- Department of Biochemistry, School of Medicine, Fujita Health University, Toyoake, Aichi, Japan
Aromatase in the mouse brain is expressed only in the nerve cells of specific brain regions with a transient peak during the neonatal period when sexual behaviors become organized. The aromatase-knockout (ArKO) mouse, generated to shed light on the physiological functions of estrogen in the brain, exhibited various abnormal behaviors, concomitant with undetectable estrogen and increased androgen in the blood. To further elucidate the effects of neurosteroidal estrogens on behavioral phenotypes, we first prepared an brain-specific aromatase transgenic (bsArTG) mouse by introduction of a human aromatase transgene controlled under a −6.5 kb upstream region of the brain-specific promoter of the mouse aromatase gene into fertilized mouse eggs, because the −6.5 kb promoter region was previously shown to contain the minimal essential element responsible for brain-specific spatiotemporal expression. Then, an ArKO mouse expressing the human aromatase only in the brain was generated by crossing the bsArTG mouse with the ArKO mouse. The resulting mice (ArKO/bsArTG mice) nearly recovered from abnormal sexual, aggressive, and locomotive (exploratory) behaviors, in spite of having almost the same serum levels of estrogen and androgen as the adult ArKO mouse. These results suggest that estrogens locally synthesized in the specific neurons of the perinatal mouse brain directly act on the neurons and play crucial roles in the organization of neuronal networks participating in the control of sexual, aggressive, and locomotive (exploratory) behaviors.
Introduction
Aromatase (the cyp19 gene product) is a key enzyme in the biosynthesis of estrogen from androgen. It is localized not only in the gonads but also in the brains of vertebrates from fish to mammals and plays important roles in the brain sexual differentiation and in the sexual behaviors through paracrine or autocrine actions of estrogen as a neurosteroid. Aromatase in the mouse brain is expressed only in the nerve cells of specific brain regions including the hypothalamus and limbic areas with a transient peak during the neonatal period when neuronal networks and imprinting of sexual behaviors are irreversibly organized (Balthazart et al., 1991; Harada and Yamada, 1992; Lauber and Lichtensteiger, 1994).
An essential direct-acting factor in the processes of sexual differentiation of the brain was formerly considered to be testosterone (T) secreted from the testes during the perinatal period (Beach, 1946; Pfaff, 1970). However, dihydrotestosterone (DHT), a biologically active androgen, was unable to restore impaired male sexual behaviors in castrated rats (McDonald et al., 1970). Moreover, some antiestrogens and aromatase inhibitors blocked androgen-induced sexual behaviors in male rats (Christensen and Clements, 1975; Beyer et al., 1976). Then, Naftolin et al. (1972) proposed an aromatase hypothesis, in which brain aromatase would be responsible for the organization of the neural circuitry underlying male sexual behaviors by conversion of T from fetal testes to 17β-estradiol (E2) in the brain (MacLusky and Naftolin, 1981).
Experimental model animals exhibiting estrogen or androgen deficiency were generated in mice by targeted disruption of estrogen receptors (ERs), androgen receptor (AR), and aromatase genes and the roles of neurosteroids were studied in reproductive physiology and behavior. A series of studies on the knockout mice of ERα (αERKO), ERβ (βERKO), or both of ERα and β (αβERKO) has revealed that ERs play essential roles in male sexual behaviors as well as aggressive and parental behaviors (Ogawa et al., 1997, 1999, 2000; Wersinger et al., 1997). On the other hand, conditional ArKO mice generated by a Cre-lox system also exhibited impaired sexual and aggressive behaviors in males and normal sexual behaviors in females, suggesting that androgen/AR are required for perinatal brain masculinization (Sato et al., 2004). Aromatase-knockout (ArKO) mice generated by several groups (Fisher et al., 1998; Honda et al., 1998; Toda et al., 2001c) had impaired reproductive functions, glucose/lipid metabolisms, and socio-emotional or sexual behaviors.
Aromatase-knockout mice showed accumulation of androgen as well as a deficiency of estrogen in the blood. Consequently, accumulated T is converted to DHT, a ligand of AR, by 5α-reductase. DHT is further metabolized to 5α-androstan-3β, 17β-diol by 3β-hydroxysteroid dehydrogenase (3β-HSD) and 17β-hydroxysteroid dehydrogenase (17β-HSD). Accumulated T is also converted to dehydroepiandrosterone (DHEA) and 5α-androsten-3β, 17β-diol by 3β-HSD, and 17β-HSD. Recently, 5α-androsten-3β, 17β-diol, and 5α-androstan-3β, 17β-diol were shown to be good ligands for ERα and ERβ, and to exert physiological functions in the brain (Lund et al., 2006; Jellinck et al., 2007). The metabolic side effects accompanied by the aromatase gene disruption and consequent genomic effects through activation of nuclear receptors by binding of androgen metabolites should be considered in the interpretation of the aberrant phenotypes of ArKO mice.
We have already identified the promoter region responsible for the brain-specific spatiotemporal expression of the mouse aromatase gene in a previous study (Harada and Honda, 2005). In this study, we generated an ArKO mouse expressing the human aromatase gene only in the brain [ArKO/ brain-specific aromatase transgenic (bsArTG) mouse] by introduction of the aromatase transgene controlled under a brain-specific promoter. We compared the behavioral phenotypes with those of the ArKO mouse as an approach to elucidate the effects of estrogen deficiency separately from the effects of androgen metabolites.
