- 1State Key Laboratory of Biocontrol, Guangdong Provincial Key Laboratory for Aquatic Economic Animals and Guangdong Provincial Engineering Technology Research Center for Healthy Breeding of Important Economic Fish, School of Life Sciences, Sun Yat-Sen University, Guangzhou, China
- 2Department of Aquaculture, National Taiwan Ocean University, Keelung, Taiwan
- 3Laboratory for Marine Fisheries Science and Food Production Processes, Qingdao National Laboratory for Marine Science and Technology, Qingdao, China
- 4College of Ocean, Key Laboratory of Tropical Biological Resources of Ministry of Education, Hainan University, Haikou, China
Sex differentiation in teleost fishes occurs in response to sex determination signals, which induce the gonad to develop as either an ovary or testis. However, sex differentiation mechanisms in fishes are diverse, and information on gonad differentiation in sex changing fishes remains limited. The orange-spotted grouper (Epinephelus coioides) is a protogynous hermaphroditic fish that provides an ideal model for investigating gonad differentiation in vertebrates. In this study, Transcriptome data showed that expression levels of amh and amhrII in gonads were increased during sex differentiation. Then we investigated the effect of overexpression anti-Müllerian hormone (Amh) on gonad development in juvenile orange-spotted groupers. Expression levels of female-related genes and serum 17β-estradiol levels were decreased, while expression of male-related genes and serum 11-ketotestosterone levels were increased in fish fed with amh-plasmid. Overexpression of Amh was also promoted the spermatogonia proliferation and induced the development of male gonads in undifferentiated orange-spotted groupers, but that this male tendency was preceded by female differentiation. In summary, these results illustrated that Amh overexpression by amh-plasmid feeding induced male gonad development in undifferentiated groupers.
Introduction
Sexual reproduction depends on the production of two types of gametes, which in turn rely on the specialized functions of the female and male gonads. Sex determination leads to a binary choice of gonad fate, to form either an ovary or a testis. The undifferentiated gonadal primordium is considered to be bipotential, and its subsequent development as either an ovary or testis in response to sex-determination signals is referred to as sex differentiation (1, 2).
The gonads of hermaphroditic, sex-changing fishes might be expected to have higher sexual plasticity than gonads of gonochoristic fishes; however, the unique and complex diversity of fish sex differentiation means that information on gonad differentiation in sex-changing fishes remains limited (1). Administration of low doses of exogenous sex steroid hormones (androgens or estrogens) leads to sex reversal in medaka, and this method has demonstrated that sex steroid hormones play an important role in gonad differentiation in a wide variety of fish species (3–5). Molecular biological studies have also shown that endogenous estrogen is a natural inducer of ovarian differentiation in gonochoristic teleosts (6–9). However, other hormones other than sex hormones can also influence sex differentiation in fish, including anti-Müllerian hormone (AMH), encoded by the amh gene (1). In female mammals, AMH acts by decreasing aromatase (cyp19a1)biosynthesis rather than by blocking enzyme activity (10). However, several of fishes show a negative correlation between amh and aromatase expression during the sex differentiation phase (11). Furthermore, higher levels of amh are correlated with lower cyp19a1a in zebrafish. These data indicate that amh is a candidate gene downregulating cyp19a1a, potentially leading to juvenile ovary-to-testis transformation (12). However, the role of amh during fish sex differentiation remains unclear.
Anti-Müllerian hormone (AMH) belongs to the transforming growth factor-beta superfamily, and has been implicated in male sex differentiation, female follicular development, and steroidogenesis in both sexes in mammals (13, 14). Although the “anti-Müllerian duct” effects of AMH have primarily been emphasized in higher vertebrates, amh has been identified in many teleost fish species that lack a Müllerian duct, such as Japanese eel (Anguilla japonica) (15), medaka (Oryzias latipes) (16), zebrafish (Danio rerio) (17), Japanese flounder (Paralichthys olivaceus) (18), and others (11), and has been shown to modulate the proliferation of spermatogonia (19). A duplicate copy of amh on the Y sex chromosome (amhY) is the master sex determinant in Atherinopsidae (Odontesthes hatcheri) (20) and Nile tilapia (Oreochromis niloticus) (21). The amh receptor type II gene (amhrII) has been identified as a sex-determining gene in Japanese pufferfish (Takifugu rubripes) (22), and amhrII mutations lead to sex reversal in medaka (O. latipes) (19, 23). These results suggest that amh may play a crucial role in sex differentiation in fish.
The orange-spotted grouper (Epinephelus coioides) is a protogynous hermaphroditic fish, which is economically valuable and widely cultured in southern Asia (24). These observations imply that ovarian differentiation may be the primary status in all sequential hermaphrodite species. In groupers, the gonads of all individuals initially differentiate directly into ovaries at approximately 47 days post-hatching (dph) (25), and most individuals initially mature as females at the age of 4–5 years, after which some individuals may undergo sex reversal from females to males (26–29), and primary oocytes were observed in the gonads of 1–3-year-old groupers (30). The orange-spotted grouper thus represents an ideal model for investigating gonad differentiation in vertebrates.
We previously showed that feeding groupers with an amh-overexpression plasmid induced female-to-male transition (31). However, the function of amh in the sex-differentiation process in groupers remains unknown. We therefore investigated the role of amh in sex differentiation in groupers using transcriptome sequencing technology to determine the amh expression patterns in gonads during the process of sex differentiation. Groupers were fed an amh-overexpression plasmid and the resulting expression patterns were investigated by immunohistochemistry (IHC), Western blotting, and real-time polymerase chain reaction (PCR) technology, to explore the potential role of amh in sex differentiation. The results will shed light on the molecular mechanisms regulating sex differentiation in marine fish and further our understanding of sex differentiation, and may ultimately provide guidance for innovations in grouper culture technology.
Materials and Methods
Animals and Tissue Sampling
Undifferentiated female groupers [75 days post-hatching (dph)] provide a useful animal model for investigating the regulation of sexual fate. Normally fed groupers were obtained from the Daya Bay Fisheries Development Center (Huizhou City, Guangdong Province, China), including fish from undifferentiated to complete differentiation stages. Gonad samples from normally fed groupers at 50, 80, 100, 130, 160, 180, and 240 days were also examined histologically.
Plasmid-feeding experiments were conducted over 125 consecutive days in orange-spotted groupers with undifferentiated gonads (age 80 days, body weight 22–35 g, body length 4.5–6.5 cm; Daya Bay Fisheries Development Center). All animal experiments were conducted in accordance with the guidelines and approval of the Animal Research and Ethics Committee of Sun Yat-Sen University.
Transcriptome Sequencing
Transcriptome sequencing has been widely used to study gene expression changes, variable splicing, post-translational modifications, and mutations, etc., especially in different samples at different time points, or to examine changes in gene expression under different processing conditions (32). Gonad samples were obtained from normally fed groupers at 50, 90, 110, 180, and 240 dph, with two biological replicates per sample. Ten mRNA libraries were constructed from purified RNA of these gonad. mRNA-Seq (mRNA sequencing) services were carried out using Illumina HiSeq 2000 platform (Illumina Inc., San Diego, CA, USA) provided by BGI-Shenzhen, China.
