- Jeffrey Cheah School of Medicine and Health Sciences, Brain Research Institute Monash Sunway, Monash University Malaysia, Sunway, Malaysia
Gonadotropin-inhibitory hormone (GnIH) is a hypothalamic neuropeptide that decreases gonadotropin synthesis and release by directly acting on the gonadotrope or by decreasing the activity of gonadotropin-releasing hormone (GnRH) neurons. GnIH is also called RFamide-related peptide in mammals or LPXRFamide peptide in fishes due to its characteristic C-terminal structure. The primary receptor for GnIH is GPR147 that inhibits cAMP production in target cells. Although most of the studies in mammals, birds, and fish have shown the inhibitory action of GnIH in the hypothalamic–pituitary–gonadal (HPG) axis, several in vivo studies in mammals and many in vivo and in vitro studies in fish have shown its stimulatory action. In mouse, although the firing rate of the majority of GnRH neurons is decreased, a small population of GnRH neurons is stimulated by GnIH. In hamsters, GnIH inhibits luteinizing hormone (LH) release in the breeding season when their endogenous LH level is high but stimulates LH release in non-breeding season when their LH level is basal. Besides different effects of GnIH on the HPG axis depending on the reproductive stages in fish, higher concentration or longer duration of GnIH administration can stimulate their HPG axis. These results suggest that GnIH action in the HPG axis is modulated by sex-steroid concentration, the action of neuroestrogen synthesized by the activity of aromatase stimulated by GnIH, estrogen membrane receptor, heteromerization and internalization of GnIH, GnRH, and estrogen membrane receptors. The inhibitory and stimulatory action of GnIH in the HPG axis may have a physiological role to maintain reproductive homeostasis according to developmental and reproductive stages.
Introduction
Gonadotropin-inhibitory hormone (GnIH) is a hypothalamic neuropeptide that was initially isolated from the brain of Japanese quail, which decreases luteinizing hormone (LH) concentration in the culture medium of the anterior pituitary gland (1). In vivo administration of quail GnIH also decreases gonadotropin synthesis as well as gonadal development and maintenance in quail (2). The C-terminal of GnIH peptides has an LPXRFamide (LPXRFa, X = L or Q) motif. Therefore, peptides orthologous to GnIH are also called RFamide-related peptide (RFRP) in mammals and LPXRFa peptides in non-mammalian and non-avian vertebrates (3). Most of the studies in mammals, birds, and fish have shown inhibitory effects of GnIH on the hypothalamic–pituitary–gonadal (HPG) axis; however, several in vivo and in vitro studies in mammals and fish show its stimulatory effects (3, 4). Here, we highlight studies that show stimulatory effects of GnIH on the HPG axis and investigate their physiological or pharmacological mechanisms.
Endogenous Mature GnIH Peptides
Human RFRP-1 and -3 (5), macaque RFRP-3 (6), Siberian hamster RFRP-1 and -3 (7), rat RFRP-3 (8), bovine RFRP-1 (9) and -3 (10), European starling GnIH (11), zebra finch GnIH (12), chicken GnIH (13), quail GnIH (1), quail GnIH-related peptide (RP) 2 (14), red-eared slider LPXRFamide-1, 2, 3 (15), frog growth hormone-releasing hormone (fGRP), fGRP-RP-1, fGRP-RP-2, and fGRP-RP-3 (16, 17), Japanese red-bellied newt LPXRFa-1, -2, -3, -4 (18), and goldfish LPXRFa-3 (19) are identified as endogenous mature LPXRFa peptides by cDNA sequencing, immunoaffinity chromatography, and mass spectrometry in gnathostomes (3). Lamprey is a jawless fish that is one of the most primitive among vertebrates. Lamprey LPXRFamide peptide precursor gene encompasses C-terminal QPQRFamide (LPXRFa-1a, 1b) and RPQRFamide peptides (LPXRFa-2) that have been identified by mass spectrometry (20). LPXRFamide peptide precursor gene is also found in amphioxus, one of the most primitive chordates (protochordates), which encompasses three mature C-terminal RPQRFamide peptides (PQRFa-1, PQRFa-2, and PQRFa-3) (21). Identified and putative amino-acid sequences of GnIH peptides are summarized in Table 1. Although the C-terminal LPXRFa structure is key for binding of GnIH to its receptor (22), the N-terminal structure may modify the action of GnIH. Studies are needed to investigate the function of the N-terminal of GnIH and the differential effect of orthologous LPXRFa peptides encoded in the precursor polypeptide (Table 1).
GnIH Receptor
Yin et al. characterized the binding activity of quail GnIH and GnIH-RPs to a G-protein-coupled receptor (GPCR) GPR147. The membrane fraction of COS-7 cells transfected with quail GPR147 cDNA specifically bound GnIH and GnIH-RPs that have a C-terminal LPXRFa motif with similar affinities (22). Hinuma et al. identified a specific receptor for GnIH (RFRP) in mammals, which was identical to GPR147 and named it OT7T022 (28). In the same year, Bonini et al. reported two GPCRs for neuropeptide FF (NPFF), a neuropeptide that has a PQRFamide (PQRFa) motif at its C-terminal that modulates pain, and designated as NPFF1 (identical to GPR147) and NPFF2 (identical to GPR74) (29). LPXRFa peptide precursor gene and PQRFa peptide precursor gene are thought to have diverged from a common ancestral gene through gene duplication (20, 21). GPR147 and GPR74 genes are also paralogous (30). The binding affinities of RFRPs to GPR147 and GPR74 and their signal transduction pathways show their higher affinity to GPR147 than NPFF that has a potent agonistic activity on GPR74 (10, 29, 31), suggesting that GPR147 (NPFF1, OT7T022) is the primary receptor for GnIH (3). However, this may not apply to teleost fishes as they generally have several subtypes of GPR147 and/or GPR74 (32).
Intracellular Signaling of GnIH Receptor
Gonadotropin-inhibitory hormone peptides suppress the production of cAMP by binding to GPR147 on the cells, suggesting that GPR147 couples to Gαi protein that inhibits adenylate cyclase (AC) (28, 33). Son et al. investigated the precise mechanism of GnIH cell-signaling pathway in a mouse gonadotrope cell line, LβT2 (34). Mouse RFRPs (mRFRPs) suppress GnRH-induced cAMP signaling. mRFRPs also inhibit GnRH-stimulated extracellular signal-regulated kinase (ERK) phosphorylation and gonadotropin subunit gene transcription by inhibiting the protein kinase A (PKA) pathway. Therefore, mRFRPs function as GnIH to inhibit GnRH-induced gonadotropin subunit gene transcription by inhibiting AC/cAMP/PKA-dependent ERK activation in gonadotropes (34) (Table 2).
Son et al. further investigated the signal transduction pathway that conveys the inhibitory action of GnIH in GnRH neurons by using a mouse GnRH neuronal cell line, GT1–7 (46). Although GnIH significantly suppressed the stimulatory effect of kisspeptin on GnRH release in hypothalamic culture, GnIH had no inhibitory effect on the protein kinase C (PKC) pathway stimulated by kisspeptin in GnRH neurons. On the other hand, GnIH eliminated the stimulatory effect of vasoactive intestinal polypeptide (VIP) on AC activity, p38 and ERK phosphorylation, and c-Fos mRNA expression in GT1–7. This shows the specific inhibitory mechanism of GnIH action on AC/cAMP/PKA pathway, and demonstrates a common mechanism of GnIH action in gonadotropes and GnRH neurons (34, 46) (Table 2).