Materials and Methods
Animals
Aromatase-knockout mice were generated by pairing heterozygous mutant animals which were generated by targeted disruption of the aromatase gene (Honda et al., 1998). The crossings were repeated over 20 generations to generate ArKO offspring with a genetic background of C57BL/6. The transgenic mice brain-specifically expressing human aromatase (bsArTG mice) were generated by microinjection of the aromatase transgene controlled under the brain-specific promoter of the mouse aromatase gene into fertilized eggs (Gorski et al., 1986; Harada and Honda, 2005). Genomic DNA was prepared from the tails of the offspring, and screened by polymerase chain reaction (PCR) to select ArKO and bsArTG mice. The ArKO/bsArTG mouse was prepared by pairing the ArKO with bsArTG mouse. Mice were singly housed under a controlled photoperiod with a 12:12-h light–dark cycle and temperature (22–24°C) throughout the tests with food and water available ad libitum. Animal care and experiments were conducted in accordance with Fujita Health University guidelines.
Behavioral Analyses
Behavioral analyses of sexual behaviors (mount, intromission, and ejaculation), aggressive behaviors (tail rattling, chasing, boxing, biting, offensive attach, wrestling), infanticide behavior, and locomotive (exploratory) behavior were performed as described previously (Matsumoto et al., 2003b). All behavioral tests were conducted during the dark phase of the light/dark cycle starting at 2 h after lights were switched off and video-recorded with infrared night scope for 15–30 min each. Male mice were tested for sexual behaviors during a 30-min test with estrous female mice (C57BL/6). As stimuli, the female mice were ovariectomized and injected with E2 benzoate (30 and 15 μg at 48 and 24 h before tests) and progesterone (500 μg at 4–7 h before tests). Males were also tested in their home cage against a group-housed olfactory-bulbectomized male intruder mouse (C57BL/6) for 15 min. For each male tested, the total number of aggressive bouts was scored. An aggressive bout was defined as a consecutive series of behavioral acts separated by less than 3 s. The locomotive (exploratory) behavior was video-recorded in the open field cage (53 cm long × 35 cm wide × 30 cm high) with an infrared night scope, and analyzed with travel distance, resting time, and parallelism index as parameters using behavior analysis software SMART (Panlab, S. L., Barcelona, Spain).
Construction of Transgenes
The DdeI and StuI fragment of the human aromatase cDNA, which contains a 5′-non-coding region of 19 bp, a complete coding region, and 3′-non-coding region of 303 bp, with a SV40 polyadenylation signal at the 3′-end was inserted into pUC118. Then, the −6.5 kb promoter region of the brain-specific exon 1f of the mouse aromatase gene (Harada and Honda, 2005) was prepared from genomic DNA and inserted into the plasmid to construct the bsArTG as shown in Figure 1. The transgene plasmid was linearized by NotI digestion, and used to generate the bsArTG mouse as described previously (Hogan et al., 1994). Four to six transgenic founders were obtained and maintained to generate progeny. Genomic DNA was prepared from the tails of the offspring and screened for the presence of the transgene by PCR (Honda et al., 1998).
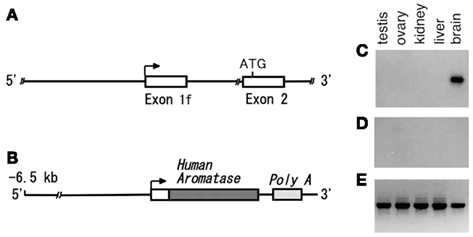
Figure 1. Construction of the brain-specific aromatase transgene. (A) The amino acid sequence of mouse aromatase is coded from the ATG translational start site in the exon 2 to termination codon in exon 10 of the gene. The exon 1f, flanked with brain-specific regulatory elements in the promoter region, is brain-specifically selected among multiple exons 1 of the gene. (B) The brain-specific aromatase transgene was constructed by linking human aromatase cDNA with the −6.5-kb promoter region of the brain-specific exon 1f. (C–E) Tissue total RNAs were prepared from testes, ovaries, kidneys, livers, and brains of ArTG mice (8–10 weeks of age) carrying the brain-specific aromatase transgene and subjected to RT–PCR specific for human aromatase in the presence (C) or absence (D) of reverse transcriptase. As controls, RT–PCRs specific for β-actin (E) were also performed.
Analysis of Expression of the Aromatase Transgene by Reverse Transcription-Polymerase Chain Reaction
The expression of the aromatase transgene in the ArTG or ArKO/bsArTG mice were examined by RT–PCR of the total RNAs which were prepared from tissue homogenates of ArTG or ArKO/bsArTG mice. The oligonucleotides of an antisense primer (5′-AACCACGATAGCACTTTGT-3′) for reverse transcription (RT) and nested antisense- (5′-TGTTAGAGGTGTCCAGCATG-3′) and sense- (5′-TACTACAACCGGGTATATGG-3′) primers for PCR were synthesized. All were specific primers for the human aromatase transgene, but not for the mouse aromatase gene. The RT–PCR was performed as described previously (Honda et al., 1998).
Assay of Serum Levels of E2 and T
The serum levels of E2 and T were determined by radio immunoassay (RIA; Mitsubishi Kagaku Bio-Clinical Laboratories, Inc., Tokyo) and enzyme immunoassay kits (Pantex, Santa Monica, CA, USA). After anesthetization, 3–4 animals of each genotype at 12–15 weeks of age were used to collect the blood by cardiac puncture. The serum samples were pooled and used for assays of serum steroids, which were performed in triplicate for each genotype.
Statistical Analysis
The frequency (mean ± SEM) of mount, intromission, and ejaculation behaviors was analyzed by a two-way analysis of variance (ANOVA) with repeated measures followed by post hoc comparisons using the Fisher’s protected least significant difference (Fisher’s PLSD) test. Differences in the incidences of behaviors were analyzed by the χ2 test or Fisher’s exact probability test. We considered differences significant, if p-values < 0.05.
