- 1Augustana Faculty, University of Alberta, Camrose, AB, Canada
- 2Physical Activity and Diabetes Laboratory, Alberta Diabetes Institute, Edmonton, AB, Canada
- 3Faculty of Kinesiology, Sport and Recreation, University of Alberta, Edmonton, AB, Canada
- 4Diabetes Research Unit and School of Sport and Exercise Science, Swansea University, Swansea, United Kingdom
Closed-loop systems for patients with type 1 diabetes are progressing rapidly. Despite these advances, current systems may struggle in dealing with the acute stress of exercise. Algorithms to predict exercise-induced blood glucose changes in current systems are mostly derived from data involving relatively young, fit males. Little is known about the magnitude of confounding variables such as sex, age, and fitness level—underlying, uncontrollable factors that might influence blood glucose control during exercise. Sex-related differences in hormonal responses to physical exercise exist in studies involving individuals without diabetes, and result in altered fuel metabolism during exercise. Increasing age is associated with attenuated catecholamine responses and lower carbohydrate oxidation during activity. Furthermore, higher fitness levels can alter hormonal and fuel selection responses to exercise. Compounding the limited research on these factors in the metabolic response to exercise in type 1 diabetes is a limited understanding of how these variables affect blood glucose levels during different types, timing and intensities of activity in individuals with type 1 diabetes (T1D). Thus, there is currently insufficient information to model a closed-loop system that can predict them accurately and consistently prevent hypoglycemia. Further, studies involving both sexes, along with a range of ages and fitness levels, are needed to create a closed-loop system that will be more precise in regulating blood glucose during exercise in a wide variety of individuals with T1D.
Introduction
Type 1 diabetes (T1D) is an autoimmune disorder that destroys an individual's insulin-producing pancreatic β-cells (1). Despite many advances over the years, T1D remains a difficult disorder to manage. Exercise is very beneficial for individuals with T1D, with known improvements in cardiovascular health, insulin sensitivity, and body composition (2). However, fear of low blood glucose, or hypoglycemia, is often a barrier to partaking in exercise in this population (3). Much research has gone into investigating ways to keep blood glucose levels in the target range during and after exercise in individuals with T1D (4), most of which has noted a great deal of variability in responses.
In the past 5 years, there has been impressive progress in the development of a closed-loop system for patients with T1D to better control blood glucose. Improvements in the accuracy of hand-held glucose monitors to meet ISO criteria (5) and continuous glucose monitoring systems (CGM) (6, 7) have made this progress possible. Device development has advanced to a degree such that both single hormone (insulin) and dual hormone (insulin and glucagon) systems show improvements in time spent in target blood glucose ranges compared to continuous subcutaneous insulin infusion (insulin pump) systems when tested in small sample groups under free-living conditions (8).
In spite of these advances, perfecting the system to deal with situations other than rest, namely physical activity/exercise is still recognized as a major challenge (9–11). The need for a smart sensor that is able to detect the onset of exercise, as well as its intensity (which can have substantially different impacts on blood glucose responses) (12), has been identified. Indeed variables such as heart rate, skin temperature, heat flux, accelerometry and galvanic skin response, have been shown to correlate with changes in blood glucose during various types of exercise (13). Simply turning off insulin delivery upon sensing the start of activity, however, may be insufficient to completely prevent declines in blood glucose, and subsequently hypoglycemia (11), unless exercise is performed shortly after a meal (14). It has also been noted that a closed loop system, or artificial pancreas, will have difficulty replicating the multitude of feed-forward and feedback responses related to blood glucose equilibrium (10), in part due to the relatively long half-life of even the shortest acting synthetic insulin.
The variability in exercise responses will also be a major challenge to creating a system that will be able to adapt to all types and durations of activity for a wide variety of individuals, with a broad range of blood glucose levels at the start of exercise. The need for a sensor that not only recognizes changes in blood glucose, but also the onset, type and intensity, of physical activity/exercise has been discussed in detail elsewhere (8, 10). Current algorithms attempting to manage changes in blood glucose during exercise are also lacking information. Acute studies of the effect of different exercise modalities on blood glucose in individuals with T1D are limited in terms of their type, timing (e.g., morning vs. evening), intensity and duration of activity. For the most part, they have small sample sizes of relatively young, fit, male participants, which may obscure some potentially important differences among blood glucose responses to exercise in physiologically different groups. While we know that insulin dosage, carbohydrate intake, time of day, and prior hypoglycemia can impact blood glucose responses to exercise in T1D (Figure 1), little is known about the relative impact of underlying physiological factors such as sex, age, and physical fitness, which are beyond the control of the individual.
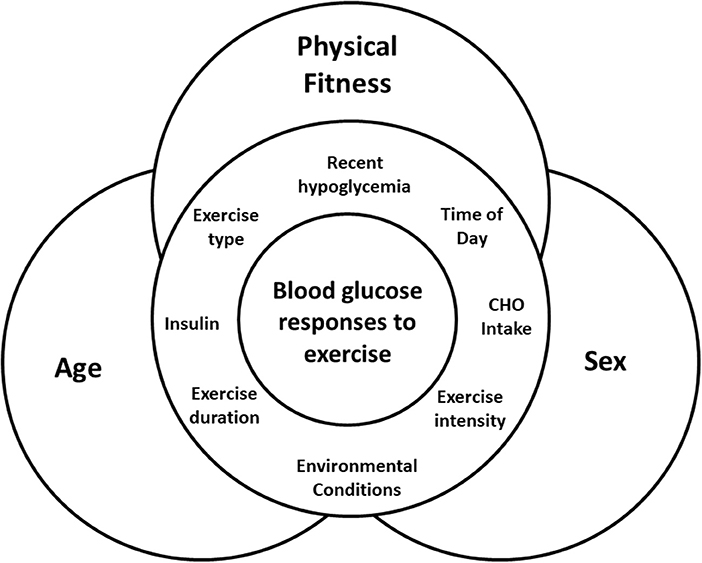
Figure 1. Interaction of factors that can impact blood glucose during exercise, with the physiological factors of age, sex, and physical fitness underlying and interacting with all of them.
What we do know about these physiological factors (age, sex, fitness) has, for the most part, only been measured in individuals without diabetes. For example, females (without diabetes) are known to have different hormonal responses to exercise than males (15–28), resulting in differences in fuel selection (15–18, 23, 29–35) that might impact changes in blood glucose during different types of exercise. The same can be said of older individuals compared to younger individuals (36–43), and those of high physical fitness compared to those of low physical fitness (37, 38, 44–50). Where tightly controlled insulin and glucagon responses will help maintain blood glucose levels in a tight range in individuals without diabetes, differences in counter-regulatory responses to exercise depending on age, sex, or physical fitness have the potential to make a greater impact where pancreatic islet function is impaired or absent. Overall, this questions the adequacy of using existing data from acute exercise studies in T1D for modeling these activities in the context of the artificial pancreas, as much of the current research with T1D participants fails to take into account underlying physiological factors that will probably interact to affect blood glucose control. This review aims to summarize the state of knowledge on these factors, and to infer their potential impact on blood glucose responses to exercise in individuals with T1D.
Effect of Sex on Exercise Responses in Individuals Without Diabetes
A large proportion of studies examining exercise metabolism have been performed uniquely in males. While limited, studies comparing the neuroendocrine and metabolic responses to exercise in healthy men and women have shown clear sex-related differences in the patterns of fuel selection (15–18, 23, 29–35), catecholamine response (15–22) and other hormonal responses such as estrogen (23–25) and growth hormone (16, 26–28). All of these factors have the potential to influence blood glucose concentrations both acutely, and in the hours following exercise (Table 1).
