- 1Institute for Experimental Medical Research, Oslo University Hospital and University of Oslo, Oslo, Norway
- 2KG Jebsen Center for Cardiac Research, Oslo, Norway
- 3Department of Pathology, School of Medicine, University of Mississippi Medical Center, Jackson, MS, United States
- 4Department of Medical, Surgical, Neurological, Metabolic and Geriatrics Sciences, University of Campania “L. Vanvitelli”, Caserta, Italy
- 5Bjørknes College, Oslo, Norway
- 6Faculty of Sciences, Department of Chemistry and Biochemistry, Lebanese University, Hadath, Lebanon
- 7Department of Ophthalmology, School of Medicine, University of Mississippi Medical Center, Jackson, MS, United States
- 8Department of Pharmacology and Toxicology, School of Medicine, University of Mississippi Medical Center, Jackson, MS, United States
Heart failure with preserved ejection fraction (HFpEF) is a major unmet medical need that is characterized by the presence of multiple cardiovascular and non-cardiovascular comorbidities. Foremost among these comorbidities are obesity and diabetes, which are not only risk factors for the development of HFpEF, but worsen symptoms and outcome. Coronary microvascular inflammation with endothelial dysfunction is a common denominator among HFpEF, obesity, and diabetes that likely explains at least in part the etiology of HFpEF and its synergistic relationship with obesity and diabetes. Thus, pharmacological strategies to supplement nitric oxide and subsequent cyclic guanosine monophosphate (cGMP)—protein kinase G (PKG) signaling may have therapeutic promise. Other potential approaches include exercise and lifestyle modifications, as well as targeting endothelial cell mineralocorticoid receptors, non-coding RNAs, sodium glucose transporter 2 inhibitors, and enhancers of natriuretic peptide protective NO-independent cGMP-initiated and alternative signaling, such as LCZ696 and phosphodiesterase-9 inhibitors. Additionally, understanding the role of adipokines in HFpEF may lead to new treatments. Identifying novel drug targets based on the shared underlying microvascular disease process may improve the quality of life and lifespan of those afflicted with both HFpEF and obesity or diabetes, or even prevent its occurrence.
Introduction
Heart failure (HF) is a major public health problem on a global scale. Historically, HF was believed to originate from long standing systolic dysfunction, as assessed by reduced ejection fraction (HFrEF), and much progress has been made in the last several decades in slowing the inevitably fatal progression of this condition with drugs and in some cases implantable devices (1–5). However, nearly as many individuals are now recognized to exhibit signs of HF, namely dyspnea, fatigue, fluid retention, and exercise intolerance, but yet have a normal or near normal ejection fraction (6–11). This condition of HF with preserved ejection fraction (HFpEF) is thought to be more common in women and more prevalent in the elderly, with similar mortality rates as HFrEF (12–15). HFpEF is documented as the leading cause of hospital admission in patients over 65 years of age and is predicted to be the leading cause of HF within a decade (16, 17). Notably, HFpEF is a leading cause of pulmonary hypertension (HTN) (18).
Diastolic dysfunction or impaired relaxation of the left ventricle (LV) is the common clinical condition of HFpEF and is attributable to both cardiac fibrosis and myofilament stiffness (19, 20). Contrary to expectations, recent clinical studies have failed to demonstrate the benefits offered by drugs effective in HFrEF to HFpEF patients (16, 21–23). Thus, HFpEF is one of the largest unmet needs in cardiovascular medicine, and there is a substantial requirement for new therapeutic approaches and strategies that target mechanisms specific for HFpEF (16). A general feature in HFpEF patients is the presence of several comorbidities (Figure 1) including HTN, anemia, atrial fibrillation (AF), obesity, and diabetes (7, 14, 16, 24–30). Moreover, comorbidities negatively affect prognosis to a greater extent in individuals with HFpEF than with HFrEF and have a greater impact on physical impairment as well (31). These observations support the proposition that aggressively targeting comorbidities may prove a more efficacious approach in the clinical management of HFpEF (32–34).
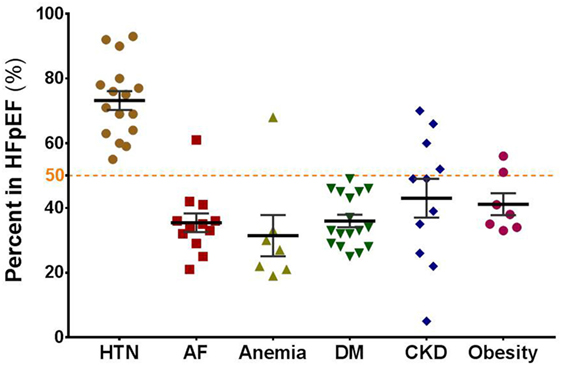
Figure 1. Major comorbidities that negatively affect prognosis in patients with HFpEF. The graph shows the prevalence of comorbidities (in percent) in HFpEF patients enrolled in different clinical studies as summarized by Triposkiadis et al. (35): hypertension (HTN), atrial fibrillation (AF), anemia, diabetes mellitus or type II diabetes (DM), chronic kidney disease (CKD), obesity.
Approximately 50% of patients with HFpEF are obese (35), and HFpEF patients with an increased body mass index (BMI) ≥35 kg/m2 are at an increased risk of an adverse outcome (death or cardiovascular hospitalization), independent of other key prognostic variables (36). Obesity is an identified risk factor for HFpEF (28, 37, 38). In a recent study on patients with HFpEF, Dalos et al. (39) found that one-third of patients over a 2-year follow-up reached the combined endpoint of HF hospitalization or cardiac death, which confirms the adverse prognosis of HFpEF. NYHA class III or IV was a strong independent predictor of outcome, along with N-terminal pro-brain natriuretic peptide (NT-proBNP). Correlates of worse NYHA class included NT-proBNP, age, increased values for diastolic dysfunction, and diastolic pulmonary artery pressure. The most novel finding was that BMI was strongly associated with worse NYHA class. The investigators also concluded that a critical contributor to symptoms of breathlessness in patients with HFpEF is increased BMI. Obesity is likely more than a prominent comorbidity for HFpEF and critically involved in its pathogenesis. Increased adiposity promotes HTN, systemic inflammation, insulin resistance, and dyslipidemia, all of which are commonly observed in patients with HFpEF (40). Obesity also impairs cardiac, vascular, and skeletal muscle function (41, 42). Adipose tissue is metabolically active and produces inflammatory cytokines or adipokines, and a number of cardiovascular active substances. Growing evidence reveals that obesity-related microvascular dysfunction, which affects all organs, contributes to exercise-intolerance, and predisposes to the development of microvascular dementia, coronary microvascular angina, chronic obstructive pulmonary disease, pulmonary HTN, and chronic kidney disease (43).
Obesity and diabetes are present in HFpEF patients with a similar proportion (35, 44). In the absence of coronary artery disease and HTN, maladaptive cardiac remodeling associated with diabetes is properly referred to as diabetic cardiomyopathy (35, 45, 46). Accumulating evidence supports the notion that there are two distinct HF phenotypes associated with diabetic cardiomyopathy. Type 1 diabetes leads to HFrEF with a dilated left ventricular phenotype. In contrast, type 2 diabetes, which is a common outcome of obesity, is associated with HFpEF and concentric remodeling of the LV. Seferović and Paulus recently presented evidence attributing the etiology of the two phenotypes to the differential principal involvement of either microvascular endothelial cells (HFpEF) or cardiac myocytes (HFrEF) in the remodeling process (45). An ancillary study of the RELAX (Phosphodiesterase-5 Inhibition to Improve Clinical Status and Exercise Capacity in Diastolic Heart Failure) trial indicated that compared to non-diabetic HFpEF patients, those with diabetes were younger, more obese and more often male, with a higher prevalence of renal dysfunction, HTN, pulmonary disease, and vascular disease (47). Analysis of the I-Preserve [Irbesartan in heart failure with preserved ejection fraction (HFpEF)] trial showed that HFpEF patients with diabetes had more signs of congestion, worse quality of life, and a poorer prognosis with a higher risk of cardiovascular mortality and hospitalization (48). On the basis of 11 clinical features, HFpEF patients who were enrolled in the I-Preserve or CHARM-Preserved (effects of candesartan in patients with chronic HF and preserved left-ventricular ejection fraction) trials were found to fall into one of six subgroups; patients with obesity and or diabetes constituted a distinctive subgroup with (along with another subgroup characterized by advanced age) the worst event-free survival (49).
The goal of our review is to highlight developments in our understanding of obesity- and diabetes-related HFpEF achieved in the last five years. Given the broad magnitude, multifaceted, and syndrome-like nature of the problem, this review is not intended to provide a comprehensive overview of obesity or diabetes and HFpEF. For instance, we do not discuss molecular signaling pathways in cardiac myocytes that are linked to hypertrophy, likely downstream of the initiating stress event (50), or that cause stiffness of myofilaments (51). We do not discuss signaling events in cardiac fibroblasts involved in collagen synthesis or turnover and fibrosis (52); nor do we deal with the importance of skeletal muscle abnormalities in HFpEF (53). Rather, we have chosen to focus on microvascular endothelial dysfunction, based on the compelling evidence that HFpEF is a manifestation of systemic vascular inflammation (54), before discussing potential pharmacological approaches (Table 1).
Role of Coronary Microvascular Inflammation
Microvascular disease appears to be a common feature of obesity, type 2 diabetes, and HFpEF. It is now recognized that obesity is associated with chronic, low-grade systemic vascular inflammation that encompasses the coronary microvasculature and entails impaired angiogenesis, microvascular rarefaction, as well as endothelial dysfunction and impaired vasodilation due to reduced endothelial nitric oxide synthase (eNOS) activity (55–60). Increased circulating levels of adipokines and cytokines contribute to the inflammatory state (57, 59–61). Similarly, both macro- and microvascular derangements are prominent in patients with type 2 diabetes (62, 63), encompassing as well inflammation, endothelial dysfunction, hypercoagubility, functional disruption of the endothelium, rarefaction, and impaired angiogenesis. Also, individuals with type 2 diabetes mellitus suffer from a higher incidence of coronary heart disease as observed in obese patients (64–66).
Coronary microvascular inflammation is now postulated to play the key role in HFpEF progression, encompassing endothelial dysfunction and impaired nitric oxide (NO)-cyclic guanosine monophosphate (cGMP)—protein kinase G (PKG) signaling and increased collagen deposition (Figure 2) (54). Increased stiffness of both myofilaments and extracellular matrix is thought to impair diastolic function (15, 54, 67). The former is postulated to result from reduced PKG-mediated phosphorylation of titin (20, 54, 67), the protein that determines passive elasticity of cardiomyocytes, and the latter from increased collagen deposition and cross-linking (fibrosis) due to inflammatory endothelium-mediated recruitment of immune cells that activate resident cardiac fibroblasts (15, 20, 67, 68). Diastolic dysfunction is likely an antecedent event that interacts synergistically with other remodeling events at the cellular level to foster development of HFpEF. Recently, levels of inflammatory cells in endomyocardial biopsy samples from HFpEF patients were found to positively correlate with diastolic dysfunction (69) and coronary microvascular dysfunction was detected by angiography in patients with HFpEF (70). Further support for the involvement of myocardial microvascular inflammatory endothelial activation in the etiology of HFpEF comes from a study by Franssen et al. (71). These investigators reported that the myocardium of both HFpEF patients and an obesity-diabetic rat model of HFpEF showed upregulation of endothelial adhesion molecules, elevated expression of the pro-oxidant protein NOX2 in macrophages and endothelial cells but not cardiomyocytes, evidence of the uncoupling of eNOS, and reduced myocardial nitrite/nitrate concentration, cGMP content, and PKG activity.