Results
Generation and Characterization of the ArKO/bsArTG Mouse
The aromatase transgene expressing the human aromatase transcriptionally regulated by the −6.5-kb promoter region of the brain-specific exon 1f of the mouse aromatase gene (Figure 1) was constructed and introduced into fertilized eggs to generate the bsArTG mouse. The ArKO/bsArTG mouse was prepared by pairing of an ArKO with a bsArTG mouse. The resulting ArKO/bsArTG mice exhibited the brain-specific expression of the aromatase transgene (Figure 1). The expression was undetectable in the testes, ovary, kidney, and liver of ArKO/bsArTG mice. ArKO/bsArTG mice showed almost the same serum levels of E2 and T as the ArKO mice (Table 1). The serum levels of E2 in wild females were changed from <5 to 30 pg/ml according to the estrous cycle in the ovary, whereas those of ArKO and ArKO/bsArTG females were below the detection limit (<5 pg/ml). The levels of T were 7.98 ng/ml in wild-type males and 0.21 ng/ml in wild-type females and were significantly increased in ArKO and ArKO/bsArTG females. There were no significant differences in T levels between ArKO and ArKO/bsArTG mice (Table 1).
Restoration of Impaired Behaviors in ArKO Mice by Introduction of bsArTG
Aromatase-knockout mice exhibited impairments of various behaviors, such as sexual behaviors of mount, intromission, and ejaculation, aggressive behaviors of tail rattling, chasing, boxing, biting, offensive attack, and wrestling, infanticide behavior, and locomotive (exploratory) behaviors as shown in Figures 2–7. Wild-type males normally displayed frequent mount and intromission, and ejaculation behaviors. In contrast, the frequency of mount and intromission behaviors in the ArKO males were observed at rates of 9 and 3% of the wild-type males’, respectively, and no ejaculation was observed in the ArKO males (Figure 2). However, the ArKO/bsArTG males almost completely recovered from the impaired ejaculation behavior observed in the ArKO males, whereas they significantly but incompletely recovered from the impaired behaviors of mount and intromission in the ArKO males, compared with the same behaviors in the wild-type males. ArKO/bsArTG males significantly recovered from disorders in the sexual behaviors of mount, intromission, and ejaculation (Figure 2). Similarly, the ArKO males showed significantly reduced aggressive behaviors against an olfactory-bulbectomized male intruder and simultaneously elevated aggression toward an estrous female, while wild-type males attack a male intruder and accept an estrous female (Figure 3). The ArKO/bsArTG males completely lacked the aggressive behavior to females in the same cage, whereas they showed partial recovery of the decreased aggressiveness to the intruder males but not complete recovery, compared with the wild-type males (Figure 3). Frequent infanticide behaviors were observed in ArKO males, whereas wild-type males did not show any infanticide (Figure 4). The infanticide behavior observed in the ArKO males almost completely disappeared in the ArKO/bsArTG males as in the wild-type males (Figure 4).
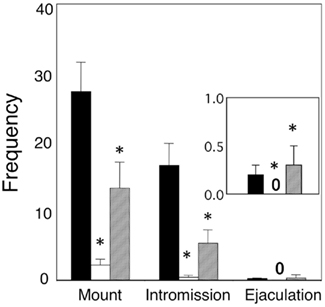
Figure 2. Impairment and restoration of male sexual behaviors in ArKO and ArKO/bsArTG mice, respectively. The behavioral tests were performed using wild-type (n = 9), ArKO (n = 11), and ArKO/bsArTG (n = 9) male mice aged 11–13 weeks. The frequency of mount, intromission, and ejaculation during a 30-min test was examined in the wild-type (solid bars), ArKO (open bars), and ArKO/bsArTG (hatched bars) mice. An insert is a enlarged graph of the ejaculation frequency. The frequency of each behavior is indicated by the mean ± SEM. Significant differences of wild-type vs. ArKO (impairments) and ArKO vs. ArKO/bsArTG (restorations) are shown (*p < 0.01).
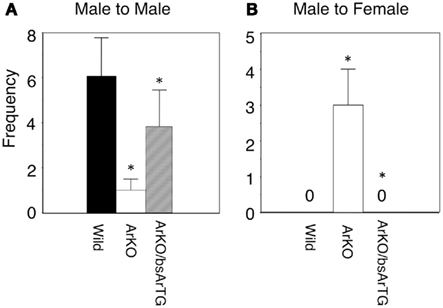
Figure 3. The resident intruder paradigm test of aggressive behaviors in wild-type (n = 9), ArKO n = 11), and ArKO/bsArTG (n = 9) male mice aged 12–14 weeks. The test male was examined in his home cage as the resident against a group-housed olfactory-bulbectomized male intruder (A) or an estrous female (B). The frequency of each aggressive behavior is indicated by the mean ± SEM. Significant differences of wild-type vs. ArKO and ArKO vs. ArKO/bsArTG are shown (*p < 0.01).
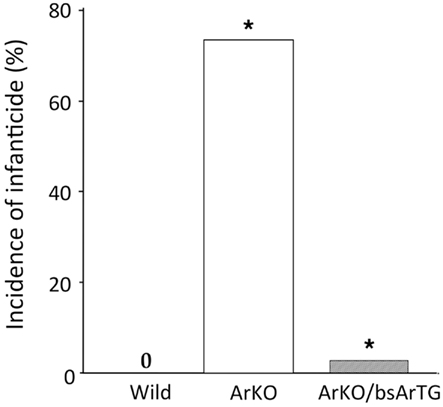
Figure 4. Infanticide behaviors in wild-type (n = 9), ArKO (n = 11), and ArKO/bsArTG (n = 9) mice aged 13–15 weeks. The incidence of the infanticide behavior is shown as a percentage, and statistically analyzed by the χ2-method. Significant differences of wild-type vs. ArKO and ArKO vs. ArKO/bsArTG are shown (*p < 0.05).
