- 1Department of Developmental and Cell Biology, University of California, Irvine, Irvine, CA, United States
- 2Department of Pharmaceutical Sciences, University of California, Irvine, Irvine, CA, United States
- 3Department of Biomedical Engineering, University of California, Irvine, Irvine, CA, United States
Obesity is a worldwide pandemic that also contributes to the increased incidence of other diseases such as type 2 diabetes. Increased obesity is generally ascribed to positive energy balance. However, recent findings suggest that exposure to endocrine-disrupting chemicals such as obesogens during critical windows of development, may play an important role in the current obesity trends. Several experimental approaches, from in vitro cell cultures to transgenerational in vivo studies, are used to better understand the mechanisms of action of obesogens, each of which contributes to answer different questions. In this review, we discuss current knowledge in the obesogen field and the existing tools developed in research laboratories using tributyltin as a model obesogen. By understanding the advantages and limitations of each of these tools, we will better focus and design experimental approaches that will help expanding the obesogen field with the objective of finding potential therapeutic targets in human populations.
In the last 40 years obesity rates have dramatically increased worldwide both in adults and in youth (1). A recent report on obesity trends in the U.S. estimated that 39.8% of adults are clinically obese (BMI > 30) (1). Importantly, obesity is a risk factor for other metabolic disorders such as type 2 diabetes whose prevalence has increased in parallel with obesity and which is predicted to affect 642 million people worldwide by 2040 (2, 3). The health costs associated with obesity were estimated at over $275 annually in the U.S alone (4). Although the major driving factor in obesity is usually considered to be a simple function of energy balance (calorie consumption higher than calorie expenditure) (5), recent reports showing the increasing trends of obesity in children under 1 year of age and in animal populations suggest that other factors may be playing important roles in obesity (6, 7). Understanding those factors, the windows of susceptibility and the mechanisms through which they to alter human metabolism and promote obesity will aid treating and preventing the increasing rates of obesity and related disorders worldwide.
Obesity is sexually dimorphic (8). Overall, females accumulate more fat than males, and it tends to be located subcutaneously, while in males, adipose tissue tends to accumulate in the visceral cavity. Subcutaneous adipose tissue is generally associated with healthier fat since it is involved in regulating thermogenesis while visceral adipose tissue is associated with increased risk of cardiovascular disease (9). Adipose tissue is now known not only for its ability to store lipids, but also for its contribution to metabolic homeostasis by secreting hormones (e.g., leptin and adiponectin) and other signaling molecules involved in the regulation of appetite and fat mobilization. This suggests that maintaining a healthy and functional adipose tissue improves the overall metabolic state of the individual (9). The sexually dimorphic content and distribution of fat is highly influenced by steroid hormones such as testosterone and estrogens (8). Therefore, alterations of the endocrine system may contribute to disturbances in the regulation of adipose tissue formation and maintenance.
Endocrine disrupting chemicals (EDCs) are exogenous chemicals that alter the natural function of hormones (10). Several human and animal studies have found associations between exposure to EDCs and increased adiposity (11–16). However, the effects of EDCs that may lead to obesity might not be linked exclusively to life style or environmental exposures during adulthood. Epidemiological studies have shown that the environment during in utero development may affect the prevalence of non-communicable diseases, including metabolic disorders, later in life (13–21). Notably, the nutritional state of the mother is strongly linked to body weight of the offspring at birth and later in life (22–26) suggesting that suboptimal environments during development may contribute to permanent alterations in the individual that will counteract any lifestyle actions to ameliorate weight gain, thereby increasing susceptibility to obesity (22, 27).
A large body of evidence shows that exposure to environmental pollutants during in utero development and early life may contribute to obesity later in life (11). Studies performed using both in vitro and in vivo models showed that a subset of endocrine disrupting chemicals, called obesogens, can have important effects on the development of adipose tissue. Obesogens are exogenous chemicals that inappropriately stimulate adipogenesis and fat storage, can disturb adipose tissue homeostasis and affect metabolic rates and/or the regulation of appetite and satiety (28). Our recent findings suggested that one consequence of these disturbances is the alteration of the metabolic setpoint of the individual, that is, the capability of the body maintain a particular weight regardless of the lifestyle changes taken to increase or reduce that weight (29). These results are supported by human studies showing an association between higher levels of perfluoroalkyl compounds in plasma and a more rapid weight regain after diet-induced weight loss plan (12). Moreover, individuals with the highest levels of perfluoroaklyl compounds has the lowest resting metabolic rate (12). Despite numerous studies demonstrating the existence and effects of obesogens, a longstanding debate about the mechanisms through which obesogens exert their obesogenic effects still persists. In this review, we discuss the current experimental approaches to study the mechanisms of function of obesogens from in vitro analysis to transgenerational in vivo studies.