Existence of GnIH and GnIH Receptor in the HPG Axis
Gonadotropin-inhibitory hormone precursor mRNA is expressed in the hypothalamus of all vertebrates investigated (3). GnIH neuronal axons terminate on GnRH1 neurons in the preoptic area (POA) that terminate at the median eminence and stimulate gonadotropin secretion from the anterior pituitary gland in birds (11, 12, 52–55) (Figure 1). In situ hybridization of GPR147 mRNA combined with GnRH immunocytochemistry shows expression of GPR147 mRNA in GnRH1 neurons in birds (11). GnIH (RFRP) axons also terminate on the hypophysiotropic type of GnRH neurons in humans (5), monkey (6), sheep (56), hamsters (7, 45), rats (39, 57), mice (58), frog (59), zebrafish (60), and lamprey (20). Double-immunohistochemistry using GPR147 and GnRH antibodies shows GPR147 on GnRH neurons in hamsters (7) (Figure 1).
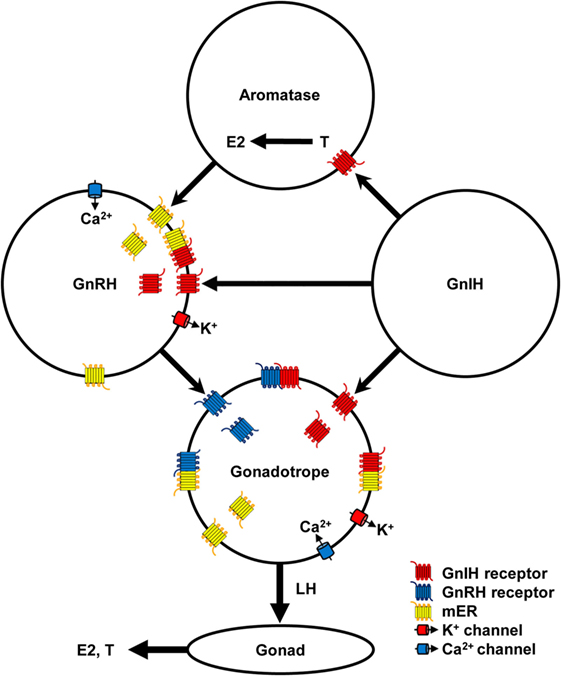
Figure 1. Schematic diagram of the mechanism of gonadotropin-inhibitory hormone (GnIH) action in the hypothalamic–pituitary–gonadal axis. GnIH neurons act on aromatase and gonadotropin-releasing hormone (GnRH) neurons in the hypothalamus and gonadotrope in the pituitary via GnIH receptor. Aromatase neurons synthesize estradiol-17β (E2) from testosterone (T) in the hypothalamus and E2 can act on GnRH neurons via membrane estrogen receptor (mER). GnIH stimulates K+ channel to hyperpolarize GnRH neurons and gonadotrope, and decrease GnRH and luteinizing hormone (LH) release, respectively. E2 stimulates Ca2+ channel to depolarize GnRH neurons and stimulates GnRH release. GnRH stimulates GnRH receptor and Ca2+ channel to depolarize gonadotrope and stimulates LH release. Low concentration of E2 inhibits Ca2+ channel on the gonadotrope and LH release stimulated by GnRH. LH stimulates synthesis and release of E2 and T from ovary and testis, respectively. GnIH and GnRH receptors and GPR30 (mER) belong to Class A G-protein coupled receptor family and may form heteromers to modulate ligand binding affinity and signal transduction. Binding of GnIH, GnRH, and E2 with their receptors can downregulate their cognate receptors by internalization. These complex stimulatory and inhibitory mechanisms may regulate reproductive homeostasis according to developmental and reproductive stages.
Abundant GnIH-immunoreactive (ir) fibers exist in the median eminence of humans (5), monkey (6), sheep (50), quail (1, 25, 61), sparrow (52, 62), and turtle (15). It has been clearly shown that GPR147 mRNA is expressed in the gonadotropes of human pituitary (5). GPR147-ir cells are located in the cephalic and caudal lobes of the chicken pituitary gland and they are colocalized with LHβ or FSHβ mRNA-containing cells (63). Therefore, it is likely that GnIH can directly act on the pituitary to inhibit gonadotropin synthesis and/or release from the pituitary in most birds and relatively large mammalian species (3) (Figure 1). On the other hand, GnIH may not act directly on the pituitary in some birds and rodents, as there are few or no GnIH-ir fibers in the median eminence of Rufous-winged sparrows (64), hamsters (7, 45), and rats (65). In teleost fishes, GnIH-ir fibers directly innervate the pituitary (4), which have been observed in goldfish (19), sockeye salmon (66), Indian major carp (67), sea bass (68), and tilapia (69). In the tilapia pituitary, LH cells were labeled by GnIH receptor antibody (69) (Figure 1).
Stimulatory Effects of GnIH on the HPG Axis
An electrophysiological study has shown that RFRP-3 exhibits rapid and repeatable inhibitory effects on the firing of 41% of GnRH neurons in adult mice (48). However, stimulatory effect of RFRP-3 was observed in 12% of GnRH neurons (Table 2). No stimulatory effect of RFRP-3 on the firing of GnRH neurons was observed in diestrus mice but 18% of GnRH neurons were stimulated by RFRP-3 in proestrus female mice (48).
To understand the physiological roles of GnIH in mammalian reproduction, GnIH precursor cDNA and endogenous mature peptides have been identified in the Siberian hamster brain (7). GnIH mRNA expression and number of GnIH-ir perikarya, fibers that innervate GnRH neurons are higher in long days (LD), breeding season, compared with short days (SD), non-breeding season. Intracerebroventricular (icv) administration of hamster RFRP-1 or RFRP-3 to male Siberian hamster inhibits plasma LH concentration 5 and 30 min after administration in LD but stimulates plasma LH concentration 30 min after administration in SD (7) (Table 2). It has been also shown that central chronic administration of RFRP-3 to male Syrian hamsters adapted to SD fully restores testicular weight and plasma testosterone concentration (44, 70) (Table 2).
Moussavi et al. investigated the effect of intraperitoneal (ip) administration of goldfish LPXRFa-3 on LHβ and FSHβ subunit mRNA levels in the pituitary and serum LH concentration during gonadal cycle in goldfish (71). Circulating 17β-estradiol (E2) level is very low at early gonadal recrudescence (gr), increasing at mid-gr, very high at mid-late gr, and decreasing at late gr stages. LPXRFa-3 increased LHβ and FSHβ mRNA levels at early to mid-late and late gr, respectively. However, serum LH level is decreased by LPXRFa-3 administration at early to mid gr (Table 3). Moussavi et al. further examined the effect of ip administration of LPXRFa-3 with two native goldfish GnRHs, salmon GnRH (sGnRH) and chicken GnRH (cGnRH)-II (72). Ip administration of gfLPXRF-3 alone elevated pituitary LHβ and FSHβ mRNA levels at early and mid-gr, and only FSHβ mRNA at late gr. Coadministration of LPXRFa-3 attenuated the stimulatory effect of sGnRH on LHβ in early recrudescence, and LHβ and FSHβ mRNA levels in mid and late gr, as well as cGnRH-II-elicited increase in LHβ mRNA expression at mid and late gr. Ip administration of gfLPXRF-3 reduced serum LH levels in early and mid gr (Table 3).
Ip administration of grouper GnIH-I, II, and III decreased GnRH1 mRNA level in the hypothalamus (77). However, GnRH3 mRNA level in the hypothalamus was increased by ip administration of GnIH-III. On the other hand, LHβ mRNA level in the pituitary was decreased by GnIH-II (Table 3). Ip administration of lamprey LPXRFa-2 increased GnRH-I and III content in the brain, gonadotropin β mRNA level in the pituitary [(20), Table 3]. A study in European sea bass has shown that intramuscular administration of sea bass GnIH-2 increased GnRH2 and kiss1 receptor mRNA levels in the brain (27). On the other hand, GnIH-1, 2 decreased pituitary LHβ mRNA level and plasma LH level. Plasma FSH level was only decreased by GnIH-1 (Table 3).