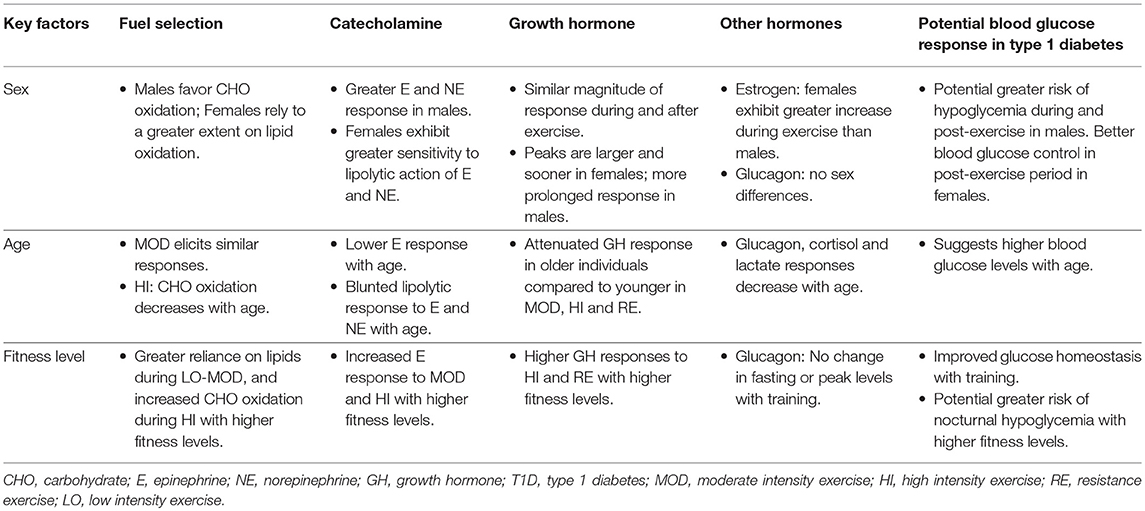
Table 1. Summary of hormonal responses to exercise by sex, age, and fitness level and their potential effect on blood glucose responses in type 1 diabetes.
Fuel Selection
There is a clear difference between males and females in fuel selection during exercise. While there appears to be no difference between the sexes at rest (51, 52), females exhibit a lower respiratory exchange ratio (RER) during exercise in the fasted state, indicating a greater reliance on lipolysis than males for energy production 15–18, 23, 30–35, 53. This trend has been observed in healthy males and females during endurance exercise (15, 29–32, 34), submaximal exercise (16, 17, 23, 53), high intensity exercise (23) and resistance exercise 34.
The RER measurements are consistent with what has been noted at the cellular level: females use more myocellular triacylglycerol than males, whereas males deplete a greater portion of their glycogen stores during various types of exercise (18, 29, 54). Additionally, it has been observed that men shift to using carbohydrates as the dominant fuel source earlier [at 45 ± 1% of the participant's maximal aerobic capacity (VO2max)] than women (at 52 ± 1% VO2max, p < 0.01) in a graded treadmill exercise test to exhaustion (55). While women still shift to oxidizing carbohydrates with higher intensity exercise, this shift is later and less extreme than in men. Less reliance on carbohydrate oxidation during exercise [53.1 ± 2.1% in men vs. 45.7 ± 1.8% (p < 0.01) in women during 2 h of cycling at 40%VO2max] has been related to less depletion of glycogen stores in women (15).
Women also seem to be better able to conserve glycogen stores after extended exercise (90 min at 65% of VO2max) (24), sparing the need for a large uptake of plasma glucose in the recovery period. One study found that women had a more precise defense of homeostasis in the recovery period post-exercise, including the control of blood glucose concentration and fuel selection (56). Due to their greater inherent capacity for lipid oxidation during exercise, women are able to regain control over glycemia and glucose flux in recovery more quickly than men (56). Therefore, men often show an increased rate of lipid mobilization post-exercise compared to women, in order to preserve glucose concentrations when restoring depleted glycogen stores from exercise (15, 33).
Important Hormones Mediating Fuel Selection
Several hormones, in addition to insulin and glucagon, are known to affect blood glucose homeostasis around exercise. Epinephrine and norepinephrine stimulate a substantial increase in hepatic glucose production. Growth hormone is known to promote lipolysis, which may in turn decrease reliance on blood glucose. Levels of estrogen, have also been shown to impact rates of lipolysis. All of these hormones respond differently between the sexes to an acute bout of exercise.
Catecholamines
There is a noticeable sex-related difference in catecholamine response to various forms and intensities of exercise. While a few exceptions exist (57, 58), the majority of studies report a significantly higher catecholamine response to various types of exercise in males compared to females 15–22. This trend has been found during moderate exercise, whether performed in the fasted state (16), or the postprandial state (18). It has also been found to hold during moderate intensity exercise of longer (2 h) duration (15), high intensity resistance exercise (59), and maximal sprints in trained individuals (19). This finding is important in blood glucose regulation, as high catecholamine levels will stimulate hepatic glycogenolysis and gluconeogenesis, increasing blood glucose levels during activity (15, 60–62), but potentially decreasing blood glucose levels post-exercise when glycogen stores are being replenished.
While men have shown an increased catecholamine response to exercise compared to women, there is also an apparent sex-related difference in the adrenergic receptor sensitivity during exercise. Horton et al. (15) found that men had significantly elevated levels of epinephrine, which stimulates lipolysis, at the end of moderate endurance exercise (post-exercise epinephrine: men = 208 ± 36 vs. women = 121 ± 15 pg/ml, p < 0.007), but had similar levels of circulating glycerol to women. This finding would suggest a greater sensitivity to the lipolytic action of the catecholamines in women. It is postulated that women may have a higher ß-adrenergic sensitivity, and decreased α-adrenergic sensitivity compared to men (17, 18, 23, 63). ß-adrenoreceptors are known to stimulate, whereas α-adrenoreceptors inhibit lipolysis, and thus a higher sensitivity of ß receptors in women during exercise results in greater net lipolysis (17). Indeed, when epinephrine was kept constant by infusion (63), women showed greater lipolytic responses than men, suggesting less activation of the α-adrenergic receptors in women. Overall, this would support the trends seen in fuel selection, where women have relatively greater levels of lipolysis and fat oxidation than men during exercise.
Estrogen
Another factor influencing fuel selection during exercise is the difference in estrogen, between men and women. Estrogen, specifically 17ß-estradiol, has been shown to promote lipid oxidation and glycogen sparing during exercise (15, 23–25). Two separate blinded placebo control studies have shown that intake of 17ß-estradiol in males shifted fuel selection in the direction of lipid oxidation in recreationally-active men so that it was similar to that of women during moderate (65% VO2max) aerobic exercise (25, 64).
While sex has a prominent effect on fuel selection, different phases of the menstrual cycle can also influence metabolism during exercise, and should thus be controlled for in studies involving women. Most studies test women in the early follicular phase, where estrogen concentrations do not differ markedly between men and women (34). This limits the proportion that estrogen contributes to determining fuel selection during exercise. During the luteal phase of the menstrual cycle, there is a higher concentration of estrogen and, consequently, a higher relative rate of fat utilization in females (31). While RER values (and therefore glucose utilization) are uniformly lower in women performing endurance exercise when compared to men, the luteal phase has been shown to produce lower glucose appearance and disappearance rates and less glycogen depletion than when exercise is performed in the follicular phase (24). There is also a greater increase in estradiol during exercise (both aerobic and resistance), which may contribute to greater fat oxidation for energy production in females during the luteal phase (35, 65, 66).
Growth Hormone
There is a lack of consensus regarding differences in growth hormone response to exercise between men and women. While some studies report a significantly increased growth hormone response in men compared to women after sprint (19) or submaximal exercise (67), others report an increased growth hormone response in women following resistance exercise (26, 68) or sprints (28). The majority of studies, however, report a similar response between the sexes in which both men and women experience an increase in growth hormone levels during and following exercise that is >10 min in length (16, 65, 69–71).
Although the majority of studies report similar relative increases in growth hormone during exercise compared to resting controls, there is a sexually dimorphic pattern in the way this occurs. Growth hormone peaks in women are reported to be larger and appear sooner than men, whereas men sustain a more prolonged response (16, 26–28). Sex-related differences in growth hormone levels can be attributed to a lack of testosterone response in women (65). Because women experience little or no increase in testosterone levels in response to exercise, growth hormone appears to compensate for the anabolic requirements stimulated by acute exercise (34, 72). Additionally, women have a higher resting basal level of growth hormone (65, 68, 70), particularly in the early follicular phase of the menstrual cycle (73). Because most exercise studies are performed on women during the early follicular phase of the menstrual cycle due to low levels of estrogen in this phase, there are marked sex differences in basal growth hormone levels (34), and subsequently higher levels of growth hormone during and after exercise. Additionally, increased levels of circulating estradiol are associated with higher growth hormone concentrations (26), as estradiol releases a growth hormone stimulating factor (65).
