- 1 Department of Cell Biology, Physiology and Immunology, University of Córdoba, Córdoba, Spain
- 2 CIBER Fisiopatología de la Obesidad y Nutrición, Córdoba, Spain
- 3 Instituto Maimonides de Investigaciones Biomédicas de Córdoba, Córdoba, Spain
- 4 Division of Endocrinology, Diabetes and Hypertension, Brigham and Women’s Hospital and Harvard Medical School, Boston, MA, USA
Gonadotropin-releasing hormone (GnRH) is the ultimate output signal of an intricate network of neuroendocrine factors that, acting on the pituitary, trigger gonadotropin release. In turn, gonadotropins exert their trophic action on the gonads to stimulate the synthesis of sex steroids thus completing the gonadotropic axis through feedback regulatory mechanisms of GnRH release. These feedback loops are predominantly inhibitory in both sexes, leading to tonic pulsatile release of GnRH from puberty onward. However, in the female, rising levels of estradiol along the estrous cycle evoke an additional positive feedback that prompts a surge-like pattern of GnRH release prior to ovulation. Kisspeptins, secreted from hypothalamic Kiss1 neurons, are poised as major conduits to regulate this dual secretory pathway. Kiss1 neurons are diverse in origin, nature, and function, convening distinct neuronal populations in two main hypothalamic nuclei: the arcuate nucleus (ARC) and the anteroventral periventricular nucleus. Recent studies from our group and others point out Kiss1 neurons in the ARC as the plausible generator of GnRH pulses through a system of pulsatile kisspeptin release shaped by the coordinated action of neurokinin B (NKB) and dynorphin A (Dyn) that are co-expressed in Kiss1 neurons (so-called KNDy neurons). In this review, we aim to document the recent findings and working models directed toward the identification of the Kiss1-dependent mechanisms of GnRH release through a synoptic overview of the state-of-the-art in the field.
Introduction
Reproductive function is an exquisitely regulated process that ensures perpetuation of the species. A plethora of central and peripheral signals interplay to modulate the coordinated action of the hypothalamus, pituitary, and gonads, which form the gonadotropic (aka HPG) axis. The hypothalamic decapeptide gonadotropin-releasing hormone (GnRH) is the ultimate central elicitor that conveys these regulatory signals down to the pituitary level to dictate the specific pattern of gonadotropin release (Fink, 2000). GnRH secretion varies significantly along the animal’s life, being subjected to circadian and ultradian regulation, i.e., seasonal and developmental changes. For instance, GnRH secretion is enhanced at the time of puberty onset (Harris and Levine, 2003), leading to high frequency pulses that induce the awakening of the reproductive function in both males and females and are maintained from that period throughout the animal’s lifespan. To note, GnRH displays a bimodal pattern of release: episodic versus surge-like (Maeda et al., 2010), where gonadal steroids play a crucial role. In this context, both sexes are subjected to the inhibitory action of these gonadal steroids through negative feedback mechanisms at the hypothalamic and pituitary level, which determine the tonic mode of GnRH secretion. In addition, adult females also exhibit a positive, estradiol-dependent, feedback of GnRH release as a unique surge-like peak that evokes ovulation by means of the consequent surge of luteinizing hormone (LH) release (Moenter et al., 1991). We are only starting to decipher the mechanisms underlying this dual regulatory process of GnRH release (Glidewell-Kenney et al., 2007), which is currently a subject of intense research in the field.
Focusing on the intermittent release of GnRH, the mechanism that dictates the amplitude and frequency of each pulse remains also poorly understood, being a matter of intense debate among neuroendocrinologists. Hence, in this review, we aim to offer a novel insight into a plausible mechanism of GnRH pulse generation through a comprehensive compilation of the latest advances in the field focusing on the interaction between kisspeptins and neurokinin B (NKB).
Neuroanatomical Distribution of GnRH Neurons
Gonadotropin-releasing hormone neurons present a scattered distribution in the brain, which has limited the thorough characterization of the physiological properties of this neuronal group. GnRH neurons originate in the olfactory placode. From embryonic day 9.5 onward (in rodents), they initiate a migratory process from this location to distinct brain areas that vary depending on the species (Schwanzel-Fukuda and Pfaff, 1989; Wray et al., 1989). In rodents, mature GnRH cell bodies are present in the medial septum, preoptic area, and the anterior hypothalamic areas (Herbison, 2006). To note, a recent study has evidenced that the anatomical distribution of these neurons depends upon their birthdate, with neurons that undergo differentiation earlier in the embryonic life populating the rostral areas of the brain (Jasoni et al., 2009). GnRH neurons, regardless of the location of their cell bodies, project to the median eminence in a process guided by yet unknown factors from the medio-basal hypothalamus (Gibson et al., 2000). Therein, GnRH is secreted into the hypophyseal portal system to stimulate gonadotropin release.
Central Control of Reproductive Function: The Role of Kiss1 Neurons
Reproduction is an extremely energy costly process for the organism and, as such, is subjected to very tight regulatory mechanisms (Hill et al., 2008). As mentioned above, GnRH is the main factor that integrates the complex array of central and peripheral cues onto pituitary gonadotropes. However, GnRH neurons are not directly receptive to most of the major regulators of reproduction, e.g., metabolic cues, such as leptin, or gonadal factors such as sex steroids. In this context, Kiss1 neurons have been extensively documented in the last few years as major gatekeepers of reproductive function. Inactivating mutations in either KISS1 or KISS1R genes (encoding kisspeptins and the Kiss1 receptor – formerly known as GPR54, respectively) lead to hypogonadotropic hypogonadism and infertility in both humans and mice (De Roux et al., 2003; Funes et al., 2003; Seminara et al., 2003). Importantly, the vast majority of GnRH neurons express the Kiss1 receptor (Han et al., 2005) and Kiss1 neurons are sensitive to sex steroids as evidenced by the presence of estrogen receptor (ER)-α, ERβ, and androgen receptor (Smith et al., 2005a,b). Thus, Kiss1 neurons have been described to play a role as a common funnel to transmit essential information for reproductive viability down to the seemingly irresponsive GnRH neurons by means of kisspeptin release (Navarro and Tena-Sempere, 2011). To note, the population of Kiss1 neurons is sexually differentiated, being predominantly present at the arcuate nucleus (ARC) in both sexes (Gottsch et al., 2004), where they mediate the negative feedback of sex steroids upon GnRH release (Smith et al., 2005a) and the anteroventral periventricular area (AVPV/PeN) with greater density in females than in males, specially in rodents, where Kiss1 neurons are poised as elicitors of the preovulatory GnRH/LH surge through positive feedback mechanisms (Smith et al., 2005a; Figure 1). Importantly, the mechanism underlying this differential activation of Kiss1 neurons in the ARC versus the AVPV relies on the ability of estradiol to activate Kiss1 transcription via estrogen response element (ERE)-independent pathways in the ARC, whereas its activation in the AVPV is mediated by the binding of the ER to the EREs in the Kiss1 promoter, so-called “classical” route of ER action (Gottsch et al., 2009).
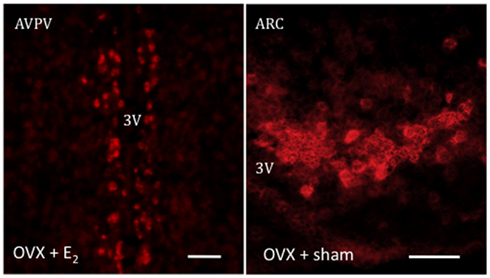
Figure 1. Representative photomicrographs depicting an in situ hybridization assay for Kiss1 mRNA, labeled using digoxigenin coupled with vector red. The expression of Kiss1 mRNA is stimulated in the presence of E2 in the AVPV or its absence in the ARC. Scale bar 50 μm.
In this vein, in the absence of the inhibitory drive of sex steroids, GnRH shows a strictly episodic pattern of release in males (Caraty and Locatelli, 1988; Tilbrook and Clarke, 2001) and during most of the ovarian cycle in females (Levine and Ramirez, 1982), suggesting that similar mechanisms for pulse generation might exist in both sexes. Noteworthy, intermittent hormone release is a feature present in many neuroendocrine systems, including GnRH release, however, determining whether the final pattern of release is dictated by the neuron itself or through a synchronized circuitry that operates upon them, remains largely controversial.
