recent use of oral contraceptive, steroid intake, vascular access device (VAD), regimen for COVID-19 treatment], lab parameters [D-dimer, prothrombin time (PT) and activated partial thromboplastin time (APPT), international normalized ratio (INR), fibrinogen, and platelet count], clinical outcome [composite thrombotic events (PE, DVT, ischemic stroke, myocardial infarction, systemic arterial embolism)] and any bleeding (minor or major), and death.
Study outcomes
The primary outcome was the occurrence of any component of composite thrombotic events in COVID-19 patients admitted to ICU who received standard, intermediate, or high anticoagulant doses for VTE prevention. Mortality rate and occurrence of major and minor bleeding were secondary outcomes of interest.
Definitions
We defined severe and critical cases of COVID-19 based on the World Health Organization (WHO) (44). Severe manifestation was defined as fever plus symptoms ≥1 of the following: respiratory rate ≥30/min, dyspnea, respiratory distress, SpO2 ≤ 93% on room air, PaO2/FiO2 ratio <300 or lung infiltrate >50% of lung field within 24–48 h. Critical illness was evidenced by symptoms ≥1 of the following: ARDS, septic shock, altered consciousness, and/or multi-organ failure.
We defined thrombotic events as a composite outcome where at least one of the following occurred: symptomatic acute PE, DVT, ischemic stroke, myocardial infarction, or systemic arterial embolism (45). Three distinct justifications for adopting composite endpoints as the primary outcome: the rate of individual response was expected to be low; the full effect could not be captured meaningfully by a single outcome; and thrombotic events were expected to present in various manifestations of the same disease. Typically, PE diagnosis was determined by computed tomography pulmonary angiography (CTPA) or a combination of high pretest clinical probability of PE with high probability ventilation-perfusion (V/Q) lung scan. DVT diagnosis was confirmed by compression ultrasonography (CUS) with doppler of lower extremities. Myocardial infarction was diagnosed by clinical means, ECG changes and cardiac criteria according to the European Society of Cardiology (ESC) (46). Ischemic stroke was confirmed if suspected patients had brain computed tomography (CT) scan and/or magnetic resonance imaging (MRI), as well as the radiology consultant's report indicated the final diagnosis in the patient file. Mortality was defined as death that occurred during hospitalization for any reason.
Major bleeding was identified by the International Society on Thrombosis and Hemostasis (ISTH) as fatal bleeding and/or symptomatic bleeding in critical areas or organs (such as intracranial, intraspinal, intraocular, retroperitoneal, intraarticular or pericardial, or intramuscular bleeding with compartment syndrome) or documented bleeding causing a decrease in hemoglobin (Hgb) level of 2 g/dL or more, and transfusion of 2 or more units of packed red blood cells (PRBCs). In contrast, minor bleeding was all bleeds that were not considered major or non-major bleeding events (47).
Sample size calculation
We calculated the sample size using Power Analysis and Sample Size (PASS) 11® and G*Power (Version 3.1.9.7) software. Because of a wide variety of thrombotic events at the time of study inception, we assumed a −15% risk difference between the standard dose, intermediate dose, and high dose arms. The total sample size was calculated to be 549 with an estimated ratio of 1:1:1 (N = 183 in each arm) as needed to provide an 80% power and significance level (α, type 1 error rate) of <0.05 to assess the hypothesis.
Statistical analysis
The three treatment groups' demographic and baseline data were compared using standard descriptive statistics. Where appropriate, continuous data were presented using means with standard deviations (±SDs) and medians with interquartile ranges (IQRs). ANOVA and Kruskal Wallis tests were used to compare normally and non-normally distributed quantitative variables between treated groups, respectively. To compare categorical variables, the chi-squared test was performed, and the results were reported as frequencies and percentages.
Missing data handling
Multiple Imputation by Chained Equations (MICE) approach with Nelson Aalen estimator was used to handle missing data, which was regarded as missing at random (MAR) (48, 49). To accommodate for uncertainty, multiple dataset predictions (5 imputed datasets with 10 iterations) were constructed for each missing value in this method, resulting in decreased variability and more accurate standard errors. Only variables with <15% missing data were considered. Convergence and density plots were visually checked for missing variables. All estimates were pooled across the generated datasets.
Matching weights procedure and survival analysis
Due to the challenge of multiple covariates and multiple treatment arms in this observational study, we applied the matching weights method, an extension of inverse probability of treatment weighting (IPTW), as a sensitivity analysis to estimate the average treatment effect (ATE) outcome across multiple treatment groups (50). The approach reweights all standard, intermediate, and high groups to simulate a propensity score-matched population. Multinomial logistic regression was used to fit all propensity scores of the covariates of interest. The use of this methodology is explained by Yoshida et al. (50). Post-weighting balance assessment is used to find the optimal balance with absolute standardized mean differences of <0.2 for all covariates, indicating better covariate balance (50). Propensity score distribution and overlap was visually inspected via a mirror diagram. A covariate balance check was demonstrated via a Love plot. To estimate the probability of survival of the three groups, we used an adjusted Kaplan-Meier (KM) model with weighted data. Time 0 was the time of anticoagulation initiation. Censoring was considered for patients with no events that were discharged alive or were still admitted patients at the time of data collection. The stratified log-rank test was used to compare the survival distributions of three samples. In order to draw weighted KM curves, we used “svykm” function from the “survey” package in R which assumes weights as sampling weights to account for the matching design. This would allow the estimation of robust standard errors for survival. For the relative effect, we fitted the Cox regression model. The assumptions were adequately checked by using Schoenfeld residuals and visual plots. We implemented robust variance estimation to estimate standard errors. In the case of low to no events, we could not estimate hazards ratios.
Statistical software
R Core Team (51) software (R Foundation for Statistical Computing, Version 4.0.1, Vienna, Austria) was used. The following packages in the R interface were used to conduct the analyses: survival (52), survey (53), mice (48), MatchThem (54), cobalt (55) and tableone (56).
