- Key Laboratory of Endocrinology, Department of Endocrinology, Translational Medicine Center, Peking Union Medical College, Peking Union Medical College Hospital, Chinese Academy of Medical Sciences, Ministry of Health, Beijing, China
Excessive white adipose tissue (WAT) accumulation due to an imbalance between caloric intake and energy expenditure (EE) characterizes obesity. However, brown adipose tissue (BAT) is highly specialized for the dissipation of energy. Recent evidence indicated that the activation of BAT and the induction of WAT browning might be promising approaches to combat obesity by increasing EE and regulating glucose and lipid metabolism. Resveratrol, which is a polyphenolic compound, has been widely acknowledged to have protective effects against obesity and related metabolic disorders. The induction of WAT browning has been considered as one of the crucial factors in the metabolic benefits of resveratrol. Nevertheless, the specific mechanism that is involved is largely unclear. As a prebiotic-like polyphenol, resveratrol is able to modulate the composition of gut microbiota. In addition, in recent years, the impact of gut microbiota on the browning of WAT has received increasing attention and has been initially confirmed to play a role. By considering all these factors, this review explores the potential link between dietary resveratrol and the browning of WAT, which may be modulated by gut microbiota and their metabolites and proposes the “gut microbiota- adipose tissue” axis plays a vital role in the anti-obesity effects of resveratrol. This observation might provide novel insights and targets that could be used for fighting against obesity and associated metabolic disorders.
Introduction
Obesity has become a worldwide epidemic and has resulted in tremendous economic and social burden. According to the latest data published by the World Health Organization (WHO), the prevalence of global obesity has almost tripled since 1975, and in 2016, more than a third of the adults in the world were overweight or obese (1), which is characterized by abnormal or excessive fat accumulation that might increase the risk of developing a number of disorders, including cardiovascular diseases (2, 3), type 2 diabetes mellitus (T2DM) (4, 5), metabolic syndrome (6, 7), musculoskeletal disorders (8, 9), and some types of cancers (10, 11). An imbalance between caloric intake and energy expenditure is considered as the underlying mechanism of obesity and results in the storage of excess energy in adipose tissue. However, current treatment strategies, such as the modification of the intake and absorption of food and the increase in physical activity, seem to be insufficient to halt the rapid progression of the obesity epidemic (12). Therefore, it is essential to find more effective approaches to fight against obesity and associated cardiometabolic disorders.
White adipose tissue (WAT) is highly specialized for storing lipids and excessively increases its storage capacity during obesity. In contrast, brown adipose tissue (BAT) is an organ that dissipates energy through adaptive thermogenesis (13, 14). Therefore, activating BAT and inducing the conversion of WAT into BAT are considered potential tools to combat obesity by increasing EE and maintaining metabolic homeostasis (15, 16). Although a large number of factors, such as pharmacological and physiological conditions, have been shown to affect the induction of the browning of WAT (17–22), here we will focus on the polyphenolic compound resveratrol. The benefits of resveratrol in obesity and metabolic disorders have been widely acknowledged (23–25). Recent evidence has also suggested that resveratrol supplementation could induce WAT browning and BAT activation (26–28). However, the mechanism by which dietary resveratrol affects adipose tissue remains unclear. In light of the largely hypothetical relationship between resveratrol and gut microbiota (29, 30), and the impact of the gut microbial community on WAT browning (31–33), this review aims to summarize the current literature to explore the association between resveratrol and the browning of WAT through the modulation of the gut microbiota and its metabolites. We propose that resveratrol has an important impact on gut microbiota and the alterations of its metabolites, which in turn influence the regulation of browning and thermogenesis, and this is known as the “gut microbiota-adipose tissue” axis.
Browning of White Adipose Tissue
Since the last century, the concept of WAT browning has gained increasing attention in academic metabolic research (34, 35). Evidence has shown that mammals have two types of adipose tissue, WAT and BAT, both of which possess unique molecular markers, cell properties, morphology, and function (36).
WAT is distributed throughout the body and mainly comprises two types: subcutaneous adipose tissue (SAT), which is located under the skin, and visceral adipose tissue (VAT), which is accumulated inside the abdominal cavity (37). WAT is highly specialized for the long-term storage of energy in the form of triglycerides (TGs) and fat-soluble substances and provides metabolic fuel in the form of fatty acids, which have great potential to buffer energy intake and consumption. Although WAT is the most plastic organ and can expand its buffering capacity by the hyperplasia or hypertrophy of adipocytes, the energy-buffering capacity would be exceeded by a long-term energy overload (38). In modern life, humans are immersed in the consumption of high-calorie food and live a sedentary lifestyle. Excessive energy intake and reduced energy expenditure leads to excess storage capacity in WAT and further results in lipid overflow from the adipose tissue, which accumulates ectopically in other major metabolic organs and ultimately causes the development of obesity and related metabolic disorders.
BAT is another important type of adipose tissue and is mainly composed of brown adipocytes, which are characterized by larger numbers of mitochondria and smaller fat droplets than WAT (14). The function of BAT has been well studied in rodents (39, 40). However, it was traditionally believed that BAT was only present in newborns and was lost after birth in humans. Recently, multiple studies have demonstrated that BAT can be found in supraclavicular, cervical, perivascular and perirenal areas in adult humans (41–43). BAT specializes in burning nutrients to dissipate energy as heat. BAT has crucial functions in adaptive thermogenesis, which is a significant component of whole-body EE, and is related to cold exposure and food intake (44). Uncoupling protein 1 (UCP1) is uniquely expressed in BAT and is responsible for inducing the adaptive thermogenesis through the uncoupling of mitochondrial oxidation of substrates from ATP production. Thus, the expression of UCP1 is the primary feature of BAT (45). Regulatory factors other than UCP1, including norepinephrine, members of the peroxisome proliferator-activated receptor family (PPAR) (46, 47), PPARγ coactivator 1 alpha (PGC1α) (45), and PRD1-BF-1-RIZ1 homologous domain protein containing protein 16 (PRDM16) (48), also play vital roles in thermogenesis and the development and differentiation of BAT. In light of the important role of EE, BAT has received considerable attention in the treatment of obesity.
Recently, a third type of adipocytes in WAT was identified, known as the beige or brite adipocytes, which has a similar phenotype to brown adipocytes; it has thermogenic features, is rich in mitochondria and expresses high levels of UCP1 and other factors that contribute to thermogenesis (e.g., PPAR, PGC1α, and PRDM16) (49, 50). The presence of brown-like adipocytes in WAT is known as WAT browning (35). In addition to increased EE, BAT, and WAT browning might exert beneficial effects on whole-body metabolism via other pathways, including increasing glucose and lipid clearance and increasing insulin sensitivity by mitigating glucolipotoxicity (51). A current animal study indicated that hyperoxia could improve glucose and lipid metabolism through browning of WAT (52). Some other evidence also showed the association between WAT browning and the improvement in glucose homeostasis, insulin sensitivity as well as lipid mobilization (17, 53, 54). Therefore, the activation of BAT and the induction of WAT browning are promising strategies for fighting against obesity and related metabolic disorders. Other than several pharmacological agents and physiological conditions, such as norepinephrine (55, 56), β3-adrenergic receptor agonist (57), exercise (21, 58), and cold exposure (59, 60), that have been shown to activate BAT and induce WAT browning, evidence has indicated that bioactive food ingredients are also key BAT activators and WAT browning inducers that have protective effects against obesity, T2DM and metabolic syndrome (61, 62).
Dietary Resveratrol, Obesity, and WAT Browning
Resveratrol and Its Role in Obesity and Related Metabolic Disorders
In recent years, bioactive dietary compounds have received increasing attention in various fields (63, 64). Resveratrol (trans-3,5,4′-trihydroxystilbene) is a natural polyphenol found mainly in plants and plant-derived products, such as Polygonum cuspidatum, fruits (including grapes and berries), peanuts and red wine (65, 66). It is estimated that nearly 75% of resveratrol is absorbed after its oral consumption in humans. However, most of it is metabolized in the intestine and liver and the major metabolites are glucuronides and sulfates of resveratrol. Thus, the free form of resveratrol is found at very low levels in serum, thereby decreasing its bioavailability (30). Clinical trials and animal experiments have shown that resveratrol has numbers of health benefits, including the prevention of cancer (67), aging (68), obesity (69), diabetes mellitus (70), and cardiovascular disease (71). Anti-inflammatory and antioxidant activity, improvement in mitochondrial function, and the inhibition of apoptosis, are all considered potential effects of resveratrol (72). This review focuses on the studies that have investigated the potential protective effects of resveratrol against obesity and obesity-related metabolic disorders in animal models and human beings.
Evidence From Experimental Animal Models
Evidence from rodents has demonstrated that resveratrol exerts beneficial effects on obesity and related metabolic disturbances (summarized in Table 1). To the best of our knowledge, the anti-obesity effects of resveratrol were first explored in mice in 2006. The research group (73) fed C57BL/6 mice a standard or high-fat diet with or without resveratrol. It was found that body weight and the number of deposits of inguinal, epididymal, and retroperitoneal WAT were significantly reduced in mice that were fed a high-fat diet with resveratrol (200 or 400 mg/kg/day) for 15 weeks. At approximately the same time, another publication (74) demonstrated that resveratrol intake (0.4% in the diet) for 6 months could dramatically improve survival and insulin sensitivity in high-fat diet fed mice, while still being independent of a significant reduction in body weight. Subsequently, numerous beneficial effects of resveratrol, including the reduction of body weight and WAT content and the regulation of lipid metabolism, were also revealed and confirmed in mice and rats fed a high-fat diet (76, 78, 86). However, some experiments showed that body weight was not decreased after resveratrol intake. One study (83) treated C57BL/6J male mice that were fed a high-fat diet for only 3 weeks with oral resveratrol (30 mg/kg/day) and did not observe a significant decrease in body weight or fat mass. The short intervention time might be the main explanation for this. In another study (84), supplementation of a high-fat diet with 0.2 and 0.4% resveratrol for 15 weeks did not reduce body or fat weight in mice. However, similar doses and treatment duration were both identified to have the positive effects in other studies (73, 75). In addition, resveratrol supplementation appeared to have no effect on reducing body weight gain and fat content in rats fed an obesogenic diet (15 mg/kg/day for 6 weeks), which indicated that this dosage was ineffective under this nutritional condition (93).