The locomotive (exploratory) behavior was observed in the open field with regard to parameters of travel distance, resting time, and parallelism index. We analyzed video-monitored locomotive (exploratory) behaviors of wild-type, ArKO, and ArKO/bsArTG mice using behavioral analysis software, SMART (Panlab). The spontaneous locomotive behavior of ArKO/bsArTG mice was compared to that of wild-type and ArKO mice. We observed that ArKO mice walked a few steps and looked around, whereas wild-type and ArKO/bsArTG mice moved more linearly. Time-series data of ArKO and wild-type mice at 200-ms intervals revealed that wild-type mice moved around constantly whereas ArKO mice move intermittently with some resting time, occasionally with resting time of more than 1 min (Figure 5). Consequently, the shorter travel distance and the longer resting time were observed in ArKO females, compared with wild-type and ArKO/bsArTG females, whereas there were no significant changes of the travel distance and the resting time among wild-type, ArKO, and ArKO/bsArTG males (Figure 6). The parallelism index, another parameter of locomotor activity, indicated characteristic features of movement in the open field of these animals (Figure 7). This parallelism index was significantly lower in ArKO mice, compared to the wild-type and ArKO/bsArTG mice (Figure 7).
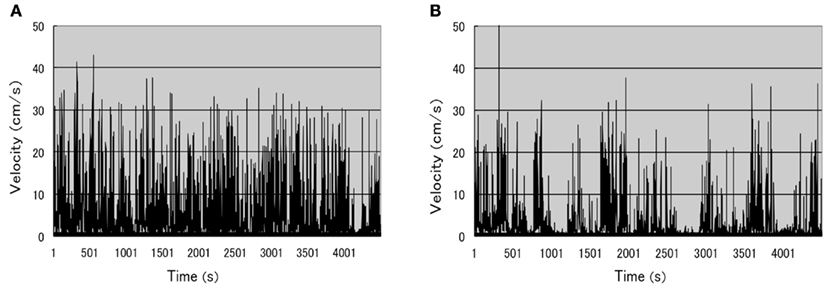
Figure 5. Typical locomotive activities of the wild-type and ArKO mice aged 15 weeks in open field tests. The locomotive behaviors of wild-type and ArKO mice were monitored in the open field cage (53 cm long × 35 cm wide × 30 cm high) by a video-recorder with infrared night scope. Time-series data of wild-type (A) and ArKO (B) mice were collected at 200 ms intervals for 15 min.
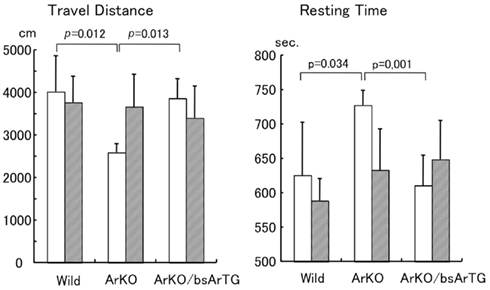
Figure 6. Impairment and restoration of locomotive activities of wild-type, ArKO, and ArKO/bsArTG mice. The travel distance and resting time during a 15-min open field test were analyzed from video-recorded locomotor activities of males (hatched bars) and females (open bars) of wild-type (n = 9), ArKO (n = 11), and ArKO/bsArTG (n = 9) mice aged 14–16 weeks.
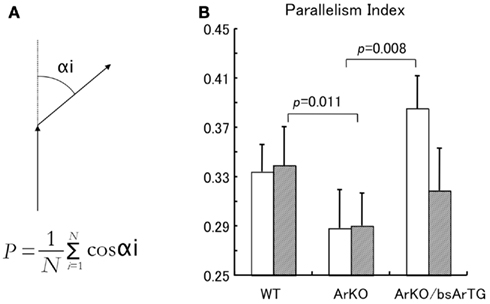
Figure 7. The parallelism index of wild-type, ArKO, and ArKO/bsArTG mice as a parameter of locomotor activity. The parallelism index, a characteristic feature of locomotor or exploratory movement, was calculated from video-recorded locomotor activities of males (hatched bars) and females (open bars) of wild-type (n = 9), ArKO (n = 11), and ArKO/bsArTG (n = 9) mice aged 14–16 weeks using behavioral analysis software SMART (B). The index value elicited from the formula shown in the (A) approaches +1 when the movement is straight ahead, and approaches 0 and −1 when the test mouse moves rightward/leftward, and backward, respectively.
Discussion
Aromatase is tissue-specifically regulated by alternative utilization of multiple exons 1 of the gene (Means et al., 1991; Harada et al., 1993; Mahendroo et al., 1993). In the brain, aromatase is brain-specifically transcribed from exon 1f (Honda et al., 1994, 1996). The promoter region of exon 1f is thought to be responsible for the brain (preoptic area, medial hypothalamus, and amygdala regions)-specific, the neuron-specific, and the developmental stage-specific expression in the brain (Harada and Honda, 2005). Such a spatiotemporal expression of aromatase in the brain is thought to be in line with the need for organization and activation of sexual behavior through local production of estrogens in specific regions of the brain. In a previous study, the −6.5-kb promoter region of exon 1f exhibited a similar pattern of spatiotemporal expression as a LacZ reporter transgene in the transgenic mouse and as expression of aromatase in the mouse brain (Harada and Honda, 2005). All transgenic mice carrying the transgene with the –6.5 kb promoter region showed significant β-galactosidase reporter activity, mainly in the limbic cortex containing the amygdala and in the diencephalon containing the preoptic area and medial hypothalamus, similar to the localization of aromatase in the mouse brain. There was almost no activity in other tissues (Harada and Honda, 2005). Furthermore, the LacZ transgene in all the transgenic mice showed a very similar developmental expression pattern as that of mouse brain aromatase with a transient peak during the perinatal period in the diencephalic and limbic regions (Harada and Honda, 2005).