In addition, 48-h incubation of grass puffer pituitary with LPXRFa-1 (10−7 M) increased LHβ and FSHβ mRNA levels [(79), Table 3]. Although LH and FSH release from Cichlasoma dimerus pituitary was decreased by 24-h incubation with LPQRFa-1 (10−6 M), FSH release was increased by LPQRFa-2 (10−6 M) [(80), Table 3]. Also, 6-h incubation of Nile tilapia pituitary with pyroglutamic-LPXRFa-2 (10−7 and 10−6 M) increased LH release and pyroglutamic-LPXRFa-2 (only 10−6 M) increased FSH release [(81), Table 3].
Effect of goldfish LPXRFa-3 on gonadotropin synthesis and release was tested in dispersed goldfish pituitary cells collected at different gr stages (71). LHβ mRNA level was decreased by LPXRFa-3 (10−8 and 10−7 M) at early gr, but increased by LPXRFa-3 (10−9 M) at mid-gr, and decreased by LPXRFa-3 (10−8 and 10−7 M) at late gr. FSHβ mRNA levels was decreased by LPXRFa-3 (10−8 and 10−7 M) at early gr, by LPXRFa-3 (10−9, 10−8, 10−7 M) at mid-gr, and by LPXRFa-3 (10−7 M) at late gr. On the other hand, LH concentration in the media was increased by LPXRFa-3 (10−8 M) at late gr (Table 3). In dispersed pituitary cells of male sockeye salmon, LH release was increased by goldfish LPXRFa-1, 2 (10−7 and 10−5 M), and LPXRFa-3 (10−9 and 10−5 M). FSH release was increased by goldfish LPXRFa-1 (10−9 and 10−5 M), LPXRFa-2 (10−7, 10−5 M), and LPXRFa-3 (10−7 M) (66, Table 3).
Possible Machnism of the Stimulatory Effects of GnIH on the HPG Axis
The mechanism of GnIH (RFRP-3) effect on the electrophysiological activity of GnRH neurons was studied in transgenic mice having vesicular glutamate transporter 2 (vGluT2)-GnRH neurons (47). GnIH and RFRP-3 produced a non-desensitizing hyperpolarization with IC50 values of 34 and 37 nM, respectively, in vGluT2-GnRH neurons via a direct postsynaptic Ba2+-sensitive K+ current mechanism (Figure 1, Table 2).
It is known that E2 secreted from the ovary negatively and positively act on the hypothalamus and pituitary to regulate the HPG axis in females. However, it is also known that E2 is synthesized from androgen by aromatase neurons in the hypothalamus (82). Recent studies have shown that E2 synthesized in the brain (neuroestrogen) directly and rapidly act on GnRH neurons via membrane estrogen receptor (mER) to regulate GnRH release (83, 84). GPR30 (85, 86), ERβ (87, 88) or other membrane receptors are thought to transduce the rapid effect of E2 on GnRH release (83, 89). E2 stimulates GnRH release by increasing intracellular Ca2+ concentration (90) and electrophysiological activity of GnRH neurons (91, 92). More recently, it has been shown that GnIH neurons terminal on aromatase neurons that express GnIH receptor and increase neuroestrogen concentration in the hypothalamus by stimulating aromatase activity in quail (93, 94). Therefore, it is possible that GnIH stimulates the electrophysiological activity of some GnRH neurones (48) by increasing neuroestrogen concentration in the hypothalamus. GnIH may further stimulate LH release that was shown in hamsters (7) by stimulating the activity of aromatase neurons and increasing neuroestrogen concentration in the hypothalamus and stimulating the electrophysiological activity of GnRH neurons and GnRH release (Figure 1).
Binding of GnRH with GnRH receptor on gonadotropes results in the activation of intracellular Gαq/11 and phospholipases and generation of the second messengers, inositol 1-, 4-, 5-tris-phosphate, diacylglycerol, and arachidonic acid, which stimulate Ca2+ mobilization and PKC activity. Ca2+ mobilization initiates gonadotropin release (Figure 1). PKC activates mitogen-activated protein kinases (MAPKs) such as ERK, jun-N-terminal kinase, and p38 MAPK, which initiate the transcriptional activity of gonadotropin subunit genes (95). GnRH receptor also couples with Gαs to stimulate AC/cAMP/PKA pathway, which was shown in LβT2 cells (96) and rat gonadotropes (97). Because GnIH signaling pathway triggered by Gαi does not interfere with Gαq/11 triggered pathway, GnIH may suppress gonadotropin subunit gene transcription by inhibiting AC/cAMP/PKA pathway stimulated by GnRH receptor and Gαs (34). GnIH may also suppress gonadotropin release by hyperpolarizing gonadotropes by activating K+ channel via GnIH receptor [(47), Figure 1].
However, recent studies of GPCR have shown that GPCR not only functions as a monomer or homodimer but also as a heterodimer with different GPCR resulting in modulation of ligand binding affinity, signal transduction, and internalization of the receptors (98, 99). It has been shown that Class A GPCRs form homo- and heteromers (100). As GnRH and GnIH receptors, and GPR30 all belong to Class A GPCR family (101), it is possible that they form heteromers in GnRH neurons and/or gonadotropes to modify the action of their ligands. Some of the stimulatory effect of GnIH on the HPG axis may be due to heteromerization of GnIH and GnRH receptor and GPR30 (Figure 1).
A recent study has shown that centrally administered GnIH can decrease plasma LH concentration in ovariectomized (OVX) prepubertal female mice that were treated with E2 but not in OVX mice that were not treated with E2 (43) (Table 2). E2 can abolish intracellular free Ca2+ concentration and LH release in ovine pituitary culture induced by GnRH (102). The inhibitory effect of low concentration of E2 on LH release was shown in bovine anterior pituitary mediated by GPR30 expressed on the gonadotrope (103, 104). These results suggest the modification of GnIH action by E2 in the hypothalamus and pituitary (Figure 1).
Finally, it is known for a long time that binding of GnRH with GnRH receptors is followed by aggregation, complex formation and internalization (105). Chronic administration of GnRH or antagonist administration can desensitize pituitary gonadotropes, downregulate GnRH receptor and suppress serum LH, FSH and sex-steroid levels (106–108). It is therefore possible that chronic central administration of GnIH (RFRP-3) to male Syrian hamsters adapted to SD restores testicular weight and plasma testosterone concentration by downregulation of GnIH receptor in the hypothalamus and pituitary (44, 70) (Table 2). It is also possible that stimulatory effect of GnIH on the pituitary of fish is due to downregulation of GnIH receptor by chronic administration (79, 80), high concentration of GnIH (66, 80, 81) or antagonistic effect of LPXRFa peptides of different species (66, 79) (Table 3). Inhibitory effects of GnIH on the HPG axis are shown when GnIH peptides are tested with relatively low concentrations in a shorter time frame (73–76) (Tables 2 and 3; Figure 1).
Complex mechanism may be involved in in vivo studies that show stimulatory and inhibitory effects of GnIH on the HPG axis in addition to downregulation of receptors and changes in the number of receptors depending on reproductive and developmental stages and endogenous sex-steroid levels (Tables 2 and 3; Figure 1). It is also important to note that GnIH peptides are produced in gonads (3, 109) and it has been shown that they have direct effects on gonadal activates in mammals (110–114), birds (115–117) and fishes (118). Most of these studies showed inhibitory effects of GnIH peptides on gonadal activities, but stimulatory activity of GnIH peptides was also shown in mouse ovary (114) and goldfish testis (118). Therefore, in vivo studies that showed effects of GnIH peptides on gonadal activates (Tables 2 and 3) may include direct effects of GnIH peptides on the gonads.
