- Pediatric Clinic, Department of Surgical and Biomedical Sciences, Università degli Studi di Perugia, Perugia, Italy
Minipuberty consists of activation of the hypothalamic-pituitary-gonadal (HPG) axis during the neonatal period, resulting in high gonadotropin and sex steroid levels, and occurs mainly in the first 3–6 months of life in both sexes. The rise in the levels of these hormones allows for the maturation of the sexual organs. In boys, the peak testosterone level is associated with penile and testicular growth and the proliferation of gonadic cells. In girls, the oestradiol levels stimulate breast tissue, but exhibit considerable fluctuations that probably reflect the cycles of maturation and atrophy of the ovarian follicles. Minipuberty allows for the development of the genital organs and creates the basis for future fertility, but further studies are necessary to understand its exact role, especially in girls. Nevertheless, no scientific study has yet elucidated how the HPG axis turns itself off and remains dormant until puberty. Additional future studies may identify clinical implications of minipuberty in selected cohorts of patients, such as premature and small for gestational age infants. Finally, minipuberty provides a fundamental 6-month window of the possibility of making early diagnoses in patients with suspected sexual reproductive disorders to enable the prompt initiation of treatment rather than delaying treatment until pubertal failure.
Introduction
Puberty is the period of life in which a child develops secondary sexual characteristics and reproductive function. Puberty requires activation of the hypothalamic-pituitary-gonadal (HPG) axis, resulting in secretion of hypothalamic gonadotropin-releasing hormone (GnRH), which in turn stimulates secretion of luteinizing hormone (LH) and follicle stimulating hormone (FSH) by the pituitary gland and the consequent maturation of gametogenesis as well as secretion of gonadal hormones. Before the onset of puberty, the HPG axis is temporary activated in two other periods of life, i.e., in the midgestational fetus and in the newborn. In recent years, many studies in the literature have referred to this latter period as minipuberty.
Minipuberty was first described in the 1970s (1, 2), but its role is still not well understood. The aim of this review is to analyse the impact and the clinical role of minipuberty. PubMed was used to search for all relevant studies published over the last 25 years using the key words “minipuberty,” “mini-puberty,” “HPG axis,” “gonadotropins,” and “sexual hormones,” combined with “fetal life,” “newborn,” “preterm,” “small for gestational age,” “growth,” “congenital hypogonadotropic hypogonadism,” “Turner syndrome,” “Klinefelter syndrome” and “CAIS”. Additional sources were found from the references of the publications that were obtained from the search. The data obtained from studies published between 1973 and 2017 are included in this review, and the most recent research is dated March 2017.
Hypothalamic-Pituitary-Gonadal (HPG) Axis Activation in Fetal Life
During embryogenesis, neurons that produce GnRH develop from the epithelium of the medial olfactory pit and move to the fetal hypothalamus by migrating along nerve fibers (3). This process occurs at ~40 days of gestation (4). Simultaneously, the pituitary gland develops and begins synthesizing both LH and FSH at 9 weeks of gestation (WG) (5), although the hormones appear in the fetal blood by 12–14 WG (6). Kisspeptin and KISS1R are factors that are involved in the regulation of fetal GnRH neuron activity. However, serum LH and FSH levels are independent of GnRH and kisspeptin at midgestation, but they become GnRH-induced after 30–31 WG (7).
The gonadotropin levels peak at midgestation in both the pituitary gland and the serum and subsequently decrease toward birth and are suppressed at term (8, 9). This pattern is probably caused by the gradual increase in the production of placental estrogens toward the end of gestation (10) that suppresses the activity of the fetal HPG axis.
Additionally, female fetuses produce higher LH and FSH levels than male fetuses (6, 11). Indeed, Debieve et al. (12) measured LH and FSH at midpregnancy (the group had median ages of 23.8 WG for the females and 22.6 WG for the males) and at term (median ages: 39.2 WG for the females and 38.9 WG for the males). Both gonadotropins were present in the first group and exhibited a clear difference between the females and males; the girls exhibited much higher levels (33.0 ± 23.2 vs. 4.4 ± 3.3 mIU/mL for LH and 54.4 ± 27.7 vs. 0.77 ± 0.49 mIU/mL for FSH). In contrast, in the term female fetuses, both LH and FSH were undetectable, and only very low FSH levels were observed in the term male fetuses. The midpregnancy gonadotropin peak coincides with the first ovarian follicle or seminiferous tubule maturation. The difference between genders is probably caused by the negative feedback that results from the higher concentrations of fetal testicular hormones (6, 13, 14). Another marked difference between the sexes is that the LH levels overcome the FSH levels in male fetuses (15), whereas the opposite situation occurs in females.
During fetal life, the masculinization of genitalia depends on the production of testosterone (T) by the Leydig cells of the fetal testicles and on its action on target organs. During the first trimester of gestation, placental human chorio-gonadotropin (hCG) induces the differentiation of testicular mesenchymal cells into Leydig cells and stimulates T production through the activation of the LH/CG receptors expressed on their surfaces (16). Indeed, mutations of the LH/CG receptor can cause the absence of virilization and feminization of the external genitalia (17). Thus, the fetal testicular T is secreted first under the control of placental hCG, and only after the 9th WG, T secretion comes under the control of pituitary LH. A clear increase in T concentration occurs between 8 and 11 WG and reaches a maximum between 11 and 14 WG. The peak level (40–580 ng/dL) is similar to the adult value (14), whereas T levels in the fetal testes can reach ~1.9–2.1 ng/mg of tissue (16). After the 20th WG, T decreases toward term (8, 14). The fetal testicles also express FSH receptors that probably control Sertoli cell proliferation, although only few studies exist regarding the effects of FSH (18–20). Anti-mullerian hormone (AMH) that is produced by the Sertoli cells in the fetal testes causes the regression of the mullerian ducts, which prevents the formation of internal feminine genitalia. T favors the development of male urogenital structures from the wolffian duct, such as the vas deferens, epididymis, and seminal vesicles, while the formation of the prostate, penis and scrotum is due to the active metabolite of T (dihydrotestosterone).