Results
Baseline characteristics
During the study period, 811 patients were screened for eligibility criteria (Figure 1). A total of 551 patients met inclusion criteria for analysis, with the remaining patients being excluded due to lack of ICU admission (n = 142), late start of thromboprophylaxis regime >48 h (n = 57), anticoagulant contraindications (n = 6), mild/moderate COVID-19 cases (n = 10), and on VTE-induced medicine (n = 6). Table 1 summarizes the main baseline characteristics of the standard dose group (n = 192), intermediate-dose group (n = 180), and high dose group (n = 179). A total of 112 (58%) patients out of the 192 standard dosing patients received heparin; whereas only 16 (8.9%) received heparin intermediate dosing and only 1 patient received heparin high dosing. None of this cohort received fondaparinux. For the pre-covariate balance of original data, there were explicit differences in the baseline characteristics of age (P = 0.032), gender (P = 0.017), ethnicity (P = 0.006), kidney function (P < 0.001) APACHE II score (P = 0.009), WHO severity (P < 0.001), mechanical ventilation (P = 0.001), and medications such as intravenous steroid (P = 0.001) and carbapenem (P = 0.021). Additionally, groups differed in the following laboratory variables: d-dimer (P = 0.014) and APTT (P < 0.001). Missing data were highest for fibrinogen (37.9%), followed by APACHE II score and D-dimer (7–10%) (Table 1, footnote).
After applying the weights matching procedure, all covariates of interest were adequately balanced (Table 2; Figure 2). We illustrated the propensity score distribution using a Love plot for absolute standardized mean difference (SMD) distribution (Figure 2). Most patients (>60%) had D-dimer >1.5 μg/ml (Supplementary Table S1).
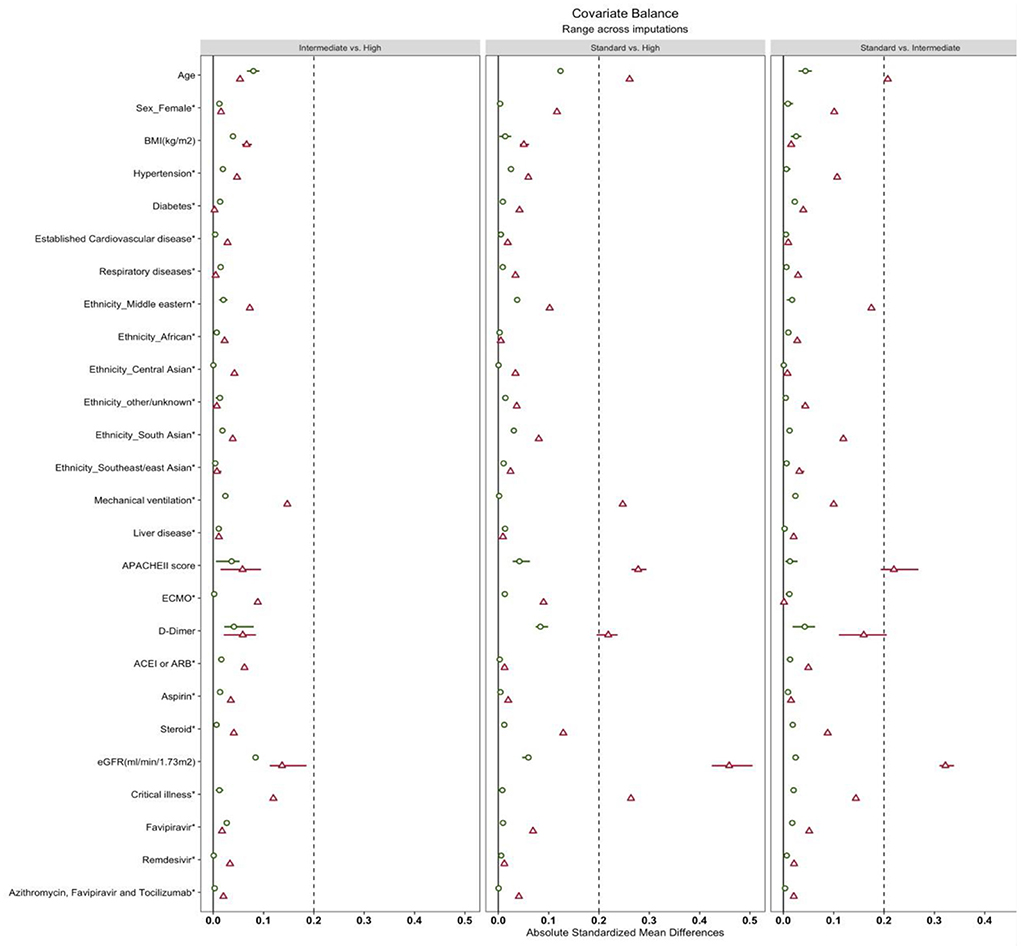
Figure 2. Love plots of covariate balance. BMI, body mass index. Established cardiovascular disease was defined as a documented history of stable angina, unstable angina, percutaneous coronary intervention (PCI), coronary artery bypass graft surgery, myocardial infarction (MI), heart failure or cerebrovascular disease included transient ischemic attack (TIA) or stroke. Respiratory disease: asthma or chronic obstructive pulmonary disease (COPD), the Acute Physiology and Chronic Health Evaluation (APACHE II). ECMO, extracorporeal membrane oxygenation; ACEI, angiotensin-converting enzyme inhibitors; ARB, angiotensin receptor blockers; eGFR, estimated Glomerular Filtration Rate.
Thrombotic events and mortality outcomes
After weights matching, the risk of a composite of thrombotic events was not significantly different between the standard-dose and intermediate-dose groups {21.6 vs. 25.2%; adjusted hazard ratio (aHR) = 1.4, [95% confidence of interval (CI), 0.88–2.33]} (Table 3; Figure 3A). Also, the standard-dose group was associated with a similar hazard of thrombotic events to the high-dose group [21.6 vs. 28.8%; aHR = 1.3 (95% CI, 0.83–2.20)] (Table 3; Figure 3A). Furthermore, each component of the composite outcome has comparative events in the three different prophylactic anticoagulant intensities (Table 3). A subgroup of patients having a D-dimer of >1.5 vs. <1.5 g/ml were associated with similar composite thrombotic events (Supplementary Table S1). For secondary outcome, patients on standard-dose and intermediate-dose arms had comparable overall in-hospital mortality [51.0 vs. 53.4%; aHR = 1.2 (95% CI, 0.88 to 1.72)] (Table 3; Figure 3B). Similarly, we found in-hospital mortality occurred more frequently in the high-dose groups (61.1%) than in the standard-dose group (51.0%), although the findings were not statistically significant [aHR = 1.3 (95% CI, 0.92–1.74)] (Table 3; Figure 3B). When the unadjusted hazard ratio was considered, analyses revealed no differences in primary outcome and mortality (Supplementary Figures S1A,B).