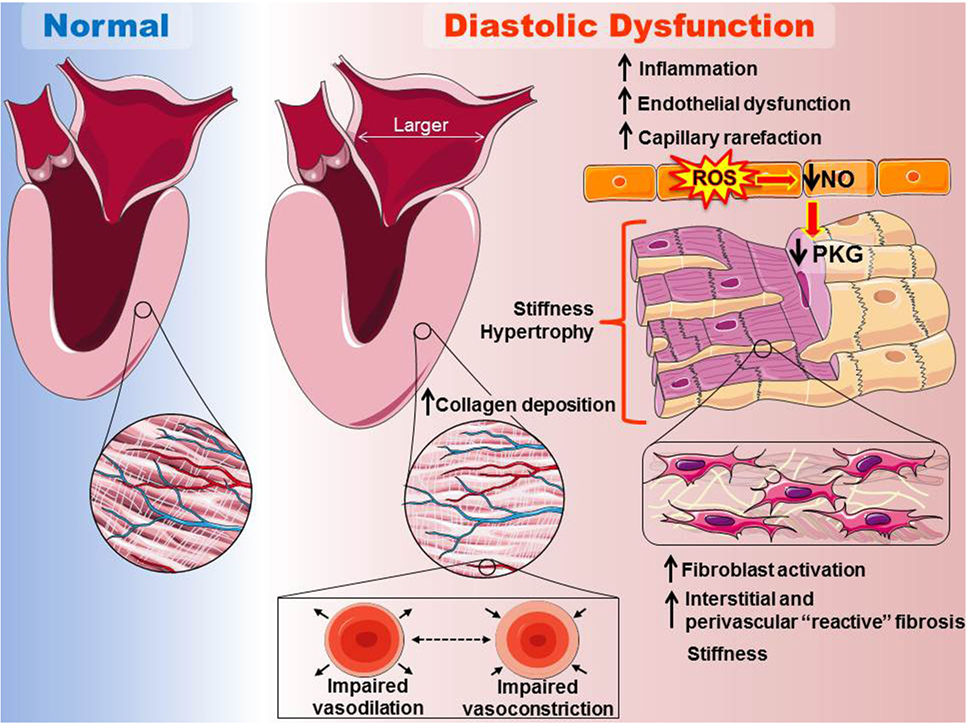
Figure 2. Scheme for the proposed etiology of heart failure with preserved ejection fraction (HFpEF) relevant to obesity and type 2 diabetes. Left side: at the organ level, HFpEF is characterized by cardiac hypertrophy and a marked increase in the left ventricular mass/volume ratio (concentric remodeling), as well as increased stiffness and often enlargement of the left atrium. Right panel: coronary microvascular inflammation is postulated to play a key role in HFpEF progression, encompassing endothelial dysfunction and reduced nitric oxide (NO)-cyclic guanosine monophosphate (cGMP)—protein kinase G (PKG) signaling. Increased stiffness of both myofilaments and the extracellular matrix is thought to impair diastolic function of the heart. The former is postulated to result in part from reduced PKG-mediated phosphorylation of titin, the protein that determines passive elasticity of cardiomyocytes. The later would result from increased collagen deposition and cross-linking (fibrosis), due to loss of cGMP/PKG anti-fibrotic signaling and increased inflammatory endothelium-mediated recruitment of immune cells that activate resident cardiac fibroblasts. Diastolic dysfunction is likely an antecedent event that interacts synergistically with other remodeling events at the cellular level to foster development of HFpEF (images adapted and reproduced with permission from the copyright holder http://servier.com/Powerpoint-image-bank).
Involvement of microvascular inflammation in HFpEF with the associated reduction in eNOS-mediated NO generation raises the possibility that enhancing cGMP-PKG signaling could be an efficacious therapeutic approach (46, 72). Potentially, this could be achieved with nitroxyl (HNO), the 1 electron-reduced congener of NO that has myocardial antihypertrophic and superoxide suppressing activity (73, 74), as well as anti-inflammatory actions on microvascular endothelial cell (75). Nitroxyl was also recently shown to inhibit TNF-induced endothelial cell and monocyte activation, as well as leukocyte adhesion to the endothelium, in isolated mouse aorta (76). Nitroxyl increases vasorelaxation and enhances cardiac contractility with positive inotropic and lusitropic effects due to a direct effect on cardiac myofilament proteins and enhancement of SERCA2a activity (77, 78). Nitroxyl may also substitute for NO in activating soluble guanylate cyclase (sGC) and increasing cGMP (79). Recently, chronic treatment with the nitroxyl donor 1-nitrosocyclohexyl acetate was found to attenuate left ventricular diastolic dysfunction in a mouse model of diabetes (80). Others have recently reported evidence indicating that inorganic nitrates and nitrites, which can be converted to NO in the body, are effective in alleviating some HFpEF symptoms (81–84). Lastly, both cardiac myocytes and endothelial cells express the third isotype of beta adrenergic receptors (β3 ARs), which couple to eNOS activation and anti-oxidant signaling (85, 86). Pre-clinical evidence suggests that β3 AR agonists, such as mirabegron, confer protection against diabetes-induced vascular dysfunction and may prove beneficial in HFpEF (85–88).
Potential Targets or Approaches
Exercise and Lifestyle Modifications
Preclinical studies have demonstrated beneficial effects of exercise training to protect the heart in obese or diabetic animals. For instance, exercise was reported to protect the hearts of obese diabetic mice from ischemia-reperfusion injury (89) and to reverse cardiac microvascular rarefaction and impaired endothelium-dependent microvascular reactivity in obese diabetic rats (90). In patients with type 2 diabetes, exercise training was reported to improve brachial artery endothelial function (91), as well as to attenuate capillary rarefaction and improve microvascular vasodilator and insulin signaling (92). In contrast, Schreuder et al. (93) did not find any improvement in endothelial function after 8 weeks of training in type 2 diabetes patients. Although a reasonable supposition, there is insufficient data to assess whether dietary and lifestyle changes offer real promise to human sufferers of HFpEF. After all, exercise intolerance is a dominant symptom of HFpEF that contributes in a major way to reduced quality of life in these patients; plus, diabetes has the associated confounding factor of myocardial metabolic inflexibility (45). In a meta-analysis of randomized control trials, physical exercise was found to improve peak oxygen uptake and quality of life in HFpEF patients; however, no significant changes in LV systolic and diastolic function were noted (94). In older adults with type 2 diabetes and chronic renal insufficiency, a moderate protein diet showed long-term effects on low-grade inflammation, and oxidative stress (95), while in elderly HFpEF patients, exercise improved peak exercise oxygen consumption, although endothelial function or arterial stiffness were not altered (96). Among obese older patients with clinically stable HFpEF, caloric restriction or aerobic exercise training increased peak oxygen consumption, and the effects appeared to be additive (97); however, neither intervention had a significant effect on quality of life as assessed by the Minnesota Living with Heart Failure Questionnaire, suggesting that the patients may still have exhibited exertional dyspnea. In any event, no improvements in cardiac function were noted and improvements in peak exercise oxygen consumption were likely due to non-cardiac peripheral adaptations (97, 98). Indeed, sarcopenic obesity may be a significant contributor to exercise intolerance in elderly HFpEF patients (99). Sustained and substantial weight loss via bariatric surgery, which was shown effective in improving left ventricular relaxation and reversing concentric LV remodeling and hypertrophy, might be considered to treat obesity-associated HFpEF in younger individuals; however, the long-term cardiovascular effects of this surgery in obese HFpEF patients would need to be assessed (33, 100).
In any case, acute exercise may serve as an important tool for detecting coronary microvascular dysfunction, which becomes more apparent when the heart is challenged in this manner (101). Additionally, exercise would cause the release of a number of hormones or cytokines in HFpEF patients that might impact on cardiac or microvascular function, an area of research that requires further exploration. Recently, exercise training was reported to increase ghrelin levels in patients with HFpEF, especially in patients with higher baseline adiponectin (102). Ghrelin is a gastric hormone that simulates appetite and is associated with weight gain. However, ghrelin was also reported to decrease blood pressure and increase cardiac output in health men (103) and to inhibit apoptosis of cardiomyocytes and endothelial cells in vitro (104). Levels of ghrelin are reduced in both obesity and type 2 diabetes (105). Irisin is a novel hormone (myokine) secreted by cardiac and skeletal myocytes in response to exercise that may regulate metabolism and limit weight gain, although its precise role is controversial (106, 107). Circulating levels of irisin are reported to be reduced or increased in obese subjects, but reduced in type 2 diabetic patients (106, 108, 109). Lower levels of irisin are associated with endothelial dysfunction (109, 110). Recently, irisin was found to improve endothelial function in obese mice via the activating 5′ adenosine monophosphate-activated protein kinase (AMPK)-eNOS pathway (110); in the spontaneously hypertensive rat, irisin-induced improvement in endothelial function, reduced blood pressure (111).
Endothelial Cell Mineralocorticoid Receptors Antagonism
Higher circulating aldosterone levels are observed in obesity (112) and type 2 diabetes (113). Moreover, aldosterone antagonism has proven effective in the clinical management of HFrEF (114, 115) and in attenuating cardiac dysfunction and maladaptive remodeling in pre-clinical animal models of obesity-associated HFpEF (116, 117). Surprisingly, the Treatment of Preserved Cardiac Function Heart Failure with an Aldosterone Antagonist (TOPCAT) study, a large randomized, double-blind clinical trial of spironolactone versus placebo in patients with symptomatic HFpEF, did not achieve a significant reduction in the primary composite outcome of time to cardiovascular death from cardiovascular causes, aborted cardiac arrest, or hospitalization for management of HF; however, TOPCAT did demonstrate that spironolactone decreases HF hospitalizations in HFpEF patients (118). Use of spironolactone for HFpEF was associated with an improvement in HF-specific health-related quality of life (119) and, in a separate study, improved exercise tolerance (120). Actually, the beneficial effects of spironolactone in HFpEF may be more significant. Subgroup analysis of TOPCAT by geographic region raised concerns about patient selection and dosing levels in the Russia/Georgia arm of the trial, whereas spironolactone was clearly superior to placebo in reducing cardiovascular events in the Americas (121). Also, spironolactone may have greater potential efficacy in HFpEF patients with lower ejection fraction (122) and, somewhat at odds with this, with lower levels of circulating natriuretic peptides and overall risk (123).
An endothelial-cell targeted strategy may optimize the beneficial actions of aldosterone antagonism in HFpEF. Based on accumulating evidence, Davel et al. recently proposed that in normal physiology, the endothelial mineralocorticoid receptor is vasoprotective; however, in the presence of cardiovascular risk factors, such as obesity and diabetes, endothelial mineralocorticoid receptor activation leads to endothelial dysfunction as a result of reduced eNOS activity and NO production, increased oxidative stress via eNOS uncoupling and NOX activation, as well as induced expression of adhesion molecules for inflammatory cells (124). Supporting this possibility is the observation that endothelial mineralocorticoid receptor deletion prevents obesity-induced diastolic dysfunction in female mice (125).
