- 1Innovative Institute of Chinese Medicine and Pharmacy, Shandong University of Traditional Chinese Medicine, Jinan, Shandong Province, China
- 2International Business School, Tianjin Foreign Studies University, Tianjin, China
- 3Department of Peripheral Vascular Disease, Affiliated Hospital of Shandong University of Traditional Chinese Medicine, Jinan, Shandong Province, China
- 4School of Clinical and Basic Medical Sciences, Shandong First Medical University& Shandong Academy of Medical Sciences, Jinan, Shandong Province, China
Background: Deep vein thrombosis (DVT) is associated with aberrant gene expression that is a common peripheral vascular disease. Here, we aimed to elucidate that the epigenetic modification of forkhead box protein 3 (FOXP3) at the post-transcriptional level, which might be the key trigger leading to the down-regulation of FOXP3 expression in DVT.
Methods: In order to explore the relationship between microRNAs (miRNAs) and FOXP3, mRNA and microRNA microarray analysis were performed. Dual luciferase reporter assay was used to verify the upstream miRNAs of FOXP3. Quantitative real-time polymerase chain reaction, flow cytometry and Western blot were used to detect the relative expression of miR-6132 and FOXP3. Additionally, DVT models were established to investigate the role of miR-6132 by Murine Doppler Ultrasound and Hematoxylin-Eosin staining.
Results: Microarray and flow cytometry results showed that the FOXP3 expression was decreased while miR-6132 level was increased substantially in DVT, and there was significant negative correlation between miR-6132 and FOXP3. Moreover, we discovered that overexpressed miR-6132 reduced FOXP3 expression and aggravated DVT formation, while miR-6132 knockdown increased FOXP3 expression and alleviated DVT formation. Dual luciferase reporter assay validated the direct binding of miR-6132 to FOXP3.
Conclusion: Collectively, our data elucidate a new avenue through which up-regulated miR-6132 contributes to the formation and progression of DVT by inhibiting FOXP3 expression.
Introduction
Deep vein thrombosis (DVT) is the formation or presence of a thrombus in the deep veins, that most commonly occurs in the large veins of the legs or pelvis. Pulmonary embolism (PE) is a terrible complication of DVT and the primary contributor to mortality, PE and DVT are known as venous thromboembolism (VTE) (1, 2). The annual incidence of DVT is about 1 per 1,000 population and it seriously affects the health and quality of patients' life (3, 4). Incidence is steadily rising due to association with many types of risk factors, such as population ageing, genetics, obesity, lifestyle, malignancy, autoimmune diseases, and infection (5, 6). Nevertheless, autoimmune diseases are frequently associated with venous thrombotic events (7). In order to find new targets for the treatment of DVT, it deeply desiderates to reveal the underlying molecular mechanism of DVT formation.
In previous studies, it was found that the proportion of Regulatory T cells (Tregs) subgroups in DVT model rats were significantly reduced (8). Tregs are a subset of T-cells that inhibit immune responses and play an important role in maintaining immune tolerance. Meanwhile, Tregs also can negatively regulate varieties of physiological and pathological immune responses (9). As well known, Tregs express surface molecules of CD4 and CD25, secret anti-inflammatory cytokines represented by IL-10 or TGF-β1 (10, 11). However, the most important transcription factor for Tregs is Forkhead box protein 3 (FOXP3), which plays an important role in their development and differentiation (12). FOXP3 is a 48 kD protein composed of 431 amino acids and is also a member of the fork head transcription factor family (13, 14). The human gene of FOXP3 consists of 11 exons and exists in the p arm of the X chromosome (Xp11.23), while the mouse gene at X A1.1 is equivalent to that of the human gene (15, 16). It has also been confirmed that FOXP3 signaling pathways regulate the process of immune, but the role of FOXP3 and its upstream regulatory factors remain ambiguous in the formation of DVT.
MicroRNAs (miRNAs) are small endogenous noncoding RNAs (typically 21–23 nucleotides in length) that negatively regulate gene expression via recognition of cognate sequences and interference of transcriptional, translational or epigenetic processes (17–19). As posttranscriptional regulators of gene expression, miRNAs have huge potential biological functions in the physiological and pathological processes of various diseases (20, 21). Interestingly, dysregulation of miRNAs expression was shown to be associated with various of cardiovascular diseases, such as atherosclerosis and DVT (22, 23). As a result, we speculated that certain miRNAs acting on FOXP3 might represent novel targets for DVT treatment.
In this study, we observed that FOXP3 expression was decreased and miR-6132 was increased in DVT patients. Notably, miR-6132 bound to its 3′UTR and thus effectively suppressed FOXP3 expression. Meanwhile, we found that increasing miR-6132 could aggravate DVT formation by inhibiting FOXP3 expression, while inhibiting miR-6132 could alleviate the formation of DVT by enhancing FOXP3 expression. Therefore, the newly discovered miR-6132/FOXP3 axis might offer new strategies for DVT treatment.
Methods and materials
Patients
A total of 36 patients diagnosed with symptomatic first idiopathic DVT of the lower limbs, and 36 control subjects in the Affiliated Hospital of Shandong University of Traditional Chinese Medicine from July 2018 to July 2022 were enrolled in this study. The involved DVT patients were made a definite diagnosis by Color Doppler Ultrasound and lower extremity angiography without a history of diabetes mellitus, hypertension or other chronic diseases (Table 1). This study was written informed consent was obtained from all participants and approved by the Ethics Committee of Shandong University of Traditional Chinese Medicine.
Specimen collection
Venous blood samples were drawn in the fasting state for approximately 12 h and the samples were encoded for blind analysis. Peripheral blood mononuclear cells (PBMCs) were separated by Ficoll density-gradient centrifugation.
Microarray analysis
The genome-wide analysis of mRNA and miRNA expression in DVT patients (n = 6) and control subjects (n = 6) were performed using Human mRNA and miRNA (4 × 180 K, Design ID: 084410, 8 × 60 K, Design ID: 070156). Sample labeling, microarray hybridization and washing were performed by OE Biotech. Co., Ltd. (Shanghai, China) according to the manufacturer's standard protocols.
Cell culture and transfection
293T cells were obtained from Procell Life Science & Technology Co., Ltd (Wuhan, China). 293T cells were transfected with miR-6132 mimics, inhibitor or respective negative control (GenePharma, Shanghai, China). The transfected cells were cultured in RPMI 1640 culture medium and harvested for 24 h. The sequences used in this study are listed in Table 2.
Quantitative real-time PCR (qRT-PCR)
Total RNA was extracted using TRIzol Reagent (Invitrogen, Carlsbad, USA) following specification. Approximately 1 µg of RNA was reverse transcribed into cDNA, which was using SYBR Green (Invitrogen, Carlsbad, USA) for qRT-PCR analysis, and the relative mRNA levels were calculated using the 2−ΔΔCt formula. The miRNA primers are designed and synthesized by RiboBio (Guangzhou, China). The PCR primer sequences are shown in Table 3.
Dual-luciferase reporter assay
The FOXP3 wild-type (WT) and FOXP3 mutant (MUT) mRNA 3′UTR luciferase reporter vectors were constructed severally. 293T cells were co-transfected with WT or MUT luciferase reporter plasmids and the miR-6132 mimics or negative controls at an ultimate concentration of 100 nM. Luciferase activity was measured in cell lysates using a dual-luciferase reporter system 24 h after transfection.