GnRH Neurons as Pulse Generators
Over the last two decades, a number of studies have suggested that GnRH neurons themselves present a pattern of firing bursts that could, indeed, account for the pulsatile release of this decapeptide. First, the GnRH cell line, GT1, exhibits spontaneous pulses of neuronal activation and GnRH secretion in the absence of any other cell line (Martinez De La Escalera et al., 1992; Bosma, 1993). Second, embryonic GnRH cells obtained from the nasal placode of rats displayed a pulsatile fashion of GnRH release (Funabashi et al., 2000).
This rhythmic pattern of GnRH release seems to be Ca2+-dependent (Krsmanovic et al., 1992). Indeed, cultured embryonic GnRH cells from monkeys exhibit synchronized Ca2+ oscillations every 50–70 min, which is similar to the interval of GnRH pulses observed in vivo (Terasawa et al., 1999). Moreover, this process is stimulated by cAMP (Krsmanovic et al., 2001) in a mechanism mediated by specific A-kinase anchoring proteins (AKAPs; Chen et al., 2010). However, a recent study by Frattarelli et al. (2011) indicates that in GT1-7 cells, only 25% of GnRH and cAMP pulses coincide, suggesting that while cAMP may positively regulate GnRH secretion, it does not participate in the mechanism of pulsatile GnRH release. Another remarkable feature of GnRH neurons is their ability to synchronize the release of GnRH with time in culture through gap junction coupling (Bose et al., 2010). This, together with the fact that GnRH neurons do express the GnRH receptor and present auto-feedback loops in approximately 50% of them (Han et al., 2010) may suggest the presence of auto-regulatory pathways. Nonetheless, these mechanisms remain to be validated in vivo since the close proximity of GnRH neurons in culture is not present in the brain where, as mentioned above, GnRH neurons occupy a disperse distribution that may compromise direct interactions between them.
Despite these evidences signifying the presence of an internal pacemaker, the inability of GnRH neurons to modify its pattern of release under critical physiologic conditions for the regulation of the reproductive axis such us puberty onset, variation in the sex steroid milieu, etc., makes the presence of additional regulatory mechanisms – able to respond to a wider array of regulators, mandatory.
GnRH Pulse Generator Outside GnRH Neurons
We could postulate that GnRH neurons, as pacemaker neurons, would need the coordinated interaction of an ensemble of regulatory factors that led to the fine-tuning of GnRH secretion. Indeed, mounting data support this contention. The presence of a master GnRH pulse generator has been localized within the medio-basal hypothalamus, pointing to the ARC as the most likely candidate. First, female rhesus monkeys bearing bilateral lesions in the medio-basal hypothalamus showed absence of endogenous GnRH/LH pulses (Pohl et al., 1983). Second, hypothalamic explants of monkeys and rats devoid of GnRH cell bodies exhibit GnRH pulses (Purnelle et al., 1997; Woller et al., 2010). Third, despite some discrepancies in the field, endogenous pulses of GT1-7 cells seem to present a cadence of 21–26 min (Bosma, 1993; Moenter et al., 2003) while in vivo, GnRH pulses present 40–60 min intervals depending on the species (Han et al., 2010). Fourth, electrophysiological recordings of multi-unic activity (MUA) volleys located in the ARC, invariably resemble LH pulses in different species (Goubillon et al., 1995; Ohkura et al., 2009). Fifth, as mentioned previously, GnRH neurons themselves do not express ER alpha (Hrabovszky et al., 2000). Therefore, they are not able to properly respond to changes in the sex steroid milieu, which constitutes a major regulatory drive to the gonadotropic axis. Indeed, in the absence of negative feedback of sex steroids after gonadectomy, or menopause in primates, GnRH pulses are significantly increased (Gore et al., 2004). Altogether, there are sufficient evidences to locate the primary generator of GnRH pulses in the ARC. However, we cannot rule out that other mechanisms may play a role in regulating GnRH discharges and that they could be placed in different brain regions, e.g., inputs coming from the suprachiasmatic nucleus (SCN) through vasoactive intestinal polypeptide (VIP; Krajnak et al., 1998; Gerhold et al., 2005) or GABAergic and glutamatergic interactions (Chu and Moenter, 2005) among those possible pathways.
Kiss1 Neurons as Holders of the GnRH Pulse Generator
The ARC presents a complex array of neuronal networks that participate in the control of reproduction and/or metabolism. Among those, the subpopulation of Kiss1 neurons located in this nucleus could serve the function of GnRH pulse generator based on the following findings: (a) GnRH neurons express the Kiss1 receptor and are activated by kisspeptins (Han et al., 2005); (b) Kiss1 neurons from the ARC contact GnRH terminals in the median eminence of rats and sheep (Matsuyama et al., 2011; Uenoyama et al., 2011); (c) kisspeptin release to the portal system in monkeys is pulsatile (Keen et al., 2008); (d) MUA volleys in goats, that closely reproduce LH pulses, are recorded from the surroundings of Kiss1 neurons and are not affected after exogenous kisspeptin treatment unlike LH pulses (Ohkura et al., 2009); (e) the number of Kiss1-expressing neurons in the ARC of lambs is greater in animals exhibiting higher frequency of LH pulses (Redmond et al., 2011); (f) the increase in GnRH neuronal firing observed in menopausal primates due to the absence of sex steroids (Gore et al., 2004) correlates with the higher level of Kiss1 expression in the infundibular nucleus of post-menopausal women and primates (Rometo et al., 2007; Eghlidi et al., 2010; Hrabovszky et al., 2011). To note, earlier reports documented that the pulsatile release of GnRH is blunted after prolonged periods of estradiol withdrawal, e.g., older post-menopausal women (Hall et al., 2000), which may reflect the potential decrease in Kiss1 expression in the ARC produced by aging, as was recently documented in old acyclic rats (Downs and Wise, 2011) although, intriguingly, it does not seem to be the case in post-menopausal women where kisspeptin immunoreactivity remains elevated well beyond menopause (Hrabovszky et al., 2011), suggesting possible species differences in the regulation of Kiss1 expression in aged individuals; (g) the administration of a kisspeptin antagonist into the ARC – but not the POA, strongly suppresses LH pulses in rats (Li et al., 2009); (h) the administration of intravenous kisspeptin pulses to agonadal juvenile monkeys elicits a sustained train of GnRH discharges (Plant et al., 2006). In addition, (i) an elegant study by Gottsch et al. (2011) has recently demonstrated by whole-cell patch recordings that Kiss1 neurons, isolated from Kiss1-CreGFP mice, present spontaneous activity as well as h- and T-type Ca2+ currents (Figure 2), which are typical features of pacemaker neurons. However, a note of caution should be added regarding possible species differences in the generation of GnRH pulses. Thus, a recent study by Chan et al. (2011) demonstrates that iv administration of kisspeptin to adult men not only stimulates LH release but delays the occurrence of the next LH pulse by an interval similar to that of normal interpulse intervals, which would suggest a reset of the GnRH pulse generator.
