- 1Department of Ancient World Studies, Sapienza University of Rome, Rome, Italy
- 2Department of Heritage Research, Ethiopian Heritage Authority, Addis Ababa, Ethiopia
- 3Department of Environmental Biology, Sapienza University of Rome, Rome, Italy
- 4Dipartimento di Scienze della Terra “Ardito Desio,” Università degli Studi di Milano, Milan, Italy
Tracing human and animal mobility behavior, land use, and exploitation strategies through strontium (Sr) isotope analysis is critical for archaeological and palaeoecological research. The development of an 87Sr/86Sr bioavailable baseline map, often termed isoscape, is a prerequisite for interpreting the Sr isotope composition of animal and human remains from an archaeological context. Despite the wealth of archaeological records dispersed across southern Ethiopia, we know little about bioavilable 87Sr/86Sr, which calls for a Sr isoscape to address key archaeological questions. Here, we present the first 87Sr/86Sr isoscape of southern Ethiopia produced using a geostatistical Ordinary Kriging approach through the analysis of water, plants, and soil leachate, combined with previously published datasets. We used the Middle Stone Age (MSA) site of Gotera as a case study and conducted 87Sr/86Sr isotope analysis of faunal tooth enamel. The results show that our novel baseline isoscape displayed heterogeneous 87Sr/86Sr isotope ratios in both the measured and predicted values (ranging from 0.703 to 0.712), consistent with geological units, with an accurate model performance evaluated through Leave-One-Out Cross-Validation (LOOCV) technique. The faunal tooth enamel analysis reveals that the Gotera fauna are of predominantly local origin, suggesting limited mobility and reliance on the exploitation of local resources across the Gotera area. This study on bioavailable isoscape and faunal 87Sr/86Sr isotopes highlights the potential of Sr isotope analysis to reconstruct past mobility patterns, spatial ecologies, and resource utilization strategies in Ethiopia.
1 Introduction
Strontium (Sr) isotope analysis has been increasingly recognized as an invaluable tool for addressing human and animal mobility patterns, seasonality, provenance, human landscape use, and spatial ecology (Ericson, 1985; Balasse et al., 2002; Janzen et al., 2020; Britton et al., 2011; Price et al., 2002; Capo et al., 1998; Alexander Bentley, 2006; Capo et al., 1998; Crowley et al., 2017; Holt et al., 2021; Zaugg et al., 2023; Lazzerini et al., 2021; Pryor et al., 2020). Sr isotope research stems from the premise that the movement of individuals, fauna, and artifacts across the landscape can be traced from geochemical markers sourced from local geologies (Ericson, 1985). The method has advanced over the past two decades (Alexander Bentley, 2006; Britton et al., 2022), with robust spatiotemporal applications, from studies of human evolution to the exploration of modern ecologies of fauna in the African savannah. Sr isotope analysis has been increasingly applied to understand hunter-gatherer land use and faunal mobility patterns in archaeology (Stewart et al., 2020; Copeland et al., 2011, 2016). In the context of emergence of Homo sapiens, Africa has been discussed as a major origin for “Out of Africa” movements (Abbas et al., 2023; Carto et al., 2009), but less explored about novel adaptive capacities and land use strategies that may have impacted these population expansions and behavioral developments using Sr isotope analysis.
Sr is an alkaline earth metal with four isotopes, 84Sr, 86Sr, 88Sr, and 87Sr, with natural abundances of 0.56, 9.87, 82.53, and 7.04%, respectively (Ericson, 1985; Capo et al., 1998; Willmes et al., 2014; Faure and Powell, 1972). All Sr isotopes are stable except for 87Sr, which is formed by the radioactive β-decay of rubidium (87Rb) with a half-life of 4.88 × 1010 years (Capo et al., 1998; Crowley et al., 2017). As Sr2+ and Ca2+ have similar chemical behaviors, Sr substitutes Ca in mineral forms, such as bioapatite (Alexander Bentley, 2006). Owing to the variations in the ages and composition of different rocks, the 87Sr/86Sr ratio exhibits predictable variations across different lithological units and geologies (Holt et al., 2021).
Beyond rocks, Sr is present in all components of soil, particularly in its bioavailable fraction (Capo et al., 1998; Alexander Bentley, 2006). Sr passes from the bedrock, regolith and soil outcropping for migrating into underground water through percolation and pedogenetic and weathering processes. Mineral agents in the subsoil typically determine the amount of Sr in soils, although additional inputs come from rivers, underground water, and atmospheric deposition, such as precipitation, aerosol, sea prays, and pulverized sediments (Ericson, 1985; Alexander Bentley, 2006; Bataille et al., 2018; Holt et al., 2021). Sr is adsorbed by plants from the soil (Bataille et al., 2020; Ericson, 1985; Holt et al., 2021). Plants with shallow root systems tend to reflect Sr ratios corresponding to the one of the uppermost soil horizons, whereas those with deeper roots exhibit isotopic ratios comparable to less superficial horizons and the bedrock (Willmes et al., 2014). Humans and animals consume plants and water and integrate Sr into their tissues through the diet in the food chain (Ericson, 1985; Alexander Bentley, 2006).
Bioavailable Sr isoscape baseline maps are required to track and interpret 87Sr/86Sr isotope ratios in biologically available organisms (Bataille et al., 2020; Britton et al., 2020; Janzen et al., 2020; Holt et al., 2021; Gigante et al., 2023). Thus, Sr isoscapes allow to explicitly predict the spatial distribution of 87Sr/86Sr isotope ratios across landscapes and diverse geolithologies (Bataille et al., 2020). The evaluation of the isotope ratios measured on the individual specimens against the bioavailable isoscape provides clues for the determination of local and non-local individuals by correlating Sr isotope ratios with the bioavailable isoscape (Bataille et al., 2020; Holt et al., 2021; Lazzerini et al., 2021).
For isoscape construction, while most studies employ a combination of different environmental archives, including microfauna, local waters, snails, and soil leachate, recent comparative studies (Crowley et al., 2017; Britton et al., 2020) suggest plant samples show consistent ratios and therefore are preferable for isoscape modeling. Previous studies (Price et al., 2002; Evans et al., 2012) have also highlighted that the Sr isotope composition of micromammals is suitable for establishing a local baseline map because of their localized home range. Further details on the advantages and disadvantages of various Sr archives have been reviewed elsewhere (Crowley et al., 2017; Bataille et al., 2020; Britton et al., 2020; Holt et al., 2021; Montgomery et al., 2010; Montgomery, 2010; Hoppe et al., 2003).
The analysis of Sr isoscape primarily relies on its geological variability which in turn determines the concentration of Sr from the areas of interest. Thus, local geological maps and lithological variations have long been utilized as baselines for Sr isotope studies (Evans et al., 2012; Pryor et al., 2020). However, an empirical study (Britton et al., 2020) has demonstrated that relying on a local geological map may overlook the discrepancies among the different bedrock reservoirs and bioavailable 87Sr/86Sr ratios. The 87Sr/86Sr ratio analyzed from environmental Sr archives including plants, animals, soils, and snails can correlate with the isotope ratios derived from the local lithologies (Holt et al., 2021). Therefore, recent studies have underscored the importance of utilizing a combination of different environmental samples from an area of interest (Bataille et al., 2020; Janzen et al., 2020; Willmes et al., 2021).
Various natural and anthropogenic parameters including sea spray, volcanic ashes, fertilizers, and human settlement can influence Sr isotope ratios (Holt et al., 2021; Britton et al., 2022). Thus, careful consideration of a multitude of factors remains critical while producing a 87Sr/86Sr isoscape for a nuanced understanding of past provenance, ecology, and mobility patterns. In the present study, some of these factors are rarely concerning given that most samples were collected from areas with limited influences of fertilizers, human settlement, and coastal sea spray effects.
Despite recent developments in African archaeology (Janzen et al., 2020; Wang et al., 2023), overall limited attention has been given to Sr isotope research. More recently, Sr isotope research has been utilized for characterizing faunal and hominin mobility behavior in eastern Africa (O'Brien et al., 2023, 2024), and hominin landscape use in southern Africa (Copeland et al., 2011, 2016). Janzen et al. (2020) produced a comprehensive Sr isoscape map of the eastern African fossiliferous and artifact-bearing localities of Kenya and Tanzania through the analysis of modern and archaeological faunal, plant, and water, as well as published Sr data. In a recent study (Wang et al., 2024), a Sr isoscape of sub-Saharan Africa has been produced investigating the geographical origin of enslaved individuals during the transatlantic slave trade. Despite its limited resolution in certain regions such as Ethiopia, the authors have produced a bioavailable isoscape for a large swath of Africa and extensive 87Sr/86Sr dataset through provenance analysis of Sr isotopes.
To date, only a few studies have highlighted the importance of Sr isotopes in addressing key archaeological questions in Ethiopia. Pryor et al. (2020) made the first important contribution in an archaeological context by utilizing 87Sr/86Sr values of human and faunal remains from the medieval site of Harlaa in eastern Ethiopia. The authors demonstrate human and faunal mobility history in the Harlaa area and its surroundings using the 87Sr/86Sr values extracted from previous geological studies. Their work showcases the range of 87Sr/86Sr values of the major geological units and human groups in the medieval period of eastern Ethiopia, demonstrating the wide potential of producing a bioavailable isoscape for the region. Other works focused mainly on the use of Sr isotope ratio as a tracer of major rock outcrops, catchment areas, and hydro-chemical compositions of Rift Valley lakes using samples from several geological units and water bodies (Teklay et al., 1998; Ayalew et al., 1999). These studies have emphasized the 87Sr/86Sr variability of major geological units, lithological characteristic and water sources. Despite their geological significance, these studies lack detailed inferences into the human past and exhibits discrepancies in 87Sr/86Sr across various biological reservoirs. This study builds on this work by presenting a novel baseline map for a key Pleistocene archaeological region of Ethiopia.
Several modeling approaches have been employed to establish a baseline bioavailable isoscape; with the most common ones being geostatistical and machine-learning approaches. Kriging is one of the widely utilized important geostatistical approaches to produce local bioavailable baseline. It relies on statistical models of spatial autocorrelation between pairs of points within a dataset by fitting a curve or “variogram model.” Subsequently, this variogram model serves as the foundation for interpolating the target variable across the entire spatial domain. The primary objective is to establish the output values for unknown locations, thereby facilitating the generation of a continuous surface map (Britton et al., 2020; James, 2022; Lugli et al., 2022; Zaugg et al., 2023). Machine Learning, particularly the Random Forest (RF) regression algorithm has become another popular Sr isoscape approach in archaeological and ecological research (Bataille et al., 2018; Gigante et al., 2023; Wang et al., 2023). RF is an ensemble-based approach that involves averaging deep decision trees and bagging (bootstrap sampling of training data) to reduce the model variance (Bataille et al., 2020; Janzen et al., 2020; Wang et al., 2023).
Southern Ethiopia, with its diverse lithological framework and landforms, has provided rich archaeological and palaeoanthropological records, making it ideal for Sr isotope research. However, the extent and nature of human and animal ecology across time and space, as well as the range of 87Sr/86Sr ratios of each lithology in the region, have yet to be established. Very limited attention has been paid to archaeological research on bioavailable baseline 87Sr/86Sr map and faunal 87Sr/86Sr isotope analysis in the archaeology of Ethiopia.
This study provides a pioneering baseline 87Sr/86Sr model for an area that currently lacks 87Sr/86Sr datasets and a bioavailable isoscape both on local and regional scales. An Ordinary Kriging geostatistical approach is employed through the analysis of plant, water, soil, and micromammal samples collected across several geobiological zones of southern Ethiopia. Additional datasets were extracted from previous studies. We chose the Gotera Middle Stone Age (MSA) site (Fusco et al., 2024) as a case study to conduct Sr isotope analysis on anthropogenically assembled faunal specimens to test assumptions about human and faunal migratory behavior and landscape use during the Late Pleistocene. We wish to emphasize that we are referring to Southern Ethiopia in this context using directional significance instead of political or regional boundaries.
2 Materials and methods
2.1 Geological setting
Southern Ethiopia is a region characterized by a complex and diverse set of rock formation histories (Abbate et al., 2015; Figure 1). The area encompasses part of the East African Rift System (EARS), mainly the Main Ethiopian Rift (MER), with significant varations in the underlying geology, ranging from pre-rifting Precambrian basement rock to the more recently dated Quaternary formations (Abbate et al., 2015). The Precambrian basement is the oldest rock outcrop in the region with lithologies consisting of deformed gneisses, amphibolite, and granulite (Yibas et al., 2002; Emishaw et al., 2017). The East African Orogen with the Arabian-Nubian Shield and the Pan-African Mozambique Belt is represented by the Precambrian of southern Ethiopia. These Precambrian rocks are exposed in the Moyale, Adola and Megado, Yabelo, Negelo, Agere Mariam, and Konso areas dominated by granite-gneiss, ophiolitic fold and thrust-out crops dated between 880 Ma and 526 Ma (Yibas et al., 2002). The rocks are interlayered with Archean to Proterozoic plutonic rocks (Philippon et al., 2014; Abbate et al., 2015). Tholeiitic basalt flows and subordinate tuffs dated between 48 Ma and 30 Ma are exposed above the basement rock in the Gamo and Amaro area (Philippon et al., 2014; Emishaw et al., 2017).
