- Department of Biology, University of Toronto Mississauga, Mississauga, ON, Canada
We have reinvestigated the possible presence or absence of the pentapeptide proctolin in Rhodnius prolixus and report here the cloning of the proctolin cDNA. The transcript is expressed in the central nervous system (CNS) and some peripheral tissues. The proctolin prepropeptide encodes a single copy of proctolin along with a possible proctolin-precursor-associated peptide. We have biochemically identified proctolin in CNS extracts and shown its distribution using proctolin-like immunoreactivity. Immunostained processes are found on the salivary glands, female and male reproductive tissues, and heart and associated alary muscles. Proctolin-like immunoreactive bipolar neurons are found on the lateral margins of the common oviduct and bursa. Proctolin is biologically active on R. prolixus tissues, stimulating increases in contraction of anterior midgut and hindgut muscles, and increasing heartbeat frequency. Contrary to the previous suggestion that proctolin is absent from R. prolixus, proctolin is indeed present and biologically active in this medically important bug.
Introduction
Proctolin was the first insect neuropeptide to be sequenced and synthesized (Starratt and Brown, 1975) and is found in a variety of arthropods (see Orchard et al., 1989; Nässel, 2002; Lange and Orchard, 2006). Unlike most neuropeptides which exist as members of extended families, proctolin is almost invariably present in the form of the pentapeptide RYLPT, although two alternative forms, AYLPT and RYLMT, along with proctolin, have been reported in Leptinotarsa decemlineata and Daphnia pulex, respectively (Spittaels et al., 1995; Dircksen et al., 2011). Proctolin appears to be unique to arthropods, and there is no convincing evidence for its presence in any other invertebrate or vertebrate. It is present in interneurons, and in motoneurons that project to skeletal and visceral muscle where it stimulates or potentiates muscle contraction (see Lange and Orchard, 2006; Nässel and Winther, 2010). It is also cardioacceleratory, and suggested to be an endocrine releasing factor, and to also act as a neurohormone (see Orchard et al., 1989; Lange, 2002; Clark et al., 2006).
Early attempts to clone the proctolin gene in insects failed, as too did searches of genome databases – even from that of Drosophila melanogaster (see Isaac et al., 2004; Taylor et al., 2004). The genes for proctolin and its receptor were, however, eventually identified and cloned in D. melanogaster (Egerod et al., 2003; Johnson et al., 2003a,b; Taylor et al., 2004), although surprisingly, proctolin has not been chemically identified in D. melanogaster (see Nässel and Winther, 2010). Subsequently, the proctolin gene was found in the genomes of only a few insect species, including Tribolium castaneum, Tenebrio molitor, and Acyrthosiphon pisum (Hauser et al., 2008; Li et al., 2008; Weaver and Audsley, 2008; Huybrechts et al., 2010). No proctolin gene has been identified in genomes of Apis mellifera, Aedes aegypti, Bombyx mori, Nasonia vitripennis, or Acromymrex echinatior and three other ant species (see Roller et al., 2008; Hauser et al., 2010; Predel et al., 2010; Nygaard et al., 2011), where proctolin and its receptor are now considered absent. Thus, proctolin is present in some insects but absent from many others. In a similar fashion, a recent study on neuropeptide precursor genes in Rhodnius prolixus failed to identify a proctolin gene, and it was suggested that R. prolixus lacks proctolin (Ons et al., 2011). This is an interesting and puzzling observation since earlier chemical assays and bioassays provided evidence for proctolin in R. prolixus. Thus, proctolin-like immunoreactive neurons are extensively distributed throughout the central nervous system (CNS) of R. prolixus, extracts of the CNS with proctolin-like bioactivity co-migrate with proctolin on two reversed phase high performance liquid chromatography (RPHPLC) separations, and proctolin itself stimulates contractions of visceral muscles of the reproductive system (Lange et al., 1988; Lange, 1990; Chiang et al., 2010). In addition, proctolin is present in another hemipteran, A. pisum (Huybrechts et al., 2010).
The blood-gorging bug, R. prolixus, is the vector of Chagas’ disease in Central and South America, wherein the protozoan parasite, Trypanosoma cruzi, is transmitted to humans in the insect’s urine following post-feeding diuresis (see Ons et al., 2011). In light of the involvement of the digestive system in the transmission of the parasite, and the important role of proctolin as a stimulator of visceral muscle contraction, we have reinvestigated the possible presence/absence of proctolin in R. prolixus. Here, we have identified the proctolin gene in R. prolixus (Rhopr-proctolin) and cloned its cDNA sequence. The mRNA, which is expressed in the CNS, encodes a proctolin precursor containing a single copy of the proctolin sequence, along with a proctolin-precursor-associated peptide. In addition, we have biochemically identified proctolin in extracts of the CNS by Matrix-assisted laser desorption/ionization (MALDI)-time-of-flight (TOF) mass spectrometry (MS)/MS sequencing. Proctolin is myostimulatory on anterior midgut, hindgut, and heart of R. prolixus, which now join reproductive tissue as a site of biological activity in R. prolixus. This work reinforces the advantages of access to the R. prolixus genome.
Materials and Methods
Insects
Unfed fifth instars or adult R. prolixus Stål 1859 were used throughout this study. The colony was reared at 25°C under 60% humidity, and maintained by feeding on defibrinated rabbit’s blood (Cedarlane Laboratories, Burlington, ON, Canada).
Isolation of the Rhopr-proctolin Sequence from Fifth Instar R. prolixus CNS cDNA Library
The complete proctolin cDNA sequence was isolated using a modified rapid amplification of cDNA ends (RACE) PCR approach as described previously (Lee et al., 2011). Initially, the A. pisum proctolin prepropeptide sequence was used as a query (Gene ID: CYPIG674496; Huybrechts et al., 2010) to perform a BLAST (tBLASTn) search of the R. prolixus preliminary genome assembly with Geneious Software, Version 4.04 (Drummond et al., 2009). The potential proctolin encoding sequence was obtained from the database search, and was used to design the 3′ and 5′RACE gene-specific primers (GSPs; Table 1). Next, 3′ or 5′ RACE GSPs were used in successive PCR reactions with plasmid-specific primers (pDNR_LIB_Rev1 and pPDNR_LIB_Rev2) or plasmid-specific primers (pDNR_LIB For1, pDNR_LIB For2), in order to increase the specificity of the amplified products (Table 1). The PCR products from the first reaction were column-purified (Biobasic, Markham, ON, USA) and used as a template for the second (nested) PCR. The PCR conditions were as follows: 5 min at 95°C, 30 s at 94°C, 30 s at 56°C, 60 s at 72°C, and 10 min at 72°C. The appropriate PCR products from the second reaction were gel extracted (Bio Basic Inc., Markham, ON, Canada) and cloned into a pGEM-T Easy Vector system (Promega, Madison, WI, USA). Positive clones containing the desired inserts were purified, and sequenced at the Applied Genomics Center at the Hospital for Sick Children (MaRS Centre, Toronto, ON, USA).
