- 1Paediatric Surgery, Royal Manchester Children's Hospital, University Manchester NHS Foundation Trust (MFT), Manchester, United Kingdom
- 2Faculty of Biology, Medicine & Health, University of Manchester, Manchester, United Kingdom
- 3Paediatric Endocrinology, Royal Manchester Children's Hospital, University Manchester NHS Foundation Trust (MFT), Manchester, United Kingdom
- 4Paediatric Histopathology, Royal Manchester Children's Hospital, University Manchester NHS Foundation Trust (MFT), Manchester, United Kingdom
- 5Nuclear Medicine, Royal Manchester Children's Hospital, University Manchester NHS Foundation Trust (MFT), Manchester, United Kingdom
- 6Molecular Genetics, Royal Devon & Exeter NHS Foundation Trust, University of Exeter Medical School, Royal Devon & Exeter Hospital, Exeter, United Kingdom
Background: Congenital Hyperinsulinism (CHI) is an important cause of severe and persistent hypoglycaemia in infancy and childhood. The focal form (CHI-F) of CHI can be potentially cured by pancreatic lesionectomy. While diagnostic characteristics of CHI-F pancreatic histopathology are well-recognized, correlation with clinical phenotype has not been established.
Aims: We aimed to correlate the diversity in clinical profiles of patients with islet cell organization in CHI-F pancreatic tissue.
Methods: Clinical datasets were obtained from 25 patients with CHI-F due to ABCC8/KCNJ11 mutations. 18F-DOPA PET-CT was used to localize focal lesions prior to surgery. Immunohistochemistry was used to support protein expression studies.
Results: In 28% (n = 7) of patient tissues focal lesions were amorphous and projected into adjoining normal pancreatic tissue without clear delineation from normal tissue. In these cases, severe hypoglycaemia was detected within, on average, 2.8 ± 0.8 (range 1–7) days following birth. By contrast, in 72% (n = 18) of tissues focal lesions were encapsulated within a defined matrix capsule. In this group, the onset of severe hypoglycaemia was generally delayed; on average 46.6 ± 14.3 (range 1–180) days following birth. For patients with encapsulated lesions and later-onset hypoglycaemia, we found that surgical procedures were curative and less complex.
Conclusion: CHI-F is associated with heterogeneity in the organization of focal lesions, which correlates well with clinical presentation and surgical outcomes.
Introduction
The most common cause of persistent or recurrent hypoglycaemia in early childhood and infancy is hyperinsulinism. Congenital Hyperinsulinism in Infancy (CHI), a rare disorder, arises from inappropriate insulin release for the level of glycaemia. Despite advances in genetic testing and management strategies, CHI carries significant morbidity and mortality associated with hypoglycaemia-induced brain injury and adverse long-term neurological outcomes (1–6). The hypoglycaemia can be unresponsive to dietary and medical interventions with diazoxide, somatostatin analogs and other medications necessitating focal lesionectomy, partial or near-total pancreatectomy (7–10). The extent of surgery performed depends upon the developmental nature of the underlying genetic cause of disease, which is broadly classified as either focal or diffuse (11). Diffuse β-cell hypersecretion requires near total pancreatectomy, and invariably results in additional complications such as diabetes mellitus and/or exocrine pancreas insufficiency (12–14). Focal disease (CHI-F) on the other hand is caused by β-cell hyperfunction and hyperplasia, which is localized to a solitary functional lesion (15). As CHI-F can be surgically managed in many cases by excision of the lesion, pre-operative localization techniques have been used to guide surgical intervention toward the removal of disease tissue from non-affected parts of the pancreas. Localization techniques range from interventional investigations, such as pancreatic venous sampling (PVS) and combined selective pancreatic arterial calcium stimulation and hepatic venous sampling to fluorine-18 labeled fluoro-L-DOPA (18F-DOPA) positron emission tomography (PET) imaging. 18F-DOPA PET currently has the highest degree of specificity in diagnosing CHI-F and has largely replaced other investigations in the localization of focal domains (16–18).