To elucidate the physiological functions of aromatase, we generated ArKO mice by targeted disruption of the mouse aromatase gene (Honda et al., 1998). The ArKO mice show a complete deficit of estrogens and exhibit various abnormalities in the gonads, bones, blood vessels, brain, etc. With regard to physiological functions in the brain, the ArKO mice exhibited abnormal behaviors, such as a propensity for infanticide, inversion of aggressive behaviors, and impairments in sexual motivation, partner preference, and performance behaviors and parental and exploratory behaviors (Bakker et al., 2002a,b; Aste et al., 2003; Matsumoto et al., 2003a,b). The observation on the sexual behaviors is consistent with phenotypes of αβERKO mice in principle (Ogawa et al., 2000). The αERKO males showed normal levels of mounts and reduced levels of intromissions, but virtually no ejaculation (Ogawa et al., 1997; Wersinger et al., 1997), whereas all three components of sexual behaviors are normal in βERKO animals (Ogawa et al., 1999). The αβ ERKO males did not exhibit any sexual behaviors. Immunoreactive ERs were also reported to increase approximately twofold in certain hypothalamic and limbic regions of ArKO mice (Agarwal et al., 2000). The facts that estrogen deficiency in the ArKO mouse resulted in apoptosis of neurons in certain brain regions (Hill et al., 2007a, 2009) may suggest important roles for estrogens in the formation of neuronal networks.
To date, ArKO mice have been generated in different laboratories, and independently observed to exhibit similar disorders in sexual partner preference (Bakker et al., 2002b), sexual performance, aggressive behavior (Toda et al., 2001a,b), and compulsive behavior (Hill et al., 2007b). The impairment in mounting behavior of ArKO males was reversed by repeated injection of E2 when initiated on the day of birth, but not when treatment was initiated on the 15th-day after birth (Toda et al., 2001a), whereas Bakker et al. (2004) have reported that the deficits in sexual behaviors of ArKO males were largely corrected following adult treatment with E2 benzoate. ArKO males also showed significantly abnormal aggressive behaviors, compared with wild-type males (Matsumoto et al., 2003a,b). Supplements of E2 soon after birth reportedly restore a complete loss of male aggressive behavior (Toda et al., 2001b).
The aromatase gene with a brain-specific promoter was introduced into the ArKO mice. Thus, aromatase in the ArKO/bsArTG mice was expressed only in the brain with a transient peak during the perinatal period, but not in other tissues including gonadal tissues (Figure 1). The ArKO/bsArTG mice showed significant recovery from impaired behaviors, suggesting that aromatase in the brain played an important role in the organization effect through local estrogen production. However, several behaviors such as mount, intromission, and aggression were incompletely recovered by the transgenic aromatase introduced in the ArKO/bsArTG mice. The facts suggest that the activation effect of these behaviors in adulthood would be exerted by high levels of estrogen from both of the brain and gonadal tissues and estrogen only from the brain in the ArKO/bsArTG mice is not enough to bring about the same effect. Alternatively, redundant or paratactic innervation systems by androgen or the other factors may also function in the organization and activation effects. The additional experiments using conditional knockout of ArKO and ArKO/bs ArTG mice and E2 implantation might provide a clear answer.
Several lines of evidence indicate that androgens directly induce male-typical aggressive behaviors (Svare and Gandelman, 1975; Simon et al., 1985). Recent experiments using the Cre-loxP system to generate ArKO male and female mice showed that ArKO males exhibited no male-typical sexual and aggressive behaviors, whereas ArKO females exhibited normal sexual behaviors (Sato et al., 2004). Moreover, administration of non-aromatized androgen, DHT to ArKO males was ineffective in restoring impaired sexual behaviors, but it was partially effective in restoring impaired aggressive behaviors, whereas DHT rescued impaired male-typical behaviors in ERKO mice (Sato et al., 2004).
Androgen metabolites such as 5-androsten-3β, 17β-diol, and 5-androtan-3β, 17β-diol were also characterized as ligands of ER in the brains (Lund et al., 2006; Jellinck et al., 2007). 5-Androsten-3β, 17β-diol, a steroid with both of estrogenic and androgenic properties, has previously been shown to promote not only human prostate cancer cells (Miyamoto et al., 1998) but also estrogen-dependent breast cancer cells (Poulin and Labrie, 1986) and to act as a ligand with a high affinity for ER (Poulin and Labrie, 1986). It has been found recently that it can also be metabolized from DHEA by microglia (Jellinck et al., 2007). Neurons in the hypothalamus significantly expressed ERβ, AR, and DHT, and its metabolite, 5-androstan-3β, 17β-diol functions in the stress-response by acting through ERβ-expressing neurons (Lund et al., 2006). The expression of all steroid-metabolizing enzymes of 5α-reductase, 17β-HSD, 3α-HSD, 3β-HSD, and aromatase in the hypothalamus was demonstrated by RT–PCR (Lund et al., 2006). The conversion of DHEA and DHT to 5-androsten-3β, 17β-diol and 5-androtan-3β, 17β-diol, respectively, would be readily catalyzed by 3β-HSD and 17β-HSD in the hypothalamus. These metabolites are non-aromatized steroids derived from androgens and therefore would also be produced in the brain of ArKO mice with elevated serum levels of androgens, which were observed in all cases of independently generated ArKO mice. To make clear whether these androgen metabolites actually act as functional steroids in the brain, the expression of steroid-metabolizing enzymes must be confirmed to elucidate metabolic profiles and local concentrations of neurosteroids in the specific brain regions of wild-type, ArKO, and ArKO/bsArTG mice. In this study, the brain-specific expression of aromatase in the ArKO/bsArTG mice was verified (Figure 1). Considering a post-translational modification of aromatase such as phosphorylation (Balthazart et al., 2005) and autophagy degradation (Zhang et al., 2010) and an estrogenic action of androgen metabolites (Poulin and Labrie, 1986; Miyamoto et al., 1998), it will also be necessary to further determine the catalytic aromatase activity and the local estrogen concentration in the specific brain regions of wild-type, ArKO, and ArKO/bsArTG mice.