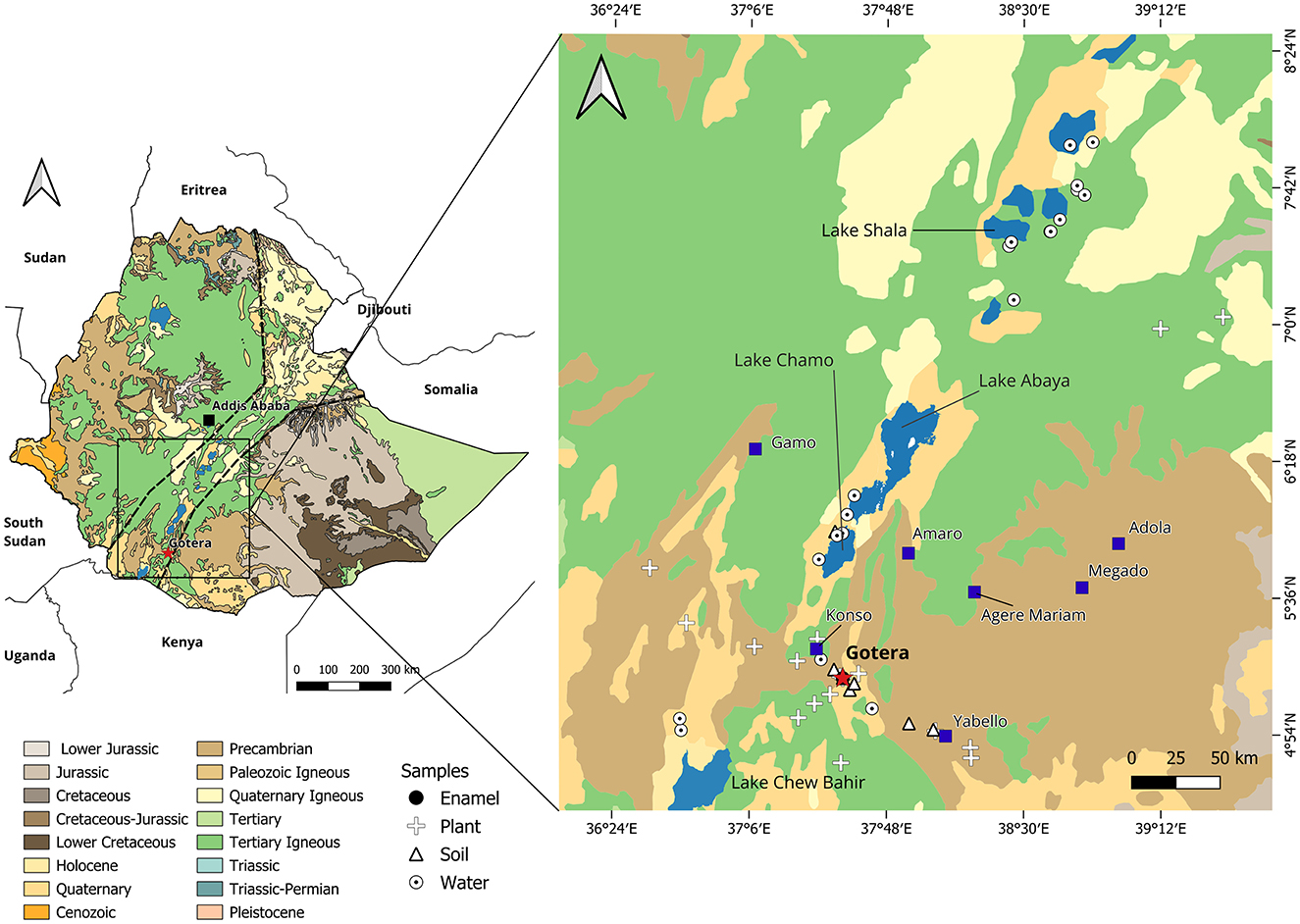
Figure 1. Geological map of southern Ethiopia with the distribution of sampling locations of different Sr archives. The area selected for this study was represented by a rectangle. Sampling locations are represented by different symbols including triangles (soil), plus sign (plants), circles (water), and black dots (tooth enamel). Geological map of Ethiopia modified from the Maps showing geology, oil and gas fields and geological provinces of Africa: U.S. Geological Survey (Persits et al., 1997).
Geomorphological, geochemical and structural investigations have shown the western and eastern MER are dominated by several fault lines. Border faults across the Chew Bahir to Gamo Gofa areas were formed through earlier rifting processes during the Miocene (WoldeGabriel et al., 2000). Volcanism progressively stretched southward from the Afar Depression between Somali and the southern Ethiopian plateau. This continued in correspondence with the formation of the EARS rifting which propagated southward. Rifting in the Broadly Rifted Zones (BRZ) of southern Ethiopia is a northward propagation of the Kenya-Turkana Rift of the southern MER which followed a different trend from the MER (Emishaw et al., 2017). In the northern and central MER, volcanism resumed around 10 Ma with exposed outcrops consisting of late Pliocene volcanic basalts and lacustrine sediments in the areas of Nech Sar, Segen, and Mursi (Teklay et al., 1998; Emishaw et al., 2017).
In the MER, tectonism, volcanism, and rifting have formed diverse sets of lithological features (WoldeGabriel et al., 2000). Inside the rift and along its shoulders, Quaternary formations corresponds to alluvial, fluvial and lake deposits. The MER divides the southern sector into the Chamo and Galana basins to the west and east, respectively, by the Amaro Horst. Outside the Ethiopian rift, Precambrian basement rock and banded gneiss are distributed in the Borena, Yabelo, and Konso areas (WoldeGabriel et al., 2000). Finally, climatic conditions triggered intense weathering of outcropping rock formations and deposits thus promoting the formation of extensive pedological cover.
2.2 Archaeological context
Southern Ethiopia is home to many palaeoanthropological and archaeological sites dating from the Plio-Pleistocene to the Holocene period, offering immense insights into hominin bio-cultural trajectories. Over the last four decades, research in southern Ethiopia has revealed several fossiliferous and artifact-bearing sites. Early palaeontological and palaeoanthropological expeditions took place in the 1970s across the lower Omo, Kibish and Fejej areas in the southernmost Ethio-Kenya border, yielding rich evidence of human bio-cultural evolution and adaptation dating back to the Plio-Pleistocene (Wood and Leakey, 2011; Shea, 2008; Asfaw et al., 1991). The Konso-Gardula Formation in southern Ethiopia has yielded the earliest Acheulean hand axes dated to the early Pleistocene (Asfaw et al., 1992). The region is also known for yielding one of the earliest records of MSA assemblages from the site of Gademotta in the MER (Sahle et al., 2014).
Owing to the rich MSA and Late Stone Age (LSA) cultural and occupational sequence, the southwestern highlands of Ethiopia were hypothesized to have acted as refugia for hunter-gatherers in the backdrop of the Late Pleistocene climatic oscillation (Brandt et al., 2017, 2012; Ossendorf et al., 2019). Evidence of population contact, long-distance raw material procurement, and trade and exchange of artifacts from the area has been documented using geochemical techniques (Brandt et al., 2017). The region has also been considered a corridor for the dispersal of H. sapiens within and beyond Africa (Brandt et al., 2017). Some of the best-known MSA and LSA sites such as Goda Buticha (Pleurdeau et al., 2014), Mochena Borago (Brandt et al., 2012), and Fincha Habera (Ossendorf et al., 2019), are situated in southern, southeastern, and southwestern Ethiopia.
Recent archaeological studies have also documented early and late Holocene occupations in the region (Assefa et al., 2014). Evidence of microlithic artifacts, plant remains and other evidence documented from sites such as Mochena Borago, Dendi and Nech Sar Park provide insights into the subsistence and technologies of hunter-gatherers (Schepers et al., 2020). Several rock art sites dated to the Holocene period are distributed across the southern Ethiopian highlands and lowlands, providing valuable insights into the symbolic and artistic expressions of past societies (Gallinaro and Zerboni, 2021; Assefa et al., 2014; Solomon, 2020). Furthermore, abundant megalithic monuments, stelae and rock art sites are found in the Gurghe, Sidama, and Gedio areas, mainly dating to the medieval period (Duff et al., 2018; Roger and Jean-Paul, 2020).
2.2.1 Case study: the Gotera MSA site
The Gotera area is situated to the southeast of the Sagan River basin, approximately 100 km east of the Chew Bahir palaeolake. This area is part of a large sedimentary basin in the Ethiopian Rift between the Omo-Turkana Basin to the west and the southern section of the MER to the east. The Gotera research area covers a portion of the northeastern Sagan River catchment. The Sagan River follows the Rift from east to west and then flows southward into the Chew-Bahir River (Foerster et al., 2018). The French archaeologist, Jean Chavaillon, initially investigated the area in the 1970s and explored the site for two successive field sessions (Chavaillon and Chavaillon, 1985). The Gotera area, along with the documented archaeological sites and particularly the GOT10 site is currently under investigation by the Italian Archaeological Mission at Gotera (GotAM), a research project run by the Sapienza University of Rome.
Gotera is one of the few unique open-air sites in the MIS3 of eastern Africa providing evidence of lithic technologies, faunal remains, fire places, with human occupational sequences spanning thousands of years (Fusco et al., 2021) in a region broadly assumed to be a structural corridor for human dispersal within and out of Africa. Lithic analysis reveals the use of multiple production methods including Levallois and bipolar on anvils with a late MSA technology (Fusco et al., 2021, 2024). Zooarchaeological evidence attests the exploitation of Suidae, hare and and most notabely, several taxa of Bovidae. The faunal assemblage at Gotera includes diverse range of other species, including small carnivores, micromammals and birds.
The materials for this study were collected through archaeological excavations of the GOT10 site in the Gotera area. Most of the faunas have been recovered from in-situ stratigraphic units, while the remaining faunal specimens are recovered from the surface (Fusco et al., 2024). The GOT10 site is securely dated between 50 and 43ka (Fusco et al., 2024), while chronological frame of other sites in the Gotera area identified through surveys remain yet to be fully assessed, however they fall broadly into the MSA technocomplex.
In the present study, Sr isotope analysis has been undertaken on the fauna consumed by MSA humans to address critical questions about Late Pleistocene human and faunal spatial ecology, and mobility patterns in southern Ethiopia. Gotera's open-air setting is rare for the time-period, offering novel insights into the understanding of Late Pleistocene human behavior and ecological adaptations. The site represents an ideal case study for testing assumptions about hunter-gatherer land-use patterns, subsistence strategies, and mobility behaviors using 87Sr/86Sr isotope analysis. By determining the origin of the Gotera assemblage, the exploitation of local fauna can indicate predictable subsistence and stable ecosystem, while the presence of non-local fauna reflects migration and ecological variability.
2.3 Sampling
In this study, a total of (n = 48) samples including plants (n = 15), water (n = 24), soil (n = 7), and tooth enamel of micromammals (n = 2) were used for building the 87Sr/86Sr isoscape. Meanwhile, a further 35 archaeological fauna specimens were sampled for Sr isotope analysis (Supplementary Table). Plant, soil, water, and enamel samples were collected from southern Ethiopia during 2022 and 2023 field. Sampling surveys were subdivided based on geological formations key to the area of interest, with locations randomly selected across the different lithologies. Soil samples were collected from 15 to 20 cm below the surface. For all samples, we attempted to avoid areas of anthropogenic disturbance and human settlements. For plant samples, grasses and leaves of trees and bushes were targeted. A Garmin ETREX 22x GPS device was used to record points set to the WGS84 reference system for the geographical coordinates. Photographic documentation was produced for each sample and the surrounding area, with additional information such as the type of geological formation and a description of the sample was also being recorded with an iPad. Plant samples were dried in dry air in the Ethiopian Heritage Authority (EHA) lab.
For water sampling, 250 ml LDPE plastic bottles with narrow necks and screw caps were used. Water samples were collected primarily from lakes located in the MER. Additional Sr isotope data (n = 19) of water 87Sr/86Sr values (Rango et al., 2010; Markowska et al., 2022) and (n = 2) plant 87Sr/86Sr values (Ghouri, 2024) were extracted from previous studies.