Non-Coding RNAs
MicroRNAs (miRNAs) are small non-coding RNAs (~21–25 nucleotides in length) that in animal cells generally bind to the 3′ UTR of mRNA to suppress gene expression by either transcript degradation or translational inhibition. The bloodstream contains multiple types of miRNAs in various types of vesicles and complexes, secreted from both healthy and dying cells of likely all tissues throughout the body (126). Since miRNA expression is dynamically regulated, circulating miRNAs are increasingly recognized as having potential utility for diagnostic and prognostic purposes. Recent reports have supported the diagnostic value of using circulating miRNA profiles to distinguish HF patients from non-HF controls and differentiating between HFrEF and HFpEF (127, 128). To date, miRNA profiles have not been defined for HFpEF patients on the basis of dominate comorbidity such as obesity or diabetes. However, both metabolic syndrome and type 2 diabetes are associated with altered circulating miRNA profiles (126, 129). The endothelium is a rich source of circulating miRNAs in both the healthy and disease states and the plasma mRNA profile provides an assessment of endothelial health (126). For instance, circulating and cardiac levels of pro-angiogenic miR-126 and miR-132 were found to be downregulated in type 2 diabetic individuals without any known history of cardiovascular disease (130). Decreased levels of these miRNAs were associated with cardiac microangiopathy as indicated by reduced capillaries and arterioles and increased endothelial cell apoptosis. Parallel findings in a mouse model of type 2 diabetes support the prognostic value of these “angiomiRs”. Interestingly, swimming training in rats was reported to increase cardiac miRNA-126 expression and angiogenesis (131). Optimistically, the identification of particular miRNA signature in diabetes- or obesity-associated HFpEF could lead to miRNA-based therapies that use tissue-targeted exosomes to deliver anti-miRNA or miRNA mimics to treat microvascular dysfunction. Some of the challenges in making miRNA-based therapy a reality are discussed elsewhere (132–134).
An emerging area in cardiovascular medicine is the study of long non-coding RNAs (lncRNAs), which are transcripts larger than 200 nucleotides that control gene expression at the epigenetic, transcriptional, and posttranscriptional levels (135). Single-nucleotide polymorphisms which alter the expression of the lncRNA ANRIL (antisense non-coding RNA in the INK4 locus) are associated with coronary artery disease and type 2 diabetes (126). Recently, circulating levels of three lncRNAs were identified as biomarkers of diastolic function and remodeling in patients with well-controlled type 2 diabetes (136): long intergenic non-coding RNA predicting cardiac remodeling (LIPCAR), myocardial infarction-associated transcript (MIAT), and endothelial cell-enriched migration/differentiation-associated long non-coding RNA (SENCR). Although the cellular source was not defined in this study, LIPCAR is thought to originate from cardiomyocyte mitochondria, whereas MIAT and SENCR have been implicated in endothelial cell function/dysfunction, including inflammation and angiogenesis (126, 136, 137). The role and diagnostic/prognostic value of lncRNAs in obesity or diabetes associated HFpEF awaits investigation.
Glucose Lowering Drugs
The drug metformin has proven highly effective in the treatment of type 2 diabetes and is currently recommended as first line treatment. Metformin has beneficial actions by reducing hepatic glucose production and by activating AMPK, which enhances cellular glucose uptake. AMPK activation in cardiac myocytes may also inhibit hypertrophy (138). Preclinical studies demonstrated that AMPK activation by metformin restores endothelial function and NO bioavailability by attenuating oxidative and endoplasmic reticulum stress and by directly increasing eNOS activity (139, 140). However, metformin does not seem to improve LV stiffness in type 2 diabetic patients (141).
Concerns of increased adverse cardiovascular outcomes, including HF, are associated with the use of sulfonylureas and thiazolidinediones (TZDs) in diabetic patients (45, 142, 143). The situation with regard dipeptidyl peptidase-4 inhibitors is unsettled (144). Although glucagon-like peptide-1 (GLP-1) receptor agonists, liraglutide and semaglutide, showed a reduction in cardiovascular events, GLP-1 agonists do not seem to have a significant effect on natriuretic peptide levels in HF (45, 145). Much excitement has been generated by the recent approval of selective sodium glucose transporter 2 (SGLT-2) inhibitors, including empagliflozin, to treat type 2 diabetes. SGLT-2 inhibitors lower blood glucose by blocking sodium-dependent reabsorption of glucose in the proximal tubule and causing glycosuria. However, the beneficial actions of SGLT-2 inhibitors in type 2 diabetes seem to extend beyond glycemic control and are not completely understood (146). SGLT-2 inhibitors are associated with weight loss and reductions in blood pressure (without an increase in heart rate), visceral adiposity, plasma urate levels, and arterial stiffness/vascular resistance, as well as improvements in microvascular/macrovascular endothelial function and cardiac metabolism (146). The recently published results of the EMPA-REG OUTCOME trial revealed a marked reduction in deaths from cardiovascular causes, HF hospitalizations, and deaths from any cause when empagliflozin was added to standard care of patients with type 2 diabetes (147). At present, insufficient evidence precludes reaching a definitive conclusion as to whether the beneficial effects of empagliflozin represent a class effect of SGLT-2 inhibitors (148).
Novel Approaches That Enhance Guanylyl Cyclase Systems
Nitric oxide deficiency is postulated to be responsible for diastolic dysfunction in HFpEF patients due to impaired cGMP generation and PKG activation. Because of issues such as tolerance and preload reduction, organic nitrates seem not to be useful in treating HFpEF (149). Alternative ways of cGMP enhancement might hold more promise for future therapeutic benefit. sGC stimulators are a relatively new class of drugs that act via an allosteric site on sGC to synergize with NO in producing cGMP, thereby offsetting decreased NO due to diminished NO synthase activity (150). The recently completed phase II SOluble guanylate Cyclase stimulatoR in heArT failurE Study (SOCRATES) program consisted of two parallel studies to assess the potential utility of the sGC stimulator, vericiguat for treating HFrEF (SOCRATES-REDUCED) and HFpEF (SOCRATES-PRESERVED). The respective primary endpoints were change in NT-proBNP at 12 weeks, and change in NT-proBNP and left atrial volume at 12 weeks (151). Vericiguat was well tolerated; however, likely because of inadequate dosage level, SOCRATES-REDUCED yielded mixed, yet promising results (152). The outcome of SOCRATES-PRESERVED has not been reported but likely is complicated by the same shortfall in dosing as the SOCRATES-REDUCED study.
Alternative NO-independent ways to increase cGMP formation, which is linked to anti-hypertrophy and anti-fibrosis signaling in the heart (153, 154), may prove beneficial in treating HFpEF. Specifically, receptors for natriuretic peptides activate membrane-bound particulate GC. Indeed, several studies have shown favorable cardiorenal effects, including improvement of diastolic function, of exogenous supplementation of the natriuretic peptides, which are known to stimulate cGMP production in the heart, kidney, and vasculature (155, 156). In contrast, deletion of the BNP gene is characterized by diastolic dysfunction, cardiac remodeling, and rising of elevated blood pressure (157). A recently approved drug for the treatment of chronic HF, LCZ696 (brand name entresto), combines an angiotensin II type 1 receptor blocker (valsartan) with a neprilysin inhibitor (sacubitril). Sacubitril suppresses proteolysis of natriuretic peptides that enhance cGMP signaling independent of NO (158). The phase III study Efficacy and Safety of LCZ696 Compared to Valsartan, on Morbidity and Mortality in Heart Failure Patients With Preserved Ejection Fraction (PARAGON-HF) (NCT01920711) is currently underway, while preliminary data from the PARAMOUNT study have shown a significant reduction of the circulating levels of NT-proBNP (a major prognostic biomarker in HF) after 12 weeks of treatment, and an improvement of both cardiac size and New York Heart Association (NYHA) class at 36 weeks as compared to valsartan (159). Selective inhibitors of phosphodiesterase-9 (PDE9), which hydrolyzes natriuretic peptide-coupled cGMP and is upregulated in HFpEF, are another potential way to increase cardiac cGMP levels (160).
Inadequate processing and activation of natriuretic peptides appears to be a signature of HTN, resulting in an impaired counter-regulatory response of the natriuretic homeostatic control system (161, 162). Notably, although natriuretic peptides are useful to stratify HFpEF patients in conjunction with the NYHA classification system, circulating levels of BNP are not elevated as much in HFpEF patients as in HFrEF (163, 164). It is now established that elevated circulating natriuretic peptides in patients with overt cardiovascular diseases, although having a significant adverse prognostic value, are constituted mainly of biologically non-active forms, while mature active forms are virtually absent in severe congestive HF patients (165). In addition, obesity has a negative impact on the elevation of circulating levels of BNP as fatty tissue expresses the clearance receptor for the natriuretic peptide (NPRC) (163, 166). Therefore, supplementation of these cardioprotective natriuretic peptides may prove to be of therapeutic importance in obesity- or diabetes-associated HFpEF. Studies report that circulating atrial natriuretic peptide (ANP) can break down into multiple peptides, each of which has distinctive actions. One of these peptides, namely proANP31–67, does not activate the cGMP pathway, but exerts a unique cardiac and renal protective response by increasing renal, as well as circulating levels of prostaglandin E2 (PGE2) (167–169). ProANP31–67 also has vasodilatory actions and induces diuresis via inhibition of the basolateral Na+–K+ ATPase of the inner medullary collecting ducts resulting in increased Na+ and renal water excretion (170, 171). Whether the potential benefits of proANP31–67 extend to HFpEF is not established, although PGE2 has protective effects on the heart via enhancement of VEGF and eNOS expression levels and anti-inflammatory actions (172, 173). Future studies are warranted to determine whether the cardiorenal protective effects and the cardiac function enhancing properties of these hormones can be explained by mechanisms different from cGMP activation.
Unresolved Issues
Adipose tissue is an endocrine organ that secretes multiple “adipokines” that have broad physiological and pathological impact throughout the body (174, 175). In obesity, the altered circulating adipokine profile contributes to systemic low-grade inflammation and the cardiovascular or obesity-related comorbidities defining the metabolic syndrome. Understanding the contribution of a particular adipokine to the disease process is a challenging task as the inflammatory milieu is a dynamic and fluid environment of multiple players with redundant or conflicting roles. A good case in point is the role of adiponectin in HFpEF. Adiponectin is the major adipokine produced by adipose tissue with anti-inflammatory, antidiabetic, anti-apoptotic, and anti-atherogenic properties (174, 176). Circulating adiponectin levels are decreased in obesity and type 2 diabetes and downregulation of adiponectin and its receptors is associated with insulin resistance and diabetes, as well as increased risk of HTN and coronary artery disease (174, 176). Animal studies have shown that adiponectin can inhibit cardiac hypertrophy and fibrosis, and reduce infarct size (174). Together these findings support the supposition that adiponectin might have therapeutic potential in HFpEF patients (176). However, circulating adiponectin levels are increased in both HFrEF and HFpEF (177). Furthermore, multiple studies have shown an association between higher adiponectin levels and increased mortality and cardiovascular disease mortality/morbidity in diverse populations (178). One confounding factor is that natriuretic peptides, which are elevated in HF due to hemodynamic stress and/or neurohormonal activation, may directly enhance adiponectin expression (178). Certainly, the question of which-time-point in the development and progression of HFpEF is an important consideration. Sex differences may play a role as well. Low adiponectin was associated with higher odds of indices of diastolic dysfunction in women, but lower odds in men, and lower adiponectin was associated with increased left ventricular mass only in women (179). Other variables that may come into play are adiponectin receptor desensitization, receptor subtypes, and the different-size molecular weight complexes of circulating adiponectin (“isoforms”) (176).