Flow cytometric analysis
Cells were resuspended in 1×PBS and stained with the following conjugated antibodies: CD4, FOXP3 (eBioscience, Ben Lomond, CA, USA) or their corresponding isotypic control for 30 min at 4°C in the dark. It was detected using the flow cytometer (Beckman Coulter, CytoFLEX, California, USA). All data were analyzed by using FlowJo _v10.8.1 (Treestar, USA).
Western blotting
Samples were lysed in RIPA buffer with protease and phosphatase inhibitor (Solarbio, Beijing, China), and the proteins were subjected to SDS-PAGE and transferred onto nitrocellulose membranes. Then, the membranes were incubated with diluted primary antibodies followed by horseradish peroxidase HRP-conjugated secondary antibody. Antibodies against FOXP3 (1:1,000, ab215206, Abcam, Cambridge, USA) and GAPDH (1:10,000, ab181603, Abcam, Cambridge, USA) were used in this study.
DVT mice model and treatment
C57BL/6J mice (8-week-old male) were purchased from Beijing HFK Bioscience Company (Beijing, China). Animal experiments were approved by the Institutional Animal Care and Use Committee of Shandong University of Traditional Chinese Medicine. The model of DVT mice was constructed according to the inferior vena cava (IVC) stenosis method, as described above (24).
The mice were stochastically falled into 4 groups (15/group): (1) DVT NC group; (2) DVT miR-6132 mimics group; (3) DVT INC group; (4) DVT miR-6132 inhibitor group.
For FOXP3 inhibitor experiments, mice were stochastically split into 3 groups (15/group): (1) DVT + INC group; (2) DVT miR-6132 inhibitor group; (3) DVT miR-6132 inhibitor + Epirubicin (FOXP3 inhibitor, MCE, USA) group. NC (5 nmol), miR-6132 mimics (5 nmol), INC (10 nmol), or miR-6132 inhibitor (10 nmol) were directly injected into the tail vein before undergoing the surgery, respectively. Epirubicin (0.3 μg/g) was injected into the tail vein before the mice underwent IVC stenosis and continued for three consecutive days (25). The fresh specimen section 2 mm below the IVC ligation site were sectioned for Hematoxylin and eosin (H&E) analysis.
Murine doppler ultrasound
Mice were anesthetized using a mixture of isoflurane-oxygen for Vascular Doppler ultrasonography. Thrombus formation images were obtained using Small Animal Ultrasound Imaging System (VINNO6 LAB, VINNO, Suzhou, China).
Enzyme-linked immunoassay (ELISA)
The protein expressions of endothelial nitric oxide synthase (eNOS) and endothelin-1 (ET-1) were detected by ELISA assay following the manufacturer's instructions with mice ELISA Kits, following the manufacturer's instructions. The absorbance of 450 and 630 nm was measured using a microplate reader.
H&E
After embedding in paraffin, the tissues were cut into 4 µm-thick sections and stained with H&E following standard procedures. All histological images were observed via light microscopy (E100, Nikon, Japan).
Statistical analyses
The results are expressed as the mean ± standard error, and the 2-tailed Student's t test or one-way ANOVA were used for comparisons. Statistical analysis was performed with SPSS 16.0 and GraphPad Prism 8.0 software. The diagnostic value was evaluated by receiver operating characteristic (ROC) curve and correlations were analyzed by Pearson correlation. All experiments were representative of at least three times independently, unless otherwise noted. The values of P < 0.05 were considered statistically significant.
Results
FOXP3 was decreased under the condition of DVT
In order to demonstrate the physiological function and the pathogenesis of DVT, we performed an analysis of the biological processes involved in the downregulation of 1,866 differentially expressed genes (P < 0.05, FC > 1.5) using Gene Ontology (GO) terms. Among the top ten significantly enriched GO terms, we found differential enrichment of pathways involved in T cell activation (Figure 1A). Interestingly, we found FOXP3 was down-regulated in T cell activation pathway (Figure 1B). To identify the role of FOXP3 in the progression of DVT, we first examined the expression of FOXP3 and found that FOXP3 is relatively low expressed in DVT patients and DVT mice (Figures 1C,D,G). Meanwhile, the expression levels of IL-10 and TGF-β1 were also significantly reduced in DVT patients (Figures 1E,F).
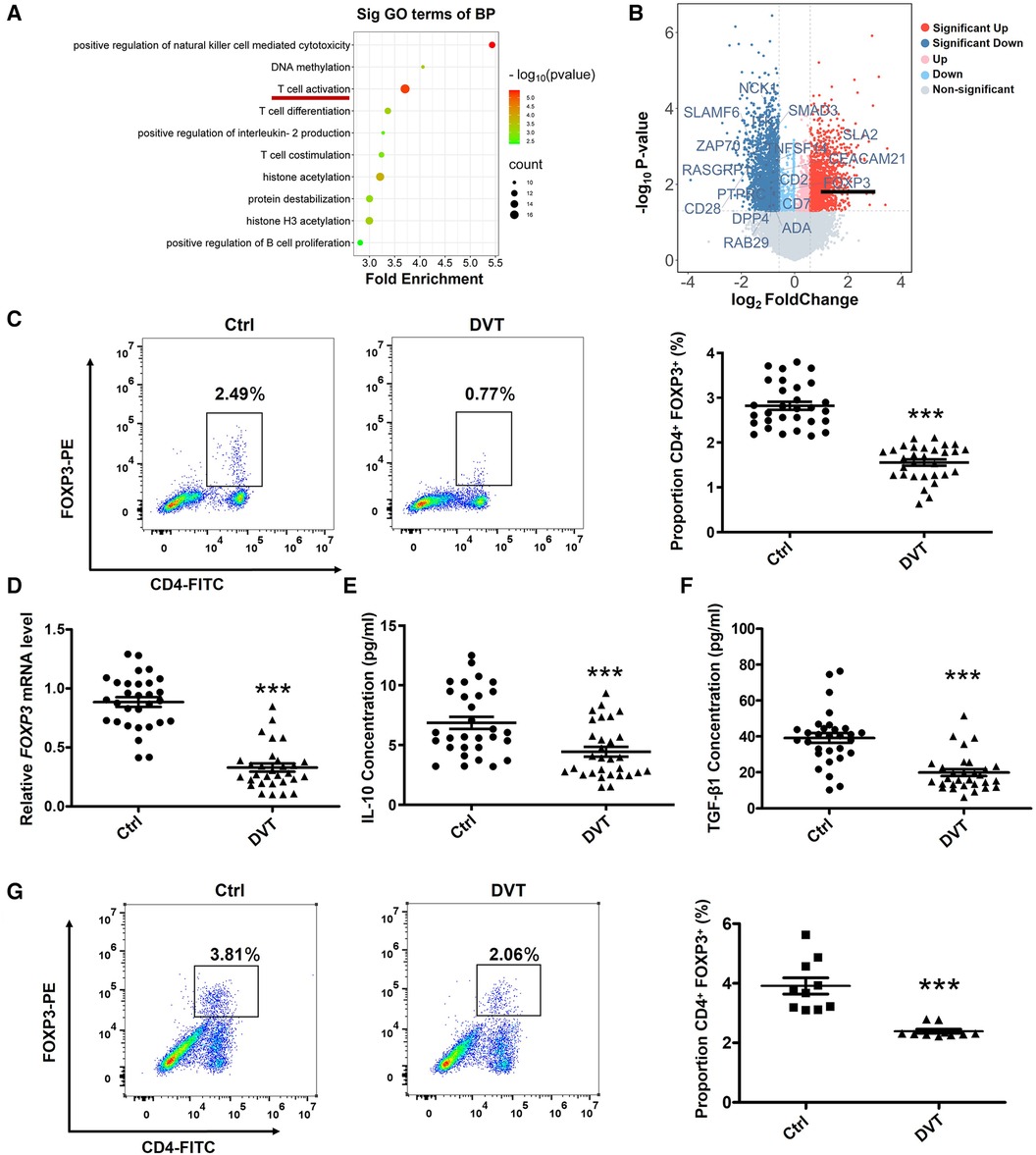
Figure 1. The expression of FOXP3, IL-10 and TGF-β1 are downregulated in DVT patients. (A) The top 10 gene ontology terms of biological process pathway enrichment analyses according to mRNA microarray analysis. (B) Volcano plot showing the profiling data of down-regulated mRNAs in the blood of six DVT patients compared with that of six controls determined by microarray analysis. Red indicates increased relative expression, while blue indicates decreased relative expression. (C,D) Relative expression levels of FOXP3 protein and mRNA in the peripheral blood mononuclear cells (PBMCs) from 30 DVT patients and 30 controls were determined by flow cytometry and quantitative real-time PCR (qRT-PCR). (E,F) IL-10 and TGF-β1 protein levels were determined by ELISA in plasma (n = 30). (G) FOXP3 protein levels were determined by flow cytometry in mice (n = 10). ***P < 0.001.