In vitro Approaches
The prototypical obesogen tributyltin (TBT) is known to activate two nuclear receptors critical for adipogenesis. These are the peroxisome proliferator-activated receptor gamma (PPARγ)(30–33), which is considered to be the master regulator of adipogenesis (34), and its heterodimeric partner the retinoid X receptor (RXR)(30), which we recently showed to be an important regulator of adipocyte commitment (35). Adipogenic commitment is the process through which multipotent mesenchymal stem cells (MSC) lose their multipotency and become irreversibly destined to the adipocyte lineage, in part via the expression of PPARγ2. Activation of PPARγ2 elicits terminal differentiation into white adipose tissue (36). Other already characterized obesogens such as fludioxonil, quinoxyfen, dibutyltin or triphenyltin, also activate PPARγ and/or RXR (37, 38), suggesting that these nuclear receptors are common targets for EDCs. However, nuclear receptors other than PPARγ2 and RXR have been shown to play important roles in adipogenesis, including the glucocorticoid receptor (GR) (39), estrogen receptor (ER) (40) and androgen receptor (AR) (41) and obesogens such as tolylfluanid, bisphenol A (BPA) or dichlorodiphenyltrichloroethane (DDT) have been shown to act through them (42–44). The fact that estrogen and androgen receptors are also involved in regulation of adipogenesis and fat storage, may explain, at least in part, the sexually dimorphic distribution of the fat in individuals from different genders. Therefore, assessing the capability of a candidate obesogen to induce adipogenic commitment, its ability to promote final adipocyte differentiation, and the mechanisms through which these processes occur requires the use of an array of assays that tests the various potential paths used by obesogens to promote fat storage.
One frequently used tool to screen for new obesogens is the murine pre-adipocyte cell line 3T3-L1 (45). This immortalized cell line has some beneficial aspects that include the short length of the assay and the amenability of cell lines to high throughput screening approaches. However, one key limitation of 3T3-L1 cells is that, since they are already pre-adipocytes, they are not useful for examining mechanisms, including screening for chemicals that may be involved in adipocyte commitment. It was recently suggested that the commercial source of the cell line (e.g., ATCC or Zenbio), the type of plates used, as well as the number of passages the cells have experienced play a critical role in the process of cell differentiation (46). 3T3-L1 cells from different commercial sources showed different expression of nuclear receptors such as PPARγ, RXRβ, and ERβ, and variable capability to differentiate into adipocytes using well-established adipogenic positive controls such as rosiglitazone (PPARγ activator) or TBT (46). These findings highlight some of the limitations of using 3T3-L1 cells as an adipogenic model when the goal is to hypothesize about in vivo outcomes after exposure to obesogens. Screening of new obesogens using this model will depend critically on poorly controlled variables such as the source and number of passages of the cells, bovine sera, inconsistencies in coatings on tissue culture plates and other culture conditions which can confound reproducibility of the results.
In contrast, assays performed with uncommitted cells such as MSCs (47), allow analyses with a broader scope. We recently described for the first time the role of RXR in adipogenic commitment using MSCs as a cell model, revealing that this phase of adipogenesis can be targeted by obesogens (35). Others have used murine cell lines such as C3H/10T1/2 which resemble MSCs in some properties (but contain a karyotype of 80 chromosomes), or BMS2 to screen for new obesogens or to dive into adipogenic mechanisms through which obesogens may act. Although both cell types are accepted adipogenic models (48, 49), they are both immortalized cell lines that do not fully recapitulate the behavior of uncommitted precursors in vivo. Both are also murine cells which can somewhat limit the extrapolation of results to other species. Therefore, conclusions and inferences made from the use of these cells should account for such limitations. One advantage of using MSCs is that they can be isolated as primary cells from different individuals in a variety of species allowing a more extensive analysis. The use of human MSCs can also allow researchers to assess the variability that exists in human populations, which would contribute to a deeper understanding of the role obesogens may play in the current obesity pandemic.
In vivo Approaches
TBT has been shown to act as an obesogen in multiple organisms including mice (30–32, 50–52) rats (53), goldfish (54), and zebrafish (55) which supports the hypothesis that obesogens are largely not species specific. However, although there are several in vitro models available to study the molecular underpinnings of obesogen exposure, the mechanisms through which obesogens contribute to obesity in animal models are likely to be more complex than those described for cell cultures. Experiments performed in adult rodents exposed to TBT showed alterations of the hypothalamic-pituitary-gonadal axis (56) as well as non-alcoholic fatty liver (52) supporting the current knowledge that the regulation of metabolism requires coordination between different organs including fat tissue, liver, muscle, pancreas, or brain.
Another source of discussion in the obesogen field is related to the functionality of the adipocytes produced after exposure to obesogens (57). As mentioned above, the adipose tissue has an important role to play in maintaining an overall healthy metabolic state (58). Interestingly, white fat cells produced by TBT exposure lack some beneficial properties of normal white adipocytes since the former show reduced levels of the antidiabetic hormone adiponectin, reduced glucose uptake capability, impaired ability to develop into thermogenic beige/brite adipocytes and increased expression of pro-inflammatory and pro-fibrotic genes (57, 59, 60). These results suggest that TBT promotes the development of dysfunctional adipocytes (57, 59, 60). Understanding which obesogens produce adipocytes of normal function and which elicit the production of dysfunctional adipocytes may also contribute to a better understanding of the potential mechanistic differences between different obesogens.
Transgenerational Approaches
It has been shown that some obesogens including TBT, DDT, and BPA, are able to induce heritable changes that are propagated through multiple generations without any further exposure (29, 61–64). The non-Mendelian transmission of these alterations suggests that epigenetic mechanisms are involved in this process. This raises yet another challenge for the obesogen field: what are the mechanisms underlying transgenerational inheritance?