Increases in growth hormone stimulate lipolysis and lipid oxidation, suppressing glucose oxidation and consequently sparing plasma glucose (74). Higher resting levels of estradiol in women stimulates growth hormone release, which inhibits glucose uptake, thereby enhancing lipolysis, and again, preserving plasma glucose levels in women. The difference in growth hormone response to exercise between the sexes, thus, has the potential to affect blood glucose control directly.
Effect of Age on Exercise Responses in Individuals Without Diabetes
While there are clear differences in exercise responses between the sexes, it would seem that declines in functional fitness with age occur evenly in both sexes, and are due mostly to the reduction in upper and lower limb muscle strength, increases in body-fat percentage, and decreases in flexibility, agility and endurance (36). There are also changes in the hormonal response to exercise, which may alter fuel selection patterns during different types of activity, resulting in differences in fuel usage during exercise between younger and older individuals. Aging is also associated with a decrease in cardio-respiratory fitness (reflected by lower maximum oxygen consumption values) (75), which in itself may impact exercise responses (discussed in the section on physical fitness below). There are minimal published data to date examining how these age-related alterations in exercise response affect changes in blood glucose during activity of any type.
Body Composition
Aging is characterized by a decrease in muscle mass and strength (76), and an increase in adiposity (77), which generally translates into declines in physical capacity (78). Older adults also have a decreased resting metabolic rate, which may only be partly due to the changes in body composition (79). A higher level of abdominal adiposity in particular is a significant predictor of insulin sensitivity in older adults (80). With decreases in muscle mass, metabolic rate and aerobic fitness, older individuals will be burning less fuel overall for the same relative intensity of exercise.
Fuel Selection
When exercise is of moderate intensity relative to the individual's peak aerobic rate, the proportion of fuel supplied by carbohydrates is similar among older and younger individuals (37, 76). However, where more intense/anaerobic exercise is concerned, carbohydrate oxidation decreases up to 20% from roughly 20–50 years of age in sedentary (but not trained) men (37). Sedentary men also have a decrease in the lactate threshold during sub-maximal exercise with age (38), however, in spite of this, younger men are able to produce more lactate during activities such as resistance exercise (39), thereby indicating a greater reliance on carbohydrates as a fuel source. This may be part of the reason why one study showed that blood glucose was ~1.0 mmol/L higher before, during and for 30 min after heavy resistance training in older (mean age ~62 years) men without diabetes compared to a younger cohort (mean age ~30 years) (39). Several of these differences between older and younger individuals can be attenuated, however, if a higher level of physical fitness is maintained through the life course. The impact of physical fitness will be discussed in greater detail in the following section.
Important Hormones Mediating Fuel Selection
Catecholamines
A lower reliance on carbohydrate with increasing age may, in part, be due to a lower epinephrine response to exercise of both moderate and high intensity with age. One study showed that men aged 60–70 years old experienced a lower epinephrine response to sub-maximal exercise (~70% VO2max) than 20–32 year old men regardless of fitness level (40). Similarly, ~15 min of exercise at ~78% VO2max resulted in a lower epinephrine response (109 ± 10 vs. 228 ± 29 pg/ml; p < 0.01) in a group of older men and women (mean 64 years) compared to younger adults (mean 24 years) (41). Very high intensity exercise (a maximal sprint cycle—Wingate test) also produced lower epinephrine responses in a group of male athletes with a mean age of 34 years (1.86 ± 0.26 mmol/l), compared to a group of athletes with a mean age of 21 years (3.24 ± 0.28 mmol/l; p < 0.05) (42). It should also be noted that in older men, there is a blunted lipolytic response to catecholamines compared to younger men, possibly due to decreased sensitivity of the hormone-sensitive lipase complex (43).
Growth Hormone
Older individuals show altered growth hormone responses to several different types and intensities of exercise. Where moderate intensity exercise is concerned, one study found that older men, whether they were trained (10.1 ± 5.5 ng/ml) or untrained (7.7 ± 6.5 ng/ml), had attenuated growth hormone response to 60 min of running at 70% VO2max compared to younger men (trained = 18.1 ± 6.7 vs. untrained = 20.4 ± 11.2 ng/ml) (40). Another study, however, found that responses were similar when exercise was sub-maximal, but that high intensity exercise produced a lower growth hormone response (11.3 ± 3.5 ng/ml vs. 21.9 ± 4.0 ng/ml; p < 0.05) in older men (mean ~64 years) compared to younger ones (mean ~23 years) (38). Differences were also found with resistance exercise, where growth hormone levels continued to increase post-exercise in young men (mean ~30 years) while decreasing in older (~62 years) men (39). Attenuated growth hormone responses to heavy resistance exercise have also been observed in older women (~62 years) in comparison to younger women (~24 years) (81), which may impact the fuels that are selected for use both during and after exercise.
Other Hormones
Glucagon and cortisol levels may also be influenced by increasing age. One study showed that glucagon levels were lower at rest in older men (60–70 years) compared to younger men (20–32 years), regardless of training status (older sedentary = 89 ± 28 pg/ml, older trained = 114 ± 34 pg/ml, younger sedentary = 141 ± 73 pg/ml, younger trained = 136 ± 35 pg/ml; p < 0.05 for both younger groups compared to both older groups) (40). Changes during exercise, however, were more dependent on physical fitness (discussed below) than age per se (40). Finally, lactate and cortisol responses to maximal exercise are also attenuated with age in both men and women (80), which would be consistent with what is known about reliance on carbohydrates as a fuel source being decreased within the same context.
Effect of Physical Fitness on Exercise Responses in Individuals Without Diabetes
While age-related declines in physical activity/exercise and hormonal responses lead to differences in fuel selection between older and younger individuals, some of these changes can be delayed or attenuated by maintaining higher levels of physical fitness (37, 38). In both younger and older men and women, higher levels of physical fitness lead to increases in hormonal responses to exercise fitness (37, 38, 46, 44), and a greater ability to mobilize and use fuels during exercise fitness (37, 38, 45–49) when compared to sedentary counterparts (Table 1). Individuals with higher fitness levels are also known to have greater insulin sensitivity than those who are sedentary (44, 50).
Fuel Selection
Improvements in physical fitness are known to change the proportion of fuels oxidized during exercise, with a greater reliance on lipids during low to moderate aerobic activities 37, 38, and an increase in carbohydrate dependence during high intensity exercise (37, 45, 59). At the same relative intensities, trained individuals will burn more calories, with the difference being made up for with fat oxidation (82), while at the same absolute intensities, trained individuals will have a greater reliance on lipid oxidation than untrained individuals (82). Male athletes are also more efficient at using lactate as a gluconeogenic precursor compared to sedentary males when exercising in the fasting state, which may spare endogenous glucose during extended and/or intense exercise (83).
The effects of physical fitness on fuel selection during exercise remain apparent as individuals age. In a study of older (~74 years) individuals, 16 weeks of endurance training caused an increase in whole body fat oxidation (from 166 ± 17 to 221 ± 28 μmol/min; p = 0.002) and a decrease in carbohydrate oxidation (from 3937 ± 483 to 3180 ± 461 μmol/min; p = 0.003) when exercise was performed at the same absolute intensity post-training (49). Another study of older (~64 years) males noted that carbohydrate oxidation increased with greater exercise intensity, and that trained men experienced a greater increase than untrained men (47). Similar to the case with sex- and age-related differences in metabolism, it is likely that fitness-related differences in hormonal responses to exercise drive these changes in fuel selection.