FOXP3 negatively correlated to miR-6132 in DVT patients
To further confirm the role of FOXP3 and miRNAs in DVT, we performed miRNA expression profiling. The mostly up-regulated 19 miRNAs were detected by at least 1.5-fold with P < 0.01 in DVT (Figure 2A). Here, we intersected the data from the TargetScan database and Chip, and displayed the possible interaction between miR-6132, miR-6785-5p, miR-574-5p, and FOXP3 (Figure 2B). Using amplified samples for validation, miR-6132 was robustly increased in DVT (Figures 2C–E). Next, miR-6132 was negatively correlated with FOXP3 (Figure 2F). With an area under the curve of 0.8989 (95% confidence interval: 0.8036–0.9942), ROC analysis indicated that miR-6132 was sensitive to distinguish DVT patients from healthy control subjects (Figure 2G). Together, our results suggested that miR-6132 and FOXP3 play important roles in DVT.
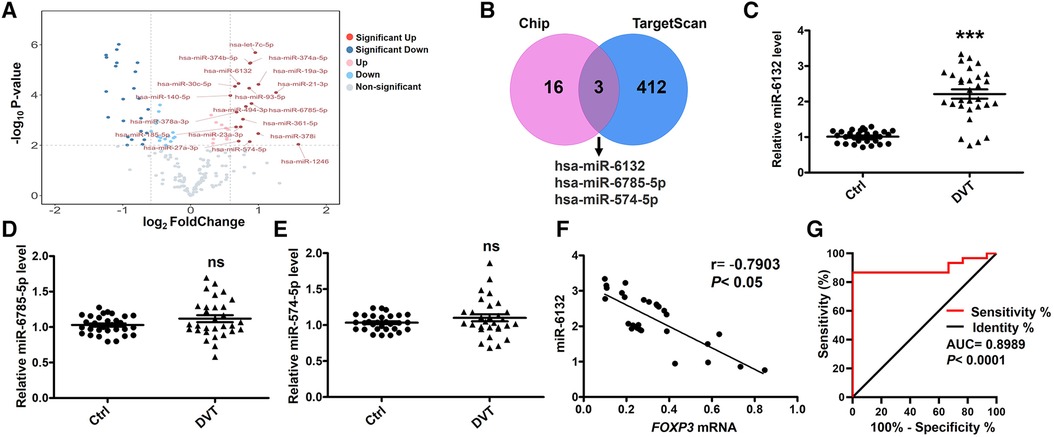
Figure 2. Negative correlation between the levels of miR-6132 and FOXP3 in patients with DVT. (A) Volcano plot showing the profiling data of up-regulated miRNAs in the blood of six DVT patients compared with that of six controls determined by microarray analysis. Red indicates increased relative expression, while blue indicates decreased relative expression. (B) The Venn diagram shows that miR-6132, miR-6785-5p and miR-574-5p were predicted by Targetscan and Chip. (C) Levels of miR-6132, miR-6785-5p and miR-574-5p in PBMCs from DVT patients (n = 30) compared with control subjects (n = 30) were measured by qRT-PCR. (D) The correlation between miR-6132 and FOXP3 was analyzed using Pearson correlation analysis (n = 30). (I) Diagnostic value of miR-6132 for DVT was evaluated by ROC curve. ***P < 0.001.
miR-6132 attenuates the expression of FOXP3 in vitro
Database prediction discovered that miR-6132 shared binding sites with FOXP3 3′UTR (Figure 3A, Supplementary Figure S2A). Based on the dual-luciferase method, miR-6132 mimics significantly repressed luciferase activity of WT FOXP3 3′UTR, whereas did not affect MUT FOXP3 3′UTR (Figure 3B, Supplementary Figure S2B). In addition, we constructed the plasmids of WT 3′UTR and performed luciferase reporter assays to investigate whether miR-6132 might also target other factors closely related to DVT, such as IL-10 or TGF-β1. Notably, miR-6132 mimics could not inhibit the luciferase activity of IL-10 and TGF-β1 (Figures 4A,B). Taken together, our luciferase reporter analysis thus confirmed that FOXP3 is a direct target of miR-6132.
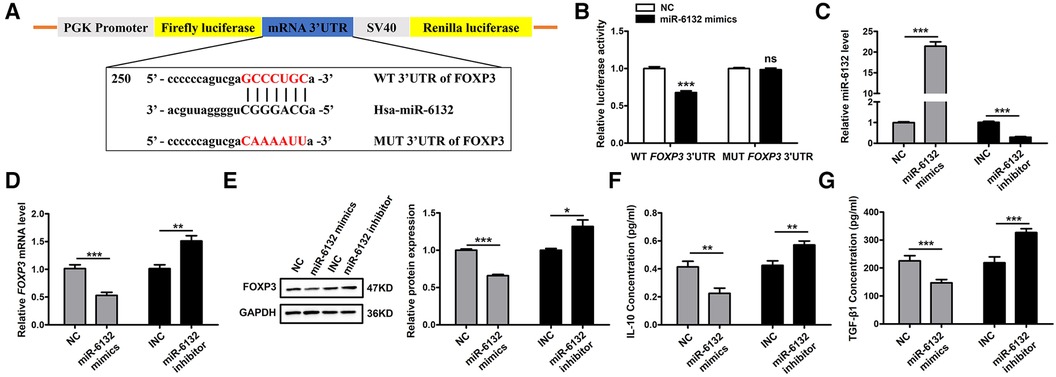
Figure 3. miR-6132 negatively regulates FOXP3 expression in 293T cells. (A) Potential miR-6132 binding sequence in FOXP3 human mRNA 3′UTR. The sequence of the corresponding wild-type (WT) and mutant (MUT) constructs used in the luciferase reporter assay is also indicated. (B) The effect of negative control (NC) or miR-6132 mimics on luciferase activity transfected 293T cells expressing the WT or MUT 3′UTR of human FOXP3. (C) The expression level of miR-6132 after NC, miR-6132 mimics, inhibitor negative control (INC) and miR-6132 inhibitor transfection as detected by qRT-PCR. (D) The expression level of FOXP3 mRNA after NC, miR-6132 mimics, INC and miR-6132 inhibitor transfection as detected by qRT-PCR. (E) The expression level of FOXP3 protein was detected by Western blot. (F,G) The expression levels of IL-10 and TGF-β1 protein were detected by ELISA. Results were quantified by Image J software. *P < 0.05, **P < 0.01, ***P < 0.001.