Packaging of Recombinant amh Plasmid for Feeding Experiments
An amh-overexpression plasmid was constructed by cloning the amh sequence into pcDNA4.0 (+) vector (Invitrogen, Carlsbad, CA, USA). The plasmids were then encapsulated in liposomes, which were formed by thin film hydration using DOTAP (Sigma-Aldrich, USA) and cholesterol (Sigma-Aldrich), based on a modification of our previous study (33). Briefly, DOTAP and cholesterol were heated to 70°C followed by heating in a water bath at 65°C for 2 h and ultrasonication at 25°C for 10 min. The mixture was then extruded through a 100-nm membrane filter and used to encapsulate empty pcDNA4.0 (+) plasmid or pcDNA4.0 (+)-amh plasmid by ultrasonication at 25°C for 30 min. The final concentration of the construct was 20 μg/μL.
The fish were divided randomly into two groups: an empty plasmid [empty pcDNA4.0 (+)] feeding group (n = 35) and an amh-plasmid [pcDNA4.0 (+)-amh] feeding group (n = 35). Both groups were cultured in separate seawater tanks. The empty plasmid group was fed a diet containing empty plasmid (1 mL/50 g food weight) and the amh-plasmid group was fed a diet mixed with amh plasmid (1 mL/50 g food weight). Seven individuals from each group were randomly sacrificed at 25, 50, 75, 100, and 125 days after treatment (dat), respectively and gonad tissues and serum samples were collected.
RNA Isolation and Gene Expression Analysis
Total RNA was extracted from the gonads of orange-spotted groupers using TRIzol reagent (Invitrogen, America) and the cDNA samples were sent to BGI (Shenzhen, China) for sequencing. cDNA was produced from 1 μg total RNA from the feeding experiment samples using a Transcriptor First Strand cDNA Synthesis Kit (Roche, Swiss) and used as the template for subsequent real-time PCR analyses. The specific primers used in this study are listed in Table 1. The transcription levels of the target genes amh, amhrII (typeII anti-Müllerian hormone receptor), sox9 (sex-determining region y-box 9), cyp11b (11beta-hydroxylase), dmrt1 (doublesex and mab-3-related transcription factor 1), hsd11b2 (11β-hydroxysteroid dehydrogenase type2), cyp19a1a (aromatase P450), and foxl2 (ForkheadboxL2) were measured using a SYBR Green PCR Master Mix Kit (ABI, Vernon, CA, USA) with an ABI Real-Time PCR Fast System (ABI). The quantitative real-time PCR conditions were as follows: denaturation at 95°C for 5 min, followed by 40 cycles of 95°C for 15 s, 58°C for 20 s, 72°C for 20 s, and then 84°C for 10 s (fluorescent data collection). After amplification, the fluorescent data were converted to threshold cycle values (Ct). The relative abundance of mRNA transcripts was then evaluated using the formula: R = 2−ΔΔCt (34).
Gonad Histology
Gonads were dissected, fixed in Bouin's solution for 24 h at room temperature, dehydrated, and embedded in paraffin. The tissue blocks were sectioned at 5 μm using a microtome (RM2235; Leica, Germany) and stained with hematoxylin and eosin for gonad histology analysis.
Western Blot and IHC Analysis
Rabbit anti-Amh and anti-Dmrt1 antibodies were produced by our laboratory previously (30, 31). Gonad tissues from plasmid-fed fish were examined by Western blotting using rabbit anti-Amh antibody (1:2,000 dilution; present study) and β-actin antibody (1:5,000 dilution; RRID: AB_2289225; Proteintech, USA) to detect the expression of amh and accumulation of the plasmid in the gonads.
IHC analyses were performed as described previously (35). Rabbit anti-Dmrt1 antibody (1:200 dilution) (30) was used to detect the emergence of spermatogonia in gonads from plasmid-fed fish. The samples were photographed under a light microscope (Nikon, Japan). The specific antibodies used in this study are listed in Table 2.
Serum 17β-estradiol (E2) and 11-ketotestosterone (11-KT) Assays
Blood samples were collected from the caudal vein of fish from each group. Serum samples were produced by centrifugation at 4°C and were stored at −20°C. Serum E2 and 11-KT levels were measured using enzyme immunoassay kits (Cayman Chemical Company, Ann Arbor, MI, USA).
Terminal Deoxynucleotidyl Transferase dUTP Nick End Labeling (TUNEL) Assay During amh Plasmid Feeding
Apoptotic cells were detected using a TUNEL Apoptosis Detection Kit (Phygene, Fuzhou, China) according to the manufacturer's instructions. The samples were photographed under a light microscope (Nikon).
Statistical Analyses
All data are expressed as the mean ± standard error of the mean (SEM). Statistical differences were estimated using Student's t-tests or one-way analysis of variance followed by Tukey's tests (SPSS Software, USA). A value of *P < 0.05 or different letters indicate significant differences.
Results
Histology of Gonads in Normal Orange-Spotted Groupers From Undifferentiated to Fully Differentiated Stage
The gonad differentiation profile in groupers is shown in Figure 1A. We observed the gonads in normally-fed groupers from the undifferentiated stage to the completion of differentiation, at 50, 80, 100, 130, 160, 180, and 240 dph by histology. The gonad primordium had appeared at 50 dph (Figure 1Ba), blood vessels were present at 80 dph (Figure 1Bb), and the gonads had begun to differentiate by 100 dph and the initial ovarian cavity appeared (Figure 1Bc). The gonads continued to differentiate from 130–160 dph, the gonia appeared and continued to increase in number, and the ovarian cavity appeared (Figures 1Bd1,d2), the gonads continued to differentiate and oogonia appeared at 180 dph (Figure 1Be), and gonad differentiation was complete and primary oocytes were present by 240 dph (start of ovarian development stage) (Figure 1Bf). These results were consistent with those of previous studies (24).