Mutations in 11 genes (ABCC8, KCNJ11, GCK, GLUD1, HADH, PGM1, HNF1a, HNF4a, INSR, FOXA2, and CACNA1D) and the inappropriate expression of SLC16A1 and HK1 have been associated with CHI (19). Loss-of-function mutations in subunits of the pancreatic ATP-sensitive potassium (KATP) channel, ABCC8 and KCNJ11, account for more than 50% of CHI cases (19–21) and for all reported CHI-F cases. CHI-F is highly unusual in its pathogenesis as it involves the inheritance of a paternal germinal mutation (ABCC8 or KCNJ11) and loss of the maternal imprinting of chromosome 11p15 allele—loss of heterozygosity (LOH). LOH is a somatic event restricted to a group of β-cells (15, 22). Amongst the genes lost from the maternal allele are ABCC8/KCNJ11, and several genes that normally result in balanced cell growth including H19, IGF-II, and CDKN1C. For CHI-F this combination of events leads to the expansion of a β-cell mass harboring defects in KATP channels within a localized domain (22). We know little about the triggers for maternal LOH during embryogenesis, and since the timing of this event is likely to be variable, it is reasonable to assume that this will lead to heterogeneity in the formation and organization of CHI-F lesions with variable clinical impact. Indeed, whilst there are several reports of the occurrence of unusual focal lesions in CHI patients, there has been no data to correlate structural aspects of lesions to the clinical profiles. Here, we report a series of 25 cases of CHI-F in which we were able to separate the organizational aspect of focal lesions into two categories that have a direct correlation to clinical datasets, surgical management, and outcomes.
Subjects and Methods
Cohort
Our study is based upon 25 subjects with CHI-F originating from a single specialized treatment center for CHI using established diagnostic criteria (20). Twenty four patients were aged between 1 and 19 months at the time of surgery, Table 1. One subject was not diagnosed with CHI-F until after death at 4 months of age (5). Pancreatic surgery was performed for alleviation of sustained hypoglycaemia in patients with poor or inappropriate responses to medical therapy, Table 2. All pancreatic tissue for research was used in accordance with ethical approval, national codes of practice and informed consent.
Tissue Samples, Histochemistry, and Immunohistochemistry
Immunohistochemistry was performed as described previously on heat-mediated antigen retrieved 5 μm thick sections of tissue, which were fixed in 4% paraformaldehyde overnight and embedded in paraffin wax as previously described (23–26). All slides were then digitized as described previously (23–26).
Statistical Analysis
Unless otherwise stated data are presented as mean ± standard error and a one-way analysis of variance (ANOVA) was used to determine whether there were significant differences among the means of datasets.
Results
Heterogeneity in the Structure of Focal Lesions
To investigate the relationship between clinical phenotype and islet lesions, we assessed the microscopic structures of focal lesions using tissues from patients following surgery. This revealed two broad types of lesions. First, islet tissues were found to be loosely connected within a focal area with extensions and organized clusters of islet cells within normal tissue, Figures 1A, 2A. In these cases (n = 7/25)—designated Type 1 lesions, the expansion of islet cell mass was not contained or encapsulated within a collagen matrix boundary that was continuous with the lesion. Second, in n = 18/25 cases we found that islet cells were localized to a discrete focal lesion and encapsulated by a defined extracellular matrix to support the confinement of cells, Figure 1B (see also Figure 2B). These lesions we designated Type 2, and we found no evidence of islet cells hyperplasia into the surrounding normal tissue. The size of the area occupied by the focal lesion was significantly larger in tissues with a poorly defined structure than those lesions with a defined organization 49.6 ± 13.6 mm2 (mean ± S.E.M., n = 7; range 6–103 mm2) vs. 23.2 ± 3 (n = 18; range 8–60.7 mm2), p < 0.02.