In female fetuses, the lack of AMH allows the mullerian ducts to develop into the fallopian tubes, uterus and upper part of the vagina (21). The development of the primordial follicle in the fetal ovaries begins before 13 WG, but the follicles are more rapidly created after 14–15 WG. The pool of primordial follicles is ~100,000 at 15 WG and then rapidly increases to reach a higher number at 34 WG (680,000). Subsequently, the pool remains stable, at least until 8 months after birth (22). This pool, which represents the foundation of female fertility, is formed when estrogen levels are high in the fetal circulation. However, the majority of estrogen production during fetal life is due to the placenta, and ovarian production can be considered irrelevant. Additionally, the roles of FSH and LH in ovarian development during pregnancy are not completely understood. It seems that normal development occurs until the 34th WG even in anencephalic fetuses. In contrast, during the last part of gestation, a marked difference can be found; in anencephalic fetuses small and growing antral follicles cannot be observed as they can in normal fetuses (23), which suggests that hypothalamic stimulation is necessary to ensure physiological ovarian development after the 7th month of gestation.
Minipuberty and Its Implications in Healthy Infants
At delivery, in healthy infants the LH and FSH levels are low in the cord blood in both sexes (24) due to the inhibitory effect of the high levels of placental estrogens. In boys, the LH level increases by ~10-fold in the few minutes after delivery, and this increase is followed by a concomitant rise in the T levels that lasts for ~12 h (25, 26). In girls, this increase does not occur.
In the first few days after birth, the fall in circulating placentally produced steroids causes a progressive lack of negative feedback on the neonatal GnRH pulse generator. In this manner, the activity of the HPG axis is reinitiated, and gonadotropin levels begin rising between days 6 and 10 after birth (27, 28). In infant boys, the serum LH level reaches the pubertal range by 1 week of age, the peak is detected between the 2nd and 10th weeks of life, and the level then decreases to the prepubertal range by 4–6 months (27–30) (Figure 1). Female infants have lower peak LH values, but the pattern is similar (Figure 2) (27–29). In contrast, the FSH levels are higher in females than in males and peaking between 1 week and 3 months. Subsequently, in males, the FSH values gradually decrease to the prepubertal range within 4 months of age, whereas in females, these values remain elevated until to 3–4 years of age (27, 29, 31).
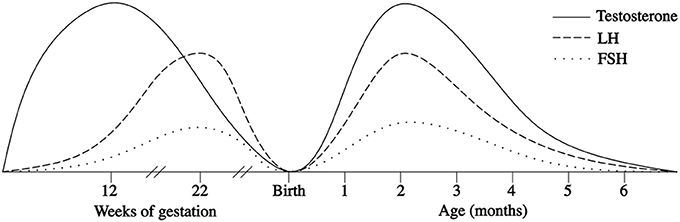
Figure 1. Patterns of fetal and postnatal luteinizing hormone (LH), follicle stimulating hormone (FSH) and testosterone (T) secretion in males.
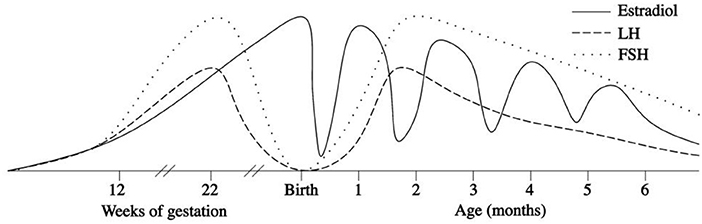
Figure 2. Patterns of fetal and postnatal luteinizing hormone (LH), follicle stimulating hormone (FSH) and oestradiol secretion in females.
Table 1 summarizes sex differences and the patterns of basal LH/FSH in the first month of life. In male neonates, the pattern of T secretion is similar to that of LH secretion: T is low in the cord blood, gradually increases to peak at 1–3 months and then declines to prepubertal values by 6–9 months of age (2, 27, 28, 30, 32). Similarly, the number of Leydig cells in the testicular tissue increases considerably until the third month and then gradually decreases due to apoptosis of the fetal Leydig cells (33–36). Sertoli cells also develop in the first months after birth under the stimulation of FSH (37), but they do not express androgen receptors during infancy, and therefore spermatogenesis does not occur (38). These cells secrete AMH in male neonates: the highest levels are observed at 3 months, and the levels subsequently decline and remain at relatively stable levels throughout childhood until puberty at which point AMH progressively decline to the adult level of 3–4% of the infant level (39). The absence of androgen receptors explains why AMH levels remain elevated in the presence of the high T levels during early infancy (38, 40). Additionally, in prepubertal boys, Sertoli cells secrete inhibin B, which represents a marker of Sertoli cell function. This hormone, which is already present in the cord blood, increases soon after birth, peaks at 3–6 months of age, reaches greater values then those observed in adults (mean +/– SD: 378 +/– 23 pg/mL), and remains elevated until at least the age of 15 months (28, 32, 41).
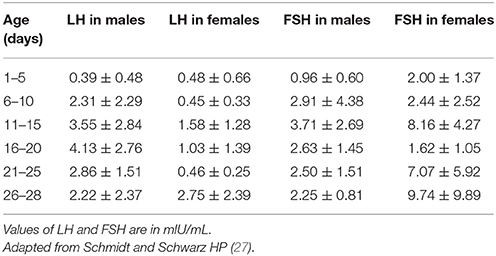
Table 1. Luteinizing hormone (LH) and follicle stimulating hormone (FSH) values (means ± SD) in neonates.
Minipuberty has been associated with physiological gonadal development processes, such as penile and testicular growth and the proliferation of gonadic cells. Cortes et al. (42) found that penile length is positively correlated with serum T and increases from birth (mean +/– SD, 3.49 +/– 0.4 cm) to 3 years of age with the highest growth velocity occurring from birth to 3 months (1 mm/month). In contrast, testicular volume increases significantly in the first 5–6 months of life, from 0.27 to 0.44 cm3, and the volume subsequently decreases to 0.31 cm3 at ~9 months (43). This pattern is positively correlated with FSH levels (30), which is probably due to the proliferation of Sertoli cells in the seminiferous tubules (37). Additionally, Hadziselimovic et al. proposed that germ cells can differentiate into adult (Ad) spermatogonia due to the transient activation of the HPG axis during the first months of life (44).