Important Hormones Mediating Fuel Selection
Catecholamines
As previously discussed, higher levels of epinephrine are associated with a greater amount of hepatic glycogenolysis, and consequently higher levels of blood glucose. Overall, higher levels of physical fitness in men are associated with increased epinephrine responses to both sub-maximal (38, 40) and high intensity exercise (46). In addition, the type of training performed will have an impact on the magnitude of epinephrine responses: male sprinters have a higher sympatho-adrenal output than endurance trained and untrained individuals when faced with supra-maximal exercise. These responses will have a direct impact on glucose metabolism.
Growth Hormone
Growth hormone responses to high intensity exercise can also be affected by the fitness levels of the participant. One study of lean and obese men found that age and physical fitness (and not adiposity) were the strongest predictors of growth hormone responses to a maximal exercise test (84). In spite of the lower response with increasing age, it has also been found that individuals with higher levels of physical fitness maintain higher growth hormone responses to maximal exercise (38, 44). In addition, trained men have demonstrated higher growth hormone responses to heavy resistance exercise, compared to untrained men (from 0.65 ± 0.47 to 5.32 ± 6.67 ng/ml in trained, from 0.50 ± 0.001 to 1.87 ± 3.47 ng/ml in untrained; p < 0.05) (85). Where moderate intensity exercise is concerned, one study observed similar growth hormone responses in trained and untrained men, when exercise was performed at the same relative intensity (38), but others have found a significantly higher response in trained men across all age groups (38, 40). There is a paucity of studies to elucidate if these trends hold true for women, or how these differences may impact glucoregulation during exercise.
Type 1 Diabetes: Effect of Sex on Exercise Responses
There is currently little exercise research examining differences between men and women with T1D. Many exercise studies involving individuals with T1D have only male participants, or very few female participants. Where studies include both men and women, they generally fail to recognize potential differences between the sexes that may affect the counter-regulatory response to exercise, and affect blood glucose homeostasis. Current studies that do look at sex-related differences are also limited to aerobic exercise, leaving a complete absence of information related to high intensity activities and resistance exercise. In addition, there is currently no information about how fluctuating levels of estrogen throughout the menstrual cycle could affect blood glucose responses to exercise in women with T1D. What we do know about sex-related differences in hormonal responses to exercise in T1D, however, tends to mirror the outcomes of previous studies in individuals without diabetes.
Fuel Selection
Very few studies have examined sex-related differences in fuel selection during exercise in individuals with T1D, and those that do involve small sample sizes (e.g., <10 individuals of each sex). One study that measured RER during 90 min of aerobic exercise (50% VO2max on a cycle ergometer) noted a tendency toward higher carbohydrate oxidation among men during the last 30 min of exercise, although differences were not statistically significant (86). As the study in question used constant dextrose and insulin infusion to maintain blood glucose, it was not possible to examine the impact of sex on changes in blood glucose during this type of activity. From what we know of studies in non-diabetic individuals, however, the greater reliance on glycogen stores in men during exercise compared to women poses a greater risk, in theory, for post-exercise hypoglycemia in men with T1D, as greater uptake of plasma glucose will be needed to replenish depleted glycogen stores during recovery.
Important Hormones Mediating Fuel Selection
While there is a current lack of information regarding sex-related differences in blood glucose responses to exercise in T1D, there is evidence that hormonal responses differ between the sexes. Similar to studies involving individuals without diabetes, Galassetti et al. (86) found that epinephrine (men = 938 ± 104; women = 628 ± 142 pmol/l; post-exercise; p < 0.05) and norepinephrine (men = 5.9 ± 0.8; women = 4.0 ± 0.7 nmol/l; post-exercise p < 0.05) responses to exercise were greater in men compared to women with T1D after 90 min of exercise on a cycle ergometer at 50% VO2max. The growth hormone response was also significantly lower in women (from 4 ± 2 to 14 ± 3 μg/l) compared to men (from 1 ± 0.4 to 24 ± 9μg/l; p < 0.05) (87). However, women still elicited a greater lipolytic response to exercise (glycerol increased from 46 ± 7 to 188 ± 40 μM compared to 31 ± 5 to 131 ± 10 μM in men; p < 0.05 area under the curve), with a suggestion of greater tissue sensitivity in women to growth hormone than men (86).
Where glucagon is concerned, sex-related differences seem to exist only where individuals have experienced hypoglycemia prior to exercise. The pancreatic β-cell death that characterizes T1D is accompanied by a progressive loss of α-cell function over time (87), resulting in an impaired glucagon response to falling blood glucose levels (88). The decline in function appears to happen evenly across the sexes, resulting in similar glucagon responses to exercise and to hypoglycemia at rest. Davis et al. (89) found that glucagon responses to hypoglycemia were similar among men and women with T1D (63 ± 18 and 58 ± 12 ng/l respectively). Similarly Galassetti et al. (86) found no sex-related differences in glucagon responses (51 ± 3 ng/l for men vs. 47 ± 7 ng/l for women) to 90 min of cycling at 50% VO2max in participants with T1D who were matched for age, glycemic control, duration of diabetes and exercise fitness.
With the presence of antecedent hypoglycemia, however, Galassetti et al. (90) discovered a greater blunting of counter-regulatory hormonal responses to exercise in men with T1D, compared to women matched for age, fitness, diabetes duration, and glycemic control. The exercise involved 90 min of euglycemic exercise (cycling at 50%VO2max) performed 24 h after a 2 h hypoglycemic period. While both groups had a noticeably blunted response, the differences in counter-regulatory hormones, particularly glucagon (11 ± 2 ng/l lower in men, but only 5 ± 2 ng/l lower in women, p < 0.05) led to a higher level of suppression of endogenous glucose production during exercise in the men (90). In the absence of a constant insulin and glucose infusion to maintain blood glucose concentration, this would most likely result in greater declines in blood glucose in males compared to females. Overall, more research in the area of sex and its effects on blood glucose responses to different types, timing, and intensity of exercise in T1D are required.
Type 1 Diabetes: Effect of Age on Exercise Responses
Within the context of T1D, aging is associated with increasing insulin dosage, as resistance to insulin increases with both age (mostly due to decreasing physical activity levels) (91) and disease duration (92). To date, it has yet to be examined whether older adults with T1D have different responses to exercise across various intensities compared to young adults. The differences in fuel selection and hormonal response seen between age groups in individuals without diabetes would indicate that age should be considered as an important factor. As improvements in diabetes care have led to a greater number of individuals with T1D living longer, healthier lives, determining the impact of age on blood glucose responses to different types and durations of exercise will become increasingly important.
Type 1 Diabetes: Effect of Physical Fitness on Exercise Responses
Where fuel selection is concerned, it would seem that improvements in physical fitness have a similar impact in individuals with T1D as they do in individuals without diabetes. One small study with T1D participants showed that improvements in physical fitness after 7 weeks of sprint training increased the participants' reliance on lipids as a fuel source (as measured by lower RER) during moderate intensity activity (93). This decreased reliance on carbohydrate as a fuel source during exercise, however, does not seem to protect against hypoglycemia: a recent study of 44 participants with T1D found that those who were more fit seemed to be more prone to hypoglycemia during moderate (60% VO2max) aerobic exercise (94).
In individuals with T1D, elevated epinephrine responses can lead to elevations of blood glucose during high intensity exercise that can persist for up to 2 h post-exercise (60). The extent to which fitness levels offer this glucose raising effect in individuals with T1D requires further examination, as current studies of high intensity exercise (either on its own or in the form of high intensity interval exercise) tend to have small sample sizes, of heterogeneous composition. It is also uncertain as to whether higher fitness levels will lead to a greater risk of nocturnal hypoglycemia in trained individuals vs. untrained individuals due to the need to replenish a greater amount of glycogen post-exercise.
Closed Loop/Artificial Pancreas Exercise Studies to Date
A small handful of studies have been completed to assess the performance of existing closed loop systems when faced with the challenge of controlling blood glucose during exercise (95–100). The task of managing blood glucose for different intensities of exercise in individuals with T1D is highlighted in a study by Jayawardene et al. (97) where a high intensity interval exercise protocol of similar energy expenditure to a moderate aerobic exercise protocol resulted in significantly different blood glucose levels 60 min post-exercise (high intensity intermittent exercise: 11.3 ± 0.5 mmol/L vs. aerobic exercise 8.9 ± mmol/L; p < 0.001) while using a hybrid closed loop system. This occurred in spite of standardization of exercise timing and food intake. One out of the 12 participants also experienced hypoglycemia (plasma glucose < 4.0 mmol/L for more than 15 min) after the moderate exercise session (97).