Perspectives and Future Directions
The role of sex as well as race in HFpEF, especially their interaction with comorbidities, is an evolving area of investigation. Early studies reported that HFpEF was more common among women than men (180, 181). Recently, the largest sex- and race-based subgroup analysis of HFpEF was published, involving data gathered from 1,889,608 hospitalizations (182). The study reported several noteworthy findings, including the following: (a) men with HFpEF were slightly younger than women with HFpEF and had a higher burden of comorbidities; (b) blacks with HFpEF were younger than whites with HFpEF, with lower rates of most comorbidities; (c) HTN, anemia, chronic renal failure, and diabetes, were more common among blacks; (d) AF was an important correlate of mortality only among women and blacks; and (e) with women, chronic pulmonary disease, and diabetes were more common among younger patients, but more common among older patients in men. Obviously, the influence of sex and race in the context of comorbidities to the heterogeneity of HFpEF is complicated and further study is needed. Another emerging area of interest is the additional classification according to the 2016 EC guidelines of HFmrEF, for HF patients exhibiting mid-range ejection fractions (183). The clinical profile, including comorbidities, and prognosis of patients diagnosed with HFmrEF, and the etiological and prognostic relationship of this HF phenotype to HFrEF and HFpEF needs to be addressed. The application of novel measures for assessing LV function such as strain imaging may be useful in this regard.
Obesity and diabetes are not only risk factors for the development of HFpEF but have significant impact on its symptoms and outcome. Therefore, focusing on these comorbid conditions in HFpEF might provide a novel therapeutic strategy. Coronary microvascular endothelial dysfunction with impaired NO-cGMP-PKG signaling is a shared condition that is thought to be the basis for diastolic stiffness, inflammation, oxidative stress, and maladaptive cardiac remodeling. Pharmacological approaches that target this signaling axis offer promise in treating or preventing HFpEF. This would include: (a) NO replenishment (inorganic nitrates/nitrites), replacement (nitroxyl donors), or enhanced generation (β3 AR agonists and AMPK agonist); and (b) enhancers of NO-independent cGMP generation (LCZ696/entresto) or prevention of its breakdown (PDE9 inhibitors). A reappraisal of clinical results supports the utility of inhibiting the mineralocorticoid receptor in treating HFpEF, but additional study is warranted. In addition, given the pronounced side effects of spironolactone at higher doses, an endothelial cell-targeted approach might be judicious. miRNA and lncRNA profiling of HFpEF patients offers the promise of not only prognostic assessment and therapeutic monitoring, but personalized treatment strategies as well. A better understanding of the role of adipokines in obesity- and diabetes-associated HFpEF may open up new pharmacological avenues. Finally, SGLT-2 inhibitors offer great promise for treating or preventing HFpEF in obese and diabetic patients. A better understanding of the physiological and molecular basis for the cardiovascular protective actions of this new drug class should foster the development of even more effective compounds.
Author Contributions
All authors contributed conceptually to the manuscript. All authors authored sections of the manuscript, contributed to figure design, and approved the final version. All appropriate permissions have been obtained from the copyright holders of any work that has been reproduced in this manuscript.
Conflict of Interest Statement
The authors declare that the research was conducted in the absence of any commercial or financial relationships that could be construed as a potential conflict of interest.
Funding
RA is supported by the South-Eastern Norway Regional Health Authority (HSØ-RHF), project #2016089. MK acknowledges the generous support of the Lebanese University (MK-4-2017) and Project Cedre (# 37303QA).
References
1. Marinescu KK, Uriel N, Mann DL, Burkhoff D. Left ventricular assist device-induced reverse remodeling: it’s not just about myocardial recovery. Expert Rev Med Devices (2017) 14:15–26. doi:10.1080/17434440.2017.1262762
2. Ng CY, Heist EK. Cardiac resynchronization therapy: maximizing the response to biventricular pacing. Cardiol Rev (2017) 25:6–11. doi:10.1097/CRD.0000000000000127
3. Marinescu KK, Uriel N, Adatya S. The future of mechanical circulatory support for advanced heart failure. Curr Opin Cardiol (2016) 31:321–8. doi:10.1097/HCO.0000000000000287
4. Aronow WS. Current treatment of heart failure with reduction of left ventricular ejection fraction. Expert Rev Clin Pharmacol (2016) 9:1619–31. doi:10.1080/17512433.2016.1242067
5. Greenberg B. Novel therapies for heart failure – where do they stand? Circ J (2016) 80:1882–91. doi:10.1253/circj.CJ-16-0742
6. Andersson C, Vasan RS. Epidemiology of heart failure with preserved ejection fraction. Heart Fail Clin (2014) 10:377–88. doi:10.1016/j.hfc.2014.04.003
7. Alsamara M, Alharethi R. Heart failure with preserved ejection fraction. Expert Rev Cardiovasc Ther (2014) 12:743–50. doi:10.1586/14779072.2014.911086
8. Borlaug BA. Mechanisms of exercise intolerance in heart failure with preserved ejection fraction. Circ J (2014) 78:20–32. doi:10.1253/circj.CJ-13-1103
9. Becher PM, Lindner D, Fluschnik N, Blankenberg S, Westermann D. Diagnosing heart failure with preserved ejection fraction. Expert Opin Med Diagn (2013) 7:463–74. doi:10.1517/17530059.2013.825246
10. Webb J, Jackson T, Claridge S, Sammut E, Behar J, Carr-White G. Management of heart failure with preserved ejection fraction. Practitioner (2015) 259:21–4.
11. Nativi-Nicolau J, Ryan JJ, Fang JC. Current therapeutic approach in heart failure with preserved ejection fraction. Heart Fail Clin (2014) 10:525–38. doi:10.1016/j.hfc.2014.04.007
12. Shakib S, Clark RA. Heart failure pharmacotherapy and supports in the elderly – a short review. Curr Cardiol Rev (2016) 12:180–5. doi:10.2174/1573403X12666160622102802
13. Rogers FJ, Gundala T, Ramos JE, Serajian A. Heart failure with preserved ejection fraction. J Am Osteopath Assoc (2015) 115:432–42. doi:10.7556/jaoa.2015.089
14. Upadhya B, Taffet GE, Cheng CP, Kitzman DW. Heart failure with preserved ejection fraction in the elderly: scope of the problem. J Mol Cell Cardiol (2015) 83:73–87. doi:10.1016/j.yjmcc.2015.02.025
15. Zouein FA, de Castro Bras LE, da Costa DV, Lindsey ML, Kurdi M, Booz GW. Heart failure with preserved ejection fraction: emerging drug strategies. J Cardiovasc Pharmacol (2013) 62:13–21. doi:10.1097/FJC.0b013e31829a4e61
16. Lam CS, Donal E, Kraigher-Krainer E, Vasan RS. Epidemiology and clinical course of heart failure with preserved ejection fraction. Eur J Heart Fail (2011) 13:18–28. doi:10.1093/eurjhf/hfq121
17. Liu Y, Haddad T, Dwivedi G. Heart failure with preserved ejection fraction: current understanding and emerging concepts. Curr Opin Cardiol (2013) 28:187–96. doi:10.1097/HCO.0b013e32835c5492
18. Meng Q, Lai YC, Kelly NJ, Bueno M, Baust J, Bachman T, et al. Development of a mouse model of metabolic syndrome, pulmonary hypertension, and heart failure with preserved ejection fraction (PH-HFpEF). Am J Respir Cell Mol Biol (2017) 56(4):497–505. doi:10.1165/rcmb.2016-0177OC
19. Franssen C, Gonzalez Miqueo A. The role of titin and extracellular matrix remodelling in heart failure with preserved ejection fraction. Neth Heart J (2016) 24:259–67. doi:10.1007/s12471-016-0812-z
20. Zile MR, Baicu CF, Ikonomidis JS, Stroud RE, Nietert PJ, Bradshaw AD, et al. Myocardial stiffness in patients with heart failure and a preserved ejection fraction: contributions of collagen and titin. Circulation (2015) 131:1247–59. doi:10.1161/CIRCULATIONAHA.114.013215
21. Tschope C, Lam CS. Diastolic heart failure: what we still don’t know. Looking for new concepts, diagnostic approaches, and the role of comorbidities. Herz (2012) 37:875–9. doi:10.1007/s00059-012-3719-5
22. Borlaug BA, Paulus WJ. Heart failure with preserved ejection fraction: pathophysiology, diagnosis, and treatment. Eur Heart J (2011) 32:670–9. doi:10.1093/eurheartj/ehq426
23. von Lueder TG, Krum H. New medical therapies for heart failure. Nat Rev Cardiol (2015) 12:730–40. doi:10.1038/nrcardio.2015.137
24. Sharma K, Hill T, Grams M, Daya NR, Hays AG, Fine D, et al. Outcomes and worsening renal function in patients hospitalized with heart failure with preserved ejection fraction. Am J Cardiol (2015) 116:1534–40. doi:10.1016/j.amjcard.2015.08.019
25. Upadhya B, Haykowsky MJ, Eggebeen J, Kitzman DW. Exercise intolerance in heart failure with preserved ejection fraction: more than a heart problem. J Geriatr Cardiol (2015) 12:294–304. doi:10.11909/j.issn.1671-5411.2015.03.013
26. Jeong EM, Dudley SC Jr. Diastolic dysfunction. Circ J (2015) 79:470–7. doi:10.1253/circj.CJ-15-0064
27. Taylor AL. Heart failure in women. Curr Heart Fail Rep (2015) 12:187–95. doi:10.1007/s11897-015-0252-x
28. Borlaug BA. The pathophysiology of heart failure with preserved ejection fraction. Nat Rev Cardiol (2014) 11:507–15. doi:10.1038/nrcardio.2014.83