The field of environmental epigenetics studies the epigenetic alterations introduced by environmental factors that contribute to phenotypic variation and/or disease (65). Studies performed in animal models showed that exposure to obesogens during development increased predisposition to obesity not only in the directly exposed F1 and F2 generations (F2 are exposed as germ cells inside the F1 fetus) but also in subsequent F3 and F4 generations (and beyond) which are not exposed (29, 61–64, 66, 67). These studies showed alterations of epigenetic factors such as DNA methylation and post-translational histone modifications in somatic tissues and/or germ cells (29, 61–64, 66–68). However, there are key knowledge gaps in this transgenerational puzzle, including the characterization of the molecular mechanisms that occur in the directly exposed stages that trigger the transgenerational phenotype, and the molecular mechanisms by which these alterations are transmitted through multiple generations.
There is extensive epigenetic reprogramming at different stages of mammalian development. Shortly after fertilization, the first erasure of epigenetic marks (DNA methylation) occurs from the zygote to the blastocyst during maternal-to-zygotic transition in a sex-specific manner (69). Between E 6.5 and E 13.5, after somatic and germ lines separate, the primordial germ cells (PGCs) undergo another round of DNA methylation erasure while they travel to the genital ridge where they will mature into sex-specific germ cells (70). Considering that from the moment of fertilization until birth there is an extensive epigenetic reprogramming of various epigenetic marks, the presence of agents that may disturb these processes, such as obesogens and other EDCs, at any of the stages may contribute to alterations in the newly determined epigenetic landscape. Since this reprogramming occurs in every generation, it is critical to determine which alterations are introduced by environmental factors and how many of these epigenomic changes can be propagated to subsequent generations in order to better understand mechanisms of transgenerational inheritance. This is currently a very controversial topic in the field (63, 65, 68, 71–73).
We recently proposed a new mechanism for the transmission of epigenetic information. In our transgenerational paradigm, we exposed pregnant F0 female mice to environmentally relevant doses of the obesogen TBT throughout pregnancy (61) or throughout pregnancy and lactation (29). Following either approach, we consistently found increased adiposity in F3 and F4 males, but not females (29, 61). Interestingly, the differences in fat storage between controls and ancestrally-TBT treated animals was exacerbated when the diet of the animals was switched from a standard diet to a diet with higher fat content (29). This supports the hypothesis that ancestral exposure to TBT alters the metabolic set point of these animals multiple generations after exposure has ceased (29). Integrative methylome and transcriptome analyses of fat tissue isolated from obese F4 males did not reveal any direct associations between changes in mRNA expression levels of genes whose promoter was either hypermethylated or hypomethylated. In contrast, we found a significant number of differentially methylated regions (DMR) located in intergenic regions. We re-analyzed the data, focusing on large blocks of the genome where the alteration in DNA methylation was in the same direction (hypo- or hyper-methylated) and denoted these as isodirectional, differentially methylated blocks (isoDMBs). IsoDMBs spanned large regions of the genome and we found a strong association between hypomethylated isoDMBs and over-expressed genes and between hypermethylated isoDMBs and under-expressed genes (29). Additionally, analysis of chromatin accessibility in sperm samples from F3 and F4 mice showed significant overlaps in accessibility between sperm samples in both generations (Figure 1A), suggesting that there is a conservation of the differentially accessible regions across generations. We also found an intriguing association between chromatin accessibility in sperm and isoDMBs in white adipose tissue of F4 male mice (Figure 1B). Regions that were inaccessible in sperm were hypomethylated in fat and regions that were accessible in sperm were hypermethylated in fat. We inferred that altered chromatin accessibility in the germ cells may promote or permit differential DNA methylation, epigenetic marks and gene expression in somatic tissues of the next generation (Figure 1C) (29).
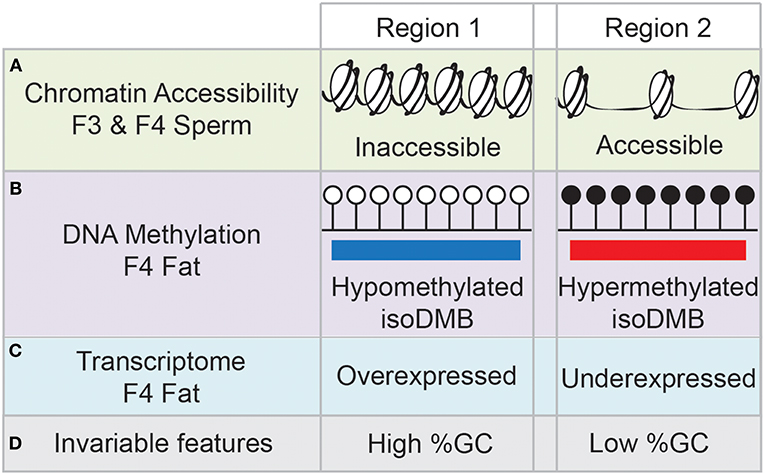
Figure 1. Schematic summary of the results from (29). Region 1 represents that genomic areas inaccessible in sperm samples of F3 and F4 mice (A) are hypomethylated in fat tissue of F4 males (B), and the genes contained in those regions tend to be overexpressed (C). Opposite trends are found in genomic areas represented by Region 2, with high accessibility in F3 and F4 sperm samples, and hypermethylation and underexpression in fat tissue. Genomic areas depicted by Region 1 have content of GCs, whereas genomic areas depicted by Region 2 have low GC content (D).