Other than high-fat diets, resveratrol consumption under several other conditions also showed anti-obesity effects. Effects of resveratrol intake (4 g/kg diet) were detected in FVB/N mice fed a high protein diet, where dramatically decreased body weight and adipose tissue weight were shown (94). The same effects were also confirmed in homozygous apoE-deficient mice fed an atherogenic diet with 0.02% resveratrol for 12 weeks (77), obese Zucker rats fed 15 mg/kg/day for 6 weeks or 10 mg/kg/day for 8 weeks (88) and Otsuka Long-Evans Tokushima fatty (OLETF) rats fed a standard diet with 0.5% resveratrol for 4 weeks (90).
Evidence From Clinical Studies in Humans
In comparison with animal models, only limited evidence from humans has shown the anti-obesity effects of resveratrol (summarized in Table 2). A randomized, double-blind, placebo-controlled clinical trial analyzed the effects of resveratrol administration (500 mg 3 times per day) for 90 days in 24 patients with metabolic syndrome (95). The results showed that total weight, body mass index (BMI), fat mass, and waist circumference (WC) were all significantly decreased after the intervention, compared with those of the placebo group. Around the same time, another clinical trial detected the effects of resveratrol supplementation (500 mg/day), along with lifestyle intervention in 50 overweight non-alcoholic fatty liver disease (NAFLD) patients for 12 weeks and found significant improvements in weight, BMI, WC, and hepatic steatosis, compared with those of the placebo control group (96). Subsequently, the long-term effects of polyphenols on metabolic health were analyzed (97). Thirty-eight overweight or obese subjects received resveratrol and epigallocatechin-3-gallate supplements (80 and 282 mg/day, respectively) or a placebo for 12 weeks. The VAT mass tended to be decreased in the intervention group compared with the placebo group (p = 0.09). Another study also demonstrated that consumption of an orlistat-resveratrol combination for 6 months could dramatically decrease BMI, WC, fat mass, and triglycerides levels in obese subjects compared with those in placebo group subjects (98).
Some other clinical trials did not observe the positive effects of resveratrol administration on body weight or fat mass, but they did observe some other metabolic benefits. Two published studies both analyzed the effects of resveratrol administration (150 mg/day for 4 weeks) in healthy obese subjects (99, 100). Although there were no alterations in body weight and fat mass, they found that resveratrol intake could reduce the sleeping metabolic rate and increase the proportion of small adipocytes. Another study also detected the influence of resveratrol consumption on healthy obese subjects, but they used a higher dosage of 1,500 mg/day for 4 weeks (101). The results showed that there were nearly no significant differences in fat mass or energy expenditure. Thus, this dosage might be too high to exert its beneficial effects on metabolism. In addition, several trials evaluated the effects of resveratrol consumption (3 g/day for 8 weeks or 1.5 g/day for 6 months) in subjects with NAFLD and showed that resveratrol intervention had almost no positive effects on the alleviation of clinical or histological NAFLD, and only a small improvement in liver fat accumulation was shown (102, 103). However, a study in which 29 non-obese, postmenopausal women with normal glucose tolerance were administered resveratrol supplementation (75 mg/day) or placebo for 12 weeks and found no changes in body and fat weight or gene expression in WAT (104). Most of the positive effects of resveratrol were observed in obese animals fed an obesogenic diet. Thus, the results of these studies might be due to the fact that they were conducted in non-obese subjects.
Overall, evidence from both experimental animal models and clinical trials in humans confirmed the benefits of dietary resveratrol intake on obesity and related metabolic disorders. Although studies have shown that several metabolic pathways, such as adipogenesis, lipogenesis, apoptosis, lipolysis, thermogenesis, and fatty acid oxidation, might be effective targets for resveratrol, the specific mechanism that explains its effect is not completely understood (69). In light of the important role of WAT browning in the prevention and treatment of obesity (105) and the dramatic anti-obesity effects of resveratrol, this review mainly discusses the mechanisms involved in BAT thermogenesis and WAT browning.
Resveratrol and WAT Browning
A number of studies have suggested the significant role of resveratrol consumption in the improvement of BAT thermogenesis and WAT browning and its effects on obesity and metabolic health. In addition to observing significant decreases in body and fat weight after 12 weeks of resveratrol intake (400 mg/kg/day), a research group also observed decreases in adipocyte size in WAT and increases in cold-induced thermogenesis, mitochondrial size and the expression of UCP1 and PGC1α in BAT, which indicated a dramatic increase in BAT thermogenesis in mice (73). Another research group treated mice fed a standard diet with resveratrol (400 mg/kg/day) for 8 weeks and found a significant increase in the expression levels of UCP1, SIRT1 (sirtuins 1), and BMP-7 in BAT (106). The induction of WAT browning and a significant increase in expression of genes encoding WAT browning biomarkers in WAT, including UCP1, PGC-1α, and PRDM16, were also identified in mice receiving a high-fat diet with 0.1% resveratrol (26). Similar results were observed in inguinal WAT-derived stromal vascular cells after treatment by resveratrol in vitro (107).Similarly, a rat model also demonstrated that resveratrol intake (30 mg/kg/day for 6 weeks) in high-fat diet-fed rats significantly upregulated the expression of PPARβ/δ, SIRT1 and PGC-1α, and UCP1 protein levels in BAT (87). Another animal study showed that supplementation with resveratrol during pregnancy and lactation could result in increased energy expenditure, insulin sensitivity, enhanced brown adipose function, and WAT browning, in male offspring challenged by a high-fat diet (27). A combination of resveratrol and quercetin has been identified to induce a browning-like remodeling effect in perirenal WAT and to increase the expression of UCP1 protein in interscapular BAT (108).
The molecular mechanism involved in the BAT thermogenesis and WAT browning induced by resveratrol was also explored. In 2006, an animal experiment (73) first showed that the resveratrol intake significantly decreased the PGC-1α acetylation and increased its activity, which was consistent with a dramatic increase in SIRT1, which is an important deacetylase. In addition, the effects of resveratrol on BAT were lost in SIRT1−/− MEFs. This finding was confirmed in 2012 with the observation that the induction of WAT browning by resveratrol was dependent on SIRT1, which deacetylated the PPARγ and led to the recruitment of the BAT program coactivator PRDM16 (109). In addition, studies demonstrated that resveratrol induced WAT browning through AMP-activated protein kinase (AMPK) α1 (26, 110). Activation of AMPK by resveratrol stimulated mitochondrial biogenesis through SIRT1. The above study showed that resveratrol can induce SIRT1 to further deacetylate PGC1α, which directly regulates the mitochondrial biogenesis and oxidative phosphorylation. Thus, the AMPK-SIRT1- PGC1α pathway might be the key to the induction of WAT browning by resveratrol, which was confirmed again by the resistance to the effects of resveratrol in AMPK-deficient mice (110). In addition, another potential mechanism that mediated the benefits of resveratrol on beige adipocytes was the direct activation of cAMP, which further activates the AMPK pathway. Increased cAMP concentrations in WAT after the oral administration of resveratrol further verified this finding (111). However, the specific mechanism and the direct effects of the activation of the cAMP pathway by resveratrol on WAT browning are still unclear.
A large body of evidence has indicated that resveratrol consumption could induce BAT activation and WAT browning, possibly through the AMPK-SIRT1- PGC1α pathway or the cAMP signaling pathway, and could also have significant benefits for the control of body weight and the improvement of metabolic disorders. However, similar evidence from studies in humans is lacking, and there is a need for more exploration. In addition, the understanding of the specific mechanism by which dietary resveratrol regulates adipose tissue is limited. In light of the important role of resveratrol in the gut microbiota in terms of weight control (112) and the cross-talk between gut microbiota and adipose tissue (113, 114), we further explored whether modifying the gut microbiota via supplementation with resveratrol might promote the BAT thermogenesis and WAT browning.
Resveratrol, gut Microbiota, and WAT Browning
Resveratrol and gut Microbiota
The gut microbiota, which is a community of 1,000 or more species of bacteria, that contains 10 times the number of cells in a human and colonizes the gastrointestinal tract, has recently become one of the most popular topics in biomedical research. It has multiple functions in the body, such as absorbing fats and fat-soluble vitamins, digesting complex carbohydrates and plant polysaccharides into short-chain fatty acids (SCFAs), and participating in bile acid-related metabolism. The commensal microbiota in the intestine is in a balanced symbiosis with the host in a healthy host and plays crucial roles in host health (115, 116). During the last few decades, emerging research has focused on the role of the gut microbiota in metabolic diseases and has shown that gut microbiota dysbiosis is intertwined with various disorders, such as obesity (112), glucose intolerance (117), insulin sensitivity (118), and disturbances in serum lipid profiles (119).
Resveratrol, a polyphenolic compound with prebiotic properties, can be metabolized by gut microbiota to produce metabolites, such as dihydroresveratrol and lunularin (30). Interestingly, numerous studies have shown that resveratrol has the potential to modulate the composition of gut microbiota, and this is related to its anti-obesity effects and its improvement of metabolic effects. Effects of resveratrol on gut microbiota were first detected in an obese mouse model induced by a high-fat diet and the results showed that resveratrol intake (200 mg/kg/day) significantly improved the gut microbial dysbiosis in high-fat diet group and decreased body and fat weight (78). The Bacteroidetes/Firmicutes ratios, which is decreased during the development of obesity, and the abundances of Lactobacillus and Bifidobacterium, were significantly increased, whereas, the abundance of Enterococcus faecalis was decreased after resveratrol intervention. Subsequently, another study also showed that the Bacteroidetes/Firmicutes ratio was significantly increased in resveratrol-treated (450 mg/kg/day for 2 weeks) mice with a high-fat diet (120). In addition, increases in the abundances of Parabacteroides, Bilophila, and Akkermansia and a decrease in the relative abundance of Lachnospiraceae were observed in high-fat diet fed mice with resveratrol. A significant increase in the relative abundances of Bacteroides, Lactobacillus, Bifidobacterium, and Akkermansia and a decrease in the Prevotella, Ruminococcaceae, Anaerotruncus, Alistipes, Helicobacter, and Peptococcaceae were also identified in mice with trimethylamine-N-oxide (TMAO)-induced atherosclerosis treated with resveratrol (400 mg/kg/day) (121). Subsequently, several experimental animal studies confirmed that resveratrol might regulate body weight and metabolism via modifying gut microbiota (summarized in Table 3). In one study, the results showed that resveratrol intake (0.4%) influenced the gut microbial composition only in obese mice and not in standard diet-fed mice, which was consistent with the positive effects of resveratrol on body weight and metabolism observed in obese animals fed an obesogenic diet (122). However, the evidence from clinical trials in humans is still limited.