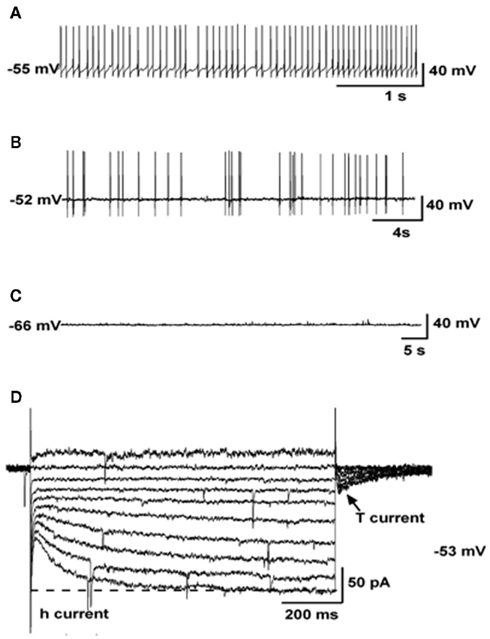
Figure 2. Electrophysiological characteristics of arcuate Kiss1 neurons in oil-treated OVX Kiss1-CreGFP mice using whole-cell patch recording. Kiss1 neurons in the ARC (of the female) rest at −63.8 ± 2.3 mV (n = 20). (A–C), Representative traces of action potentials recorded from arcuate Kiss1 neurons showing tonic (A), irregular (B), and silent (C) firing patterns. (D), Ensemble of currents in response to depolarizing/hyperpolarizing steps from −50 to −140 mV illustrating the expression of a hyperpolarization-activated cation current (h-current) and a T-type Ca2+ current (arrow) in a representative Kiss1 neuron. Vhold = −60 mV. Adapted with permission from Gottsch et al. (2011).
The Role of Neurokinin B in the Generation of Kisspeptin Pulses
Neurokinin B has recently emerged as a critical player in the central control of reproductive function. In 2009, human genetic studies showed that patients bearing inactivating mutations in the genes encoding NKB or its receptor (neurokinin 3 receptor, NK3R), TAC3 and TACR3 respectively in humans, exhibit hypogonadotropic hypogonadism and infertility (Topaloglu et al., 2009). These findings have been also (partially) recapitulated in Tacr3 null mice (Yang et al., 2012) indicating that the NKB/NK3R system plays a role in the control of gonadotropin secretion in different species. Initial reports denoted an inhibitory action of an NKB agonist, senktide, on LH release in rodents (Sandoval-Guzman and Rance, 2004; Navarro et al., 2009), however, we have recently documented high sensitivity of the NKB/NK3R system to circulating levels of sex steroids, which allows LH release under the appropriate steroid milieu in prepubertal and adult female rats (Navarro et al., 2011a, 2012). In addition, to date, a stimulatory role of NKB on LH release has also been reported in mice, monkeys, goats, and sheep (Billings et al., 2010; Ramaswamy et al., 2010; Wakabayashi et al., 2010; Navarro et al., 2011b). Recently, though, a report by Kinsey-Jones and colleagues showed otherwise. In their study, senktide exerted a decrease in LH release in ovariectomized (OVX) and estradiol-replaced female rats (Kinsey-Jones et al., 2012). Notwithstanding, their estradiol replacement seemingly failed to decrease LH release after ovariectomy, which is a required proof of the proper action of the negative feedback of estradiol and might explain this a priori controversy. Therefore, assuming that these animals reproduced an OVX+ sham model and considering the stimulation of LH release that they also observed in intact diestrus rats, these results would actually be in keeping with our previous study in female rats (Navarro et al., 2011a). Regarding its anatomical distribution, the genes encoding NKB in rodents (Tac2) and NK3R (Tacr3) are enormously spread out throughout the entire brain (Warden and Young, 1988; Shughrue et al., 1996; Navarro et al., 2011a), where they have been proposed to mediate a number of physiological processes (Pantaleo et al., 2010); however, regarding the areas known to hold neuromodulators of reproductive function, we could highlight the ARC, ventromedial (VMN), and paraventricular (PVN) nuclei as well as the lateral hypothalamic area (LHA; Navarro et al., 2011a). Importantly, not only NKB is expressed in the ARC but virtually all Kiss1 neurons in this nucleus (but not in the AVPV/PeN) co-express NKB – and Dynorphin A (Goodman et al., 2007; Navarro et al., 2009; Wakabayashi et al., 2010), which has served the scientific community to rename these neurons as KNDy neurons (Lehman et al., 2010). Moreover, KNDy neurons do express NK3R (Burke et al., 2006; Navarro et al., 2009) facilitating autosynaptic loops within the dense network of NKB/kisspeptin fibers present in the ARC as documented in rodents (Burke et al., 2006; Krajewski et al., 2010; Yeo and Herbison, 2011). Indeed, the following pieces of evidence support the contention that NKB acts upstream of, or immediately on, Kiss1 neurons in the ARC to exert its regulatory action on reproductive function: (a) central administration of the NKB agonist senktide to gonadectomized and estradiol-replaced female rats induces C-fos mRNA expression in Kiss1 neurons (Navarro et al., 2011a); (b) senktide and NKB profoundly depolarize Kiss1 neurons measured by whole-cell patch recording, phenomenon that is prevented by the NKB antagonist SB222200 (Navarro et al., 2011b); (c) animals bearing a non-functional Kiss1 receptor (Kiss1r KO mice) do not respond to the central administration of senktide in terms of LH release while wild-type controls do (García-Galiano et al., 2012); (d) central administration of NKB to goats induces a clear increase in the frequency and amplitude of MUA volleys in the ARC, which were invariably mirrored by LH pulses (Wakabayashi et al., 2010; Figure 3), yet the administration of kisspeptin modified LH pulses without modifying MUA volleys (Ohkura et al., 2009); (e) juvenile monkeys showing blunted LH responses to senktide due to NK3R desensitization still respond to kisspeptin, however, those subjected to KISS1R desensitization showed virtual absence of LH response to senktide, although admittedly, a small residual response to senktide was observed in this model suggesting a likely (minor) kisspeptin-independent action of senktide on GnRH release in the monkey (Ramaswamy et al., 2010, 2011). Altogether, these data make the case for a very likely action of NKB upon Kiss1 neurons, however, we cannot rule out the action of this peptide on additional brain areas. In this vein, preliminary data showed expression of NK3R in GnRH terminals of rats (Krajewski et al., 2005; Burke et al., 2006) and Tacr3 mRNA in GnRH neurons in the mouse (Todman et al., 2005) as well as in the immortalized GT1-7 cells – a model of differentiated GnRH neurons (Glidewell-Kenney et al., 2010). However, these results are controversial. On the one hand, a call of caution needs to be exercised when using immortalized cell lines, which may not faithfully resemble in vivo models and, on the other hand, the latter data in mice and rats seem to be rebutted by recent studies documenting the absence of NK3R immunolocalization and Tacr3 mRNA in GnRH neurons of sheep (Amstalden et al., 2009) and mice (Navarro et al., 2011b), respectively. Furthermore, the lack – or residual expression – of NK3R in GnRH neurons is supported by (1) the very illustrative studies of desensitization of NK3R versus Kiss1r in the agonadal juvenile monkey mentioned above, where kisspeptin-desensitized monkeys displayed a robust suppression (albeit not complete) of the LH response to senktide (Ramaswamy et al., 2011) and (2) the lack of action potentials in GnRH–GFP neurons subjected to whole-cell recordings after senktide treatment in the mouse (Navarro et al., 2011b). It is worth mentioning that this study shows lack of activity at the GnRH cell body level but does not rule out the presence of NK3R at the level of GnRH terminals. In this sense, (3) Corander et al. (2010) showed that, unlike kisspeptin, addition of NKB to hypothalamic explants from male rats devoid of GnRH cell bodies did not evoke any effect on GnRH release, which, initially, would preclude a direct action at the level of these terminals.