Conclusion
Gonadotropin-inhibitory hormone orthologous peptides have a characteristic LPXRFamide C-terminal motif in most vertebrate species, which is critical for receptor binding. The primary receptor for GnIH is GPR147 that inhibits cAMP production in target cells. GnIH generally decreases gonadotropin synthesis and release by directly acting on the gonadotrope or by decreasing the activity of GnRH neurons. However, one study shows stimulatory effects of GnIH on the electrophysiological activity of some GnRH neurons in mice (48). Stimulatory effect of GnIH on GnRH neurons in the hypothalamus may be explained by the action of neuroestrogen synthesized in the hypothalamus by the stimulatory action of GnIH on aromatase neurons that terminate on GnRH neurons that express estrogen membrane receptor. GnIH may further stimulate LH release that was shown in hamsters by stimulating the electrophysiological activity of GnRH neurons and GnRH release (7, 44). Peripheral sex-steroid levels may also modify the action of GnIH (7, 44, 71, 72). Some of the stimulatory effects of GnIH on the HPG axis may be due to heteromerization of GnIH and GnRH receptors and GPR30 in GnRH neurons and/or gonadotropes, which modifies ligand binding and signaling transduction mechanism. Stimulatory effect of GnIH on the HPG axis may also be due to internalization of GnIH receptor by high concentration or chronic administration of GnIH or antagonistic effect of the peptides administered (20, 66, 77, 79–81). Besides pharmacological effect of administered peptides, the general inhibitory action of GnIH by decreasing cAMP concentration and inducing hyperpolarization in target cells and the additional stimulatory action of GnIH by neuroestrogen synthesis, receptor heteromerization, and internalization may have a physiological role to maintain reproductive homeostasis according to developmental and reproductive stages.
Author Contributions
TU wrote the manuscript and IP edited the manuscript.
Conflict of Interest Statement
The authors declare that the research was conducted in the absence of any commercial or financial relationships that could be construed as a potential conflict of interest.
References
1. Tsutsui K, Saigoh E, Ukena K, Teranishi H, Fujisawa Y, Kikuchi M, et al. A novel avian hypothalamic peptide inhibiting gonadotropin release. Biochem Biophys Res Commun (2000) 275:661–7. doi:10.1006/bbrc.2000.3350
2. Ubuka T, Ukena K, Sharp PJ, Bentley GE, Tsutsui K. Gonadotropin-inhibitory hormone inhibits gonadal development and maintenance by decreasing gonadotropin synthesis and release in male quail. Endocrinology (2006) 147:1187–94. doi:10.1210/en.2005-1178
3. Ubuka T, Son YL, Tsutsui K. Molecular, cellular, morphological, physiological and behavioral aspects of gonadotropin-inhibitory hormone. Gen Comp Endocrinol (2016) 227:27–50. doi:10.1016/j.ygcen.2015.09.009
4. Muñoz-Cueto JA, PaulladaSalmerón JA, Aliaga-Guerrero M, Cowan ME, Parhar IS, Ubuka T. A journey through the gonadotropin-inhibitory hormone system of fish. Front Endocrinol (2017) 8:285. doi:10.3389/fendo.2017.00285
5. Ubuka T, Morgan K, Pawson AJ, Osugi T, Chowdhury VS, Minakata H, et al. Identification of human GnIH homologs, RFRP-1 and RFRP-3, and the cognate receptor, GPR147 in the human hypothalamic pituitary axis. PLoS One (2009) 4:e8400. doi:10.1371/journal.pone.0008400
6. Ubuka T, Lai H, Kitani M, Suzuuchi A, Pham V, Cadigan PA, et al. Gonadotropin-inhibitory hormone identification, cDNA cloning, and distribution in rhesus macaque brain. J Comp Neurol (2009) 517:841–55. doi:10.1002/cne.22191
7. Ubuka T, Inoue K, Fukuda Y, Mizuno T, Ukena K, Kriegsfeld LJ, et al. Identification, expression, and physiological functions of Siberian hamster gonadotropin-inhibitory hormone. Endocrinology (2012) 153:373–85. doi:10.1210/en.2011-1110
8. Ukena K, Iwakoshi E, Minakata H, Tsutsui K. A novel rat hypothalamic RFamide-related peptide identified by immunoaffinity chromatography and mass spectrometry. FEBS Lett (2002) 512:255–8. doi:10.1016/S0014-5793(02)02275-5
9. Fukusumi S, Habata Y, Yoshida H, Iijima N, Kawamata Y, Hosoya M, et al. Characteristics and distribution of endogenous RFamide-related peptide-1. Biochim Biophys Acta (2001) 1540:221–32. doi:10.1016/S0167-4889(01)00135-5
10. Yoshida H, Habata Y, Hosoya M, Kawamata Y, Kitada C, Hinuma S. Molecular properties of endogenous RFamide-related peptide-3 and its interaction with receptors. Biochim Biophys Acta (2003) 1593:151–7. doi:10.1016/S0167-4889(02)00389-0
11. Ubuka T, Kim S, Huang YC, Reid J, Jiang J, Osugi T, et al. Gonadotropin-inhibitory hormone neurons interact directly with gonadotropin-releasing hormone-I and -II neurons in European starling brain. Endocrinology (2008) 149:268–78. doi:10.1210/en.2007-0983
12. Tobari Y, Iijima N, Tsunekawa K, Osugi T, Okanoya K, Tsutsui K, et al. Identification of gonadotropin-inhibitory hormone in the zebra finch (Taeniopygia guttata): peptide isolation, cDNA cloning and brain distribution. Peptides (2010) 31:816–26. doi:10.1016/j.peptides.2010.01.015
13. McConn B, Wang G, Yi J, Gilbert ER, Osugi T, Ubuka T, et al. Gonadotropin-inhibitory hormone-stimulation of food intake is mediated by hypothalamic effects in chicks. Neuropeptides (2014) 48:327–34. doi:10.1016/j.npep.2014.09.001
14. Satake H, Hisada M, Kawada T, Minakata H, Ukena K, Tsutsui K. Characterization of a cDNA encoding a novel avian hypothalamic neuropeptide exerting an inhibitory effect on gonadotropin release. Biochem J (2001) 354:379–85. doi:10.1042/bj3540379
15. Ukena K, Iwakoshi-Ukena E, Osugi T, Tsutsui K. Identification and localization of gonadotropin-inhibitory hormone (GnIH) orthologs in the hypothalamus of the red-eared slider turtle, Trachemys scripta elegans. Gen Comp Endocrinol (2016) 227:69–76. doi:10.1016/j.ygcen.2015.06.009
16. Chartrel N, Dujardin C, Leprince J, Desrues L, Tonon MC, Cellier E, et al. Isolation, characterization, and distribution of a novel neuropeptide, Rana RFamide (R-RFa), in the brain of the European green frog Rana esculenta. J Comp Neurol (2002) 448:111–27. doi:10.1002/cne.10253
17. Ukena K, Koda A, Yamamoto K, Kobayashi T, Iwakoshi-Ukena E, Minakata H, et al. Novel neuropeptides related to frog growth hormone-releasing peptide: isolation, sequence, and functional analysis. Endocrinology (2003) 144:3879–84. doi:10.1210/en.2003-0359