Stable isotope sampling of fossil tooth enamel powder was conducted at EHA. Preparation was performed following the internal isotope protocols of the Laboratory of Paleoanthropology and Bioarchaeology of the Sapienza University of Rome. Prior to this, each tooth surface was carefully cleaned by gently abrading it with a diamond drill bit (>1.0 mm) to eliminate potential contaminants. Enamel from mammalian tooth was then sampled using a Dremel tool fitted with a diamond-tipped bit (>2.0 mm). Between 7 and 15 mg of enamel powder was extracted for Sr isotope analysis by drilling from the root to the crown of the tooth, ensuring an average dietary and environmental record for the period of enamel formation.
2.4 Analysis
Different sample preparation protocols were involved for soil leachate, plants, water and tooth enamel samples prior to analysis in the column chemistry. Soil samples were ground into powder using a mortar and pestle, weighed, and placed into test tubes. Between 1 and 10 g of samples were weighed and placed in a centrifuge tube. Samples were washed with Milli-Q ultrapure water to remove external dust and placed in crucibles of 50 ml quartz. The samples were subsequently added to 8 mL of 1M acetic acid using a pipette. The samples underwent sonication, followed by 12 h resting period, another round of sonication, centrifugation, and transfer into a clean 15 ml Teflon beaker using a sieve to filter out organic materials. Subsequently, 2 ml of Milli-Q Water was added, followed by further sonication and centrifugation. Finally, the substrate was transferred back into a beaker after another round of sieving. The beaker was then dried on a hotplate at approximately 125–130°C, and 1 ml of 12% hydrogen peroxide (H2O2) was added to eliminate organic materials, followed by another drying cycle. The dry sample was transferred onto weighing paper to determine the weight of the fraction leached from the original sample.
Plant samples were prepared by breaking them into small pieces using pliers over a large clean Kimwipe and placed into 15 mL centrifuge tubes. The centrifuge tubes were labeled with sample names and were placed in an oven at approximately 50°C for 12–24 h. The samples were placed in a crucible, sonicated in Milli-Q water, followed by 3 times rinse with Milli-Q water, and dried for more than 12 h. The samples were then ashed in a Thermo Lindberg/blue MTM Furnace at a different temperature starting at 100°C with gradually increasing temperature until reaching 650°C. The samples were then baked for 2 h to ensure complete combustion. The ashed samples were transferred to 1.5 mL centrifuge tubes and dissolved.
The water sample was filtered and a volume of 10–50 mL of filtered water (0.45 μm filter) was evaporated to dryness in a Teflon beaker at approximately 80°C. The residue was dissolved in 3 M nitric acid (HNO3) to remove any remaining organic material and salts. The solution was then centrifuged to remove any insoluble material prior to the transferring the supernatant to a clean Teflon vial. Strontium was isolated and purified Sr fraction was then dried, redissolved in a small volume of 0.1 M HNO3, and prepared for isotopic analysis.
Approximately 5–10 mg of enamel samples were pretreated with 1 mL of 0.1 M acetic acid for 30 min and rinsed multiple times with Milli-Q water to remove residues, carbonates and other contaminants. Samples were then dried again at 50°C and subsequently dissolved in HNO3.
Samples were processed in the Department of Geological Sciences Isotope Geochemistry Clean Laboratory at the University of North Carolina at Chapel Hill. Approximately 7–10 mg of each sample was dissolved in 550 μL 3.5 M HNO3. Sr was isolated through ion exchange chromatography on a column with EiChrom Sr-SpecTM resin using 3.5 M HNO3 to wash the columns and H2O to elute Sr. Approximately 20 μL of 0.03 N H3PO4 was added to the sample and evaporated to dryness. Sr samples were loaded onto single Re filaments with TaF5 and analyzed in triple-dynamic multi-collector mode with 88Sr = 3 V (resistor of 1011 Ω) on the VG Sector-54 thermal ionization mass spectrometer. All data are normalized to 86Sr/88Sr = 0.1194 assuming exponential fractionation behavior. Data are reported relative to a value for NBS-987 of 87Sr/86Sr = 0.710250 ± 0.000020 (2s; n = 10). The internal run precision of all analyses (<0.0018%) is far smaller than the long-term external reproducibility of standards (0.0028%). Therefore, we report all uncertainties using the larger value (± 0.000020, 2s, absolute; Supplementary Information).
2.5 Isoscape modeling
The Ordinary Kriging approach was chosen to model a bioavailable isoscape of southern Ethiopia. Ordinary Kriging offers the spatial interpolation approach with statistical autocorrelation between two pairs of data points (in this case environmental 87Sr/86Sr values) based on a variogram model. This approach has been proven to be the effective way of producing Sr isoscapes as it considers distance and degree of variation among sampled points to estimate unknown areas (Britton et al., 2020; Lugli et al., 2022). As mentioned earlier, for the implementation of Ordinary Kriging, it is necessary to develop a variogram with a specific model, which in our interpolation case is Gaussian, as it appears to best fit the shape of the point distribution. Subsequently, the raster obtained was imported into QGIS 3.34.6 to clip the interpolation through a polygon representing the area of interest in our study. We used QGIS 3.34.6 and SAGA 9.3.1 to produce the bioavailable 87Sr/86Sr isoscape model in the area of interest for the Ordinary Kriging following the import of the shapefile (shp) containing the sample points in the WGS 84 UTM 37N reference system (EPSG: 32637). To measure and evaluate model performance, we employed validation techniques and calculated statistical metrics including the Mean Square Error (MSE), Root Mean Square Error (RMSE), and R2 values. A cell size of 1,000 m was chosen, and the program was instructed to perform cross-validation, specifically Leave-One-Out Cross-Validation tests (LOOCV).
2.6 Statistical analysis
All statistical analysis, tests, and visualization were performed in the R Studio (R Core Team, 2023). Descriptive and inferential statistics were used in this study. Summary statistical, such as the mean, median, standard deviation, interquartile range, and range are provided. Inferential statistics were used to draw conclusions about the characteristics of our sampled datasets and determine whether our data followed any observed discrepancies or relationships that were statistically significant for the fauna data. A Shapiro-Wilk test confirmed the enamel data were not normally distributed while parametric tests were applied for the environmental data which met the assumption of normality. A p > 0.05 does not provide enough evidence to reject the null hypothesis. Additionally, linear regression analysis was performed to evaluate the relationships between 87Sr/86Sr values and important variables such as elevation. Spearman's correlation coefficient was performed mainly to know the relationship between two variables. The Mann-Whitney U test was used for comparing two variables, while the Wilcoxon Signed-Rank Test was used for paired independent variables. An ANOVA test was used to determine the differences among the means of multiple variables.
3 Results
3.1 Bioavailable environmental 87Sr/86Sr ratios
The summary results of the bioavailable Sr samples for plants, archaeological fauna, soil leachate, and water are presented in Table 1. The 87Sr/86Sr isotope values for the environmental samples ranged from 0.70396 to 0.71183 (mean, 0.70673 ± 0.00188). Table 2 presents the values for different geological and lithological groups. Plant values display the largest variations (range = 0.70396–0.71183 ± 0.00254) in the overall range compared to other sample types such as soil and water. This is expected as the sampling covers a wider geological rock outcrops and landscapes. The soil and water 87Sr/86Sr values also vary significantly with ranges from 0.70368–0.70898 ± 0.00189 to 0.70445–0.70887 ± 0.00119, respectively. The variation for water is interesting despite most water samples being taken from similar sources of water bodies mainly from Rift Valley lakes. Minimal isotopic offsets are observed among different substrates of soil leachate, plants and waters within similar geological formations. In all Sr sample types, specimens collected within small distances and the same geology generally show 87Sr/86Sr isotope values of the Gotera fauna are properly estimating the local Sr distributions among different lithological units and geological outcrops. The Sr isotope data for the environmental variables are normally distributed (P = 0.00070. P < 0.05).
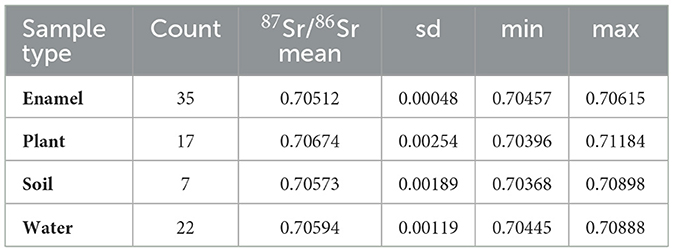
Table 1. Descriptive statistics of 87Sr/86Sr isotope ratio for different bioavailable substrates including plants, soil leachate, water, and archaeological fauna.
Analysis based on local geological formations also show large variance between them in terms of bioavailable 87Sr/86Sr values. The most radiogenic values are displayed from the Precambrian rock unit of the Yabelo and Jinka provinces with an empirical 87Sr/86Sr ratio >0.707, whereas limited values exhibited across the rift valley lakes and Quaternary deposits and soils. Quaternary sediments and Tertiary volcanic rocks have almost overlapping 87Sr/86Sr values (Figure 2) being deposits the result of the reworking of volcanoclastic material after erosion. Samples from the rift valley lakes of Langano, Shala, Abijata, Awasa, Abaya, and Chamo have generally higher Sr isotopic ratio. Lake Chew Bahir and Abaya have 87Sr/86Sr mean value of ~0.706, while Lake Langano, Awasa, Shala, and Zeway have a mean value of 0.707. Despite its geographical proximity to Lake Abaya, Lake Chamo has the lowest (~0.704) 87Sr/86Sr values from the lake samples. Within different lithological units, recent unconsolidated Quaternary sediments have the lowest 87Sr/86Sr values followed by basic volcanic rocks, while carbonate rocks of the study area show highest 87Sr/86Sr values.
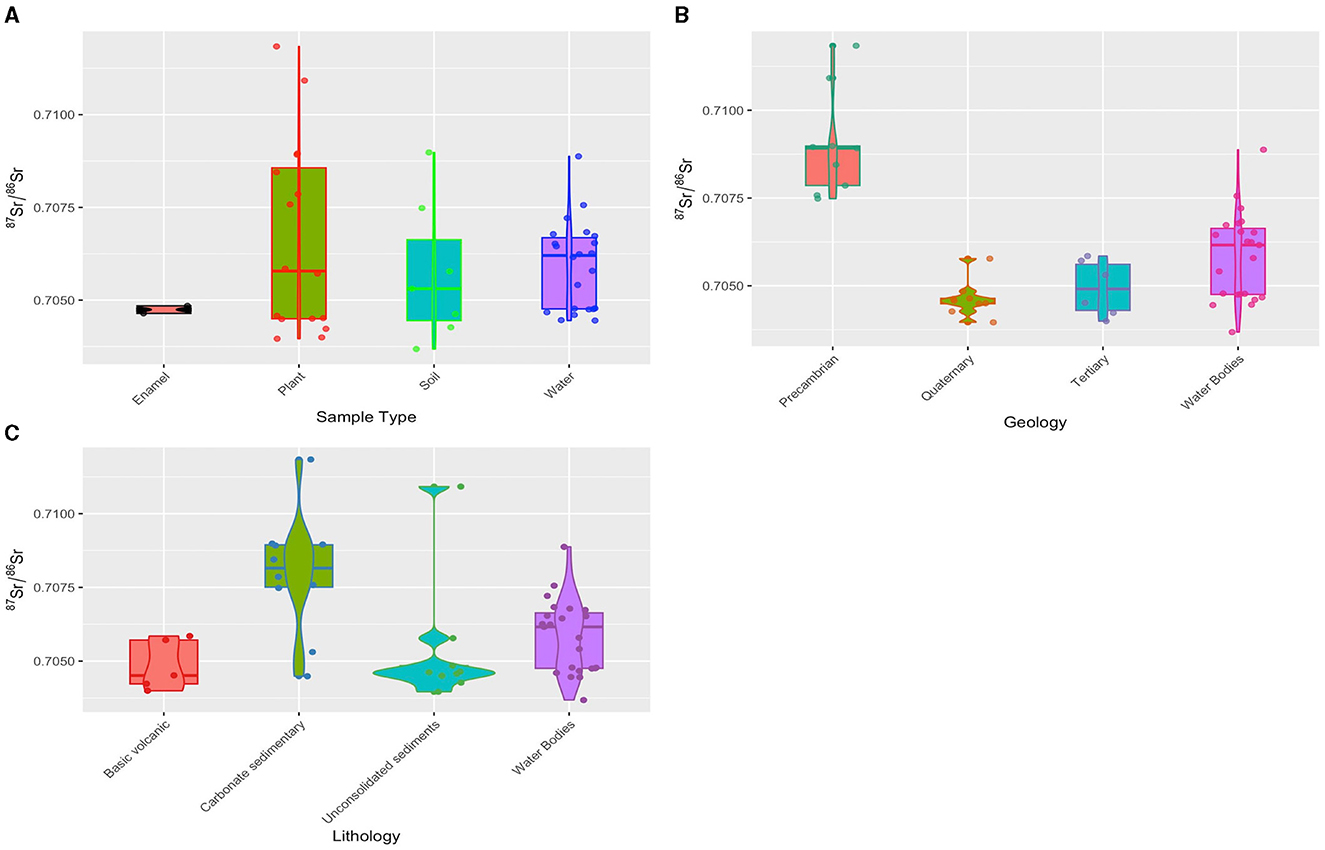
Figure 2. A box and whisker plot comparison of the 87Sr/86Sr isotope ratios of different substrates from southern Ethiopia (A), geological rock out crops (B), and lithologies (C).