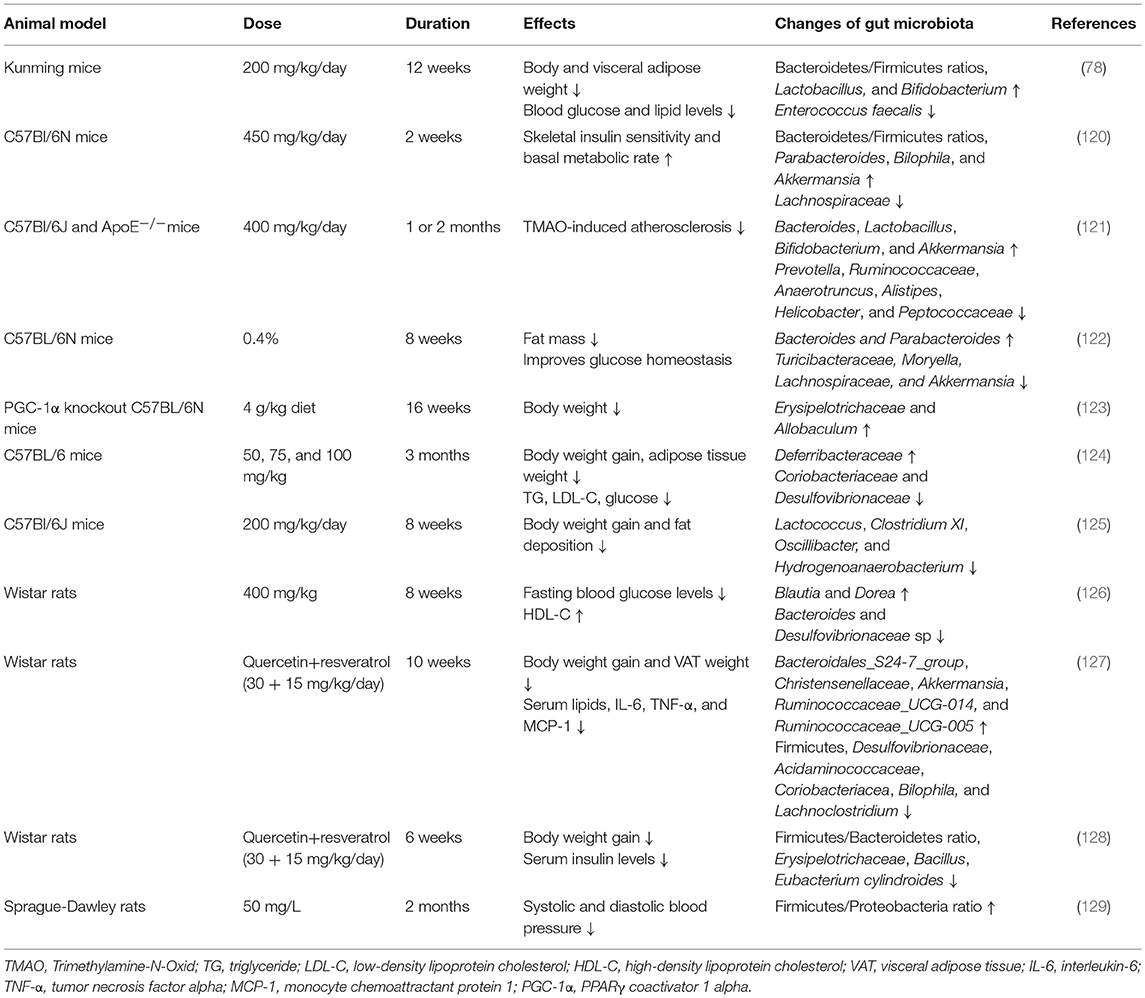
Table 3. Experimental animal studies analyzing the effects of resveratrol supplementation on gut microbiota composition.
Fecal transplantation experiments further confirmed the important role of resveratrol-mediated alterations in gut microbiota for the benefits of resveratrol. After discovering that resveratrol supplementation led to changes in the gut microbiota, a research group further explored the causal relationship between the gut microbiota and metabolism through transplanting fecal slurry from healthy resveratrol-fed donor mice to obese mice (122). The results showed that the fecal recipient mice displayed significant improvement in glucose homeostasis, which was independent of the reduction in body weight. The bacterial sequencing analysis showed that the changes in the microbial communities were similar to those observed in the donors. Thus, it is possible that alterations in the gut microbiota mediate the beneficial metabolic effects of resveratrol. Subsequently, effects of fecal microbiome transplants (FMTs) from resveratrol-fed donors (0.4%), for only 2 weeks on the metabolic parameters in recipient mice, were detected (130). Although no changes were identified in body weight and glucose metabolism in the donor mice, the resveratrol-FMTs were sufficient to improve glucose intolerance in the recipient obese mice, also independently of the changes in body weight. In addition, to determine whether live microbes were necessary for the beneficial effects, they measured the effects of heat-killed (HK) FMTs in obese mice. Interestingly, the results showed that HK slurry was also sufficient for the improvement in glucose homeostasis, which indicated that the metabolites or other components in the resveratrol-FMTs were the key factors regulating the metabolic tissues. Recently, another research group (131) transplanted the microbiota from donors treated with a resveratrol (300 mg/kg/day) diet for 16 weeks to the high-fat diet-fed mice, and found that the recipient mice had decreased body weight and improved insulin resistance. In addition, resveratrol-FMTs could induce WAT browning and BAT activation in high-fat diet-fed mice. Therefore, gut microbiota, especially their metabolites, might be a crucial factor in mediating the protective effects of resveratrol against obesity and metabolic disorders, and the modulation of WAT browning by gut microbiota may play an important role.
The Effects of gut Microbiota on WAT Browning
Recently, several studies have shown the relationship between gut microbiota and WAT browning. It is reported that germ-free mice show stimulation of BAT lipolysis and inhibition of lipogenesis (132). Therefore, they first proposed that gut microbiota could regulate BAT metabolism. A later study analyzed the role of gut microbiota in the energy homeostasis remodeling by cold exposure, which is a well-known BAT activator (133). First, cold exposure significantly changed the composition of the gut microbiota. Then, transplanting gut microbiota from cold-exposed mice to germ-free mice dramatically increased the expression of UCP1 in BAT, increased the number of UCP1-positive cells in inguinal and perigonadal WAT, and improved insulin sensitivity. Therefore, this study indicated that FMTs from cold-exposed mice increased the energy expenditure and induced the browning of WAT. Similarly, in another animal study (134), the exposure of mice to 12°C for 12 weeks could mitigate diet-induced obesity, and this was related to the increased expression of UCP1 in BAT, which was accompanied by significant increases in the abundance of Adlercreutzia, Mogibacteriaceae, Ruminococcaceae, and Desulfovibrio and decreases in the abundance of Bacilli, Erysipelotrichaceae, and the genus rc4-4. Moreover, they transplanted microbiota from donors kept at 12 or 29°C and found that germ-free mice fed a high-fat diet gained less fat mass and showed significantly increased expression of UCP1 mRNA and protein in BAT when colonized with microbiota from donors kept at 12°C instead of donors kept at 29°C. In addition to cold exposure model, intermittent fasting also significantly stimulated the beiging of WAT and improved obesity and insulin sensitivity, and it also produced a shift in the gut microbial composition. Transplantation of fecal microbiota to microbiota-depleted mice induced the browning of WAT while microbiota-depleted mice were resistant to the intermittent fasting-induced browning (32). In addition, the association between the gut microbial community and thermogenesis was also confirmed in a β-klotho KO mouse model, which is naturally resistant to diet-induced obesity due to the stimulation of energy expenditure and BAT thermogenesis (135). In addition, in mice subject to the depletion of gut microbiota by antibiotic treatment and in germ-free mice, the thermogenic capacity of BAT was impaired, and WAT browning was reduced (136). However, only one human study analyzed the relationship between gut microbiota composition and gene markers of browning in SAT and VAT. The results showed that the relative abundance of Firmicutes was positively associated with markers of WAT browning (PRDM16 and UCP1) in SAT (114). These findings all demonstrate the significant role of gut microbiota in BAT browning.
The effects of dietary gypenosides and glucoraphanin on energy metabolism were associated with increased WAT browning and BAT activity, which were accompanied by changes in gut microbiota (137, 138). Polyphenol-rich postfermented pu-erh tea could significantly improve glucose and lipid metabolism, and induced the browning of WAT in high-fat diet fed mice, which was associated with changes in the abundance of Akkermansia muciniphila (139). The evidence regarding the role of crosstalk between gut microbiota and WAT browning in the anti-obesity effects of resveratrol is limited. Recently, a study treated high-fat diet fed mice with 0.4% resveratrol for 4 weeks and found that resveratrol could significantly mitigate fat accumulation, promote WAT browning and modulate gut microbiota dysbiosis in high-fat diet-fed mice (28). They then transplanted the feces from these mice into recipient mice and showed that resveratrol-FMTs induced the browning of WAT, in which the SIRT1 signaling pathway might be a key factor. Therefore, this study demonstrated that resveratrol induced WAT browning through modifying gut microbiota.
In light of the crucial role of gut microbiota in mediating the metabolic benefits of resveratrol and the crosstalk between the gut microbial community and WAT browning, we proposed that the “gut microbiota-adipose tissue” axis might be the key to elucidating the anti-obesity effects of dietary resveratrol. Although the link between gut microbiota and WAT browning is unclear, it has been demonstrated that gut microbial metabolites might be a crucial mediator.
Metabolites Might be a Crucial Mediator Between gut Microbiota and WAT Browning That Plays a Role in the Metabolic Benefits of Resveratrol
SCFAs
Numerous studies have shown that microbial metabolites might play an important role in the crosstalk between gut microbiota and other metabolic organs, especially the SCFAs (140–144). The SCFAs, which are monocarboxylic acids containing 2–6 carbons, are generated from the degradation of non-digestible carbohydrates by specific bacteria. The major SCFAs in serum and the caecum included acetate, propionate and butyrate, which have been demonstrated to play important roles in metabolic health (145–147), including the induction of WAT browning and the activation of BAT thermogenesis (148, 149). Evidence has shown that the direct supplementation of acetate and butyrate to high-fat diet-fed mice could significantly increase the expression of UCP1 and PGC1α in WAT and BAT (150). In addition, intermittent fasting changed the composition of the gut microbial community, resulting in an increase in the fermentation products acetate and lactate, which could selectively upregulate the expression of monocarboxylate transporter 1 in beige cells and promote WAT browning (32). These effects disappeared in microbiota-depleted mice. Another study also showed that the browning process of WAT was significantly reduced by antibiotic treatment or in germ-free mice, whereas gavage of the butyrate reversed the deleterious effects (136). Thus, the SCFAs were shown to be crucial factors in mediating the effects of gut microbiota on WAT browning. A study in humans showed that plasma acetate levels were positively related to the relative abundance of Firmicutes and were also associated with PRDM16 mRNA levels in SAT (114). An animal study (131) demonstrated that resveratrol significantly increased the relative abundances of Bacteroidetes, Blautia, Ruminococcus, and Parabacteroides, which have been reported to be SCFA producers. Transplanting the resveratrol-microbiota to high-fat diet-fed mice induced the development of beige adipocytes in WAT, which was accompanied by improved metabolism. In addition, another animal study (130) quantified SCFAs in the fecal matter of the resveratrol fed mice and did not observe significant changes in the levels of major SCFAs, such as butyrate. However, they found an increased concentration the SCFA-4-hy-droxyphenylacetate in the feces, which has been shown to be involved in improving insulin sensitivity and to play a role in WAT browning (151). Therefore, the microbiota derived SCFAs might be vital factors that mediate the anti-obesity benefits of resveratrol in gut microbiota and WAT browning.