29. Andersen MJ, Borlaug BA. Heart failure with preserved ejection fraction: current understandings and challenges. Curr Cardiol Rep (2014) 16:501. doi:10.1007/s11886-014-0501-8
30. den Ruijter H, Pasterkamp G, Rutten FH, Lam CS, Chi C, Tan KH, et al. Heart failure with preserved ejection fraction in women: the Dutch Queen of Hearts program. Neth Heart J (2015) 23:89–93. doi:10.1007/s12471-014-0613-1
31. Edelmann F, Stahrenberg R, Gelbrich G, Durstewitz K, Angermann CE, Dungen HD, et al. Contribution of comorbidities to functional impairment is higher in heart failure with preserved than with reduced ejection fraction. Clin Res Cardiol (2011) 100:755–64. doi:10.1007/s00392-011-0305-4
32. Maurer MS, Mancini D. HFpEF: is splitting into distinct phenotypes by comorbidities the pathway forward? J Am Coll Cardiol (2014) 64:550–2. doi:10.1016/j.jacc.2014.06.010
33. Samson R, Jaiswal A, Ennezat PV, Cassidy M, Le Jemtel TH. Clinical phenotypes in heart failure with preserved ejection fraction. J Am Heart Assoc (2016) 5:e002477. doi:10.1161/JAHA.115.002477
34. Lindman BR. The diabetic heart failure with preserved ejection fraction phenotype: is it real and is it worth targeting therapeutically? Circulation (2017) 135:736–40. doi:10.1161/CIRCULATIONAHA.116.025957
35. Triposkiadis F, Giamouzis G, Parissis J, Starling RC, Boudoulas H, Skoularigis J, et al. Reframing the association and significance of co-morbidities in heart failure. Eur J Heart Fail (2016) 18:744–58. doi:10.1002/ejhf.600
36. Haass M, Kitzman DW, Anand IS, Miller A, Zile MR, Massie BM, et al. Body mass index and adverse cardiovascular outcomes in heart failure patients with preserved ejection fraction: results from the irbesartan in heart failure with preserved ejection fraction (I-PRESERVE) trial. Circ Heart Fail (2011) 4:324–31. doi:10.1161/CIRCHEARTFAILURE.110.959890
37. Ul Haq MA, Wong C, Hare DL. Heart failure with preserved ejection fraction: an insight into its prevalence, predictors, and implications of early detection. Rev Cardiovasc Med (2015) 16:20–7. doi:10.3909/ricm0725
38. Melenovsky V, Borlaug BA, Rosen B, Hay I, Ferruci L, Morell CH, et al. Cardiovascular features of heart failure with preserved ejection fraction versus nonfailing hypertensive left ventricular hypertrophy in the urban Baltimore community: the role of atrial remodeling/dysfunction. J Am Coll Cardiol (2007) 49:198–207. doi:10.1016/j.jacc.2006.08.050
39. Dalos D, Mascherbauer J, Zotter-Tufaro C, Duca F, Kammerlander AA, Aschauer S, et al. Functional status, pulmonary artery pressure, and clinical outcomes in heart failure with preserved ejection fraction. J Am Coll Cardiol (2016) 68:189–99. doi:10.1016/j.jacc.2016.04.052
40. von Bibra H, Paulus W, St John Sutton M. Cardiometabolic syndrome and increased risk of heart failure. Curr Heart Fail Rep (2016) 13(5):219–29. doi:10.1007/s11897-016-0298-4
41. Kitzman DW, Shah SJ. The HFpEF obesity phenotype: the elephant in the room. J Am Coll Cardiol (2016) 68:200–3. doi:10.1016/j.jacc.2016.05.019
42. Muris DM, Houben AJ, Schram MT, Stehouwer CD. Microvascular dysfunction: an emerging pathway in the pathogenesis of obesity-related insulin resistance. Rev Endocr Metab Disord (2013) 14:29–38. doi:10.1007/s11154-012-9231-7
43. Sorop O, Olver TD, van de Wouw J, Heinonen I, van Duin RW, Duncker DJ, et al. The microcirculation: a key player in obesity-associated cardiovascular disease. Cardiovasc Res (2017). doi:10.1093/cvr/cvx093
44. Echouffo-Tcheugui JB, Xu H, DeVore AD, Schulte PJ, Butler J, Yancy CW, et al. Temporal trends and factors associated with diabetes mellitus among patients hospitalized with heart failure: findings from get with the guidelines-heart failure registry. Am Heart J (2016) 182:9–20. doi:10.1016/j.ahj.2016.07.025
45. Seferovic PM, Paulus WJ. Clinical diabetic cardiomyopathy: a two-faced disease with restrictive and dilated phenotypes. Eur Heart J (2015) 36:1718–27, 1727a–c. doi:10.1093/eurheartj/ehv134
46. Lam CS. Diabetic cardiomyopathy: an expression of stage B heart failure with preserved ejection fraction. Diab Vasc Dis Res (2015) 12:234–8. doi:10.1177/1479164115579006
47. Lindman BR, Davila-Roman VG, Mann DL, McNulty S, Semigran MJ, Lewis GD, et al. Cardiovascular phenotype in HFpEF patients with or without diabetes: a RELAX trial ancillary study. J Am Coll Cardiol (2014) 64:541–9. doi:10.1016/j.jacc.2014.05.030
48. Kristensen SL, Mogensen UM, Jhund PS, Petrie MC, Preiss D, Win S, et al. Clinical and echocardiographic characteristics and cardiovascular outcomes according to diabetes status in patients with heart failure and preserved ejection fraction: a report from the i-preserve trial (irbesartan in heart failure with preserved ejection fraction). Circulation (2017) 135:724–35. doi:10.1161/CIRCULATIONAHA.116.024593
49. Kao DP, Lewsey JD, Anand IS, Massie BM, Zile MR, Carson PE, et al. Characterization of subgroups of heart failure patients with preserved ejection fraction with possible implications for prognosis and treatment response. Eur J Heart Fail (2015) 17:925–35. doi:10.1002/ejhf.327
50. Heinzel FR, Hohendanner F, Jin G, Sedej S, Edelmann F. Myocardial hypertrophy and its role in heart failure with preserved ejection fraction. J Appl Physiol (1985) (2015) 119:1233–42. doi:10.1152/japplphysiol.00374.2015
51. Methawasin M, Strom JG, Slater RE, Fernandez V, Saripalli C, Granzier H. Experimentally increasing the compliance of titin through RNA binding motif-20 (RBM20) inhibition improves diastolic function in a mouse model of heart failure with preserved ejection fraction. Circulation (2016) 134:1085–99. doi:10.1161/CIRCULATIONAHA.116.023003
52. Huerta A, Lopez B, Ravassa S, San Jose G, Querejeta R, Beloqui O, et al. Association of cystatin C with heart failure with preserved ejection fraction in elderly hypertensive patients: potential role of altered collagen metabolism. J Hypertens (2016) 34:130–8. doi:10.1097/HJH.0000000000000757
53. Wolfel EE. Exploring the mechanisms of exercise intolerance in patients with HFpEF: are we too “cardiocentric”? JACC Heart Fail (2016) 4:646–8. doi:10.1016/j.jchf.2016.06.002
54. Paulus WJ, Tschope C. A novel paradigm for heart failure with preserved ejection fraction: comorbidities drive myocardial dysfunction and remodeling through coronary microvascular endothelial inflammation. J Am Coll Cardiol (2013) 62:263–71. doi:10.1016/j.jacc.2013.02.092
55. Frisbee JC, Goodwill AG, Frisbee SJ, Butcher JT, Brock RW, Olfert IM, et al. Distinct temporal phases of microvascular rarefaction in skeletal muscle of obese Zucker rats. Am J Physiol Heart Circ Physiol (2014) 307:H1714–28. doi:10.1152/ajpheart.00605.2014
56. Ngo DT, Farb MG, Kikuchi R, Karki S, Tiwari S, Bigornia SJ, et al. Antiangiogenic actions of vascular endothelial growth factor-A165b, an inhibitory isoform of vascular endothelial growth factor-A, in human obesity. Circulation (2014) 130:1072–80. doi:10.1161/CIRCULATIONAHA.113.008171
57. Bagi Z, Broskova Z, Feher A. Obesity and coronary microvascular disease – implications for adipose tissue-mediated remote inflammatory response. Curr Vasc Pharmacol (2014) 12:453–61. doi:10.2174/1570161112666140423221843
58. Li ZL, Ebrahimi B, Zhang X, Eirin A, Woollard JR, Tang H, et al. Obesity-metabolic derangement exacerbates cardiomyocyte loss distal to moderate coronary artery stenosis in pigs without affecting global cardiac function. Am J Physiol Heart Circ Physiol (2014) 306:H1087–101. doi:10.1152/ajpheart.00052.2013
59. Tona F, Serra R, Di Ascenzo L, Osto E, Scarda A, Fabris R, et al. Systemic inflammation is related to coronary microvascular dysfunction in obese patients without obstructive coronary disease. Nutr Metab Cardiovasc Dis (2014) 24:447–53. doi:10.1016/j.numecd.2013.09.021
60. Frisbee JC, Samora JB, Peterson J, Bryner R. Exercise training blunts microvascular rarefaction in the metabolic syndrome. Am J Physiol Heart Circ Physiol (2006) 291:H2483–92. doi:10.1152/ajpheart.00566.2006
61. Neves KB, Nguyen Dinh Cat A, Lopes RA, Rios FJ, Anagnostopoulou A, Lobato NS, et al. Chemerin regulates crosstalk between adipocytes and vascular cells through NOX. Hypertension (2015) 66:657–66. doi:10.1161/HYPERTENSIONAHA.115.05616
62. Chawla A, Chawla R, Jaggi S. Microvasular and macrovascular complications in diabetes mellitus: distinct or continuum? Indian J Endocrinol Metab (2016) 20:546–51. doi:10.4103/2230-8210.183480
63. Rosenson RS, Fioretto P, Dodson PM. Does microvascular disease predict macrovascular events in type 2 diabetes? Atherosclerosis (2011) 218:13–8. doi:10.1016/j.atherosclerosis.2011.06.029
64. Wang Y, Yu Q, Fan D, Cao F. Coronary heart disease in type 2 diabetes: mechanisms and comprehensive prevention strategies. Expert Rev Cardiovasc Ther (2012) 10:1051–60. doi:10.1586/erc.12.52
65. Roberts AC, Porter KE. Cellular and molecular mechanisms of endothelial dysfunction in diabetes. Diab Vasc Dis Res (2013) 10:472–82. doi:10.1177/1479164113500680
66. Forbes JM, Cooper ME. Mechanisms of diabetic complications. Physiol Rev (2013) 93:137–88. doi:10.1152/physrev.00045.2011
67. Tschope C, Van Linthout S. New insights in (inter)cellular mechanisms by heart failure with preserved ejection fraction. Curr Heart Fail Rep (2014) 11:436–44. doi:10.1007/s11897-014-0219-3