Sequence Analysis of the Proctolin Prepropeptide
The presence and location of the potential signal peptide cleavage site in the proctolin prepropeptide sequence was examined using SignalP 3.0 (Bendtsen et al., 2004). The location and size of the introns in the proctolin gene were predicted by a BLAST search of the proctolin cDNA sequence against the R. prolixus preliminary genome assembly database using Geneious Software, Version 4.04 (Drummond et al., 2009). After selecting the best hit, the nucleotide sequence was assembled, and the proctolin cDNA sequence compared to the assembled sequence to predict the location and size of exons/introns. The splice site prediction was confirmed using Genie (Reese et al., 1997). Rhopr-proctolin prepropeptide and its homologous sequences (A. pisum, ACYPIG674496; D. melanogaster, NP609158.2; T. castaneum, EFA05689.1; Litopenaeus vannamei, FE183480; D. pulex, EFX88050) were aligned using Clustal W (Larkin et al., 2007). Within the alignment, identical and similar residues were shaded with black and gray, respectively, using the BOXSHADE3.21 server (http://www.ch.embnet.org/software/BOX form.html).
Rhopr-proctolin Expression Analysis with RT-PCR
Tissues were dissected in nuclease-free phosphate-buffered saline (PBS; Sigma-Aldrich, Oakville, ON, Canada) prepared in nuclease-free ddH2O and stored in RNA later solution (Qiagen, Toronto, ON, Canada). Total RNA was isolated from tissues using the Trizol® reagent (Life Technologies Corporation, Carlsbad, CA, USA) according to manufacturer’s protocols and quantified using a NanoDrop UV spectrophotometer (Thermo Scientific, Wilmington, Delaware, USA). An aliquot of 300 ng of total RNA from each tissue was used to synthesize cDNA using iScript™ Select cDNA Synthesis Kit (Bio-Rad, Mississauga, ON, USA). An aliquot of synthesized cDNA was used as a template for the subsequent PCR to amplify a Rhopr-proctolin fragment and the following PCR conditions were used: 5 min at 95°C, 30 s at 94°C, 30 s at 56°C, 60 s at 72°C, and 10 min at 72°C. PCR was performed as described earlier, with R. prolixus proctolin GSPs (Table 1) and a ribosomal protein 49 fragment was amplified (RP49_FOR1, 5′-TGAAACTCAGGAGAAATTGG-3′, RP49_REV1, 5′-GACACACCATGCGCTATC-3′) as a positive control. PCR products were separated on 1% agarose gel containing ethidium bromide. The predicted Rhopr-proctolin PCR product was estimated to be 331 bp in length.
Sample Preparation for Matrix-Assisted Laser Desorption/Ionization Time-of-Flight Mass Spectrometry and MALDI-TOF MS/MS
A sample of 200 CNSs from fifth instar R. prolixus was collected into 500 μl of methanol/acetic acid/water (90:9:1, by volume) and stored at −20°C. Tissues were processed and separated through a C18 column on RPHPLC (using a gradient of 9–60% acetonitrile with 0.1% TFA over 30 min) as previously described (Lee and Lange, 2011; Te Brugge et al., 2011). The fractions eluting with the same retention times (at approximately 30% acetonitrile) as synthetic proctolin (BACHEM Laboratories San Carlos, CA, USA) were dried to approximately 10 μl and then loaded onto a Zip-TipC18 (Millipore Corp, Etobicoke, ON, Canada), which was pre-equilibrated using 50% acetonitrile, to remove salts and other impurities. The samples were analyzed by MALDI-TOF MS (Q Star; Applied Biosystems Inc., Sciex, Concord, ON, Canada) at the Advanced Protein Technology Centre (Hospital for Sick Children, Toronto, ON, USA). One microliter of extract was mixed with 1 μl of matrix solution (dihydroxybenzoic acid in 50% acetonitrile with a concentration of 20 mg/ml) and then spotted on a MALDI plate and allowed to dry. Spectra were recorded in the reflection mode within a mass range from m/z 400 to m/z 3400. The peak m/z 649.358 was subjected to further analysis using tandem MS (MALDI-TOF MS/MS) to verify the R. prolixus proctolin amino acid sequence.
Immunohistochemistry
The dorsal cuticle of the head capsule and the ventral abdominal cuticle of fifth instar and adult R. prolixus were dissected under physiological saline (150 mM NaCl, 8.6 mM KCl, 2 mM CaCl2, 4 mM NaHCO3, 8.5 mM MgCl2, 5 mM HEPES, 34 mM glucose; pH 7.0) exposing the digestive tract, reproductive tissues and CNS. Fixative consisting of 2% paraformaldehyde in a Millonig’s buffer (0.13 M NaH2PO4·H2O, 0.1 M NaOH, 1.2% glucose, 0.3 mM CaCl2; pH 7) was applied for 1 h at room temperature. Following fixation, tissues were washed extensively with PBS (containing 0.9% NaCl, pH 7.2) for 1 h with rinses every 5 min. The gut, reproductive tissues, dorsal vessel, salivary gland, and CNS were removed and then incubated for 1 h at room temperature in 4% Triton X-100 made up in PBS containing 2% bovine serum albumin (BSA) and 10% normal goat serum (NGS). Tissues were then washed with PBS for 1 h with rinses every 10 min and were subsequently incubated on a shaker for 48 h at 4°C in 1:1000 rabbit anti-proctolin IgG fraction purified polyclonal antibody (provided by Hans-Jürgen Agricola, Friedrich-Schiller Universität, Jena, Germany) made up in PBS containing 0.4% Triton X-100, 2% BSA, and 2% NGS. Following incubations, the tissues were then washed in PBS for 2 h with rinses every 10 min, and then incubated for 18 h at 4°C in secondary antibody solution of purified goat anti-rabbit conjugated to Cy3 (diluted 1:600), containing 10% NGS in PBS. Tissues were then extensively washed with PBS for 8 h with rinses every 10 min. Tissues were mounted on microscope slides in 100% glycerol. Proctolin-like immunoreactivity (PLI) was observed with a Zeiss confocal laser microscope (Carl Zeiss, Jena, Germany) and Zen 2009 viewing software. Controls were performed using antiserum that had been pre-absorbed with 1 mM proctolin for 18 h. No staining was observed in these controls.
Phalloidin–TRITC staining
The muscular morphology of the posterior end of the dorsal vessel and associated alary muscles of fifth instar R. prolixus was identified by labeling the polymeric F-actin in the muscle with the phalloidin–tetramethylrhodamine B isothiocyanate conjugate (Sigma-Aldrich, Oakville, ON, Canada). A 1:1000 dilution of phalloidin–TRITC in PBS was utilized, and the staining protocol followed that of Ejaz and Lange (2008). Preparations were viewed on a Nikon Optiphot 2 Epifluorescence Microscope (Nikon Corporation, Tokyo, Japan). Images were taken with a Qimaging Monochrome QICAM 10-bit digital camera (Qimaging, Burnaby, ON, Canada).
Midgut and hindgut assays
Assays were performed on isolated tissues from fifth instar R. prolixus. A fine silk thread was tied at the most anterior end of the midgut and at its most posterior end. The most posterior end was then tied to a minuten pin secured to a well in a Sylgard-coated petri dish containing 200 μl saline, while the anterior thread was attached to a force transducer (Aksjeselskapet Mikro-elektronikk, Horten, Norway). For hindgut contraction assays the most posterior region of the hindgut was dissected with a small piece of ventral cuticle surrounding the anus. The cuticle with attached hindgut was secured to a Sylgard-coated petri dish (in a well containing 200 μl saline), while the anterior end of the hindgut was tied with a fine silk thread to a force transducer. Anterior midgut and hindgut muscle contractions were monitored on a Linear flatbed chart recorder through an amplifier. Proctolin was bath applied by removing half of the saline present in the well containing the tissue, and simultaneously replacing with an equal volume of the test solution at twice the desired, final, concentration.