Other Metabolites
In addition to the SCFAs, other metabolites may play a role. Anthocyanins, which were another type of classical polyphenol, have been confirmed to have significant anti-obesity effects; they cannot be absorbed directly but are catabolized by gut microbiota. Vanillic acid (VA) is one of the major metabolites of anthocyanins produced by microbiota. A recent study has shown that VA could reduce body weight, promote the browning of WAT and activate BAT thermogenesis in high-fat diet fed mice (152). KetoA (10-oxo-12(Z)-octadecenoic acid), which is a metabolite of linoleic acid produced by lactic acid bacteria in the intestine, has been shown to mitigate the obesity-related metabolic disturbances and upregulate the expression of UCP1 in WAT via the activation of transient receptor potential vanilloid 1 (TRPV1) (153). Resveratrol, which is a prebiotic-like polyphenol, can be catabolized by gut microbiota into various metabolites, such as dihydroresveratrol and lunularin. This study has indicated that metabolites or other components in the feces of mice given resveratrol supplementation, were sufficient to improve metabolism and induce WAT browning (130). However, the specific metabolites or other components that are involved are still unclear. The evidence for resveratrol-derived metabolites also being crucial mediators of the “gut microbiota-adipose tissue” axis is lacking, and this topic needs further exploration.
Overall, metabolites are critical mediators between gut microbiota and WAT browning and generate the “gut microbiota-adipose tissue” axis, which plays a critical role in the anti-obesity benefits of resveratrol. However, most of the current evidence is from experimental animal models. In the future, more evidence from studies in humans will be required to verify this finding.
Conclusion
Obesity has become an epidemic worldwide and has presented unprecedented challenges (154). However, the approaches used to fight against obesity and related metabolic disorders seem to be limited. Excessive energy intake and decreased energy expenditure is the main cause of obesity. BAT is highly specialized to dissipate energy as heat, which is an important component of EE. Thus, activating BAT and inducing the browning of WAT to increase EE and maintain metabolic homeostasis might be a powerful means of preventing and treating obesity (34, 35). Resveratrol, which is a polyphenolic compound, has been widely accepted as an anti-obesity agent and a metabolic effector (69, 81, 155, 156). A multitude of evidence has demonstrated that stimulating WAT browning through the AMPK-SIRT1-PGC1α pathway or the cAMP signaling pathway is one of the critical mechanisms that is utilized by resveratrol to combat obesity (157, 158). In addition, resveratrol has been confirmed to have prebiotic properties and was not only metabolized by gut microbiota but also influenced the composition of the gut microbial community (30, 128). The significant role of gut microbiota in inducing WAT browning has also been well established through correlation analysis, fecal transplantation experiments and microbiota-depleted animal models (130, 136, 139). Microbiota-derived metabolites, especially the SCFAs, might be the key to mediating the crosstalk between gut microbiota and WAT browning (130). Therefore, we conclude that resveratrol induces the browning of WAT through modulating the composition of gut microbiota and their metabolites, which play a vital role in anti-obesity effects. In other words, the “gut microbiota-adipose tissue” axis might be the key to elucidating the anti-obesity benefits of resveratrol (summarized in Figure 1).
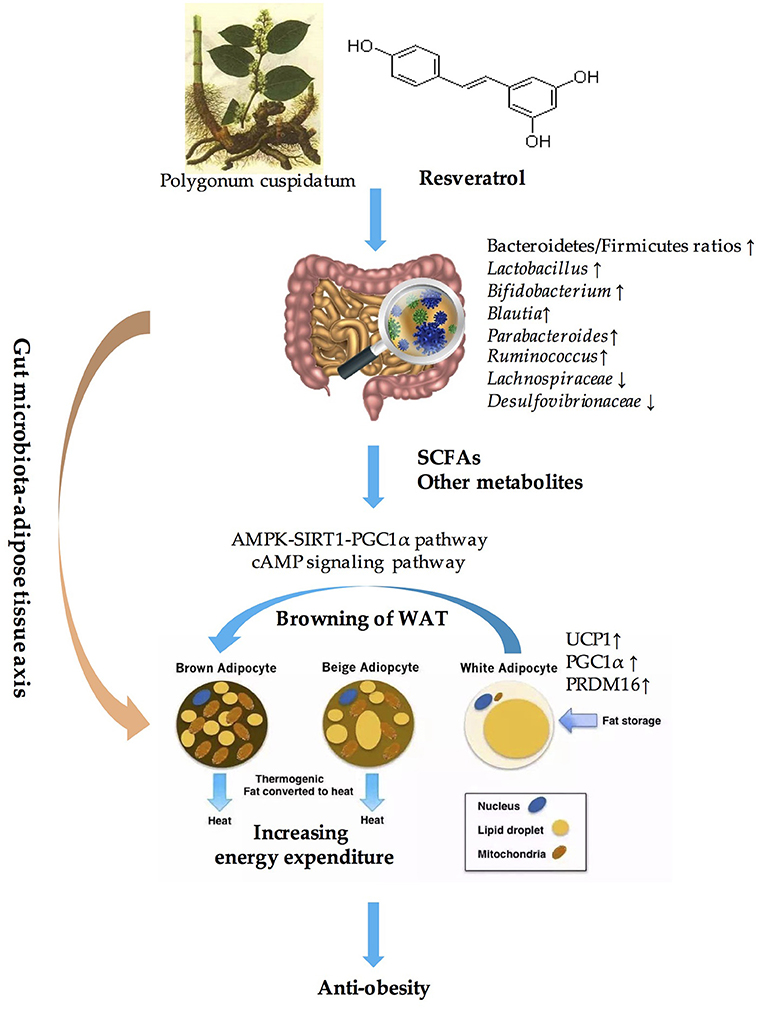
Figure 1. Overview of the role of “Gut microbiota-adipose tissue axis” in the anti-obesity benefits of resveratrol. SCFAs, short-chain fatty acids; AMPK, AMP-activated protein kinase; SIRT1, sirtuin1; UCP1, uncouple protein 1; PGC1α, PPARγ coactivator 1 alpha; PRDM16, PRD1-BF-1-RIZ1 homologous domain protein containing protein 16; WAT, white adipose tissue.
However, most of the current evidence is from experimental animal models. Because of the enormous differences between humans and animals, especially in terms of the dosages of resveratrol required, more clinical trials are warranted to confirm these findings, as well as the bioactivity and safety of these molecules. In addition, it is necessary to clarify the specific mechanisms involved in the “gut microbiota-adipose tissue” axis that prevent and treat obesity. In this review, we present a new pathway involved in the anti-obesity effects of resveratrol based on the current literature, which provides some potential ideas and targets for the treatment of obesity.
Author Contributions
LZ: writing-original draft preparation. XX, QZ, JZ, and MD: writing-review and editing. XX: supervision. XX and QZ: funding acquisition.
Funding
This work was funded by the grants from National Natural Science Foundation of China (Nos. 81170736, 81570715, 81870579, 81870545).
Conflict of Interest Statement
The authors declare that the research was conducted in the absence of any commercial or financial relationships that could be construed as a potential conflict of interest.