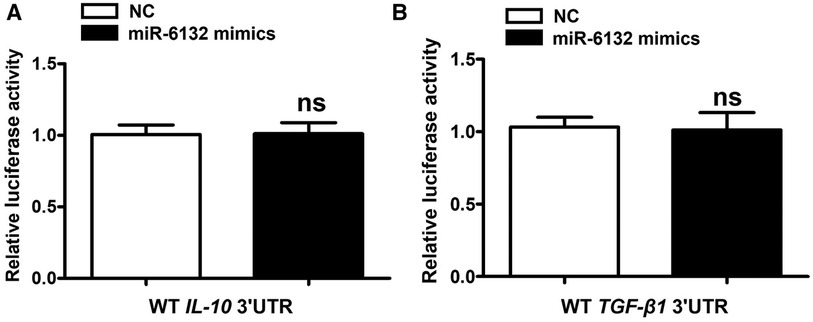
Figure 4. miR-6132 does not target IL-10, or TGF-β1 mRNA 3′UTR. (A,B) The luciferase activity was determined by co-transfecting the vectors (IL-10 3′UTR-WT or TGF-β1 3′UTR-WT) combined with negative control (NC) or miR-6132 mimics into 293T cells.
To further corroborate that FOXP3 inhibition was due to upregulation of miR-6132, we altered miR-6132 expression and determined the expression of FOXP3 in 293T cells. We observed that overexpression of miR-6132 using mimics (Figure 3C) correlated with downregulation of FOXP3 (Figures 3D,E), whereas inhibition of miR-6132 with miR-6132 inhibitor (Figure 3C) resulted in upregulation of FOXP3 (Figures 3D,E). Meanwhile, miR-6132 overexpression decreased the protein levels of IL-10 and TGF-β1 and miR-6132 knockdown promoted the protein levels of IL-10 and TGF-β1 (Figures 3F,G).
miR-6132 inhibits the expression of FOXP3, which in turn affects the formation of DVT
To extend the functional role of miR-6132 in DVT formation, miR-6132 mimics, miR-6132 inhibitor or control vectors were injected via tail vein before DVT surgery. Our results revealed that miR-6132 was significantly increased in DVT miR-6132 mimics group (Figure 5E). Similarly, H&E staining, Doppler, the thrombus length and weight showed that thrombosis was aggravated in the DVT miR-6132 mimics group at 24 h after the ligation (Figures 5A–D). We next found that Foxp3 mRNA and protein decreased in spleen of mice treated with miR-6132 mimics (Figures 5F,G), indicating that miR-6132 could negatively regulate Foxp3 expression. In contrast, miR-6132 was significantly decreased in DVT miR-6132 inhibitor group (Figure 5E), and the thrombus length and weight greatly decreased in the DVT miR-6132 inhibitor group compared with those in DVT INC group (Figures 5A–D). Meanwhile, the expression of Foxp3 increased synchronously in mice treated with miR-6132 inhibitor (Figures 5F,G).
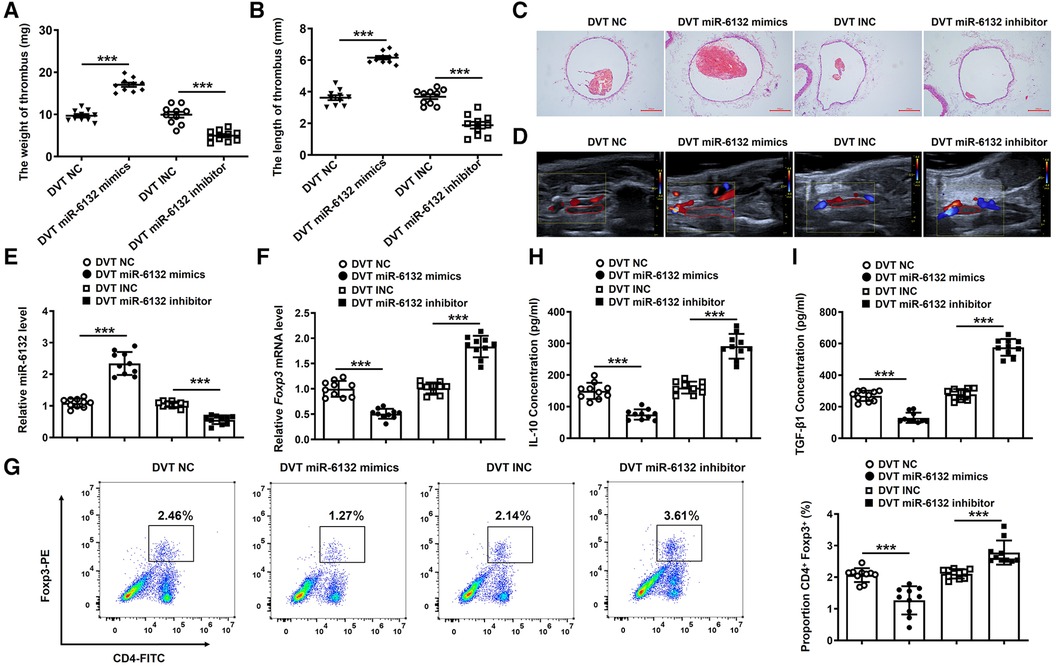
Figure 5. The regulatory effects of miR-6132 on the expression of FOXP3 in DVT formation. (A,B) The thrombosis weight and length in different groups (n = 10). (C,D) Representative images of thrombi in each group detected by H&E staining (magnification, ×100) and vascular ultrasound at 24 h post-operation. Scale bars = 200 μm. (E) Expression of miR-6132 was detected by qRT-PCR in spleen of each treatment group. (F,G) Foxp3 mRNA and protein levels were determined by qRT-PCR in spleen of each treatment group. (H,I) IL-10 and TGF-β1 protein levels were determined by ELISA in plasma of each treatment group. ***P < 0.001.