Heart assays
The ventral abdominal cuticle and visceral tissues were removed from fifth instar R. prolixus, leaving the dorsal vessel exposed. Semi-intact preparations of the abdominal region of the dorsal vessel and surrounding dorsal cuticle were secured in a dish. Heart rate was measure in saline and in various doses of proctolin using electrodes connected to an impedance converter (UFI model 2991, Morro Bay, CA, USA). Electrodes were placed on either side of the heart between the sixth and seventh abdominal segments. Preparations were maintained with 50 μl saline, and test solutions were added by removing all saline, and subsequently adding 50 μl of the desired concentration of peptide.
Results
Rhopr-proctolin Sequence
In silico screening of the R. prolixus trace archive identified a proctolin gene predicted to produce a proctolin prepropeptide containing proctolin and a proctolin-precursor-associated peptide. We first isolated the complete Rhopr-proctolin cDNA sequence (GenBank accession no. JN543225) using a modified RACE PCR approach. The Rhopr-proctolin cDNA sequence is 456 bp long and encodes a 97 amino acid long prepropeptide (Figure 1). Within the open reading frame (ORF), a putative signal peptide sequence was observed with cleavage occurring between amino acid residues 32 (S) and 33 (R). The prepropeptide encodes proctolin (RYLPT) and a proctolin-precursor-associated peptide with possible processing sites at amino acid residues 46, 48, and/or 79/80 (Figure 1). When the Rhopr-proctolin prepropeptide sequence was compared to other insects, A. pisum, T. castaneum and D. melanogaster, and the crustaceans, L. vannamei, and D. pulex, it was found that the most conserved region included the proctolin sequence and sequences immediately following it (Figure 2).
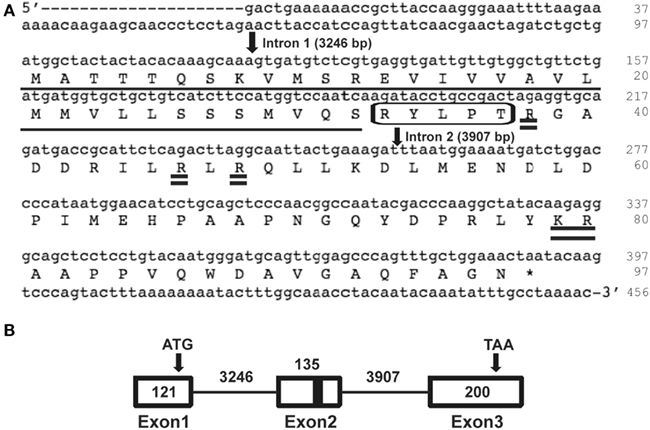
Figure 1. Rhopr-proctolin sequence and its structure. (A) Rhopr-proctolin cDNA sequence (GenBank accession no. JN543225) and the deduced amino acid sequence. Nucleotide and amino acid sequences of the coding region start at the nucleotide sequence ATG, and stop at the sequence TAA (denoted by asterisk). The proctolin coding region is boxed, and the possible post-translational cleavage sites are shown as double underlined. The highly predicted signal peptide required for processing in the secretory pathway is underlined with the predicted cleavage occurring between the amino acids S (32) and R (33). The position and the length of the two introns are indicated by arrows. (B) Schematic diagram representing the genomic organization of the proctolin gene in R. prolixus. Boxes represent exons and solid lines represent introns. Arrows represent the start codon (ATG) and the stop codon (TAA). Black bar represents region encoding proctolin. Numbers indicate the nucleotide length for the corresponding exons and introns.
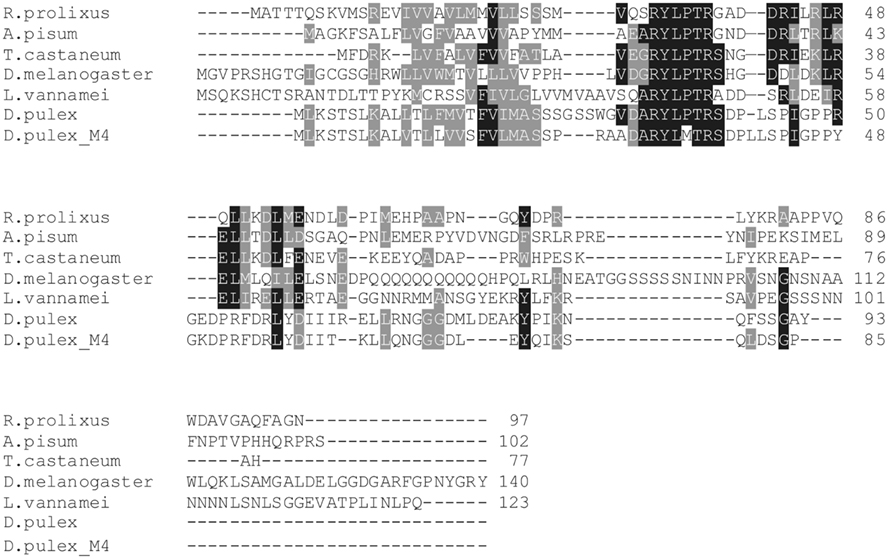
Figure 2. Alignment of known proctolin prepropeptides. Sequences from R. prolixus, A. pisum, (ACYPIG674496), T. castaneum (EFA05689.1), D. melanogaster (NP609158.2), L. vannamei (FE183480), and D. pulex (EFX88050) were aligned with Clustal W. Following a 60% majority rule, identical amino acids are highlighted in black and similar amino acids are highlighted in gray.
The Rhopr-proctolin cDNA sequence was compared with the R. prolixus preliminary genome assembly to identify the location and size of introns in Rhopr-proctolin. Rhopr-proctolin has three exons and their sizes are 121, 135, 200 bp in length (Figure 1B). These exons are separated by two introns which are 3246 and 3907 bp in length. The genomic organization of Rhopr-proctolin is similar to that in D. melanogaster (Figure 1; Taylor et al., 2004) with the Rhopr-proctolin peptide coding region located in exon 2.
Rhopr-proctolin Expression Analysis
Reverse transcription PCR was performed to determine the Rhopr-proctolin tissue expression profile. Total RNA was isolated from the CNS and peripheral tissues of fifth instar R. prolixus as well as the reproductive tissues from adult R. prolixus. Rhopr-proctolin expression was observed in the CNS and in the testes and the pooled tissue that includes the dorsal vessel, trachea, and fat body of fifth instar R. prolixus, but no expression was observed in the other tissues (Figure 3A). Interestingly, Rhopr-proctolin expression was observed in both male and female reproductive tissues of adult R. prolixus (Figure 3B). Samples that lacked the reverse transcription step were negative, as were samples deficient in template RNA.
