- 1 Department of Biochemistry, National Cerebral and Cardiovascular Center Research Institute, Suita, Osaka, Japan
- 2 Department of Biological Science, Graduated School of Science and Engineering, University of Toyama, Toyama, Japan
- 3 Laboratory of Integrative Brain Science, Department of Biology, Waseda University, Tokyo, Japan
We have identified cDNA encoding a functional growth hormone secretagogue-receptor 1a (GHS-R1a, ghrelin receptor) in two species of anuran amphibian, the bullfrog (Rana catesbeiana), and the Japanese tree frog (Hyla japonica). Deduced receptor protein for bullfrog and Japanese tree frog (tree frog) was comprised of 374- and 371-amino acids, respectively. The two receptors shared 86% identity, and are grouped to the clade of the tetrapod homologs by phylogenetic analysis. In functional analyses, ghrelin and GHS-R1a agonists increased intracellular Ca2+ concentration in GHS-R1a-transfected-HEK293 cell, but ligand selectivity of ghrelin with Ser3 and Thr3 was not observed between the two receptors. Bullfrog GHS-R1a mRNA was mainly expressed in the brain, stomach, and testis. In the brain, the gene expression was detected in the diencephalon and mesencephalon, but not in the pituitary. Tree frog GHS-R1a mRNA was predominantly expressed in the gastrointestinal tract and ovary, but not detected in the pituitary. In bullfrog stomach but not the brain, GHS-R1a mRNA expression increased after 10 days of fasting. For tree frog, GHS-R1a mRNA expression was increased in the brain, stomach and ventral skin by 10 days of fasting, and in the stomach and ventral skin by a dehydration treatment. Intracerebroventricular injection of ghrelin in dehydrated tree frog did not affect water absorption from the ventral skin. These results suggest that ghrelin is involved in energy homeostasis and possibly in osmoregulation in frogs.
Introduction
Ghrelin, a 28-amino-acid peptide in which the third serine residue (Ser3) has an n-octanoyl modification, is an endogenous ligand for the growth hormone (GH) secretagogue-receptor 1a (GHS-R1a), and was first identified in the stomach of rats and humans (Kojima et al., 1999). Ghrelin was identified as a GH-releasing peptide, but accumulating evidences have demonstrated that ghrelin plays important roles in appetite regulation, glucose metabolism, cardiovascular function and gastrointestinal functions including motility and gastric acid secretion, and memory (Kojima and Kangawa, 2005). The multiple roles of ghrelin are supported by the wide expression of mRNA and protein for GHS-R1a from the central nervous system to several peripheral tissues (Papotti et al., 2000; Gnanapavan et al., 2002; Kojima and Kangawa, 2005). In mammals, two isoforms of GHS-R, a functional receptor, GHS-R1a, and an alternative splice variant, GHS-R1b, have been known (Davenport et al., 2005).
Ghrelin is also present in non-mammalian vertebrates and shows various species-specific actions (Kaiya et al., 2007, 2008). In amphibians, ghrelin has been identified in bullfrog (Rana catesbeiana; Kaiya et al., 2001). The mature-peptide length is consists of 28 amino acids, although having the same length as the mammalian counterparts, it only shares 30% sequence identity to human ghrelin. Recently, partial sequence of prepro-ghrelin of an edible frog (Pelophylax esculentus, previously: Rana esculenta) has been reported (Izzo et al., 2011). The amino acid sequence of mature-ghrelin is almost identical; only the 13th amino acid is changed from arginine (Arg) to glutamine (Gln), while the third amino acid, threonine (Thr3), is conserved as in bullfrog. Particularly, the third acylated amino acid was changed to threonine instead of serine as seen in mammals. This substitution of amino acid affects ghrelin’s biological activity: bullfrog ghrelin is 100-times stronger in stimulating both GH and prolactin (PRL) secretion from dispersed bullfrog pituitary cells, when compared to rat ghrelin. On the other hand, a weak effect is observed in GH secretion when bullfrog ghrelin was injected intravenously into rats (Kaiya et al., 2001). This suggests that bullfrog ghrelin binds to rat GHS-R1a with different affinity to rat ghrelin, and the reversed phenomenon could be seen for the bullfrog ghrelin receptor.
Since bullfrog ghrelin has Thr3, it is speculated that ghrelin in all amphibians including anuran and urodele have Thr3. Recently, we found that only frog ghrelin of the genus, Rana, has Thr3, but other frogs such as Xenopus laevis, Hyla japonica, and Bufo japonicus and newts such as Cynops pyrrhogaster and Cynops ensicauda have Ser3 (Kaiya et al., 2010). This suggests that comparative study of the two species of frogs, which has Thr3 or Ser3, may provide valuable information on ligand–receptor interaction of the ghrelin system including ligand selectivity of the receptor.
The aim of this study is to isolate cDNA of functional ghrelin receptor (GHS-Ra) in two species of frogs, bullfrog, and Japanese tree frog (tree frog, H. japonica), which have Thr3 and Ser3 in the ghrelin, respectively, for the comparison of the differences in the structure and tissue distribution, as well as functional activity of the receptor. In addition, we examined possible involvement of the ghrelin system in energy metabolism in these frogs by food deprivation experiment. Furthermore, since tree frog is often used for the study of osmoregulation (Tokuda et al., 1995; Kohno et al., 2003; Yamada et al., 2007; Maejima et al., 2010) and ghrelin has been reported to inhibit water intake in rats, chickens and eels (Kozaka et al., 2003; Tachibana et al., 2006; Hashimoto et al., 2007; Hashimoto and Ueta, 2011), we have also examined the effect of ghrelin on water absorption in tree frog.
Materials and Methods
All experiments were performed according to the Guideline for Care and Use of Animals approved by the ethical committee of the University of Toyama, Waseda University, or National Cerebral and Cardiovascular Center.
Cloning of Ghrelin Receptor
Total RNA was extracted by TRIzol (Invitrogen, Grand Island, NY, USA) from frozen whole brain. Total RNA (1 μg) was reverse-transcribed with 3′-oligo-dT primer of the Gene Racer Kit (Invitrogen) using QuantiTect RT Kit (QIAGEN GmbH, Hilden, Germany). Primary PCR was performed with the template, a primer set (100 pmol/μl GHSR-dSES1 and GHSR-dANT1, Table 1) and ExTaq DNA polymerase (TaKaRa Bio, Shiga, Japan). The reaction conditions were 94°C for 2 min and subsequent 35 cycles of 94°C for 1 min, 53°C for 0.5 min, and 72°C for 1 min; and final extension was 72°C for 10 min. The amplified product was purified by a Wizard PCR Preps DNA Purification System (Promega, Madison, WI, USA), and 2μl (1/25 volume) of the purified product was used as template of the second-round nested PCR, which was performed under the same conditions as those of primary PCR except for using another primer set (GHSR-dSES2 and GHSR-dANT1, Table 1), PCR-prepsed template. The resultant product was subcloned into the pCRII-TOPO vector (Invitrogen), and the nucleotide sequence of the insert was determined by automated sequencing (Model 3130, Applied Biosystems, Foster City, CA, USA) according to the protocol of the BigDye™ Terminator Cycle Sequencing Kit (Applied Biosystems).
For 3′-RACE PCR, primary PCR was performed with the gene-specific primer (GSP), bfGHSR-RACE-s1 for bullfrog and hylaGHSR 3R-SES1 for tree frog (Table 1), and the 3′-primer in the kit and ExTaq DNA polymerase. The reaction conditions were 95°C for 2 min and subsequent 35 cycles of 95°C for 0.5 min, 57°C for 0.5 min, and 72°C for 1 min; and final extension was 72°C for 3 min. After PCR preps of the amplified product, nested PCR was performed with another GSP, bfGHSR-RACE-s3 for bullfrog and hylaGHSR 3R-SES2 for tree frog (Table 1), and 3′-nested primer in the kit and ExTaq DNA polymerase under the same conditions as those for the primary PCR.