Thus, the first months of life are fundamental for the development of the male reproductive organs. In contrast, it is not completely clear whether these months are also important for the reproductive functions of girls. At birth, oestradiol levels are high in the cord blood of both sexes. Umbilical cord estrogen concentrations depend on gestational age, the mode of delivery, pregnancy complications, and twinning, but not on infant sex (45). In a study conducted by Troisi et al. (46), the mean oestradiol values measured in cord blood were found to be 11,941 pg/ml in females and 12,782 pg/ml in males, and the difference between gender was not significant. During the first postnatal days, oestradiol levels gradually decrease, but after 1 week of age, they increase in girls only and remain high in the subsequent period (47–49) until at least the 6th month of life. Similar observations were made by Bidlingmaier et al. (50), who found the higher oestradiol concentration in the ovaries of 1- to 6-month-old girls compared with those at the end of the first year in postmortem samples. At 3 months of age, the median serum oestradiol level in girls is 30.0 pmol/L (range < 18–100) (47). However, individual oestradiol levels in girls exhibit considerable fluctuation in the first months of life, which may reflect the cycles of maturation and atrophy of the ovarian follicles. Indeed Kuiri-Hanninen et al. (31) reported increased numbers of antral follicles on ovarian ultrasonography in infant girls, and this fact corresponds to parallel elevations of oestradiol (48, 49) and AMH levels (31, 51). Indeed, in infant girls, there is a marked rise of AMH levels at 3 months of age (15 pmol/L; 4.5–29.5 pmol/L) compared with the levels found in cord blood (2 pmol/L; 2–15.5 pmol/L) and at 1 year of age (51). This fact may demonstrate the postnatal proliferation of granulosa cells, which produce AMH, and the contemporary development of the ovarian follicles, which probably occurs in response to the parallel FSH surge (31, 51).
The mammary glands and the uterus are also certainly estrogen target tissues in the fetus and in newborn. At birth, most full term babies of both sexes have palpable breast tissue (52) that probably results from in-uterus stimulation from placental estrogens. However, in the following months, the breast tissue in females remains larger and persists longer (52). In boys, the mammary gland diameter gradually decreases until the 6th month, whereas in full-term girls, it remains large, reflecting the activity of endogenous estrogens (48). In contrast, the uterine length increases primarily during pregnancy due to the hormones that cross the placental barrier, and after birth, it is longest at day 7 in full-term babies and then steadily decreases toward the third month and remains fairly unchanged until the second year (48). Therefore, the role of minipuberty in girls is still controversial and partially unknown.
Minipuberty in Premature Infants and in Those Small for Gestational Age (SGA)
Postnatal HPG axis activation also occurs in premature infants and is even stronger and more prolonged in time than in full-term infants (53, 54). Kuiri-Hanninen et al. (30) recently compared full-term (FT) and preterm (PT) males by measuring urinary gonadotropins and T in serial urine samples and comparing the results with testicular and penile growth. The trends of LH and T secretion are similar among the groups, but the levels are significantly higher in PT than in FT males when measured at 7 and 30 days of age. These levels then decline in both groups, but a significant difference can still be observed at month 6. Additionally, a positive association between the level of HPG axis activation and the grade of prematurity has been found, and PT males have been associated with faster penile and testicular growth after birth, which suggests that HPG axis activation plays a role in completing genital development.
In PT females, the FSH and LH values are higher than those in FT girls (55) and exhibit a more elevated and more prolonged peak; these patterns might reflect the expression of the immaturity of a negative feedback system in the HPG axis of PT girls. The greater levels of FSH in PT females are probably due to a delay in ovarian folliculogenesis; the ovaries are still immature and do not seem to be able to produce sufficient estrogens that may inhibit gonadotropin secretion. Additionally, the FSH peak is followed by transient ovarian stimulation (which reaches a maximum at ~4 weeks of age) that results in the presence of antral follicles on ultrasonography and increases in the levels of granulosa cell–derived AMH and oestradiol (31), which are higher in the serum of PT than FT girls (49). Estrogen receptor alpha is expressed in the fetal mammary glands from ~30 weeks of gestation (56), which might explain the absence of breast development in PT infants. However, in these girls, there is a stronger association between the postnatal oestradiol surge and the growth of the mammary gland diameter and uterine length (48).
A possible clinical consequence of this intensive stimulation on the genital organs that occurs in premature infants is ovarian hyperstimulation syndrome. First described in 1985 by Sedin et al. (57) in 4 very preterm neonates, ovarian hyperstimulation syndrome is characterized by oedema of the vulva, solitary or multiple cysts in the ovaries on ultrasonography, breast growth, occasional vaginal bleeding and high serum gonadotropin and oestradiol levels. This syndrome is probably the extreme consequence of the immaturity of the negative feedback mechanisms that act on the HPG axis, and this absence of feedback results in hyperstimulation of the target organs. Several cases have been reported in the literature (58–62), and all cases indicate that it is a self-limiting disease that does not require treatment if there are no complications, but follow-up until clinical resolution is necessary.
Infants born small for gestational age (SGA) are at risk of developing metabolic and endocrinological disorders (63). It is well known that SGA children are at greater risk of type 2 diabetes and cardiovascular diseases, expecially those with high catch up growth. In fact, the fetus in nutritional deficiency constantly replans his metabolism to slow growth with relative resistance to insulin, IGF-1 and GH, that persists in childhood and adult life too (64). Besides, lower insulin sensitivity has been also associated to an increased incidence of adrenal and ovarian hyperandrogenism, clinically evident as precocious pubarche and reduced ovulation rate (65).
In females, being SGA has been associated with reduced uterine and ovary size (66), whereas, in males, SGA has been linked to infertility and reduced testicular volume and T concentrations in adult life (67). Minipuberty in SGA infants is still not well defined, and the data reported in the literature are controversial. Recently, Nagai et al. (68) conducted a longitudinal study and reported lower FSH and T, as well as higher LH concentrations, in SGA infants compared with appropriate for gestational age (AGA) infants. In contrast, in previous studies, elevated serum FSH concentrations have been detected in SGA infant girls and boys (69); similarly, higher T levels have been found in SGA than in AGA boys (70). Further studies are necessary to definitely clarify the patterns of minipuberty in SGA infants and their clinical implications.
Minipuberty and Growth
Growth is influenced by different hormones depending on the period of life. In fact, in the first years, thyroid hormones play the main role, together with insulin and glucocorticoids. By contrast, GH becomes predominant during infancy until the period of puberty, when the rise of levels of sexual hormones results in the growth spurt, which is essential for final growth and bone maturation.
Recently, new studies have described an association between minipuberty and growth, particularly in males. Indeed, sex steroids and gonadotropic hormones in the first 5 months of life seem to influence somatic development in boys during the following 6 years. Becker et al. (71) conducted a prospective study of 35 healthy infants (17 males) and reported that the surge in T during the first months of life has an influence on human somatic and adipose tissue development in childhood. Indeed, boys exhibit faster increases in weight and BMI at the age of 8 weeks than do girls. At this age, the median T level has been found to be 7.37 nmol/L, which corresponds to pubertal male values. Subsequently, other trials have been conducted with greater numbers of participants. Kiviranta et al. (72) studied 84 healthy neonates (45 of which were males). The linear growth velocity was significantly faster from birth to 6 months of age in boys than in girls, and the greatest growth velocity difference, i.e., 4.1 cm per year, was observed at 1 month of age, which is simultaneous with the peak of the postnatal gonadal activation, especially in terms of T level.