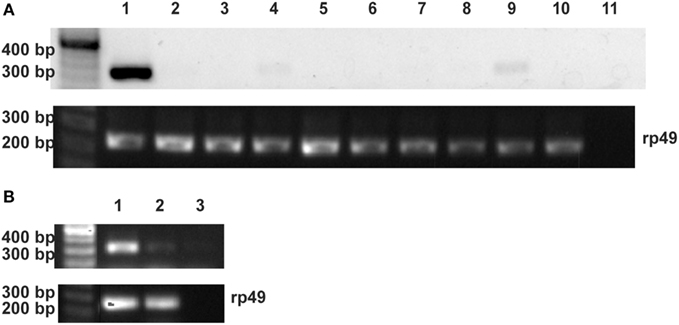
Figure 3. The expression analysis of the Rhopr-proctolin in R. prolixus. (A) Fifth instar tissues: (1) CNS, (2) salivary glands, (3) foregut, (4) dorsal vessel/fat body/trachea, (5) anterior midgut, (6) posterior midgut, (7) Malpighian tubules, (8) hindgut, (9) testis, (10) ovaries, and (11) negative control. (B) Adult reproductive tissues: (1) female, (2) male, and (3) negative control. Primers (Proctolin_Rev0 and Proctolin_For0) were designed to amplify a 331-bp fragment. For the positive control, ribosomal protein (rp) 49 primers were designed to amplify a 240-bp fragment. The negative control reactions were performed without template and position of markers are noted for size comparison.
RPHPLC and MALDI-TOF MS/MS
Aliquots of RPHPLC fractions from CNS that eluted with the same retention times as proctolin were analyzed by MALDI-TOF MS and found to contain an ion mass (m/z) in agreement with the monoisotopic mass ([M + H]+) of proctolin (649.358). This ion mass was further analyzed by MALDI-TOF MS/MS and confirmed the amino acid sequence of RYLPT (Figure 4), and therefore the phenotypic expression of proctolin in the CNS.
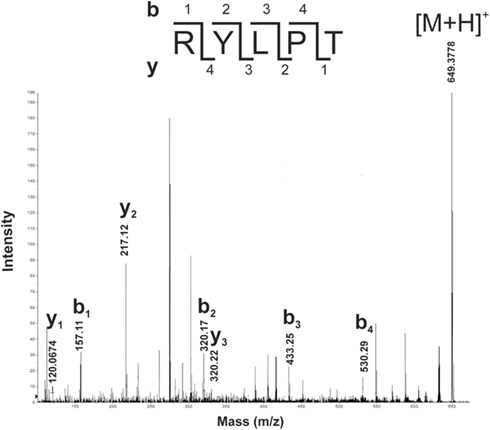
Figure 4. Detection of proctolin by MALDI-TOF MS/MS in fifth instar R. prolixus. The peptide with MH+: 649.3778 from MALDI-TOF MS of a RPHPLC fraction from CNS of R. prolixus fifth instars was selected for fragmentation experiments. Prominent y- and b-type fragments of ion signal 649.3778 were analyzed manually, and the deduced sequence, an exact match to proctolin, is shown. M, mass number; Z, atomic number.
Immunohistochemistry
As reported much earlier (Lange et al., 1988), PLI is present in neurons that are distributed extensively throughout the CNS, and in processes associated with the oviducts of R. prolixus. The general pattern of PLI in the CNS is shown in Figure 5 and confirms that there are extensive clusters of bilateral neurons throughout the CNS, with some axons projecting through the main trunk nerves that project to the hindgut and reproductive system (Figures 5C,D). Examining the digestive and reproductive systems in more detail than previously reported, we find PLI associated with the principal and accessory glands of the salivary glands, and in the nerve projecting to them (Figure 6A). There are few, if any, positively stained processes over the hindgut, and none over the midgut. The female reproductive system is well endowed with PLI, with processes over the lateral and common oviducts and over the spermatheca and bursa (Figures 6B,C). No PLI was found on the ovarioles and ovaries. Interestingly, on the lateral margins of the common oviduct and over the bursa there is a series of bipolar neurons expressing PLI (Figures 6D,E). In male R. prolixus, PLI is found in processes on the seminal vesicles and the vas deferens, but not on the testes (Figure 6F). PLI is found in processes over all of the male accessory glands (Figure 6G).
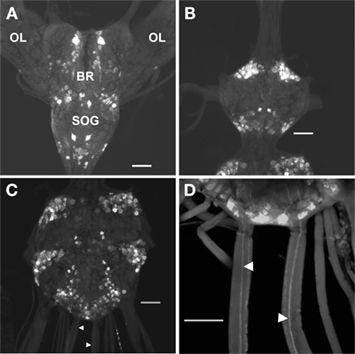
Figure 5. Proctolin-like immunoreactivity is associated with neurons in the CNS of fifth instar R. prolixus. Whole mount preparations show clusters of bilaterally paired proctolin-like immunoreactive neurons in the (A) brain (BR) and subesophageal ganglion (SOG), (B) prothoracic ganglion, and (C,D) mesothoracic ganglionic mass. Axons (arrowheads) projecting through the main trunk nerves of the mesothoracic ganglionic mass (C,D). OL, optic lobe. All scale bars = 100 μm, n = 10.
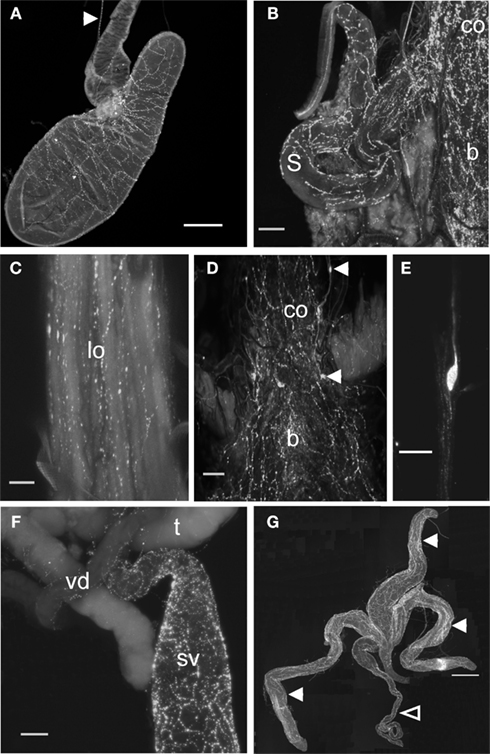
Figure 6. Proctolin-like immunoreactivity is associated with digestive and reproductive tissues of R. prolixus.(A) Proctolin-like immunoreactivity is present (arrowhead) in axons in the nerve projecting to the principal and accessory salivary gland and proctolin immunoreactive processes are associated with the salivary gland of fifth instar R. prolixus. (B–E) Proctolin-like immunoreactive processes are associated with the common oviducts (co), lateral oviducts (lo), bursa (b), and spermatheca (s) in adult female R. prolixus, with a series of immunoreactive bipolar neurons [arrowheads, (D)] localized over the common oviduct and bursa (D,E). (F,G) In adult males, proctolin-like immunoreactive processes are associated with the seminal vesicles (sv) and vas deferens (vd) (F), and over the transparent (closed arrowheads) and opaque accessory glands (open arrowhead) (G). No immunoreactivity was associated with the testis (t). Scale bars: A = 200 μm; B, D, F = 100 μm; C = 50 μm; E = 20 μm; G = 500 μm, n = 10.
Proctolin-like immunoreactivity was also found in processes along the lateral margins of the heart and on the alary muscles (Figure 7). The PLI on the heart is restricted to the most posterior part of the heart and projects anteriorly until the posterior part of the most anterior alary muscles.