Moreover, expressions of IL-10, TGF-β1, and eNOS were significantly decreased, whereas ET-1 expression was increased in plasma of mice treated with miR-6132 mimics (Figures 5H,I, 6A,B). At the same time, expressions of IL-10, TGF-β1, and eNOS were obviously increased, whereas ET-1 expression was decreased in DVT miR-6132 inhibitor group (Figures 5H,I, 6A,B). These data indicated that inhibited miR-6132 could enhance FOXP3 expression and attenuate immune response, thereby alleviating endothelial dysfunction.
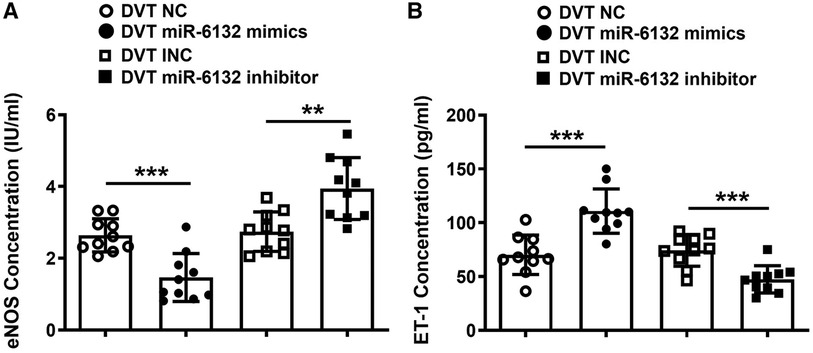
Figure 6. The regulatory effects of miR-6132 on the levels of eNOS and ET-1 were analyzed in plasma. (A,B) Expression of eNOS or ET-1 were determined by ELISA in plasma of each treatment group (n = 10). **P < 0.01, ***P < 0.001.
Neutralization of FOXP3 promoted DVT formation in vivo
Compared with the sham groups, DVT groups showed obvious thrombosis, and IL-10 and TGF-β1 were down-regulated (Supplementary Figure S1A,B). To confirm whether Foxp3 altered the DVT formation, we injected Epirubicin into mice to neutralize Foxp3 function. Notably, the size of thrombus was significantly increased (Figures 7A,B). Analogously, IL-10 and TGF-β1 was significantly decreased after injection of Epirubicin in plasma of DVT mice (Figures 7C,D). These data indicated that FOXP3 attenuated the formation of DVT.
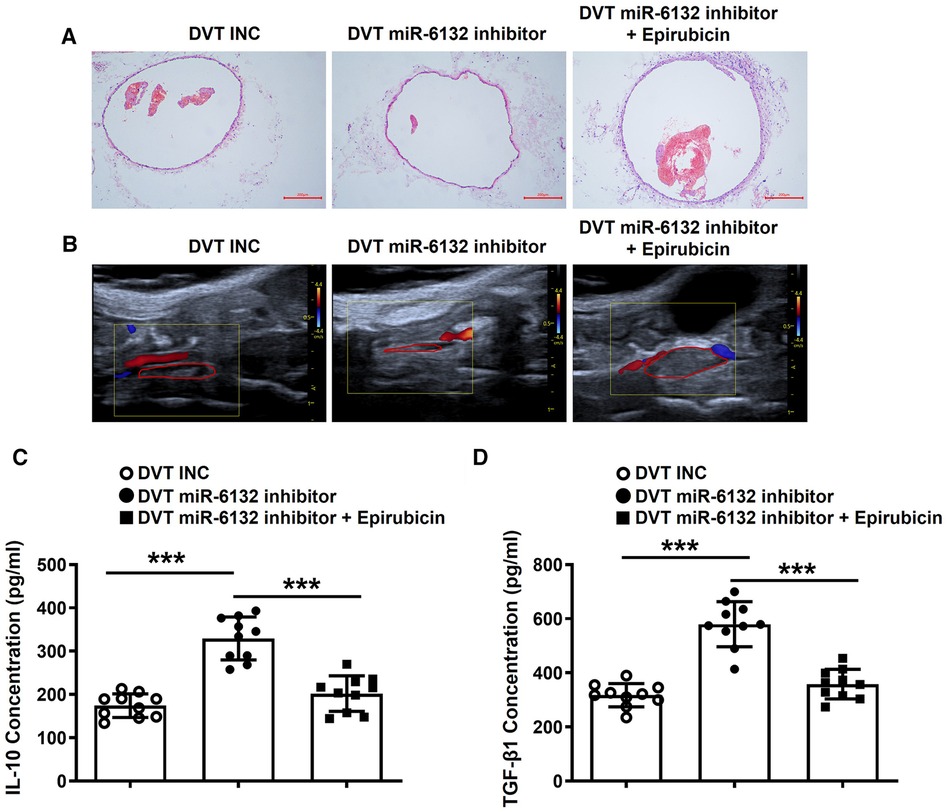
Figure 7. Neutralizing FOXP3 aggravated the formation of DVT in vivo. (A,B) Representative images of thrombi in each group detected by H&E staining and vascular ultrasound at 24 h post-operation (magnification, ×100). Scale bars = 200 μm. (C,D) IL-10 and TGF-β1 protein levels were determined by ELISA in plasma of each treatment group (n = 10). ***P < 0.001.
Discussion
DVT is a process associated with abnormal epigenetic processes, and its pathogenesis involves complex events such as blood flow, blood components, endothelial injury, genetic predisposition, immunity, and inflammatory response (26–30). Despite increasing knowledge of risk factors, one third to one half of venous thromboembolism episodes do not have a clearly provoking cause (31, 32). The latest researches have proved that immune modulation may be involved in the occurrence and prevention of DVT (33). Here, the important role of Tregs in inducing and maintaining of immune homeostasis and tolerance, and Tregs dysfunction can lead to pathology and trigger abnormal immune responses (34). Similarly, Tregs play an indispensable role in many diseases such as autoimmune diseases, infectious diseases, organ transplantation and tumors (35–37). Evidence has demonstrated that the proportion of Tregs subgroups in DVT model rats was significantly reduced (8). Studies have reported FOXP3 has a strong regulatory effect on the function and plasticity of Treg cells, and is the main lineage master regulator for Tregs development and inhibitory activity (38–40). Since FOXP3 is controlled by transcriptional and post-transcriptional mechanisms, inhibition of FOXP3 plays an important role in tissue damage. Analogously, the study provides insights into the role of FOXP3 in DVT through epigenetic modifications.
In the past, related studies have proved the importance of miRNAs in transcriptional regulation, and some miRNAs have been widely used as biomarkers and promising cancer therapeutic targets (41, 42). The pivotal role of miRNAs has become developing new diagnostic and therapeutic tools in in cardiovascular diseases, including DVT (43–45). In our previous research, it had been identified that miR-374b-5p can promote the development of DVT, and miR-338–5p inhibited the formation of DVT (46, 23). Furthermore, the expression of FOXP3 is regulated by several miRNAs such as miR-125b, miR-133a-3p, miR-34a, and miR-210. MiR-125b and miR-133a-3p are overexpressed, thereby reducing the expression of FOXP3 and further inducing autophagy in cancer (47–49). Down-regulation of miR-34a and miR-210 induces the expression of FOXP3, a major regulator that increases Tregs function, thus alleviating allergic diseases (50, 51). Further research is necessary to explore the mechanisms that the role of miRNA regulated FOXP3 in DVT. Through TargetScan database and Chip analysis, we found miRNAs that may have regulatory effects on FOXP3. We further expanded the sample for verification, and finally found that only miR-6132 may have a targeted regulatory relationship with FOXP3.