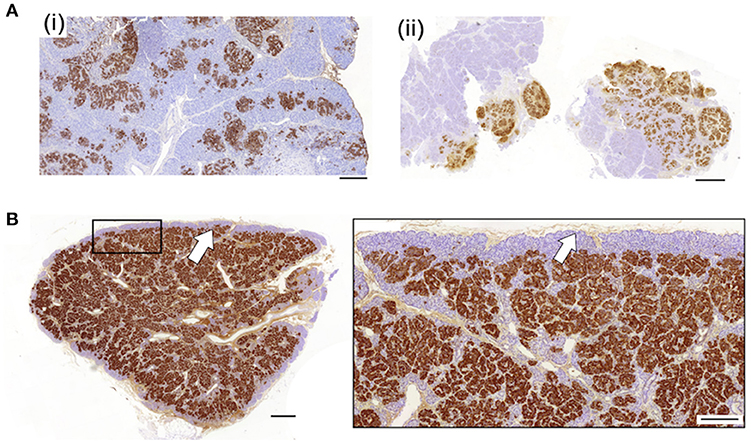
Figure 1. Diverse histopathology in focal CHI. Two discrete profiles of CHI-F are represented in this figure. Panel (A) shows typical images of Type 1 CHI-F tissue from two patients [CHI-F14 (i) and CHI-F3 (ii)]. The endocrine mass—insulin-positive cells, is poorly defined and not encapsulated. By contrast, in Type 2 tissue, Panel (B) (data obtained from CHI-F7), islet cell hyperplasia is highly localized and demarcated with a defined matrix capsule, arrow. Scales bars: Panel (A) (i) and (ii) 0.2 mm; Panel (B) (i) 0.5 mm, (ii) 0.2 mm.
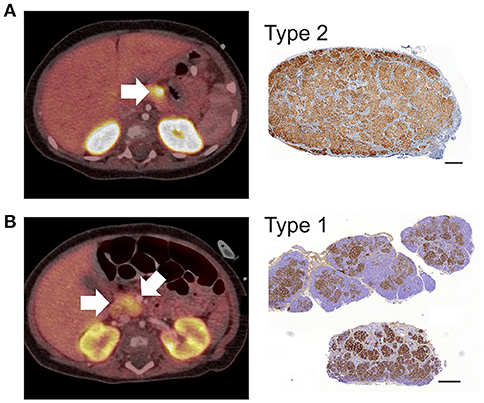
Figure 2. 18F-DOPA PET-CT images and CHI-F heterogeneity. All panels show combined positron emission tomography (PET)/computed tomography (CT) scan images and the corresponding immunohistochemical images (Ins+) of focal lesions following surgery. In Panel (A), the PET/CT scan reveals a discrete lesion with high uptake of tracer in the distal body of the pancreas. The corresponding histological data shows that the focal lesion was highly localized,—typical Type 2 lesion. Panel (B) shows a typical Type 1 lesion. The PET/CT scan reveals heterogeneous uptake of tracer with the highest SUV in the neck of pancreas (arrow). The corresponding histological data shows a heterogeneous lesion domain with expansion of islet cells into the healthy region of the pancreas. The data in (A) was obtained from patient CHI-F17 and (B) from CHI-F10. Scale bars 0.5 mm.
Early- and Late-Onset Hypoglycaemia in Focal CHI Patients
Using the tissue typing criteria of focal lesions we undertook a retrospective study of the clinical datasets of all 25 focal-CHI patients in our center, Table 1. Patients with Type 1 focal lesions had marked differences in the onset time of severe hypoglycaemia compared to patients with Type 2 focal lesions. On average, early-onset hypoglycaemia was detected 2.8 ± 0.8 days (n = 7, range 1–7 days) following birth compared to 56.1 ± 14.3 days (n = 18, range 1–180 days) in patients with Type 2 lesions. In this sub-group, patients were also younger at the time of surgery compared to late-onset patients, 2.5 ± 0.6 months (n = 6; 1 to 5 months) vs. 5.1 ± 1.1 months (n = 18; range 1 to 19 months). These differences were unrelated to birth-weight (a marker of intrauterine anabolic effects of insulin) 3.9 ± 0.2 kg (n = 7) vs. 4.4 ± 0.4 kg (n = 18), medical therapy (Table 2), genetic cause of disease (Table 3) or location of the lesion.