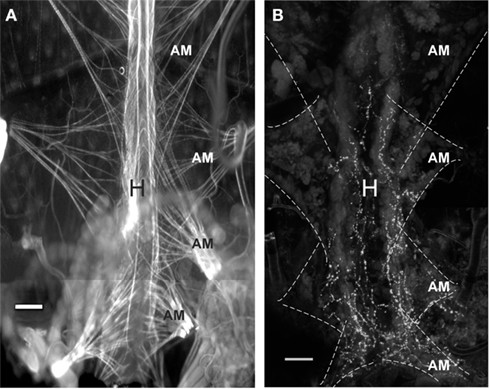
Figure 7. Proctolin-like immunoreactivity is associated with the heart of fifth instar R. prolixus.(A) TRITC-labeled phalloidin staining for F-actin in muscle cells reveals pairs of alary muscles (AM) that anchor to the body wall, providing support and facilitating contractions of the heart (H). (B) Proctolin-like immunoreactive processes are associated with processes on the lateral margin of the heart and over the alary muscles. The alary muscles (AM) are outlined in hatched lines. All scale bars = 100 μm, n = 10.
Bioassays
Proctolin stimulates dose-dependent increases in contractions of anterior midgut, hindgut, and heart (Figures 8–10). The anterior midgut is often spontaneously active and proctolin increases the frequency and amplitude of these contractions, with a threshold close to 10−9 M proctolin, with higher doses also resulting in an increase in basal tonus (Figure 8). The high dose of 10−6 M is less effective, which might indicate desensitization of the receptors. Hindgut is not spontaneously active, but proctolin results in an increase in basal tonus, with threshold approximately 10−9 M and maximum effect at 10−6 M (Figures 9A–C). Proctolin also increases heartbeat frequency, with threshold between 10−10 and 10−9 M and maximum activity at 10−7 M (Figures 10A–C). As the dose of proctolin is increased the frequency increases but amplitude of contractions is reduced due to a more tetanic response, and the inability of the preparation to return to the relaxed position.
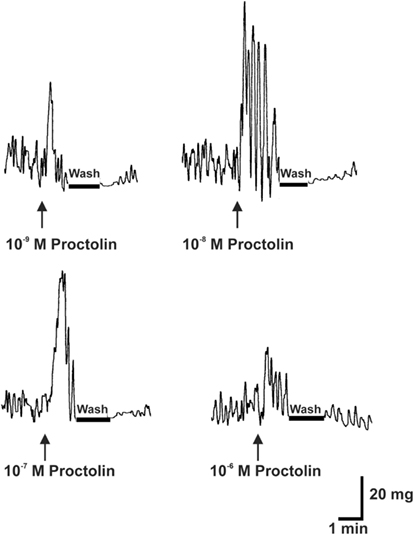
Figure 8. The effects of proctolin on spontaneous contractions of the anterior midgut of fifth instar R. prolixus. The sample trace illustrates that proctolin leads to an increases in the frequency and amplitude of anterior midgut contractions. Upward arrows indicate the addition of peptide. Note that the induced contractions are decreased after washing in saline (bar). Similar results were obtained from seven preparations.
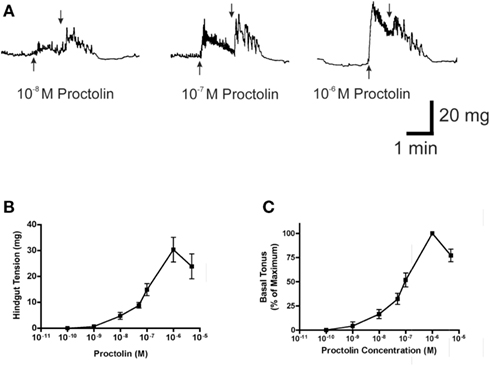
Figure 9. The effect of proctolin on spontaneous contractions of the hindgut of fifth instar R. prolixus. A sample trace (A) illustrates that proctolin leads to an increase in frequency and basal tonus of hindgut contractions. Upward arrows indicate addition of proctolin, downward arrows indicate vigorous, repeated washing with physiological saline. Dose–response curves show the total tension in mg (B) and the percent change in basal tonus of hindgut contractions relative to maximum change (C). Data are mean ± SE, n = 5.
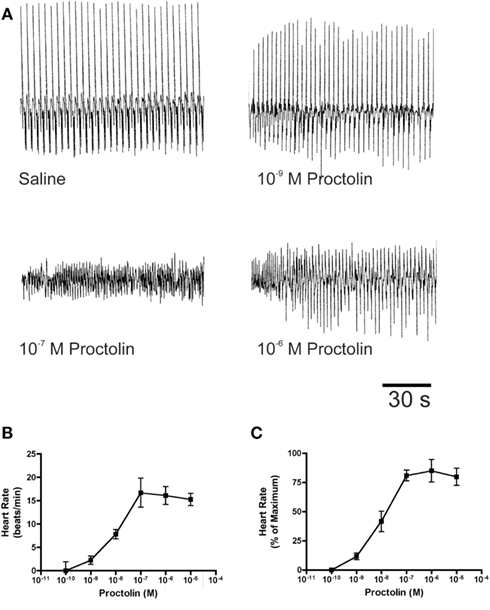
Figure 10. The effects of proctolin on the heart rate of fifth instar R. prolixus. Sample traces (A) illustrate that proctolin increases the frequency of heart contractions. Dose–response curves show that proctolin increases the heart rate in a dose-dependent manner as beats/min (B), and as the percent change in heart rate relative to maximum (C). Data are mean ± SE, n = 6.
Typically in insects, the effects of proctolin on hindgut are very strong, and often stronger than for other peptides; however, in R. prolixus the force of contraction did not appear as strong as we had observed for other peptides. Thus, as a direct comparison, we tested another myotropic peptide, the crustacean cardioactive peptide (CCAP), which is also a native peptide in R. prolixus (Lee and Lange, 2011; Lee et al., 2011). As we suspected, the response to CCAP was significantly greater than that of proctolin (see Figure 11).
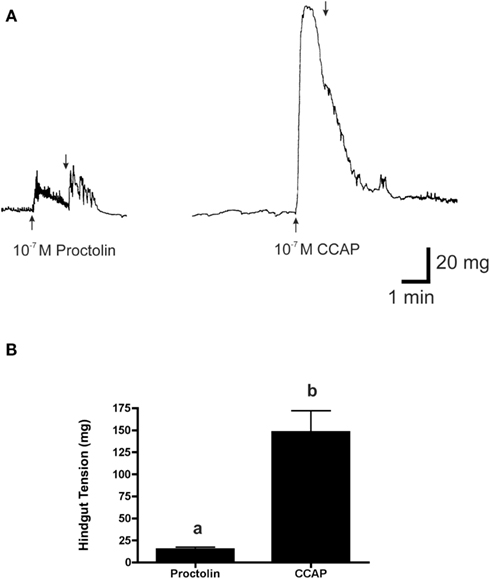
Figure 11. The effects of 10−7 M proctolin and 10−7 M CCAP on the hindgut contraction of fifth instar R. prolixus. (A) Peptides added at upward arrow and vigorously washed with saline at downward arrow. (B) Histogram showing that CCAP is more effective than proctolin at inducing contraction at 10−7 M (p < 0.001, Student’s t-test). Data are mean ± SE, n = 5.