18. Chowdhury VS, Ubuka T, Osugi T, Shimura T, Tsutsui K. Identification, localization and expression of LPXRFamide peptides, and melatonin-dependent induction of their precursor mRNA in the newt brain. J Endocrinol (2011) 209:211–20. doi:10.1530/JOE-10-0494
19. Sawada K, Ukena K, Satake H, Iwakoshi E, Minakata H, Tsutsui K. Novel fish hypothalamic neuropeptide. Eur J Biochem (2002) 269:6000–8. doi:10.1046/j.1432-1033.2002.03351.x
20. Osugi T, Daukss D, Gazda K, Ubuka T, Kosugi T, Nozaki M, et al. Evolutionary origin of the structure and function of gonadotropin-inhibitory hormone: insights from lampreys. Endocrinology (2012) 153:2362–74. doi:10.1210/en.2011-2046
21. Osugi T, Okamura T, Son YL, Ohkubo M, Ubuka T, Henmi Y, et al. Evolutionary origin of GnIH and NPFF in chordates: insights from novel amphioxus RFamide peptides. PLoS One (2014) 9:e100962. doi:10.1371/journal.pone.0100962
22. Yin H, Ukena K, Ubuka T, Tsutsui K. A novel G protein-coupled receptor for gonadotropin-inhibitory hormone in the Japanese quail (Coturnix japonica): identification, expression and binding activity. J Endocrinol (2005) 184:257–66. doi:10.1677/joe.1.05926
23. Thorson JF, Prezotto LD, Cardoso RC, Sharpton SM, Edwards JF, Welsh TH Jr, et al. Hypothalamic distribution, adenohypophyseal receptor expression, and ligand functionality of RFamide-related peptide 3 in the mare during the breeding and nonbreeding seasons. Biol Reprod (2014) 90:28. doi:10.1095/biolreprod.113.112185
24. Ikemoto T, Park MK. Chicken RFamide-related peptide (GnIH) and two distinct receptor subtypes: identification, molecular characterization, and evolutionary considerations. J Reprod Dev (2005) 51:359–77. doi:10.1262/jrd.16087
25. Ubuka T, Ueno M, Ukena K, Tsutsui K. Developmental changes in gonadotropin-inhibitory hormone in the Japanese quail (Coturnix japonica) hypothalamo-hypophysial system. J Endocrinol (2003) 178:311–8. doi:10.1677/joe.0.1780311
26. Koda A, Ukena K, Teranishi H, Ohta S, Yamamoto K, Kikuyama S, et al. A novel amphibian hypothalamic neuropeptide: isolation, localization, and biological activity. Endocrinology (2002) 143:411–9. doi:10.1210/endo.143.2.8630
27. Paullada-Salmerón JA, Cowan M, Aliaga-Guerrero M, López-Olmeda JF, Mañanós EL, Zanuy S, et al. Testicular Steroidogenesis and Locomotor Activity Are Regulated by Gonadotropin-Inhibitory Hormone in Male European Sea Bass. PLoS One (2016) 11:e0165494. doi:10.1371/journal.pone.0165494
28. Hinuma S, Shintani Y, Fukusumi S, Iijima N, Matsumoto Y, Hosoya M, et al. New neuropeptides containing carboxy-terminal RFamide and their receptor in mammals. Nat Cell Biol (2000) 2:703–8. doi:10.1038/35036326
29. Bonini JA, Jones KA, Adham N, Forray C, Artymyshyn R, Durkin MM, et al. Identification and characterization of two G protein-coupled receptors for neuropeptide FF. J Biol Chem (2000) 275:39324–31. doi:10.1074/jbc.M004385200
30. Fredriksson R, Lagerström MC, Lundin LG, Schiöth HB. The G-protein-coupled receptors in the human genome form five main families. Phylogenetic analysis, paralogon groups, and fingerprints. Mol Pharmacol (2003) 63:1256–72. doi:10.1124/mol.63.6.1256
31. Liu Q, Guan XM, Martin WJ, McDonald TP, Clements MK, Jiang Q, et al. Identification and characterization of novel mammalian neuropeptide FF-like peptides that attenuate morphine-induced antinociception. J Biol Chem (2001) 276:36961–9. doi:10.1074/jbc.M105308200
32. Ubuka T, Tsutsui K. Evolution of gonadotropin-inhibitory hormone receptor and its ligand. Gen Comp Endocrinol (2014) 209:148–61. doi:10.1016/j.ygcen.2014.09.002
33. Shimizu M, Bédécarrats GY. Activation of the chicken gonadotropin-inhibitory hormone receptor reduces gonadotropin releasing hormone receptor signaling. Gen Comp Endocrinol (2010) 167:331–7. doi:10.1016/j.ygcen.2010.03.029
34. Son YL, Ubuka T, Millar RP, Kanasaki H, Tsutsui K. Gonadotropin-inhibitory hormone inhibits GnRH-induced gonadotropin subunit gene transcriptions by inhibiting AC/cAMP/PKA-dependent ERK pathway in LβT2 cells. Endocrinology (2012) 153:2332–43. doi:10.1210/en.2011-1904
35. George JT, Hendrikse M, Veldhuis JD, Clarke IJ, Anderson RA, Millar RP. Effect of gonadotropin-inhibitory hormone on luteinizing hormone secretion in humans. Clin Endocrinol (2017) 86:731–8. doi:10.1111/cen.13308
36. Clarke IJ, Smith JT, Henry BA, Oldfield BJ, Stefanidis A, Millar RP, et al. Gonadotropin-inhibitory hormone is a hypothalamic peptide that provides a molecular switch between reproduction and feeding. Neuroendocrinology (2012) 95:305–16. doi:10.1159/000332822
37. Smith JT, Young IR, Veldhuis JD, Clarke IJ. Gonadotropin-inhibitory hormone (GnIH) secretion into the ovine hypophyseal portal system. Endocrinology (2012) 153:3368–75. doi:10.1210/en.2012-1088
38. Kadokawa H, Shibata M, Tanaka Y, Kojima T, Matsumoto K, Oshima K, et al. Bovine C-terminal octapeptide of RFamide-related peptide-3 suppresses luteinizing hormone (LH) secretion from the pituitary as well as pulsatile LH secretion in bovines. Domest Anim Endocrinol (2009) 36:219–24. doi:10.1016/j.domaniend.2009.02.001
39. Johnson MA, Tsutsui K, Fraley GS. Rat RFamide-related peptide-3 stimulates GH secretion, inhibits LH secretion, and has variable effects on sex behavior in the adult male rat. Horm Behav (2007) 51:171–80. doi:10.1016/j.yhbeh.2006.09.009
40. Pineda R, Garcia-Galiano D, Sanchez-Garrido MA, Romero M, Ruiz-Pino F, Aguilar E, et al. Characterization of the inhibitory roles of RFRP3, the mammalian ortholog of GnIH, in the control of gonadotropin secretion in the rat: in vivo and in vitro studies. Am J Physiol Endocrinol Metab (2010) 299:E39–46. doi:10.1152/ajpendo.00108.2010
41. Murakami M, Matsuzaki T, Iwasa T, Yasui T, Irahara M, Osugi T, et al. Hypophysiotropic role of RFamide-related peptide-3 in the inhibition of LH secretion in female rats. J Endocrinol (2008) 199:105–12. doi:10.1677/JOE-08-0197
42. Anderson GM, Relf HL, Rizwan MZ, Evans JJ. Central and peripheral effects of RFamide-related peptide-3 on luteinizing hormone and prolactin secretion in rats. Endocrinology (2009) 150:1834–40. doi:10.1210/en.2008-1359
43. Xiang W, Zhang B, Lv F, Ma Y, Chen H, Chen L, et al. The inhibitory effects of RFamide-related peptide 3 on luteinizing hormone release involves an estradiol-dependent manner in prepubertal but not in adult female mice. Biol Reprod (2015) 93:30. doi:10.1095/biolreprod.115.128777