So far, there have been reports of aromatase/E2/ER-mediated, 5α-reducatase/DHT/AR-mediated, and androgen metabolites/ER-mediated innervation of areas implicated in the control of sexual behaviors. Although this study provided evidence in support of aromatase/E2/ER-mediated innervation of some behaviors, the other behaviors were partially impaired in the ArKO mice and partially recovered in the ArKO/bsArTG mice. The introduction of ArTG into ArKO males led to complete recovery of the abnormal ejaculation, aggressiveness to the intruder females, and infanticide in the ArKO males, and only partial recovery of abnormal mount and intromission behaviors in the ArKO males. These facts may suggest the presence of redundant or paratactic innervation system which could bypass or compensate for the aromatase/E2/ER-mediated innervation.
We observed the undetectable serum levels of E2 and the increased serum levels of T in the ArKO mice. These facts cast doubt on the side effects resulting from elevated androgens with respect to the abnormal behaviors observed in the ArKO mice because androgens were reported to induce male-typical aggressive behaviors (Svare and Gandelman, 1975; Simon et al., 1985) and sexual behaviors (Sato et al., 2004). Thus, we designed recovery experiments for behavioral disorders in ArKO mouse by introduction of the aromatase transgene, regulated by the −6.5-kb brain-specific promoter of the exon 1f, into the ArKO mouse. Because the resulting ArKO/bsArTG mice express aromatase only in the brains with a transient peak during the perinatal period, adult ArKO/bsArTG mice showed almost the same serum levels of E2 and T as adult ArKO mice. However, ArKO/bsArTG mice nearly recovered from impairments of sexual behaviors including mounting, intromission, and ejaculation behaviors observed in the ArKO mice. Similarly, both the aggressive behavior and the infanticide behavior observed in ArKO males were normalized in the ArKO/bsArTG males. ArKO/bsArTG mice also showed good recovery of locomotor (exploratory) activities measured by travel distance, resting time, and the parallelism index. The results obtained in this study support the supposition that estrogens produced in localized brain areas during the perinatal period may directly act on the neurons and be essential for organization of various behaviors in the brain, and that aromatase may play an important role in the biological processes underlying brain functions. To evaluate the accurate physiological function of 5-androsten-3β, 17β-diol, 5-androtan-3β, 17β-diol, and the other androgen metabolites in the brain, we would need to establish the generation of steroid 17α, 20-lyase deficient mouse maintaining steroid 17α-hydroxylase activity as reported as a case of the human genetic disease (Zachmann et al., 1982), and to analyze behavioral disorders of steroid 17α, 20-lyase knockout mice and restoration of the impairments by administration of estrogens, androgens, and their metabolites.
Conflict of Interest Statement
The authors declare that the research was conducted in the absence of any commercial or financial relationships that could be construed as a potential conflict of interest.
Acknowledgments
This work was supported in part by Grants-in-Aid for Scientific Research from the Ministry of Education, Science, Sports, and Culture of Japan; Grants-in-Aid for Research from Fujita Health University; Medical Science Promotion Fund (the 24th General Assembly of the Japanese Association of Medical Sciences). The research was conducted in the absence of any commercial or financial relationship that could be construed as a potential conflict of interest.
References
Agarwal, V. R., Sinton, C. M., Liang, C., Fisher, C., German, D. C., and Simpson, E. R. (2000). Upregulation of estrogen receptors in the forebrain of aromatase knockout (ArKO) mice. Mol. Cell. Endocrinol. 162, 9–16.
Aste, N., Honda, S., and Harada, N. (2003). Forebrain Fos responses to reproductively related chemosensory cue in aromatase knockout mice. Brain Res. Bull. 60, 191–200.
Bakker, J., Baillien, M., Honda, S., Harada, N., and Balthazart, J. (2004). Relationships between aromatase activity in the brain and gonads and behavioural deficits in homozygous and heterozygous aromatase knockout mice. J. Neuroendocrinol. 16, 483–490.
Bakker, J., Honda, S., Harada, N., and Balthazart, J. (2002a). The aromatase knock-out mouse provides new evidence that estradiol is required during development in the female for the expression of sociosexual behaviors in adulthood. J. Neurosci. 22, 9104–9112.
Bakker, J., Honda, S., Harada, N., and Balthazart, J. (2002b). Sexual partner preference requires a functional aromatase (cyp19) gene in male mice. Horm. Behav. 42, 158.
Balthazart, J., Baillien, M., and Ball, G. F. (2005). Interactions between kinases and phosphatases in the rapid control of brain aromatase. J. Neuroendocrinol. 17, 553–559.
Balthazart, J., Foidart, A., Surlemont, C., and Harada, N. (1991). Distribution of aromatase-immunoreactive cells in the mouse forebrain. Cell Tissue Res. 263, 71–79.