Discussion
The pentapeptide proctolin has some interesting features. It is present in some species of arthropods (including Insecta, Crustacea, and Chelicerata) where it has been identified by MS (although surprisingly not yet in Drosophila species), and may be unique to arthropods since there is no convincing evidence for its presence in other invertebrates or vertebrates. In addition, unlike most biologically active peptides, proctolin is not amidated, and also does not occur as an extended family of peptides; it has an identical amino acid sequence in all arthropods studied, although two alternative sequences have also been reported.
Proctolin is a small peptide, and although it was the first insect neuropeptide to be sequenced, the cloning of the gene was considerably delayed relative to that of other insect neuropeptide families. The gene has now been cloned, but in only a small number of insect species, including D. melanogaster, D. pseudoobscura, T. castaneum, A. pisum, and now R. prolixus (Taylor et al., 2004; Hauser et al., 2008; Weaver and Audsley, 2008; Huybrechts et al., 2010). Recently, the first non-insect arthropod proctolin genes were reported in the Pacific white shrimp, L. vannamei, and the water flea, D. pulex (Ma et al., 2010; Dircksen et al., 2011). Interestingly, the proctolin and receptor genes appear to be absent from the genomes of A. mellifera, A. aegypti, B. mori, N. vitripennis, and A. echinatior plus three other ant species (see Hauser et al., 2008, 2010; Roller et al., 2008; Predel et al., 2010; Nygaard et al., 2011). Thus, proctolin is cataloged under the “variable set” of insect neuropeptide genes, that are found in some insects but absent from others (see Hauser et al., 2010; Nygaard et al., 2011). Presumably for the insects in which proctolin is absent, the nervous system has accommodated to its loss through coopting other neuroactive chemicals. The R. prolixus transcript shows similarities with the other proctolin transcripts, and contains a single copy of the proctolin sequence. The biochemistry of proctolin biosynthesis and processing from its precursor has been described in D. melanogaster (Isaac et al., 2004; Taylor et al., 2004). Cleavage appears to be sequential with the signal peptide cleaved to expose the N-terminal R of proctolin, and a second cleavage to create RYLPTR (an R-extended proctolin intermediate) that would then be converted to proctolin in secretory granules by a carboxypeptidase D. Of note, with regard to the transcript, is a second peptide that might be processed from the prepropeptide. This second peptide immediately follows the cleavage of the RYLPTR sequence in the prepropeptide and appears to be relatively conserved, at least in the insect species. It is unknown if this proctolin-precursor-associated peptide in any of the arthropods is actually processed (including two to three possible cleavage sites), or whether it has any biological relevance, but its relative conservation might indicate some importance.
Expression of the R. prolixus proctolin gene as revealed by RT-PCR is found in the CNS, confirming the immunohistochemical data which reveals large numbers of neurons throughout the CNS. Interestingly, expression was also detected in peripheral tissues, namely testes of fifth instars and reproductive tissues of adults. In addition, pooled samples of dorsal vessel trachea and fat body also revealed some expression. Expression in peripheral tissues is intriguing. However, it is important to realize that peptides (including proctolin) can occur in sensory neurons in arthropods, including peripheral bipolar neurons in this study (Siwicki et al., 1985; Pasztor et al., 1988; Persson and Nässel, 1999). The full extent of the distribution of such neurons is not known, and so it is difficult to state that the expression we see is from the actual peripheral tissues, or from neurons associated with these tissues. However, it is clear that more extensive studies need to be performed in this area.
Proctolin is present in interneurons (suggesting central actions) and in numerous motoneurons that project to skeletal, visceral, and cardiac muscle where it stimulates contractions (see Konopinska and Rosinski, 1999; Nässel, 2002; Lange and Orchard, 2006). Proctolin-like immunoreactive staining reveals the peripheral tissues that might be targets for proctolin. Thus, in R. prolixus, PLI is present in processes associated with the salivary gland, both female and male reproductive organs, and the heart. The salivary glands of R. prolixus are unusual for insects in that they possess a double layer of visceral muscle surrounding the large secretory cavity. The muscles are under polyneuronal control from the salivary nerve and a variety of neuropeptides and serotonin have been shown to stimulate or inhibit contractions (Orchard and Te Brugge, 2002). Clearly, proctolin might also be involved in the neural control of salivary gland muscle contraction, or might participate in the process of salivary secretion.
Proctolin has previously been shown to be associated with insect reproductive tissues, and to participate in activities associated with reproduction. Proctolin influences oviposition digging behavior when applied to abdominal ganglion VIII and alters the rhythmic bursting of centrally generated action potentials recorded from the oviducal nerves in L. migratoria (Kwok and Orchard, 2002; see Lange, 2002); however, the central roles of proctolin in insects are not well understood. Most research on proctolin has concentrated on its peripheral actions. In the oviducts of L. migratoria, proctolin is a co-transmitter, along with a more conventional transmitter (possibly glutamate). Proctolin increases the amplitude of neurally evoked contractions, the amplitude and frequency of myogenic contractions, and stimulates a tonic contraction (see Orchard et al., 1989; Lange, 2002). Proctolin also acts at locust ovipositor muscles, enhancing neuromuscular transmission and muscle contraction (Belanger and Orchard, 1993). Proctolin appears to be a potent modulator of insect reproductive tissues, stimulating contractions of the oviducts of P. americana, L. maderae, L. migratoria and R. prolixus (see Lange, 1990; Lange and Orchard, 2006), and spermatheca in L. migratoria and R. prolixus (see Lange, 1990, 2002). Proctolin also influences egg-laying activity in A. mellifera queens, and promotes the onset of vitellogenesis in Blaberus craniifer (Goudey-Perrière et al., 1994). The extensive distribution of PLI in R. prolixus oviducts, spermatheca, and bursa illustrates proctolin’s likely key role in modulating these female reproductive tissues. Interestingly, a series of bipolar neurons displaying PLI occurs over the lateral margins of the common oviduct and bursa. Peripheral bipolar neurons in insects are typically sensory and so this raises the question of sensory proctolinergic projections to the CNS that might provide feedback on movements associated with these reproductive tissues, or even local release of proctolin in response to stretch. Peripheral proctolin sensory neurons have previously been shown to release proctolin in response to stretch in the oval organs of Crustacea (Pasztor et al., 1988).
Proctolin-like immunoreactive processes are also found over the male reproductive tissues in R. prolixus; namely the seminal vesicles, vas deferens, and all of the accessory glands. The neural control of male reproductive tissue has not been extensively studied in insects (unlike female reproductive tissues), and so these R. prolixus male tissues might prove useful models for future studies.
The lateral margins of the heart and alary muscles possess processes with PLI, and proctolin stimulates increases in heartbeat frequency but no increase in amplitude. Indeed, the amplitude was reduced because the contractions were more tetanic and the heart was observed to not fully relax. Proctolin has previously been shown to be a cardioacceleratory peptide in arthropods increasing the rate and amplitude of contractions of hearts in several insects and crustaceans (see Orchard et al., 1989; Konopinska and Rosinski, 1999; Lange and Orchard, 2006). Thus, proctolin appears to be present in neurons projecting to the heart in some insects and to control heartbeat. In D. melanogaster, PLI is not associated with processes on the heart, but proctolin receptors are expressed on axon terminals on the adult heart muscle. It has been suggested that proctolin acts as a neurohormone in this insect; stimulating the release of other cardioactive factors from the nerve terminals to control heart rate (see Taylor et al., 2004). Over expression of the proctolin gene in D. melanogaster increased heart rate in pupae lending some support to the biological role of proctolin in controlling heart rate (Taylor et al., 2004).