For 5′-RACE PCR, first-strand cDNAs were synthesized from 5 μg of total RNA with an anti-sense GSP, bfGHSR-AS1 for bullfrog and hylaGHSR 5R-ANT-X (Table 1). Primary PCR was conducted using anti-sense GSP, bfGHSR-AS3 for bullfrog or hylaGHSR 5R-ANT-Y for tree frog (Table 1), 5′ primer in the kit, ExTaq DNA polymerase, and 2.5% DMSO with amplification conditions at 95°C for 2 min and subsequent 35 cycles of 95°C for 0.5 min, 55°C for 0.5 min, and 72°C for 1 min and final extension was 72°C for 3 min. After PCR preps of the product, nested PCR was performed using another anti-sense GSP, bfGHSR-AS2 for bullfrog and hylaGHSR 5R-ANT-Y for tree frog (Table 1), and 5′-nested primer in the kit only changing annealing temperature to 58°C. For bullfrog, a 1715-bp product that contains an unexpected stop codon within a candidate nucleotide sequence was isolated. Hence, we designed a sense primer within the sequence at the 5′-end of the product (bfGHSR-G-s1, Table 1), and genomic PCR was performed with bfGHSR-G-s1, bfGHSR-AS4, ExTaq DNA polymerase and 2.5% DMSO under the amplification conditions at 95°C for 2 min and subsequent 35 cycles of 95°C for 0.5 min, 56°C for 0.5 min, and 72°C for 1 min; and final extension was 72°C for 3 min. As a result, a 1.4-kb product was isolated, and two initiating ATG codon were found in the candidate sequence. Therefore, we designed two sense primers for cloning the coding region of bfGHS-Ra (bfGHSR-code-s1 and -s2, Table 1).
Functional Analysis of Ghrelin Receptor
To examine functional activity of identified receptor protein, we constructed expression vector for mammalian cells containing the coding region of frog GHS-Ra proteins. The cDNA that encodes coding region of frog GHS-Ra proteins was amplified and subcloned into the pcDNA3.1-V5-His-TOPO vector (Invitrogen) after overhang reaction if necessary. RT-PCR for bfGHS-Ra was performed using whole brain cDNA template, a primer set, bfGHSR-code-s1 and bfGHSR-code-AS, pyrobest DNA polymerase (TaKaRa Bio) under the following reaction conditions: 95°C for 2 min and subsequent 35 cycles of 95°C for 0.5 min, 57°C for 0.5 min and 72°C for 1 min, and final extension was 72°C for 3 min. hjGHS-Ra cDNA was amplified using a primer set, hjGHSR-code-s and hjGHSR-code-AS, HotSTAR Taq Plus mix (QIAGEN GmbH) with reaction conditions at 95°C for 5 min and subsequent 35 cycles of 95°C for 0.5 min, 55°C for 0.5 min, and 72°C for 1.5 min and final extension at 72°C for 3 min. The amplified cDNA was subcloned, and sequenced for correct orientation, and subsequently cultured at 50-ml scale. The plasmid vector was then isolated using a HiSpeed Plasmid Midi Kit (QIAGEN GmbH) and was diluted to 1 μg/μl for transfection experiment.
Changes in intracellular Ca2+ concentrations were measured using FLIPRtetra (Molecular Devices, Menlo Park, CA, USA). Human embryonic kidney 293 (HEK293) cells were cultured in DMEM containing 10% fetal calf serum at a density of 1 × 106 cells in a collagen-coated 10-cm dish for 24 h. An expression vector (2.5 μg) was transfected with FuGENE6 (Roche Diagnostics, Mannheim, Germany) according to the manufacturer’s protocol. After twenty-four hours of transfection, the cells were plated onto a poly-D-lysine- (Sigma Chemical, St. Louis, MO, USA) coated 96-well black plate (Corning Inc., Wilkes Barre, PA, USA) at a density of 3 × 104 cells per well. After twenty hours of plating, cultured medium was aspirated, and 100 μl fluorescent dye solution containing 4.4 μM Fluo-4AM (Invitrogen) and 1% FCS, 1% Power load concentrate (Invitrogen) in a working buffer [1 × Hanks’ BSS (Invitrogen)-20 mM HEPES buffer containing 250 μM probenecid (Sigma Chemical)] was loaded into each well. The plate was incubated for 1 h at room temperature, and the plate was washed three times with a working buffer by an automatic washing machine, followed by the addition of 100 μl synthetic octanoylated bullfrog ghrelin 28-C8, decanoylated bullfrog ghrelin 28-C10 (custom synthesis at ASBIO Pharma Co. Ltd., Gunma, Japan), rat ghrelin (Peptide Institute Inc., Osaka, Japan), GHRP-6 (Bachem AG, Bubendorf, Switzerland), or hexarelin (Phoenix Pharmaceutical Inc., Belmont, CA, USA) at doses of 0, 0.1, 1, 10, 100, and 1000 nM in a working buffer containing 0.001% Triton X-100 using the automated FLIPR system. Changes in intracellular Ca2+ concentrations were measured by excitation at 488 nm and emission at 500–560 nm.
Quantitative Real-Time PCR
Quantitative real-time PCR (qPCR) was used for quantification of gastric and brain mRNA of GHS-Ra in the starvation experiment for bullfrog. First-strand DNA was synthesized from 1 μg of total RNA using QuantiTect RT Kit (QIAGEN GmbH) with oligo-dT primer. qPCR was performed for GHS-Ra and β-actin with two primer sets, bfGHSR-Q-s and bfGHSR-Q-AS, and bfB-actin-s and bfB-actin-AS, respectively (Table 1). The expected amplicon size was 240 bp and 255 bp, respectively.
Quantitative real-time PCR was performed using a LightCycler 480 (Roche Applied Science, Mannheim, Germany) and a QuantiFAST SYBR Green PCR Kit (QIAGEN GmbH). The amplification conditions were 95°C for 5 min, and subsequent 40 cycles at 95°C for 10 s and 60°C for 30 s. The reaction mixture consisted of 1× master mix, 250 nM of each primer and template (100 ng total RNA equivalent). For quantification of GHS-Ra mRNA copy number, a linear regression line was generated using a serially diluted pCRII vector containing each desired cloned cDNA that was linearized by restriction with XbaI.
Tissue Distribution of GHS-Ra mRNA
For bullfrog tissues, first-strand DNA was synthesized from 1 μg of total RNA using QuantiTect RT Kit (QIAGEN GmbH) with oligo-dT primer. PCR was performed with HotSTAR Taq Plus mix (QIAGEN GmbH) using bfGHSR-code-s1 and bfGHSR-AS4 for GHS-Ra, bfB-actin-s and bfB-actin-AS for β-actin, and Frog GRL-s2 and Frog GRL-AS2 for ghrelin (Table 1). The expected amplicon size was 240, 255, and 237 bp, respectively. The reaction conditions were 95°C for 5 min and subsequent 35 cycles of 95°C for 0.5 min, 57°C for 0.5 min, and 72°C for 0.5 min.
For tree frog, first-strand DNA was synthesized from 1 μg of total RNA using QuantiTect RT Kit (QIAGEN GmbH) with oligo-dT primer. PCR was performed for GHS-Ra and β-actin with hjGHSR-Q-s and hjGHSR-Q-AS, and hjB-actin-s and hjB-actin-AS were used for the analyses (Table 1). The expected amplicon size was 195 and 227 bp, respectively. PCR was performed using a LightCycler 480 and a QuantiFAST SYBR Green PCR Kit under condition described above.
Starvation Experiment in Bullfrog
Juvenile bullfrogs of both sexes, weighing 22–25 g, were randomly divided into four control and four experimental groups. Animals were kept in eight plastic containers (73 cm × 42 cm × 20 cm) under artificial illumination (12:12h dark–light cycle) and constant temperature (23°C). All frogs were provided with food (approximately 0.3 g of bovine liver) once daily at early evening (5:00–6:30 P.M.) for the 3 days before experiment. Control animals were provided with food throughout the experimental period. Frogs in food-deprived group were sacrificed 0, 5, 10, and 20 days after scheduled food deprivation. Frogs in the control groups were sacrificed according to the same schedule as the experimental groups. After pithing each animal, stomach and whole brain were excised from each frog, frozen in liquid nitrogen and stored at −80°C until use. The mRNA expression level of GHS-Ra was quantified by qPCR as described above.
Starvation or Dehydration Experiment in Tree Frogs
Adult tree frogs of both sexes, weighing 1.3–2.3 g, were bred in breading cages under artificial illumination (12:12h dark–light cycle) and temperature (23–28°C) for at least more than 1 week before experiment. They were allowed free access to water, and were fed with mealworm or cricket twice a week.