Beach, F. A. (1946). A review of physiological and psychological studies of the sexual behavior in mammals. Physiol. Rev. 27, 240–306.
Beyer, C., Morali, G., Naftolin, F., Larsson, K., and Perez-Palacios, G. (1976). Effects of some antiestrogens and aromatase inhibitors on androgen induced sexual behavior in castrated male rats. Horm. Behav. 7, 353–363.
Christensen, L. W., and Clements, L. G. (1975). Blockage of testosterone-induced mounting behavior in the male rat with intracranial application of the aromatase inhibitor androst-1,3,6-triene-3, 17-dione. Endocrinology 97, 1545–1551.
Fisher, C. R., Graves, K. H., Parlow, A. F., and Simpson, E. R. (1998). Characterization of mice deficient in aromatase (ArKO) because of targeted disruption of the cyp19 gene. Proc. Natl. Acad. Sci. U.S.A. 95, 6965–6970.
Gorski, K., Carneiro, M., and Schibler, U. (1986). Tissue-specific in vitro transcription from the mouse albumin promoter. Cell 47, 767–776.
Harada, N., and Honda, S. (2005). Analysis of spatiotemporal regulation of aromatase in the brain using transgenic mice. J. Steroid Biochem. Mol. Biol. 95, 49–55.
Harada, N., Utsumi, T., and Takagi, Y. (1993). Tissue-specific expression of the human aromatase cytochrome P-450 gene by alternative use of multiple exons 1 and promoters, and switching of tissue-specific exons 1 in carcinogenesis. Proc. Natl. Acad. Sci. U.S.A. 90, 11312–11316.
Harada, N., and Yamada, K. (1992). Ontogeny of aromatase messenger ribonucleic acid in mouse brain: fluorometric quantitation by polymerase chain reaction. Endocrinology 131, 2306–2312.
Hill, R. A., Chow, J., Fritzemeier, K., Simpson, E. R., and Boon, W. C. (2007a). Fas/FasL-mediated apoptosis in the arcuate nucleus and medial preoptic area of male ArKO mice is ameliorated by selective estrogen receptor alpha and estrogen receptor beta agonist treatment, respectively. Mol. Cell. Neurosci. 36, 146–157.
Hill, R. A., McInnes, K. J., Gong, E. C., Jones, M. E., Simpson, E. R., and Boon, W. C. (2007b). Estrogen deficient male mice develop compulsive behavior. Biol. Psychiatry 61, 359–366.
Hill, R. A., Chua, H. K., Jones, M. E., Simpson, E. R., and Boon, W. C. (2009). Estrogen deficiency results in apoptosis in the frontal cortex of adult female aromatase knockout mice. Mol. Cell. Neurosci. 41, 1–7.
Hogan, B., Beddington, R., Constantini, F., and Lacy, E. (1994). Manipulating the Mouse Embryo: A Laboratory Manual. New York: Cold Spring Harbor Laboratory.
Honda, S., Harada, N., Ito, S., Takagi, Y., and Maeda, S. (1998). Disruption of sexual behavior in male aromatase-deficient mice lacking exons 1 and 2 of the cyp19 gene. Biochem. Biophys. Res. Commun. 252, 445–449.
Honda, S., Harada, N., and Takagi, Y. (1994). Novel exon 1 of the aromatase gene specific for aromatase transcripts in human brain. Biochem. Biophys. Res. Commun. 198, 1153–1160.
Honda, S., Harada, N., and Takagi, Y. (1996). The alternative exons 1 of the mouse aromatase cytochrome P-450 gene. Biochim. Biophys. Acta 1305, 145–150.
Jellinck, P. H., Kaufmann, M., Gottfried-Blackmore, A., McEwen, B. S., Jones, G., and Bulloch, K. (2007). Selective conversion by microglia of dehydroepiandrosterone to 5-androstenediol-A steroid with inherent estrogenic properties. J. Steroid Biochem. Mol. Biol. 107, 156–162.
Lauber, M. E., and Lichtensteiger, W. (1994). Pre- and postnatal ontogeny of aromatase cytochrome P450 messenger ribonucleic acid expression in the male rat brain studied by in situ hybridization. Endocrinology 135, 1661–1668.
Lund, T. D., Hinds, L. R., and Handa, R. J. (2006). The androgen 5α-dihydrotestosterone and its metabolite 5α-androstan-3β,17/beta-diol inhibit the hypothalamo-pituitary-adrenal response to stress by acting through estrogen receptor β-expressing neurons in the hypothalamus. J. Neurosci. 26, 1448–1456.
MacLusky, N. J., and Naftolin, F. (1981). Sexual differentiation of the central nervous system. Science 211, 1294–1303.
Mahendroo, M. S., Mendelson, C. R., and Simpson, E. R. (1993). Tissue-specific and hormonally controlled alternative promoters regulate aromatase cytochrome P450 gene expression in human adipose tissue. J. Biol. Chem. 268, 19463–19470.
Matsumoto, T., Honda, S., and Harada, N. (2003a). Alteration in sex-specific behaviors in male mice lacking the aromatase gene. Neuroendocrinology 77, 416–424.
Matsumoto, T., Honda, S., and Harada, N. (2003b). Neurological effects of aromatase deficiency in the mouse. J. Steroid Biochem. Mol. Biol. 86, 357–365.
McDonald, P., Beyer, C., Newton, F., Brien, B., Baker, R., Tan, H. S., Sampson, C., Kitching, P., Greenhill, R., and Pritchard, D. (1970). Failure of 5alpha-dihydrotestosterone to initiate sexual behaviour in the castrated male rats. Nature 227, 964–965.