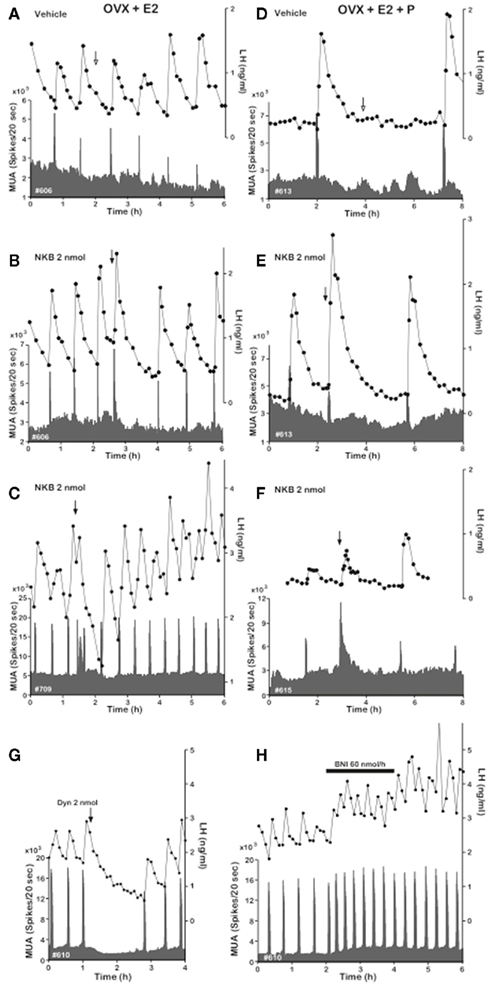
Figure 3. Effect of NKB on MUA and plasma LH in OVX-plus-E2- and OVX-plus-E2-plus-P-treated goats. Representative profiles of MUA and plasma LH concentrations in OVX-plus-E2(A–C) and OVX-plus-E2-plus-P (D–F) goats that received an intracerebroventricular injection of vehicle (A,D) or 2 nmol (B,C,E,F) of NKB are shown. Also, effects of Dyn and nor-BNI on the MUA and plasma LH in the OVX goat are included. (G), Representative profiles of MUA and plasma LH concentrations in one animal that received an intracerebroventricular injection of 2 nmol of Dyn are shown. The arrow indicates timing of injection. (H), Representative profiles of MUA and plasma LH concentrations in one animal that received an intracerebroventricular infusion of nor-BNI at a rate of 60 nmol 600 μl−1 h−1 for 2 h are shown. The bar indicates the period of infusion. Adapted with permission from Wakabayashi et al. (2010).
In any case, the number of NKB neurons in the ARC of male mice seems to be twice that of the Kiss1 neurons (Navarro et al., 2011b). This, taken together with the fact that NKB fibers, at least in mice and rats, project to neighboring hypothalamic areas of the ARC – including bilateral projections within the ARC and to the median eminence, periventricular nucleus, BNST, dorsomedial nucleus, lateral hypothalamus, and rostral preoptic area (Burke et al., 2006; Krajewski et al., 2010; Yeo and Herbison, 2011) – suggests the existence of possible, yet unknown, additional regulatory pathways.
In this intricate machinery of co-transmitters of kisspeptin, the role of dynorphin remains far less characterized. Dynorphin has been long recognized as an inhibitor of gonadotropin release in a number of species (Schulz et al., 1981; Kinoshita et al., 1982). Importantly, based on recent studies in the goat, the role of dynorphin seems to lay upstream of the Kiss1 neuron. Thus, central administration of the dynorphin receptor (Kappa opiod receptor, KOR) agonist, U50488, strongly suppresses MUA volleys in the ARC and LH pulses (Wakabayashi et al., 2010; Figure 3). In this same direction, the administration of a KOR antagonist, nor-BNI, increases the frequency of MUA volleys that is mirrored by the same increase in LH pulses (Wakabayashi et al., 2010; Figure 3). Therefore, these data suggest that dynorphin participates to shut down Kiss1 neurons, hence blocking kisspeptin–GnRH–LH release. Nonetheless, in this scenario, how (and where) dynorphin plays its role remains controversial. It is clear that the action of dynorphin eventually turns back to Kiss1 neurons through direct (autosynaptic contact) or indirect (intermediate neurons) mechanisms. Latest evidences seem to support the latter alternative. We have recently documented that Kiss1 neurons in the ARC of male mice are virtually devoid of KOR (less than 6%; Navarro et al., 2011b), while females displayed a slightly greater percentage of colocalization (∼20%; Navarro et al., 2009), indicating the possible existence of a marginal subset of Kiss1 neurons in the ARC expressing KOR. In any case, even if KOR is present in a reduced number of Kiss1 neurons, the primary place of action of dynorphin seems to reside outside and upstream of Kiss1 neurons (Figure 4) since GnRH neurons are also devoid of KOR (Mitchell et al., 1997; Sannella and Petersen, 1997); however, the identity of this neuronal population – that must project back to Kiss1 neurons, remains a mystery.
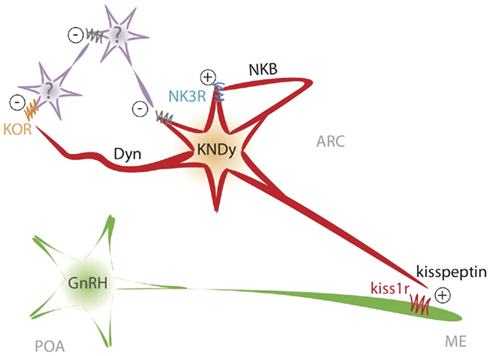
Figure 4. Schematic representation of the proposed model of interaction between NKB and kisspeptin in the ARC to shape GnRH/LH pulses.
Altogether, there is compelling evidence to infer that kisspeptin release is pulsatile and that those pulses correlate with GnRH/LH pulses, which, presumably, are dependent on the former. Consequently, we have proposed a model whereby KNDy neurons, in the absence of the inhibitory drive of sex steroids, would activate the NKB pathway and release this neuropeptide on the same KNDy neuron through recurrent collaterals as well as to nearby NKDy neurons (Figure 4). This feature might be of crucial importance for the proper functioning of this model, playing a critical role in the synchronization of KNDy neurons. In this sense, in order to present unique GnRH pulses, the population of KNDy neurons must evoke a unique kisspeptin pulse, which is initiated by a spreading NKB activating wave across the ARC. This possibility is in keeping with the dense network of NKB fibers surrounding KNDy neurons in the ARC, as commented above (Burke et al., 2006; Krajewski et al., 2010; Yeo and Herbison, 2011). Next, the activation of the NKB receptor, NK3R, initiates the process of kisspeptin and dynorphin secretion. Kisspeptin would then reach the kisspeptin receptor at the GnRH terminals to induce GnRH release to the median eminence (Figure 4). To note, there is no direct evidence of the presence of Kiss1r at the level of GnRH terminals, however, this could be inferred based on the observation that kisspeptin can elicit GnRH secretion from explants of the mediobasal hypothalamus, which contains few, if any, GnRH cells bodies (Irwig et al., 2004; Thompson et al., 2004; Castellano et al., 2006; D’Anglemont De Tassigny et al., 2008) and the close apposition of kisspeptin fibers at the GnRH terminals (Matsuyama et al., 2011; Uenoyama et al., 2011). At the same time, dynorphin, acting mainly on yet unknown intermediate neurons, feeds back to Kiss1 neurons through inhibitory signals – dependent on Gi/G0 protein coupling (Schoffelmeer et al., 1988) – that eventually shut down NKB and kisspeptin release. In this situation, the cease in NKB secretion terminates the release of dynorphin and, therefore, the action of the inhibitory drive. As a consequence, NKDy neurons would become readily available to re-start NKB secretion, then leading to a new pulse of kisspeptin (Figure 4). However, one could be tempted to hypothesize that KNDy neurons are, most likely, not able to discriminate when to secrete one neurotransmitter or another after a process of neuronal activation and, therefore, all these factors (kisspeptin, NKB, and dynorphin) could be released at the same time after a common burst comprising all KNDy neurons. If this were true, it could mean a critical limitation of the model. Although this is merely speculative, current data indicates that this limitation could, at least partially, be overcome because of the restricted sites of action of each of those factors in the context of the generation of GnRH pulses. (a) Kisspeptin would act only on GnRH neurons, since KNDy neurons themselves in the ARC are devoid of Kiss1 receptor (D’Anglemont De Tassigny et al., 2008) and therefore do not respond to kisspeptin (Navarro et al., 2011b); (b) NKB would act mainly on KNDy neurons since GnRH neurons (at least in mice and sheep) do not express detectable levels of NK3R (Billings et al., 2010; Navarro et al., 2011b); (c) GnRH neurons do not express KOR (Mitchell et al., 1997; Sannella and Petersen, 1997) and KNDy neurons might do it only in a low percentage (Navarro et al., 2009, 2011b), then indicating that dynorphin must act upon intermediate neurons, increasing the turn around time of the inhibitory signal onto KNDy neurons (Figure 4). This would lead to a short delay period after the stimulation of NKB, therefore allowing for the appearance of a kisspeptin pulse.