A linear regression analysis (Figure 3) of the Sr isotope values by elevation shows that isotopic values increase as elevation increases. This is confirmed by a statistically significant correlation coefficient analysis (P < 0.05, P = 0.024). Overall, 87Sr/86Sr values show large variabilities across our selected areas of interest in southern Ethiopia. These variabilities are correlated with geological and lithological units whereby younger lithologies display lower 87Sr/86Sr values compared to older Precambrian units with higher 87Sr/86Sr values.
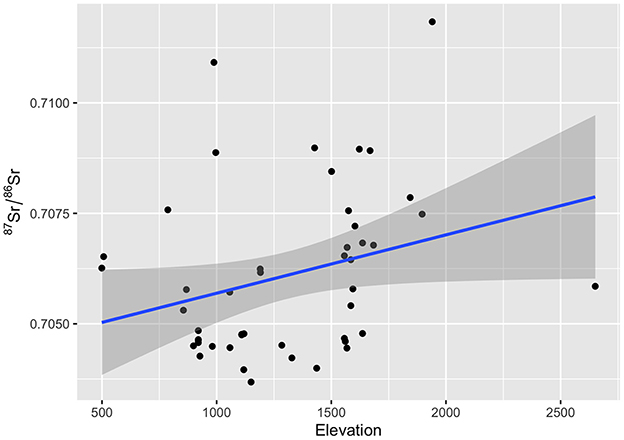
Figure 3. Linear regression analysis graph of the 87Sr/86Sr ratios of our areas of interest plotted against elevation showing a positive correlation (P = 0.024).
3.2 Predicted isoscape
The 87Sr/86Sr range of the predicted values in the study area of southern Ethiopia is < 0.705 to > 0.709 (Figure 4). The Sr isoscape reflects the spatial heterogeneity of our area of interest (Figure 5A), with the lowest values displayed to the direction transecting the Gotera area to the northeast and southwest (0.704–0.705). The bioavailable isoscape shows more radiogenic 87Sr/86Sr values (>0.708) predicted for the eastern and western uplands of Jinka and Yabello areas. Relatively lower values were associated with Quaternary sediments and Tertiary volcanics of the rift floor around the Arba Minch, Lake Chamo, and Gotera areas. Most of the lake water bodies in the MER such as Lake Langano, Zeway, Abijata, Shala, and Abaya displayed predicted 87Sr/86Sr values between 0.706 and 0.707 (Figure 4). Lake Chamo area displays the lowest predicted 87Sr/86Sr values. Areas around Lake Langano with environmental samples from hot springs in the central MER show relatively higher 87Sr/86Sr values compared to other areas in the rift setting.
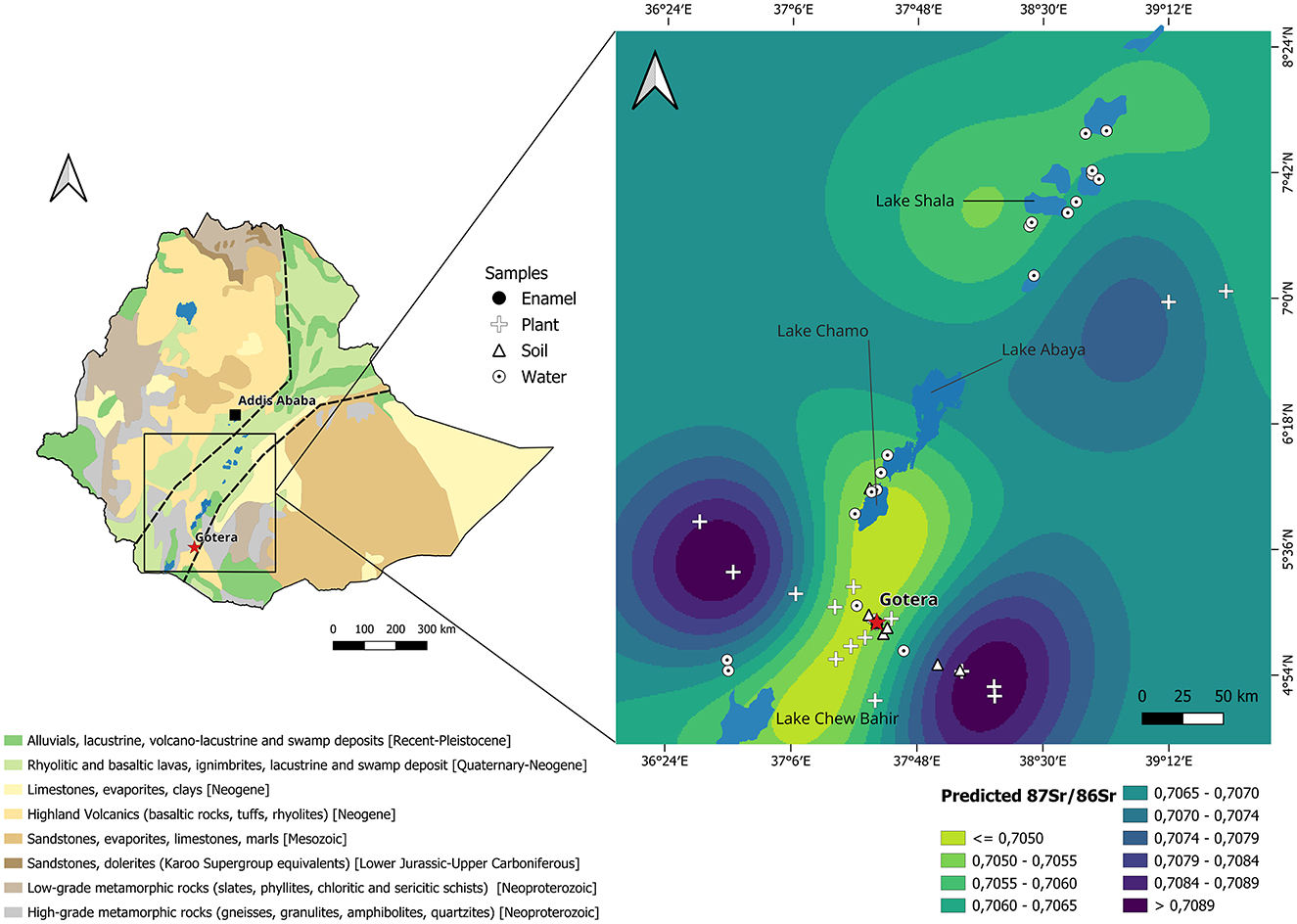
Figure 4. Bioavailable 87Sr/86Sr isoscape for the studied portion of southern Ethiopia. The Kriging model was produced QGIS 3.34.6 and SAGA 9.3.1 softwares. Symbols represent the location of different environmental samples. Geological map of Ethiopia modified from the Geological Atlas of Africa (Schlüter, 2008).
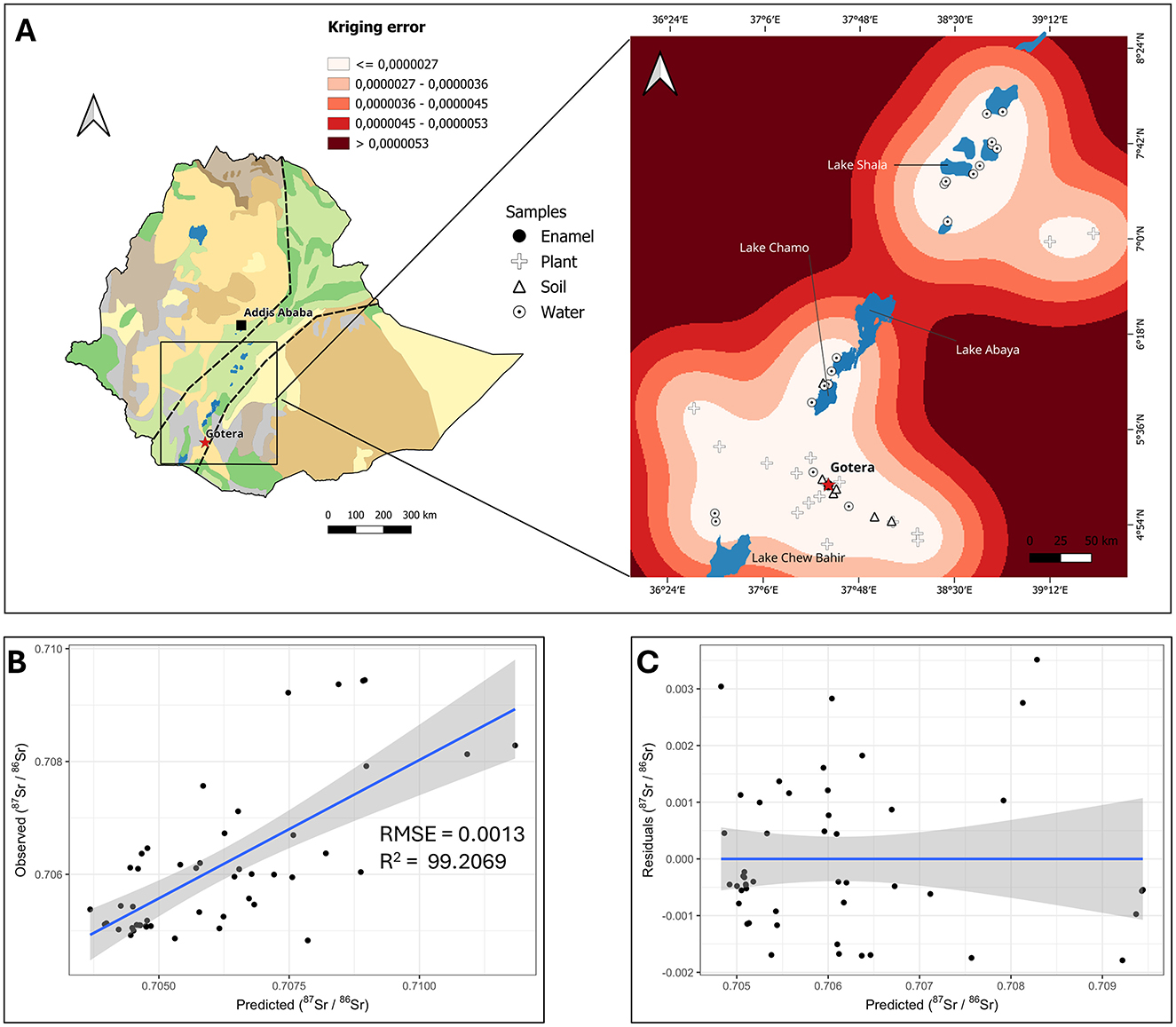
Figure 5. A prediction error isoscape showing the models good performance particularly in the densely sampled areas with (A). Linear regression between observed and predicted 87Sr/86Sr values (B). The residuals against the predicted value (C) using the Leave-One-Out Cross-Validation tests. Symbols represent the location of different environmental samples. Geological map of Ethiopia modified from the Geological Atlas of Africa (Schlüter, 2008).
The RMSE value of 0.00132 indicates a high model accuracy while the R2 of 99.206862 reflects over 99% of the variance in the dataset can be explained by the model, implying an excellent model fit. This study models the prediction standard error of the isoscape model to evaluate the prediction performance (Figure 5). The predicted standard error from the model is relatively low (SE = 0.0000000032) and evenly distributed in the sampled locations. We found that the prediction error (Figure 5A) becomes larger in the areas with fewer or no samples. This is expected because the Ordinary Kriging overlooks the consideration of auxiliary environmental predictors such as local geologies, climates, and lithological factors. Overall, our model demonstrates strong predictive performance with a high R2 (99.206862) and low MSE (0.000002) and RMSE (0.00132), representing accurate and precise prediction.