Proctolin was first isolated based upon its ability to stimulate hindgut contractions in P. americana (Starratt and Brown, 1975), and subsequently shown to be a potent stimulator of contractions of the digestive system in a variety of arthropods (see Lange and Orchard, 2006). Proctolin stimulates contractions of foregut in Schistocerca gregaria, and midgut of D. punctata, and L. migratoria (see Lange and Orchard, 2006). In a similar fashion proctolin stimulates contractions of the anterior midgut and hindgut of R. prolixus, although there are few if any proctolin-like immunoreactive processes projecting to these tissues. Proctolin might act as a neurohormone on these tissues, as shown in some other insect preparations (Lange, 2002; Taylor et al., 2004), and it is possible that proctolin might be released peripherally from the extensive proctolin-like immunoreactive processes that are found over the salivary glands and reproductive tissues, or from the peripheral bipolar neurons that stain for PLI. Typically in insects, proctolin is a most potent stimulator of hindgut contractions but in R. prolixus we found proctolin to be not particularly effective. Indeed, it is a weak stimulator compared to another insect peptide, CCAP (Lee et al., 2011). The weak response of R. prolixus gut contractions to proctolin and the apparent absence of PLI suggest the possibility that only a subset of muscles in these tissues might be sensitive to proctolin and that proctolin is not a major player in the control of forceful contractions of these tissues.
The data clearly shows that, contrary to an earlier report (Ons et al., 2011), proctolin is present in the blood-gorging bug R. prolixus. Thus, we have sequenced the cDNA encoding proctolin, shown proctolin to be present within the CNS, mapped the distribution of proctolin-like immunoreactive cells and processes within the periphery, and demonstrated biological effects of proctolin on a variety of tissues.
Conflict of Interest Statement
The authors declare that the research was conducted in the absence of any commercial or financial relationships that could be construed as a potential conflict of interest.
Acknowledgments
This research was funded by the Natural Sciences and Engineering Research Council of Canada grants to Angela B. Lange and Ian Orchard. We are most grateful to Professor Hans-Jürgen Agricola for providing the proctolin antibody.
References
Belanger, J. H., and Orchard, I. (1993). The locust ovipositor opener muscle: proctolinergic central and peripheral neuromodulation in a centrally driven motor system. J. Exp. Biol. 174, 343–362.
Bendtsen, J. D., Nielsen, H., von Heijne, G., and Brunak, S. (2004). Improved prediction of signal peptides: SignalP 3.0 . J. Mol. Biol. 340, 783–795.
Chiang, R. G., Martens, J. D., and O’Donnell, M. J. (2010). The vagina muscles of the blood-sucking insect Rhodnius prolixus as a model for exploring the physiological effects of proctolin. Physiol. Entomol. 35, 154–159.
Clark, L., Zhang, J. R., Tobe, S., and Lange, A. B. (2006). Proctolin: a possible releasing factor in the corpus cardiacum/corpus allatum of the locust. Peptides 27, 559–566.
Dircksen, H., Neupert, S., Predel, R., Verleyen, P., Huybrechts, J., Strauss, J., Hauser, F., Stafflinger, E., Schneider, M., Pauwels, K., Schoofs, L., and Grimmelikhuijzen, C. J. P. (2011). Genomics, transcriptomics, and peptidomics of Daphnia pulex neuropeptides and protein hormones. J. Proteome Res. 10, 4478–4504.
Drummond, A. J., Ashton, B., Cheung, M., Heled, J., Kearse, M., Moir, R., Stones-Havas, S., Thierer, T., and Wilson, A. (2009). Geneious v4.8. Available at: http://www.geneious.com/
Egerod, K., Reynisson, E., Hauser, F., Williamson, M., Cazzamali, G., and Grimmelikhuijzen, C. J. P. (2003). Molecular identification of the first insect proctolin receptor. Biochem. Biophys. Res. Commun. 306, 437–442.
Ejaz, A., and Lange, A. B. (2008). Peptidergic control of the heart of the stick insect, Baculum extradentatum. Peptides 29, 214–225.
Goudey-Perrière, F., Perrière, C., and Brousse-Gaury, P. (1994). Proctolin promotes vitellogenesis onset in the imaginal molt decapitated cockroach Blaberus craniifer. Comp. Biochem. Physiol. Comp. Physiol. 108, 533–542.
Hauser, F., Cazzamali, G., Williamson, M., Park, Y., Li, B., Tanaka, Y., Predel, R., Neupert, S., Schachtner, J., Verleyen, P., and Grimmelikhuijzen, C. J. P. (2008). A genome-wide inventory of neurohormone GPCRs in the red flour beetle Tribolium castaneum. Front. Neuroendocrinol. 29, 142–165.
Hauser, F., Neupert, S., Williamson, M., Predel, R., Tanaka, Y., and Grimmelikhuijzen, C. P. J. (2010). Genomes and peptidomics of neuropeptides and protein hormones present in the parasitic wasp Nasonia vitripennis. J. Proteome Res. 9, 5296–5310.
Huybrechts, J., Bonhomme, J., Minoli, S., Prunier-Leterme, N., Dombrovsky, A., Abdel-Latief, M., Robichon, A., Veenstra, J. A., and Tagu, D. (2010). Neuropeptide and neurohormone precursors in the pea aphid, Acyrthosiphon pisum. Insect Mol. Biol. 19, 87–95.
Isaac, R. E., Taylor, C. A., Hamasaka, Y., Nässel, D. R., and Shirras, A. D. (2004). Proctolin in the post-genomic era: new insights and challenges. Invert. Neurosci. 5, 51–64.
Johnson, E. C., Garczynski, S. F., Park, D., Crim, J. W., Nässel, D. R., and Taghert, P. H. (2003a). Identification and characterization of a G protein-coupled receptor for the neuropeptide proctolin in Drosophila melanogaster. Proc. Natl. Acad. Sci. U.S. A. 100, 6198–6203.
Johnson, E. C., Bohn, L. M., Barak, L. S., Birse, R. T., Nässel, D. R., Caron, M. G., and Taghert, P. H. (2003b). Identification of Drosophila neuropeptide receptors by G protein-coupled receptors-(β-Arrestin2 interactions. J. Biol. Chem. 278, 52172–52178.
Konopinska, D., and Rosinski, G. (1999). Proctolin, an insect neuropeptide. J. Pept. Sci. 5, 533–546.
Kwok, R., and Orchard, I. (2002). Central effects of the peptides, SchistoFLRFamide and proctolin, on locust oviduct contraction. Peptides 23, 1925–1932.
Lange, A. B. (1990). The presence of proctolin in the reproductive system of Rhodnius prolixus. J. Insect Physiol. 36, 345–351.
Lange, A. B. (2002). A review of the involvement of proctolin as a cotransmitter and local neurohormone in the oviduct of the locust, Locusta migratoria. Peptides 23, 2063–2070.