Conclusion and Future Perspectives
During the last few years, the data supporting the pivotal role of kisspeptin in the regulation of GnRH release in a wide range of species is so consistent that we can ensure this phenomenon without a doubt. In this manuscript, we have offered a comprehensive review of the latest advances in the physiology and mechanism of action of Kiss1 neurons in the ARC, adding an intriguing new role to this neuronal group: serve as the GnRH pulse generator, whose characterization has remained elusive to neuroendocrinologists for decades. As a note of caution, we do not position this mechanism as the only center governing GnRH release. There are, indeed, evidences to believe that in the absence of Kiss1 signaling there is a residual, but significant, stimulatory drive onto GnRH neurons from an unknown source (Chan et al., 2009). Thus, it is feasible that a more complex network of neuronal circuits interplays to exert their regulation on GnRH release under specific physiologic conditions, ensuring the appropriate response of the gonadotropic axis to those circumstances. For this reason, we do believe that the tandem Kisspeptin/NKB is essential for the tonic episodic release of GnRH while yet there are significant open questions in this model that await to be addressed in the upcoming years. For example, it would be of great interest to decipher what the genuine role of dynorphin in this model is and what interneurons it is acting on. In addition, it is known that GnRH neurons exhibit a prolonged period of activation after a single kisspeptin pulse (Han et al., 2005), therefore, there must be a factor that actively block kisspeptin’s action on GnRH neurons after every pulse, whose nature is to date unidentified.
In sum, kisspeptin and NKB play a fundamental role in the tonic release of GnRH; however, further research is needed to offer a more detailed insight into the exact process of generation of GnRH pulses.
Conflict of Interest Statement
The author declares that the research was conducted in the absence of any commercial or financial relationships that could be construed as a potential conflict of interest.
Acknowledgments
This research was supported by the EU Marie Curie Outgoing International fellowship within the Seventh Framework Program of the EU. The author is grateful to Drs. Manuel Tena-Sempere and Leonor Pinilla for their constructive comments on the manuscript. The author reports no conflicts of interest.
References
Amstalden, M., Coolen, L. M., Hemmerle, A. M., Billings, H. J., Connors, J. M., Goodman, R. L., and Lehman, M. N. (2009). Neurokinin 3 receptor immunoreactivity in the septal region, preoptic area and hypothalamus of the female sheep: colocalisation in neurokinin B cells of the arcuate nucleus but not in gonadotropin-releasing hormone neurones. J. Neuroendocrinol. 22, 1–12.
Billings, H. J., Connors, J. M., Altman, S. N., Hileman, S. M., Holaskova, I., Lehman, M. N., Mcmanus, C. J., Nestor, C. C., Jacobs, B. H., and Goodman, R. L. (2010). Neurokinin B acts via the neurokinin-3 receptor in the retrochiasmatic area to stimulate luteinizing hormone secretion in sheep. Endocrinology 151, 3836–3846.
Bose, S., Leclerc, G. M., Vasquez-Martinez, R., and Boockfor, F. R. (2010). Administration of connexin43 siRNA abolishes secretory pulse synchronization in GnRH clonal cell populations. Mol. Cell. Endocrinol. 314, 75–83.
Bosma, M. M. (1993). Ion channel properties and episodic activity in isolated immortalized gonadotropin-releasing hormone (GnRH) neurons. J. Membr. Biol. 136, 85–96.
Burke, M. C., Letts, P. A., Krajewski, S. J., and Rance, N. E. (2006). Coexpression of dynorphin and neurokinin B immunoreactivity in the rat hypothalamus: morphologic evidence of interrelated function within the arcuate nucleus. J. Comp. Neurol. 498, 712–726.
Caraty, A., and Locatelli, A. (1988). Effect of time after castration on secretion of LHRH and LH in the ram. J. Reprod. Fertil. 82, 263–269.
Castellano, J. M., Navarro, V. M., Fernandez-Fernandez, R., Castano, J. P., Malagon, M. M., Aguilar, E., Dieguez, C., Magni, P., Pinilla, L., and Tena-Sempere, M. (2006). Ontogeny and mechanisms of action for the stimulatory effect of kisspeptin on gonadotropin-releasing hormone system of the rat. Mol. Cell. Endocrinol. 257–258, 75–83.
Chan, Y. M., Broder-Fingert, S., Wong, K. M., and Seminara, S. B. (2009). Kisspeptin/Gpr54-independent gonadotropin-releasing hormone activity in Kiss1 and Gpr54 mutant mice. J. Neuroendocrinol. 21, 1015–1023.
Chan, Y. M., Butler, J. P., Pinnell, N. E., Pralong, F. P., Crowley, W. F. Jr., Ren, C., Chan, K. K., and Seminara, S. B. (2011). Kisspeptin resets the hypothalamic GnRH clock in men. J. Clin. Endocrinol. Metab. 96, E908–E915.
Chen, Q., Weiner, R. I., and Blackman, B. E. (2010). Decreased expression of A-kinase anchoring protein 150 in GT1 neurons decreases neuron excitability and frequency of intrinsic gonadotropin-releasing hormone pulses. Endocrinology 151, 281–290.
Chu, Z., and Moenter, S. M. (2005). Endogenous activation of metabotropic glutamate receptors modulates GABAergic transmission to gonadotropin-releasing hormone neurons and alters their firing rate: a possible local feedback circuit. J. Neurosci. 25, 5740–5749.
Corander, M. P., Challis, B. G., Thompson, E. L., Jovanovic, Z., Loraine Tung, Y. C., Rimmington, D., Huhtaniemi, I. T., Murphy, K. G., Topaloglu, A. K., Yeo, G. S., O’Rahilly, S., Dhillo, W. S., Semple, R. K., and Coll, A. P. (2010). The effects of neurokinin B upon gonadotropin release in male rodents. J. Neuroendocrinol. 22, 181–187.
D’Anglemont De Tassigny, X., Fagg, L. A., Carlton, M. B., and Colledge, W. H. (2008). Kisspeptin can stimulate gonadotropin-releasing hormone (GnRH) release by a direct action at GnRH nerve terminals. Endocrinology 149, 3926–3932.
De Roux, N., Genin, E., Carel, J. C., Matsuda, F., Chaussain, J. L., and Milgrom, E. (2003). Hypogonadotropic hypogonadism due to loss of function of the Kiss1-derived peptide receptor GPR54. Proc. Natl. Acad. Sci. U.S.A. 100, 10972–10976.
Downs, J. L., and Wise, P. M. (2011). “Age-related resistance to 17β-estradiol-mediated inhibition of Kiss1 in the arcuate nucleus,” in Society for Neuroscience Annual Meeting, Washington, DC.
Eghlidi, D. H., Haley, G. E., Noriega, N. C., Kohama, S. G., and Urbanski, H. F. (2010). Influence of age and 17beta-estradiol on kisspeptin, neurokinin B, and prodynorphin gene expression in the arcuate-median eminence of female rhesus macaques. Endocrinology 151, 3783–3794.
Fink, G. (2000). “Neuroendocrine regulation of pituitary function: general principles,” in Neuroendocrinology in Physiology and Medicine, eds P. M. Conn and M. E. Freeman (Totowa: Humana Press), 107–134.
Frattarelli, J. L., Krsmanovic, L. Z., and Catt, K. J. (2011). The relationship between pulsatile GnRH secretion and cAMP production in immortalized GnRH neurons. Am. J. Physiol. Endocrinol. Metab. 300, E1022–E1030.
Funabashi, T., Daikoku, S., Shinohara, K., and Kimura, F. (2000). Pulsatile gonadotropin-releasing hormone (GnRH) secretion is an inherent function of GnRH neurons, as revealed by the culture of medial olfactory placode obtained from embryonic rats. Neuroendocrinology 71, 138–144.