3.3 87Sr/86Sr isotope values of the Gotera fauna
The 87Sr/86Sr isotope range of archaeological fauna displays a smaller range (range = 0.704565–0.706145) (Table 3) compared to the different environmental archives utilized in the current study. The mean value for the Gotera fauna of all analyzed taxa appears to be identical (mean ~0.705). The 87Sr/86Sr values of the Alecelaphini (range = 0.70465–0.70587), Antilopini (range = 0.70470–0.70566), Bovidae (range = 0.70479–0.70615), Equidae (range = 0.70471–0.70585), and Suidae (range = 0.70462–0.70592) are not significantly different (Figure 5). While relatively larger variations are observed for Suidae taxa (sd = 0.00072), limited variation is documented for Antilopini (sd = 0.00035) (Table 3). Other individuals including a rodent (0.70485), a tragelaphini (0.70457), and a Viverridae (0.70464) show more or less similar 87Sr/86Sr ranges (Figure 6) corresponding to the GOT10 site plant and soil 87Sr/86Sr values.
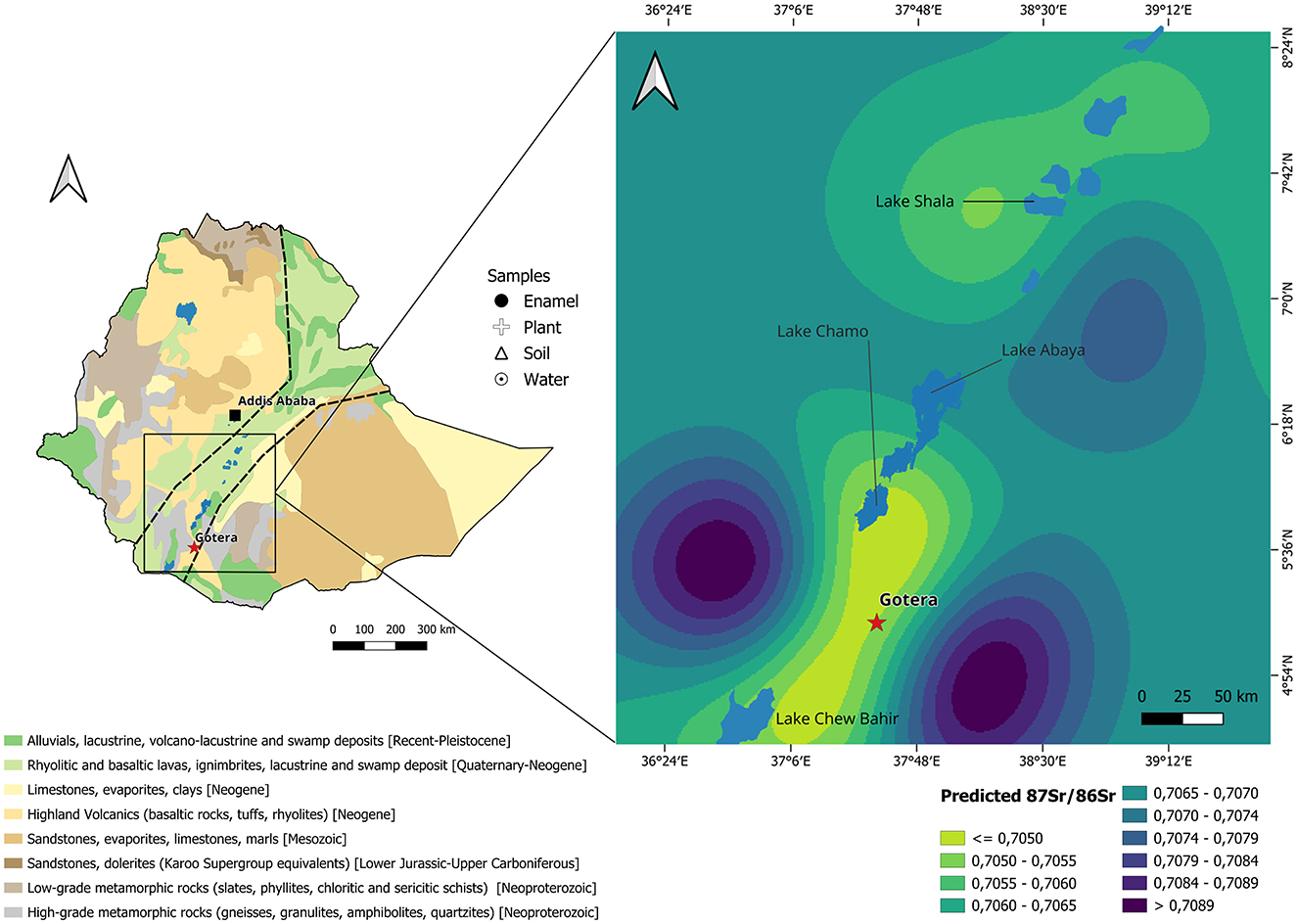
Figure 6. The bioavailable isoscape of southern Ethiopia, produced through Ordinary Kriging using QGIS 3.34.6 and SAGA 9.3.1 softwares. Geological map of Ethiopia modified from the Geological Atlas of Africa (Schlüter, 2008).
4 Discussion
4.1 Bioavailable 87Sr/86Sr isoscape of southern Ethiopia
Bioavailable Sr isoscape is key to being able to properly interpreting 87Sr/86Sr ratios across different geographic areas and its relevance for past mobility (Maurer et al., 2012; Bataille et al., 2018; Wang et al., 2023). Over the past decades, studies have increasingly employed different modeling approaches to construct a bioavailable 87Sr/86Sr isoscape and Sr isotope studies to understand the origin and mobility patterns of archaeological fauna and human groups in the past as well as for ecological studies (Price et al., 2002; Alexander Bentley, 2006; Maurer et al., 2012; Crowley et al., 2017; Bataille et al., 2018; Britton et al., 2020; Gigante et al., 2023; Wang et al., 2023). Such studies interpret 87Sr/86Sr values by comparing them with the predicted bioavailable 87Sr/86Sr isoscape (Bataille et al., 2018).
This study demonstrates that the bioavailable isoscape produced for southern Ethiopia here appears to correspond closely to the underlying local lithology. Though limited, previous Sr isotope researches in Ethiopia on major lithological units (e.g., Pryor et al., 2020) has revealed 87Sr/86Sr values across the Miocene/Pliocene and Oligocene basalts (0.703–0.706), Cretaceous terrestrial sandstones (0.7068–0.7078), Jurassic limestones (0-709-0.710), and Precambrian basement geology (0.7098–0.7105). Such measured variations in the 87Sr/86Sr values across the different topographic features reflect the contribution and interplay between various geological formation histories (Crowley et al., 2017). For instance, volcanic rocks generally display low 87Sr/86Sr values while plutonic rocks display high 87Sr/86Sr values. In the present study, the geolithologies of the area, according to the GLiM database (Hartmann and Moosdorf, 2012) covers the Precambrian basement rock outcrops prominently exposed across both sides of the rift valley, while the rift floor is comprised of Quaternary sediments and Tertiary volcanic outcrops. In areas to the west and southeast of Gotera, the Precambrian deposit seems to display similar and higher 87Sr/86Sr values, whereas the Pleistocene unconsolidated sediments show lower 87Sr/86Sr values. Both the measured and predicted 87Sr/86Sr values agree with the 87Sr/86Sr values documented in previous studies across Ethiopia where plutonic rocks display > 0.709 (Teklay et al., 1998). Similarly, the Miocene-Pliocene volcanic basalts from the MER produced very low 87Sr/86Sr values of 0.70354–0.70431. This is consistent with our measured 87Sr/86Sr values and the predicted model for the Tertiary Miocene volcanic-dominated areas of Segen and part of Konso.
The observed minimal offsets in the empirical 87Sr/86Sr values in some areas, for instance, at Lake Abaya and Lake Chamo can perhaps be explained by variations in sediment load and runoff sources. It has been documented that Lake Abaya is known for its high turbidity due to the large amount of radiogenic sediment flow and riverine Sr flux from its catchment areas (Teffera et al., 2019; Markowska et al., 2022). Similarly, the high radiogenic 87Sr/86Sr isotopic composition of Lake Chew Bahir, is due to the substantial contribution of the Precambrian basement catchment area (Markowska et al., 2022). The low 87Sr/86Sr values (~0.704) recorded from Lake Chamo is probably due to the Neogene/Paleogene volcanic contributions and limited sediment flow from the high radiogenic catchment areas. Likewise, the relatively high 87Sr/86Sr values of hot spring waters documented across the MER are likely due to strong influences from bedrock geology. Boschetti et al. (2018) demonstrate that groundwater passes through mineral rich bedrock substrates tends to display high radiogenic Sr values compared to lake waters formed as a result of surface runoffs.
Our bioavailable isoscape (Figure 7) suggests Ordinary Kriging appears to be effective in the areas of interest as demonstrated by the excellent model performance fit and low prediction standard errors (Figures 5B, C). Furthermore, the prediction model works well with higher precision and accuracy particularly in areas with the availability of dense samples. This shows that Ordinary Kriging approach produces a high-resolution bioavailable isoscape which is useful for modeling variations in 87Sr/86Sr across the landscape. Furthermore, the spatial variations in the predicted 87Sr/86Sr isoscape correspond well with the geological map of the study area (Figure 1), an alternative map traditionally utilized for Sr isotope studies. Previous studies (Lugli et al., 2019; Wang et al., 2023) have demonstrated the potential of Ordinary Kriging to generate detailed bioavailable isoscapes by integrating different Sr pools including soil, water, plants, and biological samples. Willmes et al. (2021) has also highlighted that Ordinary Kriging works best for small-scale analysis like the present study where with significant localized variations in 87Sr/86Sr values.
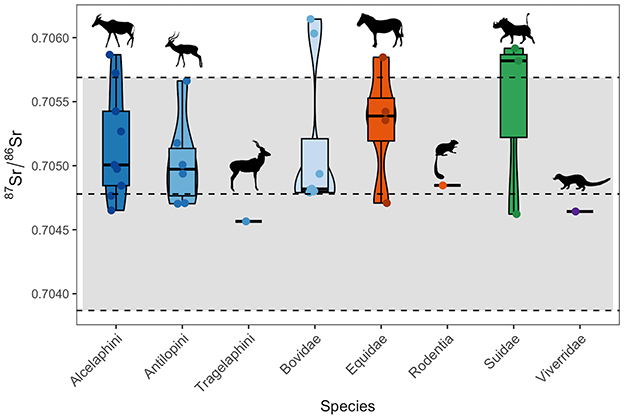
Figure 7. The 87Sr/86Sr isotope ratio of Gotera fauna by taxa with the shaded area represents the local values defined as ± 2 sd of micro-fauna, plant, and soil leachate from Gotera and its surroundings. The dotted lines represent the minimum and maximum values of the range while the middle dotted line shows the mean value related to ± 2 sd. Different range of blue colors represent taxa from the Bovidae family.
It is, however, important to note that while our Ordinary Kriging model generally works well to produce 87Sr/86Sr isotope map, it may overlook integrating Sr isotope influences, such as catchment area contributions and other factors highlighted above. This is reflected in the predicted and observed high 87Sr/86Sr values, while still geologically situated within the Quaternary sediment, for instance, some lakes and hot springs in the central MER. In this study, plant and soil samples perform well and generally reflect the local geology, while water samples display limited discrepancies which is also captured in the predicted 87Sr/86Sr isoscape.
An Sr isoscape for Ethiopia is available as part of the global isoscape modeled in Bataille et al. (2020) produced using the random forest (RF) machine learning technique, it has been observed that this isoscape shows a poor modeling performance in the areas of Ethiopia compared to the bioavailable isoscape in the part of Ethiopia studied here based on our new data. Bataille et al. (2020) has shown that the global isoscape expected to display low accuracies in the regions with fewer environmental samples regardless of the RF modeling. This suggests that a large sample size remains a fundamental prerequisite coupled with an appropriate choice of modeling approaches. Recent studies demonstrate the better modeling performance of the RF technique for constructing bioavailable isoscapes with the potential of exploring environmental covariates to predict values for less-sampled areas.
In this study, we explored factors including the role of elevation in influencing the bioavailable 87Sr/86Sr isotopes. Regression analysis (P < 0.05, P = 0.024) indicates that elevation appears to have a positive influence for the high 87Sr/86Sr values across the high-altitude areas situated just outside the EARS. This can be expected as younger lithological units are across the rift floor with lower elevation while old bedrock lies outside the rift or across the rift shoulders.
