- Barts and the London School of Medicine and Dentistry, Centre for Endocrinology, William Harvey Research Institute, Queen Mary University of London, London, United Kingdom
Glucocorticoid production in mammals is principally regulated by the action of the pituitary hormone adrenocorticotropin (ACTH) acting on its cognate membrane receptor on the zona fasciculata cells of the adrenal cortex. The receptor for ACTH consists of two essential components, a small seven transmembrane domain G protein-coupled receptor of the melanocortin receptor subgroup known as the melanocortin 2 receptor (MC2R) and a small single transmembrane domain protein that adopts a antiparallel homodimeric form and which is known as the melanocortin 2 receptor accessory protein (MRAP). MRAP is essential for the trafficking of the MC2R to the cell surface as well as being required for receptor responsiveness to ACTH at physiological concentrations—probably by facilitating ACTH binding, but possibly also by supporting G protein interaction with the MC2R. A number of studies have shown that ACTH stimulates the expression of functional receptor at the cell surface and the transcription of both MC2R and MRAP mRNA. However, the time course of these transcriptional effects differs such that MRAP is expressed relatively rapidly whereas MC2R transcription responds much more slowly. Furthermore, recent data suggests that MRAP protein is turned over with a short half-life whereas MC2R has a significantly longer half-life. These findings imply that these two ACTH receptor proteins have distinct trajectories and that it is likely that MRAP-independent MC2R is present at the cell surface. In such a situation newly transcribed and translated MRAP could enable the rapid recruitment of functional receptor at the plasma membrane without the need for new MC2R translation. This may be advantageous in circumstances of significant stress in that the potentially complex and perhaps inefficient process of de novo MC2R translation, folding, post-translational modification and trafficking can be avoided.
The pituitary-adrenal axis provides a vital mechanism for protection against stress, allowing higher centers to rapidly generate increases in circulating glucocorticoids. These in turn adjust the metabolic and immunological functions of the body to cope with the altered environment. Failure of this system is potentially fatal. It is surprising therefore that there is not greater redundancy among the components of this system. For example, the key messenger hormone, adrenocorticotropin (ACTH) is the product of a single gene and is secreted from a single population of corticotroph cells in a single anatomical organ—the anterior pituitary gland. ACTH in turn acts through a single receptor located almost entirely on the surface of cells of the two adrenal glands, resulting in the production of a single glucocorticoid hormone—cortisol or corticosterone. Although the ACTH receptor was one of the first receptors to be shown to signal through stimulation of cyclic AMP (1), and one of the first receptors for which ligand binding was demonstrated (2), the molecular characterization of this entity was relatively delayed. Protein purification approaches were largely unsuccessful, and it was not until a gene was identified through reduced stringency PCR which was shown to be closely related to a gene encoding a receptor for α-melanocyte stimulating hormone (α-MSH). This gene was expressed almost exclusively in the adrenal cortex and hence was described as the melanocortin 2 receptor (MC2R) and proposed to encode the ACTH receptor (3). Subsequent demonstration of mutations in this gene in patients with ACTH resistance lent support to this identification (4, 5).
With most receptors for which a gene or cDNA is identified in this way the usual practice is to transfect the candidate DNA into a cell type that lacks the receptor in question and to show the cell acquires the characteristics of the receptor. In the case of the ACTH receptor however this proved very difficult. Some evidence of expression could be found in cell types that already possessed MSH receptors (3, 6), but expression in a true null cell devoid of any MSH receptor was not achievable.
The first evidence that the MC2R required a co-factor came from the demonstration that it could be expressed in adrenal cell lines that lacked endogenous ACTH receptors—implying that there was something else about the adrenal cell required for successful expression (7). However, it was not until a new gene was found that was mutated in patients with ACTH resistance but a normal MC2R gene that a solution to this problem was identified. This new gene encoded a small single transmembrane domain protein that when co-expressed with the MC2R in transfected cells conferred typical ACTH responsiveness to those cells. This gene was named the melanocortin 2 receptor accessory protein—MRAP (8).