Minipuberty and Hypogonadism
In the last 20 years, studies have been conducted to establish an association between minipuberty and hypogonadotropic hypogonadism (HH), especially in males, and have found that the disease is characterized by the absence of the postnatal FSH, LH, and T surge. For this reason, minipuberty provides a short-time window of opportunity to make an early diagnosis (41). At birth, HH can be revealed by a micropenis with or without associated cryptorchidism, and male neonates exhibiting these “red flags” should undergo a single serum sample examination to identify congenital gonadotropin deficiency (73, 74). A novel study conduced in a large cohort of HH patients, in fact, confirmed the importance of identifying male genital tract anomalies in prepubertal age, in particular showing that micropenis or cryptorchidism are significantly more represented in HH resulting from Kallman syndrome (75). The best period for the measurement of the serum concentrations of reproductive hormone is between 4 and 8 weeks of life, but it can be practically performed until 6 months of age (73). Besides, a recent study suggested that testicular position increases from birth to 3 months of age and decreases thereafter, overlapping with the period of minipuberty. Therefore, testicular distance to pubic bone may be a useful biomarker of postnatal testicular function, both for Leyding and Sertoli cell activity (76).
Hadziselimovic et al. identified impaired minipuberty as the main reason for cryptorchidism-induced infertility (77). Indeed, the lack of secretion of LH and T in the perinatal period prevents the differentiation of germ cells, which results in infertility after puberty (78).
Furthermore, the importance of minipuberty has been highlighted by evidence that orchiopexy alone does not necessary improve fertility in cryptorchid males, whereas in 6-month-olds, long targeted therapy with LH-Rh analogs following successful surgery results in the normalization of the sperm parameters in adult life (79). Table 2 presents case reports and descriptions of small trials of patients with HH who were treated during the first year of life with recombinant human LH and FSH or with T in attempts to imitate physiological minipuberty. In all cases, the effects were beneficial. The substitutive therapy with T resulted in a marked increase in T level and penile length, whereas recombinant gonadotropin administration caused increase not only in T level, but also in LH, FSH, as well as inhibin B and AMH. This hormonal pattern results both in the increase of penile length and testicular volume and in fertility potential later in life. The administration of gonadotropins is safe, well tolerated and effective. Finally, new evidences suggest also the possibility of treatment with a gonadotropin-releasing hormone agonist, which induces gonocytes to differentiate into Ad spermatogonia and rescues fertility (84). As regards hypergonadotropic hypogonadism, one of the most frequent causes is Turner syndrome (TS). In these patients, perinatal FSH secretion is similar to that in healthy girls (85), however, during infancy, the pattern of FSH secretion is strictly related to karyotype. Young girls with monosomy TS exhibit a persistent elevation of FSH up to 6 years, whereas those with 45,X/46,XX mosaicism have only minimally elevated FSH values, which suggests the presence of feedback effects on the HPG axis due to retained ovarian function (86, 87).
Contrasting data can be found in the literature about males with Klinefelter syndrome (KS). Lahlou et al. (88) found that, in their cohort of KS patients 0–3 years old, the FSH, LH, and inhibin B levels were similar to those in healthy controls with the exception of T, which exhibited a physiological increase during the first trimester, but always remained at a lower level thereafter when compared with controls. Similarly, Ross et al. underlined that the neonatal surge in T is attenuated in the KS population (89). In contrast, Aksglaede et al. (90) found elevated LH levels and high-normal serum T levels in KS infants, as well as evidence of subtle Sertoli cell dysfunction with low-normal inhibin B levels. This situation may predict the postpubertal resistance of Sertoli cells to FSH action in subjects with KS in adult life (91). Table 3 summarizes studies on minipuberty in KS.
Finally, androgen receptors also play an important role in HPG axis activity. Indeed, infants with complete androgen insensitivity who present with a mutation in the androgen receptor do not exhibit the physiological peaks in LH and consequently T during minipuberty, whereas neonates with partial androgen insensitivity exhibit high-normal levels of postnatal T and LH (92).
Conclusions
The HPG axis is physiologically activated in the fetus during midgestation and gradually turns off toward term due to the negative feedback of placental hormones on the fetal hypothalamus. At birth, when the restriction is removed, the HPG axis reactivates, which results in a T peak in males between months 1 and 3; by contrast, the oestradiol levels in females fluctuate until 6 months of age. Minipuberty allows for the development of the genital organs and creates the basis for future fertility, but further studies are necessary to understand its exact role, especially in girls. Nevertheless, no scientific study has yet elucidated how the HPG axis turns itself off and remains dormant until puberty. Additional future studies may identify clinical implications of minipuberty in selected cohorts of patients, such as premature and small for gestational age infants. Finally, minipuberty provides a fundamental 6-month window of the possibility of making early diagnoses in patients with suspected sexual reproductive disorders to enable the prompt initiation of treatment rather than delaying treatment until pubertal failure.
Author Contributions
LL drafted the manuscript. MC and AL performed the literature review. LP supervised the project. SE revised the manuscript and made substantial scientific contributions.
Funding
This review was partially supported by the World Association for Infectious Diseases and Immunological Disorders (grant n. WAidid2017_06). WAidid has no role in literature analysis and manuscript preparation, but only gave an unrestricted grant for covering expenses.
Conflict of Interest Statement
The authors declare that the research was conducted in the absence of any commercial or financial relationships that could be construed as a potential conflict of interest.
References
1. Forest MG, Cathiard AM. Pattern of plasma testosterone and A4 androstenedione in normal newborns: evidence for testicular activity at birth. J Clin Endocrinol Metab. (1975) 1:977–80. doi: 10.1210/jcem-41-5-977
2. Forest MG, Cathiard AM, Bertrand JA. Evidence of testicular activity in early infncy. J Clin Endocrinol Metab. (1973) 1:148–51. doi: 10.1210/jcem-37-1-148
3. Schwanzel-Fukada M, Pfaff D. Origin of luteinizing hormone-releasing hormone neurons. Nature (1989) 338:161–4. doi: 10.1038/338161a0
4. Crossin KL, Pfaff DW, Bouloux PMG, Pasteur I, France JH. Migration of luteinizing hormone-releasing hormone (LHRH) neurons in early human embryos. J Clin Invest. (1996) 1996:547657.