Apart from the good model performance, limited offsets have been observed between the measured and predicted 87Sr/86Sr ratios. Possible variations would be due to several factors such as atmospheric deposition, pedogenic, and geological factors (Maurer et al., 2012; Crowley et al., 2017). Similarly, given that Ethiopia's position to the coast is greater than 100 km, the influence of sea salt aerosols on the isotopic values of southern Ethiopia is expected to be minimal and, therefore, less of a concern in this study. Copeland et al. (2016) suggests areas with over 100 km distance from the coastal lines are less likely to be influenced by the sea spray effect. Similarly, anthropogenic factors including the use of fertilizer and human settlement may have minimal effects as most sampling areas are farther away from human settlements. Therefore, variations and/or similarities in the predicted isoscape may be explained by several factors including atmospheric dust deposition, lithological variations, climate and precipitation patterns.
This is reflected in the overlapping 87Sr/86Sr values of the Quaternary and Tertiary geological formations which have been shown in the predicted bioavailable isoscape of southern Ethiopia making our interpretation somewhat challenging particularly in areas with Miocene and Pliocene volcanic rocks. Furthermore, as Ordinary Kriging inherently overlooks the influence of geolithological factors, this may have precluded defining better resolution isoscape in the geological boundaries between two or more units (Zaugg et al., 2023). In this regard, complementing and utilizing alternative modeling approaches such as random forest regression (Bataille et al., 2020; Janzen et al., 2020) may overcome caveats as the technique considers environmental, climatic, lithological and geological factors.
4.2 Local 87Sr/86S range and provenance assignment
Researchers have employed several approaches to define a local bioavailable baseline (Price et al., 2002; Maurer et al., 2012; Lugli et al., 2022). 87Sr/86Sr values that fall within the estimated local range are considered “local” while those which fall outside are considered “non-local” or migratory (Alexander Bentley, 2006). We employed a combination of different local vs. non-local provenance assignment approaches. The most common method of estimating the local range for a given archaeological material is to define the ± 2 sd of the mean of local 87Sr/86Sr ratios (Price et al., 2002; Alexander Bentley, 2006; Lugli et al., 2022).
To define the local baseline, we considered the range of microfauna, local plants, and the soil 87Sr/86Sr ratio of the Gotera area. We estimated the local range from soil (n = 3), small mammals (n = 2), and plants (n = 3) from the Gotera area. The local ± 2 sd range equivalent to the 95% confidence interval of the Gotera area falls between 0.70386 and 0.70568. This study compared the 87Sr/86Sr ratios of small mammals from the Gotera archaeological site to the local modern baseline (plants and soil). We found that the 87Sr/86Sr values are consistent over time. Unsurprisingly, the micro-fauna, plant, and soil values from our case study area show relatively limited baseline 87Sr/86Sr range implying lower variance in the local range and excellent agreement among the different Sr sources. Figure 7 shows the provenance assignment graph for the local archives, reflecting the fact that most of the faunal values fall within the local range. We also compared the corresponding faunal 87Sr/86Sr values against the empirical and predicted values for the Gotera area. Accordingly, the 87Sr/86Sr values of most of the Gotera faunal falls within the local range. Recent studies on the fossils and their modern counterparts has documented that species with savannah and open woodland habitats such as the Gotera fauna tend to have wider home ranges (Copeland et al., 2016; O'Brien et al., 2024).
The 87Sr/86Sr values of the Gotera fauna predominatly aligns well with the the predicted Sr isoscape within 30 km radii of the Gotera landscape, suggesting faunal exploitation most likely occurred in the vicinity of the site. Nearly all faunal 87Sr/86Sr values falls within the local range established by local fauna and bioavailable isoscape, suggesting limited mobility with local foraging radii of fauna and reliance on local resources. Alternatively, faunal movement could occur from regions or geographical areas with similar 87Sr/86Sr values. However, given the absence of high 87Sr/86Sr values exclusively for the archaeological faunal, which were observed in both measured and predicted bioavailable isoscapes of environmental samples, more plausible scenario is likely to be the limited mobility patterns. 87Sr/86Sr values similar to the fauna in the predicted isoscape is found mainly across the rift setting of the Arba Minch area. However, movement into the area would likely be hindered by the Konso ranges and uplands with Precambrian rock outcrops which have limited sign of isotopic similarity or mobility into the adjacent Precambrian basement areas exposed mainly in the high-altitude vicinities. Nevertheless, it is crucial to highlight the issue of equifinality which obfuscates the discussion of 87Sr/86Sr data.
The 87Sr/86Sr values of small fauna such as viverrids and hares in Gotera show values identical to those of modern plant and soil samples from the Gotera area. One individual Tragelaphus (ID, 532) shows similar Sr isotope ratios to the local plant and soil values. Except for micro-mammals, the relatively higher 87Sr/86Sr ranges of Alcelaphini, and Bovidae (indet.), Equidae and Suidae display almost similar variances. The sparse and limited distribution (Figure 4; Table 3) of the faunal 87Sr/86Sr isotope ratios is suggestive of the nature of different home ranges for each species. Higher ranges within the same taxon indicate different home-range behavior and mobility patterns both within and probably outside Gotera. Different faunas occupy varying degrees of home ranges from a couple of square kilo meters to hundreds of kilo meters depending on habitat preference, seasonality, and social structure (O'Brien et al., 2024). At Gotera, these animals could have had similar dietary and drinking sources and likely resided in a similar locality. Based on their 87Sr/86Sr values, none of the Gotera faunas were accessing the Precambrian basement formations across the uphill surrounding area.
Careful consideration, however, should be given to the small sample size (n=35) compared to the anthropogenically assembled large faunas from the GOT10 site. Similarly, integrating Sr isotopic composition against the bioavailable isoscape can be challenging due to issues of equifinality (Holt et al., 2021). Furthermore, it is worth bearing in mind that bulk samples used in this study have provided time-averaged 87Sr/86Sr values of faunal mobility history during tooth mineralization. One issue is that animals whose tooth 87Sr/86Sr matches the “local” Sr signature could be quite migratory, traveling to geologies with much higher and much lower 87Sr/86Sr values, but when homogenized during isotopic sampling, this will “average” to a value that appears identical to the local range. In this regard, sequentially sampled tooth enamel often provides better resolution, mainly to elucidate the seasonal mobilities of game animals. However, this study intends to test whether the faunas are broadly mobile across major geologies and topographies in search of resources, or whether the Gotera area may have offered adequate resources for faunas and humans to be residentially stable. While acknowledging the issue of equifinality, bulk samples have provided important clues about landscape use and mobility patterns particularly in areas such as the Gotera depression with diverse geological and topographic variabilities across the immediate vicinities.
The 87Sr/86Sr values for some taxa outside the shaded area in Figure 7, including Alcelaphini, and Equidae, could suggest that these individuals are “non-local”. These faunas may have been hunted from the Tertiary rock outcrops probably within the plain area southwest of Gotera toward Yabelo direction with relatively wider 87Sr/86Sr ranges of 0.70399 to 0.70820. The escarpments across the adjacent highlands are a less likely origin for the non-local fauna. This shows that either at least some individuals within the identified taxa could be migratory or hunter-gatherers may have traveled to hunt them.
4.3 Implication for faunal mobility and hominin land use
The modern savannah ecosystem in eastern Africa hosts one of the largest seasonal wildlife migrations (O'Brien et al., 2023). Migration and mobility patterns in fossil records analogous to the modern fauna have not yet been properly researched except for an ongoing effort (O'Brien et al., 2023, 2024). Likewise, research on the impacts of faunal mobility behavior and seasonal migrations on hunter-gatherer lifestyle is minimal.
The faunal specimens that were exploited by MSA hunter-gatherers in Gotera did not show evidence of significant mobility, suggesting that faunal specimens were accessible resources throughout the year. We argue that the different taxa would have had access to resources year-round in the study area suggesting that MSA human groups may have had adequate resources across the Gotera landscape, which may explain the persistence of intense human occupation in the area. The availability of local resources mainly water and vegetation may also have controlled faunal home ranges by offering productive semi-arid lake-margin environments. The limited 87Sr/86Sr variations for faunas as shown in Figure 7 indicates MSA hunter-gatherers would have explored a wider yet still home-range-sized, area of the paleolandscape of the Gotera depression. Our current Sr isotope results reveal that the fauna may have roamed within the Gotera area possibly following the palaeobasin watercourses. The Gotera palaeolake offshore as interpreted by sedimentological analysis (Fusco et al., 2021) may have provided suitable palaeoecological niches for diverse faunal and human groups.
Tracking faunal mobility across nearby Tertiary rock outcrops, such as those in the Konso area, is hampered by the overlapping nature of Sr isotope values between the Quaternary sediments and Tertiary volcanics. The bioavailable isoscape also performs less well in this part of the study area. Based on the bioavailable Sr isoscape, low 87Sr/86Sr values are predicted in the refit floor from the Arba Minch in a north-to-south direction, transecting Gotera. Thus, it is likely that north-to-south small-scale faunal movement would have been possible following the rift basin within an isotopically homogeneous area.
O'Brien et al.'s (2024) recent Sr and δ13C stable isotope investigation on the extant fossils from four archaeological sites in Kenya suggests limited herbivore migration during the Late Pleistocene. Migratory and non-migratory behaviors of animals have been recognized from modern and archaeological fauna through intra-tooth sequential Sr isotope analysis (e.g., O'Brien et al., 2023). Fauna may migrate seasonally for different reasons, including searches for food, water, and mating (O'Brien et al., 2024). Future research on the intra-tooth analysis of 87Sr/86Sr ratios coupled with other stable isotopes will contribute additional insights into the nature of migration and seasonal site use of Late Pleistocene human groups and faunal communities in the region.
Our Sr isotope record indicates the low residential mobility of hunter-gatherers at Gotera. This can be related to the occurrence of rich material records at the site, indicating high occupational intensity and site-use of the Gotera area. Our interpretation corresponds to the exploitation of over 90% of locally sourced lithic raw materials at the GOT10 site (Fusco et al., 2024), suggesting that hunter-gatherers were predominantly relied on local resources. This study supports the ecological assumption that environmental setting with evenly distributed resources and diverse faunal availability characterize prehistoric hunter-gatherers residential stability and territorial lifestyles (Harpending and Davis, 1977). This study further supports Tryon and Faith's (2016) proposed model that suggests reduced residential mobility during the MSA to LSA transition period due to high population density and intensive exploitation of immediate local landscape.
5 Conclusions and future directions
This bioavailable isoscape contributes to the characterization of human and faunal mobility patterns and land use in Late Pleistocene eastern Africa. This study offers the first environmental, and empirical data on bioavailable Sr isoscape for southern Ethiopia. Our research contributes to the ecological and archaeological assessment of human and faunal migratory behaviors, human occupation and resources utilization strategies of the MSA human groups during the Late Plesitocene. The 87Sr/86Sr isoscape of southern Ethiopia will aid future provenance, mobility, and ecological studies and serve as a baseline for building further empirical and process-based Sr isoscape models on local, regional, and global scales. It can also serve as a foundation for future scientific enquiries and will serve as a baseline map construction in Ethiopia and elsewhere.
The bioavailable isoscape for a section of southern Ethiopia indicates that using geostatistical Ordinary Kriging is an alternative approach for producing small-scale high-resolution isoscapes, particularly in areas with dense sampling. To compensate for some of the observed caveats, we suggest that future research should integrating multiple modeling techniques as well as multiple lines of evidence.
The consistency of the archaeological faunal 87Sr/86Sr values with the local baseline map for Gotera suggests the majority of these individuals were resided or exploited in the Gotera environment. Our interpretation of the 87Sr/86Sr values of the fauna suggesting Gotera may have offered reliable animal resources and environmental conditions during the Late Pleistocene. Furthermore, we argue that with an open-to-wooded habitat structure and palaeolake sediment, the Gotera area may have represented an environmental refugium within a wider region of climatic change and unpredictability.
The main limitations of this study include a relatively small archaeological sample size, accessibility, and analytical cost. Producing high-resolution, extensive Sr isoscapes inherently requires exhaustive datasets from different lithologies and within the same lithologies as well as from different environmental substrates. Given that this study represents the first isoscape of its kind which is unprecedented in Ethiopia, it had faced the challenges of work with a small sample size in a complex topographical region. It has also been challenging to collect samples from all targeted areas due to security issues in some parts of southern Ethiopia and inaccessibility either due to dense vegetation cover or lack of road infrastructure.
Overall, the present study demonstrates the potential applicability of Sr isotope research for future archaeological and palaeoecological investigations in southern Ethiopia and the region of eastern Africa. We plan to extend the study area eventually throughout Ethiopia with the sampling of diverse Sr reservoirs across different lithological units.
Data availability statement
The original contributions presented in the study are included in the article/Supplementary material, further inquiries can be directed to the corresponding authors.