References
1. World Health Organization. Obesity and Overweight. (2018). Available online at: http://www.who.int/mediacentre/factsheets/fs311/en/
2. Kachur S, Lavie CJ, De Schutter A, Milani RV, Ventura HO. Obesity and cardiovascular diseases. Minerva Med. (2017) 108:212–28. doi: 10.23736/S0026-4806.17.05022-4
3. Parto P, Lavie CJ. Obesity and cardiovascular diseases. Curr Probl Cardiol. (2017) 42:376–94. doi: 10.1016/j.cpcardiol.2017.04.004
4. Eckel RH, Kahn SE, Ferrannini E, Goldfine AB, Nathan DM, Schwartz MW, et al. Obesity and type 2 diabetes: what can be unified and what needs to be individualized? J Clin Endocrinol Metab. (2011) 96:1654–63. doi: 10.1210/jc.2011-0585
5. Verma S, Hussain ME. Obesity and diabetes: an update. Diabetes Metab Syndr. (2017) 11:73–9. doi: 10.1016/j.dsx.2016.06.017
6. Andersen CJ, Murphy KE, Fernandez ML. Impact of obesity and metabolic syndrome on immunity. Adv Nutr. (2016) 7:66–75. doi: 10.3945/an.115.010207
7. Engin A. The definition and prevalence of obesity and metabolic syndrome. Adv Exp Med Biol. (2017) 960:1–17. doi: 10.1007/978-3-319-48382-5_1
8. Jordani PC, Campi LB, Circeli GZ, Visscher CM, Bigal ME, Goncalves DA. Obesity as a risk factor for temporomandibular disorders. J Oral Rehabil. (2017) 44:1–8. doi: 10.1111/joor.12453
9. Shultz SP, Ambrose KR. “Where Does it Hurt?” Implications of obesity on musculoskeletal health. N C Med J. (2017) 78:326–31. doi: 10.18043/ncm.78.5.326
10. Font-Burgada J, Sun B, Karin M. Obesity and cancer: the oil that feeds the flame. Cell Metab. (2016) 23:48–62. doi: 10.1016/j.cmet.2015.12.015
11. Ackerman SE, Blackburn OA, Marchildon F, Cohen P. Insights into the link between obesity and cancer. Curr Obes Rep. (2017) 6:195–203. doi: 10.1007/s13679-017-0263-x
12. Astrup A, Lundsgaard C. What do pharmacological approaches to obesity management offer? Linking pharmacological mechanisms of obesity management agents to clinical practice. Exp Clin Endocrinol Diabetes. (1998) 106(Suppl. 2):29–34. doi: 10.1055/s-0029-1212034
13. Cypess AM, Lehman S, Williams G, Tal I, Rodman D, Goldfine AB, et al. Identification and importance of brown adipose tissue in adult humans. N Engl J Med. (2009) 360:1509–17. doi: 10.1056/NEJMoa0810780
14. Oelkrug R, Polymeropoulos ET, Jastroch M. Brown adipose tissue: physiological function and evolutionary significance. J Comp Physiol B. (2015) 185:587–606. doi: 10.1007/s00360-015-0907-7
15. Cui XB, Chen SY. White adipose tissue browning and obesity. J Biomed Res. (2016) 31:1–2. doi: 10.7555/JBR.31.20160101
16. Jankovic A, Otasevic V, Stancic A, Buzadzic B, Korac A, Korac B. Physiological regulation and metabolic role of browning in white adipose tissue. Horm Mol Biol Clin Investig. (2017) 31. doi: 10.1515/hmbci-2017-0034
17. Choi SS, Kim ES, Jung JE, Marciano DP, Jo A, Koo JY, et al. PPARgamma antagonist gleevec improves insulin sensitivity and promotes the browning of white adipose tissue. Diabetes. (2016) 65:829–39. doi: 10.2337/db15-1382
18. Carino A, Cipriani S, Marchiano S, Biagioli M, Santorelli C, Donini A, et al. BAR502, a dual FXR and GPBAR1 agonist, promotes browning of white adipose tissue and reverses liver steatosis and fibrosis. Sci Rep. (2017) 7:42801. doi: 10.1038/srep42801
19. Weiner J, Hankir M, Heiker JT, Fenske W, Krause K. Thyroid hormones and browning of adipose tissue. Mol Cell Endocrinol. (2017) 458:156–9. doi: 10.1016/j.mce.2017.01.011
20. Yao L, Cui X, Chen Q, Yang X, Fang F, Zhang J, et al. Cold-inducible SIRT6 regulates Thermogenesis of brown and beige fat. Cell Rep. (2017) 20:641–54. doi: 10.1016/j.celrep.2017.06.069
21. Aldiss P, Betts J, Sale C, Pope M, Budge H, Symonds ME. Exercise-induced ‘browning' of adipose tissues. Metabolism. (2018) 81:63–70. doi: 10.1016/j.metabol.2017.11.009
22. Paschos GK, Tang SY, Theken KN, Li X, Verginadis I, Lekkas D, et al. Cold-induced browning of inguinal white adipose tissue is independent of adipose tissue cyclooxygenase-2. Cell Rep. (2018) 24:809–14. doi: 10.1016/j.celrep.2018.06.082
23. Fernandez-Quintela A, Carpene C, Fernandez M, Aguirre L, Milton-Laskibar I, Contreras J, et al. Anti-obesity effects of resveratrol: comparison between animal models and humans. J Physiol Biochem. (2016) 73:417–29. doi: 10.1007/s13105-016-0544-y
24. Fernandez-Quintela A, Milton-Laskibar I, Gonzalez M, Portillo MP. Antiobesity effects of resveratrol: which tissues are involved? Ann N Y Acad Sci. (2017) 1403:118–31. doi: 10.1111/nyas.13413
25. Springer M, Moco S. Resveratrol and its human metabolites-effects on metabolic health and obesity. Nutrients. (2019) 11:E143. doi: 10.3390/nu11010143
26. Wang S, Liang X, Yang Q, Fu X, Rogers CJ, Zhu M, et al. Resveratrol induces brown-like adipocyte formation in white fat through activation of AMP-activated protein kinase (AMPK) alpha1. Int J Obes. (2015) 39:967–76. doi: 10.1038/ijo.2015.23
27. Zou T, Chen D, Yang Q, Wang B, Zhu MJ, Nathanielsz PW, et al. Resveratrol supplementation of high-fat diet-fed pregnant mice promotes brown and beige adipocyte development and prevents obesity in male offspring. J Physiol. (2017) 595:1547–62. doi: 10.1113/JP273478
28. Liao W, Yin X, Li Q, Zhang H, Liu Z, Zheng X, et al. Resveratrol-induced white adipose tissue browning in obese mice by remodeling fecal microbiota. Molecules. (2018) 23:E3356. doi: 10.3390/molecules23123356
29. Bird JK, Raederstorff D, Weber P, Steinert RE. Cardiovascular and antiobesity effects of resveratrol mediated through the gut microbiota. Adv Nutr. (2017) 8:839–49. doi: 10.3945/an.117.016568
30. Chaplin A, Carpene C, Mercader J. Resveratrol, metabolic syndrome, and gut microbiota. Nutrients. (2018) 10:E1651. doi: 10.3390/nu10111651
31. Geurts L, Everard A, Van Hul M, Essaghir A, Duparc T, Matamoros S, et al. Adipose tissue NAPE-PLD controls fat mass development by altering the browning process and gut microbiota. Nat Commun. (2015) 6:6495. doi: 10.1038/ncomms7495
32. Li G, Xie C, Lu S, Nichols RG, Tian Y, Li L, et al. Intermittent fasting promotes white adipose browning and decreases obesity by shaping the gut microbiota. Cell Metab. (2017) 26:672–85.e674. doi: 10.1016/j.cmet.2017.08.019
33. Reynes B, Palou M, Rodriguez AM, Palou A. Regulation of adaptive thermogenesis and browning by prebiotics and postbiotics. Front Physiol. (2018) 9:1908. doi: 10.3389/fphys.2018.01908
34. Bargut TCL, Souza-Mello V, Aguila MB, Mandarim-De-Lacerda CA. Browning of white adipose tissue: lessons from experimental models. Horm Mol Biol Clin Investig. (2017) 31. doi: 10.1515/hmbci-2016-0051
35. Jeremic N, Chaturvedi P, Tyagi SC. Browning of white fat: novel insight into factors, mechanisms, and therapeutics. J Cell Physiol. (2017) 232:61–8. doi: 10.1002/jcp.25450
36. Lee YH, Mottillo EP, Granneman JG. Adipose tissue plasticity from WAT to BAT and in between. Biochim Biophys Acta. (2014) 1842:358–69. doi: 10.1016/j.bbadis.2013.05.011
37. Gil A, Olza J, Gil-Campos M, Gomez-Llorente C, Aguilera CM. Is adipose tissue metabolically different at different sites? Int J Pediatr Obes. (2011) 6(Suppl. 1):13–20. doi: 10.3109/17477166.2011.604326
38. Esteve Rafols M. Adipose tissue: cell heterogeneity and functional diversity. Endocrinol Nutr. (2014) 61:100–12. doi: 10.1016/j.endonu.2013.03.011
39. Lim S, Honek J, Xue Y, Seki T, Cao Z, Andersson P, et al. Cold-induced activation of brown adipose tissue and adipose angiogenesis in mice. Nat Protoc. (2012) 7:606–15. doi: 10.1038/nprot.2012.013
40. Tao C, Huang S, Wang Y, Wei G, Zhang Y, Qi D, et al. Changes in white and brown adipose tissue microRNA expression in cold-induced mice. Biochem Biophys Res Commun. (2015) 463:193–9. doi: 10.1016/j.bbrc.2015.05.014
41. Saito M, Okamatsu-Ogura Y, Matsushita M, Watanabe K, Yoneshiro T, Nio-Kobayashi J, et al. High incidence of metabolically active brown adipose tissue in healthy adult humans: effects of cold exposure and adiposity. Diabetes. (2009) 58:1526–31. doi: 10.2337/db09-0530
42. Van Marken Lichtenbelt WD, Vanhommerig JW, Smulders NM, Drossaerts JM, Kemerink GJ, Bouvy ND, et al. Cold-activated brown adipose tissue in healthy men. N Engl J Med. (2009) 360:1500–8. doi: 10.1056/NEJMoa0808718
43. Virtanen KA, Lidell ME, Orava J, Heglind M, Westergren R, Niemi T, et al. Functional brown adipose tissue in healthy adults. N Engl J Med. (2009) 360:1518–25. doi: 10.1056/NEJMoa0808949
44. Cannon B, Nedergaard J. Brown adipose tissue: function and physiological significance. Physiol Rev. (2004) 84:277–359. doi: 10.1152/physrev.00015.2003
45. Bargut TC, Aguila MB, Mandarim-De-Lacerda CA. Brown adipose tissue: updates in cellular and molecular biology. Tissue Cell. (2016) 48:452–60. doi: 10.1016/j.tice.2016.08.001
46. Penna-De-Carvalho A, Graus-Nunes F, Rabelo-Andrade J, Mandarim-De-Lacerda CA, Souza-Mello V. Enhanced pan-peroxisome proliferator-activated receptor gene and protein expression in adipose tissue of diet-induced obese mice treated with telmisartan. Exp Physiol. (2014) 99:1663–78. doi: 10.1113/expphysiol.2014.081596
47. Rachid TL, Penna-De-Carvalho A, Bringhenti I, Aguila MB, Mandarim-De-Lacerda CA, Souza-Mello V. PPAR-alpha agonist elicits metabolically active brown adipocytes and weight loss in diet-induced obese mice. Cell Biochem Funct. (2015) 33:249–56. doi: 10.1002/cbf.3111
48. Kim WK, Choi HR, Park SG, Ko Y, Bae KH, Lee SC. Myostatin inhibits brown adipocyte differentiation via regulation of Smad3-mediated beta-catenin stabilization. Int J Biochem Cell Biol. (2012) 44:327–34. doi: 10.1016/j.biocel.2011.11.004