44. Ancel C, Bentsen AH, Sébert ME, Tena-Sempere M, Mikkelsen JD, Simonneaux V. Stimulatory effect of RFRP-3 on the gonadotrophic axis in the male Syrian hamster: the exception proves the rule. Endocrinology (2012) 153:1352–63. doi:10.1210/en.2011-1622
45. Kriegsfeld LJ, Mei DF, Bentley GE, Ubuka T, Mason AO, Inoue K, et al. Identification and characterization of a gonadotropin-inhibitory system in the brains of mammals. Proc Natl Acad Sci U S A (2006) 103:2410–5. doi:10.1073/pnas.0511003103
46. Son YL, Ubuka T, Soga T, Yamamoto K, Bentley GE, Tsutsui K. Inhibitory action of gonadotropin-inhibitory hormone on the signaling pathways induced by kisspeptin and vasoactive intestinal polypeptide in GnRH neuronal cell line, GT1-7. FASEB J (2016) 30:2198–210. doi:10.1096/fj.201500055
47. Wu M, Dumalska I, Morozova E, van den Pol AN, Alreja M. Gonadotropin inhibitory hormone inhibits basal forebrain vGluT2-gonadotropin-releasing hormone neurons via a direct postsynaptic mechanism. J Physiol (2009) 587:1401–11. doi:10.1113/jphysiol.2008.166447
48. Ducret E, Anderson GM, Herbison AE. RFamide-related peptide-3, a mammalian gonadotropin-inhibitory hormone ortholog, regulates gonadotropin-releasing hormone neuron firing in the mouse. Endocrinology (2009) 150:2799–804. doi:10.1210/en.2008-1623
49. Gojska NM, Friedman Z, Belsham DD. Direct regulation of gonadotrophin-releasing hormone (GnRH) transcription by RF-amide-related peptide-3 and kisspeptin in a novel GnRH-secreting cell line, mHypoA-GnRH/GFP. J Neuroendocrinol (2014) 26:888–97. doi:10.1111/jne.12225
50. Clarke IJ, Sari IP, Qi Y, Smith JT, Parkington HC, Ubuka T, et al. Potent action of RFamide-related peptide-3 on pituitary gonadotropes indicative of a hypophysiotropic role in the negative regulation of gonadotropin secretion. Endocrinology (2008) 149:5811–21. doi:10.1210/en.2008-0575
51. Sari IP, Rao A, Smith JT, Tilbrook AJ, Clarke IJ. Effect of RF-amide-related peptide-3 on luteinizing hormone and follicle-stimulating hormone synthesis and secretion in ovine pituitary gonadotropes. Endocrinology (2009) 150:5549–56. doi:10.1210/en.2009-0775
52. Bentley GE, Perfito N, Ukena K, Tsutsui K, Wingfield JC. Gonadotropin-inhibitory peptide in song sparrows (Melospiza melodia) in different reproductive conditions, and in house sparrows (Passer domesticus) relative to chicken-gonadotropin-releasing hormone. J Neuroendocrinol (2003) 15:794–802. doi:10.1046/j.1365-2826.2003.01062.x
53. Ubuka T, Bentley GE. Identification, localization, and regulation of passerine GnRH-I messenger RNA. J Endocrinol (2009) 201:81–7. doi:10.1677/JOE-08-0508
54. Ubuka T, Bentley GE. Neuroendocrine control of reproduction in birds. In: Norris DO, Lopez KH, editors. Hormones and Reproduction of Vertebrates, Vol. 4. Birds. Academic Press (2010). p. 1–25.
55. Ubuka T, Cadigan PA, Wang A, Liu J, Bentley GE. Identification of European starling GnRH-I precursor mRNA and its seasonal regulation. Gen Comp Endocrinol (2009) 162:301–6. doi:10.1016/j.ygcen.2009.04.001
56. Qi Y, Oldfield BJ, Clarke IJ. Projections of RFamide-related peptide-3 neurones in the ovine hypothalamus, with special reference to regions regulating energy balance and reproduction. J Neuroendocrinol (2009) 21:690–7. doi:10.1111/j.1365-2826.2009.01886.x
57. Soga T, Kitahashi T, Clarke IJ, Parhar IS. Gonadotropin-inhibitory hormone promoter-driven enhanced green fluorescent protein expression decreases during aging in female rats. Endocrinology (2014) 155:1944–55. doi:10.1210/en.2013-1786
58. Rizwan MZ, Poling MC, Corr M, Cornes PA, Augustine RA, Quennell JH, et al. RFamide-related peptide-3 receptor gene expression in GnRH and kisspeptin neurons and GnRH-dependent mechanism of action. Endocrinology (2012) 153:3770–9. doi:10.1210/en.2012-1133
59. Pinelli C, Jadhao AG, Biswas SP, Tsutsui K, D’Aniello B. Neuroanatomical organization of the brain gonadotropin-inhibitory hormone and gonadotropin-releasing hormone systems in the frog Pelophylax esculentus. Brain Behav Evol (2015) 85:15–28. doi:10.1159/000368594
60. Spicer OS, Zmora N, Wong TT, Golan M, Levavi-Sivan B, Gothilf Y, et al. The gonadotropin-inhibitory hormone (Lpxrfa) system’s regulation of reproduction in the brain-pituitary axis of the zebrafish (Danio rerio). Biol Reprod (2017) 96:1031–42. doi:10.1093/biolre/iox032
61. Ukena K, Ubuka T, Tsutsui K. Distribution of a novel avian gonadotropin-inhibitory hormone in the quail brain. Cell Tissue Res (2003) 312:73–9. doi:10.1007/s00441-003-0700-x
62. Osugi T, Ukena K, Bentley GE, O’Brien S, Moore IT, Wingfield JC, et al. Gonadotropin-inhibitory hormone in Gambel’s white-crowned sparrow (Zonotrichia leucophrys gambelii): cDNA identification, transcript localization and functional effects in laboratory and field experiments. J Endocrinol (2004) 182:33–42. doi:10.1677/joe.0.1820033
63. Maddineni S, Ocón-Grove OM, Krzysik-Walker SM, Hendricks GL III, Proudman JA, Ramachandran R. Gonadotrophin-inhibitory hormone receptor expression in the chicken pituitary gland: potential influence of sexual maturation and ovarian steroids. J Neuroendocrinol (2008) 20:1078–88. doi:10.1111/j.1365-2826.2008.01765.x
64. Small TW, Sharp PJ, Bentley GE, Millar RP, Tsutsui K, Mura E, et al. Photoperiod-independent hypothalamic regulation of luteinizing hormone secretion in a free-living Sonoran desert bird, the Rufous-winged Sparrow (Aimophila carpalis). Brain Behav Evol (2008) 71:127–42. doi:10.1159/000111459
65. Rizwan MZ, Porteous R, Herbison AE, Anderson GM. Cells expressing RFamide-related peptide-1/3, the mammalian gonadotropin-inhibitory hormone orthologs, are not hypophysiotropic neuroendocrine neurons in the rat. Endocrinology (2009) 150:1413–20. doi:10.1210/en.2008-1287
66. Amano M, Moriyama S, Iigo M, Kitamura S, Amiya N, Yamamori K, et al. Novel fish hypothalamic neuropeptides stimulate the release of gonadotrophins and growth hormone from the pituitary of sockeye salmon. J Endocrinol (2006) 188:417–23. doi:10.1677/joe.1.06494
67. Biswas S, Jadhao AG, Pinelli C, Palande NV, Tsutsui K. GnIH and GnRH expressions in the central nervous system and pituitary of Indian major carp, Labeo rohita during ontogeny: an immunocytochemical study. Gen Comp Endocrinol (2015) 220:88–92. doi:10.1016/j.ygcen.2014.06.005
68. Paullada-Salmerón JA, Cowan M, Aliaga-Guerrero M, Gómez A, Zanuy S, Mañanos E, et al. LPXRFa peptide system in the European sea bass: a molecular and immunohistochemical approach. J Comp Neurol (2016) 524:176–98. doi:10.1002/cne.23833