We also noted that hypomethylated isoDMBs tend to be located in areas of the genome with high GC content, whereas hypermethylated isoDMBs are located in areas of the genome with low GC content (Figure 1D). In other words, rather than being located randomly distributed throughout the genome, changes in methylation were enriched in regions with specific base composition (GC content) (29). Others have shown that there is an association between base composition and higher order chromatin organization in the nucleus (74). This led us to hypothesize that in utero exposure to TBT causes heritable alterations in chromatin architecture that will contribute to changes in the epigenetic landscape that are reflected in the transcriptome (29). This new model provides a potential molecular mechanism that embraces previously described results showing alterations of epigenetic marks, such as DNA methylation, histone modification and expression of small non-coding RNAs in germ cells and somatic cells after ancestral exposure to EDCs such obesogens (62–64, 66, 67).
Windows of Susceptibility
There are different windows of susceptibility to obesity after obesogen exposure. Rodents exposed to obesogens such as TBT or nonylphenol during early adulthood showed increased ectopic lipid storage in liver elevated body weight, and altered levels of metabolic hormones (52, 75). BPA or tolylfluanid exposure led to alterations in glucose metabolism and adiposity in adult mice (76, 77). Some of the metabolic alterations are only observed when the animals are exposed to high fat diets or high fat/high sugar diets (75, 77), suggesting that obesogen exposure may be increasing susceptibility to obesity in the presence of other metabolic challenges. This has important implications for the human obesity pandemic.
Exposures during in utero development have the potential to affect critical developmental steps that may have dramatic effects not only in the offspring, but in subsequent generations. Two different approaches have been used to study the effects of obesogens within early developmental windows: exposure of females throughout pregnancy (from fertilization to birth) (29, 37, 61) and exposure at discrete embryonic stages (E8–E14) (62–64, 67). The objective of the latter is to expose the embryos only during the time the primordial germ cells (PGCs) are traveling to the genital ridge, and during which DNA methylation is largely erased (70). However, the benefit of studying the former approach is that it allows the exposure to obesogens throughout the different phases of embryonic development, all of which may be important for obesity in later generations.
Another important factor that must be considered when assessing the effect of environmental pollutants using animal models is the concentration the animals are receiving. In order to be able to extrapolate the information obtained from animal studies to humans, it is necessary to work with concentrations of EDCs that are in the realm of what human exposure is measured or estimated to be. Typically, the chemical doses at which the endocrine system is altered are significantly lower than the concentrations that induce toxic effects in the body (44).
Summary and Future Directions
Since the obesogen hypothesis was first proposed, studies in many laboratories have supported the existence and effects of obesogens (78). The list of bona fide obesogens (those shown to influence obesity, in vivo) continues to grow and mechanisms through which these chemicals act to promote obesity are emerging. Experimental approaches to study these mechanisms are improving. In vitro studies continue to provide a strong foundation to evaluate the effects of obesogens in cells, particularly as more investigators adopt stem cell models, over the limited studies possible in 3T3-L1 preadipocytes. These cell culture-based studies offer the possibility to increase the numbers of chemicals screened and may reveal new mechanisms or hypotheses about the effects of these chemicals using in vivo animal models. Current technology based on deep sequencing in bulk or in single cells will allow extensive whole-genome analyses that can provide information critical to understanding the epigenomic and transcriptomal state of the cells in different tissues and life stages. A major challenge in this area will be to integrate and interpret these large, multi-omic data sets to provide the most useful information.
Another major obstacle in understanding the effects of EDCs and obesogens in humans is the paucity of exposure data, particularly data from longitudinal prospective cohort studies. In addition, humans are exposed to mixtures of EDCs throughout the life course. Fortunately, experimental researchers and epidemiologists are teaming up and the advent of “exposome” studies that assess and analyze levels of exposure to obesogens together with a multitude of other chemicals, including EDCs, will enable strong mechanistic links to be made between chemical exposure and human disease (79). This will inform and guide future laboratory and epidemiological approaches that will overcome the current limitations. In turn, this will allow us to assess the costs to society of EDC and obesogen exposure more accurately. In an optimistic view, this information might influence policy makers to take appropriate steps to protect the public health.
Author Contributions
RC-G and BB participated in the discussion of and wrote the ideas reflected in this review.
Conflict of Interest Statement
The authors declare that the research was conducted in the absence of any commercial or financial relationships that could be construed as a potential conflict of interest.
Acknowledgments
This work was supported by awards from the National Institutes of Health (ES023316, ES021832) to BB. BB is a named inventor on patents related to nuclear receptors some of which have been licensed to for profit entities.
References
1. Hales C, Carroll M, Fryar CD, Ogden CL. Prevalence of Obesity Among Adults and Youth: United States, 2015–2016, NCHS Data Brief., Hyattsville, MD: U.S. Department of Health & Human Services (2017).