Funes, S., Hedrick, J. A., Vassileva, G., Markowitz, L., Abbondanzo, S., Golovko, A., Yang, S., Monsma, F. J., and Gustafson, E. L. (2003). The Kiss-1 receptor GPR54 is essential for the development of the murine reproductive system. Biochem. Biophys. Res. Commun. 312, 1357–1363.
García-Galiano, D., van Ingen Schenau, D., Leon, S., Krajnc-Franken, M. A., Manfredi-Lozano, M., Romero-Ruiz, A., Navarro, V. M., Gaytan, F., van Noort, P. I., Pinilla, L., Blomenröhr, M., and Tena-Sempere, M. (2012). Kisspeptin signaling is indispensable for neurokinin B, but not glutamate, stimulation of gonadotropin secretion in mice. Endocrinology 153, 316–328.
Gerhold, L. M., Rosewell, K. L., and Wise, P. M. (2005). Suppression of vasoactive intestinal polypeptide in the suprachiasmatic nucleus leads to aging-like alterations in cAMP rhythms and activation of gonadotropin-releasing hormone neurons. J. Neurosci. 25, 62–67.
Gibson, M. J., Ingraham, L., and Dobrjansky, A. (2000). Soluble factors guide gonadotropin-releasing hormone axonal targeting to the median eminence. Endocrinology 141, 3065–3071.
Glidewell-Kenney, C., Hurley, L. A., Pfaff, L., Weiss, J., Levine, J. E., and Jameson, J. L. (2007). Nonclassical estrogen receptor alpha signaling mediates negative feedback in the female mouse reproductive axis. Proc. Natl. Acad. Sci. U.S.A. 104, 8173–8177.
Glidewell-Kenney, C. A., Grove, A. M. H., Iyer, K., and Mellon, P. L. (2010). “The neurokinin 3 receptor agonist, senktide, reduces gonadotropin releasing hormone synthesis and secretion in the immortalized GT1-7 hypothalamic cell model,” in Society for Neuroscience 40th Annual Meeting, San Diego.
Goodman, R. L., Lehman, M. N., Smith, J. T., Coolen, L. M., De Oliveira, C. V., Jafarzadehshirazi, M. R., Pereira, A., Iqbal, J., Caraty, A., Ciofi, P., and Clarke, I. J. (2007). Kisspeptin neurons in the arcuate nucleus of the ewe express both dynorphin A and neurokinin B. Endocrinology 148, 5752–5760.
Gore, A. C., Windsor-Engnell, B. M., and Terasawa, E. (2004). Menopausal increases in pulsatile gonadotropin-releasing hormone release in a nonhuman primate (Macaca mulatta). Endocrinology 145, 4653–4659.
Gottsch, M. L., Cunningham, M. J., Smith, J. T., Popa, S. M., Acohido, B. V., Crowley, W. F., Seminara, S., Clifton, D. K., and Steiner, R. A. (2004). A role for kisspeptins in the regulation of gonadotropin secretion in the mouse. Endocrinology 145, 4073–4077.
Gottsch, M. L., Navarro, V. M., Zhao, Z., Glidewell-Kenney, C., Weiss, J., Jameson, J. L., Clifton, D. K., Levine, J. E., and Steiner, R. A. (2009). Regulation of Kiss1 and dynorphin gene expression in the murine brain by classical and nonclassical estrogen receptor pathways. J. Neurosci. 29, 9390–9395.
Gottsch, M. L., Popa, S. M., Lawhorn, J. K., Qiu, J., Tonsfeldt, K. J., Bosch, M. A., Kelly, M. J., Ronnekleiv, O. K., Sanz, E., Mcknight, G. S., Clifton, D. K., Palmiter, R. D., and Steiner, R. A. (2011). Molecular properties of Kiss1 neurons in the arcuate nucleus of the mouse. Endocrinology 152, 4298–4309.
Goubillon, M. L., Kaufman, J. M., and Thalabard, J. C. (1995). Hypothalamic multiunit activity and pulsatile luteinizing hormone release in the castrated male rat. Eur. J. Endocrinol. 133, 585–590.
Hall, J. E., Lavoie, H. B., Marsh, E. E., and Martin, K. A. (2000). Decrease in gonadotropin-releasing hormone (GnRH) pulse frequency with aging in postmenopausal women. J. Clin. Endocrinol. Metab. 85, 1794–1800.
Han, S. K., Gottsch, M. L., Lee, K. J., Popa, S. M., Smith, J. T., Jakawich, S. K., Clifton, D. K., Steiner, R. A., and Herbison, A. E. (2005). Activation of gonadotropin-releasing hormone neurons by kisspeptin as a neuroendocrine switch for the onset of puberty. J. Neurosci. 25, 11349–11356.
Han, S. K., Lee, K., Bhattarai, J. P., and Herbison, A. E. (2010). Gonadotrophin-releasing hormone (GnRH) exerts stimulatory effects on GnRH neurons in intact adult male and female mice. J. Neuroendocrinol. 22, 188–195.
Harris, G. C., and Levine, J. E. (2003). Pubertal acceleration of pulsatile gonadotropin-releasing hormone release in male rats as revealed by microdialysis. Endocrinology 144, 163–171.
Herbison, A. (2006). “Physiology of the GnRH neuronal network,” in Physiology of Reproduction, eds J. Neil and E. Knobil (San Diego, CA: Academic Press), 1415–1482.
Hill, J. W., Elmquist, J. K., and Elias, C. F. (2008). Hypothalamic pathways linking energy balance and reproduction. Am. J. Physiol. Endocrinol. Metab. 294, E827–E832.
Hrabovszky, E., Molnar, C. S., Sipos, M., Vida, B., Ciofi, P., Borsay, B. A., Sarkadi, L., Herczeg, L., Bloom, S. R., Ghatei, M. A., Dhillo, W. S., Kallo, I., and Liposits, Z. (2011). Sexual dimorphism of kisspeptin and neurokinin B immunoreactive neurons in the infundibular nucleus of aged men and women. Front. Endocrinol. 2:80. doi:10.3389/fendo.2011.00080
Hrabovszky, E., Shughrue, P. J., Merchenthaler, I., Hajszan, T., Carpenter, C. D., Liposits, Z., and Petersen, S. L. (2000). Detection of estrogen receptor-beta messenger ribonucleic acid and 125I-estrogen binding sites in luteinizing hormone-releasing hormone neurons of the rat brain. Endocrinology 141, 3506–3509.
Irwig, M. S., Fraley, G. S., Smith, J. T., Acohido, B. V., Popa, S. M., Cunningham, M. J., Gottsch, M. L., Clifton, D. K., and Steiner, R. A. (2004). Kisspeptin activation of gonadotropin releasing hormone neurons and regulation of Kiss-1 mRNA in the male rat. Neuroendocrinology 80, 264–272.
Jasoni, C. L., Porteous, R. W., and Herbison, A. E. (2009). Anatomical location of mature GnRH neurons corresponds with their birthdate in the developing mouse. Dev. Dyn. 238, 524–531.
Keen, K. L., Wegner, F. H., Bloom, S. R., Ghatei, M. A., and Terasawa, E. (2008). An increase in kisspeptin-54 release occurs with the pubertal increase in luteinizing hormone-releasing hormone-1 release in the stalk-median eminence of female rhesus monkeys in vivo. Endocrinology 149, 4151–4157.
Kinoshita, F., Nakai, Y., Katakami, H., and Imura, H. (1982). Suppressive effect of dynorphin-(1-13) on luteinizing hormone release in conscious castrated rats. Life Sci. 30, 1915–1919.
Kinsey-Jones, J. S., Grachev, P., Li, X. F., Lin, Y. S., Milligan, S. R., Lightman, S. L., and O’Byrne, K. T. (2012). The inhibitory effects of neurokinin B on GnRH pulse generator frequency in the female rat. Endocrinology 153, 307–315.
Krajewski, S. J., Anderson, M. J., Iles-Shih, L., Chen, K. J., Urbanski, H. F., and Rance, N. E. (2005). Morphologic evidence that neurokinin B modulates gonadotropin-releasing hormone secretion via neurokinin 3 receptors in the rat median eminence. J. Comp. Neurol. 489, 372–386.