69. Ogawa S, Sivalingam M, Biran J, Golan M, Anthonysamy RS, Levavi-Sivan B, et al. Distribution of LPXRFa, a gonadotropin-inhibitory hormone ortholog peptide, and LPXRFa receptor in the brain and pituitary of the tilapia. J Comp Neurol (2016) 524:2753–75. doi:10.1002/cne.23990
70. Henningsen JB, Gauer F, Simonneaux V. RFRP neurons – the doorway to understanding seasonal reproduction in mammals. Front Endocrinol (2016) 7:36. doi:10.3389/fendo.2016.00036
71. Moussavi M, Wlasichuk M, Chang JP, Habibi HR. Seasonal effect of GnIH on gonadotrope functions in the pituitary of goldfish. Mol Cell Endocrinol (2012) 350:53–60. doi:10.1016/j.mce.2011.11.020
72. Moussavi M, Wlasichuk M, Chang JP, Habibi HR. Seasonal effect of gonadotrophin inhibitory hormone on gonadotrophin-releasing hormone-induced gonadotroph functions in the goldfish pituitary. J Neuroendocrinol (2013) 25:506–16. doi:10.1111/jne.12024
73. Paullada-Salmerón JA, Cowan M, Aliaga-Guerrero M, Morano F, Zanuy S, Muñoz-Cueto JA. Gonadotropin Inhibitory Hormone Down-Regulates the Brain-Pituitary Reproductive Axis of Male European Sea Bass (Dicentrarchus labrax). Biol Reprod (2016) 94:121. doi:10.1095/biolreprod.116.139022
74. Zhang Y, Li S, Liu Y, Lu D, Chen H, Huang X, et al. Structural diversity of the GnIH/GnIH receptor system in teleost: its involvement in early development and the negative control of LH release. Peptides (2010) 31:1034–43. doi:10.1016/j.peptides.2010.03.003
75. Qi X, Zhou W, Li S, Lu D, Yi S, Xie R, et al. Evidences for the regulation of GnRH and GTH expression by GnIH in the goldfish, Carassius auratus. Mol Cell Endocrinol (2013) 366:9–20. doi:10.1016/j.mce.2012.11.001
76. Choi YJ, Kim NN, Habibi HR, Choi CY. Effects of gonadotropin inhibitory hormone or gonadotropin-releasing hormone on reproduction-related genes in the protandrous cinnamon clownfish, Amphiprion melanopus. Gen Comp Endocrinol (2016) 235:89–99. doi:10.1016/j.ygcen.2016.06.010
77. Wang Q, Qi X, Guo Y, Li S, Zhang Y, Liu X, et al. Molecular identification of GnIH/GnIHR signal and its reproductive function in protogynous hermaphroditic orange-spotted grouper (Epinephelus coioides). Gen Comp Endocrinol (2015) 216:9–23. doi:10.1016/j.ygcen.2015.04.016
78. Aliaga-Guerrero M, Paullada-Salmerón JA, Piquer V, Mañanós EL, Muñoz-Cueto JA. Gonadotropin-inhibitory hormone in the flatfish, Solea senegalensis: molecular cloning, brain localization and physiological effects. J Comp Neurol (2017) 526(2):349–70. doi:10.1002/cne.24339
79. Shahjahan M, Ikegami T, Osugi T, Ukena K, Doi H, Hattori A, et al. Synchronised expressions of LPXRFamide peptide and its receptor genes: seasonal, diurnal and circadian changes during spawning period in grass puffer. J Neuroendocrinol (2011) 23:39–51. doi:10.1111/j.1365-2826.2010.02081.x
80. Di Yorio MP, Pérez Sirkin DI, Delgadin TH, Shimizu A, Tsutsui K, Somoza GM, et al. Gonadotrophin-inhibitory hormone in the cichlid fish cichlasoma dimerus: structure, brain distribution and differential effects on the secretion of gonadotrophins and growth hormone. J Neuroendocrinol (2016) 28:12377. doi:10.1111/jne.12377
81. Biran J, Golan M, Mizrahi N, Ogawa S, Parhar IS, Levavi-Sivan B. LPXRFa, the piscine ortholog of GnIH, and LPXRF receptor positively regulate gonadotropin secretion in Tilapia (Oreochromis niloticus). Endocrinology (2014) 155:4391–401. doi:10.1210/en.2013-2047
82. Naftolin F, Ryan KJ, Petro Z. Aromatization of androstenedione by the diencephalon. J Clin Endocrinol Metab (1971) 33:368–70. doi:10.1210/jcem-33-2-368
83. Terasawa E, Kenealy BP. Neuroestrogen, rapid action of estradiol, and GnRH neurons. Front Neuroendocrinol (2012) 33:364–75. doi:10.1016/j.yfrne.2012.08.001
84. Kenealy BP, Kapoor A, Guerriero KA, Keen KL, Garcia JP, Kurian JR, et al. Neuroestradiol in the hypothalamus contributes to the regulation of gonadotropin releasing hormone release. J Neurosci (2013) 33:19051–9. doi:10.1523/JNEUROSCI.3878-13.2013
85. Filardo EJ, Quinn JA, Bland KI, Frackelton AR Jr. Estrogen-induced activation of Erk-1 and Erk-2 requires the G protein-coupled receptor homolog, GPR30, and occurs via trans-activation of the epidermal growth factor receptor through release of HB-EGF. Mol Endocrinol (2000) 14:1649–60. doi:10.1210/mend.14.10.0532
86. Filardo EJ, Quinn JA, Frackelton AR Jr, Bland KI. Estrogen action via the G protein-coupled receptor, GPR30: stimulation of adenylyl cyclase and cAMP-mediated attenuation of the epidermal growth factor receptor-to-MAPK signaling axis. Mol Endocrinol (2002) 16:70–84. doi:10.1210/mend.16.1.0758
87. Kuiper GG, Enmark E, Pelto-Huikko M, Nilsson S, Gustafsson JA. Cloning of a novel receptor expressed in rat prostate and ovary. Proc Natl Acad Sci U S A (1996) 93:5925–30. doi:10.1073/pnas.93.12.5925
88. Hrabovszky E, Shughrue PJ, Merchenthaler I, Hajszán T, Carpenter CD, Liposits Z, et al. Detection of estrogen receptor-beta messenger ribonucleic acid and 125I-estrogen binding sites in luteinizing hormone-releasing hormone neurons of the rat brain. Endocrinology (2000) 141:3506–9. doi:10.1210/endo.141.9.7788
89. Noel SD, Keen KL, Baumann DI, Filardo EJ, Terasawa E. Involvement of G protein-coupled receptor 30 (GPR30) in rapid action of estrogen in primate LHRH neurons. Mol Endocrinol (2009) 23:349–59. doi:10.1210/me.2008-0299
90. Abe H, Keen KL, Terasawa E. Rapid action of estrogens on intracellular calcium oscillations in primate luteinizing hormone-releasing hormone-1 neurons. Endocrinology (2008) 149:1155–62. doi:10.1210/en.2007-0942
91. Abe H, Terasawa E. Firing pattern and rapid modulation of activity by estrogen in primate luteinizing hormone releasing hormone-1 neurons. Endocrinology (2005) 146:4312–20. doi:10.1210/en.2005-0435
92. Chu Z, Andrade J, Shupnik MA, Moenter SM. Differential regulation of gonadotropin-releasing hormone neuron activity and membrane properties by acutely applied estradiol: dependence on dose and estrogen receptor subtype. J Neurosci (2009) 29:5616–27. doi:10.1523/JNEUROSCI.0352-09.2009
93. Ubuka T, Haraguchi S, Tobari Y, Narihiro M, Ishikawa K, Hayashi T, et al. Hypothalamic inhibition of socio-sexual behaviour by increasing neuroestrogen synthesis. Nat Commun (2014) 5:3061. doi:10.1038/ncomms4061
94. Ubuka T, Tsutsui K. Review: neuroestrogen regulation of socio-sexual behavior of males. Front Neurosci (2014) 8:323. doi:10.3389/fnins.2014.00323