Author contributions
SA: Conceptualization, Data curation, Formal analysis, Funding acquisition, Investigation, Methodology, Resources, Software, Visualization, Writing – original draft, Writing – review & editing, Project administration, Supervision, Validation. FL: Data curation, Investigation, Methodology, Software, Validation, Writing – review & editing, Visualization. MT: Supervision, Validation, Writing – review & editing. CA: Data curation, Validation, Writing – review & editing. MG: Supervision, Validation, Writing – review & editing. AZ: Conceptualization, Validation, Writing – review & editing. MF: Validation, Writing – review & editing. ES: Funding acquisition, Resources, Supervision, Validation, Writing – review & editing.
Funding
The author(s) declare that financial support was received for the research and/or publication of this article. This study forms part of Seminew Asrat's doctoral thesis and received support from funding sources including the Leakey Foundation for SA's doctoral research, Sapienza University of Rome “Avvio alla ricerca” grant, the Italian Ministry of Research (PRIN 2022), “Grandi Scavi Sapienza,” and Italian Ministry of Foreign Affairs (MAE).
Acknowledgments
We would like to acknowledge the Ethiopian Heritage Authority and Borena Culture and Tourism Office for the continued cooperation during our successful fieldwork and laboratory research in Ethiopia. We extend our acknowledgment to the Laboratory of Palaeoanthropology and Bioarchaeology of the Sapienza University of Rome and the Isotopic Geochemistry Laboratory of the Department of Geological Sciences at the University of North Carolina for sample preparation and isotope analysis. We would like to express our gratitude to the anonymous reviewers and Dr. Patrick Roberts of the Max Planck Institute of Geoanthropology for their invaluable feedback on earlier versions of the manuscript. The authors also wish to thank Sahle Melaku, Silvia Soncin, Yilkal Demis, Wogen Amerga, and Halake Shoba for their assistance and cooperation during field work, sampling and laboratory analysis.
Conflict of interest
The authors declare that the research was conducted in the absence of any commercial or financial relationships that could be construed as a potential conflict of interest.
Publisher's note
All claims expressed in this article are solely those of the authors and do not necessarily represent those of their affiliated organizations, or those of the publisher, the editors and the reviewers. Any product that may be evaluated in this article, or claim that may be made by its manufacturer, is not guaranteed or endorsed by the publisher.
Supplementary material
The Supplementary Material for this article can be found online at: https://www.frontiersin.org/articles/10.3389/fearc.2025.1499291/full#supplementary-material
References
Abbas, M., Lai, Z., Jansen, J. D., Tu, H., Alqudah, M., Xu, X., et al. (2023). Human dispersals out of Africa via the Levant. Sci. Adv. 9:eadi6838. doi: 10.1126/sciadv.adi6838
Abbate, E., Bruni, P., and Sagri, M. (2015). “Geology of Ethiopia: a review and geomorphological perspectives,” in Landscapes and Landforms of Ethiopia, eds. P. Billi (World Geomorphological Landscapes. Dordrecht: Springer Netherlands), 33–64. doi: 10.1007/978-94-017-8026-1_2
Alexander Bentley, R. (2006). Strontium isotopes from the earth to the archaeological skeleton: a review. J. Archaeol. Method Theory 13, 135–187. doi: 10.1007/s10816-006-9009-x
Asfaw, B., Beyene, Y., Semaw, S., Suwa, G., White, T., WoldeGabriel, G., et al. (1991). Fejej: a new paleoanthropological research area in Ethiopia. J. Hum. Evol. 21, 137–143. doi: 10.1016/0047-2484(91)90004-F
Asfaw, B., Beyene, Y., Suwa, G., Walter, R. C., White, T. D., WoldeGabriel, G., et al. (1992). The earliest acheulean from Konso-Gardula. Nature 360, 732–735. doi: 10.1038/360732a0
Assefa, Z., Pleurdeau, D., Duquesnoy, F., Hovers, E., Pearson, O., Asrat, A., et al. (2014). Survey and explorations of caves in southeastern ethiopia: middle stone age and later stone age archaeology and holocene rock art. Quat. Int. 343, 136–147. doi: 10.1016/j.quaint.2013.07.132
Ayalew, D., Yirgu, G., and Pik, R. (1999). Geochemical and isotopic (Sr, Nd and Pb) characteristics of volcanic rocks from Southwestern Ethiopia. J. Afr. Earth Sci. 29, 381–391. doi: 10.1016/S0899-5362(99)00104-9
Balasse, M., Ambrose, S. H., Smith, A. B., and Price, T. D. (2002). The seasonal mobility model for prehistoric herders in the South-Western Cape of South Africa assessed by isotopic analysis of sheep tooth enamel. J. Archaeol. Sci. 29, 917–932. doi: 10.1006/jasc.2001.0787
Bataille, C. P., Crowley, B. E., Wooller, M. J., and Bowen, G. J. (2020). Advances in global bioavailable strontium isoscapes. Palaeogeogr. Palaeoclimatol. Palaeoecol. 555:109849. doi: 10.1016/j.palaeo.2020.109849
Bataille, C. P., von Holstein, I. C., Laffoon, J. E., Willmes, M., Liu, X. M., and Davies, G. R. (2018). A bioavailable strontium isoscape for Western Europe: a machine learning approach. PLoS ONE 13:e0197386. doi: 10.1371/journal.pone.0197386
Boschetti, T., Awaleh, M., and Barbieri, M. (2018). Waters from the Djiboutian Afar: a review of strontium isotopic composition and a comparison with Ethiopian waters and red sea brines. Water 10:1700. doi: 10.3390/w10111700
Brandt, S., Hildebrand, E., Vogelsang, R., Wolfhagen, J., and Wang, H. (2017). A new MIS 3 radiocarbon chronology for mochena borago rockshelter, SW Ethiopia: implications for the interpretation of late pleistocene chronostratigraphy and human behavior. J. Archaeol. Sci. 11, 352–369. doi: 10.1016/j.jasrep.2016.09.013
Brandt, S. A., Fisher, E. C., Hildebrand, E. A., Vogelsang, R., Ambrose, S. H., Lesur, J., et al. (2012). Early MIS 3 occupation of mochena borago rockshelter, southwest ethiopian highlands: implications for late pleistocene archaeology, paleoenvironments and modern human dispersals. Quat. Int. 274, 38–54. doi: 10.1016/j.quaint.2012.03.047
Britton, K., Crowley, B. E., Bataille, C. P., Miller, J. H., and Wooller, M. J. (2022). Editorial: a golden age for strontium isotope research? Current advances in paleoecological and archaeological research. Front. Ecol. Evol. 9:820295. doi: 10.3389/fevo.2021.820295
Britton, K., Grimes, V., Niven, L., Steele, T. E., McPherron, S., Soressi, M., et al. (2011). Strontium isotope evidence for migration in late pleistocene rangifer: implications for neanderthal hunting strategies at the middle palaeolithic site of Jonzac, France. J. Hum. Evol. 61, 176–185. doi: 10.1016/j.jhevol.2011.03.004
Britton, K., Le Corre, M., Willmes, M., Moffat, I., Grün, R., Mannino, M. A., et al. (2020). Sampling plants and malacofauna in 87Sr/86Sr bioavailability studies: implications for isoscape mapping and reconstructing of past mobility patterns. Front. Ecol. Evol. 8:579473. doi: 10.3389/fevo.2020.579473
Capo, R. C., Stewart, B. W., and Chadwick, O. A. (1998). Strontium isotopes as tracers of ecosystem processes: theory and methods. Geoderma 82, 197–225. doi: 10.1016/S0016-7061(97)00102-X
Carto, S. L., Weaver, A. J., Hetherington, R., Lam, Y., and Wiebe, E. C. (2009). Out of Africa and into an Ice Age: on the role of global climate change in the late Pleistocene migration of early modern humans out of Africa. J. Hum. Evol. 56, 139–151. doi: 10.1016/j.jhevol.2008.09.004
Chavaillon, N., and Chavaillon, J. (1985). Gotera: Un Site Paléolithique Récent d'Éthiopie. Paris: Editions Recherche sur les civilisations.
Copeland, S. R., Cawthra, H. C., Fisher, E. C., Lee-Thorp, J. A., Cowling, R. M., Le Roux, P. J., et al. (2016). Strontium isotope investigation of ungulate movement patterns on the pleistocene paleo-agulhas plain of the greater cape floristic region, South Africa. Quat. Sci. Rev. 141, 65–84. doi: 10.1016/j.quascirev.2016.04.002
Copeland, S. R., Sponheimer, M., de Ruiter, D. J., Lee-Thorp, J. A., Codron, D., le Roux, P. J., et al. (2011). Strontium isotope evidence for landscape use by early hominins. Nature 474, 76–78. doi: 10.1038/nature10149
Crowley, B. E., Miller, J. H., and Bataille, C. P. (2017). Strontium isotopes (87 Sr/86 Sr) in terrestrial ecological and palaeoecological research: empirical efforts and recent advances in continental-scale models: Sr isotopes in terrestrial biological applications. Biol. Rev. 92, 43–59. doi: 10.1111/brv.12217
Duff, A. I., Zena, A., Melesse, A., Wolff, J. A., Neill, O. K., and Shackley, M. S. (2018). Recent research on megalithic stele sites of the gedeo zone, Southern Ethiopia. J. Archaeol. Sci. Rep. 19, 856–863. doi: 10.1016/j.jasrep.2018.03.034
Emishaw, L., Laó-Dávila, D. A., Abdelsalam, M. G., Atekwana, E. A., and Gao, S. S. (2017). Evolution of the broadly rifted zone in Southern Ethiopia through gravitational collapse and extension of dynamic topography. Tectonophysics 699, 213–226. doi: 10.1016/j.tecto.2016.12.009
Ericson, J. E. (1985). Strontium isotope characterization in the study of prehistoric human ecology. J. Hum. Evol. 14, 503–514. doi: 10.1016/S0047-2484(85)80029-4
Evans, J. A., Chenery, C. A., and Montgomery, J. (2012). A Summary of strontium and oxygen isotope variation in archaeological human tooth enamel excavated from britain. J. Anal. At. Spectrom. 27, 754. doi: 10.1039/c2ja10362a
Faure, G., and Powell, J. L. (1972). Strontium Isotope Geology. Berlin, Heidelberg: Springer. doi: 10.1007/978-3-642-65367-4
Foerster, V., Deocampo, D. M., Asrat, A., Günter, C., Junginger, A., Krämer, K. H., et al. (2018). Towards an understanding of climate proxy formation in the chew bahir basin, southern ethiopian rift. Palaeogeogr. Palaeoclimatol. Palaeoecol. 501, 111–123. doi: 10.1016/j.palaeo.2018.04.009
Fusco, M., Asrat, S., Aureli, C., Zerboni, A., Lemorini, C., Gallinaro, M., et al. (2024). The gotera archaeological mission in southern ethiopia: a preliminary field report on the ongoing research at the middle stone age site of gotera. 53, 17–33.
Fusco, M., Carletti, E., Gallinaro, M., Zerboni, A., and Spinapolice, E. E. (2021). Lithic variability and raw material exploitation at the middle stone age (MSA) site of gotera, southern ethiopia: a combined technological and quantitative approach. J. Lithic Stud. 8:29. doi: 10.2218/jls.6530
Gallinaro, M., and Zerboni, A. (2021). Rock, Pigments, and Weathering. A preliminary assessment of the challenges and potential of physical and biochemical studies on rock art from southern ethiopia. Quat. Int. 572, 41–51. doi: 10.1016/j.quaint.2020.05.056
Ghouri, S. (2024). Hydrogen and Strontium Isoscapes for the African Palearctic Range to Reconstruct Insect Migration and Connectivity (Unpublished MSc Thesis). University of Ottawa, Ottawa, ON.