49. Castro E, Silva TEO, Festuccia WT. Critical review of beige adipocyte thermogenic activation and contribution to whole-body energy expenditure. Horm Mol Biol Clin Investig. (2017) 31. doi: 10.1515/hmbci-2017-0042
50. Gao Z, Daquinag AC, Su F, Snyder B, Kolonin MG. PDGFRalpha/PDGFRbeta signaling balance modulates progenitor cell differentiation into white and beige adipocytes. Development. (2018) 145:dev155861. doi: 10.1242/dev.155861
51. Kaisanlahti A, Glumoff T. Browning of white fat: agents and implications for beige adipose tissue to type 2 diabetes. J Physiol Biochem. (2019) 75:1–10. doi: 10.1007/s13105-018-0658-5
52. Norouzirad R, Ghanbari M, Bahadoran Z, Abdollahifar MA, Rasouli N, Ghasemi A. Hyperoxia improves carbohydrate metabolism by browning of white adipocytes in obese type 2 diabetic rats. Life Sci. (2019) 220:58–68. doi: 10.1016/j.lfs.2019.01.045
53. Pardo R, Blasco N, Vila M, Beiroa D, Nogueiras R, Canas X, et al. EndoG knockout mice show increased brown adipocyte recruitment in white adipose tissue and improved glucose homeostasis. Endocrinology. (2016) 157:3873–87. doi: 10.1210/en.2015-1334
54. Guan L, Gong D, Yang S, Shen N, Zhang S, Li Y, et al. Genipin ameliorates diet-induced obesity via promoting lipid mobilization and browning of white adipose tissue in rats. Phytother Res. (2018) 32:723–32. doi: 10.1002/ptr.6022
55. Wang S, Wang X, Ye Z, Xu C, Zhang M, Ruan B, et al. Curcumin promotes browning of white adipose tissue in a norepinephrine-dependent way. Biochem Biophys Res Commun. (2015) 466:247–53. doi: 10.1016/j.bbrc.2015.09.018
56. Hu J, Christian M. Hormonal factors in the control of the browning of white adipose tissue. Horm Mol Biol Clin Investig. (2017) 31. doi: 10.1515/hmbci-2017-0017
57. Merlin J, Sato M, Chia LY, Fahey R, Pakzad M, Nowell CJ, et al. Rosiglitazone and a beta3-adrenoceptor agonist are both required for functional browning of white adipocytes in culture. Front Endocrinol. (2018) 9:249. doi: 10.3389/fendo.2018.00249
58. Sepa-Kishi DM, Ceddia RB. Exercise-mediated effects on white and brown adipose tissue plasticity and metabolism. Exerc Sport Sci Rev. (2016) 44:37–44. doi: 10.1249/JES.0000000000000068
59. Dempersmier J, Sambeat A, Gulyaeva O, Paul SM, Hudak CS, Raposo HF, et al. Cold-inducible Zfp516 activates UCP1 transcription to promote browning of white fat and development of brown fat. Mol Cell. (2015) 57:235–46. doi: 10.1016/j.molcel.2014.12.005
60. Gao Y, Qimuge NR, Qin J, Cai R, Li X, Chu GY, et al. Acute and chronic cold exposure differentially affects the browning of porcine white adipose tissue. Animal. (2018) 12:1435–41. doi: 10.1017/S1751731117002981
61. Bonet ML, Mercader J, Palou A. A nutritional perspective on UCP1-dependent thermogenesis. Biochimie. (2017) 134:99–117. doi: 10.1016/j.biochi.2016.12.014
62. El Hadi H, Di Vincenzo A, Vettor R, Rossato M. Food ingredients involved in white-to-brown adipose tissue conversion and in calorie burning. Front Physiol. (2018) 9:1954. doi: 10.3389/fphys.2018.01954
63. Kris-Etherton PM, Hecker KD, Bonanome A, Coval SM, Binkoski AE, Hilpert KF, et al. Bioactive compounds in foods: their role in the prevention of cardiovascular disease and cancer. Am J Med. (2002) 113(Suppl. 9B):71s−88s. doi: 10.1016/S0002-9343(01)00995-0
64. Godoy LD, Lucas JE, Bender AJ, Romanick SS, Ferguson BS. Targeting the epigenome: screening bioactive compounds that regulate histone deacetylase activity. Mol Nutr Food Res. (2017) 61. doi: 10.1002/mnfr.201600744
65. Walle T. Bioavailability of resveratrol. Ann N Y Acad Sci. (2011) 1215:9–15. doi: 10.1111/j.1749-6632.2010.05842.x
66. Gambini J, Ingles M, Olaso G, Lopez-Grueso R, Bonet-Costa V, Gimeno-Mallench L, et al. Properties of resveratrol: in vitro and in vivo studies about metabolism, bioavailability, and biological effects in animal models and humans. Oxid Med Cell Longev. (2015) 2015:837042. doi: 10.1155/2015/837042
67. Ko JH, Sethi G, Um JY, Shanmugam MK, Arfuso F, Kumar AP, et al. The role of resveratrol in cancer therapy. Int J Mol Sci. (2017) 18:E2589. doi: 10.3390/ijms18122589
68. Diaz M, Degens H, Vanhees L, Austin C, Azzawi M. The effects of resveratrol on aging vessels. Exp Gerontol. (2016) 85:41–7. doi: 10.1016/j.exger.2016.09.016
69. Aguirre L, Fernandez-Quintela A, Arias N, Portillo MP. Resveratrol: anti-obesity mechanisms of action. Molecules. (2014) 19:18632–55. doi: 10.3390/molecules191118632
70. Szkudelski T, Szkudelska K. Resveratrol and diabetes: from animal to human studies. Biochim Biophys Acta. (2015) 1852:1145–54. doi: 10.1016/j.bbadis.2014.10.013
71. Bonnefont-Rousselot D. Resveratrol and cardiovascular diseases. Nutrients. (2016) 8:E250. doi: 10.3390/nu8050250
72. Rauf A, Imran M, Suleria HAR, Ahmad B, Peters DG, Mubarak MS. A comprehensive review of the health perspectives of resveratrol. Food Funct. (2017) 8:4284–305. doi: 10.1039/C7FO01300K
73. Lagouge M, Argmann C, Gerhart-Hines Z, Meziane H, Lerin C, Daussin F, et al. Resveratrol improves mitochondrial function and protects against metabolic disease by activating SIRT1 and PGC-1alpha. Cell. (2006) 127:1109–22. doi: 10.1016/j.cell.2006.11.013
74. Baur JA, Pearson KJ, Price NL, Jamieson HA, Lerin C, Kalra A, et al. Resveratrol improves health and survival of mice on a high-calorie diet. Nature. (2006) 444:337–42. doi: 10.1038/nature05354
75. Kim S, Jin Y, Choi Y, Park T. Resveratrol exerts anti-obesity effects via mechanisms involving down-regulation of adipogenic and inflammatory processes in mice. Biochem Pharmacol. (2011) 81:1343–51. doi: 10.1016/j.bcp.2011.03.012
76. Cho SJ, Jung UJ, Choi MS. Differential effects of low-dose resveratrol on adiposity and hepatic steatosis in diet-induced obese mice. Br J Nutr. (2012) 108:2166–75. doi: 10.1017/S0007114512000347
77. Jeon SM, Lee SA, Choi MS. Antiobesity and vasoprotective effects of resveratrol in apoE-deficient mice. J Med Food. (2014) 17:310–6. doi: 10.1089/jmf.2013.2885
78. Qiao Y, Sun J, Xia S, Tang X, Shi Y, Le G. Effects of resveratrol on gut microbiota and fat storage in a mouse model with high-fat-induced obesity. Food Funct. (2014) 5:1241–9. doi: 10.1039/c3fo60630a
79. Andrade JM, Paraiso AF, De Oliveira MV, Martins AM, Neto JF, Guimaraes AL, et al. Resveratrol attenuates hepatic steatosis in high-fat fed mice by decreasing lipogenesis and inflammation. Nutrition. (2014) 30:915–9. doi: 10.1016/j.nut.2013.11.016
80. Gu H, Li K, Li X, Yu X, Wang W, Ding L, et al. Oral resveratrol prevents osteoarthritis progression in C57BL/6J mice fed a high-fat diet. Nutrients. (2016) 8:233. doi: 10.3390/nu8040233
81. Chang CC, Lin KY, Peng KY, Day YJ, Hung LM. Resveratrol exerts anti-obesity effects in high-fat diet obese mice and displays differential dosage effects on cytotoxicity, differentiation, and lipolysis in 3T3-L1 cells. Endocr J. (2016) 63:169–78. doi: 10.1507/endocrj.EJ15-0545
82. Cheng K, Song Z, Zhang H, Li S, Wang C, Zhang L, et al. The therapeutic effects of resveratrol on hepatic steatosis in high-fat diet-induced obese mice by improving oxidative stress, inflammation and lipid-related gene transcriptional expression. Med Mol Morphol. (2019). doi: 10.1007/s00795-019-00216-7. [Epub ahead of print].
83. Voigt A, Ribot J, Sabater AG, Palou A, Bonet ML, Klaus S. Identification of Mest/Peg1 gene expression as a predictive biomarker of adipose tissue expansion sensitive to dietary anti-obesity interventions. Genes Nutr. (2015) 10:27. doi: 10.1007/s12263-015-0477-z
84. Tauriainen E, Luostarinen M, Martonen E, Finckenberg P, Kovalainen M, Huotari A, et al. Distinct effects of calorie restriction and resveratrol on diet-induced obesity and Fatty liver formation. J Nutr Metab. (2011) 2011:525094. doi: 10.1155/2011/525094
85. Macarulla MT, Alberdi G, Gomez S, Tueros I, Bald C, Rodriguez VM, et al. Effects of different doses of resveratrol on body fat and serum parameters in rats fed a hypercaloric diet. J Physiol Biochem. (2009) 65:369–76. doi: 10.1007/BF03185932
86. Alberdi G, Rodriguez VM, Miranda J, Macarulla MT, Arias N, Andres-Lacueva C, et al. Changes in white adipose tissue metabolism induced by resveratrol in rats. Nutr Metab. (2011) 8:29. doi: 10.1186/1743-7075-8-29
87. Alberdi G, Rodriguez VM, Miranda J, Macarulla MT, Churruca I, Portillo MP. Thermogenesis is involved in the body-fat lowering effects of resveratrol in rats. Food Chem. (2013) 141:1530–5. doi: 10.1016/j.foodchem.2013.03.085
88. Rivera L, Moron R, Zarzuelo A, Galisteo M. Long-term resveratrol administration reduces metabolic disturbances and lowers blood pressure in obese Zucker rats. Biochem Pharmacol. (2009) 77:1053–63. doi: 10.1016/j.bcp.2008.11.027
89. Gomez-Zorita S, Fernandez-Quintela A, Lasa A, Hijona E, Bujanda L, Portillo MP. Effects of resveratrol on obesity-related inflammation markers in adipose tissue of genetically obese rats. Nutrition. (2013) 29:1374–80. doi: 10.1016/j.nut.2013.04.014
90. Nagao K, Jinnouchi T, Kai S, Yanagita T. Effect of dietary resveratrol on the metabolic profile of nutrients in obese OLETF rats. Lipids Health Dis. (2013) 12:8. doi: 10.1186/1476-511X-12-8
91. Trepiana J, Gomez-Zorita S, Fernandez-Quintela A, Gonzalez M, Portillo MP. Effects of resveratrol and its analogue pterostilbene, on NOV/CCN3 adipokine in adipose tissue from rats fed a high-fat high-sucrose diet. J Physiol Biochem. (2019). doi: 10.1007/s13105-019-00680-w. [Epub ahead of print].