Krajewski, S. J., Burke, M. C., Anderson, M. J., Mcmullen, N. T., and Rance, N. E. (2010). Forebrain projections of arcuate neurokinin B neurons demonstrated by anterograde tract-tracing and monosodium glutamate lesions in the rat. Neuroscience 166, 680–697.
Krajnak, K., Kashon, M. L., Rosewell, K. L., and Wise, P. M. (1998). Aging alters the rhythmic expression of vasoactive intestinal polypeptide mRNA but not arginine vasopressin mRNA in the suprachiasmatic nuclei of female rats. J. Neurosci. 18, 4767–4774.
Krsmanovic, L. Z., Mores, N., Navarro, C. E., Tomic, M., and Catt, K. J. (2001). Regulation of Ca2+-sensitive adenylyl cyclase in gonadotropin-releasing hormone neurons. Mol. Endocrinol. 15, 429–440.
Krsmanovic, L. Z., Stojilkovic, S. S., Merelli, F., Dufour, S. M., Virmani, M. A., and Catt, K. J. (1992). Calcium signaling and episodic secretion of gonadotropin-releasing hormone in hypothalamic neurons. Proc. Natl. Acad. Sci. U.S.A. 89, 8462–8466.
Lehman, M. N., Coolen, L. M., and Goodman, R. L. (2010). Minireview: kisspeptin/neurokinin B/dynorphin (KNDy) cells of the arcuate nucleus: a central node in the control of gonadotropin-releasing hormone secretion. Endocrinology 151, 3479–3489.
Levine, J. E., and Ramirez, V. D. (1982). Luteinizing hormone-releasing hormone release during the rat estrous cycle and after ovariectomy, as estimated with push-pull cannulae. Endocrinology 111, 1439–1448.
Li, X. F., Kinsey-Jones, J. S., Cheng, Y., Knox, A. M., Lin, Y., Petrou, N. A., Roseweir, A., Lightman, S. L., Milligan, S. R., Millar, R. P., and O’Byrne, K. T. (2009). Kisspeptin signalling in the hypothalamic arcuate nucleus regulates GnRH pulse generator frequency in the rat. PLoS ONE 4, e8334. doi:10.1371/journal.pone.0008334
Maeda, K., Ohkura, S., Uenoyama, Y., Wakabayashi, Y., Oka, Y., Tsukamura, H., and Okamura, H. (2010). Neurobiological mechanisms underlying GnRH pulse generation by the hypothalamus. Brain Res. 1364, 103–115.
Martinez De La Escalera, G., Choi, A. L., and Weiner, R. I. (1992). Generation and synchronization of gonadotropin-releasing hormone (GnRH) pulses: intrinsic properties of the GT1-1 GnRH neuronal cell line. Proc. Natl. Acad. Sci. U.S.A. 89, 1852–1855.
Matsuyama, S., Ohkura, S., Mogi, K., Wakabayashi, Y., Mori, Y., Tsukamura, H., Maeda, K., Ichikawa, M., and Okamura, H. (2011). Morphological evidence for direct interaction between kisspeptin and gonadotropin-releasing hormone neurons at the median eminence of the male goat: an immunoelectron microscopic study. Neuroendocrinology 94, 323–332.
Mitchell, V., Prevot, V., Jennes, L., Aubert, J. P., Croix, D., and Beauvillain, J. C. (1997). Presence of mu and kappa opioid receptor mRNAs in galanin but not in GnRH neurons in the female rat. Neuroreport 8, 3167–3172.
Moenter, S. M., Caraty, A., Locatelli, A., and Karsch, F. J. (1991). Pattern of gonadotropin-releasing hormone (GnRH) secretion leading up to ovulation in the ewe: existence of a preovulatory GnRH surge. Endocrinology 129, 1175–1182.
Moenter, S. M., Defazio, A. R., Pitts, G. R., and Nunemaker, C. S. (2003). Mechanisms underlying episodic gonadotropin-releasing hormone secretion. Front. Neuroendocrinol. 24, 79–93.
Navarro, V. M., Castellano, J. M., Mcconkey, S. M., Pineda, R., Ruiz-Pino, F., Pinilla, L., Clifton, D. K., Tena-Sempere, M., and Steiner, R. A. (2011a). Interactions between kisspeptin and neurokinin B in the control of GnRH secretion in the female rat. Am. J. Physiol. Endocrinol. Metab. 300, E202–E210.
Navarro, V. M., Gottsch, M. L., Wu, M., Garcia-Galiano, D., Hobbs, S. J., Bosch, M. A., Pinilla, L., Clifton, D. K., Dearth, A., Ronnekleiv, O. K., Braun, R. E., Palmiter, R. D., Tena-Sempere, M., Alreja, M., and Steiner, R. A. (2011b). Regulation of NKB pathways and their roles in the control of Kiss1 neurons in the arcuate nucleus of the male mouse. Endocrinology 152, 4265–4275.
Navarro, V. M., Gottsch, M. L., Chavkin, C., Okamura, H., Clifton, D. K., and Steiner, R. A. (2009). Regulation of gonadotropin-releasing hormone secretion by kisspeptin/dynorphin/neurokinin B neurons in the arcuate nucleus of the mouse. J. Neurosci. 29, 11859–11866.
Navarro, V. M., Ruiz-Pino, F., Sanchez-Garrido, M. A., Garcia-Galiano, D., Hobbs, S. J., Manfredi-Lozano, M., León, S., Sangiao-Alvarellos, S., Castellano, J. M., Clifton, D. K., Pinilla, L., Steiner, R. A., and Tena-Sempere, M. (2012). Role of neurokinin B in the control of female puberty and its modulation by metabolic status. J. Neurosci. 32, 2388–2397.
Navarro, V. M., and Tena-Sempere, M. (2011). Neuroendocrine control by kisspeptins: role in metabolic regulation of fertility. Nat. Rev. Endocrinol. 8, 40–53.
Ohkura, S., Takase, K., Matsuyama, S., Mogi, K., Ichimaru, T., Wakabayashi, Y., Uenoyama, Y., Mori, Y., Steiner, R. A., Tsukamura, H., Maeda, K. I., and Okamura, H. (2009). Gonadotrophin-releasing hormone pulse generator activity in the hypothalamus of the goat. J. Neuroendocrinol. 21, 813–821.
Pantaleo, N., Chadwick, W., Park, S. S., Wang, L., Zhou, Y., Martin, B., and Maudsley, S. (2010). The mammalian tachykinin ligand-receptor system: an emerging target for central neurological disorders. CNS Neurol. Disord. Drug Targets 9, 627–635.
Plant, T. M., Ramaswamy, S., and Dipietro, M. J. (2006). Repetitive activation of hypothalamic G protein-coupled receptor 54 with intravenous pulses of kisspeptin in the juvenile monkey (Macaca mulatta) elicits a sustained train of gonadotropin-releasing hormone discharges. Endocrinology 147, 1007–1013.
Pohl, C. R., Richardson, D. W., Hutchison, J. S., Germak, J. A., and Knobil, E. (1983). Hypophysiotropic signal frequency and the functioning of the pituitary-ovarian system in the rhesus monkey. Endocrinology 112, 2076–2080.
Purnelle, G., Gerard, A., Czajkowski, V., and Bourguignon, J. P. (1997). Pulsatile secretion of gonadotropin-releasing hormone by rat hypothalamic explants without cell bodies of GnRH neurons [corrected]. Neuroendocrinology 66, 305–312.
Ramaswamy, S., Seminara, S. B., Ali, B., Ciofi, P., Amin, N. A., and Plant, T. M. (2010). Neurokinin B stimulates GnRH release in the male monkey (Macaca mulatta) and is colocalized with kisspeptin in the arcuate nucleus. Endocrinology 151, 4494–4503.
Ramaswamy, S., Seminara, S. B., and Plant, T. M. (2011). Evidence from the agonadal juvenile male rhesus monkey (Macaca mulatta) for the view that the action of neurokinin B to trigger gonadotropin-releasing hormone release is upstream from the kisspeptin receptor. Neuroendocrinology 94, 237–245.