Lange, A. B., and Orchard, I. (2006). “Proctolin in insects,” in Handbook of Biologically Active Peptides, ed. A. J. Kastin (New York: Academic Press, Elsevier), 177–180.
Lange, A. B., Orchard, I., and Barrett, F. M. (1988). The presence and distribution of proctolin in the blood-feeding bug, Rhodnius prolixus. J. Insect Physiol. 34, 379–386.
Larkin, M. A., Blackshields, G., Brown, N. P., Chenna, R., McGettigan, P. A., McWilliam, H., Valentin, R., Wallace, I. M., Wilm, A., Lopez, R., Thompson, J. D., Gibson, T. J., and Higgins, D. G. (2007). Clustal W and Clustal X version 2.0 . Bioinformatics 23, 2947–2948.
Lee, D. H., Paluzzi, J. P., Orchard, I., and Lange, A. B. (2011). Isolation, cloning and expression of the crustacean cardioactive peptide gene in the Chagas’ disease vector, Rhodnius prolixus. Peptides 32, 475–482.
Lee, D. H., and Lange, A. B. (2011). Crustacean cardioactive peptide in the Chagas’ disease vector, Rhodnius prolixus: presence, distribution and physiological effects. Gen. Comp. Endocrinol. 174, 36–43.
Li, B., Predel, R., Neupert, S., Hauser, F., Tanaka, Y., Cazzamali, G., Williamson, M., Arakane, Y., Verleyen, P., Schoofs, L., Schachtner, J., Grimmelikhuijzen, C. J. P., and Park, Y. (2008). Genomics, transcriptomics, and peptidomics of neuropeptides and protein hormones in the red flour beetle Tribolium castaneum. Genome Res. 18, 113–122.
Ma, M., Gard, A. L., Xiang, F., Wang, J., Davoodian, N., Lenz, P. H., Malecha, S. R., Christie, A. E., and Li, L. (2010). Combining in silico transcriptome mining and biological mass spectrometry for neuropeptide discovery in the Pacific white shrimp Litopenaeus vannamei. Peptides 31, 27–43.
Nässel, D. R. (2002). Neuropeptides in the nervous system of Drosophila and other insects: multiple roles as neuromodulators and neurohormones. Prog. Neurobiol. 68, 1–84.
Nässel, D. R., and Winther, A. M. E. (2010). Drosophila neuropeptides in regulation of physiology and behavior. Prog. Neurobiol. 92, 42–104.
Nygaard, S., Zhang, G., Schiøtt, M., Li, C., Wurm, Y., Hu, H., Zhou, J., Ji, L., Qiu, F., Rasmussen, M., Pan, H., Hauser, F., Krogh, A., Grimmelikhuijzen, C. J. P, Wang, J., and Boomsma, J. J. (2011). The genome of the leaf-cutting ant Acromyrmex echinatior suggests key adaptations to advanced social life and fungus farming. Genome Res. 8, 1339–1348.
Ons, S., Sterkel, M., Diambra, L., Urlaub, H., and Rivera-Pomar, R. (2011). Neuropeptide precursor gene discovery in the Chagas disease vector Rhodnius prolixus. Insect Mol. Biol. 20, 29–44.
Orchard, I., Belanger, J. H., and Lange, A. B. (1989). Proctolin: a review with emphasis on insects. J. Neurobiol. 20, 470–496.
Orchard, I., and Te Brugge, V. (2002). Contractions associated with the salivary glands of the blood-feeding bug, Rhodnius prolixus: evidence for both a neural and neurohormonal coordination. Peptides 23, 693–700.
Pasztor, V., Lange, A. B., and Orchard, I. (1988). Stretch-induced release of proctolin from the dendrites of a lobster sense organ. Brain Res. 458, 199–203.
Persson, M. G. S., and Nässel, D. R. (1999). Neuropeptides in insect sensory neurones: tachykinin-, FMRFamide- and allatotropin-related peptides in terminals of locust thoracic sensory afferents. Brain Res. 816, 131–141.
Predel, R., Neupert, S., Garczynski, S. F., Crim, J. W., Brown, M. R., Russell, W. K., Kahnt, J., Russell, D. H., and Nachman, R. J. (2010). Neuropeptidomics of the mosquito Aedes aegypti. J. Proteome Res. 9, 2006–2015.
Reese, M. G., Eeckman, F. H., Kulp, D., and Haussler, D. (1997). Improved splice site detection in Genie. J. Comput. Biol. 4, 311–323.
Roller, L., Yamanaka, N., Watanabe, K., Daubnerova, I., Žitňan, D., Kataoka, H., and Tanaka, Y. (2008). The unique evolution of neuropeptide genes in the silkworm Bombyx mori. Insect Biochem. Mol. Biol. 38, 1147–1157.
Siwicki, K. K., Beltz, B. S., Schwartz, T. L., and Kravitz, E. A. (1985). Proctolin in the lobster nervous system. Peptides 6, 393–402.
Spittaels, K., Vankeerberghen, A., Torrekens, S., Devreese, B., Grauwels, L., Van Leuven, F., Hunt, D., Shabanowitz, J., Schoofs, L., Van Beeumene, J., and De Loof, A. (1995). Isolation of Ala1-proctolin, the first natural analogue of proctolin, from the brain of the Colorado potato beetle. Mol. Cell. Endocrinol. 110, 119–124.
Starratt, A. N., and Brown, B. E. (1975). Structure of pentapeptide proctolin, a proposed neurotransmitter in insects. Life Sci. 17, 1253–1256.
Taylor, C. A. M., Winther, A. M. E., Siviter, R. J., Shirras, A. D., Isaac, R. E., and Nässel, D. R. (2004). Identification of a proctolin preprohormone gene (Proct) of Drosophila melanogaster: expression and predicted prohormone processing. J. Neurobiol. 58, 379–391.
Te Brugge, V., Paluzzi, J. P., Schooley, D. A., and Orchard, I. (2011). Identification of the elusive peptidergic diuretic hormone in the blood-feeding bug, Rhodnius prolixus: a CRF-related peptide. J. Exp. Biol. 214, 371–381.
Keywords: insect, mRNA, MALDI-TOF, heart, salivary gland, reproductive tissues, immunohistochemistry, muscle contraction
Citation: Orchard I, Lee DH, da Silva R and Lange AB (2011) The proctolin gene and biological effects of proctolin in the blood-feeding bug, Rhodnius prolixus. Front. Endocrin. 2:59. doi: 10.3389/fendo.2011.00059
Received: 17 August 2011; Accepted: 04 October 2011;
Published online: 21 October 2011.
Edited by:
Eric W. Roubos, Radboud University Nijmegen, NetherlandsReviewed by:
Cornelis J. P. Grimmelikhuijzen, University of Copenhagen, DenmarkLiliane Schoofs, Catholic University of Leuven, Belgium
Copyright: © 2011 Orchard, Lee, da Silva and Lange. This is an open-access article subject to a non-exclusive license between the authors and Frontiers Media SA, which permits use, distribution and reproduction in other forums, provided the original authors and source are credited and other Frontiers conditions are complied with.
*Correspondence: Ian Orchard, Department of Biology, University of Toronto Mississauga, 3359 Mississauga Road, Mississauga, ON, Canada L5L 1C6. e-mail: ian.orchard@utoronto.ca