Gigante, M., Mazzariol, A., Bonetto, J., Armaroli, E., Cipriani, A., and Lugli, F. (2023). Machine learning-based sr isoscape of southern sardinia: a tool for bio-geographic studies at the phoenician-punic site of nora. PLoS ONE 18:e0287787. doi: 10.1371/journal.pone.0287787
Harpending, H., and Davis, H. (1977). Some implications for hunter-gatherer ecology derived from the spatial structure of resources. World Archaeol. 8, 275–286. doi: 10.1080/00438243.1977.9979673
Hartmann, J., and Moosdorf, N. (2012). The new global lithological map database GLiM: a representation of rock properties at the earth surface. Geochem. Geophys. Geosyst. 13:2012GC004370. doi: 10.1029/2012GC004370
Holt, E., Evans, J. A., and Madgwick, R. (2021). Strontium (87Sr/86Sr) mapping: a critical review of methods and approaches. Earth Sci. Rev. 216:103593. doi: 10.1016/j.earscirev.2021.103593
Hoppe, K. A., Koch, P. L., and Furutani, T. T. (2003). Assessing the preservation of biogenic strontium in fossil bones and tooth enamel. Int. J. Osteoarchaeol. 13, 20–28. doi: 10.1002/oa.663
James, H. F. (2022). A large-scale environmental strontium isotope baseline map of portugal for archaeological and paleoecological provenance studies. J. Archaeol. Sci. 142:105595. doi: 10.1016/j.jas.2022.105595
Janzen, A., Bataille, C., Copeland, S. R., Quinn, R. L., Ambrose, S. H., Reed, D., et al. (2020). Spatial variation in bioavailable strontium isotope ratios (87Sr/86Sr) in Kenya and Northern Tanzania: implications for ecology, paleoanthropology, archaeology. Palaeogeogr. Palaeoclimatol. Palaeoecol. 560:109957. doi: 10.1016/j.palaeo.2020.109957
Lazzerini, N., Balter, V., Coulon, A., Tacail, T., Marchina, C., Lemoine, M., et al. (2021). Monthly mobility inferred from isoscapes and laser ablation strontium isotope ratios in caprine tooth enamel. Sci. Rep. 11:2277. doi: 10.1038/s41598-021-81923-z
Lugli, F., Cipriani, A., Bruno, L., Ronchetti, F., Cavazzuti, C., and Benazzi, S. (2022). A strontium isoscape of italy for provenance studies. Chem. Geol. 587:120624. doi: 10.1016/j.chemgeo.2021.120624
Lugli, F., Cipriani, A., Capecchi, G., Ricci, S., Boschin, F., Boscato, P., et al. (2019). Strontium and stable isotope evidence of human mobility strategies across the last glacial maximum in Southern Italy. Nat. Ecol. Evol. 3, 905–911. doi: 10.1038/s41559-019-0900-8
Markowska, M., Martin, A. N., Vonhof, H. B., Guinoiseau, D., Fischer, M. L., Zinaye, B., et al. (2022). A multi-isotope and modelling approach for constraining hydro-connectivity in the east african rift system, southern ethiopia. Quat. Sci. Rev. 279:107387. doi: 10.1016/j.quascirev.2022.107387
Maurer, A. F., Galer, S. J., Knipper, C., Beierlein, L., Nunn, E. V., Peters, D., et al. (2012). Bioavailable 87Sr/86Sr in different environmental samples—effects of anthropogenic contamination and implications for isoscapes in past migration studies. Sci. Total Environ. 433, 216–229. doi: 10.1016/j.scitotenv.2012.06.046
Montgomery, J. (2010). Passports from the past: investigating human dispersals using strontium isotope analysis of tooth enamel. Ann. Hum. Biol. 37, 325–346. doi: 10.3109/03014461003649297
Montgomery, J., Evans, J., and Horstwood, M. (2010). Evidence for long-term averaging of strontium in bovine enamel using TIMS and LA-MC-ICP-MS strontium isotope intra-molar profiles. Environ. Archaeol. 15, 32–42. doi: 10.1179/146141010X12640787648694
O'Brien, K., Podkovyroff, K., Fernandez, D. P., Tryon, C. A., Ashioya, L., and Faith, J. T. (2023). Migratory behavior in the enigmatic late pleistocene bovid rusingoryx atopocranion. Front. Environ. Archaeol 2:1237714. doi: 10.3389/fearc.2023.1237714
O'Brien, K., Podkovyroff, K., Fernandez, D. P., Tryon, C. A., Cerling, T. E., Ashioya, L., et al. (2024). Limited herbivore migration during the last glacial period of Kenya. Nat. Ecol. Evol. 8, 1191–1198. doi: 10.1038/s41559-024-02413-9
Ossendorf, G., Groos, A. R., Bromm, T., Tekelemariam, M. G., Glaser, B., Lesur, J., et al. (2019). Middle stone age foragers resided in high elevations of the glaciated bale mountains, Ethiopia. Science 365, 583–587. doi: 10.1126/science.aaw8942
Persits, F. M., Ahlbrandt, T. S., Tuttle, M. L., Charpentier, R. R., Brownfield, M. E., and Takahashi, K. I. (1997). “Maps showing geology, oil and gas fields and geological provinces of Africa,” in U.S. Geological Survey Open-File Report 97-470-A. doi: 10.3133/ofr97470A
Philippon, M., Corti, G., Sani, F., Bonini, M., Balestrieri, M. L., Molin, P., et al. (2014). Evolution, distribution, and characteristics of rifting in southern ethiopia. Tectonics 33, 485–508. doi: 10.1002/2013TC003430
Pleurdeau, D., Hovers, E., Assefa, Z., Asrat, A., Pearson, O., Bahain, J. J., et al. (2014). Cultural change or continuity in the late msa/early lsa of southeastern ethiopia? The site of goda buticha, dire dawa area. Quat. Int. 343, 117–135. doi: 10.1016/j.quaint.2014.02.001
Price, T. D., Burton, J. H., and Bentley, R. A. (2002). The characterization of biologically available strontium isotope ratios for the study of prehistoric migration. Archaeometry 44, 117–135. doi: 10.1111/1475-4754.00047
Pryor, A. J. E., Insoll, T., and Evis, L. (2020). Laser ablation strontium isotope analysis of human remains from harlaa and sofi, eastern ethiopia, and the implications for islamisation and mobility. STAR 6, 113–136. doi: 10.1080/20548923.2020.1843266
R Core Team (2023). R: A Language and Environment for Statistical Computing. R Foundation for Statistical Computing, Vienna. Available online at: https://Www.R-Project.Org/-Search; https://www.bing.com/search?q=R+Core+Team+(2023)+R%3A+A+Language+and+Environment+for+Statistical+Computing.+R+Foundation+for+Statistical+Computing%2C+Vienna.+https%3A%2F%2F (accessed September 7, 2024).
Rango, T., Petrini, R., Stenni, B., Bianchini, G., Slejko, F., Beccaluva, L., et al. (2010). The dynamics of central main ethiopian rift waters: evidence from δD, δ18O and 87Sr/86Sr Ratios. Appl. Geochem. 25, 1860–1871. doi: 10.1016/j.apgeochem.2010.10.001
Roger, J., and Jean-Paul, C. (2020). Art rupestre dans la Corne de l'Afrique. Chauvigny: Association des publications chauvinoises (Mémoire LIII), 312. Available online at: https://journals.openedition.org/rao/8880 (accessed December 21, 2024).
Sahle, Y., Morgan, L. E., Braun, D. R., Atnafu, B., and Hutchings, W. K. (2014). Chronological and behavioral contexts of the earliest middle stone age in the gademotta formation, main ethiopian rift. Quat. Int. 331, 6–19. doi: 10.1016/j.quaint.2013.03.010
Schepers, C., Lesur, J., and Vogelsang, R. (2020). Hunter-gatherers of the high-altitude afromontane forest – the holocene occupation of mount dendi, Ethiopia. Azania 55, 329–359. doi: 10.1080/0067270X.2020.1792709
Schlüter, T. (2008). Geological Atlas of Africa: With Notes on Stratigraphy, Tectonics, Economic Geology, Geohazards and Geosites of Each Country, 2nd Edn revised and enlarged. Cham: Springer.
Shea, J. J. (2008). The middle stone age archaeology of the lower omo valley kibish formation: excavations, lithic assemblages, and inferred patterns of early homo sapiens behavior. J. Hum. Evol. 55, 448–485. doi: 10.1016/j.jhevol.2008.05.014
Solomon, T. (2020). Understanding the Beginning of Herding through the Study of Rock Art: The Case of Borana in Southern Ethiopia. Ferrara: University of Ferrara.
Stewart, B. A., Zhao, Y., Mitchell, P. J., Dewar, G., Gleason, J. D., and Blum, J. D. (2020). Ostrich eggshell bead strontium isotopes reveal persistent macroscale social networking across late quaternary southern africa. Proc. Natl. Acad. Sci. U.S.A. 117, 6453–6462. doi: 10.1073/pnas.1921037117
Teffera, F. E., Lemmens, P., Deriemaecker, A., Deckers, J., Bauer, H., Gamo, F. W., et al. (2019). Why are lake abaya and lake chamo so different? A limnological comparison of two neighboring major ethiopian rift valley lakes. Hydrobiologia 829, 113–124. doi: 10.1007/s10750-018-3707-8
Teklay, M., Kröner, A., Mezger, K., and Oberhänsli, R. (1998). Geochemistry, Pb Pb single zircon ages and Nd Sr isotope composition of precambrian rocks from southern and eastern ethiopia: implications for crustal evolution in east africa. J. Afr. Earth Sci. 26, 207–227. doi: 10.1016/S0899-5362(98)00006-2
Tryon, C. A., and Faith, J. T. (2016). A demographic perspective on the middle to later stone age transition from nasera rockshelter, Tanzania. Philos. Trans. R. Soc. B Biol. Sci. 371:20150238. doi: 10.1098/rstb.2015.0238
Wang, X., Bocksberger, G., Arandjelovic, M., Agbor, A., Angedakin, S., Aubert, F., et al. (2024). Strontium isoscape of sub-Saharan Africa allows tracing origins of victims of the transatlantic slave trade. Nat. Commun. 15:10891. doi: 10.1038/s41467-024-55256-0
Wang, X., Bocksberger, G., Lautenschläger, T., Finckh, M., Meller, P., O'Malley, G. E., et al. (2023). A bioavailable strontium isoscape of angola with implications for the archaeology of the transatlantic slave trade. J. Archaeol. Sci. 154:105775. doi: 10.1016/j.jas.2023.105775
Willmes, M., Bataille, C. P., James, H. F., Moffat, I., McMorrow, L., Kinsley, L., et al. (2021). Mapping of bioavailable strontium isotope ratios in france for archaeological provenance studies. Open Science Framework. 90, 75–86. doi: 10.31219/osf.io/txasq
Willmes, M., McMorrow, L., Kinsley, L., Armstrong, R., Aubert, M., Eggins, S., et al. (2014). The IRHUM (isotopic reconstruction of human migration) database – bioavailable strontium isotope ratios for geochemical fingerprinting in France. Earth Syst. Sci. Data 6, 117–122. doi: 10.5194/essdd-6-761-2013
WoldeGabriel, G., Heiken, G., White, T. D., Asfaw, B., Hart, W. K., and Renne, P. R. (2000). “Volcanism, tectonism, sedimentation, and the paleoanthropo-logical record in the Ethiopian Rift System,” in Volcanic Hazards and Disasters in Human Antiquity, eds. F. W. McCoy, and G. Heiken (Boulder, CO: Geological Society of America), Special Paper 345. doi: 10.1130/0-8137-2345-0.83
Wood, B., and Leakey, M. (2011). The omo-turkana basin fossil hominins and their contribution to our understanding of human evolution in Africa. Evol. Anthropol. Issues News Rev. 20, 264–292. doi: 10.1002/evan.20335
Yibas, B., Reimold, W. U., Armstrong, R., Koeberl, C., Anhaeusser, C. R., Phillips, D., et al. (2002). The tectonostratigraphy, granitoid geochronology and geological evolution of the precambrian of Southern Ethiopia. J. Afr. Earth Sci. 34, 57–84. doi: 10.1016/S0899-5362(01)00099-9
Keywords: strontium isotopes, bioavailable isoscape, faunal mobility, hominin land use, MSA, Southern Ethiopia, paleoecology
Citation: Asrat S, Lucchini F, Tafuri MA, Aureli C, Gallinaro M, Zerboni A, Fusco M and Spinapolice EE (2025) A strontium (87Sr/86Sr) isoscape of Southern Ethiopia: implications for hominin land use and faunal mobility patterns. Front. Environ. Archaeol. 4:1499291. doi: 10.3389/fearc.2025.1499291
Received: 20 September 2024; Accepted: 11 March 2025;
Published: 22 April 2025.
Edited by:
Sally Christine Reynolds, Bournemouth University, United KingdomReviewed by:
Emmanuel Ndiema, National Museums of Kenya, KenyaCharlotte King, University of Otago, New Zealand
Copyright © 2025 Asrat, Lucchini, Tafuri, Aureli, Gallinaro, Zerboni, Fusco and Spinapolice. This is an open-access article distributed under the terms of the Creative Commons Attribution License (CC BY). The use, distribution or reproduction in other forums is permitted, provided the original author(s) and the copyright owner(s) are credited and that the original publication in this journal is cited, in accordance with accepted academic practice. No use, distribution or reproduction is permitted which does not comply with these terms.
*Correspondence: Seminew Asrat, c2VtaW5ld2FzcmF0Lm1vZ2VzaWVAdW5pcm9tYTEuaXQ=; Enza E. Spinapolice, ZW56YWVsZW5hLnNwaW5hcG9saWNlQHVuaXJvbWExLml0