For hypoglycemia prevention during and after exercise, it has been suggested that closed loop systems should include both insulin and glucagon so that they can more closely replicate the hormonal changes that usually take place. Unfortunately, stable liquid glucagon formulations are currently lacking (101), making the widespread use of bi-hormonal devices impossible at this point. While dual hormone systems have generally been more successful at hypoglycemia prevention during exercise when compared to systems using insulin alone, they still cannot fully prevent hypoglycemia. Taleb et al. (96), for example, examined the performance of dual and single hormone systems in relation to a bout of aerobic exercise (60 min at 60% VO2max) and high intensity intermittent exercise (alternating between 50 and 85% VO2max every 2 min). While the dual hormone system resulted in a trend toward fewer participants experiencing hypoglycemia during the aerobic exercise trial (17.6 vs. 52.9%, p = 0.07), it still did not prevent it. Findings were similar for the high intensity intermittent protocol with respect to the number of participants experiencing hypoglycemia during exercise (6.25 for dual vs. 40% for single hormone, p = 0.07). Castle et al. (100) also had similar findings for a 45 min exercise session at 60% VO2max where a dual hormone system decreased time spent in hypoglycemia (3.4 ± 4.5% for dual hormone, 8.3 ± 12.6% for single hormone, p = 0.009). Hypoglycemia still occurred, albeit in lesser amounts. It is likely that there simply is not enough information available on exercise in T1D for these systems to predict exercise responses accurately.
Summary
Changes in blood glucose during the 24 h after physical activity/exercise (i.e., the recovery period) are likely to be slow enough that a closed loop system will be able to manage them appropriately. The variability in responses to exercise and the magnitude of blood glucose changes over short periods, however, may prove to be a challenging obstacle due to the diversity of responses in a very heterogeneous population. A highly fit, young, female, may have very different responses to various types and intensities of exercise than an older, sedentary male. In the context of how little is currently known about all of the possible variables that might affect blood glucose responses to exercise in individuals with T1D, there may be insufficient information to produce a model that fully predicts it. Additional studies in this area involving participants with a greater range of ages, sexes, and fitness levels are required in order to provide the necessary information to successfully model physical activity/exercise in the context of the artificial pancreas.
Author Contributions
All authors JY, NB and RB made equal contributions in the research, drafting and editing of this manuscript.
Funding
NB was supported by an Alberta Innovates Health Solutions Summer Studentship. This collaborative work was made possible by Swansea University Research Grant Enabler (SURGE) funding.
Conflict of Interest Statement
JY has received speaker's fees from Animas Canada and Dexcom Canada, financial and in kind research support from Medtronic Canada, and in-kind research support from Ascensia Canada, Lifescan Canada, Dexcom Canada, and Abbott Nutrition Canada (Glucerna). RB has received speaker fees and educational support from Sanofi, Boehringer-Ingelheim, Novo Nordisk, Beneo.
The remaining author declares that the research was conducted in the absence of any commercial or financial relationships that could be construed as a potential conflict of interest.
References
1. Bluestone JA, Herold K, Eisenbarth G. Genetics, pathogenesis and clinical interventions in type 1 diabetes. Nature (2010) 464:1293–300. doi: 10.1038/nature08933
2. Chimen M, Kennedy A, Nirantharamkumar K, Pang TT, Andrews R, Narendran P. What are the health benefits of physical activity in type 1 diabetes mellitus? A literature review. Diabetologia (2012) 55:542–51. doi: 10.1007/s00125-011-2403-2
3. Brazeau AS, Rabasa-Lhoret R, Strychar I, Mircescu H. Barriers to physical activity among patients with type 1 diabetes. Diabetes Care (2008) 31:2108–9. doi: 10.2337/dc08-0720
4. Riddell MC, Gallen IW, Smart CE, Taplin CE, Adlofsson P, Lumb AN, et al. Exercise management in type 1 diabetes: a consensus statement. Lancet Diabetes Endocrinol. (2017) 5:377–90. doi: 10.1016/S2213-8587(17)30014-1
5. Freckmann G, Schmid C, Baumstark A, Pleus S, Link M, Haug C. System accuracy evaluation of 43 blood glucose monitoring systems for self-monitoring of blood glucose according to DIN EN ISO 15197. J Diabetes Sci Technol. (2012) 6:1060–75. doi: 10.1177/193229681200600510
6. Laffel L. Improved accuracy of continuous glucose monitoring systems in pediatric patients with diabetes mellitus: results from two studies. Diabetes Technol Ther. (2016) 18:S223–33. doi: 10.1089/dia.2015.0380
7. Bailey TS. Clinical implications of accuracy measurements of continuous glucose sensors. Diabetes Technol Ther. (2017) 19:S51–4. doi: 10.1089/dia.2017.0050
8. Weisman A, Bai JW, Cardinez M, Kramer CK, Perkins BA. Effect of artificial pancreas systems on glycaemic control in patients with type 1 diabetes: a systematic review and meta-analysis of outpatient randomised controlled trials. Lancet Diabetes Endocrinol. (2017) 5:501–12. doi: 10.1016/S2213-8587(17)30167-5
9. Riddell MC, Zaharieva DP, Yavelberg L, Cinar A, Jamnik VK. Exercise and the development of the artificial pancreas: one of the more difficult series of hurdles. J Diabetes Sci Technol. (2015) 9:1217–26. doi: 10.1177/1932296815609370
10. Colberg SR, Laan R, Dassau E, Kerr D. Physical activity and type 1 diabetes: time for a rewire? J Diabetes Sci Technol. (2015) 9:609–18. doi: 10.1177/1932296814566231
11. Zaharieva D, Yavelberg L, Jamnik V, Cinar A, Turksoy K, Riddell MC. The effects of basal insulin suspension at the start of exercise on blood glucose levels during continuous versus circuit-based exercise in individuals with type 1 diabetes on continuous subcutaneous insulin infusion. Diabetes Technol Ther. (2017) 19:370–8. doi: 10.1089/dia.2017.0010
12. Colberg SR, Sigal RJ, Yardley JE, Riddell MC, Dunstan DW, Dempsey PC, et al. Physical activity/exercise and diabetes: a position statement of the American Diabetes Association. Diabetes Care (2016) 39:2065–79. doi: 10.2337/dc16-1728
13. Turksoy K, Monforti C, Park M, Griffith G, Quinn L, Cinar A. Use of wearable sensors and biometric variables in an artificial pancreas system. Sensors (Basel). (2017) 17:E532. doi: 10.3390/s17030532
14. Franc S, Daoudi A, Pochat A, Petit MH, Randazzo C, Petit C, et al. Insulin-based strategies to prevent hypoglycaemia during and after exercise in adult patients with type 1 diabetes on pump therapy: the DIABRASPORT randomized study. Diabetes Obes Metab. (2015) 17:1150–7. doi: 10.1111/dom.12552
15. Horton TJ, Pagliassotti MJ, Hobbs K, Hill JO. Fuel metabolism in men and women during and after long-duration exercise. J. Appl. Physiol (1985) (1998) 85:1823–32. doi: 10.1152/jappl.1998.85.5.1823