In patients harboring discrete encapsulated focal lesions, a localized/focal uptake of 18F-DOPA in PET was diagnostic in 94% of cases (n = 16/17), Table 1 and Figure 2A. During surgery (n = 17), encapsulated lesions were visible, palpable (laparotomy), and easily dissected (laparoscopy), thereby making surgery less complicated. There were no post-operative complications in this group. Apart from one patient, normoglycaemia was achieved soon after lesionectomy. We found that 88% of children had no long-term adverse consequences (n = 15/17), Table 1.
For patients with poorly demarcated/unencapsulated focal lesions—Type 1, 75% of cases (n = 3/4) were also found to have a focal uptake pattern following PET-CT. Only one patient (CHI-F10) had a heterogeneous pattern of 18F-DOPA, Figure 2B. For these patients surgical procedures were more complex as only 33% (n = 2/6) of lesions were visible at the time of surgery and no lesion was palpable in open laparotomy. This led to a requirement for greater tissue dissection and more than one frozen section biopsy to find the anatomical location of the focal lesion. All patients who underwent surgery for Type 1 lesions (n = 6) required either an extended resection or a subtotal pancreatectomy to stabilize blood glucose levels (Table 1). Long-term outcomes were less favorable for these patients. Recurrent but transient hypoglycaemia was noted in three (50%) patients; both short- and long-term complications ranging from developmental delay, blindness, and hyperglycaemia, were also noted, Table 1.
Discussion
Inappropriate insulin release from the CHI pancreas results from abnormalities in either all islets of Langerhans—diffuse disease, or from localized defects originating from a small focal lesion, CHI-F. The genetic basis of CHI-F has been widely reported, but the pathobiology of the lesion and impact upon the pancreas has not been thoroughly addressed. Further, whilst clinical heterogeneity in CHI-F has been reported (27, 28), the underlying associations or causes of this is unknown. In this study, we describe a direct correlation between the severity of focal CHI and the extent of lesion encapsulation. Islet tissue that is loosely organized and devoid of a matrix capsule—designated Type 1, causes early-onset hypoglycaemia, requiring complex surgery and suboptimal surgical outcomes. By contrast, later-onset disease with optimal outcome is directly correlated with marked islet cell encapsulation, designated Type 2. These observations may form the basis of a diagnostic process and are a step forward to understanding not only the pathobiology of the disease but also correlating histopathology with clinical phenotype.
CHI-F is a somatic-recessive endocrine disorder arising through a combination of events involving deregulation of imprinting in region Ch.11p15.5 and a somatic reduction to homozygosity of a paternal gene defect in either ABCC8 or KCNJ11. At least 12 imprinted genes are known to be encoded by the Ch.11p15.5 imprinting domain and several are thought to be involved with β-cell overgrowth—H19, P57KIP2, and IGF2, in CHI-F (11, 15). Thus, at some stage during development and subsequent to islet cell formation, loss of maternal imprinting results in the inappropriate proliferation of β-cells harboring loss of function defects in KATP channel genes.
Normally, islets of Langerhans have a highly organized structure involving the spatial arrangement of endocrine cells, which are encapsulated and vascularized. The interface between islet and exocrine tissue is composed of an interstitial matrix and a basement membrane. Whilst the islet basement membrane acts as a functional barrier separating the exocrine and endocrine domains, the connective tissue/extracellular matrix (ECM) also serves a diverse number of functions (29–31). These include organizing the connections between endocrine cells, vascular endothelial cells, neural cells, and immune cells. These interactions have functional significance by facilitating the rapid exchange of oxygen, nutrients, metabolites, signaling hormones, and islet hormones (32, 33). In focal disease, not only does β-cell proliferation cause a marked increase in β-cell numbers, but it will also lead to a deregulation of the interactions between the ECM and islet cells. This is highly likely to lead to loss of functional integrity and may contribute to the pathobiology of disease.