92. Ardid-Ruiz A, Ibars M, Mena P, Del Rio D, Muguerza B, Blade C, et al. Potential involvement of peripheral leptin/STAT3 signaling in the effects of resveratrol and its metabolites on reducing body fat accumulation. Nutrients. (2018) 10:E1757. doi: 10.3390/nu10111757
93. Arias N, Macarulla MT, Aguirre L, Milton I, Portillo MP. The combination of resveratrol and quercetin enhances the individual effects of these molecules on triacylglycerol metabolism in white adipose tissue. Eur J Nutr. (2016) 55:341–8. doi: 10.1007/s00394-015-0854-9
94. Mendes KL, De Pinho L, Andrade JM, Paraiso AF, Lula JF, Macedo SM, et al. Distinct metabolic effects of resveratrol on lipogenesis markers in mice adipose tissue treated with high-polyunsaturated fat and high-protein diets. Life Sci. (2016) 153:66–73. doi: 10.1016/j.lfs.2016.04.014
95. Mendez-Del Villar M, Gonzalez-Ortiz M, Martinez-Abundis E, Perez-Rubio KG, Lizarraga-Valdez R. Effect of resveratrol administration on metabolic syndrome, insulin sensitivity, and insulin secretion. Metab Syndr Relat Disord. (2014) 12:497–501. doi: 10.1089/met.2014.0082
96. Faghihzadeh F, Adibi P, Rafiei R, Hekmatdoost A. Resveratrol supplementation improves inflammatory biomarkers in patients with nonalcoholic fatty liver disease. Nutr Res. (2014) 34:837–43. doi: 10.1016/j.nutres.2014.09.005
97. Most J, Timmers S, Warnke I, Jocken JW, Van Boekschoten M, De Groot P, et al. Combined epigallocatechin-3-gallate and resveratrol supplementation for 12 wk increases mitochondrial capacity and fat oxidation, but not insulin sensitivity, in obese humans: a randomized controlled trial. Am J Clin Nutr. (2016) 104:215–27. doi: 10.3945/ajcn.115.122937
98. Arzola-Paniagua MA, Garcia-Salgado Lopez ER, Calvo-Vargas CG, Guevara-Cruz M. Efficacy of an orlistat-resveratrol combination for weight loss in subjects with obesity: a randomized controlled trial. Obesity. (2016) 24:1454–63. doi: 10.1002/oby.21523
99. Timmers S, Konings E, Bilet L, Houtkooper RH, Van De Weijer T, Goossens GH, et al. Calorie restriction-like effects of 30 days of resveratrol supplementation on energy metabolism and metabolic profile in obese humans. Cell Metab. (2011) 14:612–22. doi: 10.1016/j.cmet.2011.10.002
100. Konings E, Timmers S, Boekschoten MV, Goossens GH, Jocken JW, Afman LA, et al. The effects of 30 days resveratrol supplementation on adipose tissue morphology and gene expression patterns in obese men. Int J Obes. (2014) 38:470–3. doi: 10.1038/ijo.2013.155
101. Poulsen MM, Vestergaard PF, Clasen BF, Radko Y, Christensen LP, Stodkilde-Jorgensen H, et al. High-dose resveratrol supplementation in obese men: an investigator-initiated, randomized, placebo-controlled clinical trial of substrate metabolism, insulin sensitivity, and body composition. Diabetes. (2013) 62:1186–95. doi: 10.2337/db12-0975
102. Chachay VS, Macdonald GA, Martin JH, Whitehead JP, O'moore-Sullivan TM, Lee P, et al. Resveratrol does not benefit patients with nonalcoholic fatty liver disease. Clin Gastroenterol Hepatol. (2014) 12:2092–103.e1–6. doi: 10.1016/j.cgh.2014.02.024
103. Heeboll S, Kreuzfeldt M, Hamilton-Dutoit S, Kjaer Poulsen M, Stodkilde-Jorgensen H, Moller HJ, et al. Placebo-controlled, randomised clinical trial: high-dose resveratrol treatment for non-alcoholic fatty liver disease. Scand J Gastroenterol. (2016) 51:456–64. doi: 10.3109/00365521.2015.1107620
104. Yoshino J, Conte C, Fontana L, Mittendorfer B, Imai S, Schechtman KB, et al. Resveratrol supplementation does not improve metabolic function in nonobese women with normal glucose tolerance. Cell Metab. (2012) 16:658–64. doi: 10.1016/j.cmet.2012.09.015
105. Vargas-Castillo A, Fuentes-Romero R, Rodriguez-Lopez LA, Torres N, Tovar AR. Understanding the biology of thermogenic fat: is browning a new approach to the treatment of obesity? Arch Med Res. (2017) 48:401–13. doi: 10.1016/j.arcmed.2017.10.002
106. Andrade JM, Frade AC, Guimaraes JB, Freitas KM, Lopes MT, Guimaraes AL, et al. Resveratrol increases brown adipose tissue thermogenesis markers by increasing SIRT1 and energy expenditure and decreasing fat accumulation in adipose tissue of mice fed a standard diet. Eur J Nutr. (2014) 53:1503–10. doi: 10.1007/s00394-014-0655-6
107. Wang S, Liang X, Yang Q, Fu X, Zhu M, Rodgers BD, et al. Resveratrol enhances brown adipocyte formation and function by activating AMP-activated protein kinase (AMPK) alpha1 in mice fed high-fat diet. Mol Nutr Food Res. (2017) 61:1600746. doi: 10.1002/mnfr.201600746
108. Arias N, Pico C, Teresa Macarulla M, Oliver P, Miranda J, Palou A, et al. A combination of resveratrol and quercetin induces browning in white adipose tissue of rats fed an obesogenic diet. Obesity. (2017) 25:111–21. doi: 10.1002/oby.21706
109. Qiang L, Wang L, Kon N, Zhao W, Lee S, Zhang Y, et al. Brown remodeling of white adipose tissue by SirT1-dependent deacetylation of Ppargamma. Cell. (2012) 150:620–32. doi: 10.1016/j.cell.2012.06.027
110. Um JH, Park SJ, Kang H, Yang S, Foretz M, Mcburney MW, et al. AMP-activated protein kinase-deficient mice are resistant to the metabolic effects of resveratrol. Diabetes. (2010) 59:554–63. doi: 10.2337/db09-0482
111. Park SJ, Ahmad F, Philp A, Baar K, Williams T, Luo H, et al. Resveratrol ameliorates aging-related metabolic phenotypes by inhibiting cAMP phosphodiesterases. Cell. (2012) 148:421–33. doi: 10.1016/j.cell.2012.01.017
112. Gerard P. Gut microbiota and obesity. Cell Mol Life Sci. (2016) 73:147–62. doi: 10.1007/s00018-015-2061-5
113. Morris A. Gut microbiota: link between the gut and adipose tissues. Nat Rev Endocrinol. (2017) 13:501. doi: 10.1038/nrendo.2017.92
114. Moreno-Navarrete JM, Serino M, Blasco-Baque V, Azalbert V, Barton RH, Cardellini M, et al. Gut microbiota interacts with markers of adipose tissue browning, insulin action and plasma acetate in morbid obesity. Mol Nutr Food Res. (2018) 62:1700721. doi: 10.1002/mnfr.201700721
115. Jandhyala SM, Talukdar R, Subramanyam C, Vuyyuru H, Sasikala M, Nageshwar Reddy D. Role of the normal gut microbiota. World J Gastroenterol. (2015) 21:8787–803. doi: 10.3748/wjg.v21.i29.8787
116. Zhou L, Xiao X. The role of gut microbiota in the effects of maternal obesity during pregnancy on offspring metabolism. Biosci Rep. (2018) 38:BSR20171234. doi: 10.1042/BSR20171234
117. Blandino G, Inturri R, Lazzara F, Di Rosa M, Malaguarnera L. Impact of gut microbiota on diabetes mellitus. Diabetes Metab. (2016) 42:303–15. doi: 10.1016/j.diabet.2016.04.004
118. Saad MJ, Santos A, Prada PO. Linking gut microbiota and inflammation to obesity and insulin resistance. Physiology. (2016) 31:283–93. doi: 10.1152/physiol.00041.2015
119. Ghazalpour A, Cespedes I, Bennett BJ, Allayee H. Expanding role of gut microbiota in lipid metabolism. Curr Opin Lipidol. (2016) 27:141–7. doi: 10.1097/MOL.0000000000000278
120. Sung MM, Byrne NJ, Robertson IM, Kim TT, Samokhvalov V, Levasseur J, et al. Resveratrol improves exercise performance and skeletal muscle oxidative capacity in heart failure. Am J Physiol Heart Circ Physiol. (2017) 312:H842–H853. doi: 10.1152/ajpheart.00455.2016
121. Chen ML, Yi L, Zhang Y, Zhou X, Ran L, Yang J, et al. Resveratrol attenuates trimethylamine-N-Oxide (TMAO)-induced atherosclerosis by regulating TMAO synthesis and bile acid metabolism via remodeling of the gut microbiota. MBio. (2016) 7:e02210–02215. doi: 10.1128/mBio.02210-15
122. Sung MM, Kim TT, Denou E, Soltys CM, Hamza SM, Byrne NJ, et al. Improved glucose homeostasis in obese mice treated with resveratrol is associated with alterations in the gut microbiome. Diabetes. (2017) 66:418–25. doi: 10.2337/db16-0680
123. Brandt N, Kotowska D, Kristensen CM, Olesen J, Lutzhoft DO, Halling JF, et al. The impact of exercise training and resveratrol supplementation on gut microbiota composition in high-fat diet fed mice. Physiol Rep. (2018) 6:e13881. doi: 10.14814/phy2.13881
124. Campbell CL, Yu R, Li F, Zhou Q, Chen D, Qi C, et al. Modulation of fat metabolism and gut microbiota by resveratrol on high-fat diet-induced obese mice. Diabetes Metab Syndr Obes. (2019) 12:97–107. doi: 10.2147/DMSO.S192228
125. Jung MJ, Lee J, Shin NR, Kim MS, Hyun DW, Yun JH, et al. Chronic repression of mTOR complex 2 induces changes in the gut microbiota of diet-induced obese mice. Sci Rep. (2016) 6:30887. doi: 10.1038/srep30887
126. Yang C, Deng Q, Xu J, Wang X, Hu C, Tang H, et al. Sinapic acid and resveratrol alleviate oxidative stress with modulation of gut microbiota in high-fat diet-fed rats. Food Res Int. (2019) 116:1202–11. doi: 10.1016/j.foodres.2018.10.003
127. Zhao L, Zhang Q, Ma W, Tian F, Shen H, Zhou M. A combination of quercetin and resveratrol reduces obesity in high-fat diet-fed rats by modulation of gut microbiota. Food Funct. (2017) 8:4644–56. doi: 10.1039/C7FO01383C
128. Etxeberria U, Arias N, Boque N, Macarulla MT, Portillo MP, Martinez JA, et al. Reshaping faecal gut microbiota composition by the intake of trans-resveratrol and quercetin in high-fat sucrose diet-fed rats. J Nutr Biochem. (2015) 26:651–60. doi: 10.1016/j.jnutbio.2015.01.002
129. Tain YL, Lee WC, Wu KLH, Leu S, Chan JYH. Resveratrol prevents the development of hypertension programmed by maternal plus post-weaning high-fructose consumption through modulation of oxidative stress, nutrient-sensing signals, and gut microbiota. Mol Nutr Food Res. (2018) e1800066. doi: 10.1002/mnfr.201800066. [Epub ahead of print].