68. Gallet R, de Couto G, Simsolo E, Valle J, Sun B, Liu W, et al. Cardiosphere-derived cells reverse heart failure with preserved ejection fraction (HFpEF) in rats by decreasing fibrosis and inflammation. JACC Basic Transl Sci (2016) 1:14–28. doi:10.1016/j.jacbts.2016.01.003
69. Westermann D, Lindner D, Kasner M, Zietsch C, Savvatis K, Escher F, et al. Cardiac inflammation contributes to changes in the extracellular matrix in patients with heart failure and normal ejection fraction. Circ Heart Fail (2011) 4:44–52. doi:10.1161/CIRCHEARTFAILURE.109.931451
70. Sucato V, Evola S, Novo G, Sansone A, Quagliana A, Andolina G, et al. Angiographic evaluation of coronary microvascular dysfunction in patients with heart failure and preserved ejection fraction. Microcirculation (2015) 22:528–33. doi:10.1111/micc.12223
71. Franssen C, Chen S, Unger A, Korkmaz HI, De Keulenaer GW, Tschope C, et al. Myocardial microvascular inflammatory endothelial activation in heart failure with preserved ejection fraction. JACC Heart Fail (2016) 4:312–24. doi:10.1016/j.jchf.2015.10.007
72. Kovacs A, Alogna A, Post H, Hamdani N. Is enhancing cGMP-PKG signalling a promising therapeutic target for heart failure with preserved ejection fraction? Neth Heart J (2016) 24:268–74. doi:10.1007/s12471-016-0814-x
73. Arcaro A, Lembo G, Tocchetti CG. Nitroxyl (HNO) for treatment of acute heart failure. Curr Heart Fail Rep (2014) 11:227–35. doi:10.1007/s11897-014-0210-z
74. Bullen ML, Miller AA, Andrews KL, Irvine JC, Ritchie RH, Sobey CG, et al. Nitroxyl (HNO) as a vasoprotective signaling molecule. Antioxid Redox Signal (2011) 14:1675–86. doi:10.1089/ars.2010.3327
75. Zgheib C, Kurdi M, Zouein FA, Gunter BW, Stanley BA, Zgheib J, et al. Acyloxy nitroso compounds inhibit LIF signaling in endothelial cells and cardiac myocytes: evidence that STAT3 signaling is redox-sensitive. PLoS One (2012) 7:e43313. doi:10.1371/journal.pone.0043313
76. Andrews KL, Sampson AK, Irvine JC, Shihata WA, Michell DL, Lumsden NG, et al. Nitroxyl (HNO) reduces endothelial and monocyte activation and promotes M2 macrophage polarization. Clin Sci (Lond) (2016) 130:1629–40. doi:10.1042/CS20160097
77. Sivakumaran V, Stanley BA, Tocchetti CG, Ballin JD, Caceres V, Zhou L, et al. HNO enhances SERCA2a activity and cardiomyocyte function by promoting redox-dependent phospholamban oligomerization. Antioxid Redox Signal (2013) 19:1185–97. doi:10.1089/ars.2012.5057
78. Gao WD, Murray CI, Tian Y, Zhong X, DuMond JF, Shen X, et al. Nitroxyl-mediated disulfide bond formation between cardiac myofilament cysteines enhances contractile function. Circ Res (2012) 111:1002–11. doi:10.1161/CIRCRESAHA.112.270827
79. Zhu G, Groneberg D, Sikka G, Hori D, Ranek MJ, Nakamura T, et al. Soluble guanylate cyclase is required for systemic vasodilation but not positive inotropy induced by nitroxyl in the mouse. Hypertension (2015) 65:385–92. doi:10.1161/HYPERTENSIONAHA.114.04285
80. Cao N, Wong YG, Rosli S, Kiriazis H, Huynh K, Qin C, et al. Chronic administration of the nitroxyl donor 1-nitrosocyclo hexyl acetate limits left ventricular diastolic dysfunction in a mouse model of diabetes mellitus in vivo. Circ Heart Fail (2015) 8:572–81. doi:10.1161/CIRCHEARTFAILURE.114.001699
81. Zamani P, Tan VX, Soto-Calderon H, Beraun M, Brandimarto J, Trieu L, et al. Pharmacokinetics and pharmacodynamics of inorganic nitrate in heart failure with preserved ejection fraction. Circ Res (2016) 120(7):1151–61. doi:10.1161/CIRCRESAHA.116.309832
82. Eggebeen J, Kim-Shapiro DB, Haykowsky M, Morgan TM, Basu S, Brubaker P, et al. One week of daily dosing with beetroot juice improves submaximal endurance and blood pressure in older patients with heart failure and preserved ejection fraction. JACC Heart Fail (2016) 4:428–37. doi:10.1016/j.jchf.2015.12.013
83. Chirinos JA, Zamani P. The nitrate-nitrite-NO pathway and its implications for heart failure and preserved ejection fraction. Curr Heart Fail Rep (2016) 13:47–59. doi:10.1007/s11897-016-0277-9
84. Borlaug BA, Koepp KE, Melenovsky V. Sodium nitrite improves exercise hemodynamics and ventricular performance in heart failure with preserved ejection fraction. J Am Coll Cardiol (2015) 66:1672–82. doi:10.1016/j.jacc.2015.07.067
85. Cannavo A, Koch WJ. Targeting beta3-adrenergic receptors in the heart: selective agonism and beta-blockade. J Cardiovasc Pharmacol (2017) 69:71–8. doi:10.1097/FJC.0000000000000444
86. Michel LY, Balligand JL. New and emerging therapies and targets: beta-3 agonists. Handb Exp Pharmacol (2016) 243:205–23. doi:10.1007/164_2016_88
87. Karimi Galougahi K, Liu CC, Garcia A, Gentile C, Fry NA, Hamilton EJ, et al. Beta3 adrenergic stimulation restores nitric oxide/redox balance and enhances endothelial function in hyperglycemia. J Am Heart Assoc (2016) 5:e002824. doi:10.1161/JAHA.115.002824
88. Karimi Galougahi K, Liu CC, Garcia A, Fry NA, Hamilton EJ, Figtree GA, et al. Beta3-adrenoceptor activation relieves oxidative inhibition of the cardiac Na+-K+ pump in hyperglycemia induced by insulin receptor blockade. Am J Physiol Cell Physiol (2015) 309:C286–95. doi:10.1152/ajpcell.00071.2015
89. Kleindienst A, Battault S, Belaidi E, Tanguy S, Rosselin M, Boulghobra D, et al. Exercise does not activate the beta3 adrenergic receptor-eNOS pathway, but reduces inducible NOS expression to protect the heart of obese diabetic mice. Basic Res Cardiol (2016) 111:40. doi:10.1007/s00395-016-0559-0
90. Machado MV, Martins RL, Borges J, Antunes BR, Estato V, Vieira AB, et al. Exercise training reverses structural microvascular rarefaction and improves endothelium-dependent microvascular reactivity in rats with diabetes. Metab Syndr Relat Disord (2016) 14:298–304. doi:10.1089/met.2015.0146
91. Francois ME, Durrer C, Pistawka KJ, Halperin FA, Little JP. Resistance-based interval exercise acutely improves endothelial function in type 2 diabetes. Am J Physiol Heart Circ Physiol (2016) 311:H1258–67. doi:10.1152/ajpheart.00398.2016
92. Olver TD, Laughlin MH. Endurance, interval sprint, and resistance exercise training: impact on microvascular dysfunction in type 2 diabetes. Am J Physiol Heart Circ Physiol (2016) 310:H337–50. doi:10.1152/ajpheart.00440.2015
93. Schreuder TH, Duncker DJ, Hopman MT, Thijssen DH. Randomized controlled trial using bosentan to enhance the impact of exercise training in subjects with type 2 diabetes mellitus. Exp Physiol (2014) 99:1538–47. doi:10.1113/expphysiol.2014.081182
94. Pandey A, Parashar A, Kumbhani DJ, Agarwal S, Garg J, Kitzman D, et al. Exercise training in patients with heart failure and preserved ejection fraction: meta-analysis of randomized control trials. Circ Heart Fail (2015) 8:33–40. doi:10.1161/CIRCHEARTFAILURE.114.001615
95. Giordano M, Ciarambino T, Castellino P, Cataliotti A, Malatino L, Ferrara N, et al. Long-term effects of moderate protein diet on renal function and low-grade inflammation in older adults with type 2 diabetes and chronic kidney disease. Nutrition (2014) 30:1045–9. doi:10.1016/j.nut.2014.03.007
96. Kitzman DW, Brubaker PH, Herrington DM, Morgan TM, Stewart KP, Hundley WG, et al. Effect of endurance exercise training on endothelial function and arterial stiffness in older patients with heart failure and preserved ejection fraction: a randomized, controlled, single-blind trial. J Am Coll Cardiol (2013) 62:584–92. doi:10.1016/j.jacc.2013.04.033
97. Kitzman DW, Brubaker P, Morgan T, Haykowsky M, Hundley G, Kraus WE, et al. Effect of caloric restriction or aerobic exercise training on peak oxygen consumption and quality of life in obese older patients with heart failure with preserved ejection fraction: a randomized clinical Trial. JAMA (2016) 315:36–46. doi:10.1001/jama.2015.17346
98. Wenger NK. Lifestyle interventions to improve exercise tolerance in obese older patients with heart failure and preserved ejection fraction. JAMA (2016) 315:31–3. doi:10.1001/jama.2015.17347
99. Upadhya B, Haykowsky MJ, Eggebeen J, Kitzman DW. Sarcopenic obesity and the pathogenesis of exercise intolerance in heart failure with preserved ejection fraction. Curr Heart Fail Rep (2015) 12:205–14. doi:10.1007/s11897-015-0257-5
100. Owan T, Avelar E, Morley K, Jiji R, Hall N, Krezowski J, et al. Favorable changes in cardiac geometry and function following gastric bypass surgery: 2-year follow-up in the Utah obesity study. J Am Coll Cardiol (2011) 57:732–9. doi:10.1016/j.jacc.2010.10.017
101. Heinonen I, Sorop O, de Beer VJ, Duncker DJ, Merkus D. What can we learn about treating heart failure from the heart’s response to acute exercise? Focus on the coronary microcirculation. J Appl Physiol (1985) (2015) 119:934–43. doi:10.1152/japplphysiol.00053.2015
102. Trippel TD, Holzendorf V, Halle M, Gelbrich G, Nolte K, Duvinage A, et al. Ghrelin and hormonal markers under exercise training in patients with heart failure with preserved ejection fraction: results from the Ex-DHF pilot study. ESC Heart Fail (2017) 4:56–65. doi:10.1002/ehf2.12109
103. Nagaya N, Kojima M, Uematsu M, Yamagishi M, Hosoda H, Oya H, et al. Hemodynamic and hormonal effects of human ghrelin in healthy volunteers. Am J Physiol Regul Integr Comp Physiol (2001) 280:R1483–7.