There is little information on how timing of the loss of maternal imprinting impacts on the sequential organization of the islet cell architecture involving endocrine cellular proliferation, encapsulation and vascularization. Indeed, since loss of heterozygosity can occur at any time during embryogenesis, our data show that CHI-F is underpinned by heterogeneity in both structure and organization of the lesion domain. We found that in ~30% of patients, the organizational aspects of focal lesion were poor and the limits of the endocrine cell mass were difficult to define (5). This form of CHI-F has been alluded too by others in both large cohorts of patients or unusual case reports without reference to clinical severity of pathobiology (27, 28, 34–37). In this study, we found no correlation between the genetic cause of disease and the type of focal condition, but we did make the important observation that children with poorly formed lesions had much earlier onset of clinical symptoms than those children with discrete focal lesions. This may suggest a slower rate of endocrine cell and vascular proliferation in the latter group, but it seems more likely that this relates to differences in the manner of interactions with the ECM within the growing lesions. Because of the underlying architecture, it was easier to localize discrete lesions by 18F-Dopa PET-CT, undertake curative surgery and minimize long-term complications.
In summary, we have systematically examined the clinical profiles and the structure and organization of focal lesions in children with CHI-F. We found a degree of heterogeneity that was strongly correlated with disease severity, surgical management, and long-term clinical outcomes. The organization of islet cell structures is strongly determined by endocrine cells and their release of growth factors for vascular events. We therefore, conclude that the β-cell pathology in CHI-F extends beyond the loss of function defects in ATP-sensitive K+ channel genes and includes heterogeneity in interactions between islet cells and extracellular matrix organization.
Ethics Statement
This study was carried out in accordance with the recommendations of National Research Ethics Service (NRES) with written informed consent from all subjects. All subjects gave written informed consent in accordance with the Declaration of Helsinki. The protocol was approved by the North West Research Ethics Committee—Project Reference Number: 07/H1010/88.
Author Contributions
All authors contributed to the study and writing of the manuscript. RC, MSE, MS, KC, IB, and MD conceived the design of the study. MSE, DY, BH, WM, MN, EC, SB, ZM, RS, RP, SF, and SE collected data with the assistance of RC, IB, EC, and MD. Data were analyzed by IB and MD. The draft manuscript was prepared by MD and IB with critical review and agreement with all authors. The final manuscript was prepared by IB and MD.
Funding
This work was funded by NORCHI Charitable Fund, Manchester University Hospitals and University of Manchester, The National Institute for Health, The Million Dollar Bike Fund, Diabetes UK, Wellcome Trust, The RCUK.
Conflict of Interest Statement
The authors declare that the research was conducted in the absence of any commercial or financial relationships that could be construed as a potential conflict of interest.
Acknowledgments
The authors received support from the NORCHI Charitable Fund (MD, IB, RP, and KC), and innovation supporting funds from Manchester University Hospitals and University of Manchester (MD and IB), The National Institute for Health Research (MD, IB, RP, and KC), The Million Dollar Bike Fund (Pilot Award Number: MDBR-16-100-CHI; MD, IB, and KC), and Diabetes UK (Award Number: 12/0004608; MD and KC). KC was supported by a RCUK Academic Fellowship. We are grateful to Roger Meadows (Bioimaging Core Facility), and Peter Walker (Histology Core Facility) the University of Manchester for their inputs to the datasets. We are also grateful to research nurses and clinical colleagues at Central Manchester University Hospitals NHS Trust and the Manchester Biomedical Research Centre.
References
1. Menni F, Lonlay P, de Sevin C, Touati G, Peigné C, Barbier V, et al. Neurologic outcomes of 90 neonates and infants with persistent hyperinsulinemic hypoglycemia. Pediatrics (2001) 107:476–9. doi: 10.1542/peds.107.3.476
2. Meissner T, Wendel U, Burgard P, Schaetzle S, Mayatepek E. Long-term follow-up of 114 patients with congenital hyperinsulinism. Eur. J. Endocrinol. (2003) 149:43–51.