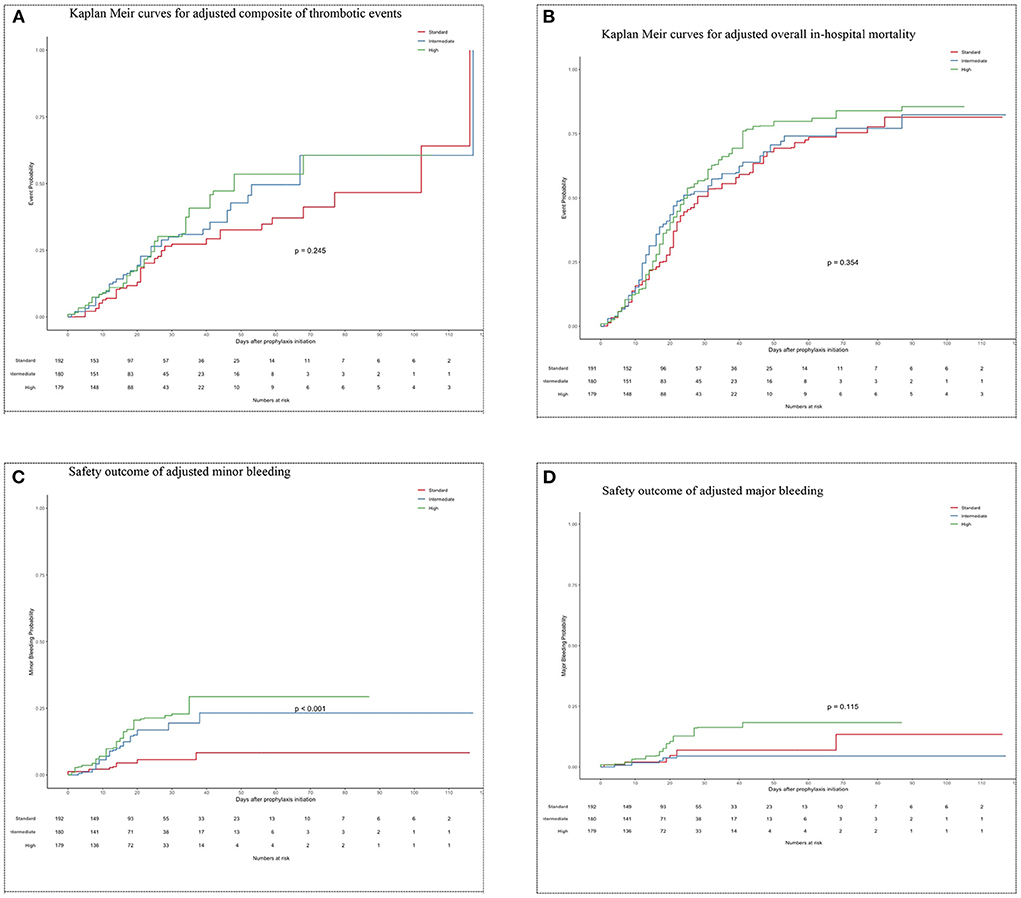
Figure 3. (A) Kaplan Meir curves for adjusted composite of thrombotic events. (B) Kaplan Meir curves for adjusted overall in-hospital mortality. (C) Safety outcome of adjusted minor bleeding. (D) Safety outcome of adjusted major bleeding.
Bleeding outcome
Minor bleeding in the intermediate-dose group (12.6%) was higher compared to the standard-dose group (4.9%) [aHR = 2.9 (95% CI, 1.26–6.80)] (Table 3; Figure 3C). Also, higher proportion of patients experienced minor bleeding in high-dose group (17.3%) vs. the standard-dose group (4.9%) [aHR = 3.9 (95% CI, 1.73–8.76)] (Table 3; Figure 3C). No significant differences regarding major bleeding were observed between the 3 study groups (standard: 4.9 vs. intermediate: 2.9 vs. high: 8.6%) (Table 3; Figure 3D). The unadjusted analysis can be seen in Table 3 and Supplementary Figures S1C,D).
Discussion
This retrospective cohort study compared three different thromboprophylactic regimens in COVID-19 ICU patients. We found that these three regimens (standard-dose, intermediate-dose, and high-dose groups) had comparable primary composite of thrombotic events, including the major components. Furthermore, no significant differences were observed between the three study groups with respect to hospital mortality. Those who received intermediate-dose and high-dose groups had a similar frequency of major bleeding events as those who received standard-dose. However, there were more minor bleeding events in the intermediate-dose and high-dose groups compared with the standard-dose group.
The effectiveness of anticoagulant dose escalation in COVID-19 ICU patients continues to be debated, even among RCT studies. Our findings are in line with the hypothesis that high-dose thromboprophylaxis has a similar composite of thrombotic events in critically ill patients with COVID-19 as intermediate-dose and standard-dose thromboprophylaxis regimens (29, 31, 32). In addition, parallel to observational and RCT studies (29, 31, 32, 57, 58), different thromboprophylaxis doses did not eliminate the risk of overall mortality. This cast doubt on other studies that show a superior survival rate with escalated doses (37, 59, 60). Of note, a meta-analysis of observational studies, has also confirmed that increasing the anticoagulation regimen to the therapeutic dose, resulted in an increase in bleeding events (28).
To the best of our knowledge, only one study investigated the efficacy of three different anticoagulation dose regimens in COVID-19-infected critically ill patients (60). It concluded that high-dose thromboprophylaxis was associated with a lower risk of cumulative incidence of thromboembolic events and fewer bleeding events compared with lower doses. However, the study's findings should be interpreted with caution because of study limitations that may lead to misleading estimates of treatment effect. This includes small sample size and that almost half (45.4%) of patients underwent dose adjustment of the anticoagulant during ICU stay (60).