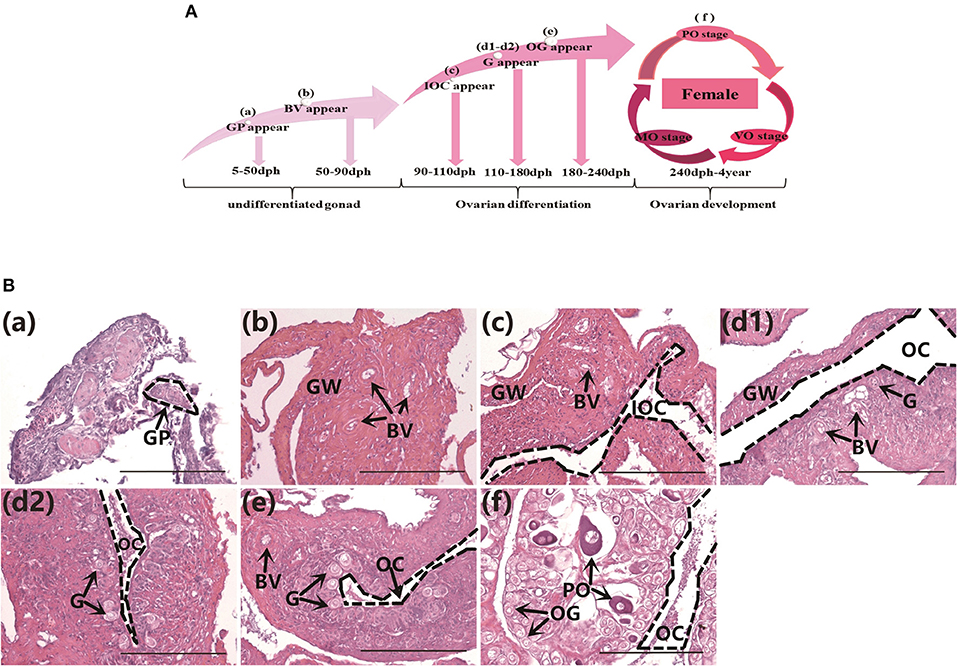
Figure 1. Gonad histology in normal orange-spotted groupers. (A) Gonad differentiation profiles in normal groupers. (B) Gonad histology in normal groupers at different developmental stages. (Ba) Gonad primordium appeared 50 days post-hatching (dph). (Bb) Blood vessels appeared 80 dph. (Bc) Initial ovarian cavity appeared 100 dph. (Bd1,Bd2) Gonia appeared 130–160 dph. (Be) Oogonia appeared 180 dph. (Bf) Primary oocytes appeared 240 dph. GP, gonad primordium; GW, gonad wall; BV, blood vessel; IOC, initial ovarian cavity; G, gonia; OC, ovarian cavity; OG, oogonium; PO, primary oocyte. Scale bars, 100 μm.
Identification of Genes Related to sex Differentiation by Transcriptome Sequencing
We previously revealed the approximate sex differentiation period in groupers (Yong Zhang & Qing Wang, unpublished observations). In the current study, we performed transcriptome analysis of gonads at different time intervals to screen for sex differentiation-related gene expression, an carried out transcriptome sequencing at 50, 90, 110, 180, and 240 days post-hatching (dph) from undifferentiated gonads to complete gonad differentiation. Regarding the expression levels of genes related to sex differentiation in vivo, expression levels of the female-related genes cyp19a1a and foxl2 continued to rise, while the male-related genes hsd11b2 and dmrt1 remained at persistently low levels or were not expressed. Previous studies suggested that amh plays an important role in testis development (16, 21). However, we found that expression levels of both amh and amhrII were increased during sex differentiation in gonads (Figure 2). This suggested that amh expression may be essential for sex differentiation in gonads, but more in-depth studies were needed to determine the function of amh in the process of sex differentiation.
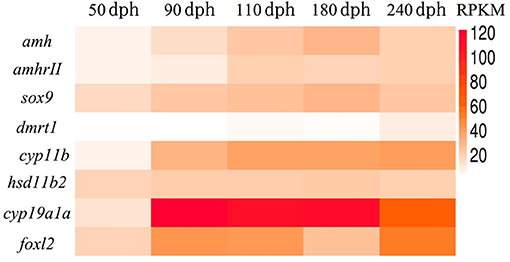
Figure 2. Transcriptome sequencing analysis of genes related to sex differentiation in normal orange-spotted grouper. All data were sampled from normal feeding grouper. RPKM (Reads Per Kilobase Million) of genes related to sex differentiation after transcriptome sequencing.
Detection of Amh Levels by Western Blotting Following Plasmid Feeding
We detected Amh levels in vivo by Western blotting in groupers fed with amh plasmid, using β-actin as an internal control. Amh expression was significantly increased in the amh-plasmid group on all sampled days, but not in the empty-plasmid group. These results confirmed that feeding groupers with amh plasmid increased Amh protein expression in the gonad in vivo (Figures 3A,B).
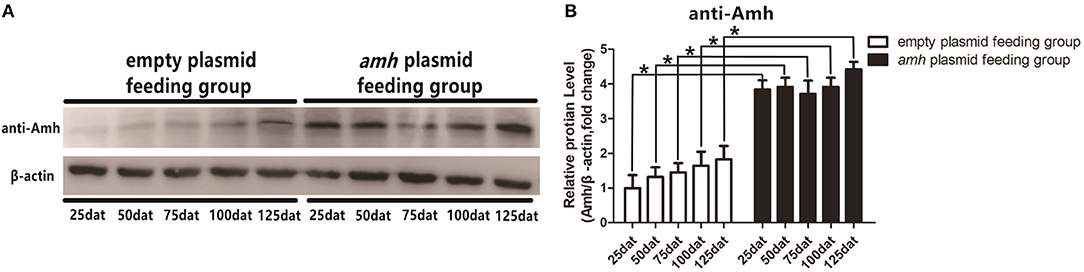
Figure 3. Western blot analysis of Amh expression in orange-spotted groupers following amh-plasmid feeding. (A) Amh expression was detected by western blot using rabbit anti-Amh antibody in gonads from groupers fed with amh plasmid. (B) Densitometric analysis of amh expression levels in gonads. Statistical differences were estimated using the Student's t-test analysis of variance followed by Tukey's tests (SPSS Software). *P < 0.05 indicate significant differences.
Amh Over-expression Changed the Direction of Sex Differentiation
The gonadal status of each individual was examined by histology during the feeding experiments. The gonads developed normally in the empty-plasmid group from 25–50 dat (Figures 4A,B), and a few gonia appeared at 75 dat (Figure 4C), and some oogonia and primary oocytes appeared from 100–125 dat (Figures 4D,E). In contrast, gonad structure differed in the amh-plasmid group: the gonads developed normally at 25 dat (Figure 4F), but gonia formation was only observed in one fish at 50 dat, while no gonia were present in the other fish gonads (Figure 4G). About a third of gonads had a small amount gonia by 75 dat (Figures 4H,K), and about two-thirds had numerous gonia by 100 dat, while the remainder still had few gonia (Figures 4I,L). All gonads had abundant gonia by 125 dat (Figures 4J,M). In conclusion, normal gonads in empty-plasmid-fed fish developed abundant oogonia and primary oocytes, while amh-overexpressing fish (amh-plasmid-fed) developed a greater number of gonia, without primary oocytes (Table 3).
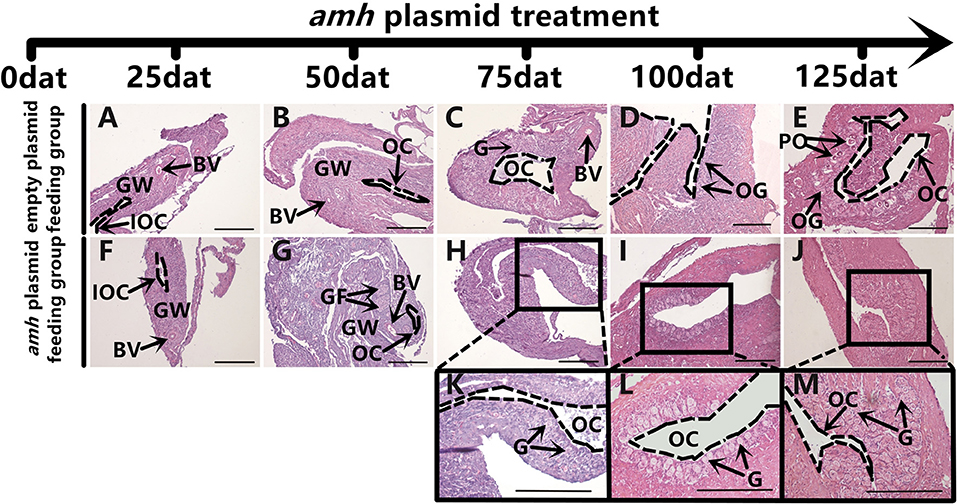
Figure 4. Gonad histology in orange-spotted groupers following amh-plasmid feeding. (A–E) Gonad histology in groupers fed empty plasmid at different sampling times. (F–M) Gonad histology in groupers fed amh plasmid at different sampling times. Panels (K–M) show high magnification views of boxed areas in (H–J), respectively. GW, gonad wall; BV, blood vessel; IOC, initial ovarian cavity; GF, gonium formation; G, gonia; OC, ovarian cavity; OG, oogonium; PO, primary oocyte. Scale bars, 100 μm.