In many cases, microRNAs derived from the maternal gene, and the process was initiated by Drosha complex. Drosha's cleavage strictly depends on the secondary structure of the stem-loop base of pri-miRNA, as well as the sequence within the 125 nt range on the outer side of the stem-loop base. Therefore, the extremely specific nucleotides lying in the small region of sequence determined the generation of specific microRNAs. Given the large frequency of sequence variation across the species, some microRNA existed in human, but not mice, and hsa-miR-6132 is such a microRNA. To date, a mouse variant of hsa-miR-6132 has not annotated/determined. Although microRNAs are not conserved in mice, the functions of human microRNAs are usually validated in the mice model by acting on the same target gene (52, 53). In this study, we confirmed the regulatory function of hsa-miR-6132 on mouse Foxp3 with the same manner for human gene of FOXP3. To the best of our knowledge, our work provided that decreased miR-6132 increased FOXP3 expression and inhibited DVT formation (Figure 8). Thus, targeting miR-6132 may be a potentially effective strategy for DVT.
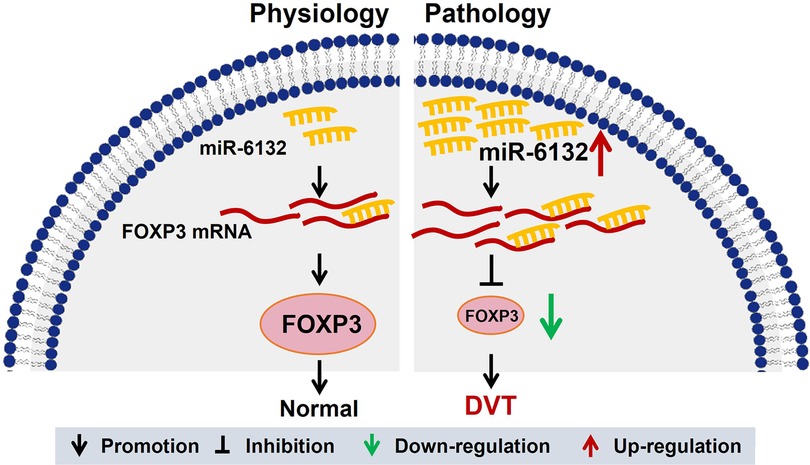
Figure 8. A graphical abstract showing the mechanism of miR-6132 and FOXP3 in DVT. The elevation of miR-6132 inhibited FOXP3 expression in DVT.
To gain a deeper understanding of the biological functions, Gene Ontology terms in Biological Process revealed that the markedly enriched pathways were the T cell activation signaling pathway. Here, our results showed that FOXP3 expression was obviously decreased in DVT. Coincidently, dual luciferase assay demonstrated the immediate bonding of miR-6132 to FOXP3 mRNA 3′UTR. Our pilot study showed that upregulation of miR-6132 could promote DVT formation by inhibiting FOXP3 expression. Based on the above findings, we further studied that inhibition of FOXP3 mRNA and protein levels by overexpression of miR-6132. Similarly, inhibition of miR-6132 could attenuate the formation of DVT by enhancing FOXP3 expression.
In conclusion, we first demonstrated that miR-6132 could contribute to DVT by suppressing the expression of FOXP3. The purpose of the study is to explore the biological role of miR-6132/FOXP3 axis, which will open up new potential marker for the diagnosis and treatment of DVT diseases in the future.
Data availability statement
The original contributions presented in the study are included in the article/Supplementary Materials, further inquiries can be directed to the corresponding authors.
Ethics statement
The studies involving humans were approved by the Ethics Committee of Shandong University of Traditional Chinese Medicine. The studies were conducted in accordance with the local legislation and institutional requirements. The participants provided their written informed consent to participate in this study. The animal study was approved by the Ethics Committee of Shandong University of Traditional Chinese Medicine. The study was conducted in accordance with the local legislation and institutional requirements.
Author contributions
YZ: Methodology, Project administration, Writing – original draft, Data curation, Investigation. ZZ: Conceptualization, Investigation, Writing – original draft. CC: Data curation, Supervision, Writing – original draft. GL: Project administration, Validation, Writing – original draft. NF: Data curation, Formal Analysis, Writing – original draft. HL: Resources, Validation, Writing – original draft. RW: Project administration, Writing – original draft. TZ: Methodology, Writing – original draft. LL: Data curation, Writing – original draft. BW: Funding acquisition, Supervision, Writing – review & editing. XL: Funding acquisition, Writing – review & editing.
Funding
The author(s) declare that financial support was received for the research, authorship, and/or publication of this article.
This work was supported by the Natural Science Foundation of China (82274575), Co-construction project of State Administration of TCM (GZY-KJS-SD-2023-034, GZY-KJS-SD-2023-046), Major Basic Research Project of Natural Science Foundation of Shandong Province (ZR2023ZD56), the Natural Science Foundation of Shandong Province (ZR2022LZY011), the Central Government Guides Local Science and Technology Development Fund Projects of Shandong Province (YDZX20203700001407), Taishan Scholars (Tsqn201812125), National Youth Qihuang Scholar Training Program, Shandong Province Traditional Chinese Medicine High Level Talent Cultivation Project and the Project of Science and Technology of traditional Chinese Medicine of Shandong Province (2021Q084).
Conflict of interest
The authors declare that the research was conducted in the absence of any commercial or financial relationships that could be construed as a potential conflict of interest.
Publisher's note
All claims expressed in this article are solely those of the authors and do not necessarily represent those of their affiliated organizations, or those of the publisher, the editors and the reviewers. Any product that may be evaluated in this article, or claim that may be made by its manufacturer, is not guaranteed or endorsed by the publisher.
Supplementary material
The Supplementary Material for this article can be found online at: https://www.frontiersin.org/articles/10.3389/fcvm.2024.1356286/full#supplementary-material
Supplementary Figure S1
The expression of IL-10 and TGF-β1 in DVT formation. (A) Representative images of thrombi in each group detected by H&E staining and vascular ultrasound at 24 h post-operation (magnification, ×100). Scale bars = 200 μm. (B) The levels of IL-10 and TGF-β1 were detected by ELISA in plasma. ***P < 0.001.
Supplementary Figure S2
Foxp3 was targeted and regulated by miR-6132. (A) Potential miR-6132 binding sequence in mouse Foxp3 mRNA 3′UTR. (B) The effect of negative control (NC) or miR-6132 mimics on luciferase activity expressing the wild-type (WT) and mutant (MUT) 3′UTR of mouse Foxp3. **P < 0.01.