Evidence from a number of labs showed that the MRAP protein interacted with the MC2R as a homodimer and then trafficked with the receptor to the plasma membrane (9, 10). This appeared to be the primary function of MRAP in that without MRAP, the MC2R protein accumulated at the ER and did not appear at the cell surface.
At the cell surface MRAP maintained an association with the receptor protein and the evidence suggests it is required for ACTH binding or possibly in signal transduction (11, 12). Perhaps the most unusual phenomenon associated with MRAP is that it exists as an antiparallel homodimer spanning the plasma membrane such that one N-terminus is extracellular and one is intracellular (9). This topology is probably unique in eukaryotic biology, and suggests a very unusual mode of action that remains poorly understood.
These aspects of MRAP have been reviewed elsewhere (13, 14) and are not the prime focus of this paper. Instead, we will consider the dynamic aspects of MRAP which may challenge the notion that it is the “loyal and lifelong” partner of the MC2R as may be assumed, or whether it has a more complex and perhaps transient role with its partner protein.
Induction of MC2R mRNA by ACTH
It was recognized some years before the ACTH receptor was cloned and characterized that ACTH could induce increased responsiveness to itself and an increased number of receptors in the adrenal. Thus, for example, Durand and Locatelli (15) demonstrated in rabbits that ACTH treatment increased the number of its adrenal receptors. Similar findings were reported from fetal sheep adrenals (16). Penhoat et al. (17) showed that 48 h treatment with physiological concentrations of ACTH increased ACTH ligand binding on bovine adrenal cells four-fold.
Following the cloning of the MC2R several groups explored the influence of ACTH and other stimuli on MC2R mRNA. Thus Mountjoy et al. (18) showed a 6-fold increase in Mc2r mRNA in mouse Y1 cells and a 2-fold increase in human H-295 cells after 24 h of treatment with ACTH. Lebrethon et al. (19) found a 21-fold increase in MC2R mRNA and a 4-fold increase in ACTH binding in human primary adrenal cells, initial responses being seen within 12 h of treatment, and similar changes after 48 h treatment with ACTH in human fetal adrenal cells (20). Rehman et al. (21) showed induction of MC2R mRNA after ACTH after 6 h in cultured human fetal adrenal cells. More recently Liu et al described the effect of restraint stress or ACTH injection on rat adrenal Mc2r mRNA over a short time period and failed to show any changes over the initial 4 h (in response to restraint) or over the first 60 min in response to ACTH (22). These and other similar studies are summarized in Table 1.
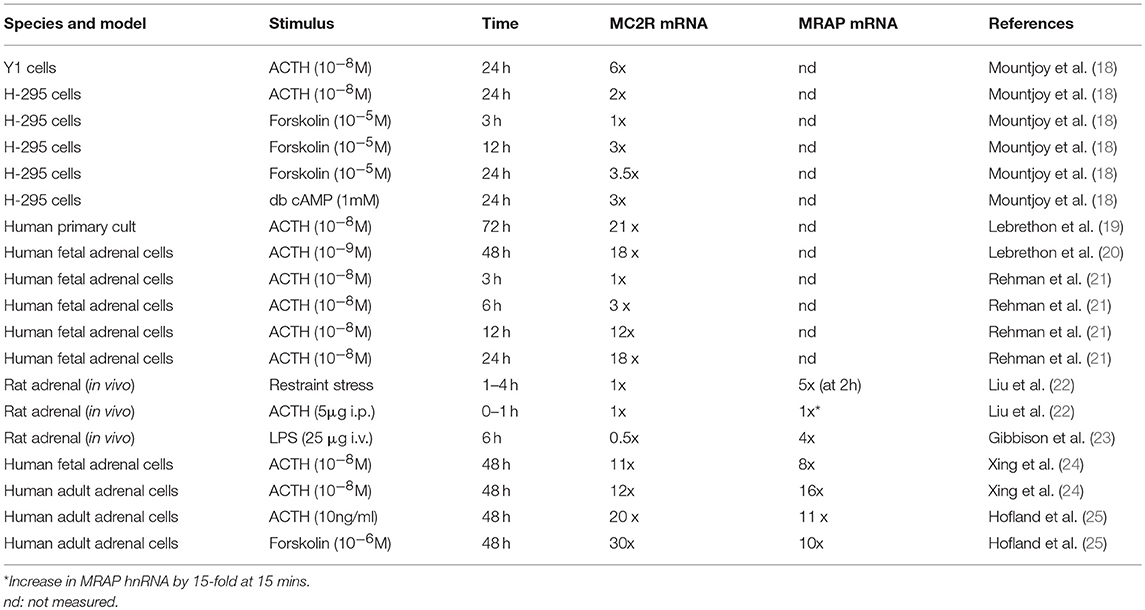
Table 1. Summary of studies of MC2R and MRAP mRNA expression changes in response to various stimuli.