IHC Identification of Gonia in amh-Over-Expressing Fish
It is difficult to distinguish between oogonia and spermatogonia based on shape and size using light microscopy, and we therefore identified them by IHC. Dmrt1 is a male-specific gene in groupers (36), and Dmrt1 protein only exists in spermatogonia and in primary and secondary spermatocytes in orange-spotted groupers (37). We therefore confirmed the presence of spermatogonia by IHC detection of Dmrt1 expression. Hematoxylin and eosin staining of gonads sampled at different times as a control (Figures 5A,C,E,G,I,K). No Dmrt1 signals were detected in the gonads in the empty-plasmid-fed group (Figures 5B,D,F), but a positive Dmrt1 signal was found in the amh-plasmid-fed group (Figures 5H,J,L). Furthermore, strong Dmrt1-positive signals were observed in the gonads from 75–125 dat (Figures 5H,J,L–O). These results demonstrated that amh-plasmid feeding increased the male tendency of gonad development, thus confirming the idea that Amh overexpression caused undifferentiated orange-spotted groupers to develop into males.
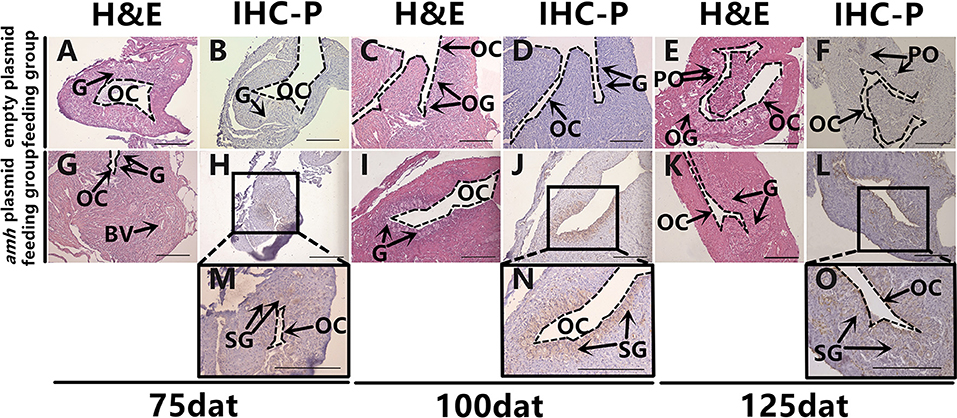
Figure 5. Dmrt1 signals detection in sex-differentiation process in orange-spotted grouper following amh-plasmid feeding. Hematoxylin and eosin staining of gonads sampled at different times (A,C,E,G,I,K). IHC staining of gonads at different sampling times using rabbit anti-Dmrt1 antibody (B,D,F,H,J,L–O). Panels (M–O) show high magnification views of boxed areas in (H,J,L), respectively. BV, blood vessel; OC, ovarian cavity; G, gonia; OG, oogonium; PO, primary oocyte; SG, spermatogonia. Scale bars, 100 μm.
Gene Expression and Serum Steroid Hormone Level Changes During amh-Plasmid Feeding
We also analyzed the expression profiles of key sex-differentiation genes during amh-plasmid feeding. Amh and amhrII expression levels were significantly increased from 100–125 dat (Figures 6A,B), dmrt1 and cyp11b were significantly increased from 75–125 dat (Figures 6D,E), and sox9 and hsd11b2 were significantly increased from 50–125 dat (Figures 6C,F), while cyp19a1a and foxl2 expression levels were significantly decreased from 75–125 dat (Figures 6G,H). These results indicated that amh-plasmid feeding stimulated male-pathway gene expression (sox9, dmrt1, cyp11b, and hsd11b2) and suppressed female-pathway gene expression (cyp19a1a, foxl2).
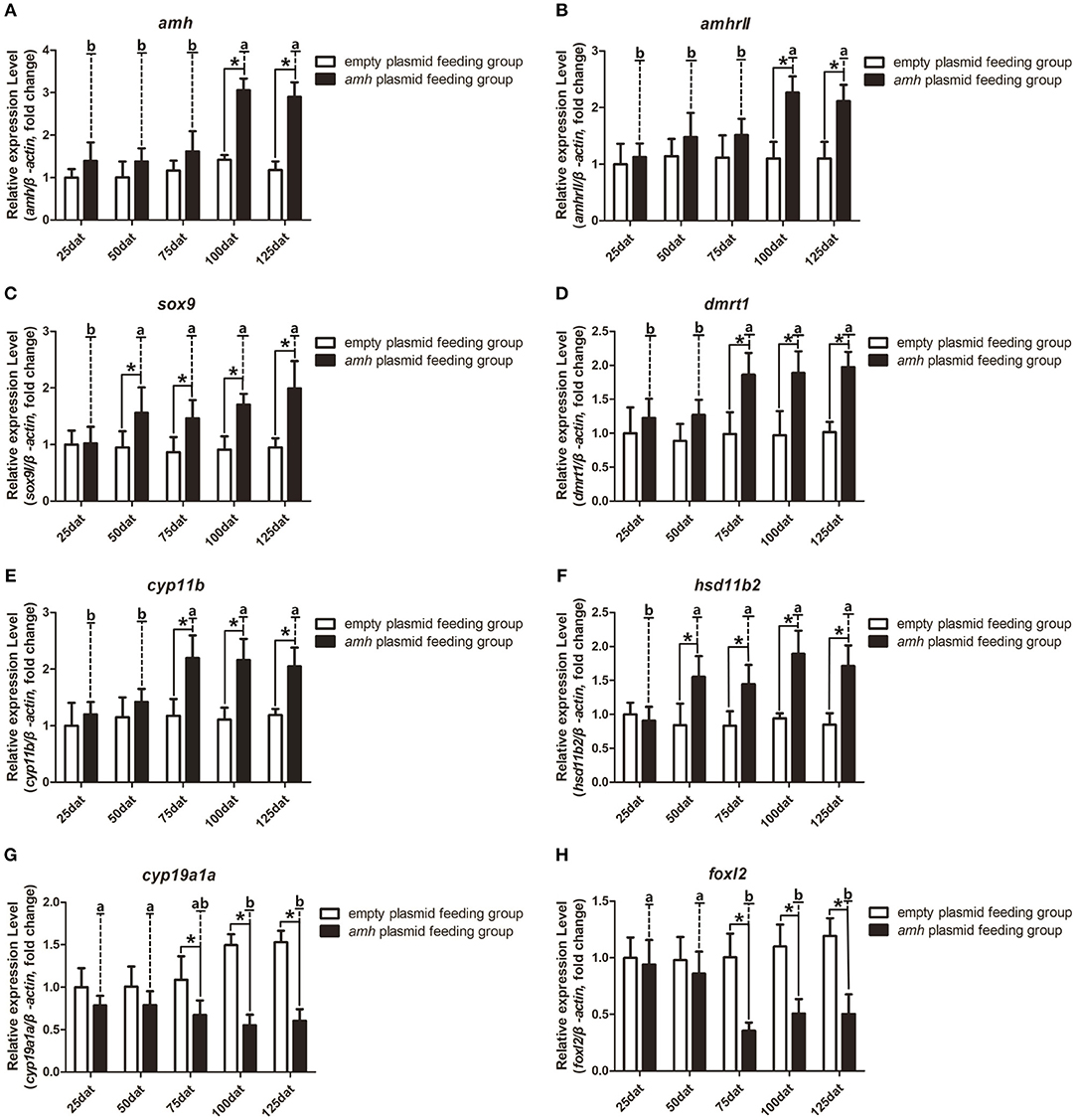
Figure 6. Expression profiles of key sex-differentiation genes in orange-spotted groupers following amh-plasmid feeding. (A–F) Gene expression of amh (A), amhrII (B), sox9 (C), dmrt1 (D), cyp11b (E), hsd11b2 (F), cyp19a1a (G), and foxl2 (H) during amh-overexpression plasmid-induced sex reversal. β-actin was used as the internal control. Data expressed as the mean ± S.E.M from seven fish samples. Statistical differences were estimated using the Student's t-test or one-way analysis of variance followed by Tukey's tests (SPSS Software). *P < 0.05 or different letters indicate significant differences.