References
1. Nicklas JM, Gordon AE, Henke PK. Resolution of deep venous thrombosis: proposed immune paradigms. Int J Mol Sci. (2020) 21(6):2080. doi: 10.3390/ijms21062080
2. Tritschler T, Kraaijpoel N, Le Gal G, Wells PS. Venous thromboembolism: advances in diagnosis and treatment. JAMA. (2018) 320(15):1583–94. doi: 10.1001/jama.2018.14346
3. Heil J, Miesbach W, Vogl T, Bechstein WO, Reinisch A. Deep vein thrombosis of the upper extremity. Dtsch Arztebl Int. (2017) 114(14):244–9.28446351
4. Stone J, Hangge P, Albadawi H, Wallace A, Shamoun F, Knuttien MG, et al. Deep vein thrombosis: pathogenesis, diagnosis, and medical management. Cardiovasc Diagn Ther. (2017) 7(Suppl 3):S276–84. doi: 10.21037/cdt.2017.09.01
5. Montagnana M, Favaloro EJ, Franchini M, Guidi GC, Lippi G. The role of ethnicity, age and gender in venous thromboembolism. J Thromb Thrombolysis. (2010) 29(4):489–96. doi: 10.1007/s11239-009-0365-8
6. Fuchs TA, Brill A, Wagner DD. Neutrophil extracellular trap (NET) impact on deep vein thrombosis. Arterioscler Thromb Vasc Biol. (2012) 32(8):1777–83. doi: 10.1161/ATVBAHA.111.242859
7. Silvestri E, Scalera A, Emmi G, Squatrito D, Ciucciarelli L, Cenci C, et al. Thrombosis in autoimmune diseases: a role for immunosuppressive treatments? Semin Thromb Hemost. (2016) 42(6):650–61. doi: 10.1055/s-0036-1579642
8. Sun T, Li X, Wang B. Effect of chlorogenic acid on Th17/Treg shift in deep vein thrombasis rats. Shandong Med J. (2015) 55(08):4–6.
9. Sakaguchi S, Ono M, Setoguchi R, Yagi H, Hori S, Fehervari Z, et al. Foxp3+ CD25+ CD4 + natural regulatory T cells in dominant self-tolerance and autoimmune disease. Immunol Rev. (2006) 212:8–27. doi: 10.1111/j.0105-2896.2006.00427.x
10. Talaat RM, Mohamed SF, Bassyouni IH, Raouf AA. Th1/Th2/Th17/Treg cytokine imbalance in systemic lupus erythematosus (SLE) patients: correlation with disease activity. Cytokine. (2015) 72(2):146–53. doi: 10.1016/j.cyto.2014.12.027
11. Trojan K, Zhu L, Aly M, Weimer R, Bulut N, Morath C, et al. Association of peripheral NK cell counts with Helios(+) IFN-gamma(−) T(regs) in patients with good long-term renal allograft function. Clin Exp Immunol. (2017) 188(3):467–79. doi: 10.1111/cei.12945
12. Kim CH. FOXP3 and its role in the immune system. Adv Exp Med Biol. (2009) 665:17–29. doi: 10.1007/978-1-4419-1599-3_2
13. Kronenberg M, Rudensky A. Regulation of immunity by self-reactive T cells. Nature. (2005) 435(7042):598–604.15931212
14. Schubert LA, Jeffery E, Zhang Y, Ramsdell F, Ziegler SF. Scurfin (FOXP3) acts as a repressor of transcription and regulates T cell activation. J Biol Chem. (2001) 276(40):37672–9. doi: 10.1074/jbc.M104521200
15. Bennett CL, Yoshioka R, Kiyosawa H, Barker DF, Fain PR, Shigeoka AO, et al. X-linked syndrome of polyendocrinopathy, immune dysfunction, and diarrhea maps to Xp11.23–Xq13.3. Am J Hum Genet. (2000) 66(2):461–8. doi: 10.1086/302761
16. Bennett CL, Christie J, Ramsdell F, Brunkow ME, Ferguson PJ, Whitesell L, et al. The immune dysregulation, polyendocrinopathy, enteropathy, X-linked syndrome (IPEX) is caused by mutations of FOXP3. Nat Genet. (2001) 27(1):20–21.11137993
18. Chen L, Heikkinen L, Wang C, Yang Y, Sun H, Wong G. Trends in the development of miRNA bioinformatics tools. Brief Bioinform. (2019) 20(5):1836–52. doi: 10.1093/bib/bby054
19. Tiwari A, Mukherjee B, Dixit M. MicroRNA key to angiogenesis regulation: miRNA biology and therapy. Curr Cancer Drug Targets. (2018) 18(3):266–77. doi: 10.2174/1568009617666170630142725
20. Fan Y, Chen H, Huang Z, Zheng H, Zhou J. Emerging role of miRNAs in renal fibrosis. RNA Biol. (2020) 17(1):1–12. doi: 10.1080/15476286.2019.1667215
21. Condrat CE, Thompson DC, Barbu MG, Bugnar OL, Boboc A, Cretoiu D, et al. miRNAs as biomarkers in disease: latest findings regarding their role in diagnosis and prognosis. Cells. (2020) 9(2):276. doi: 10.3390/cells9020276
22. Solly EL, Dimasi CG, Bursill CA, Psaltis PJ, Tan JTM. MicroRNAs as therapeutic targets and clinical biomarkers in atherosclerosis. J Clin Med. (2019) 8(12):2199. doi: 10.3390/jcm8122199
23. Zhang Y, Zhang Z, Wei R, Miao X, Sun S, Liang G, et al. IL (Interleukin)-6 contributes to deep vein thrombosis and is negatively regulated by miR-338-5p. Arterioscler Thromb Vasc Biol. (2020) 40(2):323–34. doi: 10.1161/ATVBAHA.119.313137
24. Schönfelder T, Jäckel S, Wenzel P. Mouse models of deep vein thrombosis. Gefasschirurgie. (2017) 22:28–33. doi: 10.1007/s00772-016-0227-6
25. Kashima H, Momose F, Umehara H, Miyoshi N, Ogo N, Muraoka D, et al. Epirubicin, identified using a novel luciferase reporter assay for Foxp3 inhibitors, inhibits regulatory T cell activity. PLoS One. (2016) 11(6):e0156643. doi: 10.1371/journal.pone.0156643
26. Alessio AM, Beltrame MP, Nascimento MC, Vicente CP, de Godoy JA, Silva JC, et al. Circulating progenitor and mature endothelial cells in deep vein thrombosis. Int J Med Sci. (2013) 10(12):1746–54. doi: 10.7150/ijms.6887
27. Branchford BR, Carpenter SL. The role of inflammation in venous thromboembolism. Front Pediatr. (2018) 6:142. doi: 10.3389/fped.2018.00142
28. Roumen-Klappe EM, den Heijer M, van Uum SH, van der Ven-Jongekrijg J, van der Graaf F, Wollersheim H. Inflammatory response in the acute phase of deep vein thrombosis. J Vasc Surg. (2002) 35(4):701–6. doi: 10.1067/mva.2002.121746
29. Plancher KD, Chan JJ, Bishai SK, Silane M, Ibrahim TF, Petterson SC. DVT and pulmonary embolism following knee arthroscopy: the role of genetic predisposition and autoimmune antibodies: a report of 3 cases. JBJS Case Connect. (2020) 10(2):e0514. doi: 10.2106/JBJS.CC.19.00514
30. Koupenova M, Kehrel BE, Corkrey HA, Freedman JE. Thrombosis and platelets: an update. Eur Heart J. (2017) 38(11):785–91.28039338