Frogs were kept at constant temperature (23°C) when moved to the experimental condition, and were divided into three groups: (a) dehydration group, which decreased their body weight (BW) to 20% from the initial weight with feeding after 3 to 4 days (Maejima et al., 2008), (b) fasting group, which were food deprived for 10 days with free access to water, and (c) intact group, which were kept in free access to water, and feed was given at once every 2 days. The 10-days of fasting period was referred in the result of bullfrog (section Starvation experiment in bullfrog). A 10-days fasting is considered to be enough to induce negative energy balance because of the smaller body size of tree frogs when compared to bullfrog. Tissues (brain, heart, stomach, intestine, kidney, urinary bladder, and ventral skin) were collected from each group and stored at −80°C. Total RNA was isolated using ISOGEN (Nippon gene, Tokyo, Japan), and DNase-I-treated total RNA (1 μg) was reverse-transcribed with the PrimeScript first-strand cDNA Synthesis Kit (TaKaRa Bio). RT-PCR for GHS-Ra (311 bp) was performed using hylaGHSR 3R-SES2 and hyGHSR-spANT for 28 cycles, while for GAPDH (362 bp), frog GAPDH-SES and ANT were used with 25 cycles of amplification (Table 1). The PCR products were electrophoresed by 2% agarose gel containing 0.005% ethidium bromide, and the band densities were analyzed using Scion Image software (Scion Corporation, MD, USA). The mRNA expression level of GHS-Ra was normalized by that of GAPDH. Three to four RNA samples were used for the analysis, and repeated three times to examine the reproducibility of expression patterns.
Effect of Intracerebroventricular (ICV) Injection of Ghrelin on Water Absorption in Tree Frogs
This experiment was carried out in the autumn (October and November), and was based on the methods previously described, except dehydration treatment after fully hydration (Maejima et al., 2008, 2010). Frogs of both sexes were continuously kept in tap water for 24 h in order to maintain a fully hydrated condition. Dehydration treatment was then done until their BW lost approximately 13% from the initial level. For ICV injection, a small part of the parietal bone was carefully removed under anesthesia with 0.1% MS-222 (3-aminobenzoic acid ethyl ester, Sigma, St. Louis, MO, USA). Under a dissecting microscope, 0.2 μl/g BW of rat ghrelin solution [82.5 ng (25 pmol)/g BW] containing Evans blue dye was injected into the third ventricle (a middle portion between the telencephalon and mesencephalon with 0.8 mm depth) by a manipulator and 10-μl Hamilton syringe. Injected dose of ghrelin referred the previous study for arginine vasotocin (Maejima et al., 2008). Frogs in the control group were injected with frog Ringer’s solution which contained (mM): NaCl, 111; CaCl2, 2.70; KCl, 3.35; NaHCO3, 2.38; glucose, 5.50; pH 7.4. In addition, intact group without injection treatment was also prepared. After allowing recovery time for 15 min, the treated frog was put into the water dish (5 cm × 5 cm) containing tap water in the plastic cage (L20 cm × W30 cm × H20 cm). The behavior was recorded for 360 min with a video-camcorder since the frog tended to stay for longer time than previous experiments even in the hydrated condition (Maejima et al., 2008, 2010). After recording, the time staying in the water dish, which is called residence time, was analyzed. This residence time was defined as water absorption through the pelvic skin, and was compared among ghrelin-injected, frog Ringer’s solution-injected and intact groups. After termination of the experiment, position of Evans blue dye was confirmed to ensure that the injected solution was accurately administered into the designated position.
Statistical Analysis
The results are expressed as means ± SEM. The significance of differences between the values was determined at P < 0.05 using unpaired Student’s t-test for single comparisons or one-way ANOVA followed by Bonferroni/Dunn test for multiple comparisons.
Results
Identification of Ghrelin Receptor-Like Receptor cDNA
We identified cDNA encoding GHS-Ra-like protein from each frog. The open reading frame (ORF) of bullfrog cDNA was 1125 bp, which encodes a 374-amino acid protein (Figure 1, acc# AB626731), while ORF of tree frog cDNA was 1116 bp, which encodes a 371-amino acid protein (Figure 2, acc# AB626732). Comparison of the deduced amino acid sequences to other GHS-Ra sequences showed that numerous consensus sequences for GHS-Ra were found in the identified proteins (Figure 3). Frog GHS-Ra-like proteins shared 86% identity with each other (Figure 4A). Chicken GHS-R1a and quail GHS-R1aS showed the highest identity (74%) to the frog proteins (Figure 4B). Phylogenetic analysis showed that bullfrog and tree frog GHS-Ra-like proteins belong to the same branch with clawed frog (X. laevis), and other tetrapods such as birds and mammals were contained in the same sub-clade (Figure 4B). Interestingly, a branch including frog GHS-Ra-like proteins belongs to a sub-clade of fish GHS-R2a proteins.
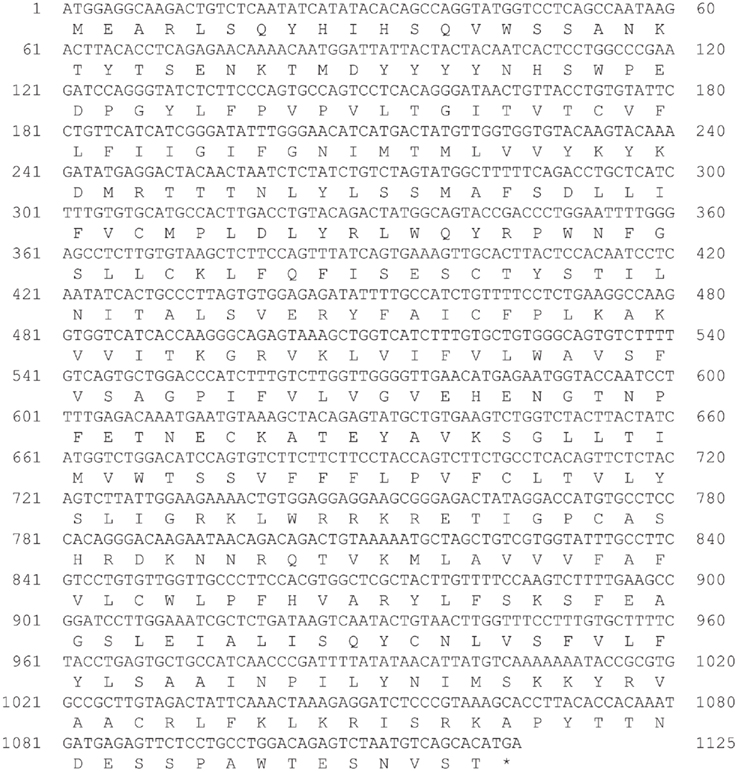
Figure 1. Nucleotide and deduced amino acid sequences of growth hormone secretagogue-receptor (GHS-R) a-like receptor for bullfrog. Asterisk indicates the termination codon (TGA).
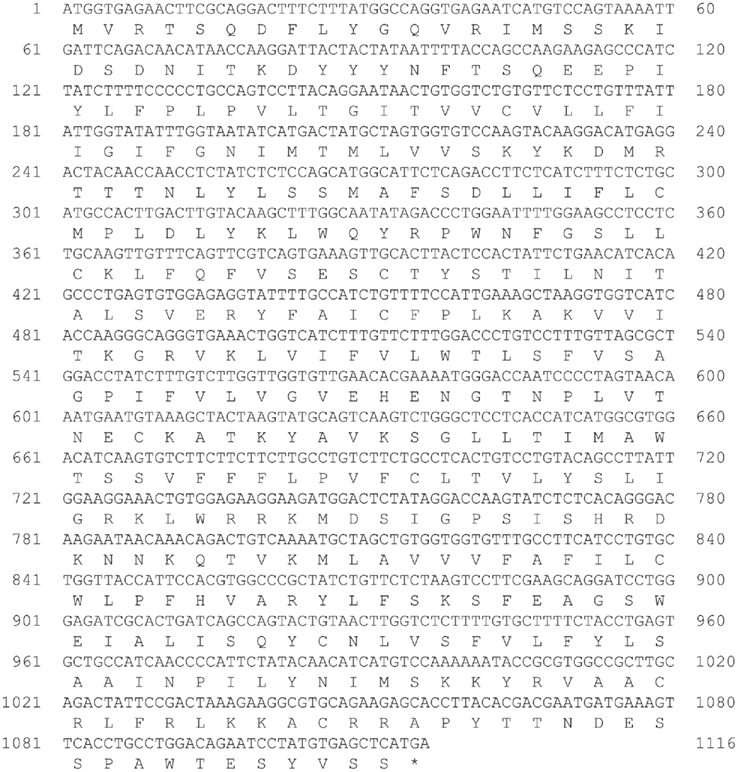
Figure 2. Nucleotide and deduced amino acid sequences of growth hormone secretagogue-receptor (GHS-R) a-like receptor for Japanese tree frog. Asterisk indicates the termination codon (TGA).