A balance between endogenous estrogens and androgens is known to be required to maintain the sexual identity of many fish species, and steroidogenesis plays a critical role in fish sex differentiation (30). amh-plasmid feeding significantly decreased serum E2 levels and significantly increased 11-KT levels from 75–125 dat compared with empty-plasmid-fed fish (Figures 7A,B). Estrogen is known to play an important role in maintaining ovarian development (30), and gradually reduced expression of female sex-differentiation genes in the gonads could lead to decreased E2 levels, resulting in degradation of female germ cells or disrupted ovarian development. In contrast, 11-KT plays a critical role in maintaining the testes, and high expression of male sex differentiation genes in the gonads could change the tendency of sex differentiation. The above results suggested that gonads may tend to develop in a male direction due to decreased estrogen levels and increased androgen levels in amh-plasmid-fed groupers.
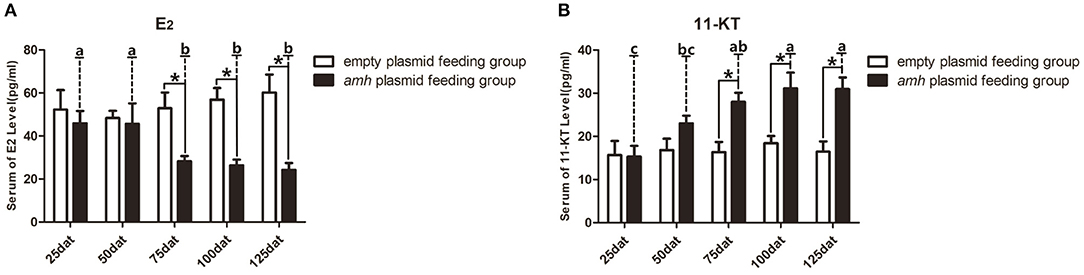
Figure 7. Serum sex steroid hormone levels in orange-spotted groupers following amh-plasmid feeding. (A) E2 levels in the empty-plasmid- and amh-plasmid-fed groups. (B) 11-KT levels in the empty-plasmid- and amh-plasmid-fed groups. Data expressed as the mean ± SEM from seven fish samples. Statistical differences were estimated using the Student's t-test or one-way analysis of variance followed by Tukey's tests (SPSS Software). *P < 0.05 or different letters indicate significant differences.
Apoptosis During Sex Differentiation in amh-plasmid-fed Groupers
Groupers are well-known to be protogynous fish, with primary oocytes observed in the gonads of 1–3-year-old orange-spotted groupers (30). Most orange-spotted groupers first mature as females at 4–5 years old, and some may then undergo sex reversal from females to males (26–29). We aimed to determine if amh-plasmid feeding before sex differentiation induced the gonads to develop directly into males, or if the gonads first developed as females before showing male differentiation. We therefore detected gonadal apoptosis in amh-plasmid-fed fish during differentiation. No apoptosis was detected in the empty plasmid group from 25–125 dat (Figures 8A–E), while gonads in the amh-plasmid-fed group showed an apoptotic signal at 25 dat (Figures 8F,K), which increased from 50–75 dat (Figures 8G,H,L,M), but was absent from 100–125 dat (Figures 8I,J,N,O). There are also positive and negative controls (Figures 8P,Q). These results suggested that Amh overexpression induced the development of male gonads in undifferentiated orange-spotted groupers, but that this male tendency was preceded by female differentiation (Table 4).
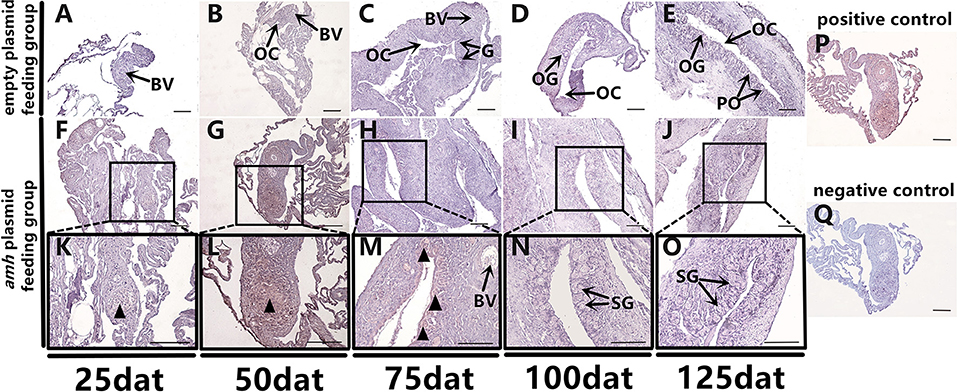
Figure 8. Apoptosis detection in sex-differentiation process in orange-spotted grouper following amh-plasmid feeding. Apoptosis in gonads sampled at different times. Black triangular arrow indicates apoptotic signal. (A–E) Gonad apoptosis in empty-plasmid-fed groupers at different sampling times. (F–O) Gonad apoptosis in amh-plasmid-fed groupers at different sampling times. (P,Q) Positive and negative controls. Panels (K–O) Show high magnification views of the boxed areas in (F–J) respectively. BV, blood vessel; OC, ovarian cavity; G, gonia; OG, oogonium; PO, primary oocyte; SG, spermatogonia. Scale bars, 50 μm.