16. Davis SN, Galassetti P, Wasserman DH, Tate D. Effects of gender on neuroendocrine and metabolic counterregulatory responses to exercise in normal man. J Clin Endocrinol Metab. (2000) 85:224–30. doi: 10.1210/jcem.85.1.6328
17. Hedrington MS, Davis SN. Sexual dimorphism in glucose and lipid metabolism during fasting, hypoglycemia, and exercise. Front Endocrinol (Lausanne) (2015) 6:61. doi: 10.3389/fendo.2015.00061
18. Steffensen CH, Roepstorff C, Madsen M, Kiens B. Myocellular triacylglycerol breakdown in females but not in males during exercise. Am J Physiol Endocrinol Metab. (2002) 282:E634–42. doi: 10.1152/ajpendo.00078.2001
19. Justice TD, Hammer GL, Davey RJ, Paramalingam N, Guelfi KJ, Lewis L, et al. Effect of antecedent moderate-intensity exercise on the glycemia-increasing effect of a 30-sec maximal sprint: a sex comparison. Physiol. Rep. (2015) 3:e12386. doi: 10.14814/phy2.12386
20. Gratas-Delamarche A, Le Cam R, Delamarche P, Monnier M, Koubi H. Lactate and catecholamine responses in male and female sprinters during a Wingate test. Eur J Appl Physiol Occup Physiol. (1994) 68:362–6. doi: 10.1007/BF00571458
21. Amiel SA, Maran A, Powrie JK, Umpleby AM, Macdonald IA. Gender differences in counterregulation to hypoglycaemia. Diabetologia (1993) 36:460–4. doi: 10.1007/BF00402284
22. Pullinen T, Nicol C, MacDonald E, Komi PV. Plasma catecholamine responses to four resistance exercise tests in men and women. Eur J Appl Physiol Occup Physiol. (1999) 80:125–31. doi: 10.1007/s004210050568
23. Isacco L, Duche P, Boisseau N. Influence of hormonal status on substrate utilization at rest and during exercise in the female population. Sports Med. (2012) 42:27–42. doi: 10.2165/11598900-000000000-00000
24. Devries MC, Hamadeh MJ, Phillips SM, Tarnopolsky MA. Menstrual cycle phase and sex influence muscle glycogen utilization and glucose turnover during moderate-intensity endurance exercise. Am J Physiol Regul Integr Comp Physiol. (2006) 291:R1120–R1128. doi: 10.1152/ajpregu.00700.2005
25. Hamadeh MJ, Devries MC, Tarnopolsky MA. Estrogen supplementation reduces whole body leucine and carbohydrate oxidation and increases lipid oxidation in men during endurance exercise. J Clin Endocrinol Metab. (2005) 90:3592–9. doi: 10.1210/jc.2004-1743
26. Luk HY, Kraemer WJ, Szivak TK, Flanagan SD, Hooper DR, Kupchak BR, et al. Acute resistance exercise stimulates sex-specific dimeric immunoreactive growth hormone responses. Growth Horm IGF Res. (2015) 25:136–40. doi: 10.1016/j.ghir.2015.02.002
27. Esbjornsson M, Norman B, Suchdev S, Viru M, Lindghren A, Jansson E. Greater growth hormone and insulin response in women than in men during repeated bouts of sprint exercise. Acta Physiol (Oxf). (2009) 197:107–15. doi: 10.1111/j.1748-1716.2009.01994.x
28. Eliakim A, Nemet D, Most G, Rakover N, Pantanowitz M, Meckel Y. Effect of gender on the GH-IGF-I response to anaerobic exercise in young adults. J Strength Cond Res. (2014) 28:3411–5. doi: 10.1519/JSC.0000000000000605
29. Devries MC. Sex-based differences in endurance exercise muscle metabolism: impact on exercise and nutritional strategies to optimize health and performance in women. Exp Physiol. (2016) 101:243–9. doi: 10.1113/EP085369
30. Tarnopolsky MA. Sex differences in exercise metabolism and the role of 17-beta estradiol. Med Sci Sports Exerc. (2008) 40:648–54. doi: 10.1249/MSS.0b013e31816212ff
31. Riddell MC, Partington SL, Stupka N, Armstrong D, Rennie C, Tarnopolsky MA. Substrate utilization during exercise performed with and without glucose ingestion in female and male endurance trained athletes. Int J Sport Nutr Exerc Metab. (2003) 13:407–21. doi: 10.1123/ijsnem.13.4.407
32. Mittendorfer B, Horowitz JF, Klein S. Effect of gender on lipid kinetics during endurance exercise of moderate intensity in untrained subjects. Am J Physiol Endocrinol Metab. (2002) 283:E58–65. doi: 10.1152/ajpendo.00504.2001
33. Henderson GC, Fattor JA, Horning MA, Faghihnia N, Johnson ML, Mau TL, et al. Lipolysis and fatty acid metabolism in men and women during the postexercise recovery period. J Physiol. (2007) 584:963–81. doi: 10.1113/jphysiol.2007.137331
34. Fragala MS, Kraemer WJ, Denegar CR, Maresh CM, Mastro AM, Volek JS. Neuroendocrine-immune interactions and responses to exercise. Sports Med. (2011) 41:621–39. doi: 10.2165/11590430-000000000-00000
35. Carter SL, Rennie C, Tarnopolsky MA. Substrate utilization during endurance exercise in men and women after endurance training. Am J Physiol Endocrinol Metab. (2001) 280:E898–907. doi: 10.1152/ajpendo.2001.280.6.E898
36. Milanovic Z, Pantelic S, Trajkovic N, Sporis G, Kostic R, James N. Age-related decrease in physical activity and functional fitness among elderly men and women. Clin Interv Aging (2013) 8:549–56. doi: 10.2147/CIA.S44112
37. Manetta J, Brun JF, Prefaut C, Mercier J. Substrate oxidation during exercise at moderate and hard intensity in middle-aged and young athletes vs. sedentary men. Metabolism (2005) 54:1411–9. doi: 10.1016/j.metabol.2004.12.002
38. Silverman HG, Mazzeo RS. Hormonal responses to maximal and submaximal exercise in trained and untrained men of various ages. J Gerontol A Biol Sci Med Sci. (1996) 51:B30–B37.
39. Kraemer WJ, Hakkinen K, Newton RU, McCormick M, Nindl BC, Volek JS, et al. Acute hormonal responses to heavy resistance exercise in younger and older men. Eur J Appl Physiol Occup Physiol. (1998) 77:206–11.
40. Hagberg JM, Seals DR, Yerg JE, Gavin J, Gingerich R, Premachandra B, et al. Metabolic responses to exercise in young and older athletes and sedentary men. J. Appl. Physiol. (1985) (1988) 65:900–8. doi: 10.1152/jappl.1988.65.2.900
41. Kohrt WM, Spina RJ, Ehsani AA, Cryer PE, Holloszy JO. Effects of age, adiposity, and fitness level on plasma catecholamine responses to standing and exercise. J. Appl. Physiol. (1985). (1993) 75, 1828–35. doi: 10.1152/jappl.1993.75.4.1828
42. Zouhal H, Vincent S, Moussa E, Botcazou M, Delamarche P, Gratas-Delamarche A. Early advancing age alters plasma glucose and glucoregulatory hormones in response to supramaximal exercise. J Sci Med Sport. (2009) 12:652–6. doi: 10.1016/j.jsams.2008.03.003
43. Lonnqvist F, Nyberg B, Wahrenberg H, Arner P. Catecholamine-induced lipolysis in adipose tissue of the elderly. J Clin Invest. (1990) 85:1614–21. doi: 10.1172/JCI114612
44. Manetta J, Brun JF, Maimoun L, Callis A, Prefaut C, Mercier J. Effect of training on the GH/IGF-I axis during exercise in middle-aged men: relationship to glucose homeostasis. Am J Physiol Endocrinol Metab. (2002) 283:E929–E936. doi: 10.1152/ajpendo.00539.2001
45. Manetta J, Brun JF, Maimoun L, Galy O, Coste O, Maso F, et al. Carbohydrate dependence during hard-intensity exercise in trained cyclists in the competitive season: importance of training status. Int J Sports Med. (2002) 23:516–23. doi: 10.1055/s-2002-35074
46. Manetta J, Brun JF, Perez-Martin A, Callis A, Prefaut C, Mercier J. Fuel oxidation during exercise in middle-aged men: role of training and glucose disposal. Med Sci Sports Exerc. (2002) 34:423–9.