130. Kim TT, Parajuli N, Sung MM, Bairwa SC, Levasseur J, Soltys CM, et al. Fecal transplant from resveratrol-fed donors improves glycaemia and cardiovascular features of the metabolic syndrome in mice. Am J Physiol Endocrinol Metab. (2018) 315:E511–E519. doi: 10.1152/ajpendo.00471.2017
131. Wang P, Li D, Ke W, Liang D, Hu X, Chen F. Resveratrol-induced gut microbiota reduces obesity in high-fat diet-fed mice. Int J Obes. (2019) doi: 10.1038/s41366-019-0332-1. [Epub ahead of print].
132. Mestdagh R, Dumas ME, Rezzi S, Kochhar S, Holmes E, Claus SP, et al. Gut microbiota modulate the metabolism of brown adipose tissue in mice. J Proteome Res. (2012) 11:620–30. doi: 10.1021/pr200938v
133. Chevalier C, Stojanovic O, Colin DJ, Suarez-Zamorano N, Tarallo V, Veyrat-Durebex C, et al. Gut microbiota orchestrates energy homeostasis during cold. Cell. (2015) 163:1360–74. doi: 10.1016/j.cell.2015.11.004
134. Zietak M, Kovatcheva-Datchary P, Markiewicz LH, Stahlman M, Kozak LP, Backhed F. Altered microbiota contributes to reduced diet-induced obesity upon cold exposure. Cell Metab. (2016) 23:1216–23. doi: 10.1016/j.cmet.2016.05.001
135. Somm E, Henry H, Bruce SJ, Aeby S, Rosikiewicz M, Sykiotis GP, et al. beta-Klotho deficiency protects against obesity through a crosstalk between liver, microbiota, and brown adipose tissue. JCI Insight. (2017) 2:91809. doi: 10.1172/jci.insight.91809
136. Li B, Li L, Li M, Lam SM, Wang G, Wu Y, et al. Microbiota depletion impairs thermogenesis of brown adipose tissue and browning of white adipose tissue. Cell Rep. (2019) 26:2720–2737.e2725. doi: 10.1016/j.celrep.2019.02.015
137. Liu J, Li Y, Yang P, Wan J, Chang Q, Wang TTY, et al. Gypenosides reduced the risk of overweight and insulin resistance in C57BL/6J mice through modulating adipose thermogenesis and gut microbiota. J Agric Food Chem. (2017) 65:9237–46. doi: 10.1021/acs.jafc.7b03382
138. Nagata N, Xu L, Kohno S, Ushida Y, Aoki Y, Umeda R, et al. Glucoraphanin ameliorates obesity and insulin resistance through adipose tissue browning and reduction of metabolic endotoxemia in mice. Diabetes. (2017) 66:1222–36. doi: 10.2337/db16-0662
139. Gao X, Xie Q, Kong P, Liu L, Sun S, Xiong B, et al. Polyphenol- and caffeine-rich postfermented pu-erh tea improves diet-induced metabolic syndrome by remodeling intestinal homeostasis in mice. Infect Immun. (2018) 86:e00601-17. doi: 10.1128/IAI.00601-17
140. Fu ZD, Cui JY. Remote sensing between liver and intestine: importance of microbial metabolites. Curr Pharmacol Rep. (2017) 3:101–13. doi: 10.1007/s40495-017-0087-0
141. Matheus VA, Monteiro L, Oliveira RB, Maschio DA, Collares-Buzato CB. Butyrate reduces high-fat diet-induced metabolic alterations, hepatic steatosis and pancreatic beta cell and intestinal barrier dysfunctions in prediabetic mice. Exp Biol Med. (2017) 242:1214–26. doi: 10.1177/1535370217708188
142. Morris G, Berk M, Carvalho A, Caso JR, Sanz Y, Walder K, et al. The role of the microbial metabolites including tryptophan catabolites and short chain fatty acids in the pathophysiology of immune-inflammatory and neuroimmune disease. Mol Neurobiol. (2017) 54:4432–51. doi: 10.1007/s12035-016-0004-2
143. Ohira H, Tsutsui W, Fujioka Y. Are short chain fatty acids in gut microbiota defensive players for inflammation and atherosclerosis? J Atheroscler Thromb. (2017) 24:660–72. doi: 10.5551/jat.RV17006
144. Sun MF, Shen YQ. Dysbiosis of gut microbiota and microbial metabolites in Parkinson's disease. Ageing Res Rev. (2018) 45:53–61. doi: 10.1016/j.arr.2018.04.004
145. Hald S, Schioldan AG, Moore ME, Dige A, Laerke HN, Agnholt J, et al. Effects of arabinoxylan and resistant starch on intestinal microbiota and short-chain fatty acids in subjects with metabolic syndrome: a randomised crossover study. PLoS ONE. (2016) 11:e0159223. doi: 10.1371/journal.pone.0159223
146. Chambers ES, Preston T, Frost G, Morrison DJ. Role of gut microbiota-generated short-chain fatty acids in metabolic and cardiovascular health. Curr Nutr Rep. (2018) 7:198–206. doi: 10.1007/s13668-018-0248-8
147. Hu J, Lin S, Zheng B, Cheung PCK. Short-chain fatty acids in control of energy metabolism. Crit Rev Food Sci Nutr. (2018) 58:1243–9. doi: 10.1080/10408398.2016.1245650
148. Hanatani S, Motoshima H, Takaki Y, Kawasaki S, Igata M, Matsumura T, et al. Acetate alters expression of genes involved in beige adipogenesis in 3T3-L1 cells and obese KK-Ay mice. J Clin Biochem Nutr. (2016) 59:207–14. doi: 10.3164/jcbn.16-23
149. Sahuri-Arisoylu M, Brody LP, Parkinson JR, Parkes H, Navaratnam N, Miller AD, et al. Reprogramming of hepatic fat accumulation and ‘browning' of adipose tissue by the short-chain fatty acid acetate. Int J Obes. (2016) 40:955–63. doi: 10.1038/ijo.2016.23
150. Lin HV, Frassetto A, Kowalik EJ Jr, Nawrocki AR, Lu MM, Kosinski JR, et al. Butyrate and propionate protect against diet-induced obesity and regulate gut hormones via free fatty acid receptor 3-independent mechanisms. PLoS ONE. (2012) 7:e35240. doi: 10.1371/journal.pone.0035240
151. Kawanaka M, Nishino K, Oka T, Urata N, Nakamura J, Suehiro M, et al. Tyrosine levels are associated with insulin resistance in patients with nonalcoholic fatty liver disease. Hepat Med. (2015) 7:29–35. doi: 10.2147/HMER.S79100
152. Han X, Guo J, You Y, Yin M, Liang J, Ren C, et al. Vanillic acid activates thermogenesis in brown and white adipose tissue. Food Funct. (2018) 9:4366–75. doi: 10.1039/C8FO00978C
153. Kim M, Furuzono T, Yamakuni K, Li Y, Kim YI, Takahashi H, et al. 10-oxo-12(Z)-octadecenoic acid, a linoleic acid metabolite produced by gut lactic acid bacteria, enhances energy metabolism by activation of TRPV1. FASEB J. (2017) 31:5036–48. doi: 10.1096/fj.201700151R
154. Zobel EH, Hansen TW, Rossing P, Von Scholten BJ. Global changes in food supply and the obesity epidemic. Curr Obes Rep. (2016) 5:449–55. doi: 10.1007/s13679-016-0233-8
155. Wang S, Moustaid-Moussa N, Chen L, Mo H, Shastri A, Su R, et al. Novel insights of dietary polyphenols and obesity. J Nutr Biochem. (2014) 25:1–18. doi: 10.1016/j.jnutbio.2013.09.001
156. Jimoh A, Tanko Y, Ahmed A, Mohammed A, Ayo JO. Resveratrol prevents high-fat diet-induced obesity and oxidative stress in rabbits. Pathophysiology. (2018) 25:359–64. doi: 10.1016/j.pathophys.2018.07.003
157. Okla M, Kim J, Koehler K, Chung S. Dietary factors promoting brown and beige fat development and thermogenesis. Adv Nutr. (2017) 8:473–83. doi: 10.3945/an.116.014332
Keywords: resveratrol, browning of white adipose tissue, brown adipose tissue, gut microbiota, metabolites, obesity, metabolic disorders
Citation: Zhou L, Xiao X, Zhang Q, Zheng J and Deng M (2019) Deciphering the Anti-obesity Benefits of Resveratrol: The “Gut Microbiota-Adipose Tissue” Axis. Front. Endocrinol. 10:413. doi: 10.3389/fendo.2019.00413
Received: 22 April 2019; Accepted: 07 June 2019;
Published: 27 June 2019.
Edited by:
Margaret Morris, University of New South Wales, AustraliaReviewed by:
Carmelo Quarta, Helmholtz Center Munich, GermanyClaire Joanne Stocker, University of Buckingham, United Kingdom
Copyright © 2019 Zhou, Xiao, Zhang, Zheng and Deng. This is an open-access article distributed under the terms of the Creative Commons Attribution License (CC BY). The use, distribution or reproduction in other forums is permitted, provided the original author(s) and the copyright owner(s) are credited and that the original publication in this journal is cited, in accordance with accepted academic practice. No use, distribution or reproduction is permitted which does not comply with these terms.
*Correspondence: Xinhua Xiao, eGlhb3hoMjAxNEB2aXAuMTYzLmNvbQ==