There are several possible explanations for the high incidence of venous thromboembolism (VTE) in the ICU population. One possible explanation is that the bioavailability of subcutaneous thromboprophylaxis is reduced, especially in edematous patients or those who receive vasoactive medications concurrently, thereby potentially providing reduced efficacy (61). Another possibility is low cardiac output in a population with pre-existing cardiovascular disease (62). Furthermore, significant VTE occurrence was observed not only with COVID-19, but also with other epidemic respiratory virus infections. When relevant data from previous virus infections is reviewed, it provides a lesson from the past about the magnitude of coagulation disorders' severity when compared to COVID-19 infection. Critically ill patients with H1N1 virus and SARS-CoV-1 showed substantial VTE rates of 44 and 30%, respectively (63, 64).
Some studies speculated that abnormal coagulation parameters, such as elevated D-dimer in COVID-19 (D-dimer > 1.5 μg/ml), were predictors of ICU admission, mortality and the development of VTE (12, 13, 65–67). However, the generalizability of these results is subject to certain limitations. First, those studies were limited by a small sample size, lack of serial D-dimer monitoring, absence of laboratory methodology details for the D-dimer assay, and lack of validation. Also, in one study, continuous D-dimer data was empirically categorized (levels of ≤ 0.5 μg/ml, >0.5 to ≤ 1 μg/ml, and >1 μg/ml) instead of using receiver operating characteristic (ROC) analysis to determine the optimal cutoff predictive value of D-dimer for poor prognosis and mortality (67). Second, the D-dimer assay test is limited by low specificity and high rate of false-positive results in a variety of non-thrombotic conditions, such as inflammation, infection, sepsis, female gender, black race, increased aging, active malignancy, sickle cell disease, lupus, chronic liver disease, trauma or surgical status (68). Third, compared to the current and previous pandemic and epidemic viruses, we noticed that D-dimer levels were elevated in both severely infected COVID-19 and SARS-CoV-1 patients, at 59.6 and 45%, respectively (69, 70). Thus, using D-dimer levels as a marker to inform anticoagulant dosing regimens in ICU patients may be inadequate for clinical decision-making.
The definition of prophylactic anticoagulation intensity played a vital role in determining the extent to which study results would be affected (71). Notably, dosing regimens of anticoagulant varied widely across different studies, where it was defined according to local site protocols or trial protocol (28, 33). The therapeutic dose in the large REMAP-CAP/ACTIV-4a/ATTACC multiplatform trial was defined as to meet the target for aPTT of 1.5 to 2.5 times the upper limit of normal (for unfractionated heparin) or therapeutic anti-Xa levels (for enoxaparin). (32). The study concluded that therapeutic dose was associated with significantly lower rates of VTE and higher rates of major and minor bleeding (32). However, these results were inconsistent with what we found. Considering that thrombotic events were not regularly screened during hospital stay in our institutions, but rather, were prompted by the treating physician upon suspicion. This would underestimate the rate of thrombotic events in our cohort. However, given local protocol-based practice, this would be in line with current COVID-19 treatment guidelines panel recommendations (71).
In addition, we noted in our study significant variations in heparin and enoxaparin proportions between groups. Comparing how each anticoagulant type might affect the magnitude of clinical outcomes was beyond the scope of this study. Additionally, practical constraints might prevent designing different study groups with various anticoagulant dosing regimens and types. Obviously, a still open question is whether the anticoagulant type will affect the efficacy and safety outcomes.
Many studies compare just two different thromboprophylaxis regimens. Our study shares many features with others, but the combination used is unique. First, it represents a comprehensive examination of the most frequent three different thromboprophylaxis dosing regimens used in ICU admitted COVID-19 patients. Being familiar with the same efficacy of three prophylactic-dose regimes empowers clinicians to make decisions and recommend a standard dose of thromboprophylaxis in non-obese ICU COVID-19 patients. Second, this study was conducted at 4 centers (multicenter) in two Saudi Arabian cities. Third, in our analysis, we considered all possible covariates that may influence the thrombotic events and mortality findings for ICU patients when developing the Cox regression prediction model. Fourth, this study offers valuable insight into high-risk patients, such as those with elevated D-dimer levels, with patients receiving standard, intermediate, and high-dose thromboprophylaxis, having mean D-dimer baselines of 5.65, 6.44, and 7.54 μg/ml, respectively.
The American Society of Hematology guidelines and Saudi Critical Care Society practice guidelines suggest using the standard prophylactic dose for adults with critical COVID-19 who had no clinical suspicion of VTE (72, 73). However, our findings may counter the uncertainty about using standard prophylactic dose in patients with high suspicion of VTE, namely those with D-dimer level >1.5 μg/ml (in >60% of our cohort). More research using randomized controlled trials is needed to investigate the efficacy and safety of three different anticoagulation regimens in critically ill COVID-19 patients with D-dimer levels >1.5 μg/ml.
The present study was subject to a several potential weaknesses. First, it was an observational cohort study, which could have included unmeasured confounding factors that could not be accounted for without a randomized study design. Second, despite many of our patients having D-dimer levels above 1.5 μg/ml, around 30–35% of each group had D-dimer levels <1.5 μg/ml, which gives further caution on the generalizability of these findings in this populations of patients. Lastly, missing data could bias our estimates, despite using the powerful statistical tool MICE.
Conclusion
This current study shows that standard, intermediate, high anticoagulation dose targets for thromboprophylaxis of critically ill COVID-19 patients have a comparable composite of thrombotic events and mortality. An escalated dose of thromboprophylaxis (intermediate and high dose) could increase the rate of minor bleeding but not major bleeding when compared to the standard dose. Thus, these data recommend the standard-dose as preferred regimen.
Data availability statement
The original contributions presented in the study are included in the article/Supplementary material, further inquiries can be directed to the corresponding author/s.
Ethics statement
The study approval was granted by the Institutional Review Boards at KFMC and PMAH (IRB: 20-666), KSMC (IRB: H1RI-16-Nov20-01), and Almoosa Hospital (IRB: ARC-20-12-4). Written informed consent for participation was not required for this study in accordance with the national legislation and the institutional requirements.