Discussion
In this study, we observed the gonads in normally-fed groupers from the undifferentiated stage to the completion of differentiation (Figure 1). These results were consistent with those of previous studies (24). Despite the fact that hermaphroditism is widespread in many fish lineages (38), but only studies on sex differences have been conducted in only a few hermaphrodites. As the grouper is a protogynous hermaphroditic fish, therefore, the expression level of amh in the gonad differentiation of grouper is different from the gonochoristic fish.
In gonochoristic fish, elevated amh levels in male gonads are a common feature in fish during sex differentiation phase. That indicates an involvement of amh in male sex differentiation. Therefore, in recent gene expression studies elevated amh levels has been used as an indirect method for sexing developing gonads from Japanese flounder (39), Southern flounder (40), Atlantic cod (41), Nile tilapia (42), and pejerrey (43, 44). The divergent amh expression patterns in different species reflect the different modes of sex differentiation in fish (11). Through transcriptome sequencing data, we found that expression levels of both amh and amhrII were increased during sex differentiation in gonads (Figure 2). We speculate that although the expression of amh in the ovary is much lower than that in the testis, amh also plays a role in regulating ovarian development, but the role of amh in ovary require a more in-depth study. Besides, through transcriptome sequencing data, we also found regarding the expression levels of the female-related genes cyp19a1a and foxl2 continued to rise, while the male-related genes hsd11b2 and dmrt1 remained at persistently low levels or were not expressed.
Despite the diversity of sex-differentiation processes among fishes, ovarian differentiation can be directed by estrogens and their synthetic aromatase enzymes in most teleosts. P450 aromatase (cyp19a1a) is the most important steroidogenic enzyme for ovarian differentiation and plays an essential role in the production of E2, which is believed to be the major sex hormone for inducing and maintaining ovarian development in fish (4). In female mammals, AMH acts by decreasing aromatase (cyp19a1)biosynthesis rather than by blocking enzyme activity (10). However, several of fishes show a negative correlation between amh and aromatase expression during the sex differentiation phase (11). An inverse association between cyp19a1a and amh expression has been reported in numerous fishes, including Japanese flounder (P. olivaceus) (45), rainbow trout (Oncorhynchus mykiss) (46), zebrafish (D. rerio) (12), southern flounder (Paralichthys lethostigma) (40), and pejerrey (Odontesthes bonariensis) (43). Although a causal relationship cannot be established from these studies, the results suggest that amh is a candidate gene down-regulating cyp19a (12). We also found an inverse association between amh and cyp19a1a expression in our current study, and amh overexpression in vivo inhibited cyp19a1a gene expression and led to significantly decreased serum E2; however, it remains unclear if amh directly downregulates cyp19a1a expression. We also analyzed the expression profiles of other key sex-differentiation-related genes during gonad differentiation in groupers. Feeding with an amh plasmid stimulated male-pathway gene expression (sox9, dmrt1, cyp11b, and hsd11b2) and suppressed female-pathway gene expression (cyp19a1a, foxl2), and induced a male tendency during sex differentiation in undifferentiated groupers. These results suggested that amh may have an important role in sex reversal in protogynous fish, by suppressing gonadal aromatase expression and/or activating a male-specific expression pathway.
The AMH gene is widely found in the gonads in invertebrates and vertebrates, and plays an important regulatory role in gonad development in vertebrates, and AMH is important for primordial follicle recruitment and folliculogenesis in mammals (47, 48). In contrast to mammals however, amh expression differs among different species of fish and its role in the ovary in teleosts, especially protogynous hermaphroditic species, remains largely unknown. A previous study showed that Amh influenced germ cell number, and high expression levels during early male development suggested that it played a role in male sex determination (11). Furthermore, feeding groupers with an amh-overexpression plasmid induced female-to-male transition (31). No sex chromosomes have yet been identified in groupers, which may be due to the special sex differentiation mechanism of female prematuration of hermaphrodites, and further sex differentiation research at the gene level is still required (49). Increasing evidence suggests that the amh gene plays an indispensable role in the process of fish sex differentiation (20, 50), though its involvement in early sex differentiation in the orange-spotted grouper remains unclear. The current results showed that fish fed an amh plasmid developed a male tendency, thus supporting the idea that Amh overexpression could induce male sex in undifferentiated orange-spotted groupers, while male tendency was preceded by female differentiation. However, further studies are needed to establish the time required to achieve complete male sex differentiation, and whether withdrawal of amh supplementation would lead to reversion to ovaries.
In summary, amh played an important role in sex differentiation in groupers, including orange-spotted groupers that Amh overexpression caused undifferentiated orange-spotted groupers to develop into males. A molecular-level understanding of sex differentiation, including the upstream factors responsible for initiating this process, would enable the development of efficient, low cost genetic tools for controlling sex ratios, thereby avoiding the use of steroid treatments, which pose significant environmental contamination risks. However, further studies are needed to clarify the mechanism and regulatory networks of amh in the process of sex differentiation in bony fish.
Author Contributions
YH, MZ, and YZ contributed conception and design of the study. YH and LW organized the database. ZY, JW, and QY performed the statistical analysis. ML provides the packaging of recombinant amh plasmid. YH wrote the first draft of the manuscript. MZ, LX, SL, YZ, and HL wrote sections of the manuscript. All authors contributed to manuscript revision, read, and approved the submitted version.
Funding
This work is supported by National Natural Science Foundation of China (Nos. 31702324, 31802274, 31572596, 31672631), Guangdong Provincial Natural Science Foundation (2018B030311026, 2018A030313890), Guangdong Provincial Science and Technology Program (2013B090400017, 2017B090904022), Science and Technology Planning Project of Guangzhou (201804020013), Special Fund for Fisheries-Scientific Research of Guangdong Province (SDYY-2018-04), Special Fund of State Key Laboratory of Developmental Biology of Freshwater (2018KF001), and the Fundamental Research Funds for the Central Universities (17lgpy115).
Conflict of Interest Statement
The authors declare that the research was conducted in the absence of any commercial or financial relationships that could be construed as a potential conflict of interest.