104. Baldanzi G, Filigheddu N, Cutrupi S, Catapano F, Bonissoni S, Fubini A, et al. Ghrelin and des-acyl ghrelin inhibit cell death in cardiomyocytes and endothelial cells through ERK1/2 and PI 3-kinase/AKT. J Cell Biol (2002) 159:1029–37. doi:10.1083/jcb.200207165
105. Churm R, Davies JS, Stephens JW, Prior SL. Ghrelin function in human obesity and type 2 diabetes: a concise review. Obes Rev (2017) 18:140–8. doi:10.1111/obr.12474
106. Panati K, Suneetha Y, Narala VR. Irisin/FNDC5 – an updated review. Eur Rev Med Pharmacol Sci (2016) 20:689–97.
107. Perakakis N, Triantafyllou GA, Fernandez-Real JM, Huh JY, Park KH, Seufert J, et al. Physiology and role of irisin in glucose homeostasis. Nat Rev Endocrinol (2017) 13(6):324–37. doi:10.1038/nrendo.2016.221
108. Shoukry A, Shalaby SM, El-Arabi Bdeer S, Mahmoud AA, Mousa MM, Khalifa A. Circulating serum irisin levels in obesity and type 2 diabetes mellitus. IUBMB Life (2016) 68:544–56. doi:10.1002/iub.1511
109. Hou N, Han F, Sun X. The relationship between circulating irisin levels and endothelial function in lean and obese subjects. Clin Endocrinol (Oxf) (2015) 83:339–43. doi:10.1111/cen.12658
110. Han F, Zhang S, Hou N, Wang D, Sun X. Irisin improves endothelial function in obese mice through the AMPK-eNOS pathway. Am J Physiol Heart Circ Physiol (2015) 309:H1501–8. doi:10.1152/ajpheart.00443.2015
111. Fu J, Han Y, Wang J, Liu Y, Zheng S, Zhou L, et al. Irisin lowers blood pressure by improvement of endothelial dysfunction via AMPK-Akt-eNOS-NO pathway in the spontaneously hypertensive rat. J Am Heart Assoc (2016) 5:e003433. doi:10.1161/JAHA.116.003433
112. Poddar M, Chetty Y, Chetty VT. How does obesity affect the endocrine system? A narrative review. Clin Obes (2017) 7(3):136–44. doi:10.1111/cob.12184
113. Buglioni A, Cannone V, Sangaralingham SJ, Heublein DM, Scott CG, Bailey KR, et al. Aldosterone predicts cardiovascular, renal, and metabolic disease in the general community: a 4-year follow-up. J Am Heart Assoc (2015) 4:e002505. doi:10.1161/JAHA.115.002505
114. De Vecchis R, Cantatrione C, Mazzei D, Barone A, Maurea N. The impact exerted on clinical outcomes of patients with chronic heart failure by aldosterone receptor antagonists: a meta-analysis of randomized controlled trials. J Clin Med Res (2017) 9:130–42. doi:10.14740/jocmr2851w
115. Elguindy AM. TOPCAT misses its primary endpoint: should spironolactone be abandoned in HFpEF? Glob Cardiol Sci Pract (2013) 2013:357–60. doi:10.5339/gcsp.2013.42
116. Bender SB, DeMarco VG, Padilla J, Jenkins NT, Habibi J, Garro M, et al. Mineralocorticoid receptor antagonism treats obesity-associated cardiac diastolic dysfunction. Hypertension (2015) 65:1082–8. doi:10.1161/HYPERTENSIONAHA.114.04912
117. Youcef G, Olivier A, Nicot N, Muller A, Deng C, Labat C, et al. Preventive and chronic mineralocorticoid receptor antagonism is highly beneficial in obese SHHF rats. Br J Pharmacol (2016) 173:1805–19. doi:10.1111/bph.13479
118. Pitt B, Pfeffer MA, Assmann SF, Boineau R, Anand IS, Claggett B, et al. Spironolactone for heart failure with preserved ejection fraction. N Engl J Med (2014) 370:1383–92. doi:10.1056/NEJMoa1313731
119. Lewis EF, Kim HY, Claggett B, Spertus J, Heitner JF, Assmann SF, et al. Impact of spironolactone on longitudinal changes in health-related quality of life in the treatment of preserved cardiac function heart failure with an aldosterone antagonist trial. Circ Heart Fail (2016) 9:e001937. doi:10.1161/CIRCHEARTFAILURE.114.001937
120. Kosmala W, Rojek A, Przewlocka-Kosmala M, Wright L, Mysiak A, Marwick TH. Effect of aldosterone antagonism on exercise tolerance in heart failure with preserved ejection fraction. J Am Coll Cardiol (2016) 68:1823–34. doi:10.1016/j.jacc.2016.07.763
121. Pfeffer MA, Claggett B, Assmann SF, Boineau R, Anand IS, Clausell N, et al. Regional variation in patients and outcomes in the treatment of preserved cardiac function heart failure with an aldosterone antagonist (TOPCAT) trial. Circulation (2015) 131:34–42. doi:10.1161/CIRCULATIONAHA.114.013255
122. Solomon SD, Claggett B, Lewis EF, Desai A, Anand I, Sweitzer NK, et al. Influence of ejection fraction on outcomes and efficacy of spironolactone in patients with heart failure with preserved ejection fraction. Eur Heart J (2016) 37:455–62. doi:10.1093/eurheartj/ehv464
123. Anand IS, Claggett B, Liu J, Shah AM, Rector TS, Shah SJ, et al. Interaction between spironolactone and natriuretic peptides in patients with heart failure and preserved ejection fraction: from the TOPCAT trial. JACC Heart Fail (2017) 5:241–52. doi:10.1016/j.jchf.2016.11.015
124. Davel AP, Anwar IJ, Jaffe IZ. The endothelial mineralocorticoid receptor: mediator of the switch from vascular health to disease. Curr Opin Nephrol Hypertens (2017) 26:97–104. doi:10.1097/MNH.0000000000000306
125. Jia G, Habibi J, DeMarco VG, Martinez-Lemus LA, Ma L, Whaley-Connell AT, et al. Endothelial mineralocorticoid receptor deletion prevents diet-induced cardiac diastolic dysfunction in females. Hypertension (2015) 66:1159–67. doi:10.1161/HYPERTENSIONAHA.115.06015
126. Njock MS, Fish JE. Endothelial miRNAs as cellular messengers in cardiometabolic diseases. Trends Endocrinol Metab (2017) 28:237–46. doi:10.1016/j.tem.2016.11.009
127. Watson CJ, Gupta SK, O’Connell E, Thum S, Glezeva N, Fendrich J, et al. MicroRNA signatures differentiate preserved from reduced ejection fraction heart failure. Eur J Heart Fail (2015) 17:405–15. doi:10.1002/ejhf.244
128. Wong LL, Armugam A, Sepramaniam S, Karolina DS, Lim KY, Lim JY, et al. Circulating microRNAs in heart failure with reduced and preserved left ventricular ejection fraction. Eur J Heart Fail (2015) 17:393–404. doi:10.1002/ejhf.223
129. Beltrami C, Angelini TG, Emanueli C. Noncoding RNAs in diabetes vascular complications. J Mol Cell Cardiol (2015) 89:42–50. doi:10.1016/j.yjmcc.2014.12.014
130. Rawal S, Munasinghe PE, Shindikar A, Paulin J, Cameron V, Manning P, et al. Down-regulation of proangiogenic microRNA-126 and microRNA-132 are early modulators of diabetic cardiac microangiopathy. Cardiovasc Res (2017) 113:90–101. doi:10.1093/cvr/cvw235
131. da Silva ND Jr, Fernandes T, Soci UP, Monteiro AW, Phillips MI, de Oliveira EM. Swimming training in rats increases cardiac microRNA-126 expression and angiogenesis. Med Sci Sports Exerc (2012) 44:1453–62. doi:10.1249/MSS.0b013e31824e8a36
132. Sethupathy P. The promise and challenge of therapeutic microRNA silencing in diabetes and metabolic diseases. Curr Diab Rep (2016) 16:52. doi:10.1007/s11892-016-0745-3
133. Deiuliis JA. MicroRNAs as regulators of metabolic disease: pathophysiologic significance and emerging role as biomarkers and therapeutics. Int J Obes (Lond) (2016) 40:88–101. doi:10.1038/ijo.2015.170
134. Peng Y, Yu S, Li H, Xiang H, Peng J, Jiang S. MicroRNAs: emerging roles in adipogenesis and obesity. Cell Signal (2014) 26:1888–96. doi:10.1016/j.cellsig.2014.05.006
135. Boon RA, Jae N, Holdt L, Dimmeler S. Long noncoding RNAs: from clinical genetics to therapeutic targets? J Am Coll Cardiol (2016) 67:1214–26. doi:10.1016/j.jacc.2015.12.051
136. de Gonzalo-Calvo D, Kenneweg F, Bang C, Toro R, van der Meer RW, Rijzewijk LJ, et al. Circulating long-non coding RNAs as biomarkers of left ventricular diastolic function and remodelling in patients with well-controlled type 2 diabetes. Sci Rep (2016) 6:37354. doi:10.1038/srep37354
137. Boulberdaa M, Scott E, Ballantyne M, Garcia R, Descamps B, Angelini GD, et al. A role for the long noncoding RNA SENCR in commitment and function of endothelial cells. Mol Ther (2016) 24:978–90. doi:10.1038/mt.2016.41
138. Li T, Jiang S, Yang Z, Ma Z, Yi W, Wang D, et al. Targeting the energy guardian AMPK: another avenue for treating cardiomyopathy? Cell Mol Life Sci (2017) 74:1413–29. doi:10.1007/s00018-016-2407-7
139. Cheang WS, Tian XY, Wong WT, Lau CW, Lee SS, Chen ZY, et al. Metformin protects endothelial function in diet-induced obese mice by inhibition of endoplasmic reticulum stress through 5′ adenosine monophosphate-activated protein kinase-peroxisome proliferator-activated receptor delta pathway. Arterioscler Thromb Vasc Biol (2014) 34:830–6. doi:10.1161/ATVBAHA.113.301938
140. Donato AJ, Morgan RG, Walker AE, Lesniewski LA. Cellular and molecular biology of aging endothelial cells. J Mol Cell Cardiol (2015) 89:122–35. doi:10.1016/j.yjmcc.2015.01.021
141. van der Meer RW, Rijzewijk LJ, de Jong HW, Lamb HJ, Lubberink M, Romijn JA, et al. Pioglitazone improves cardiac function and alters myocardial substrate metabolism without affecting cardiac triglyceride accumulation and high-energy phosphate metabolism in patients with well-controlled type 2 diabetes mellitus. Circulation (2009) 119:2069–77. doi:10.1161/CIRCULATIONAHA.108.803916
142. Liao HW, Saver JL, Wu YL, Chen TH, Lee M, Ovbiagele B. Pioglitazone and cardiovascular outcomes in patients with insulin resistance, pre-diabetes and type 2 diabetes: a systematic review and meta-analysis. BMJ Open (2017) 7:e013927. doi:10.1136/bmjopen-2016-013927
143. Cheng JW, Badreldin HA, Patel DK, Bhatt SH. Antidiabetic agents and cardiovascular outcomes in patients with heart diseases. Curr Med Res Opin (2017) 33(6):985–92. doi:10.1080/03007995.2017.1284052
144. Luconi M, Cantini G, Ceriello A, Mannucci E. Perspectives on cardiovascular effects of incretin-based drugs: from bedside to bench, return trip. Int J Cardiol (2017) 241:302–10. doi:10.1016/j.ijcard.2017.02.126
145. Munaf M, Pellicori P, Allgar V, Wong K. A meta-analysis of the therapeutic effects of glucagon-like peptide-1 agonist in heart failure. Int J Pept (2012) 2012:249827. doi:10.1155/2012/249827
146. Martens P, Mathieu C, Verbrugge FH. Promise of SGLT2 inhibitors in heart failure: diabetes and beyond. Curr Treat Options Cardiovasc Med (2017) 19:23. doi:10.1007/s11936-017-0522-x