Author contributions
Conceptualization was done by AAlr, YM, and AAlam. Statistics, methodology, software, and interpretation of the results were done by AAlam and AAlr. Study supervision was done by PC. Consultation on statistics was provided by PC and IA. The first draft of the paper was done and the abstract was drafted by AAlr. All authors contributed to the final version of the manuscript.
Acknowledgments
The authors would like to thank the Research Center at King Fahd Medical City, Riyadh, for their valuable technical support provided for the manuscript. This publication was supported by the Deanship of Scientific Research at Prince Sattam Bin Abdulaziz University.
Conflict of interest
The authors declare that the research was conducted in the absence of any commercial or financial relationships that could be construed as a potential conflict of interest.
Publisher's note
All claims expressed in this article are solely those of the authors and do not necessarily represent those of their affiliated organizations, or those of the publisher, the editors and the reviewers. Any product that may be evaluated in this article, or claim that may be made by its manufacturer, is not guaranteed or endorsed by the publisher.
Supplementary material
The Supplementary Material for this article can be found online at: https://www.frontiersin.org/articles/10.3389/fcvm.2022.978420/full#supplementary-material
References
1. Lu R, Zhao X, Li J, Niu P, Yang B, Wu H, et al. Genomic characterisation and epidemiology of 2019 novel coronavirus: implications for virus origins and receptor binding. Lancet. (2020) 395:565–74. doi: 10.1016/S0140-6736(20)30251-8
2. Zhu N, Zhang D, Wang W, Li X, Yang B, Song J, et al. A novel coronavirus from patients with pneumonia in China (2019). N Engl J Med. (2020) 382:727–733. doi: 10.1056/NEJMoa2001017
3. Gupta A, Madhavan MV, Sehgal K, Nair N, Mahajan S, Sehrawat TS, et al. Extrapulmonary manifestations of COVID-19. Nat Med. (2020) 26:1017–32. doi: 10.1038/s41591-020-0968-3
4. Wichmann D, Sperhake JP, Lütgehetmann M, Steurer S, Edler C, Heinemann A, et al. Autopsy findings and venous thromboembolism in patients with COVID-19: a prospective cohort study. Ann Intern Med. (2020) 173:268–277. doi: 10.7326/L20-1206
5. Helms J, Tacquard C, Severac F, Leonard-Lorant I, Ohana M, Delabranche X, et al. High risk of thrombosis in patients with severe SARS-CoVour-2 infection: a multicenter prospective cohort study. Intensive Care Med. 46, 1089–98. (2020). doi: 10.1007/s00134-020-06062-x
6. Llitjos JF, Leclerc M, Chochois C, Monsallier JM, Ramakers M, Auvray M, et al. High incidence of venous thromboembolic events in anticoagulated severe COVID-19 patients. J Thromb Haemost. (2020) 18:1743–6. doi: 10.1111/jth.14869
7. Klok FA, Kruip MJHA, Van der Meer NJM, Arbous MS, Gommers DAMPJ, Kant KM, et al. Incidence of thrombotic complications in critically ill ICU patients with COVID-19. Thromb Res. (2020) 191:145–7. doi: 10.1016/j.thromres.2020.04.013
8. Middeldorp S, Coppens M, van Haaps TF, Foppen M, Vlaar AP, Müller MC, et al. Incidence of venous thromboembolism in hospitalized patients with COVID-19. J Thromb Haemost. (2020) 18:1995–2002. doi: 10.20944/preprints202004.0345.v1
9. Klok FA, Kruip MJHA, Van der Meer NJM, Arbous MS, Gommers DAMPJ, Kant KM, et al. Confirmation of the high cumulative incidence of thrombotic complications in critically ill ICU patients with COVID-19: an updated analysis. Thromb Res. (2020) 191:148–50. doi: 10.1016/j.thromres.2020.04.041
10. Mansory EM, Srigunapalan S, Lazo-Langner Venous A. thromboembolism in hospitalized critical and noncritical COVID-19 patients: a systematic review and meta-analysis. TH Open. (2021) 5:e286–94. doi: 10.1055/s-0041-1730967
11. Zhang R, Ni L, Di X, Wang X, Ma B, Niu S, et al. Systematic review and meta-analysis of the prevalence of venous thromboembolic events in novel coronavirus disease-2019 patients. J Vasc Surgery Venous Lymphat Disord. (2021) 9:289. doi: 10.1016/j.jvsv.2020.11.023
12. Cui S, Chen S, Li X, Liu S, Wang Prevalence F. of venous thromboembolism in patients with severe novel coronavirus pneumonia. J Thromb Haemost. (2020) 18:1421–1424. doi: 10.1111/jth.14830
13. Tang N, Li D, Wang X, Sun Abnormal Z. coagulation parameters are associated with poor prognosis in patients with novel coronavirus pneumonia. J Thromb Haemost. (2020) 18:844–7. doi: 10.1111/jth.14768
14. Liao SC, Shao SC, Chen YT, Chen YC, Hung M-J. Incidence and mortality of pulmonary embolism in COVID-19: a systematic review and meta-analysis. Crit Care. (2020) 24:1–5. doi: 10.1186/s13054-020-03175-z
15. Spyropoulos AC, Levy JH, Ageno W, Connors JM, Hunt BJ, Iba T, et al. Subcommittee on perioperative, critical care thrombosis, haemostasis of the scientific, standardization committee of the international society on thrombosis and haemostasis. Scientific and standardization committee communication: clinical guidance on the diagnosis, prevention, and treatment of venous thromboembolism in hospitalized patients with COVID-19. J Thromb Haemost. (2020) 18:1859–65. doi: 10.1111/jth.14929