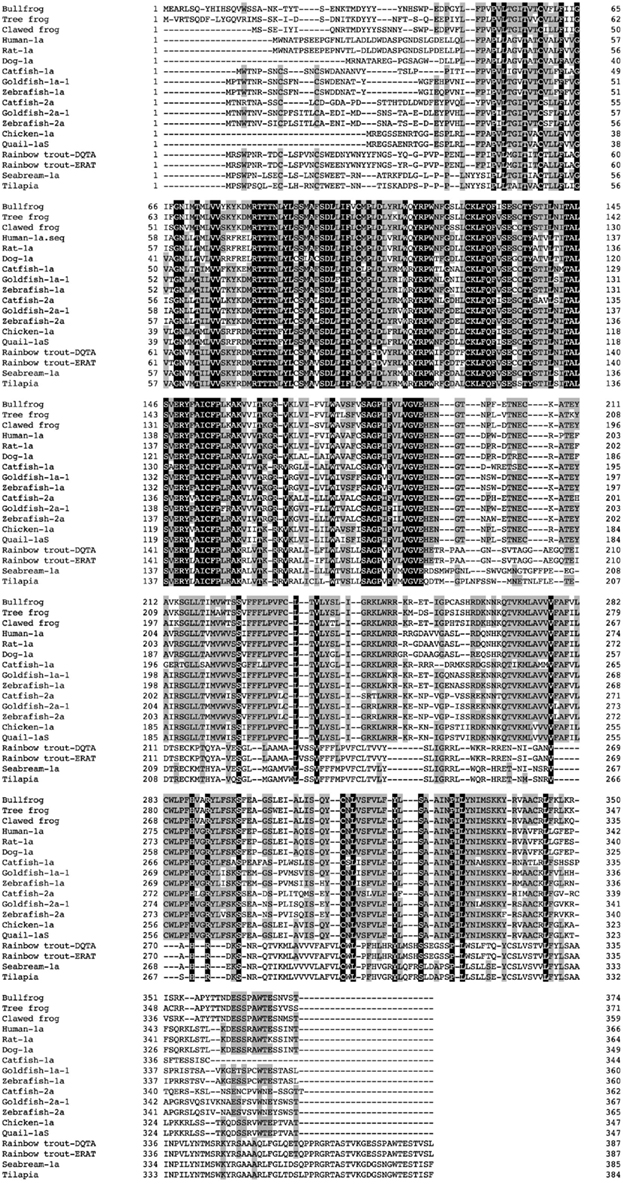
Figure 3. Comparison of the amino acid sequence of frog GHS-Ra-like receptor with other GHS-Ra and GHSR1a-like receptors. The boxed letters indicate identical amino acids across all species, and the shaded letters show identical amino acids in more than half of the examined species. Amino acid sequences are available from the DDBJ/EMBL/GenBank databases: clawed frog (XM_002931572), human-1a (NM_198407), rat-1a (NM_032075), dog-1a (NM_001099945), catfish-1a (FJ707319), goldfish-1a-1 (AB504275), zebrafish-1a (XM001335981), catfish-2a (FJ707320), goldfish-2a-1 (AB504277), zebrafish-2a (XM001340372), chicken-1a (AB095995), quail-1aS (AB469019), rainbow trout GHSR1a-like receptor (GHSR1a-LR)-DQTA (AB362479), rainbow trout GHSR1a-LR-ERAT (AB362480), seabream-1a (AY151040), tilapia GHSR1a-LR (AB361053).
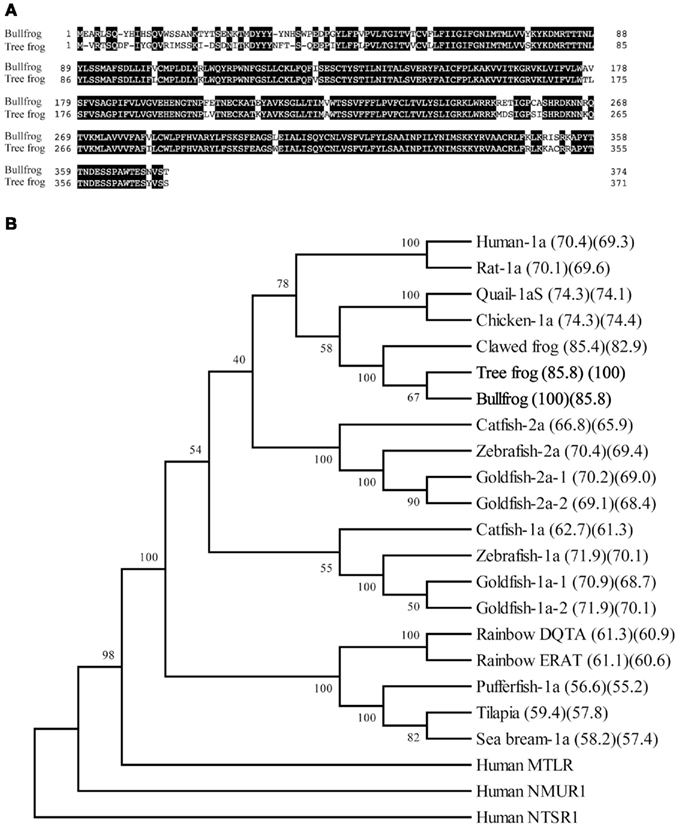
Figure 4. Comparison of the amino acid sequence of GHS-Ra-like receptor between bullfrog and Japanese tree frog (A), and phylogenetic analysis of GHS-Ra and related receptors (B). (A) The boxed letters indicate identical amino acids between the two species. The sequence identity is 86%. (B) A phylogenetic tree was generated by the NJ method of MEGA4 (http://www.megasoftware.net/). Human motilin receptor (MTLR), neuromedin U receptor-1 (NMUR1), and neurotensin receptor-1 (NTSR1) were included as on out-group. The numbers at branch points represent bootstrap values (1000 repetitions). The value in parenthesis indicates amino acid sequence identity to bullfrog (left) and tree frog (right). Amino acid sequences except those indicated in Figure 3 are available in the DDBJ/EMBL/GenBank databases: human MTLR (NM_001507), human NMUR1 (NM_006056), human NTSR1 (NM_002531).
Functional Analyses of Identified Ghrelin Receptor-Like Proteins
The two identified GHS-Ra-like receptor cDNAs were transiently expressed in HEK 293 cells, and the cells were treated with bullfrog ghrelin with n-octanoyl (C8) or n-decanoyl (C10) modification and rat ghrelin (Figure 5A,B), and two different GHSs, GHRP-6 or hexarelin (Figure 5C,D). In both bullfrog (Figure 5A) and tree frog (Figure 5B), GHS-Ra-like receptors showed the same degree of responses to bullfrog and rat ghrelin at concentrations from 0 to 1000 nM. No differences in the response pattern were seen among the three ghrelin species. Both GHRP-6 and hexarelin showed 1/10 less potency for inducing intracellular Ca2+ signaling in bullfrog (Figure 5C) and tree frog (Figure 5D) GHS-Ra-like receptors, when compared with bullfrog ghrelin 28-C8, although the pattern of the dose response was similar between bullfrog and tree frog receptors. Substantiated by these results, we can confirmed that the presently identified receptors in bullfrog and tree frog were functional ghrelin receptor (GHS-Ra).
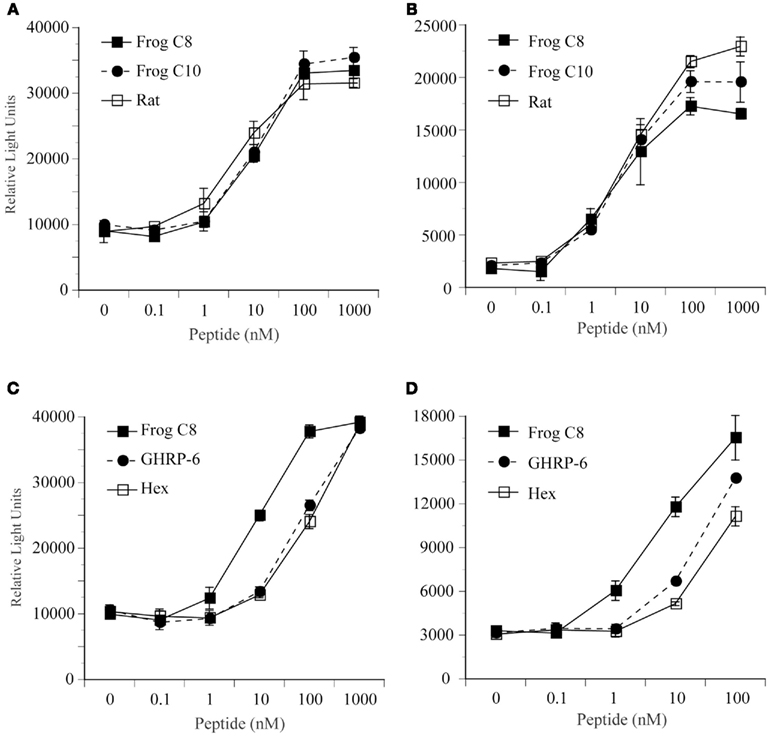
Figure 5. Functional analysis of GHS-R1a for bullfrog and Japanese tree frog. Changes in intracellular Ca2+ concentrations were examined in human embryonic kidney (HEK) 293 cells that were transfected with GHS-R1a cDNA for bullfrog (A,C) and for Japanese tree frog (B,D). These cells were treated with bullfrog ghrelin 28-C8, 28-C10, and rat ghrelin (A,B), and bullfrog ghrelin 28-C8, GHRP-6, and hexarelin (C,D) at concentrations of 0, 0.1, 1, 10, 100, or 1000 nM. Values represent the means ± SEM of triplicate experiments by one assay.