References
1. Siegfried KR. In search of determinants: gene expression during gonadal sex differentiation. J Fish Biol. (2010) 76:1879–902. doi: 10.1111/j.1095-8649.2010.02594.x
2. Capel B. Vertebrate sex determination: evolutionary plasticity of a fundamental switch. Nat Publ Gr. (2017) 18:675–89. doi: 10.1038/nrg.2017.60
3. Yamamoto TO. Artificial induction of functional sex-reversal in genotypic females of the medaka (Oryzias latipes). J Exp Zool. (1958) 137:227–63. doi: 10.1002/jez.1401370203
4. Devlin RH, Nagahama Y. Sex determination and sex differentiation in fish: an overview of genetic, physiological, and environmental influences. Aquaculture. (2002) 208:191–364. doi: 10.1016/S0044-8486(02)00057-1
5. Guiguen Y, Fostier A, Piferrer F, Chang CF. Ovarian aromatase and estrogens: a pivotal role for gonadal sex differentiation and sex change in fish. Gen Comp Endocrinol. (2010) 165:352–66. doi: 10.1016/j.ygcen.2009.03.002
6. Sudhakumari CC, Senthilkumaran B, Kobayashi T, Kajiura-Kobayashi H, Wang DS, Yoshikuni M, et al. Ontogenic expression patterns of several nuclear receptors and cytochrome P450 aromatases in brain and gonads of the Nile tilapia Oreochromis niloticus suggests their involvement in sex differentiation. in Fish Physiol Biochem.(2005) 129–135. doi: 10.1007/s10695-006-0014-5
7. Ijiri S, Kaneko H, Kobayashi T, Wang D-S, Sakai F, Paul-Prasanth B, et al. Sexual dimorphic expression of genes in gonads during early differentiation of a teleost fish, the nile tilapia oreochromis niloticus1. Biol Reprod. (2008) 78:333–41. doi: 10.1095/biolreprod.107.064246
8. Ruksana S, Pandit NP, Nakamura M. Efficacy of exemestane, a new generation of aromatase inhibitor, on sex differentiation in a gonochoristic fish. Comp Biochem Physiol C Toxicol Pharmacol. (2010) 152:69–74. doi: 10.1016/j.cbpc.2010.02.014
9. Piferrer F, Zanuy S, Carrillo M, Solar II, Devlin RH, Donaldson EM. Brief treatment with an aromatase inhibitor during sex differentiation causes chromosomally female salmon to develop as normal, functional males. J Exp Zool. (1994) 270:255–62. doi: 10.1002/jez.1402700304
10. Vigier B, Forest MG, Eychenne B, Bézard J, Garrigou O, Robel P, et al. Anti-Müllerian hormone produces endocrine sex reversal of fetal ovaries. Proc Natl Acad Sci USA. (1989) 86:3684–8. doi: 10.1073/pnas.86.10.3684
11. Pfennig F, Standke A, Gutzeit HO. The role of Amh signaling in teleost fish - Multiple functions not restricted to the gonads. Gen Comp Endocrinol. (2015) 223:87–107. doi: 10.1016/j.ygcen.2015.09.025
12. Wang XG, Orban L. Anti-Müllerian hormone and 11 β-hydroxylase show reciprocal expression to that of aromatase in the transforming gonad of zebrafish males. Dev Dyn. (2007) 236:1329–38. doi: 10.1002/dvdy.21129
13. Behringer RR, Finegold MJ, Cate RL. Müllerian-inhibiting substance function during mammalian sexual development. Cell. (1994) 79:415–25. doi: 10.1016/0092-8674(94)90251-8
14. Josso N, Racine C, Di Clemente N, Rodolfo Rey, Xavier F. The role of anti-Mullerian hormone in gonadal development. Mol Cell Endocrinol. (1998) 145:3–7.
15. Miura T, Miura C, Konda Y, Yamauchi K. Spermatogenesis-preventing substance in Japanese eel. Development. (2002) 129:2689–97. Available online at: http://dev.biologists.org/content/129/11/2689.short
16. Klüver N, Pfennig F, Pala I, Storch K, Schlieder M, Froschauer A, et al. Differential expression of anti-Müllerian hormone (amh) and anti-Müllerian hormone receptor type II (amhrII) in the teleost Medaka. Dev Dyn. (2007) 236:271–81. doi: 10.1002/dvdy.20997
17. Rodríguez-Marí A, Yan YL, BreMiller RA, Wilson C, Cañestro C, Postlethwait JH. Characterization and expression pattern of zebrafish anti-Müllerian hormone (amh) relative to sox9a, sox9b, and cyp19a1a, during gonad development. Gene Expr Pattern. (2005) 5:655–67. doi: 10.1016/j.modgep.2005.02.008
18. Yoshinaga N, Shiraishi E, Yamamoto T, Iguchi T, Abe SI, Kitano T. Sexually dimorphic expression of a teleost homologue of Müllerian inhibiting substance during gonadal sex differentiation in Japanese flounder, Paralichthys olivaceus. Biochem Biophys Res Commun. (2004) 322:508–13. doi: 10.1016/j.bbrc.2004.07.162
19. Morinaga C, Saito D, Nakamura S, Sasaki T, Asakawa S, Shimizu N, et al. The hotei mutation of medaka in the anti-Mullerian hormone receptor causes the dysregulation of germ cell and sexual development. Proc Natl Acad Sci USA. (2007) 104:9691–96. doi: 10.1073/pnas.0611379104
20. Hattori RS, Murai Y, Oura M, Masuda S, Majhi SK, Sakamoto T, et al. A Y-linked anti-Mullerian hormone duplication takes over a critical role in sex determination. Proc Natl Acad Sci USA. (2012) 109:2955–59. doi: 10.1073/pnas.1018392109
21. Li M, Sun Y, Zhao J, Shi H, Zeng S, Ye K, et al. A Tandem duplicate of anti-müllerian hormone with a missense SNP on the Y chromosome is essential for male sex determination in nile tilapia, oreochromis niloticus. PLoS Genet. (2015) 11:e1005678. doi: 10.1371/journal.pgen.1005678
22. Kamiya T, Kai W, Tasumi S, Oka A, Matsunaga T, Mizuno N, et al. A trans-species missense SNP in Amhr2 is associated with sex determination in the tiger Pufferfish, Takifugu rubripes (Fugu). PLoS Genet. (2012) 8:e1002798. doi: 10.1371/journal.pgen.1002798
23. Nakamura S, Watakabe I, Nishimura T, Picard J-Y, Toyoda A, Taniguchi Y, et al. Hyperproliferation of mitotically active germ cells due to defective anti-Mullerian hormone signaling mediates sex reversal in medaka. Development. (2012) 139:2283–7. doi: 10.1242/dev.076307
24. Liu M, de Mitcheson YS. Gonad development during sexual differentiation in hatchery-produced orange-spotted grouper (Epinephelus coioides) and humpback grouper (Cromileptes altivelis) (Pisces: Serranidae, Epinephelinae). Aquaculture. (2009) 287:191–202. doi: 10.1016/j.aquaculture.2008.10.027
25. Murata R, Karimata H, Ashraful Alam M, Nakamura M. Gonadal sex differentiation in the Malabar grouper, Epinephelus malabaricus. Aquaculture. (2009) 293:286–9. doi: 10.1016/j.aquaculture.2009.04.031
26. TAN M. S. Biology of the tropical grouper, Epinephelus tauvina (FORSKAL) I. Apreliminary study on hermaphroditism in E. tavina. Singapore J Pri Ind. (1974) 2:123–33
27. Chen FY, Chow M, Chao TM, Lim R. Artificial spawning and larval rearing of the grouper, Epinephelus tauvina (Forskal) in Singapore. Singapore J Prim Ind. (1977) 5:1–21