2. Menke A, Casagrande S, Geiss L, Cowie CC. Prevalence of and trends in diabetes among adults in the United States, 1988-2012. JAMA. (2015) 314:1021–9. doi: 10.1001/jama.2015.10029
3. Kahn SE, Hull RL, Utzschneider KM. Mechanisms linking obesity to insulin resistance and type 2 diabetes. Nature. (2006) 444:840–6. doi: 10.1038/nature05482
4. Biener A, Cawley J, Meyerhoefer C. The high and rising costs of obesity to the US health care system. J Gen Intern Med. (2017) 32:6–8. doi: 10.1007/s11606-016-3968-8
5. Hall KD, Heymsfield SB, Kemnitz JW, Klein S, Schoeller DA, Speakman JR. Energy balance and its components: implications for body weight regulation. Am J Clin Nutr. (2012) 95:989–94. doi: 10.3945/ajcn.112.036350
6. Ng M, Fleming T, Robinson M, Thomson B, Graetz N, Margono C, et al., Global, regional, and national prevalence of overweight and obesity in children and adults during 1980-2013: a systematic analysis for the Global Burden of Disease Study 2013. Lancet. (2014) 384:766–81. doi: 10.1016/S0140-6736(14)60460-8
7. Klimentidis YC, Beasley TM, Lin HY, Murati G, Glass GE, Guyton M, et al. Canaries in the coal mine: a cross-species analysis of the plurality of obesity epidemics. Proc Biol Sci. (2011) 278:1626–32. doi: 10.1098/rspb.2010.1890
8. Palmer BF, Clegg DJ. The sexual dimorphism of obesity. Mol Cell Endocrinol. (2015) 402:113–9. doi: 10.1016/j.mce.2014.11.029
9. Trayhurn P. Endocrine and signalling role of adipose tissue: new perspectives on fat. Acta Physiol Scand. (2005) 184:285–93. doi: 10.1111/j.1365-201X.2005.01468.x
10. Zoeller RT, Brown TR, Doan LL, Gore AC, Skakkebaek NE, Soto AM, et al. Endocrine-disrupting chemicals and public health protection: a statement of principles from the endocrine society. Endocrinology. (2012) 153:4097–110. doi: 10.1210/en.2012-1422
11. Darbre PD. Endocrine disruptors and obesity. Curr Obes Rep. (2017) 6:18–27. doi: 10.1007/s13679-017-0240-4
12. Liu G, Dhana K, Furtado JD, Rood J, Zong G, Liang L, et al. Perfluoroalkyl substances and changes in body weight and resting metabolic rate in response to weight-loss diets: a prospective study. PLoS Med. (2018) 15:e1002502. doi: 10.1371/journal.pmed.1002502
13. Mendez MA, Garcia-Esteban R, Guxens M, Vrijheid M, Kogevinas M, Goni F, et al. Prenatal organochlorine compound exposure, rapid weight gain, and overweight in infancy. Environ Health Perspect. (2011) 119:272–8. doi: 10.1289/ehp.1002169
14. Valvi D, Mendez MA, Garcia-Esteban R, Ballester F, Ibarluzea J, Goni F, et al. Prenatal exposure to persistent organic pollutants and rapid weight gain and overweight in infancy. Obesity (Silver Spring). (2014) 22:488–96. doi: 10.1002/oby.20603
15. Valvi D, Mendez MA, Martinez D, Grimalt JO, Torrent M, Sunyer J, et al. Prenatal concentrations of polychlorinated biphenyls, DDE, and DDT and overweight in children: a prospective birth cohort study. Environ Health Perspect. (2012) 120:451–7. doi: 10.1289/ehp.1103862
16. Erkin-Cakmak A, Harley KG, Chevrier J, Bradman A, Kogut K, Huen K, et al. In utero and childhood polybrominated diphenyl ether exposures and body mass at age 7 years: the CHAMACOS study. Environ Health Perspect. (2015) 123:636–42. doi: 10.1289/ehp.1408417
17. Hanson MA, Gluckman PD. Early developmental conditioning of later health and disease: physiology or pathophysiology? Physiol Rev. (2014) 94:1027–76. doi: 10.1152/physrev.00029.2013
18. Stein Z. Famine and Human Development : The Dutch Hunger Winter of 1944-1945, New York, NY: Oxford University Press (1975).
19. Barker DJ, Bull AR, Osmond C, Simmonds SJ. Fetal and placental size and risk of hypertension in adult life. BMJ. (1990) 301:259–62. doi: 10.1136/bmj.301.6746.259
20. Barker DJ, Winter PD, Osmond C, Margetts B, Simmonds SJ. Weight in infancy and death from ischaemic heart disease. Lancet. (1989) 2:577–80. doi: 10.1016/S0140-6736(89)90710-1
21. Hales CN, Barker DJ, Clark PM, Cox LJ, Fall C, Osmond C, et al. Fetal and infant growth and impaired glucose tolerance at age 64. BMJ. (1991) 303:1019–22. doi: 10.1136/bmj.303.6809.1019
22. Gluckman PD, Hanson M, Zimmet P, Forrester T. Losing the war against obesity: the need for a developmental perspective. Sci Transl Med. (2011) 3:93cm19. doi: 10.1126/scitranslmed.3002554
23. Godfrey KM, Reynolds RM, Prescott SL, Nyirenda M, Jaddoe VW, Eriksson JG, et al. of maternal obesity on the long-term health of offspring. Lancet Diabetes Endocrinol. (2017) 5:53–64. doi: 10.1016/S2213-8587(16)30107-3