Induction of MRAP mRNA by ACTH
As the MRAP gene was only identified in 2005 these earlier studies did not examine its response to ACTH and related stimuli, and it is only in later studies that both MC2R and MRAP gene expression in the same model has been reported.
Thus in their study Liu et al. (22) demonstrated the rapid induction of adrenal Mrap mRNA by about 5-fold within 2 h in their model of restraint stress, and a 15-fold induction of Mrap heteronuclear RNA within 15 min of ACTH injection (although no change in mature Mrap mRNA was observed in this time frame). These increases were not sustained and in the restraint stress model Mrap mRNA had almost normalized after 4 h. Gibbinson et al. (23) found a 4-fold increase in adrenal Mrap mRNA 6 h after LPS injection in the rat (at a time when Mc2r mRNA levels had fallen).
Over a longer time period Xing et al. (24) used a microarray approach to explore gene expression changes after 48 h of treatment of human fetal or adult adrenals demonstrating a 16-fold and 12-fold change of MRAP and MC2R expression (respectively) in adult adrenal, and 8-fold and 11-fold increases in these two genes in fetal adrenal. Hofland et al. (25) demonstrated 11 and 20-fold increases in MRAP and MC2R in human normal adrenal primary cultures exposed to 48 h treatment with ACTH. These various studies are summarized in Table 1.
In summary, although a range of models and stimuli have been used, it is unfortunate that few have examined the response of both MC2R and MRAP over a time course that encompasses both acute and more delayed time periods. However, it does seem from the available data that while MC2R is almost universally induced over a longer time period of 12−24 h or more, MRAP is capable of making a more rapid response to ACTH, restraint stress or LPS stimulation.
ACTH Receptor Proteins
The next question that arises is what happens to the ACTH receptor protein complex after its translation. Very little data has been collected on the behavior of the MC2R and MRAP proteins—in large part because of absence of good quality antibodies.
It seems that the MRAP homodimer forms at the same time as, or very soon after translation, and that the MRAP molecule in which the N-terminus is in the extracellular position is then glycosylated—probably stabilizing the homodimer at this time. This then interacts with the MC2R protein at the level of the endoplasmic reticulum or Golgi (26). This complex is capable of trafficking to the cell surface, although whether MRAP has an additional role in supporting correct folding of the MC2R is not clear. It is also unclear what the fate of un-complexed MC2R is at the ER—for example in circumstances in which MRAP is not expressed. In studies in cells lacking MRAP, transfected MC2R accumulated substantially at the ER, although the relationship of such overexpression systems to physiology is uncertain (27). It is also clear that in the absence of MC2R, MRAP will traffic efficiently to the cell surface (8, 9). The key elements of this conventional model are shown in Figure 1.