47. Bassami M, Ahmadizad S, Doran D, MacLaren DP. Effects of exercise intensity and duration on fat metabolism in trained and untrained older males. Eur J Appl Physiol. (2007) 101:525–32. doi: 10.1007/s00421-007-0523-7
48. Boon H, Jonkers RA, Koopman R, Blaak EE, Saris WH, Wagenmakers AJ, et al. Substrate source use in older, trained males after decades of endurance training. Med Sci Sports Exerc. (2007) 39:2160–70. doi: 10.1249/mss.0b013e3181572ace
49. Sial S, Coggan AR, Hickner RC, Klein S. Training-induced alterations in fat and carbohydrate metabolism during exercise in elderly subjects. Am J Physiol. (1998) 274:E785–E90. doi: 10.1152/ajpendo.1998.274.5.E785
50. Huth C, Pigeon E, Riou ME, St-Onge J, Arguin H, Couillard E, et al. Fitness, adiposopathy, and adiposity are independent predictors of insulin sensitivity in middle-aged men without diabetes. J Physiol Biochem. (2016) 72:435–44. doi: 10.1007/s13105-016-0488-2
51. Sarafian D, Schutz Y, Montani JP, Dulloo AG, Miles-Chan JL. Sex difference in substrate oxidation during low-intensity isometric exercise in young adults. Appl Physiol Nutr Metab. (2016) 41:977–84. doi: 10.1139/apnm-2016-0127
52. Tremblay J, Peronnet F, Massicotte D, Lavoie D. Carbohydrate supplementation and sex differences in fuel selection during exercise. Med Sci Sports Exerc. (2010) 42:1314–23. doi: 10.1249/MSS.0b013e3181cbba0b
53. Wiecek M, Maciejczyk M, Szymura J, Syzgula Z. Sex differences in oxidative stress after eccentric and concentric exercise. Redox Rep. (2017) 22:478–85. doi: 10.1080/13510002.2017.1304195
54. Roepstorff C, Steffensen CH, Madsen M, Stallknecht B, Kanstrup IL, Richter EA, et al. Gender differences in substrate utilization during submaximal exercise in endurance-trained subjects. Am. J. Physiol. Endocrinol. Metab. (2002) 282:E435–47. doi: 10.1152/ajpendo.00266.2001
55. Venables MC, Achten J, Jeukendrup AE. Determinants of fat oxidation during exercise in healthy men and women: a cross-sectional study. J. Appl. Physiol (1985) (2005) 98:160–7. doi: 10.1152/japplphysiol.00662.2003
56. Henderson GC, Fattor JA, Horning MA, Faghihnia N, Johnson ML, Luke-Zeitoun M, et al. Glucoregulation is more precise in women than in men during postexercise recovery. Am J Clin Nutr. (2008) 87:1686–94. doi: 10.1093/ajcn/87.6.1686
57. Vincent S, Berthon P, Zouhal H, Moussa E, Catheline M, Bentue-Ferrer D, et al. Plasma glucose, insulin and catecholamine responses to a Wingate test in physically active women and men. Eur J Appl Physiol. (2004) 91:15–21. doi: 10.1007/s00421-003-0957-5
58. Marliss EB, Kreisman SH, Manzon A, Halter JB, Vranic M, and Nessim SJ. Gender differences in glucoregulatory responses to intense exercise. J. Appl. Physiol (1985). (2000) 88:457–66. doi: 10.1152/jappl.2000.88.2.457
59. Pullinen T, Mero A, Huttunen P, Pakarinen A, Komi PV. Resistance exercise-induced hormonal responses in men, women, and pubescent boys. Med Sci Sports Exerc. (2002) 34:806–13. doi: 10.1097/00005768-200205000-00013
60. Mitchell TH, Abraham G, Schiffrin A, Leiter LA, Marliss EB. Hyperglycemia after intense exercise in IDDM subjects during continuous subcutaneous insulin infusion. Diabetes Care (1988) 11:311–7. doi: 10.2337/diacare.11.4.311
61. Bussau VA, Ferreira LD, Jones TW, Founier PA. The 10-s maximal sprint: a novel approach to counter an exercise-mediated fall in glycemia in individuals with type 1 diabetes. Diabetes Care (2006) 29:601–6. doi: 10.2337/diacare.29.03.06.dc05-1764
62. Bussau VA, Ferreira LD, Jones TW, Founier PA. A 10-s sprint performed prior to moderate-intensity exercise prevents early post-exercise fall in glycaemia in individuals with type 1 diabetes. Diabetologia (2007) 50:1815–8. doi: 10.1007/s00125-007-0727-8
63. Schmidt SL, Bessesen DH, Stotz S, Peelor FF, Miller BF, and Horton TJ. Adrenergic control of lipolysis in women compared with men. J. Appl. Physiol (1985) (2014) 117:1008–19. doi: 10.1152/japplphysiol.00003.2014
64. Devries MC, Hamadeh MJ, Graham TE, Tarnopolsky MA. 17beta-estradiol supplementation decreases glucose rate of appearance and disappearance with no effect on glycogen utilization during moderate intensity exercise in men. J Clin Endocrinol Metab. (2005) 90:6218–25. doi: 10.1210/jc.2005-0926
65. Consitt LA, Copeland JL, Tremblay MS. Endogenous anabolic hormone responses to endurance versus resistance exercise and training in women. Sports Med. (2002) 32:1–22. doi: 10.2165/00007256-200232010-00001
66. Copeland JL, Consitt LA, Tremblay MS. Hormonal responses to endurance and resistance exercise in females aged 19-69 years. J Gerontol A Biol Sci Med Sci. (2002) 57:B158–B165.
67. Vislocky LM, Gaine PC, Pikosky MA, Martin WF, Rodriguez NR. Gender impacts the post-exercise substrate and endocrine response in trained runners. J Int Soc Sports Nutr. (2008) 5:7. doi: 10.1186/1550-2783-5-7
68. Wideman L, Weltman JY, Shah N, Story S, Veldhuis JD, Weltman A. Effects of gender on exercise-induced growth hormone release. J. Appl. Physiol (1985) (1999) 87:1154–62. doi: 10.1152/jappl.1999.87.3.1154
69. Benini R, Prado Nunes PR, Orsatti CL, Barcelos LC, Orsatti FL. Effects of acute total body resistance exercise on hormonal and cytokines changes in men and women. J Sports Med Phys Fitness. (2015) 55:337–44.
70. Kraemer WJ, Staron RS, Hagerman FC, Hikida RS, Fry AC, Gordon SE, et al. The effects of short-term resistance training on endocrine function in men and women. Eur J Appl Physiol Occup Physiol. (1998) 78:69–76.