5. Hagen C, McNeilly AS. The gonadotrophins and their subunits in foetal pituitary glands and circulation. J Steroid Biochem. (1977) 8:537–44. doi: 10.1016/0022-4731(77)90259-X
6. Clements JA, Reyes FI, Winter JSD, Faiman C. Studies on human sexual development. III. Fetal pituitary and serum, and amniotic fluid concentrations of LH, CG, and FSH. J Clin Endocrinol Metab. (1976) 42:9. doi: 10.1210/jcem-42-1-9
7. Guimiot F, Chevrier L, Dreux S, Chevenne D, Caraty A, Delezoide AL, et al. Negative fetal FSH/LH regulation in late pregnancy is associated with declined Kisspeptin/KISS1R expression in the tuberal hypothalamus. J Clin Endocrinol Metab. (2012) 97:2221–9. doi: 10.1210/jc.2012-2078
8. Takagi S, Yoshida T, Tsubata K, Ozaki H, Fujii TK, Nomura Y, et al. Sex differences in fetal gonadotropins and androgens. J Steroid Biochem. (1977) 8:609–20. doi: 10.1016/0022-4731(77)90270-9
9. Massa G, de Zegher F, Vanderschueren-Lodeweyckx M. Serum levels of immunoreactive inhibin, FSH, and LH in human infants at preterm and term birth. Biol Neonate. (1992) 61:150–5. doi: 10.1159/000243737
10. Troisi R, Potischman N, Roberts JM, Harger G, Markovic N, Cole B, et al. Hoover RN. Correlation of serum hormone concentrations in maternal and umbilical cord samples. Cancer Epidemiol Biomarkers Prev. (2003) 12:452–6.
11. Kaplan SL, Grumbach MM. The ontogenesis of human foetal hormones. II. Luteinizing hormone (LH) and follicle stimulating hormone (FSH). Acta Endocrinol. (1976) 81:808–29. doi: 10.1530/acta.0.0810808
12. Debieve F, Beerlandt S, Hubinont C, Thomas K. Gonadotropins, prolactin, inhibin A, inhibin B, and activin A in human fetal serum from midpregnancy and term pregnancy. J Clin Endocrinol Metab. (2000) 85:270–4. doi: 10.1210/jcem.85.1.6249
13. Winter JS. Hypothalamic-pituitary function in the fetus and infant. Clin Endocrinol Metab. (1982) 11:41–55. doi: 10.1016/S0300-595X(82)80037-6
14. Reyes FI, Borodits RS, Winter JSD, Faiman C. Studies on human sexual development .2. Fetal and maternal serum gonadotropin and sex steroid concentrations. J Clin Endocrinol Metab. (1974) 38:612–7. doi: 10.1210/jcem-38-4-612
15. Beck-Peccoz P, Padmanabhan V, Baggiani AM, Cortelazzi D, Buscaglia M, Medri G, et a. Maturation of hypothalamic-pituitary-gonadal function in normal human fetuses: circulating levels of gonadotropins, their common a-subunit and free testosterone, and discrepancy between immunological and biological activities of circulating follicle-stimu. J Clin Endocrinol Metab. (1991) 73:525–32. doi: 10.1210/jcem-73-3-525
16. Tapanainen J, Kellokumpu-lehtinen P, Pelliniemi L. Age-related changes in endogenous steroids of human. J Clin Endocrinol Metab. (1981) 52:98–102. doi: 10.1210/jcem-52-1-98
17. Latronico AC, Anasti J, Arnhold IJ, Rapaport R, Mendonca BB, Bloise W, et al. Testicular and ovarian resistance to luteinizing hormone caused by inactivating mutations of the luteinizing hormone. N Engl J Med. (1996) 334:507–12. doi: 10.1056/NEJM199602223340805
18. Zhang S, Li W, Zhu C, Wang X, Li Z, Zhang J, et al. Sertoli cell-specific expression of metastasis-associated protein 2 (MTA2) is required for transcriptional regulation of the follicle-stimulating hormone receptor (FSHR) gene during spermatogenesis. J Biol Chem. (2012) 287:40471–83. doi: 10.1074/jbc.M112.383802
19. Plant TM, Marshall GR. The functional significance of FSH in spermatogenesis and the control of its secretion in male primates. Endocr Rev. (2001) 22:764–86. doi: 10.1210/edrv.22.6.0446
20. O'Shaughnessy PJ, Baker PJ, Monteiro A, Cassie S, Bhattacharya S, Fowler PA. Developmental changes in human fetal testicular cell numbers and messenger ribonucleic acid levels during the second trimester. J Clin Endocrinol Metab. (2007) 92:4792–801. doi: 10.1210/jc.2007-1690
21. Bowles J, Koopman P. Sex determination in mammalian germ cells: extrinsic versus intrinsic factors. Reproduction (2010) 139:943–58. doi: 10.1530/REP-10-0075
22. Forabosco A, Sforza C. Establishment of ovarian reserve: a quantitative morphometric study of the developing human ovary. Fertil Steril. (2007) 88:675–83. doi: 10.1016/j.fertnstert.2006.11.191
23. Baker TG, Scrimgeour JB. Development of the gonad in normal and anencephalic human fetuses. J Reprod Fertil. (1980) 60:193–9. doi: 10.1530/jrf.0.0600193
24. Varvarigou AA, Liatsis SG, Vassilakos P, Decavalas G, Beratis NG. Effect of maternal smoking on cord blood estriol, placental lactogen, chorionic gonadotropin, FSH, LH, and cortisol. J Perinat Med. (2009) 37:364–9. doi: 10.1515/JPM.2009.028
25. Corbier P, Dehennin L, Castanier M, Mebazaa A, Edwards DA, Roffi J. Sex differences in serum luteinizing hormone and testosterone in the human neonate during the first few hours after birth. J Clin Endocrinol Metab. (1990) 71:1344–8. doi: 10.1210/jcem-71-5-1344
26. de Zegher F, Devlieger H, Veldhuis JD. Pulsatile and sexually dimorphic secretion of luteinizing hormone in the human infant on the day of birth. Pediatr Res. (1992) 32:605–7. doi: 10.1203/00006450-199211000-00025
27. Schmidt H, Schwarz HP. Serum concentrations of LH and FSH in the healthy newborn. Eur J Endocrinol. (2000) 143:213–5. doi: 10.1530/eje.0.1430213
28. Bergadá I, Milani C, Bedecarrás P, Andreone L, Ropelato MG, Gottlieb S, et al. Time course of the serum gonadotropin surge, inhibins, and anti-Müllerian hormone in normal newborn males during the first month of life. J Clin Endocrinol Metab. (2006) 91:4092–8. doi: 10.1210/jc.2006-1079
29. Winter JSD, Faiman C, Hobson WC, Prasad AV, Reyes FI. Pituitary-gonadal relations in infancy. I. patterns of serum gonadotropin concentrations from birth to four years of age in man and chimpanzee. J Clin Endocrinol Metab. (1975) 40:545–51. doi: 10.1210/jcem-40-4-545
30. Kuiri-Hänninen T, Seuri R, Tyrväinen E, Turpeinen U, Hämäläinen E, Stenman UH, et al. Increased activity of the hypothalamic-pituitary-testicular axis in infancy results in increased androgen action in premature boys. J Clin Endocrinol Metab. (2011) 96:98–105. doi: 10.1210/jc.2010-1359
31. Kuiri-Hänninen T, Kallio S, Seuri R, Tyrväinen E, Liakka A, Tapanainen J, Sankilampi U, Dunkel L. Postnatal developmental changes in the pituitary-ovarian axis in preterm and term infant girls. J Clin Endocrinol Metab. (2011) 96:3432–9. doi: 10.1210/jc.2011-1502
32. Andersson AM, Toppari J, Haavisto AM, Petersen JH, Simell T, Simell O, et al. Longitudinal reproductive hormone profiles in infants: Peak of inhibin B levels in infant boys exceeds levels in adult men. J Clin Endocrinol Metab. (1998) 83:675–81. doi: 10.1210/jc.83.2.675
33. Chemes HE. Infancy is not a quiescent period of testicular development. Int J Androl. (2001) 24:2–7. doi: 10.1046/j.1365-2605.2001.00260.x
34. Codesal J, Regadera J, Nistal M, Regadera-Sejas J, Paniagua R. Involution of human fetal Leydig cells. An immunohistochemical, ultrastructural and quantitative study. J Anat. (1990) 172:103–14.