3. Lord K, Dzata E, Snider KE, Gallagher PR, De León DD. Clinical presentation and management of children with diffuse and focal hyperinsulinism: a review of 223 Cases. J Clin Endocrinol Metab. (2013) 98:1786–9. doi: 10.1210/jc.2013-2094
4. Dyer C. Death of baby from hyperinsulinaemic hypoglycaemia prompts safety upgrade at Barts trust. BMJ (2018) 360:k1370. doi: 10.1136/bmj.k1370
5. Chinoy A, Banerjee I, Flanagan SE, Ellard S, Han B, Mohamed Z, et al. Focal congenital hyperinsulinism as a cause for sudden infant death. Pediatr Dev Pathol. (2018). doi: 10.1177/1093526618765376. [Epub ahead of print].
6. Guyot A, Moreau F, Eberhard M, Gaulier JM, Paraf F. Congenital hyperinsulinism revealed by sudden infant death. Ann Pathol. (2017) 37:429–32. doi: 10.1016/j.annpat.2017.07.003
7. Avatapalle HB, Banerjee I, Shah S, Pryce M, Nicholson J, Rigby L, et al. Abnormal neurodevelopmental outcomes are common in children with transient congenital hyperinsulinism. Front Endocrinol. (2013) 4:60. doi: 10.3389/fendo.2013.00060
8. De Leon DD, Stanley CA. Mechanisms of disease: advances in diagnosis and treatment of hyperinsulinism in neonates. Nat Clin Pract Endocrinol Metab. (2007) 3:57–68. doi: 10.1038/ncpendmet0368
9. Yorifuji T. Congenital hyperinsulinism: current status and future perspectives. Ann Pediatr Endocrinol Metab. (2014) 19:57–68. doi: 10.6065/apem.2014.19.2.57
10. Banerjee I, Skae MS, Flanagan SE, Rigby L, Patel L, Didi M, et al. The contribution of rapid KATP channel gene mutation analysis to the clinical management of children with congenital hyperinsulinism. Eur J Endocrinol. (2011) 164:733–40. doi: 10.1530/EJE-10-1136
11. de Lonlay-Debeney P, Poggi-Travert F, Fournet JC, Sempoux C, Dionisi Vici C, Brunelle F, et al. Clinical features of 52 neonates with hyperinsulinism. N Engl J Med. (1999) 340:1169–75.
12. Lovvorn HN III, Nance ML, Ferry RJ Jr Stolte L, Baker L, O'Neill JA Jr, et al. Congenital hyperinsulinism and the surgeon: lessons learned over 35 years. J Pediatr Surg. (1999) 34:786–92, discussion 792–3.
13. Pierro A, Nah SA. Surgical management of congenital hyperinsulinism of infancy. Semin Pediatr Surg. (2011) 20:50–3. doi: 10.1053/j.sempedsurg.2010.10.009
14. Beltrand J, Caquard M, Arnoux JB, Laborde K, Velho G, Verkarre V, et al. Glucose metabolism in 105 children and adolescents after pancreatectomy for congenital hyperinsulinism. Diab Care (2012) 35:198–203. doi: 10.2337/dc11-1296
15. de Lonlay P, Fournet JC, Rahier J, Gross-Morand MS, Poggi-Travert F, Foussier V, et al. Somatic deletion of the imprinted 11p15 region in sporadic persistent hyperinsulinemic hypoglycemia of infancy is specific of focal adenomatous hyperplasia and endorses partial pancreatectomy. J Clin Invest. (1997) 100:802–7.