Expression of Ghrelin Receptor mRNA in Bullfrog and Tree Frog
In bullfrog, RT-PCR revealed that GHS-Ra mRNA was highly expressed in the brain, stomach and testis, and to a lesser extent in the small and large intestines, adrenal gland, and kidney (Figure 6A), while ghrelin mRNA was only detected in the stomach. We examined detail distribution of GHS-Ra mRNA in bullfrog brain (Figure 6B). mRNA expression was detected in the diencephalon and mesencephalon, but not in the telencephalon and pituitary in both male and female frogs.
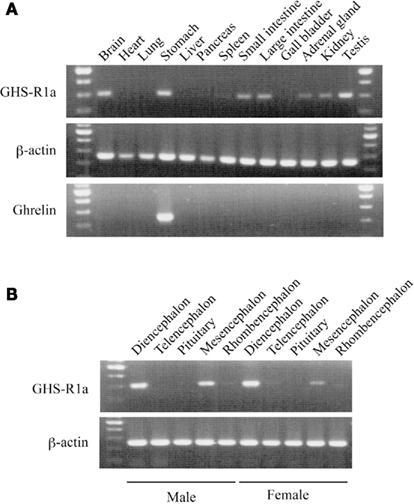
Figure 6. Growth hormone secretagogue-receptor 1a mRNA expression in bullfrog tissues. (A) Body tissues of a male frog. Upper, middle and lower columns indicate GHS-R1a, β-actin and ghrelin, respectively. (B) Five parts of brain region. The former five tissues are obtained from a male and the latter five are from a female. Upper and lower columns indicate the result of GHS-R1a and β-actin, respectively. All gel views are obtained by 35 cycles of PCR.
In tree frog, RT-PCR revealed that GHS-Ra mRNA was expressed in almost all tissues examined, and relatively high expression was seen in the intestines such as duodenum, small and large intestines in both male and female (Figure 7). In female frogs, a high expression of GHS-Ra mRNA was detected in the ovary.
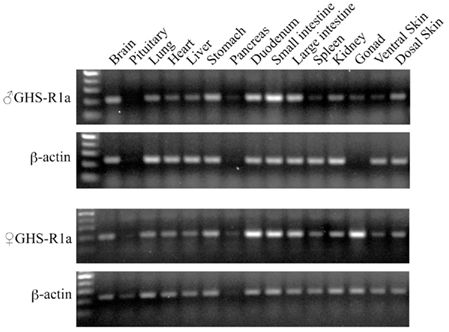
Figure 7. Growth hormone secretagogue-receptor 1a mRNA expression in Japanese tree frog tissues. The gel views represent the result of GHS-R1a (28 cycles PCR) and β-actin (18 cycles PCR), respectively, in body tissues of a male (upper two columns) and a female (lower two columns).
Effects of Fasting on the Ghrelin Receptor mRNA Expression in Bullfrog Stomach and Brain
Expression of GHS-Ra mRNA in the stomach for fed frogs did not show significant changes during feed deprivation period for 20 days (Figure 8A). Expression of GHS-Ra mRNA in the stomach for fasted frogs significantly increased after 10 days of fasting when compared with the respective control, although it was not statistically significant when compared with the level at day 0. The increased levels returned to the control level after 20 days of fasting. Expression of GHS-Ra mRNA in the whole brain did not change for 20 days of fasting period (Figure 8B).
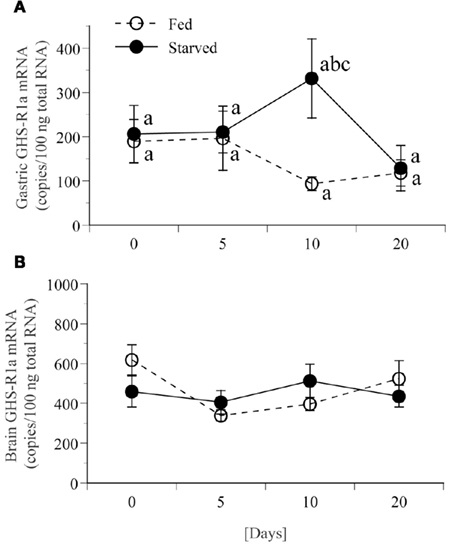
Figure 8. Changes in GHS-R1a mRNA expression in stomach and brain of bullfrog for 20 days food deprivation. mRNA expression levels were quantified by real-time PCR in stomach (A) or brain (B) with duplicate measurements. White and black circles indicate the result for fed and fasted frogs, respectively. Values are the means ± SEM (n = 8). Statistical analysis (non-repeated ANOVA followed by Bonferroni/Dunn test) was conducted. Different letters indicate statistical differences (P < 0.05): b, 10-days fed group vs. 10-days fasted group; c, 20-days fed group vs. 10-days fasted group.
Effects of Fasting and Dehydration on the Ghrelin Receptor mRNA Expression in Tree Frog
Ten-days of food deprivation significantly increased GHS-Ra mRNA expression in the stomach and ventral skin of tree frog, when compared with the respective control (Figure 9A). Dehydration by decreased BW to 20% significantly increased mRNA expression of GHS-Ra in the whole brain, stomach and ventral skin, compared with the respective control (Figure 9B).
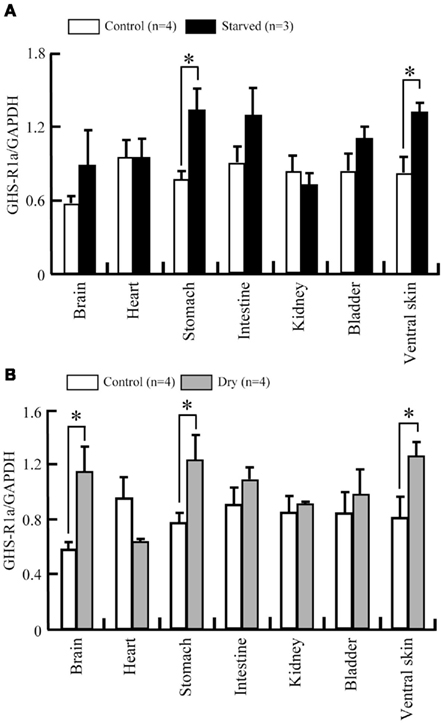
Figure 9. Changes in GHS-R1a mRNA expression in a 10-days starvation and dehydration treatment of Japanese tree frog. mRNA expression levels were estimated semi-quantitatively as a ratio relative to GAPDH mRNA expression in a 10-days starvation experiment (A) and dehydration experiment (B). Values are the means + SEM. *P < 0.05 compared to the control (unpaired Student’s t-test).
Effects of ICV Injection of Ghrelin on Water Absorption from the Ventral Skin in Tree Frog
Dehydration increased GHS-Ra mRNA expression in the ventral skin (Figure 9B). Ghrelin may affect water absorption in dehydrated frog. We injected rat ghrelin (Ser3-ghrelin) into the lateral ventricle of the brain in 13% dehydrated tree frogs, and examined the effect on water absorption. There was no difference in the residence time among ghrelin-injected, frog Ringer’s-injected and intact frogs (Figure 10).
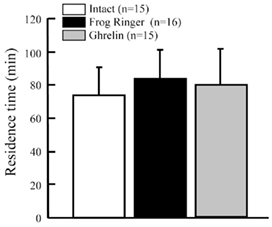
Figure 10. Effect of intracerebroventricular injection of rat ghrelin on residence time in water of Japanese tree frogs. Values are the means + SEM. Statistical analysis (non-repeated ANOVA followed by Bonferroni/Dunn test) was conducted, but significant difference was not detected.
Discussion
This is the first report to have identified ghrelin receptor in amphibian. cDNAs from two species of frogs that encoded functional GHS-Ra protein as demonstrated by functional assay were isolated.