35. Lejeune H, Habert R, Saez JM. Origin, proliferation and differentiation of Leydig cells. J Mol Endocrinol. (1998) 20:1–25. doi: 10.1677/jme.0.0200001
36. Nistal M, Paniagua R, Regadera J, Santamaria L, Amat P. A quantitative morphological study of human Leydig cells from birth to adulthood. Cell Tissue Res. (1986) 246:229–36. doi: 10.1007/BF00215884
37. Cortes D, Muller J, Skakkebaek NE. Proliferation of Sertoli cells during development of the human testis assessed by stereological methods. Int J Androl. (1987) 10:589–96. doi: 10.1111/j.1365-2605.1987.tb00358.x
38. Chemes HE, Rey RA, Nistal M, Regadera J, Musse M, González-Peramato P, et al. Physiological androgen insensitivity of the fetal, neonatal, and early infantile testis is explained by the ontogeny of the androgen receptor expression in sertoli cells. J Clin Endocrinol Metab. (2008) 93:4408–12. doi: 10.1210/jc.2008-0915
39. Aksglaede L, Sørensen K, Boas M, Mouritsen A, Hagen CP, Jensen RB, et al. Changes in anti-mullerian hormone (AMH) throughout the life span: a population-based study of 1027 healthy males from birth (cord blood) to the age of 69 years. J Clin Endocrinol Metab. (2010) 95:5357–64. doi: 10.1210/jc.2010-1207
40. Boukari K, Meduri G, Brailly-Tabard S, Guibourdenche J, Ciampi ML, Massin N, et al. Lack of androgen receptor expression in sertoli cells accounts for the absence of anti-Mullerian hormone repression during early human testis development. J Clin Endocrinol Metab. (2009) 94:1818–25. doi: 10.1210/jc.2008-1909
41. Grumbach MM. A window of opportunity: the diagnosis of gonadotropin deficiency in the male infant. J Clin Endocrinol Metab. (2005) 90:3122–7. doi: 10.1210/jc.2004-2465
42. Boas M, Boisen KA, Virtanen HE, Kaleva M, Suomi AM, Schmidt IM, et al. Postnatal penile length and growth rate correlate to serum testosterone levels: a longitudinal study of 1962 normal boys. Eur J Endocrinol. (2006) 154:125–9. doi: 10.1530/eje.1.02066
43. Kuijper EA, van Kooten J, Verbeke JI, van Rooijen M, Lambalk CB. Ultrasonographically measured testicular volumes in 0- to 6-year-old boys. Hum Reprod. (2008) 23:792–6. doi: 10.1093/humrep/den021
44. Hadziselimovic F, Hadziselimovic NO, Demougin P, Krey G, Oakeley E. Piwi-pathway alteration induces LINE-1 transposon derepression and infertility development in cryptorchidism. Sex Dev. (2015) 9:98–104. doi: 10.1159/000375351
45. Hickey M, Hart R, Keelan JA. The relationship between umbilical cord estrogens and perinatal characteristics. Cancer Epidemiol Biomarkers Prev. (2014) 23:946–52. doi: 10.1158/1055-9965.EPI-13-1321
46. Troisi R, Potischman N, Roberts J, Siiteri P, Daftary A, Sims C, et al. Associations of maternal and umbilical cord hormone concentrations with maternal, gestational and neonatal factors (United States). Cancer Causes Control (2003) 14:347–55.