24. Forsen T, Eriksson JG, Tuomilehto J, Teramo K, Osmond C, Barker DJ. Mother's weight in pregnancy and coronary heart disease in a cohort of Finnish men: follow up study. BMJ. (1997) 315:837–40. doi: 10.1136/bmj.315.7112.837
25. Lawlor DA, Relton C, Sattar N, Nelson SM. Maternal adiposity–a determinant of perinatal and offspring outcomes? Nat Rev Endocrinol. (2012) 8:679–88. doi: 10.1038/nrendo.2012.176
26. Desai M, Hales CN. Role of fetal and infant growth in programming metabolism in later life. Biol Rev Camb Philos Soc. (1997) 72:329–48. doi: 10.1017/S0006323196005026
27. Lin XY, Lim IY, Wu YH, Teh AL, Chen L, Aris IM, et al. Developmental pathways to adiposity begin before birth and are influenced by genotype, prenatal environment and epigenome. BMC Med. (2017) 15:50. doi: 10.1186/s12916-017-0800-1
28. Janesick AS, Blumberg B. Obesogens: an emerging threat to public health. Am J Obstet Gynecol. (2016) 214:559–65. doi: 10.1016/j.ajog.2016.01.182
29. Chamorro-Garcia R, Diaz-Castillo C, Shoucri BM, Kach H, Leavitt R, Shioda T, et al. Ancestral perinatal obesogen exposure results in a transgenerational thrifty phenotype in mice. Nat Commun. (2017) 8:2012. doi: 10.1038/s41467-017-01944-z
30. Grun F, Watanabe H, Zamanian Z, Maeda L, Arima K, Cubacha R, et al. Endocrine-disrupting organotin compounds are potent inducers of adipogenesis in vertebrates. Mol Endocrinol. (2006) 20:2141–55. doi: 10.1210/me.2005-0367
31. Kirchner S, Kieu T, Chow C, Casey S, Blumberg B. Prenatal exposure to the environmental obesogen tributyltin predisposes multipotent stem cells to become adipocytes. Mol Endocrinol. (2010) 24:526–39. doi: 10.1210/me.2009-0261
32. Li X, Ycaza J, Blumberg B. The environmental obesogen tributyltin chloride acts via peroxisome proliferator activated receptor gamma to induce adipogenesis in murine 3T3-L1 preadipocytes. J Steroid Biochem Mol Biol. (2011) 127:9–15. doi: 10.1016/j.jsbmb.2011.03.012
33. Kanayama T, Kobayashi N, Mamiya S, Nakanishi T, Nishikawa J. Organotin compounds promote adipocyte differentiation as agonists of the peroxisome proliferator-activated receptor gamma/retinoid X receptor pathway. Mol Pharmacol. (2005) 67:766–74. doi: 10.1124/mol.104.008409
34. Tontonoz P, Spiegelman BM. Fat and beyond: the diverse biology of PPARgamma. Annu Rev Biochem. (2008) 77:289–312. doi: 10.1146/annurev.biochem.77.061307.091829
35. Shoucri BM, Martinez ES, Abreo TJ, Hung VT, Moosova Z, Shioda T, et al. Retinoid X receptor activation alters the chromatin landscape to commit mesenchymal stem cells to the adipose lineage. Endocrinology. (2017) 158:3109–25. doi: 10.1210/en.2017-00348
36. Cristancho AG, Lazar MA. Forming functional fat: a growing understanding of adipocyte differentiation. Nat Rev Mol Cell Biol. (2011) 12:722–34. doi: 10.1038/nrm3198
37. Chamorro-Garcia R, Shoucri BM, Willner S, Kach H, Janesick A, Blumberg B. Effects of perinatal exposure to dibutyltin chloride on fat and glucose metabolism in mice, and molecular mechanisms, in vitro. Environ Health Perspect. (2018) 126:057006. doi: 10.1289/EHP3030
38. Janesick AS, Dimastrogiovanni G, Vanek L, Boulos C, Chamorro-Garcia R, Tang W, et al. On the utility of toxcast and toxpi as methods for identifying new obesogens. Environ Health Perspect. (2016) 124:1214–26. doi: 10.1289/ehp.1510352
39. Steger DJ, Grant GR, Schupp M, Tomaru T, Lefterova MI, Schug J, et al. Propagation of adipogenic signals through an epigenomic transition state. Genes Dev. (2010) 24:1035–44. doi: 10.1101/gad.1907110
40. Cooke PS, Naaz A. Role of estrogens in adipocyte development and function. Exp Biol Med (Maywood). (2004) 229:1127–35. doi: 10.1177/153537020422901107
41. Dieudonne MN, Pecquery R, Boumediene A, Leneveu MC, Giudicelli Y. Androgen receptors in human preadipocytes and adipocytes: regional specificities and regulation by sex steroids. Am J Physiol. (1998) 274:C1645–52. doi: 10.1152/ajpcell.1998.274.6.C1645
42. Sargis RM, Johnson DN, Choudhury RA, Brady MJ. Environmental endocrine disruptors promote adipogenesis in the 3T3-L1 cell line through glucocorticoid receptor activation. Obesity (Silver Spring). (2010) 18:1283–8. doi: 10.1038/oby.2009.419
43. Newbold RR, Padilla-Banks E, Snyder RJ, Jefferson WN. Perinatal exposure to environmental estrogens and the development of obesity. Mol Nutr Food Res. (2007) 51:912–7. doi: 10.1002/mnfr.200600259