31. Kearon C, Ageno W, Cannegieter SC, Cosmi B, Geersing GJ, Kyrle PA, et al. Categorization of patients as having provoked or unprovoked venous thromboembolism: guidance from the SSC of ISTH. J Thromb Haemost. (2016) 14(7):1480–3. doi: 10.1111/jth.13336
32. Di Nisio M, van Es N, Büller HR. Deep vein thrombosis and pulmonary embolism. Lancet. (2016) 388(10063):3060–73. doi: 10.1016/S0140-6736(16)30514-1
33. Budnik I, Brill A. Immune factors in deep vein thrombosis initiation. Trends Immunol. (2018) 39(8):610–23. doi: 10.1016/j.it.2018.04.010
34. Meng X, Yang J, Dong M, Zhang K, Tu E, Gao Q, et al. Regulatory T cells in cardiovascular diseases. Nat Rev Cardiol. (2016) 13(3):167–79. doi: 10.1038/nrcardio.2015.169
35. Mukhatayev Z, Ostapchuk YO, Fang D, Le Poole IC. Engineered antigen-specific regulatory T cells for autoimmune skin conditions. Autoimmun Rev. (2021) 20(3):102761. doi: 10.1016/j.autrev.2021.102761
36. Kondĕlková K, Vokurková D, Krejsek J, Borská L, Fiala Z, Ctirad A. Regulatory T cells (TREG) and their roles in immune system with respect to immunopathological disorders. Acta Medica (Hradec Kralove). (2010) 53(2):73–7. doi: 10.14712/18059694.2016.63
37. Du Y, Fang Q, Zheng SG. Regulatory T cells: concept, classification, phenotype, and biological characteristics. Adv Exp Med Biol. (2021) 1278:1–31. doi: 10.1007/978-981-15-6407-9_1
38. Deng G, Song X, Fujimoto S, Piccirillo CA, Nagai Y, Greene MI. Foxp3 post-translational modifications and treg suppressive activity. Front Immunol. (2019) 10:2486.31681337
39. Rudensky AY. Regulatory T cells and Foxp3. Immunol Rev. (2011) 241(1):260–8. doi: 10.1111/j.1600-065X.2011.01018.x
40. Shahneh F, Grill A, Klein M, Frauhammer F, Bopp T, Schäfer K, et al. Specialized regulatory T cells control venous blood clot resolution through SPARC. Blood. (2021) 137(11):1517–26. doi: 10.1182/blood.2020005407
41. Cui X, Liu Y, Sun W, Ding J, Bo X, Wang H. Comprehensive analysis of miRNA-gene regulatory network with clinical significance in human cancers. Sci China Life Sci. (2020) 63(8):1201–12. doi: 10.1007/s11427-019-9667-0
42. Wolska-Gawron K, Bartosińska J, Rusek M, Kowal M, Raczkiewicz D, Krasowska D. Circulating miRNA-181b-5p, miRNA-223-3p, miRNA-210-3p, let 7i-5p, miRNA-21-5p and miRNA-29a-3p in patients with localized scleroderma as potential biomarkers. Sci Rep. (2020) 10(1):20218. doi: 10.1038/s41598-020-76995-2
43. Wojciechowska A, Braniewska A, Kozar-Kamińska K. MicroRNA in cardiovascular biology and disease. Adv Clin Exp Med. (2017) 26(5):865–74. doi: 10.17219/acem/62915
44. Zhou SS, Jin JP, Wang JQ, Zhang ZG, Freedman JH, Zheng Y, et al. miRNAS in cardiovascular diseases: potential biomarkers, therapeutic targets and challenges. Acta Pharmacol Sin. (2018) 39(7):1073–84. doi: 10.1038/aps.2018.30
45. Morelli VM, Brækkan SK, Hansen JB. Role of microRNAs in venous thromboembolism. Int J Mol Sci. (2020) 21(7):2602. doi: 10.3390/ijms21072602
46. Zhang Y, Miao X, Zhang Z, Wei R, Sun S, Liang G, et al. miR-374b-5p is increased in deep vein thrombosis and negatively targets IL-10. J Mol Cell Cardiol. (2020) 144:97–108. doi: 10.1016/j.yjmcc.2020.05.011
47. Xu N, Zhao Y, Bu H, Tan S, Dong G, Liu J, et al. Cochlioquinone derivative CoB1 induces cytostatic autophagy in lung cancer through miRNA-125b and Foxp3. Phytomedicine. (2021) 93:153742. doi: 10.1016/j.phymed.2021.153742
48. Li JP, Zhang HM, Liu MJ, Xiang Y, Li H, Huang F, et al. miR-133a-3p/FOXP3 axis regulates cell proliferation and autophagy in gastric cancer. J Cell Biochem. (2020) 121(5–6):3392–405. doi: 10.1002/jcb.29613
49. Wang S, Wu J, Ren J, Vlantis AC, Li MY, Liu SYW, et al. MicroRNA-125b interacts with Foxp3 to induce autophagy in thyroid cancer. Mol Ther. (2018) 26(9):2295–303. doi: 10.1016/j.ymthe.2018.06.015
50. Alharris E, Alghetaa H, Seth R, Chatterjee S, Singh NP, Nagarkatti M, et al. Resveratrol attenuates allergic asthma and associated inflammation in the lungs through regulation of miRNA-34a that targets FoxP3 in mice. Front Immunol. (2018) 9:2992. doi: 10.3389/fimmu.2018.02992
51. Chen J, Zhao L, Wang D, Xu Y, Gao H, Tan W, et al. Contribution of regulatory T cells to immune tolerance and association of microRNA-210 and Foxp3 in preeclampsia. Mol Med Rep. (2019) 19(2):1150–8.30569125
52. Xu X, Zhu X, Wang C, Li Y, Fan C, Kao X. microRNA-650 promotes inflammation induced apoptosis of intestinal epithelioid cells by targeting NLRP6. Biochem Biophys Res Commun. (2019) 517(4):551–6. doi: 10.1016/j.bbrc.2019.06.077
Keywords: deep vein thrombosis, FOXP3, miR-6132, immune response, post-transcriptional regulation
Citation: Zhang Y, Zhang Z, Li H, Chu C, Liang G, Fan N, Wei R, Zhang T, Li L, Wang B and Li X (2024) Increased miR-6132 promotes deep vein thrombosis formation by downregulating FOXP3 expression. Front. Cardiovasc. Med. 11:1356286. doi: 10.3389/fcvm.2024.1356286
Received: 15 December 2023; Accepted: 8 March 2024;
Published: 20 March 2024.
Edited by:
Cristina Tudoran, Victor Babes University of Medicine and Pharmacy, RomaniaReviewed by:
Coen van Solingen, New York University, United StatesHemant Giri, Excitant Therapeutics LLC, United States
© 2024 Zhang, Zhang, Li, Chu, Liang, Fan, Wei, Zhang, Li, Wang and Li. This is an open-access article distributed under the terms of the Creative Commons Attribution License (CC BY). The use, distribution or reproduction in other forums is permitted, provided the original author(s) and the copyright owner(s) are credited and that the original publication in this journal is cited, in accordance with accepted academic practice. No use, distribution or reproduction is permitted which does not comply with these terms.
*Correspondence: Bin Wang NzEwMDA4NDhAc2R1dGNtZWR1LmNu Xia Li NjAyMzAwMzNAc2R1dGNtLmVkdS5jbg==