Sequence alignment and phylogenetic analyses of ghrelin receptor proteins including GHS-Ra-like receptor (GHSRa-LR) showed that the two frog proteins were grouped to the same branch with clawed frog, X. laevis. However, the branch was found to belong to the sub-clade that also included the fish GHS-R2a as well as GHS-R1a of birds and mammals. GHS-R2a is a relatively newly ghrelin receptor that was recently found in some teleost fish such as zebrafish, channel catfish and goldfish, and the sequence identities between GHS-R1a and 2a are 67–74% (Kaiya et al., 2008, 2010; Small et al., 2009). It is considered to be a paralog generated by the second-round whole genome duplication (2R WGD) or fish-specific 3R WGD (Meyer and Van de Peer, 2005). Therefore, fish GHS-R1a has created another sub-group from GHS-R1a for birds and mammals. Furthermore, GHSRa-LR is considered to be another sub-group. Receptors that are not functionally confirmed by signal transduction study with ghrelin or GHSs, or that needs a high dose of ghrelin or GHSs to induce intracellular Ca2+ signaling but are sharing high sequence identity with GHS-Ra (Palyha et al., 2000; Chen and Cheng, 2004; Kaiya et al., 2009a,b) are regarded as GHSRa-LR. When the amino acid sequence of fish GHS-Ra are compared to human GHS-R1a, fish GHS-R2a shows higher identity than fish GHS-R1a [catfish-2a (65.7%) > catfish-1a (62.1%); goldfish-1a-1 (68.2%) > goldfish-2a-1 (66.8%); goldfish 1a-2 (68.1%) > goldfish 2a-2 (65.6%); zebrafish-1a (68.1%) > zebrafish-2a (67.4%)]. If the identity toward human GHS-R1a takes precedence over in terms of naming of GHS-R, it should call (re-name) present fish GHS-R2a as GHS-R1a. Based on these facts, we designate our identified receptor GHS-R1a for frogs.
Expressed frog GHS-R1a proteins were found to be functional, which were able to bind bullfrog and rat ghrelin, and two GHS-R1a agonists, and induced intracellular Ca2+ signaling in transfected HEK293 cells. There are two types of acyl modification in bullfrog ghrelin: octanoylation (C8) and decanoylation (C10; Kaiya et al., 2001). The present result showed that the two modified peptides have equipotency toward bullfrog GHS-R1a, indicating that the two peptides can bind the receptor with the same affinity to the receptor without lipid selectivity. On the other hand, the two GHS-R1a agonists that were equipotent in stimulating calcium signalling, exhibited 1/10 less potency when compared to that of bullfrog ghrelin 28-C8. In goldfish (gf) ghrelin receptor (gfGHS-Ra), a similar interaction to ghrelin or GHSs has been reported between gfGHSR2a-1 and gfGHS-R1a-1: gfGHSR2a-1 shows high affinity to all ligands examined such as goldfish ghrelin, GHRP-6 and hexarelin, but gfGHS-R1a-1 has low affinity to hexarelin. One reason would be due to the specific agonistic structure to human (mammalian) GHS-R1a in the evolutionary process. Therefore, low affinity to GHS-R1a agonists observed in bullfrog GHS-R1a could be due to the substitutions of key amino acid in positions that are important for determining the affinity to GHS-R1a agonists (Feighner et al., 1998). In frog receptors, Val131 for human GHS-R1a was substituted with Ile; which could be responsible for the low affinity. Recently, Ueda et al. (2010) reported several important amino acids in the human GHS-R1a structure for ghrelin or GHRP-6-inducing intracellular Ca2+ signaling and ghrelin binding, which include Cys116, Cys198 and Glu187, and they are conserved in both frog receptors.
Ligand selectivity between bullfrog and tree frog GHS-R1a was not observed as expected. We speculated that Thr3-ghrelin (bullfrog ghrelin) could show much higher affinity than Ser3-ghrelin (rat ghrelin) in bullfrog GHS-R1a, and inversely Ser3-ghelin could activate tree frog GHS-R1a more potently than Thr3-ghrelin. The reason may be related to the fact that identified GHS-R1a mRNA has not been detected (expressed) in the pituitary of both frogs. It was very strange because a previous in vitro experiment showed that bullfrog ghrelin has more potent GH- and PRL-releasing activities than rat ghrelin in bullfrog pituitary cells (Kaiya et al., 2001), indicating the direct effect of ghrelin to the pituitary, and it strongly suggests the presence of ghrelin receptor that selectively binds bullfrog ghrelin (Thr3-ghrelin) in the bullfrog pituitary. Ghrelin receptor mRNA has mainly been detected in the pituitary of almost all animals examined so far (Gnanapavan et al., 2002; Geelissen et al., 2003; Tanaka et al., 2003; Chen and Cheng, 2004; Kaiya et al., 2009a,b; Small et al., 2009). The present result suggests that our identified ghrelin receptor is the brain (hypothalamic) type ghrelin receptor, and it is possible that another (hypophyseal) type of ghrelin receptor is present, which preferentially binds Thr3-ghrelin in the pituitary of both frogs. However, despite several attempts, we were not able to identify the hypophyseal type until now. Further study would be required for the demonstration of the presence of a hypophyseal ghrelin receptor in frogs.
Growth hormone secretagogue-receptor 1a mRNA was detected in various tissues of each frog, and was mainly expressed in the brain, gastrointestinal tract, and kidney. Although its mRNA distribution is highly similar to the pattern detected in other animals, its involvement in physiological events have to be further elucidated. In Japanese quail and chickens, GHS-R1a mRNA are expressed in the gastrointestinal tract, with the expression level differs in each region of the intestine (Kitazawa et al., 2009). In goldfish, tissue distribution of ghrelin receptor mRNA is different depending on the receptor type; GHS-R1a is mainly expressed in the brain and intestines, whereas GHS-R2a is not present in the intestine (Kaiya et al., 2010). GHS-R1a mRNA expression in frog tissues directly indicates that these are ghrelin’s effectors, and it suggests the possible involvement of ghrelin in regulation of brain functions (Olszewski et al., 2008; Briggs and Andrews, 2011), gut motility (Peeters, 2005; Kitazawa et al., 2007, 2009) and renal function (Mori et al., 2000).
Specific GHS-R1a mRNA expression of frog was found in testis of bullfrog, ovary, heart and liver of tree frog. Recently, Izzo et al. (2011) reported testicular expression of ghrelin mRNA in a frog, P. esculentus. They showed localization of ghrelin mRNA in the cytoplasm of the interstitial Leydig cells and in the germinal compartment of Sertoli cells. In addition, the mRNA expression varies during reproductive cycle, and 17β-estradiol affects testicular ghrelin mRNA expression. These results suggest an involvement of the ghrelin system in frog reproduction. Rak et al. (2009) have suggested functional roles of ghrelin in the porcine ovarian follicles such as estradiol secretion, aromatase activity and cell proliferation. Sun et al. (2010) reported that ghrelin contributes to positive inotropic effect on cardiomyocytes. Recently, hepatoprotective effect of ghrelin has been suggested (Golestan Jahromi et al., 2010; Moreno et al., 2010). Further study is needed to clarify physiological actions of ghrelin in frogs. Recently, presence of ghrelin and GHS-R1a proteins have been demonstrated in the pancreas of X. laevis (Suzuki and Yamamoto, 2011). We could not confirm mRNA expression of GHS-R1a in the pancreas of bullfrog and tree frog in this study. Further detailed examination is necessary by taking the presence of another receptor type from identified GHS-R1a into consideration.
In bullfrog, GHS-R1a mRNA expression in the stomach increased 10 days after fasting. Similarly, in tree frog, a 10-days food deprivation led to increase in GHS-R1a mRNA expression in the stomach. Similar changes in plasma ghrelin levels, gastric ghrelin content and gastric ghrelin mRNA have been observed in our previous study using bullfrog (Kaiya et al., 2006). This may be because that stomach is the primary source for ghrelin production, the gastric GHS-R1a could first detect the ghrelin change. Taking together with the results from the current study, we hypothesized that this could be the metabolic regulation of the ghrelin system in bullfrog. A similar change observed in tree frog may suggest a physiological involvement of the ghrelin system during negative energy balance. In goldfish, GHS-R1a and 2a mRNA expressions do not change in the intestine for 7 days of food deprivation although intestine is the primary source for ghrelin production (Kaiya et al., 2010).