16. Blomberg BA, Moghbel MC, Saboury B, Stanley CA, Alavi A. The value of radiologic interventions and (18)F-DOPA PET in diagnosing and localizing focal congenital hyperinsulinism: systematic review and meta-analysis. Mol Imaging Biol. (2013) 15:97–105. doi: 10.1007/s11307-012-0572-0
17. Zani A, Nah SA, Ron O, Totonelli G, Ismail D, Smith VV, et al. The predictive value of preoperative fluorine-18-L-3,4-dihydroxyphenylalanine positron emission tomography-computed tomography scans in children with congenital hyperinsulinism of infancy. J Pediatr Surg. (2011) 46:204–8. doi: 10.1016/j.jpedsurg.2010.09.093
18. Masue M, Nishibori H, Fukuyama S, Yoshizawa A, Okamoto S, Doi R, et al. Diagnostic accuracy of [18F]-fluoro-L-dihydroxyphenylalanine positron emission tomography scan for persistent congenital hyperinsulinism in Japan. Clin Endocrinol. (2011) 75:342–6. doi: 10.1111/j.1365-2265.2011.04072.x
19. Vajravelu ME, De León DD. Genetic characteristics of patients with congenital hyperinsulinism. Curr Opin Pediatr. (2018) 30:568–75. doi: 10.1097/MOP.0000000000000645
20. Banerjee I, Avatapalle B, Padidela R, Stevens A, Cosgrove KE, Clayton PE, et al. Integrating genetic and imaging investigations into the clinical management of congenital hyperinsulinism. Clin Endocrinol. (2013) 78:803–13. doi: 10.1111/cen.12153
21. Stanley CA. Perspective on the genetics and diagnosis of congenital hyperinsulinism disorders. J Clin Endocrinol Metab. (2016) 101:815–26. doi: 10.1210/jc.2015-3651
22. Fournet JC, Mayaud C, de Lonlay P, Gross-Morand MS, Verkarre V, Castanet M, et al. Unbalanced expression of 11p15 imprinted genes in focal forms of congenital hyperinsulinism: association with a reduction to homozygosity of a mutation in ABCC8 or KCNJ11. Am J Pathol. (2001) 158:2177–84. doi: 10.1016/S0002-9440(10)64689-5
23. Han B, Newbould M, Batra G, Cheesman E, Craigie RJ, Mohamed Z, et al. Enhanced islet cell nucleomegaly defines diffuse congenital hyperinsulinism in infancy but not other forms of the disease. Am J Clin Pathol. (2016) 145:757–68. doi: 10.1093/ajcp/aqw075
24. Salisbury RJ, Han B, Jennings RE, Berry AA, Stevens A, Mohamed Z, et al. Altered phenotype of β-cells and other pancreatic cell lineages in patients with diffuse congenital hyperinsulinism in infancy due to mutations in the ATP-sensitive K-channel. Diabetes (2015) 64:3182–8. doi: 10.2337/db14-1202
25. Szymanowski M, Estebanez MS, Padidela R, Han B, Mosinska K, Stevens A, et al. mTOR inhibitors for the treatment of severe congenital hyperinsulinism: perspectives on limited therapeutic success. J Clin Endocrinol Metab. (2016) 101:4719–29. doi: 10.1210/jc.2016-2711
26. Han B, Mohamed Z, Salomon Estebanez M, Craigie RJ, Newbould M, Cheesman E, et al. Atypical forms of congenital hyperinsulinism in infancy are associated with mosaic patterns of immature islet cells. J Clin Endocrinol Metab. (2017) 102:3261–7. doi: 10.1210/jc.2017-00158
27. Ismail D, Kapoor RR, Smith VV, Ashworth M, Blankenstein O, Pierro A, et al. The heterogeneity of focal forms of congenital hyperinsulinism. J Clin Endocrinol Metab. (2012) 97:E94–9. doi: 10.1210/jc.2011-1628
28. Suchi M, MacMullen CM, Thornton PS, Adzick NS, Ganguly A, Ruchelli ED, et al. Molecular and immunohistochemical analyses of the focal form of congenital hyperinsulinism. Mod Pathol. (2006) 19:122–9. doi: 10.1038/modpathol.3800497