47. Schmidt IM, Chellakooty M, Haavisto AM, Boisen KA, Damgaard IN, Steendahl U, et al. Gender difference in breast tissue size in infancy: correlation with serum estradiol. Pediatr Res. (2002) 52:682–6. doi: 10.1203/00006450-200211000-00012
48. Kuiri-Hänninen T, Haanpää M, Turpeinen U. Postnatal ovarian activation has effects in estrogen target tissues in infant girls. J Clin Endocrinol Metab. (2013) 98:4709–16. doi: 10.1210/jc.2013-1677
49. Chellakooty M, Schmidt IM, Haavisto AM, Boisen KA, Damgaard IN, Mau C, et al. Inhibin A, inhibin B, follicle-stimulating hormone, luteinizing hormone, estradiol, and sex hormone-binding globulin levels in 473 healthy infant girls. J Clin Endocrinol Metab. (2003) 88:3515–20. doi: 10.1210/jc.2002-021468
50. Bidlingmaier F, Strom TM, Dorr HG, Eisenmenger W, Knorr D. Estrone and estradiol concentrations in human ovaries, testes, and adrenals during the first two years of life. J Clin Endocrinol Metab. (1987) 65:862–7. doi: 10.1210/jcem-65-5-862
51. Hagen CP, Aksglaede L, Sørensen K, Main KM, Boas M, Cleemann L, et al., Juul A. Serum levels of anti-müllerian hormone as a marker of ovarian function in 926 healthy females from birth to adulthood and in 172 turner syndrome patients. J Clin Endocrinol Metab. (2010) 95:5003–10. doi: 10.1210/jc.2010-0930
52. Jayasinghe Y, Cha R, Horn-Ommen J, O'Brien P, Simmons PS. Establishment of normative data for the amount of breast tissue present in healthy children up to two years of age. J Pediatr Adolesc Gynecol. (2010) 23:305–11. doi: 10.1016/j.jpag.2010.03.002
53. Tapanainen J, Koivisto M, Vihko R, Huhtaniemi I. Enhanced activity of the pituitary-gonadal axis in premature human infants. J Clin Endocrinol Metab. (1981) 52:235–8. doi: 10.1210/jcem-52-2-235
54. Shinkawa O, Furuhashi N, Fukaya T, Suzuki M, Kono H, Tachibana Y. Changes of Serum Gonadotropin Levels and Sex Differences in Premature and Mature Infant during Neonatal Life. J Clin Endocrinol Metab. (1983) 56:1327–31. doi: 10.1210/jcem-56-6-1327
55. De Jong M, Rotteveel J, Heijboer AC, Cranendonk A, Twisk JWR, Van Weissenbruch MM. Urine gonadotropin and estradiol levels in female very-low-birth-weight infants. Early Hum Dev. (2013) 89:131–5. doi: 10.1016/j.earlhumdev.2012.09.007
56. Keeling JW, Ozer E, King G, Walker F. Oestrogen receptor alpha in female fetal, infant, and child mammary tissue. J Pathol. (2000) 191:449–51. doi: 10.1002/1096-9896(2000)9999:9999<::AID-PATH661>3.0.CO;2-#
57. Sedin G, Bergquist C, Lindgren PG. Ovarian hyperstimulation syndrome in preterm infants. Pediatr Res. (1985) 19:548–52. doi: 10.1203/00006450-198506000-00009
58. Marinkovic M, Rasmussen M, Jones K. Feminizing changes in a prematurely born infant. Clin Pediatr (Phila). (2010) 49:188–9. doi: 10.1177/0009922809337624
59. Starzyk J, Wojcik M, Wojtys J, Tomasik P, Mitkowska Z, Pietrzyk JJ. Ovarian hyperstimulation syndrome in newborns–a case presentation and literature review. Horm Res. (2009) 71:60–4. doi: 10.1159/000173743
60. Altuntas N, Turkyilmaz C, Yuce O, Kulali F, Hirfanoglu IM, Onal E, et al. Preterm ovarian hyperstimulation syndrome presented with vaginal bleeding: a case report. J Pediatr Endocrinol Metab. (2014) 27:355–8. doi: 10.1515/jpem-2013-0166
61. Durst MA, Wicklow B, Narvey M. Atypical case of preterm ovarian hyperstimulation syndrome. BMJ Case Rep. (2017) 1:2017. doi: 10.1136/bcr-2016-217517
62. Vochem M. [Ovarian hyperstimulation syndrome in preterm infants]. Z Geburtshilfe Neonatol. (2002) 206:156–60. doi: 10.1055/s-2002-33669
63. Gluckman PD, Hanson MA. Living with the past: evolution, development, and patterns of disease. Science. (2004) 305:1733–6. doi: 10.1126/science.1095292
64. Mericq V, Ong KK, Bazaes R, Peña V, Avila A, Salazar T, et al. Longitudinal changes in insulin sensitivity and secretion from birth to age three years in small- and appropriate-for-gestational-age children. Diabetologia (2005) 48:2609–14. doi: 10.1007/s00125-005-0036-z
65. Ibáñez L, de Zegher F. Puberty after prenatal growth restraint. Horm Res. (2006) 65(Suppl. 3):112–115.
66. Ibanez L, Potau N, Enriquez G, Marcos MV, de Zegher F. Hypergonadotrophinaemia with reduced uterine and ovarian size in women born small-for-gestational-age. Hum Reprod. (2003) 18:1565–9. doi: 10.1093/humrep/deg351
67. Cicognani A, Alessandroni R, Pasini A, Pirazzoli P, Cassio A, Barbieri E, Cacciari E. Low birth weight for gestational age and subsequent male gonadal function. J Pediatr. (2002) 141:376. doi: 10.1067/mpd.2002.126300
68. Nagai S, Kawai M, Myowa-Yamakoshi M, Morimoto T, Matsukura T, Heike T. Gonadotropin levels in urine during early postnatal period in small for gestational age preterm male infants with fetal growth restriction. J Perinatol. (2017) 37:843–7. doi: 10.1038/jp.2017.55
69. Ibáñez L, Valls C, Cols M, Ferrer A, Marcos MV, De Zegher F. Hypersecretion of FSH in infant boys and girls born small for gestational age. J Clin Endocrinol Metab. (2002) 87:1986–8. doi: 10.1210/jcem.87.5.8459
70. Forest MG, de Peretti E, Bertrand J. Testicular and adrenal androgens and their binding to plasma proteins in the perinatal period: developmental patterns of plasma testosterone, 4-androstenedione, dehydroepiandrosterone and its sulfate in premature and small for date infants as compared wit. J Steroid Biochem. (1980) 12:25–36. doi: 10.1016/0022-4731(80)90247-2
71. Becker M, Oehler K, Partsch CJ, Ulmen U, Schmutzler R, Cammann H, et al. Hormonal “minipuberty” influences the somatic development of boys but not of girls up to the age of 6 years. Clin Endocrinol. (2015) 83:694–701. doi: 10.1111/cen.12827
72. Kiviranta P, Kuiri-Hänninen T, Saari A, Lamidi M-L, Dunkel L, Sankilampi U. Transient postnatal gonadal activation and growth velocity in infancy. Pediatrics (2016) 138:e20153561. doi: 10.1542/peds.2015-3561
73. Quinton R, Mamoojee Y, Jayasena CN, Young J, Howard S, Dunkel L, et al. Society for Endocrinology UK guidance on the evaluation of suspected disorders of sexual development: emphasizing the opportunity to predict adolescent pubertal failure through a neonatal diagnosis of absent minipuberty. Clin Endocrinol. (2017) 86:305–6. doi: 10.1111/cen.13257
74. Dwyer AA, Jayasena CN, Quinton R. Congenital hypogonadotropic hypogonadism: implications of absent mini-puberty. Minerva Endocrinol. (2016) 41:188–95.