Growth hormone secretagogue-receptor 1a mRNA expression did not change in the brain of both frogs. In fact, little is known about expression changes in GHS-R1a mRNA in the brain of other animals. In rats, receptor density had not changed by nutritional state in the hypothalamus despite of relevant alterations in plasma ghrelin level observed (Harrold et al., 2008). Riley et al. (2008) reported no change in GHSR1a-LR mRNA expression in tilapia brain for 7 days fasting. On the other hand, in rats, GHS-R1a mRNA expression in arcuate nuclei of the hypothalamus increased 48 h after fasting (Nogueiras et al., 2004). Furthermore, Riley et al. (2008) have showed later that a high level of expression of GHSR1a-LR mRNA in whole brain has been observed in short-term 3-h before meal, although the increase is not evident 1–2 h before meal (Peddu et al., 2009). In goldfish, 7 days of fasting decreased gfGHS-R1a-1 in the vagal lobe, whereas GHS-R1a-2 did not change. On the other hand, no changes were observed for both receptor mRNA expressions in the olfactory lobe (Kaiya et al., 2010). These results indicate that regulation of mRNA expression in goldfish brain during food deprivation is region-specific. In the present study, we used whole brain for gene expression analysis. More detail analyses including region-specific or time-dependent regulation may be required for detecting changes in receptor mRNA expression.
In tree frog, dehydration treatment led to increase in GHS-R1a mRNA expression in the brain, stomach and ventral skin. Little is known about the effect of the ghrelin system on dehydration. Recently, it becomes evident that ghrelin inhibits water intake in rats, chickens and seawater-acclimated eels (Kozaka et al., 2003; Tachibana et al., 2006; Hashimoto et al., 2007; Hashimoto and Ueta, 2011; Nobata and Takei, 2011). In tree frog, ICV injection of AVT or angiotensin II (Ang II) extended the residence time in water dish, indicating increased water absorption from the ventral skin (Maejima et al., 2008, 2010). These results are supported by the presence of receptor for those hormones in the tree frog brain. Results from the present study show that GHS-R1a mRNA expression increased in the brain after dehydration, suggesting a possible involvement of ghrelin on water absorption in tree frog. However, ICV injection of ghrelin did not affect the residence time although the injected dose (25 pmol/g BW) was considered to be sufficient. As ghrelin is a peripheral, circulating gastric hormone rather than a neuroendocrine hormone, further study is required to examine the effect of peripherally injected ghrelin on water absorption.
In summary, we determined nucleotide sequence of ghrelin receptor (GHS-R1a) cDNA in the two species of anuran amphibians. The two GHS-R1a are capable of binding ghrelin and inducing intracellular Ca2+ signaling, but expected ligand selectivity between Ser3-ghrelin and Thr3-ghrelin was not observed. Since the identified GHS-R1a mRNA was not detected in the pituitary, it is possible that another type of ghrelin receptor could be found in the pituitary. In frogs, GHS-R1a mRNA expression was up-regulated by fasting or dehydration, suggesting that ghrelin is involved in the regulation of appetite and osmoregulation. Further studies are required for understanding the physiological relevance of the ghrelin system in amphibian.
Conflict of Interest Statement
The authors declare that the research was conducted in the absence of any commercial or financial relationships that could be construed as a potential conflict of interest.
Acknowledgments
We thank Mrs. Azumi Ooyama for excellent technical assistance, and Ms. Janice Tam for critical reading of this manuscript. This work was supported in part by a Grant-in-Aid for Scientific Research from MEXT of Japan to Hiroyuki Kaiya, Kenji Kangawa, and Mikiya Miyazato, and by the Takeda Scientific Foundation of Japan to Kenji Kangawa.
References
Briggs, D. I., and Andrews, Z. B. (2011). Metabolic status regulates ghrelin function on energy homeostasis. Neuroendocrinology 93, 48–57.
Chen, C. B., and Cheng, C. H. (2004). Identification and functional characterization of two alternatively spliced growth hormone secretagogue receptor transcripts from the pituitary of black seabream, Acanthopagrus schlegeli. Mol. Cell. Endocrinol. 214, 81–95.
Davenport, A. P., Bonner, T. I., Foord, S. M., Harmar, A. J., Neubig, R. R., Pin, J. P., Spedding, M., Kojima, M., and Kangawa, K. (2005). International Union of Pharmacology LVI. Ghrelin receptor nomenclature, distribution, and function. Pharmacol. Rev. 57, 541–46.
Feighner, S. D., Howard, A. D., Prendergast, K., Palyha, O. C., Hreniuk, D. L., Nargund, R., Underwood, D., Tata, J. R., Dean, D. C., Tan, C. P., McKee, K. K., Woods, J. W., Patchett, A. A., Smith, R. G., and Van der Ploeg, L. H. (1998). Structural requirements for the activation of the human growth hormone secretagogue receptor by peptide and nonpeptide secretagogues. Mol. Endocrinol. 12, 137–145.
Geelissen, S., Beck, I. M., Darras, V. M., Kühn, E., and van der Geyten, S. (2003). Distribution and regulation of chicken growth hormone secretagogue receptor isoforms. Gen. Comp. Endocrinol. 134, 167–174.
Gnanapavan, S., Kola, B., Bustin, S. A., Morris, D. G., McGee, P., Fairclough, P., Bhattacharya, S., Carpenter, R., Grossman, A. B., and Korbonits, M. (2002). The tissue distribution of the mRNA of ghrelin and subtypes of its receptor, GHS-R, in humans. J. Clin. Endocrinol. Metab. 87, 2988–2991.
Golestan Jahromi, M., Nabavizadeh, F., Vahedian, J., Nahrevanian, H., Dehpour, A. R., and Zare-Mehrjardi, A. (2010). Protective effect of ghrelin on acetaminophen-induced liver injury in rat. Peptides 31, 2114–2117.
Harrold, J. A., Dovey, T., Cai, X. J., Halford, J. C., and Pinkney, J. (2008). Autoradiographic analysis of ghrelin receptors in the rat hypothalamus. Brain Res. 1196, 59–64.
Hashimoto, H., Fujihara, H., Kawasaki, M., Saito, T., Shibata, M., Otsubo, H., Takei, Y., and Ueta, Y. (2007). Centrally and peripherally administered ghrelin potently inhibits water intake in rats. Endocrinology 148, 1638–1647.
Hashimoto, H., Otsubo, H., Fujihara, H., Suzuki, H., Ohbuchi, T., Yokoyama, T., Takei, Y., and Ueta, Y. (2010). Centrally administered ghrelin potently inhibits water intake induced by angiotensin II and hypovolemia in rats. J. Physiol. Sci. 60, 19–25.
Hashimoto, H., and Ueta, Y. (2011). Central effects of ghrelin, a unique peptide, on appetite and fluid/water drinking behavior. Curr. Protein Pept. Sci. 12, 280–287.
Izzo, G., Ferrara, D., Napolitano, F., Crispo, A. A., d’Istria, M., Aniello, F., and Minucci, S. (2011). Identification of a cDNA encoding for Ghrelin in the testis of the frog Pelophylax esculentus and its involvement in spermatogenesis. Comp. Biochem. Physiol. A 158, 367–373.
Kaiya, H., Darras, V. M., and Kangawa, K. (2007). Ghrelin in birds; its structure, distribution and function. J. Poult. Sci. 44, 1–18.
Kaiya, H., Hasunuma, I., Ttsutsui, K., Kangawa, K., and Miyazato, M. (2010). “Only frog ghrelin of the genus, Rana, has threonine residue at the N-terminal position 3,” in Proceedings of the Fifth International Peptide Symposium, eds N. Fujimoto, and Y. Kiso (Kyoto: Peptide Science 2010), 74.
Kaiya, H., Kojima, M., Hosoda, H., Koda, A., Yamamoto, K., Kitajima, Y., Matsumoto, M., Minamitake, Y., Kikuyama, S., and Kangawa, K. (2001). Bullfrog ghrelin is modified by n-octanoic acid at its third threonine residue. J. Biol. Chem. 276, 40441–40448.
Kaiya, H., Miyazato, M., Kangawa, K., Peter, R. E., and Unniappan, S. (2008). Ghrelin: a multifunctional hormone in non-mammalian vertebrates. Comp. Biochem. Physiol. A 149, 109–128.
Kaiya, H., Riley, L. G., Janzen, W., Hirano, T., Grau, E. G., Miyazato, M., and Kangawa, K. (2009a). Identification and genomic sequence of a ghrelin receptor (GHS-R)-like receptor in the Mozambique tilapia, Oreochromis mossambicus. Zool. Sci. 26, 330–337.
Kaiya, H., Mori, T., Miyazato, M., and Kangawa, K. (2009b). Ghrelin receptor (GHS-R)-like receptor and its genomic organisation in rainbow trout, Oncorhynchus mykiss. Comp. Biochem. Physiol. A 153, 438–450.
Kaiya, H., Sakata, I., Yamamoto, K., Koda, A., Sakai, T., Kangawa, K., and Kikuyama, S. (2006). Identification of immunoreactive plasma and stomach ghrelin, and expression of stomach ghrelin mRNA in the bullfrog, Rana catesbeiana. Gen. Comp. Endocrinol. 148, 236–244.