95. Naor Z. Signaling by G-protein-coupled receptor (GPCR): studies on the GnRH receptor. Front Neuroendocrinol (2009) 30:10–29. doi:10.1016/j.yfrne.2008.07.001
96. Liu F, Usui I, Evans LG, Austin DA, Mellon PL, Olefsky JM, et al. Involvement of both G(q/11) and G(s) proteins in gonadotropin-releasing hormone receptor-mediated signaling in L beta T2 cells. J Biol Chem (2002) 277:32099–108. doi:10.1074/jbc.M203639200
97. Stanislaus D, Ponder S, Ji TH, Conn PM. Gonadotropin-releasing hormone receptor couples to multiple G proteins in rat gonadotrophs and in GGH3 cells: evidence from palmitoylation and overexpression of G proteins. Biol Reprod (1998) 59:579–86. doi:10.1095/biolreprod59.3.579
98. Ferré S, Baler R, Bouvier M, Caron MG, Devi LA, Durroux T, et al. Building a new conceptual framework for receptor heteromers. Nat Chem Biol (2009) 5:131–4. doi:10.1038/nchembio0309-131
99. Satake H, Matsubara S, Aoyama M, Kawada T, Sakai T. GPCR heterodimerization in the reproductive system: functional regulation and implication for biodiversity. Front Endocrinol (2013) 4:100. doi:10.3389/fendo.2013.00100
100. Ferré S, Casadó V, Devi LA, Filizola M, Jockers R, Lohse MJ, et al. G protein-coupled receptor oligomerization revisited: functional and pharmacological perspectives. Pharmacol Rev (2014) 66:413–34. doi:10.1124/pr.113.008052
101. Kakarala KK, Jamil K. Sequence-structure based phylogeny of GPCR Class A Rhodopsin receptors. Mol Phylogenet Evol (2014) 74:66–96. doi:10.1016/j.ympev.2014.01.022
102. Iqbal J, Latchoumanin O, Sari IP, Lang RJ, Coleman HA, Parkington HC, et al. Estradiol-17beta inhibits gonadotropin-releasing hormone-induced Ca2+ in gonadotropes to regulate negative feedback on luteinizing hormone release. Endocrinology (2009) 150:4213–20. doi:10.1210/en.2009-0092
103. Rudolf FO, Kadokawa H. Expression of estradiol receptor, GPR30, in bovine anterior pituitary and effects of GPR30 agonist on GnRH-induced LH secretion. Anim Reprod Sci (2013) 139(1–4):9–17. doi:10.1016/j.anireprosci.2013.04.003
104. Rudolf FO, Kadokawa H. Cytoplasmic kinases downstream of GPR30 suppress gonadotropin-releasing hormone (GnRH)-induced luteinizing hormone secretion from bovine anterior pituitary cells. J Reprod Dev (2016) 62:65–9. doi:10.1262/jrd.2015-104
105. Conn PM, Staley D, Harris C, Andrews WV, Gorospe WC, McArdle CA, et al. Mechanism of action of gonadotropin releasing hormone. Annu Rev Physiol (1986) 48:495–513. doi:10.1146/annurev.ph.48.030186.002431
106. Katt JA, Duncan JA, Herbon L, Barkan A, Marshall JC. The frequency of gonadotropin-releasing hormone stimulation determines the number of pituitary gonadotropin-releasing hormone receptors. Endocrinology (1985) 116:2113–5. doi:10.1210/endo-116-5-2113
107. Conn PM, Crowley WF Jr. Gonadotropin-releasing hormone and its analogues. N Engl J Med (1991) 324:93–103. doi:10.1056/NEJM199101103240205
108. Halmos G, Schally AV. Changes in subcellular distribution of pituitary receptors for luteinizing hormone-releasing hormone (LH-RH) after treatment with the LH-RH antagonist cetrorelix. Proc Natl Acad Sci U S A (2002) 99:961–5. doi:10.1073/pnas.012598399
109. Bentley GE, Ubuka T, McGuire NL, Chowdhury VS, Morita Y, Yano T, et al. Gonadotropin-inhibitory hormone and its receptor in the avian reproductive system. Gen Comp Endocrinol (2008) 156:34–43. doi:10.1016/j.ygcen.2007.10.003
110. Singh P, Krishna A, Tsutsui K. Effects of gonadotropin-inhibitory hormone on folliculogenesis and steroidogenesis of cyclic mice. Fertil Steril (2011) 95:1397–404. doi:10.1016/j.fertnstert.2010.03.052
111. Oishi H, Klausen C, Bentley GE, Osugi T, Tsutsui K, Gilks CB, et al. The human gonadotropin-inhibitory hormone ortholog RFamide-related peptide-3 suppresses gonadotropin-induced progesterone production in human granulosa cells. Endocrinology (2012) 153:3435–45. doi:10.1210/en.2012-1066
112. Anjum S, Krishna A, Tsutsui K. Inhibitory roles of the mammalian GnIH ortholog RFRP3 in testicular activities in adult mice. J Endocrinol (2014) 223:79–91. doi:10.1530/JOE-14-0333
113. Zheng L, Su J, Fang R, Jin M, Lei Z, Hou Y, et al. Developmental changes in the role of gonadotropin-inhibitory hormone (GnIH) and its receptors in the reproductive axis of male Xiaomeishan pigs. Anim Reprod Sci (2015) 154:113–20. doi:10.1016/j.anireprosci.2015.01.004
114. Dave A, Krishna A, Tsutsui K. Direct effects of RFRP-1, a mammalian GnIH ortholog, on ovarian activities of the cyclic mouse. Gen Comp Endocrinol (2017) 252:193–9. doi:10.1016/j.ygcen.2017.06.024
115. Maddineni SR, Ocón-Grove OM, Krzysik-Walker SM, Hendricks GL III, Ramachandran R. Gonadotropin-inhibitory hormone (GnIH) receptor gene is expressed in the chicken ovary: potential role of GnIH in follicular maturation. Reproduction (2008) 135:267–74. doi:10.1530/REP-07-0369
116. McGuire NL, Bentley GE. A functional neuropeptide system in vertebrate gonads: gonadotropin-inhibitory hormone and its receptor in testes of field-caught house sparrow (Passer domesticus). Gen Comp Endocrinol (2010) 166:565–72. doi:10.1016/j.ygcen.2010.01.010
117. McGuire NL, Kangas K, Bentley GE. Effects of melatonin on peripheral reproductive function: regulation of testicular GnIH and testosterone. Endocrinology (2011) 152:3461–70. doi:10.1210/en.2011-1053
Keywords: gonadotropin-releasing hormone, GPR147, aromatase, neuroestrogen, GPR30, receptor heteromerization, receptor internalization, sex steroids
Citation: Ubuka T and Parhar I (2018) Dual Actions of Mammalian and Piscine Gonadotropin-Inhibitory Hormones, RFamide-Related Peptides and LPXRFamide Peptides, in the Hypothalamic–Pituitary–Gonadal Axis. Front. Endocrinol. 8:377. doi: 10.3389/fendo.2017.00377
Received: 21 November 2017; Accepted: 22 December 2017;
Published: 11 January 2018
Edited by:
Honoo Satake, Suntory Foundation for Life Sciences, JapanReviewed by:
Gregoy Y. Bedecarrats, University of Guelph, CanadaKazuyoshi Ukena, Hiroshima University, Japan
Copyright: © 2018 Ubuka and Parhar. This is an open-access article distributed under the terms of the Creative Commons Attribution License (CC BY). The use, distribution or reproduction in other forums is permitted, provided the original author(s) or licensor are credited and that the original publication in this journal is cited, in accordance with accepted academic practice. No use, distribution or reproduction is permitted which does not comply with these terms.
*Correspondence: Takayoshi Ubuka, dGFrYXlvc2hpLnVidWthQG1vbmFzaC5lZHU=