29. Cross SE, Vaughan RH, Willcox AJ, McBride AJ, Abraham AA, Han B, et al. Key Matrix proteins within the pancreatic islet basement membrane are differentially digested during human islet isolation. Am J Trans. (2017) 17:451–61. doi: 10.1111/ajt.13975
30. Wang RN, Rosenberg L. Maintenance of beta-cell function and survival following islet isolation requires re-establishment of the islet-matrix relationship. J Endocrinol. (1999) 163:181–10. doi: 10.1677/joe.0.1630181
31. Smink AM, de Vos P. Therapeutic strategies for modulating the extracellular matrix to improve pancreatic islet function and survival after transplantation. Curr Diab Rep. (2018) 18:39. doi: 10.1007/s11892-018-1014-4
32. Aamodt KI, Powers AC. Signals in the pancreatic islet microenvironment influence beta-cell proliferation. Diab Obes Metab. (2017) 19(Suppl. 1):124–36. doi: 10.1111/dom.13031
33. Bosco D, Meda P, Halban PA, Rouiller DG. Importance of cell-matrix interactions in rat islet beta-cell secretion in vitro: role of alpha6beta1 integrin. Diabetes (2000) 49:233–43. doi: 10.2337/diabetes.49.2.233
34. Kühnen P, Matthae R, Arya V, Hauptmann K, Rothe K, Wächter S, et al. Occurrence of giant focal forms of congenital hyperinsulinism with incorrect visualization by (18) F DOPA-PET/CT scanning. Clin Endocrinol. (2014) 81:847–54. doi: 10.1111/cen.12473
35. Laje P, States LJ, Zhuang H, Becker SA, Palladino AA, Stanley CA, et al. Accuracy of PET/CT Scan in the diagnosis of the focal form of congenital hyperinsulinism. J Pediatr Surg. (2013) 48:388–93. doi: 10.1016/j.jpedsurg.2012.11.025
36. Barthlen W, Varol E, Empting S, Wieland I, Zenker M, Mohnike W, et al. Surgery in focal congenital hyperinsulinism (CHI) - the “Hyperinsulinism Germany International” Experience in 30 Children. Pediatr Endocrinol Rev. (2016) 14:129–37. doi: 10.17458/PER.2016.BVE.Surgeryinfocal
Keywords: congenital hyperinsulinism, insulin, hypoglycaemia, islet, positron emission tomography, focal, pancreas, β-cell
Citation: Craigie RJ, Salomon-Estebanez M, Yau D, Han B, Mal W, Newbould M, Cheesman E, Bitetti S, Mohamed Z, Sajjan R, Padidela R, Skae M, Flanagan S, Ellard S, Cosgrove KE, Banerjee I and Dunne MJ (2018) Clinical Diversity in Focal Congenital Hyperinsulinism in Infancy Correlates With Histological Heterogeneity of Islet Cell Lesions. Front. Endocrinol. 9:619. doi: 10.3389/fendo.2018.00619
Received: 17 July 2018; Accepted: 27 September 2018;
Published: 17 October 2018.
Edited by:
Fabrizio Barbetti, Università degli Studi di Roma Tor Vergata, ItalyReviewed by:
Eli Hershkovitz, Soroka Medical Center, IsraelLuigi R. Garibaldi, University of Pittsburgh, United States
Copyright © 2018 Craigie, Salomon-Estebanez, Yau, Han, Mal, Newbould, Cheesman, Bitetti, Mohamed, Sajjan, Padidela, Skae, Flanagan, Ellard, Cosgrove, Banerjee and Dunne. This is an open-access article distributed under the terms of the Creative Commons Attribution License (CC BY). The use, distribution or reproduction in other forums is permitted, provided the original author(s) and the copyright owner(s) are credited and that the original publication in this journal is cited, in accordance with accepted academic practice. No use, distribution or reproduction is permitted which does not comply with these terms.
*Correspondence: Mark J. Dunne, bWFyay5qLmR1bm5lQG1hbmNoZXN0ZXIuYWMudWs=