71. Linnamo V, Pakarinen A, Komi PV, Kraemer WJ, Hakkinen K. Acute hormonal responses to submaximal and maximal heavy resistance and explosive exercises in men and women. J Strength Cond Res. (2005) 19:566–71. doi: 10.1519/R-15404.1
72. Kraemer WJ, Fleck SJ, Dziados JE, Harman EA, Marchitelli LJ, Gordon SE, et al. Changes in hormonal concentrations after different heavy-resistance exercise protocols in women. J. Appl. Physiol (1985) (1993) 75:594–604. doi: 10.1152/jappl.1993.75.2.594
73. Kraemer WJ, Gordon SE, Fleck SJ, Marchitelli LJ, Mello R, Dziados JE, et al. Endogenous anabolic hormonal and growth factor responses to heavy resistance exercise in males and females. Int J Sports Med. (1991) 12:228–35. doi: 10.1055/s-2007-1024673
74. Jorgensen JO, Krag M, Kanaley J, Moller J, Hansen TK, Moller N, et al. Exercise, hormones, and body temperature. Regulation and action of GH during exercise J Endocrinol Invest. (2003) 26:838–42. doi: 10.1007/BF03345233
75. Fleg JL, Morrell CH, Bos AG, Brant LJ, Talbot LA, Wright JG, et al. Accelerated longitudinal decline of aerobic capacity in healthy older adults. Circulation (2005) 112:674–82. doi: 10.1161/CIRCULATIONAHA.105.545459
76. Doherty TJ. The influence of aging and sex on skeletal muscle mass and strength. Curr Opin Clin Nutr Metab Care (2001) 4:503–8. doi: 10.1097/00075197-200111000-00007
77. Poehlman ET, Toth MJ, Bunyard LB, Gardner AW, Donaldson KE, Colman E, et al. Physiological predictors of increasing total and central adiposity in aging men and women. Arch Intern Med. (1995) 155:2443–8. doi: 10.1001/archinte.1995.00430220101011
78. Bouchard DR, Beliaeff S, Dionne UK, Brochu M. Fat mass but not fat-free mass is related to physical capacity in well-functioning older individuals: nutrition as a determinant of successful aging (NuAge)–the Quebec Longitudinal Study. J Gerontol A Biol Sci Med Sci. (2007) 62:1382–8. doi: 10.1093/gerona/62.12.1382
79. Krems C, Luhrmann PM, Strassburg A, Hartmann B, Neuhauser-Berthold M. Lower resting metabolic rate in the elderly may not be entirely due to changes in body composition. Eur J Clin Nutr. (2005) 59:255–62. doi: 10.1038/sj.ejcn.1602066
80. Racette SB, Evans EM, Weiss EP, Hagberg JM, Holloszy JO. Abdominal adiposity is a stronger predictor of insulin resistance than fitness among 50-95 year olds. Diabetes Care (2006) 29:673–8. doi: 10.2337/diacare.29.03.06.dc05-1605
81. Gordon SE, Kraemer WJ, Looney DP, Flanagan SD, Comstock BA, Hymer WC. The influence of age and exercise modality on growth hormone bioactivity in women. Growth Horm IGF Res. (2014) 24:95–103. doi: 10.1016/j.ghir.2014.03.005
82. van Loon LJ, Jeukendrup AE, Saris WH, Wagenmakers AJ. Effect of training status on fuel selection during submaximal exercise with glucose ingestion. J. Appl. Physiol (1985) (1999) 87:1413–20. doi: 10.1152/jappl.1999.87.4.1413
83. Emhoff CA, Messonnier LA, Horning MA, Fattor JA, Carlson TJ, and Brooks GA. Gluconeogenesis and hepatic glycogenolysis during exercise at the lactate threshold. J. Appl. Physiol (1985) (2013) 114:297–306. doi: 10.1152/japplphysiol.01202.2012
84. Holt RI, Webb E, Pentecost C, Sonksen PH. Aging and physical fitness are more important than obesity in determining exercise-induced generation of GH. J Clin Endocrinol Metab. (2001) 86:5715–20. doi: 10.1210/jcem.86.12.8092
85. Hasani-Ranjbar S, Soleymani Far E, Heshmat R, Rajabi H, Kosari H. Time course responses of serum GH, insulin, IGF-1, IGFBP1, and IGFBP3 concentrations after heavy resistance exercise in trained and untrained men. Endocrine (2012) 41:144–51. doi: 10.1007/s12020-011-9537-3
86. Galassetti P, Tate D, Neill RA, Morrey S, Davis SN. Effect of gender on counterregulatory responses to euglycemic exercise in type 1 diabetes. J Clin Endocrinol Metab. (2002) 87:5144–50. doi: 10.1210/jc.2002-020757
87. Banarer S, McGregor VP, Cryer PE. Intraislet hyperinsulinemia prevents the glucagon response to hypoglycemia despite an intact autonomic response. Diabetes (2002) 51:958–65. doi: 10.2337/diabetes.51.4.958
88. Gerich JE, Langlois M, Noacco C, Karam JH, Forsham PH. Lack of glucagon response to hypoglycemia in diabetes: evidence for an intrinsic pancreatic alpha cell defect. Science (1973) 182:171–3. doi: 10.1126/science.182.4108.171
89. Davis SN, Fowler S, Costa F. Hypoglycemic counterregulatory responses differ between men and women with type 1 diabetes. Diabetes (2000) 49:65–72. doi: 10.2337/diabetes.49.1.65
90. Galassetti P, Tate D, Neill RA, Morrey S, Wasserman DH, Davis SN. Effect of sex on counterregulatory responses to exercise after antecedent hypoglycemia in type 1 diabetes. Am J Physiol Endocrinol Metab. (2004) 287:E16–24. doi: 10.1152/ajpendo.00480.2002
91. Amati F, Dube JJ, Coen PM, Stefanovic-Racic M, Toledo FG, Goodpaster BH. Physical inactivity and obesity underlie the insulin resistance of aging. Diabetes Care (2009) 32:1547–9. doi: 10.2337/dc09-0267
92. Bulum T, Duvnjak L. Insulin resistance in patients with type 1 diabetes: relationship with metabolic and inflammatory parameters. Acta Clin Croat. (2013) 52:43–51.
93. Harmer AR, Chisholm DJ, McKenna MJ, Hunter SK, Ruell PA, Naylor JM, et al. Sprint training increases muscle oxidative metabolism during high-intensity exercise in patients with type 1 diabetes. Diabetes Care (2008) 31:2097–102. doi: 10.2337/dc08-0329
94. Al Khalifah RA, Suppere C, Haidar A, Rabasa-Lhoret R, Ladouceur M, Legault L. Association of aerobic fitness level with exercise-induced hypoglycaemia in Type 1 diabetes. Diabet Med. (2016) 33:1686–90. doi: 10.1111/dme.13070
95. Van Bon AC, Jonker LD, Koebrugge R, Koops R, Hoekstra JB, DeVries JH. Feasibility of a bihormonal closed-loop system to control postexercise and postprandial glucose excursions. J Diabetes Sci Technol. (2012) 6:1114–22. doi: 10.1177/193229681200600516
96. Taleb N, Emami A, Suppere C, Messier V, Legault L, Ladouceur M, et al. Efficacy of single-hormone and dual-hormone artificial pancreas during continuous and interval exercise in adult patients with type 1 diabetes: randomised controlled crossover trial. Diabetologia (2016) 59:2561–71. doi: 10.1007/s00125-016-4107-0
97. Jayawardene DC, McAuley SA, Horsburgh JC, Gerche A, Jenkins AJ, Ward GM, et al. Closed-loop insulin delivery for adults with type 1 diabetes undertaking high-intensity interval exercise versus moderate-intensity exercise: A randomized, crossover study. Diabetes Technol Ther. (2017) 19:340–8. doi: 10.1089/dia.2016.0461
98. Abraham MB, Davey R, O'Grady MJ, Ly TT, Paramalingam N, Fournier PA, et al. Effectiveness of a predictive algorithm in the prevention of exercise-induced hypoglycemia in type 1 diabetes. Diabetes Technol Ther. (2016) 18:543–50. doi: 10.1089/dia.2016.0141
99. Jacobs PG, El Youssef J, Reddy R, Resalat N, Branigan D, Condon J, et al. Randomized trial of a dual-hormone artificial pancreas with dosing adjustment during exercise compared with no adjustment and sensor-augmented pump therapy. Diabetes Obes Metab. (2016) 18:1110–9. doi: 10.1111/dom.12707
100. Castle JR, El Youssef J, Wilson LM, Reddy R, Resalat N, Branigan D, et al. Randomized outpatient trial of single- and dual-hormone closed-loop systems that adapt to exercise using wearable sensors. Diabetes Care (2018) 41:1471–7. doi: 10.2337/dc18-0228
Keywords: type 1 diabetes, physical activity, closed loop, sex, age, fitness
Citation: Yardley JE, Brockman NK and Bracken RM (2018) Could Age, Sex and Physical Fitness Affect Blood Glucose Responses to Exercise in Type 1 Diabetes? Front. Endocrinol. 9:674. doi: 10.3389/fendo.2018.00674
Received: 16 July 2018; Accepted: 29 October 2018;
Published: 15 November 2018.
Edited by:
Timothy William Jones, University of Western Australia, AustraliaCopyright © 2018 Yardley, Brockman and Bracken. This is an open-access article distributed under the terms of the Creative Commons Attribution License (CC BY). The use, distribution or reproduction in other forums is permitted, provided the original author(s) and the copyright owner(s) are credited and that the original publication in this journal is cited, in accordance with accepted academic practice. No use, distribution or reproduction is permitted which does not comply with these terms.
*Correspondence: Jane E. Yardley, amFuZS55YXJkbGV5QHVhbGJlcnRhLmNh