Kitazawa, T., Kaiya, H., and Taneike, T. (2007). Contractile effects of ghrelin-related peptides on the chicken gastrointestinal tract in vitro. Peptides 28, 617–624.
Kitazawa, T., Maeda, Y., and Kaiya, H. (2009). Molecular cloning of growth hormone secretagogue-receptor and effect of quail ghrelin on gastrointestinal motility in Japanese quail. Regul. Pept. 158, 132–142.
Kohno, S., Kamishima, Y., and Iguchi, T. (2003). Molecular cloning of an anuran V(2) type [Arg(8)] vasotocin receptor and mesotocin receptor: functional characterization and tissue expression in the Japanese tree frog (Hyla japonica). Gen. Comp. Endocrinol. 132, 485–498.
Kojima, M., Hosoda, H., Date, Y., Nakazato, M., Matsuo, H., and Kangawa, K. (1999). Ghrelin is a growth-hormone-releasing acylated peptide from stomach. Nature 402, 656–660.
Kozaka, T., Fujii, Y., and Ando, M. (2003). Central effects of various ligands on drinking behavior in eels acclimated to seawater. J. Exp. Biol. 206, 687–692.
Maejima, S., Konno, N., Matsuda, K., and Uchiyama, M. (2010). Central angiotensin II stimulates cutaneous water intake behavior via an angiotensin II type-1 receptor pathway in the Japanese tree frog Hyla japonica. Horm. Behav. 58, 457–464.
Maejima, S., Yamada, T., Hamada, T., Matsuda, K., and Uchiyama, M. (2008). Effects of hypertonic stimuli and arginine vasotocin (AVT) on water absorption response in Japanese tree frog, Hyla japonica. Gen. Comp. Endocrinol. 157, 196–202.
Meyer, A., and Van de Peer, Y. (2005). From 2R to 3R: evidence for a fish-specific genome duplication (FSGD). Bioessays 27, 937–945.
Moreno, M., Chaves, J. F., Sancho-Bru, P., Ramalho, F., Ramalho, L. N., Mansego, M. L., Ivorra, C., Dominguez, M., Conde, L., Millán, C., Marí, M., Colmenero, J., Lozano, J. J., Jares, P., Vidal, J., Forns, X., Arroyo, V., Caballería, J., Ginès, P., and Bataller, R. (2010). Ghrelin attenuates hepatocellular injury and liver fibrogenesis in rodents and influences fibrosis progression in humans. Hepatology 51, 974–985.
Mori, K., Yoshimoto, A., Takaya, K., Hosoda, K., Ariyasu, H., Yahata, K., Mukoyama, M., Sugawara, A., Hosoda, H., Kojima, M., Kangawa, K., and Nakao, K. (2000). Kidney produces a novel acylated peptide, ghrelin. FEBS Lett. 486, 213–216.
Nobata, S., and Takei, Y. (2011). The area postrema in hindbrain is a central player for regulation of drinking behavior in Japanese eels. Am. J. Physiol. Regul. Integr. Comp. Physiol. 300, R1569–R1577.
Nogueiras, R., Tovar, S., Mitchell, S. E., Rayner, D. V., Archer, Z. A., Dieguez, C., and Williams, L. M. (2004). Regulation of growth hormone secretagogue receptor gene expression in the arcuate nuclei of the rat by leptin and ghrelin. Diabetes 53, 2552–2558.
Olszewski, P. K., Schiöth, H. B., and Levine, A. S. (2008). Ghrelin in the CNS: from hunger to a rewarding and memorable meal? Brain Res. Rev. 58, 160–170.
Palyha, O. C., Feighner, S. D., Tan, C. P., McKee, K. K., Hreniuk, D. L., Gao, Y. D., Schleim, K. D., Yang, L., Morriello, G. J., Nargund, R., Patchett, A. A., Howard, A. D., and Smith, R. G. (2000). Ligand activation domain of human orphan growth hormone (GH) secretagogue receptor (GHS-R) conserved from Pufferfish to humans. Mol. Endocrinol. 14, 160–169.
Papotti, M., Ghè, C., Cassoni, P., Catapano, F., Deghenghi, R., Ghigo, E., and Muccioli, G. (2000). Growth hormone secretagogue binding sites in peripheral human tissues. J. Clin. Endocrinol. Metab. 85, 3803–3807.
Peddu, S. C., Breves, J. P., Kaiya, H., Gordon Grau, E., and Riley, L. G. Jr. (2009). Pre- and postprandial effects on ghrelin signalling in the brain and on the GH/IGF-I axis in the Mozambique tilapia (Oreochromis mossambicus). Gen. Comp. Endocrinol. 161, 412–418.
Peeters, T. L. (2005). Ghrelin: a new player in the control of gastrointestinal functions. Gut 54, 1638–1649.
Rak, A., Szczepankiewicz, D., and Gregoraszczuk, E. L. (2009). Expression of ghrelin receptor, GHSR-1a, and its functional role in the porcine ovarian follicles. Growth Horm. IGF Res. 19, 68–76.
Riley, L. G., Fox, B. K., Breves, J. P., Kaiya, H., Dorough, C. P., Hirano, T., and Grau, E. G. (2008). Absence of effects of short-term fasting on plasma ghrelin and brain expression of ghrelin receptors in the tilapia, Oreochromis mossambicus. Zool. Sci. 225, 821–827.
Small, B. C., Quiniou, S. M., and Kaiya, H. (2009). Sequence, genomic organization and expression of two channel catfish, Ictalurus punctatus, ghrelin receptors. Comp. Biochem. Physiol. A 154, 451–464.
Sun, Q., Ma, Y., Zhang, L., Zhao, Y. F., Zang, W. J., and Chen, C. (2010). Effects of GH secretagogues on contractility and Ca2+ homeostasis of isolated adult rat ventricular myocytes. Endocrinology 151, 4446–4454.
Suzuki, H., and Yamamoto, T. (2011). Ghrelin- and growth hormone secretagogue receptor-immunoreactive cells in Xenopus pancreas. Regul. Pept. 169, 64–69.
Tachibana, T., Kaiya, H., Denbow, D. M., Kangawa, K., and Furuse, M. (2006). Central ghrelin acts as an anti-dipsogenic peptide in chicks. Neurosci. Lett. 405, 241–245.
Tanaka, M., Miyazaki, T., Yamamoto, I., Nakai, N., Ohta, Y., Tsushima, N., Wakita, M., and Shimada, K. (2003). Molecular characterization of chicken growth hormone secretagogue receptor gene. Gen. Comp. Endocrinol. 134, 198–202.
Tokuda, C., Kimura, K., and Kamishima, Y. (1995). Angiotensin II suppresses water absorption through the ventral skin of Japanese tree-frogs in vitro. Zool. Sci. 12, 203–206.
Ueda, T., Matsuura, B., Miyake, T., Furukawa, S., Abe, M., Hiasa, Y., and Onji, M. (2010). Mutational analysis of predicted extracellular domains of human growth hormone secretagogue receptor 1a. Regul. Pept. 166, 28–35.
Keywords: bullfrog, characterization, cloning, ghrelin, growth hormone secretagogue-receptor 1a, Japanese tree frog
Citation: Kaiya H, Koizumi Y, Konno N, Yamamoto K, Uchiyama M, Kangawa K and Miyazato M (2011) Ghrelin receptor in two species of anuran amphibian, bullfrog (Rana catesbeiana), and Japanese tree frog (Hyla japonica). Front. Endocrin. 2:31. doi: 10.3389/fendo.2011.00031
Received: 08 July 2011; Accepted: 26 August 2011;
Published online: 26 September 2011.
Edited by:
Gustavo M. Somoza, Instituto de Investigaciones Biotecnologicas-Instituto Tecnologico de Chascomus, ArgentinaReviewed by:
Suraj Unniappan, York University, CanadaFabian Canosa, Instituto de Investigaciones Biotecnologicas-Instituto Tecnologico de Chascomus, Argentina
Copyright: © 2011 Kaiya, Koizumi, Konno, Yamamoto, Uchiyama, Kangawa and Miyazato. This is an open-access article subject to a non-exclusive license between the authors and Frontiers Media SA, which permits use, distribution and reproduction in other forums, provided the original authors and source are credited and other Frontiers conditions are complied with.
*Correspondence: Hiroyuki Kaiya, Department of Biochemistry, National Cerebral and Cardiovascular Center Research Institute, 5-7-1 Fujishirodai, Suita, Osaka 565-8565, Japan. e-mail:a2FpeWFAcmkubmN2Yy5nby5qcA==