44. Vandenberg LN, Colborn T, Hayes TB, Heindel JJ, Shioda T, Soto AM, et al. Hormones and endocrine-disrupting chemicals: low-dose effects and nonmonotonic dose responses. Endocr Rev. (2012) 33:378–455. doi: 10.1210/er.2011-1050
45. Ruiz-Ojeda FJ, Ruperez AI, Gomez-Llorente C, Gil A, Aguilera CM. Cell models and their application for studying adipogenic differentiation in relation to obesity: a review. Int J Mol Sci. (2016) 17:e1040. doi: 10.3390/ijms17071040
46. Kassotis CD, Masse L, Kim S, Schlezinger JJ, Webster TF, Stapleton HM Characterization of adipogenic chemicals in three different cell culture systems: implications for reproducibility based on cell source and handling. Sci Rep. (2017) 7:42104. doi: 10.1038/srep42104
47. Singer NG, Caplan AI. Mesenchymal stem cells: mechanisms of inflammation. Annu Rev Pathol. (2011) 6:457–78. doi: 10.1146/annurev-pathol-011110-130230
48. Tang QQ, Otto TC, Lane MD. Commitment of C3H10T1/2 pluripotent stem cells to the adipocyte lineage. Proc Natl Acad Sci USA. (2004) 101:9607–11. doi: 10.1073/pnas.0403100101
49. Kelly KA, Tanaka S, Baron R, Gimble JM. Murine bone marrow stromally derived BMS2 adipocytes support differentiation and function of osteoclast-like cells in vitro. Endocrinology. (1998) 139:2092–101. doi: 10.1210/endo.139.4.5915
50. Bo E, Viglietti-Panzica C, Panzica GC. Acute exposure to tributyltin induces c-fos activation in the hypothalamic arcuate nucleus of adult male mice. Neurotoxicology. (2011) 32:277–80. doi: 10.1016/j.neuro.2010.12.011
51. Penza M, Jeremic M, Marrazzo E, Maggi A, Ciana P, Rando G, et al. The environmental chemical tributyltin chloride (TBT) shows both estrogenic and adipogenic activities in mice which might depend on the exposure dose. Toxicol Appl Pharmacol. (2011) 255:65–75. doi: 10.1016/j.taap.2011.05.017
52. Zuo Z, Chen S, Wu T, Zhang J, Su Y, Chen Y, et al. Tributyltin causes obesity and hepatic steatosis in male mice. Environ Toxicol. (2011) 26:79–85. doi: 10.1002/tox.20531
53. He K, Zhang J, Chen Z. Effect of tributyltin on the food intake and brain neuropeptide expression in rats. Endokrynol Pol. (2014) 65:485–90. doi: 10.5603/EP.2014.0068
54. Zhang J, Sun P, Yang F, Kong T, Zhang R. Tributyltin disrupts feeding and energy metabolism in the goldfish (Carassius auratus). Chemosphere. (2016) 152:221–8. doi: 10.1016/j.chemosphere.2016.02.127
55. Tingaud-Sequeira A, Ouadah N, Babin PJ. Zebrafish obesogenic test: a tool for screening molecules that target adiposity. J Lipid Res. (2011) 52:1765–72. doi: 10.1194/jlr.D017012
56. Sena GC, Freitas-Lima LC, Merlo E, Podratz PL, de Araujo JF, Brandao PA, et al. Environmental obesogen tributyltin chloride leads to abnormal hypothalamic-pituitary-gonadal axis function by disruption in kisspeptin/leptin signaling in female rats. Toxicol Appl Pharmacol. (2017) 319:22–38. doi: 10.1016/j.taap.2017.01.021
57. Regnier SM, El-Hashani E, Kamau W, Zhang X, Massad NL, Sargis RM. Tributyltin differentially promotes development of a phenotypically distinct adipocyte. Obesity (Silver Spring). (2015) 23:1864–71. doi: 10.1002/oby.21174
58. Asarian L, Geary N. Sex differences in the physiology of eating. Am J Physiol Regul Integr Comp Physiol. (2013) 305:R1215–67. doi: 10.1152/ajpregu.00446.2012
59. Shoucri BM, Hung VT, Chamorro-Garcia R, Shioda T, Blumberg B. Retinoid X receptor activation during adipogenesis of female mesenchymal stem cells programs a dysfunctional adipocyte. Endocrinology. (2018) 159:2863–83. doi: 10.1210/en.2018-00056
60. Kim S, Li A, Monti S, Schlezinger JJ. Tributyltin induces a transcriptional response without a brite adipocyte signature in adipocyte models. Arch Toxicol. (2018) 92:2859–74. doi: 10.1007/s00204-018-2268-y
61. Chamorro-Garcia R, Sahu M, Abbey RJ, Laude J, Pham N, Blumberg B. Transgenerational inheritance of increased fat depot size, stem cell reprogramming, and hepatic steatosis elicited by prenatal exposure to the obesogen tributyltin in mice. Environ Health Perspect. (2013) 121:359–66. doi: 10.1289/ehp.1205701
62. Manikkam M, Tracey R, Guerrero-Bosagna C, Skinner MK Plastics derived endocrine disruptors (BPA DEHP and DBP) induce epigenetic transgenerational inheritance of obesity reproductive disease and sperm epimutations. PLoS ONE. (2013) 8:e55387. doi: 10.1371/journal.pone.0055387
63. Skinner MK, Manikkam M, Tracey R, Guerrero-Bosagna C, Haque M, Nilsson EE. Ancestral dichlorodiphenyltrichloroethane (DDT) exposure promotes epigenetic transgenerational inheritance of obesity. Bmc Med. (2013) 11:228. doi: 10.1186/1741-7015-11-228