16. Marietta M, Ageno W, Artoni A, Candia E, Gresele P, Marchetti M, et al. COVID-19 and haemostasis: a position paper from Italian Society on Thrombosis and Haemostasis (SISET). Blood Transfus. (2020) 18:167. doi: 10.2450/2020.0083-20
17. Poissy J, Goutay J, Caplan M, Parmentier E, Duburcq T, Lassalle F, et al. Pulmonary embolism in COVID-19 patients: awareness of an increased prevalence. Circulation. (2020) 142:184–6. doi: 10.1161/CIRCULATIONAHA.120.047430
18. Cattaneo M, Bertinato EM, Birocchi S, Brizio C, Malavolta D, Manzoni M, et al. Pulmonary embolism or pulmonary thrombosis in COVID-19? Is the recommendation to use high-dose heparin for thromboprophylaxis justified? Thromb Haemost. (2020) 120:1230–2. doi: 10.1055/s-0040-1712097
19. Hamming I, Timens W, Bulthuis MLC, Lely AT, van Navis GJ, van Goor H, et al. Tissue distribution of ACE2 protein, the functional receptor for SARS coronavirus. A first step in understanding SARS pathogenesis. J Pathol Soc Gt Britain Ireland. (2004) 203:631–7. doi: 10.1002/path.1570
20. Jackson SP, Darbousset R, Schoenwaelder Thromboinflammation SM. challenges of therapeutically targeting coagulation and other host defense mechanisms. Blood. (2019) 133:906–18. doi: 10.1182/blood-2018-11-882993
21. Subramaniam S, Jurk K, Hobohm L, Jäckel S, Saffarzadeh M, Schwierczek K, et al. Distinct contributions of complement factors to platelet activation and fibrin formation in venous thrombus development. Blood. (2017) 129:2291–302. doi: 10.1182/blood-2016-11-749879
22. Cook D, Attia J, Weaver B, McDonald E, Meade M, Crowther Venous M, et al. thromboembolic disease: an observational study in medical-surgical intensive care unit patients. J Crit Care. (2000) 15:127–32. doi: 10.1053/jcrc.2000.19224
23. Minet C, Lugosi M, Savoye PY, Menez C, Ruckly S, Bonadona A, et al. Pulmonary embolism in mechanically ventilated patients requiring computed tomography: prevalence, risk factors, and outcome. Crit Care Med. (2012) 40:3202–8. doi: 10.1097/CCM.0b013e318265e461
24. Geerts WH, Bergqvist D, Pineo GF, Heit JA, Samama CM, Lassen MR, et al. Prevention of venous thromboembolism: American College of Chest Physicians evidence-based clinical practice guidelines. Chest. (2008) 133:381S−453S. doi: 10.1378/chest.08-0656
25. Cook D, Crowther M, Meade M, Rabbat C, Griffith L, Schiff D, et al. Deep venous thrombosis in medical-surgical critically ill patients: prevalence, incidence, and risk factors. Crit Care Med. (2005) 33:1565–71. doi: 10.1097/01.CCM.0000171207.95319.B2
26. Yao Y, Cao J, Wang Q, Shi Q, Liu K, Luo Z, et al. D-dimer as a biomarker for disease severity and mortality in COVID-19 patients: a case control study. J Intensive Care. (2020) 8:1–11. doi: 10.1186/s40560-020-00466-z
27. Zermatten MG, Pantet O, Gomez F, Schneider A, Méan M, Mazzolai L, et al. Utility of D-dimers and intermediate-dose prophylaxis for venous thromboembolism in critically ill patients with COVID-19. Thromb Res. (2020) 196:222–6. doi: 10.1016/j.thromres.2020.08.027
28. Parisi R, Costanzo S, Di Castelnuovo A, De Gaetano G, Donati MB, Iacoviello L. Different anticoagulant regimens, mortality, and bleeding in hospitalized patients with COVID-19: a systematic review and an updated meta-analysis. in Seminars in Thrombosis and Hemostasis. (2021). doi: 10.1055/s-0041-1726034
29. Bikdeli B, Talasaz AH, Rashidi F, Bakhshandeh H, Rafiee F, Rezaeifar P, et al. Intermediate-dose versus standard-dose prophylactic anticoagulation in patients with COVID-19 admitted to the intensive care unit: 90-day results from the inspiration randomized trial. Thromb Haemost. (2021) 122:131–41. doi: 10.1055/a-1485-2372
30. Lopes RD, Furtado RH, Macedo AVS, Bronhara B, Damiani LP, Barbosa LM, et al. Therapeutic versus prophylactic anticoagulation for patients admitted to hospital with COVID-19 and elevated D-dimer concentration (ACTION): an open-label, multicentre, randomised, controlled trial. Lancet. (2021) 397:2253–63. doi: 10.1016/S0140-6736(21)01203-4
31. Perepu US, Chambers I, Wahab A, Ten Eyck P, Wu C, Dayal S, et al. Standard prophylactic versus intermediate dose enoxaparin in adults with severe COVID-19: a multi-center, open-label, randomised controlled trial. Open-Label Randomised Control Trial. (2021) 19:2225–34. doi: 10.2139/ssrn.3840099
32. REMAP-CAP ACTIV-4a and A. Investigators therapeutic anticoagulation with heparin in critically ill patients with COVID-19. N Engl J Med. (2022) 385:777–89. doi: 10.1056/NEJMoa2103417
33. Ortega-Paz L, Galli M, Capodanno D, Franchi F, Rollini F, Bikdeli B, et al. Safety and efficacy of different prophylactic anticoagulation dosing regimens in critically and non-critically ill patients with COVID-19: a systematic review and meta-analysis of randomized controlled trials. Eur Hear J Cardiovasc Pharmacother. (2021) 197:65–68. doi: 10.1093/ehjcvp/pvab070