75. Bonomi M, Vezzoli V, Krausz C, Guizzardi F, Vezzani S, Simoni M, et al. Italian Network on Central Hypogonadism. Characteristics of a nationwide cohort of patients presenting with isolated hypogonadotropic hypogonadism (IHH). Eur J Endocrinol. (2018) 178:23–32. doi: 10.1530/EJE-17-0065
76. Koskenniemi JJ, Virtanen HE, Wohlfahrt-Veje C, Löyttyniemi E, Skakkebaek NE, Juul A, et al. Postnatal changes in testicular position are associated with IGF-I and function of Sertoli and Leydig cells. J Clin Endocrinol Metab. (2018) 103:1429–37. doi: 10.1210/jc.2017-01889
77. Hadziselimovic F, Zitvkovic D, Bica DTG, Emmons LR. The importance of mini-puberty for fertility in Cryptorchidism. J Urol. (2005) 174:1536–9. doi: 10.1097/01.ju.0000181506.97839.b0
78. Hadziselimovic F, Emmons LR, Buser MW. A diminished postnatal surge of Ad spermatogonia in cryptorchid infants is additional evidence for hypogonadotropic hypogonadism. Swiss Med Wkly. (2004) 134:381–4.
79. Hadziselimovic F, Gegenschatz-Schmid K, Verkauskas G, Demougin P, Bilius V, Dasevicius D, et al. Gene expression changes underlying idiopathic central hypogonadism in cryptorchidism with defective mini-puberty. Sex Dev. (2016) 10:136–46. doi: 10.1159/000447762
80. Main KM, Schmidt IM, Skakkebæk NE. A possible role for reproductive hormones in newborn boys: Progressive hypogonadism without the postnatal testosterone peak. J Clin Endocrinol Metab. (2000) 85:4905–7. doi: 10.1210/jcem.85.12.7058
81. Main KM, Schmidt IM, Toppari J, Skakkebæk NE. Early postnatal treatment of hypogonadotropic hypogonadism with recombinant human FSH and LH. Eur J Endocrinol. (2002) 146:75–9. doi: 10.1530/eje.0.1460075
82. Bougnères P, François M, Pantalone L, Rodrigue D, Bouvattier C, Demesteere E, et al. Effects of an Early Postnatal Treatment of Hypogonadotropic Hypogonadism with a Continuous Subcutaneous Infusion of Recombinant Follicle-Stimulating Hormone and Luteinizing Hormone. J Clin Endocrinol Metab. (2008) 93:2202–5. doi: 10.1210/jc.2008-0121
83. Stoupa A, Samara-Boustani D, Flechtner I, Pinto G, Jourdon I, González-Briceño L, et al. Efficacy and safety of continuous subcutaneous infusion of recombinant human gonadotropins for congenital micropenis during early infancy. Horm Res Paediatr. (2017) 87:103–10. doi: 10.1159/000454861
84. Gegenschatz-Schmid K, Verkauskas G, Demougin P, Bilius V, Dasevicius D, Stadler MB, et al. Curative GnRHa treatment has an unexpected repressive effect on Sertoli cell specific genes. Basic Clin Androl. (2018) 28:2. doi: 10.1186/s12610-018-0067-1
85. Heinrichs C, Bourdoux P, Saussez C, Vis HL, Bourguignon JP. Blood spot follicle-stimulating hormone during early postnatal life in normal girls and Turner's syndrome. J Clin Endocrinol Metab. (1994) 78:978–81.
86. Fechner PY, Davenport ML, Qualy RL, Ross JL, Gunther DF, et al. Toddler Turner Study Group. Differences in follicle-stimulating hormone secretion between 45,X monosomy Turner syndrome and 45,X/46,XX mosaicism are evident at an early age. J Clin Endocrinol Metab. (2006) 91:4896–902. doi: 10.1210/jc.2006-1157
87. Chrysis D, Spiliotis BE, Stene M, Cacciari E, Davenport ML. Gonadotropin secretion in girls with Turner syndrome measured by an ultrasensitive immunochemiluminometric assay. Horm Res. (2006) 65:261–6. doi: 10.1159/000092516
88. Lahlou N, Fennoy I, Carel J-C, Roger M. Inhibin B and anti-müllerian hormone, but not testosterone levels, are normal in infants with nonmosaic Klinefelter syndrome. J Clin Endocrinol Metab. (2004) 89:1864–8. doi: 10.1210/jc.2003-031624
89. Ross JL, Samango-Sprouse C, Lahlou N, Kowal K, Elder FF, Zinn A. Early androgen deficiency in infants and young boys with 47,XXY Klinefelter syndrome. Horm Res. (2005) 64:39–45. doi: 10.1159/000087313
90. Aksglaede L, Petersen JH, Main KM, Skakkebæk NE, Juul A. High normal testosterone levels in infants with non-mosaic Klinefelter's syndrome. Eur J Endocrinol. (2007) 157:345–50. doi: 10.1530/EJE-07-0310
91. Cabrol S, Ross JL, Fennoy I, Bouvattier C, Roger M, Lahlou N. Assessment of leydig and sertoli cell functions in infants with nonmosaic klinefelter syndrome: Insulin-like peptide 3 levels are normal and positively correlated with lh levels. J Clin Endocrinol Metab. (2011) 96:746–53. doi: 10.1210/jc.2010-2103
Keywords: gonadotropin, hypothalamic-pituitary-gonadal, minipuberty, oestradiol, testosterone
Citation: Lanciotti L, Cofini M, Leonardi A, Penta L and Esposito S (2018) Up-To-Date Review About Minipuberty and Overview on Hypothalamic-Pituitary-Gonadal Axis Activation in Fetal and Neonatal Life. Front. Endocrinol. 9:410. doi: 10.3389/fendo.2018.00410
Received: 22 April 2018; Accepted: 02 July 2018;
Published: 23 July 2018.
Edited by:
Valentino Cherubini, Azienda Ospedaliero Universitaria Ospedali Riuniti, ItalyReviewed by:
Stefano Zucchini, Policlinico S. Orsola Malpighi, ItalyJarmo Jääskeläinen, Kuopio University Hospital, Finland
Marco Bonomi, Istituto Auxologico Italiano (IRCCS), Italy
Copyright © 2018 Lanciotti, Cofini, Leonardi, Penta and Esposito. This is an open-access article distributed under the terms of the Creative Commons Attribution License (CC BY). The use, distribution or reproduction in other forums is permitted, provided the original author(s) and the copyright owner(s) are credited and that the original publication in this journal is cited, in accordance with accepted academic practice. No use, distribution or reproduction is permitted which does not comply with these terms.
*Correspondence: Susanna Esposito, susanna.esposito@unimi.it