Means, G. D., Kilgore, M. W., Mahendroo, M. S., Mendelson, C. R., and Simpson, E. R. (1991). Tissue-specific promoters regulate aromatase cytochrome P450 gene expression in human ovary and fetal tissues. Mol. Endocrinol. 5, 2005–2013.
Miyamoto, H., Yeh, S., Lardy, H. E. M., and Chang, C. (1998). Δ5-Androstenediol is a natural hormone with androgenic activity in human prostate cancer cells. Proc. Natl. Acad. Sci. U.S.A. 95, 11083–11088.
Naftolin, F., Ryan, K. J., and Petro, Z. (1972). Aromatization of androstenedione by the anterior hypothalamus of adult male and female rats. Endocrinology 90, 295–298.
Ogawa, S., Chan, J., Chester, A. E., Gustafsson, J. A., Korach, K. S., and Pfaff, D. W. (1999). Survival of reproductive behaviors in estrogen receptor beta gene-deficient (betaERKO) male and female mice. Proc. Natl. Acad. Sci. U.S.A. 96, 12887–12892.
Ogawa, S., Chester, A. E., Hewitt, S. C., Walker, V. R., Gustafsson, J. A., Smithies, O., Korach, K. S., and Pfaff, D. W. (2000). Abolition of male sexual behaviors in mice lacking estrogen receptors alpha and beta (alpha beta ERKO). Proc. Natl. Acad. Sci. U.S.A. 97, 14737–14741.
Ogawa, S., Lubahn, D. B., Korach, K. S., and Pfaff, D. W. (1997). Behavioral effects of estrogen receptor gene disruption in male mice. Proc. Natl. Acad. Sci. U.S.A. 94, 1476–1481.
Pfaff, D. (1970). Nature of sex hormone effects on rat sex behavior: specificity of effects and individual patterns of response. J. Comp. Physiol. Psychol. 73, 349–358.
Poulin, R., and Labrie, F. (1986). Stimulation of cell proliferation and estrogenic response by adrenal C19-Δ5-steroids in the ZR-75-1 human breast cancer cell line. Cancer Res. 46, 4933–4937.
Sato, T., Matsumoto, T., Kawano, H., Watanabe, T., Uematsu, Y., Sekine, K., Fukuda, T., Aihara, K., Krust, A., Yamada, T., Nakamichi, Y., Yamamoto, Y., Nakamura, T., Yoshimura, K., Yoshizawa, T., Metzger, D., Chambon, P., and Kato, S. (2004). Brain masculinization requires androgen receptor function. Proc. Natl. Acad. Sci. U.S.A. 101, 1673–1678.
Simon, N. G., Whalen, R. E., and Tate, M. P. (1985). Induction of male-typical aggression by androgens but not by estrogens in adult female mice. Horm. Behav. 19, 204–212.
Svare, B., and Gandelman, R. (1975). Aggressive behavior of juvenile mice: influence of androgen and olfactory stimuli. Dev. Psychobiol. 8, 405–415.
Toda, K., Okada, T., Takeda, K., Akira, S., Saibara, T., Shiraishi, M., Onishi, S., and Shizuta, Y. (2001a). Oestrogen at the neonatal stage is critical for the reproductive ability of male mice as revealed by supplementation with 17beta-oestradiol to aromatase gene (Cyp19) knockout mice. J. Endocrinol. 168, 455–463.
Toda, K., Saibara, T., Okada, T., Onishi, S., and Shizuta, Y. (2001b). A loss of aggressive behaviour and its reinstatement by oestrogen in mice lacking the aromatase gene (Cyp19). J. Endocrinol. 168, 217–220.
Toda, K., Saibara, T., Okada, T., Onishi, S., and Shizuta, Y. (2001c). A loss of aggressive behaviour and its reinstatement by oestrogen in mice lacking the aromatase gene (Cyp19). J. Endocrinol. 168, 217–220.
Wersinger, S. R., Sannen, K., Villalba, C., Lubahn, D. B., Rissman, E. F., and De Vries, G. J. (1997). Masculine sexual behavior is disrupted in male and female mice lacking a functional estrogen receptor alpha gene. Horm. Behav. 32, 176–183.
Zachmann, M., Werder, E. A., and Prader, A. (1982). Two types of male pseudohermaphroditism due to 17, 20-desmolase deficiency. J. Clin. Endocrinol. Metab. 55, 487–490.
Keywords: knockout mouse, transgenic mouse, brain-specific expression, aromatase, estrogen, androgen, sexual behavior
Citation: Honda S-I, Wakatsuki T and Harada N (2011) Behavioral analysis of genetically modified mice indicates essential roles of neurosteroidal estrogen. Front. Endocrin. 2:40. doi: 10.3389/fendo.2011.00040
Received: 01 July 2011;
Paper pending published: 25 July 2011;
Accepted: 07 September 2011;
Published online: 26 September 2011.
Edited by:
Kazuyoshi Tsutsui, Waseda University, JapanReviewed by:
Barney A. Schlinger, University of California at Los Angeles, USASuguru Kawato, University of Tokyo University of Tokyo, Japan
Copyright: © 2011 Honda, Wakatsuki and Harada. This is an open-access article subject to a non-exclusive license between the authors and Frontiers Media SA, which permits use, distribution and reproduction in other forums, provided the original authors and source are credited and other Frontiers conditions are complied with.
*Correspondence: Nobuhiro Harada, Department of Biochemistry, School of Medicine, Fujita Health University, Toyoake, Aichi 470-1192, Japan. e-mail:bmhhcmFkYUBmdWppdGEtaHUuYWMuanA=