147. Zinman B, Wanner C, Lachin JM, Fitchett D, Bluhmki E, Hantel S, et al. Empagliflozin, cardiovascular outcomes, and mortality in type 2 diabetes. N Engl J Med (2015) 373:2117–28. doi:10.1056/NEJMoa1504720
148. Ampudia-Blasco FJ, Romera I, Arino B, Gomis R. Following the results of the EMPA-REG OUTCOME trial with empagliflozin, is it possible to speak of a class effect? Int J Gen Med (2017) 10:23–6. doi:10.2147/IJGM.S115566
149. Redfield MM, Anstrom KJ, Levine JA, Koepp GA, Borlaug BA, Chen HH, et al. Isosorbide mononitrate in heart failure with preserved ejection fraction. N Engl J Med (2015) 373:2314–24. doi:10.1056/NEJMoa1510774
150. Stasch JP, Schlossmann J, Hocher B. Renal effects of soluble guanylate cyclase stimulators and activators: a review of the preclinical evidence. Curr Opin Pharmacol (2015) 21:95–104. doi:10.1016/j.coph.2014.12.014
151. Pieske B, Butler J, Filippatos G, Lam C, Maggioni AP, Ponikowski P, et al. Rationale and design of the soluble guanylate cyclase stimulator in heart failure studies (SOCRATES). Eur J Heart Fail (2014) 16:1026–38. doi:10.1002/ejhf.135
152. Gheorghiade M, Greene SJ, Butler J, Filippatos G, Lam CS, Maggioni AP, et al. Effect of vericiguat, a soluble guanylate cyclase stimulator, on natriuretic peptide levels in patients with worsening chronic heart failure and reduced ejection fraction: the SOCRATES-REDUCED randomized trial. JAMA (2015) 314:2251–62. doi:10.1001/jama.2015.15734
153. Kass DA. Cardiac role of cyclic-GMP hydrolyzing phosphodiesterase type 5: from experimental models to clinical trials. Curr Heart Fail Rep (2012) 9:192–9. doi:10.1007/s11897-012-0101-0
154. Lukowski R, Krieg T, Rybalkin SD, Beavo J, Hofmann F. Turning on cGMP-dependent pathways to treat cardiac dysfunctions: boom, bust, and beyond. Trends Pharmacol Sci (2014) 35:404–13. doi:10.1016/j.tips.2014.05.003
155. Cataliotti A, Costello-Boerrigter LC, Chen HH, Textor SC, Burnett JC Jr. Sustained blood pressure-lowering actions of subcutaneous B-type natriuretic peptide (nesiritide) in a patient with uncontrolled hypertension. Mayo Clin Proc (2012) 87:413–5. doi:10.1016/j.mayocp.2012.02.003
156. Cataliotti A, Tonne JM, Bellavia D, Martin FL, Oehler EA, Harders GE, et al. Long-term cardiac pro-B-type natriuretic peptide gene delivery prevents the development of hypertensive heart disease in spontaneously hypertensive rats. Circulation (2011) 123:1297–305. doi:10.1161/CIRCULATIONAHA.110.981720
157. Holditch SJ, Schreiber CA, Nini R, Tonne JM, Peng KW, Geurts A, et al. B-type natriuretic peptide deletion leads to progressive hypertension, associated organ damage, and reduced survival: novel model for human hypertension. Hypertension (2015) 66:199–210. doi:10.1161/HYPERTENSIONAHA.115.05610
158. Hsu S, Kass DA. Can nitrite AMPk up sirt-ainty to treat heart failure with preserved ejection fraction? Circulation (2016) 133:692–4. doi:10.1161/CIRCULATIONAHA.116.021409
159. Solomon SD, Zile M, Pieske B, Voors A, Shah A, Kraigher-Krainer E, et al. The angiotensin receptor neprilysin inhibitor LCZ696 in heart failure with preserved ejection fraction: a phase 2 double-blind randomised controlled trial. Lancet (2012) 380:1387–95. doi:10.1016/S0140-6736(12)61227-6
160. Shah SJ, Kitzman DW, Borlaug BA, van Heerebeek L, Zile MR, Kass DA, et al. Phenotype-specific treatment of heart failure with preserved ejection fraction: a multiorgan roadmap. Circulation (2016) 134:73–90. doi:10.1161/CIRCULATIONAHA.116.021884
161. Belluardo P, Cataliotti A, Bonaiuto L, Giuffre E, Maugeri E, Noto P, et al. Lack of activation of molecular forms of the BNP system in human grade 1 hypertension and relationship to cardiac hypertrophy. Am J Physiol Heart Circ Physiol (2006) 291:H1529–35. doi:10.1152/ajpheart.00107.2006
162. Macheret F, Heublein D, Costello-Boerrigter LC, Boerrigter G, McKie P, Bellavia D, et al. Human hypertension is characterized by a lack of activation of the antihypertensive cardiac hormones ANP and BNP. J Am Coll Cardiol (2012) 60:1558–65. doi:10.1016/j.jacc.2012.05.049
163. Khalid U, Wruck LM, Quibrera PM, Bozkurt B, Nambi V, Virani SS, et al. BNP and obesity in acute decompensated heart failure with preserved vs. reduced ejection fraction: the atherosclerosis risk in communities surveillance study. Int J Cardiol (2017) 233:61–6. doi:10.1016/j.ijcard.2017.01.130
164. van Veldhuisen DJ, Linssen GC, Jaarsma T, van Gilst WH, Hoes AW, Tijssen JG, et al. B-type natriuretic peptide and prognosis in heart failure patients with preserved and reduced ejection fraction. J Am Coll Cardiol (2013) 61:1498–506. doi:10.1016/j.jacc.2012.12.044
165. Hawkridge AM, Heublein DM, Bergen HR III, Cataliotti A, Burnett JC Jr, Muddiman DC. Quantitative mass spectral evidence for the absence of circulating brain natriuretic peptide (BNP-32) in severe human heart failure. Proc Natl Acad Sci U S A (2005) 102:17442–7. doi:10.1073/pnas.0508782102
166. Madamanchi C, Alhosaini H, Sumida A, Runge MS. Obesity and natriuretic peptides, BNP and NT-proBNP: mechanisms and diagnostic implications for heart failure. Int J Cardiol (2014) 176:611–7. doi:10.1016/j.ijcard.2014.08.007
167. Yan W, Wu F, Morser J, Wu Q. Corin, a transmembrane cardiac serine protease, acts as a pro-atrial natriuretic peptide-converting enzyme. Proc Natl Acad Sci U S A (2000) 97:8525–9. doi:10.1073/pnas.150149097
168. Vesely DL, Perez-Lamboy GI, Schocken DD. Vessel dilator, long acting natriuretic peptide, and kaliuretic peptide increase circulating prostaglandin E2. Life Sci (2000) 66:905–13. doi:10.1016/S0024-3205(99)00674-8
169. Clark LC, Farghaly H, Saba SR, Vesely DL. Amelioration with vessel dilator of acute tubular necrosis and renal failure established for 2 days. Am J Physiol Heart Circ Physiol (2000) 278:H1555–64.
170. Zeidel ML. Regulation of collecting duct Na+ reabsorption by ANP 31-67. Clin Exp Pharmacol Physiol (1995) 22:121–4. doi:10.1111/j.1440-1681.1995.tb01967.x
171. Gunning ME, Brady HR, Otuechere G, Brenner BM, Zeidel ML. Atrial natriuretic peptide(31-67) inhibits Na+ transport in rabbit inner medullary collecting duct cells. Role of prostaglandin E2. J Clin Invest (1992) 89:1411–7. doi:10.1172/JCI115730
172. Zhou Y, Yang P, Li A, Ye X, Ren S, Li X. Prostaglandin E2 reduces swine myocardial ischemia reperfusion injury via increased endothelial nitric oxide synthase and vascular endothelial growth factor expression levels. Biomed Rep (2017) 6:188–94. doi:10.3892/br.2016.834
173. Pang L, Cai Y, Tang EH, Irwin MG, Ma H, Xia Z. Prostaglandin E receptor subtype 4 signaling in the heart: role in ischemia/reperfusion injury and cardiac hypertrophy. J Diabetes Res (2016) 2016:1324347. doi:10.1155/2016/1324347
174. Goncalves N, Falcao-Pires I, Leite-Moreira AF. Adipokines and their receptors: potential new targets in cardiovascular diseases. Future Med Chem (2015) 7:139–57. doi:10.4155/fmc.14.147
176. Francisco C, Neves JS, Falcao-Pires I, Leite-Moreira A. Can adiponectin help us to target diastolic dysfunction? Cardiovasc Drugs Ther (2016) 30:635–44. doi:10.1007/s10557-016-6694-x
177. Faxen UL, Hage C, Andreasson A, Donal E, Daubert JC, Linde C, et al. HFpEF and HFrEF exhibit different phenotypes as assessed by leptin and adiponectin. Int J Cardiol (2017) 228:709–16. doi:10.1016/j.ijcard.2016.11.194
178. Witberg G, Ayers CR, Turer AT, Lev E, Kornowski R, de Lemos J, et al. Relation of adiponectin to all-cause mortality, cardiovascular mortality, and major adverse cardiovascular events (from the Dallas Heart Study). Am J Cardiol (2016) 117:574–9. doi:10.1016/j.amjcard.2015.11.067
179. Norvik JV, Schirmer H, Ytrehus K, Jenssen TG, Zykova SN, Eggen AE, et al. Low adiponectin is associated with diastolic dysfunction in women: a cross-sectional study from the Tromso Study. BMC Cardiovasc Disord (2017) 17:79. doi:10.1186/s12872-017-0509-2
180. Meta-analysis Global Group in Chronic Heart Failure (MAGGIC). The survival of patients with heart failure with preserved or reduced left ventricular ejection fraction: an individual patient data meta-analysis. Eur Heart J (2012) 33:1750–7. doi:10.1093/eurheartj/ehr254
181. Owan TE, Hodge DO, Herges RM, Jacobsen SJ, Roger VL, Redfield MM. Trends in prevalence and outcome of heart failure with preserved ejection fraction. N Engl J Med (2006) 355:251–9. doi:10.1056/NEJMoa052256
182. Goyal P, Paul T, Almarzooq ZI, Peterson JC, Krishnan U, Swaminathan RV, et al. Sex- and race-related differences in characteristics and outcomes of hospitalizations for heart failure with preserved ejection fraction. J Am Heart Assoc (2017) 6:e003330. doi:10.1161/JAHA.116.003330
Keywords: metabolic disease, heart function, diastolic dysfunction, endothelial and microvascular dysfunction, inflammation, hypertension
Citation: Altara R, Giordano M, Nordén ES, Cataliotti A, Kurdi M, Bajestani SN and Booz GW (2017) Targeting Obesity and Diabetes to Treat Heart Failure with Preserved Ejection Fraction. Front. Endocrinol. 8:160. doi: 10.3389/fendo.2017.00160
Received: 03 April 2017; Accepted: 23 June 2017;
Published: 17 July 2017
Edited by:
Gaetano Santulli, Columbia University, United StatesReviewed by:
Ilkka H. A. Heinonen, University of Turku, FinlandHaroldo A. Toque, Georgia Health Sciences University, United States
Copyright: © 2017 Altara, Giordano, Nordén, Cataliotti, Kurdi, Bajestani and Booz. This is an open-access article distributed under the terms of the Creative Commons Attribution License (CC BY). The use, distribution or reproduction in other forums is permitted, provided the original author(s) or licensor are credited and that the original publication in this journal is cited, in accordance with accepted academic practice. No use, distribution or reproduction is permitted which does not comply with these terms.
*Correspondence: Raffaele Altara, raffaele.altara@medisin.uio.no