28. Heemstra PC, Randall JE. Groupers of the world (Family Serranidae, Subfamily Epinephelinae). (1993). doi: 10.1016/0025-326X(92)90600-B
29. Quinitio GF, Caberoy NB, Reyes DM. Induction of sex change in female Epinephelus coioides by social control. Isr J Aquac Bamidgeh. (1997) 49:77–83
30. Wang Q, Liu Y, Peng C, Wang X, Xiao L, Wang D, et al. Molecular regulation of sex change induced by methyltestosterone -feeding and methyltestosterone -feeding withdrawal in the protogynous orange-spotted grouper. Biol Reprod. (2017) 97:324–33. doi: 10.1093/biolre/iox085
31. Han Y, Peng C, Wang L, Guo J, Lu M, Chen J, et al. Female-to-male sex reversal in orange-spotted grouper (Epinephelus coioides) caused by overexpressing of Amh in vivo. Biol Reprod. (2018) 7:1205–15. doi: 10.1093/biolre/ioy157
32. Maher CA, Kumar-Sinha C, Cao X, Kalyana-Sundaram S, Han B, Jing X, et al. Transcriptome sequencing to detect gene fusions in cancer. Nature. (2009) 458:97–101. doi: 10.1038/nature07638
33. Su FJ, Lu M. Application and efficacy test of siRNA vaccine against nervous necrosis virus infection. Fish Shellfish Immunol. (2016) 53:123–4. doi: 10.1016/j.fsi.2016.04.122
34. Shved N, Berishvili G, Cotta HD, Eppler E, Reinecke M. Ethinylestradiol differentially interferes with IGF-I in liver and extrahepatic sites during development of male and female bony fish. J Endocrinol. (2005) 195:513–23. doi: 10.1677/JOE-07-0295
35. Kobayashi T, Chang X-T, Nakamura M, Kajiura H, Nagahama Y. Isomerase: antibody production and their use for the immunohistochemical detection of fish steroidogenic tissues. Zoolog Sci. (1996) 13:909–14. doi: 10.2108/zsj.13.909
36. Sandra GE, Norma MM. Sexual determination and differentiation in teleost fish. Rev Fish Biol Fish. (2010) 20:101–21. doi: 10.1007/s11160-009-9123-4
37. Xia W, Zhou L, Yao B, Li CJ, Gui JF. Differential and spermatogenic cell-specific expression of DMRT1 during sex reversal in protogynous hermaphroditic groupers. Mol Cell Endocrinol. (2007) 263:156–72. doi: 10.1016/j.mce.2006.09.014
38. Erisman BE, Petersen CW, Hastings PA, Warner RR. Phylogenetic Perspectives on the Evolution of Functional Hermaphroditism in Teleost Fishes. Integrat. Comparat. Biol. (2013) 53:736–54. doi: 10.1093/icb/ict077
39. Yamaguchi T, Yoshinaga N, Yazawa T, Gen K, Kitano T. Cortisol Is Involved in Temperature-Dependent Sex Determination in the Japanese Flounder. Endocrinology. (2010) 151:3900–3908. doi: 10.1210/en.2010-0228
40. Mankiewicz JL, Godwin J, Holler BL, Turner PM, Murashige R, Shamey R, et al. Masculinizing effect of background color and cortisol in a flatfish with environmental sex-determination. Integr Comp Biol. (2013) 53:755–65. doi: 10.1093/icb/ict093
41. Haugen T, Almeida FFL, Andersson E, Bogerd J, Male R, Skaar KS, et al. Sex differentiation in Atlantic cod ( Gadus morhua L . ): morphological and gene expression studies. Reprod Biol Endocrinol. (2012) 10:1–13. doi: 10.1186/1477-7827-10-47
42. Poonlaphdecha S, Pepey E, Canonne M, Verdal H De, Baroiller J, Cotta HD. General and comparative endocrinology temperature induced-masculinisation in the nile tilapia causes rapid up-regulation of both dmrt1 and amh expressions. Gen Comp Endocrinol. (2013) 193:234–242. doi: 10.1016/j.ygcen.2013.06.007
43. Fernandino JI, Hattori RS, Kimura H, Strüssmann CA, Somoza GM. Expression profile and estrogenic regulation of anti-müllerian hormone during gonadal development in pejerrey Odontesthes bonariensis, a teleost fish with strong temperature-dependent sex determination. Dev Dyn. (2008) 237:3192–9. doi: 10.1002/dvdy.21731
44. Hattori RS, Fernandino JI, Kishii A, Kimura H, Kinno T, Oura M. Cortisol-induced masculinization: does thermal stress affect gonadal fate in pejerrey , a teleost fish with temperature-dependent sex determination? PLos ONE. (2009) 4:e6548. doi: 10.1371/journal.pone.0006548
45. Kitano T, Yoshinaga N, Shiraishi E, Koyanagi T, Abe SI. Tamoxifen induces masculinization of genetic females and regulates P450 aromatase and Müllerian inhibiting substance mRNA expression in Japanese flounder (Paralichthys olivaceus). Mol Reprod Dev. (2007) 74:1171–7. doi: 10.1002/mrd.20603
46. Vizziano D, Baron D, Randuineau G, Mahè S, Cauty C, Guiguen Y. Rainbow trout gonadal masculinization induced by inhibition of estrogen synthesis is more physiological than masculinization induced by androgen supplementation1. Biol Reprod. (2008) 78:939–46. doi: 10.1095/biolreprod.107.065961
47. Weenen C, Laven JSE, von Bergh ARM, Cranfield M, Groome NP, Visser JA, et al. Anti-müllerian hormone expression pattern in the human ovary: potential implications for initial and cyclic follicle recruitment. Mol Hum Reprod. (2004) 10:77–83. doi: 10.1093/molehr/gah015
48. Durlinger ALL, Kramer P, Karels B, De Jong FH, Uilenbroek JTJ, Anton Grootegoed J, et al. Control of primordial follicle recruitment by anti-mullerian hormone in the mouse ovary. Endocrinology. (1999) 140:5789–5796. doi: 10.1210/endo.140.12.7204
49. Galetti PM, Aguilar CT, Molina WF. An overview of marine fish cytogenetics. Hydrobiologia. (2000) 420:55–62. doi: 10.1023/A:1003977418900
Keywords: sex differentiation, amh, grouper, protogynous, hermaphroditic
Citation: Han Y, Zhao M, Wang L, Yu Z, Wang J, Yu Q, Xiao L, Lu M, Li S, Zhang Y and Lin H (2019) Overexpression of Anti-müllerian Hormone Gene in vivo Affects Gonad Sex Differentiation in Undifferentiated Orange-Spotted Groupers (Epinephelus coioides). Front. Endocrinol. 10:210. doi: 10.3389/fendo.2019.00210
Received: 28 December 2018; Accepted: 15 March 2019;
Published: 05 April 2019.
Edited by:
Vance L. Trudeau, University of Ottawa, CanadaReviewed by:
Juan Ignacio Fernandino, CONICET Institute of Biotechnological Research (IIB-INTECH), ArgentinaDeshou Wang, Southwest University, China
Copyright © 2019 Han, Zhao, Wang, Yu, Wang, Yu, Xiao, Lu, Li, Zhang and Lin. This is an open-access article distributed under the terms of the Creative Commons Attribution License (CC BY). The use, distribution or reproduction in other forums is permitted, provided the original author(s) and the copyright owner(s) are credited and that the original publication in this journal is cited, in accordance with accepted academic practice. No use, distribution or reproduction is permitted which does not comply with these terms.
*Correspondence: Mi Zhao, emhhb20zN0BtYWlsLnN5c3UuZWR1LmNu
Yong Zhang, bHNzenlAbWFpbC5zeXN1LmVkdS5jbg==