Redmond, J. S., Baez-Sandoval, G. M., Spell, K. M., Spencer, T. E., Lents, C. A., Williams, G. L., and Amstalden, M. (2011). Developmental changes in hypothalamic Kiss1 expression during activation of the pulsatile release of luteinising hormone in maturing Ewe lambs. J. Neuroendocrinol. 23, 815–822.
Rometo, A. M., Krajewski, S. J., Voytko, M. L., and Rance, N. E. (2007). Hypertrophy and increased kisspeptin gene expression in the hypothalamic infundibular nucleus of postmenopausal women and ovariectomized monkeys. J. Clin. Endocrinol. Metab. 92, 2744–2750.
Sandoval-Guzman, T., and Rance, N. E. (2004). Central injection of senktide, an NK3 receptor agonist, or neuropeptide Y inhibits LH secretion and induces different patterns of Fos expression in the rat hypothalamus. Brain Res. 1026, 307–312.
Sannella, M. I., and Petersen, S. L. (1997). Dual label in situ hybridization studies provide evidence that luteinizing hormone-releasing hormone neurons do not synthesize messenger ribonucleic acid for mu, kappa, or delta opiate receptors. Endocrinology 138, 1667–1672.
Schoffelmeer, A. N., Rice, K. C., Jacobson, A. E., Van Gelderen, J. G., Hogenboom, F., Heijna, M. H., and Mulder, A. H. (1988). Mu-, delta- and kappa-opioid receptor-mediated inhibition of neurotransmitter release and adenylate cyclase activity in rat brain slices: studies with fentanyl isothiocyanate. Eur. J. Pharmacol. 154, 169–178.
Schulz, R., Wilhelm, A., Pirke, K. M., Gramsch, C., and Herz, A. (1981). Beta-endorphin and dynorphin control serum luteinizing hormone level in immature female rats. Nature 294, 757–759.
Schwanzel-Fukuda, M., and Pfaff, D. W. (1989). Origin of luteinizing hormone-releasing hormone neurons. Nature 338, 161–164.
Seminara, S. B., Messager, S., Chatzidaki, E. E., Thresher, R. R., Acierno, J. S. Jr., Shagoury, J. K., Bo-Abbas, Y., Kuohung, W., Schwinof, K. M., Hendrick, A. G., Zahn, D., Dixon, J., Kaiser, U. B., Slaugenhaupt, S. A., Gusella, J. F., O’Rahilly, S., Carlton, M. B., Crowley, W. F. Jr., Aparicio, S. A., and Colledge, W. H. (2003). The GPR54 gene as a regulator of puberty. N. Engl. J. Med. 349, 1614–1627.
Shughrue, P. J., Lane, M. V., and Merchenthaler, I. (1996). In situ hybridization analysis of the distribution of neurokinin-3 mRNA in the rat central nervous system. J. Comp. Neurol. 372, 395–414.
Smith, J. T., Cunningham, M. J., Rissman, E. F., Clifton, D. K., and Steiner, R. A. (2005a). Regulation of Kiss1 gene expression in the brain of the female mouse. Endocrinology 146, 3686–3692.
Smith, J. T., Dungan, H. M., Stoll, E. A., Gottsch, M. L., Braun, R. E., Eacker, S. M., Clifton, D. K., and Steiner, R. A. (2005b). Differential regulation of Kiss-1 mRNA expression by sex steroids in the brain of the male mouse. Endocrinology 146, 2976–2984.
Terasawa, E., Schanhofer, W. K., Keen, K. L., and Luchansky, L. (1999). Intracellular Ca(2+) oscillations in luteinizing hormone-releasing hormone neurons derived from the embryonic olfactory placode of the rhesus monkey. J. Neurosci. 19, 5898–5909.
Thompson, E. L., Patterson, M., Murphy, K. G., Smith, K. L., Dhillo, W. S., Todd, J. F., Ghatei, M. A., and Bloom, S. R. (2004). Central and peripheral administration of kisspeptin-10 stimulates the hypothalamic-pituitary-gonadal axis. J. Neuroendocrinol. 16, 850–858.
Tilbrook, A. J., and Clarke, I. J. (2001). Negative feedback regulation of the secretion and actions of gonadotropin-releasing hormone in males. Biol. Reprod. 64, 735–742.
Todman, M. G., Han, S. K., and Herbison, A. E. (2005). Profiling neurotransmitter receptor expression in mouse gonadotropin-releasing hormone neurons using green fluorescent protein-promoter transgenics and microarrays. Neuroscience 132, 703–712.
Topaloglu, A. K., Reimann, F., Guclu, M., Yalin, A. S., Kotan, L. D., Porter, K. M., Serin, A., Mungan, N. O., Cook, J. R., Ozbek, M. N., Imamoglu, S., Akalin, N. S., Yuksel, B., O’Rahilly, S., and Semple, R. K. (2009). TAC3 and TACR3 mutations in familial hypogonadotropic hypogonadism reveal a key role for Neurokinin B in the central control of reproduction. Nat. Genet. 41, 354–358.
Uenoyama, Y., Inoue, N., Pheng, V., Homma, T., Takase, K., Yamada, S., Ajiki, K., Ichikawa, M., Okamura, H., Maeda, K. I., and Tsukamura, H. (2011). Ultrastructural evidence of kisspeptin-gonadotropin-releasing hormone interaction in the median eminence of female rats: implication of axo-axonal regulation of GnRH release. J. Neuroendocrinol. 23, 863–870.
Wakabayashi, Y., Nakada, T., Murata, K., Ohkura, S., Mogi, K., Navarro, V. M., Clifton, D. K., Mori, Y., Tsukamura, H., Maeda, K., Steiner, R. A., and Okamura, H. (2010). Neurokinin B and dynorphin A in kisspeptin neurons of the arcuate nucleus participate in generation of periodic oscillation of neural activity driving pulsatile gonadotropin-releasing hormone secretion in the goat. J. Neurosci. 30, 3124–3132.
Warden, M. K., and Young, W. S. III. (1988). Distribution of cells containing mRNAs encoding substance P and neurokinin B in the rat central nervous system. J. Comp. Neurol. 272, 90–113.
Woller, M. J., Tannenbaum, P. L., Schultz-Darken, N. J., Eshelman, B. D., and Abbott, D. H. (2010). Pulsatile gonadotropin-releasing hormone release from hypothalamic explants of male marmoset monkeys compared with male rats. Am. J. Physiol. Regul. Integr. Comp. Physiol. 298, R70–R78.
Wray, S., Grant, P., and Gainer, H. (1989). Evidence that cells expressing luteinizing hormone-releasing hormone mRNA in the mouse are derived from progenitor cells in the olfactory placode. Proc. Natl. Acad. Sci. U.S.A. 86, 8132–8136.
Yang, J. J., Caligioni, C. S., Chan, Y. M., and Seminara, S. B. (2012). Uncovering novel reproductive defects in neurokinin b receptor null mice: closing the gap between mice and men. Endocrinology 153, 1498–1508.
Keywords: GnRH, kisspeptin, neurokinin B, estradiol, KNDy
Citation: Navarro VM (2012) New insights into the control of pulsatile GnRH release: the role of Kiss1/neurokinin B neurons. Front. Endocrin. 3:48. doi: 10.3389/fendo.2012.00048
Received: 26 October 2011; Accepted: 12 March 2012;
Published online: 02 April 2012.
Edited by:
Henryk Urbanski, Oregon National Primate Research Center, USAReviewed by:
Yoshitaka Oka, University of Tokyo, JapanErik Hrabovszky, Hungarian Academy of Sciences, Hungary
Copyright: © 2012 Navarro. This is an open-access article distributed under the terms of the Creative Commons Attribution Non Commercial License, which permits non-commercial use, distribution, and reproduction in other forums, provided the original authors and source are credited.
*Correspondence: Víctor M. Navarro, Division of Endocrinology, Diabetes and Hypertension, Brigham and Women’s Hospital and Harvard Medical School, Boston, MA 02115, USA. e-mail:bmFsb3ZpY0BnbWFpbC5jb20=