64. Tracey R, Manikkam M, Guerrero-Bosagna C, Skinner MK. Hydrocarbons (jet fuel JP-8) induce epigenetic transgenerational inheritance of obesity, reproductive disease and sperm epimutations. Reprod Toxicol. (2013) 36:104–16. doi: 10.1016/j.reprotox.2012.11.011
65. Skinner MK, Environmental epigenetics and a unified theory of the molecular aspects of evolution: a neo-lamarckian concept that facilitates neo-darwinian evolution. Genome Biol Evol. (2015) 7:1296–302. doi: 10.1093/gbe/evv073
66. Ben Maamar M, Sadler-Riggleman I, Beck D, McBirney M, Nilsson E, Klukovich R, et al. Alterations in sperm DNA methylation, non-coding RNA expression, and histone retention mediate vinclozolin-induced epigenetic transgenerational inheritance of disease. Environ Epigenet. (2018) 4:dvy010. doi: 10.1093/eep/dvy010
67. Skinner MK, Ben Maamar M, Sadler-Riggleman I, Beck D, Nilsson E, McBirney M, et al. Alterations in sperm DNA methylation, non-coding RNA and histone retention associate with DDT-induced epigenetic transgenerational inheritance of disease. Epigenetics Chromatin. (2018) 11:8. doi: 10.1186/s13072-018-0178-0
68. Haque MM, Nilsson EE, Holder LB, Skinner MK. Genomic clustering of differential DNA methylated regions (epimutations) associated with the epigenetic transgenerational inheritance of disease and phenotypic variation. BMC Genomics. (2016) 17:418. doi: 10.1186/s12864-016-2748-5
69. Eckersley-Maslin MA, Alda-Catalinas C, Reik W. Dynamics of the epigenetic landscape during the maternal-to-zygotic transition. Nat Rev Mol Cell Biol. (2018) 19:436–50. doi: 10.1038/s41580-018-0008-z
70. Saitou M, Yamaji M. Primordial germ cells in mice. Cold Spring Harb Perspect Biol. (2012) 4:a008375. doi: 10.1101/cshperspect.a008375
71. Iqbal K, Tran DA, Li AX, Warden C, Bai AY, Singh P, et al. Deleterious effects of endocrine disruptors are corrected in the mammalian germline by epigenome reprogramming. Genome Biol. (2015) 16:59. doi: 10.1186/s13059-015-0619-z
72. Whitelaw E Disputing lamarckian epigenetic inheritance in mammals. Genome Biol. (2015) 16:60. doi: 10.1186/s13059-015-0626-0
73. Heard E, Martienssen RA. Transgenerational epigenetic inheritance: myths and mechanisms. Cell. (2014) 157:95–109. doi: 10.1016/j.cell.2014.02.045
74. Flyamer IM, Gassler J, Imakaev M, Brandao HB, Ulianov SV, Abdennur N, et al. Single-nucleus Hi-C reveals unique chromatin reorganization at oocyte-to-zygote transition. Nature. (2017) 544:110–4. doi: 10.1038/nature21711
75. Yu J, Yang X, Yang X, Yang M, Wang P, Yang Y, et al. Nonylphenol aggravates non-alcoholic fatty liver disease in high sucrose-high fat diet-treated rats. Sci Rep. (2018) 8:3232. doi: 10.1038/s41598-018-21725-y
76. Batista TM, Alonso-Magdalena P, Vieira E, Amaral ME, Cederroth CR, Nef S, et al. Short-term treatment with bisphenol-A leads to metabolic abnormalities in adult male mice. PLoS ONE. (2012) 7:e33814. doi: 10.1371/journal.pone.0033814
77. Regnier SM, Kirkley AG, Ruiz D, Kamau W, Wu Q, Kannan K, et al. Diet-dependence of metabolic perturbations mediated by the endocrine disruptor tolylfluanid. Endocr Connect. (2018) 7:159–68. doi: 10.1530/EC-17-0320
78. Heindel JJ, Blumberg B, Cave M, Machtinger R, Mantovani A, Mendez MA, et al. Metabolism disrupting chemicals and metabolic disorders. Reprod Toxicol. (2017) 68:3–33. doi: 10.1016/j.reprotox.2016.10.001
Keywords: obesogens, obesity, MSCs, 3T3-L1, transgeneration, isoDMBs, tributyltin, metabolism
Citation: Chamorro-Garcia R and Blumberg B (2019) Current Research Approaches and Challenges in the Obesogen Field. Front. Endocrinol. 10:167. doi: 10.3389/fendo.2019.00167
Received: 21 November 2018; Accepted: 28 February 2019;
Published: 22 March 2019.
Edited by:
Angel Nadal, Universidad Miguel Hernández de Elche, SpainReviewed by:
Isabel Navarro, University of Barcelona, SpainPaloma Alonso-Magdalena, Universidad Miguel Hernández de Elche, Spain
Copyright © 2019 Chamorro-Garcia and Blumberg. This is an open-access article distributed under the terms of the Creative Commons Attribution License (CC BY). The use, distribution or reproduction in other forums is permitted, provided the original author(s) and the copyright owner(s) are credited and that the original publication in this journal is cited, in accordance with accepted academic practice. No use, distribution or reproduction is permitted which does not comply with these terms.
*Correspondence: Bruce Blumberg, Ymx1bWJlcmdAdWNpLmVkdQ==