34. Lynn L, Reyes JA, Hawkins K, Panda A, Linville L, Aldhahri W, et al. The effect of anticoagulation on clinical outcomes in novel Coronavirus (COVID-19) pneumonia in a US cohort. Thromb Res. (2021) 197:65–8. doi: 10.1016/j.thromres.2020.10.031
35. Ayerbe L, Risco C, Ayis S. The association between treatment with heparin and survival in patients with Covid-19. J Thromb Thrombolysis. (2020) 50:298–301. doi: 10.1007/s11239-020-02162-z
36. Bolzetta F, Maselli M, Formilan M, Busonera F, Albanese P, Chiaromanni F, et al. Prophylactic or therapeutic doses of heparins for COVID-19 infection? A retrospective study. Aging Clin Exp Res. (2021) 33:213–7. doi: 10.1007/s40520-020-01750-6
37. Martinelli I, Ciavarella A, Abbattista M, Aliberti S, De Zan V, Folli C, et al. Increasing dosages of low-molecular-weight heparin in hospitalized patients with Covid-19. Intern Emerg Med. (2021) 16:1223–9. doi: 10.1007/s11739-020-02585-9
38. Liu X, Zhang X, Xiao Y, Gao T, Wang G, Wang Z, et al. Heparin-induced thrombocytopenia is associated with a high risk of mortality in critical COVID-19 patients receiving heparin-involved treatment. MedRxiv. (2020). doi: 10.1101/2020.04.23.20076851
39. Tremblay D, Van Gerwen M, Alsen M, Thibaud S, Kessler A, Venugopal S, et al. Impact of anticoagulation prior to COVID-19 infection: a propensity score–matched cohort study. Blood. (2020) 136:144–7. doi: 10.1182/blood.2020006941
40. Nadkarni GN, Lala A, Bagiella E, Chang HL, Moreno PR, Pujadas E, et al. Anticoagulation, bleeding, mortality, and pathology in hospitalized patients with COVID-19. J Am Coll Cardiol. (2020) 76:1815–26. doi: 10.1016/j.jacc.2020.08.041
41. Von Elm E, Altman DG, Egger M, Pocock SJ, Gøtzsche PC, Vandenbroucke JP, et al. The strengthening the reporting of observational studies in epidemiology (STROBE) statement: guidelines for reporting observational studies. Bull World Health Organ. (2007) 85:867–72. doi: 10.2471/BLT.07.045120
42. Matt V, Matthew H. The retrospective chart review: important methodological considerations. J Educ Eval Health Prof. (2013) 10:12. doi: 10.3352/jeehp.2013.10.12
43. Harris PA, Taylor R, Thielke R, Payne J, Gonzalez N, Conde JG, et al. Research electronic data capture (REDCap)-a metadata-driven methodology and workflow process for providing translational research informatics support. J Biomed Inform. (2009) 42:377–81. doi: 10.1016/j.jbi.2008.08.010
44. WHO. Clinical Management of Severe Acute Respiratory Infection When Novel Coronavirus (2019-nCoV) Infection is Suspected: Interim Guidance. (2020). Geneva: WHO. p. 21.
45. Elbadawi A, Elgendy IY, Sahai A, Bhandari R, McCarthy M, Gomes M, et al. Incidence and outcomes of thrombotic events in symptomatic patients with COVID-19. Arterioscler Thromb Vasc Biol. (2021) 41:545–7. doi: 10.1161/ATVBAHA.120.315304
46. Roffi M, Patrono C, Collet JP, Mueller C, Valgimigli M, Andreotti F, et al. 2015 ESC Guidelines for the management of acute coronary syndromes in patients presenting without persistent ST-segment elevation. Kardiol Pol (Polish Hear J). (2015) 73:1207–94. doi: 10.5603/KP.2015.0243
47. Schulman S Kearon C Subcommittee Subcommittee on Control of Anticoagulation of the Scientific and Standardization Committee of the International Society on Thrombosis and Haemostasis. Definition of major bleeding in clinical investigations of antihemostatic medicinal products in non-surgical patients. J Thromb Haemost. (2005) 3:692–4. doi: 10.1111/j.1538-7836.2005.01204.x
48. Van Buuren S, Groothuis-Oudshoorn K. Mice: multivariate imputation by chained equations in R. J Stat Softw. (2011) 45:1–67. doi: 10.18637/jss.v045.i03
49. Sterne JA, White IR, Carlin JB, Spratt M, Royston P, Kenward MG, et al. Multiple imputation for missing data in epidemiological and clinical research: potential and pitfalls. BMJ. (2009) 338:b2393. doi: 10.1136/bmj.b2393
50. Yoshida K, Hernández-Díaz S, Solomon DH, Jackson JW, Gagne JJ, Glynn RJ, et al. Matching weights to simultaneously compare three treatment groups: comparison to three-way matching. Epidemiology. (2017) 28:387. doi: 10.1097/EDE.0000000000000627
51. R Core Team. R: A language and environment for statistical computing. R Foundation for Statistical Computing, Vienna, Austria (2013).
52. Therneau TM, Grambsch PM. The cox model. in Modeling Survival Data: Extending the Cox Model. Berlin: Springer (2000), 39–77. doi: 10.1007/978-1-4757-3294-8_3
53. Wickham H. ggplot2: Elegant Graphics for Data Analysis. Berlin: Springer (2016). doi: 10.1007/978-3-319-24277-4
54. Pishgar F, Greifer N, Leyrat CSE. MatchThem: Matching and Weighting Multiply Imputed Datasets. R package version 09, 3. (2020).
55. Greifer N. Cobalt: Covariate Balance Tables Plots. R package cobalt version 4, 3.1 (2021). Available online at: https://ngreifer.github.io/cobalt/ (accessed March 23, 2022).
56. Yoshida K,. Tableone: Create 'Table 1' to Describe Baseline Characteristics with or Without Propensity Score Weights. Version 0, 13.0 (2021). Available online at: https://cran.r-project.org/web/packages/tableone/index.html (accessed March 23, 2022).
57. Lemos AC, do Espírito Santo DA, Salvetti MC, Gilio RN, Agra LB, Pazin-Filho A, Miranda CH. Therapeutic versus prophylactic anticoagulation for severe COVID-19: A randomized phase II clinical trial (HESACOVID). Thromb Res. 196:359–66. doi: 10.1016/j.thromres.2020.09.026
58. Ferguson J, Volk S, Vondracek T, Flanigan J, Chernaik Empiric A. therapeutic anticoagulation and mortality in critically ill patients with respiratory failure from SARS-CoV-2: a retrospective cohort study. J Clin Pharmacol. (2020) 60:1411–5. doi: 10.1002/jcph.1749
59. Trinh MA, Chang DR, Govindarajulu US, Kane E, Fuster V, Kohli-Seth R, et al. Therapeutic anticoagulation is associated with decreased mortality in mechanically ventilated COVID-19 patients. medRxiv. (2020). doi: 10.1101/2020.05.30.20117929
60. Jonmarker S, Hollenberg J, Dahlberg M, Stackelberg O, Litorell J, Everhov AH, et al. Dosing of thromboprophylaxis and mortality in critically ill COVID-19 patients. Crit Care. (2020) 24:1–10. doi: 10.1186/s13054-020-03375-7
61. Dörffler-Melly J, de Jonge E, de Pont AC, Meijers J, Vroom MB, Büller HR, et al. Bioavailability of subcutaneous low-molecular-weight heparin to patients on vasopressors. Lancet. (2002) 359:849–50. doi: 10.1016/S0140-6736(02)07920-5
62. Robinson S, Zincuk A, Strøm T, Larsen TB, Rasmussen B, Toft Enoxaparin P, et al. effective dosage for intensive care patients: double-blinded, randomised clinical trial. Crit Care. (2010) 14:1–7. doi: 10.1186/cc8924
63. Giannis D, Ziogas IA, Gianni Coagulation P. disorders in coronavirus infected patients: COVID-19, SARS-CoV-1, MERS-CoV and lessons from the past. J Clin Virol. (2020) 127:104362. doi: 10.1016/j.jcv.2020.104362
64. Obi AT, Tignanelli CJ, Jacobs BN, Arya S, Park PK, Wakefield TW, et al. Empirical systemic anticoagulation is associated with decreased venous thromboembolism in critically ill influenza A H1N1 acute respiratory distress syndrome patients. J Vasc Surg Venous Lymphat Disord. (2019) 7:317–24. doi: 10.1016/j.jvsv.2018.08.010
65. Miesbach W, Makris M. COVID-19: coagulopathy, risk of thrombosis, and the rationale for anticoagulation. Clin Appl Thromb. (2020) 26:1076029620938149. doi: 10.1177/1076029620938149
66. Jiménez D, García-Sanchez A, Rali P, Muriel A, Bikdeli B, Ruiz-Artacho P, et al. Incidence of VTE and bleeding among hospitalized patients with coronavirus disease 2019: a systematic review and meta-analysis. Chest. (2021) 159:1182–96. doi: 10.1016/j.chest.2020.11.005
67. Zhou F, Yu T, Du R, Fan G, Liu Y, Liu Z, et al. Clinical course and risk factors for mortality of adult inpatients with COVID-19 in Wuhan, China: a retrospective cohort study. Lancet. (2020) 395:1054–62. doi: 10.1016/S0140-6736(20)30566-3
68. Kabrhel C, Mark Courtney D, Camargo CA Jr, Plewa MC, Nordenholz KE, Moore CL, et al. Factors associated with positive D-dimer results in patients evaluated for pulmonary embolism. Acad Emerg Med. (2010) 17:589–97. doi: 10.1111/j.1553-2712.2010.00765.x
69. Guan WJ, Ni ZY, Hu Y, Liang WH, Ou CQ, He JX, et al. China medical treatment expert group for Covid-19. Clin Charact Coronavirus Dis. (2019) 382:1708–20.
70. Lee N, Hui D, Wu A, Chan P, Cameron P, Joynt GM, et al. A major outbreak of severe acute respiratory syndrome in Hong Kong. N Engl J Med. (2003) 348:1986–94. doi: 10.1056/NEJMoa030685
71. COVID-19 Treatment Guidelines Panel,. Coronavirus Disease 2019 (COVID-19) Treatment Guidelines. National Institutes of Health (2022). Available online at: https://www.covid19treatmentguidelines.nih.gov/therapies/antithrombotic-therapy/ (accessed July 12, 2022).
72. Cuker EK, Tseng R, Nieuwlaat P, Angchaisuksiri C, Blair K, Dane K, et al. American Society of Hematology 2021 guidelines on the use of anticoagulation for thromboprophylaxis in patients with COVID-19. Blood Adv. (2021) 5:872–88. doi: 10.1182/bloodadvances.2020003763
Keywords: thromboprophylaxis doses, critically ill patients, COVID-19, mortality, thromboprophylaxis
Citation: Alrashed A, Cahusac P, Mohzari YA, Bamogaddam RF, Alfaifi M, Mathew M, Alrumayyan BF, Alqahtani BF, Alshammari A, AlNekhilan K, Binrokan A, Alamri K, Alshahrani A, Alshahrani S, Alanazi AS, Alhassan BM, Alsaeed A, Almutairi W, Albujaidy A, AlJuaid L, Almalki ZS, Ahmed N, Alajami HN, Aljishi HM, Alsheef M, Alajlan SA, Almutairi F, Alsirhani A, Alotaibi M, Aljaber MA, Bahammam HA, Aldandan H, Almulhim AS, Abraham I and Alamer A (2022) A comparison of three thromboprophylaxis regimens in critically ill COVID-19 patients: An analysis of real-world data. Front. Cardiovasc. Med. 9:978420. doi: 10.3389/fcvm.2022.978420
Received: 26 June 2022; Accepted: 18 July 2022;
Published: 16 August 2022.
Edited by:
Luca Spiezia, University of Padua, ItalyCopyright © 2022 Alrashed, Cahusac, Mohzari, Bamogaddam, Alfaifi, Mathew, Alrumayyan, Alqahtani, Alshammari, AlNekhilan, Binrokan, Alamri, Alshahrani, Alshahrani, Alanazi, Alhassan, Alsaeed, Almutairi, Albujaidy, AlJuaid, Almalki, Ahmed, Alajami, Aljishi, Alsheef, Alajlan, Almutairi, Alsirhani, Alotaibi, Aljaber, Bahammam, Aldandan, Almulhim, Abraham and Alamer. This is an open-access article distributed under the terms of the Creative Commons Attribution License (CC BY). The use, distribution or reproduction in other forums is permitted, provided the original author(s) and the copyright owner(s) are credited and that the original publication in this journal is cited, in accordance with accepted academic practice. No use, distribution or reproduction is permitted which does not comply with these terms.
*Correspondence: Hussain Aldandan, aHVzc2llbi5hbGRhbmRhbkBnbWFpbC5jb20=; Ahmad Alamer, YWEuYWFsbWVyQHBzYXUuZWR1LnNh