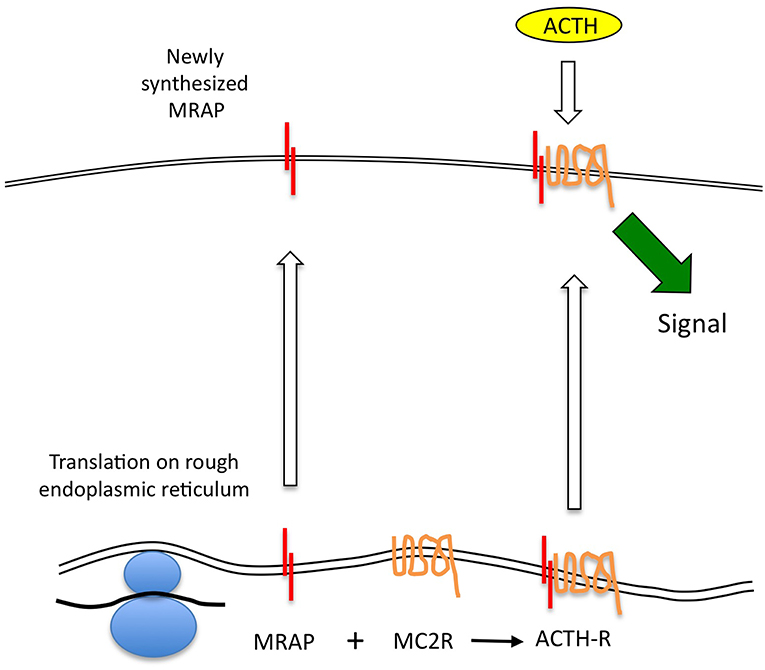
Figure 1. ACTH receptor protein expression. MC2R mRNA is translated at the endoplasmic reticulum (ER), and may independently adopt a correctly folded and orientated receptor conformation. However, it is unable to traffic beyond this point to the plasma membrane. MRAP mRNA is translated and adopts an anti-parallel homodimeric conformation at the ER. This complexes with the MC2R protein and may assist with its correct conformational folding before enabling the complex to traffic to the cell surface. Only the MRAP-MC2R complex is competent to bind ACTH at physiological concentrations and thence to generate a steroidogenic signal.
ACTH Receptor Turnover
In an article published in this journal in 2016, Maben et al traced the fate of MRAP in transfected CHO cells (28). They concluded that MRAP has a half-life of 1.7 h and is not stabilized by the presence of MC2R expression. Using similar techniques they estimated that the MC2R protein half-life was between 3 and 8 h. This is a surprising finding as it implies that after reaching the plasma membrane MRAP and MC2R turn over independently of each other. This is apparently in contradiction to the “lifetime partnership” model and implies that MRAP-independent MC2R is likely to be present at the cell surface under most circumstances and is presumably unresponsive to ACTH.
Clearly this half-life data needs replication although it was obtained using a robust but relatively novel biotin-labeling technique as well as by a more traditional cycloheximide technique with similar findings. It has the disadvantage of deriving from transfected CHO cells rather than cells in which MRAP and MC2R were endogenously expressed. Unquantitated data from transfected OS3 adrenocortical cells was also shown in the same paper which appeared to suggest a substantially longer half life for the MRAP protein—particularly in the absence of MC2R. OS3 cells express endogenous and functional MRAP, but no endogenous MC2R (29). Maben et al. argued that OS3 cells grow more slowly than CHO cells and that this may explain the slower turnover, but clearly an alternative explanation is that adrenal cells protect the turnover of these proteins for functional reasons. This question needs further exploration.
If we accept that MRAP protein does turnover rapidly and at a faster rate than MC2R, it seems probable that the ACTH receptor complex is not a tight one and that free MRAP (presumably in its homodimeric form) and MC2R may be present at the cell surface. Unfortunately there is no published data on the relative quantities of these two proteins in adrenal cells expressing functional ACTH receptors. There is also no data on the affinities of the MRAP dimer for the receptor. Roy et al provided immunofluorescent imaging data to support the existence of non-complexed MC2R and MRAP in transfected cells (30). If there is free MC2R on the cell surface this would form a reservoir of “spare” receptor that was incapable of responding to ACTH. Consequently, the relatively rapid transcription of MRAP in response to the various stimuli described earlier may enable functionally “new” receptor to be recruited at the cell surface in the absence of rapid MC2R gene expression. This would provide an attractive and efficient mechanism for the rapid upregulation of the ACTH receptor without having to depend on the accurate folding of new MC2R protein. Elements of this model are shown in Figure 2.
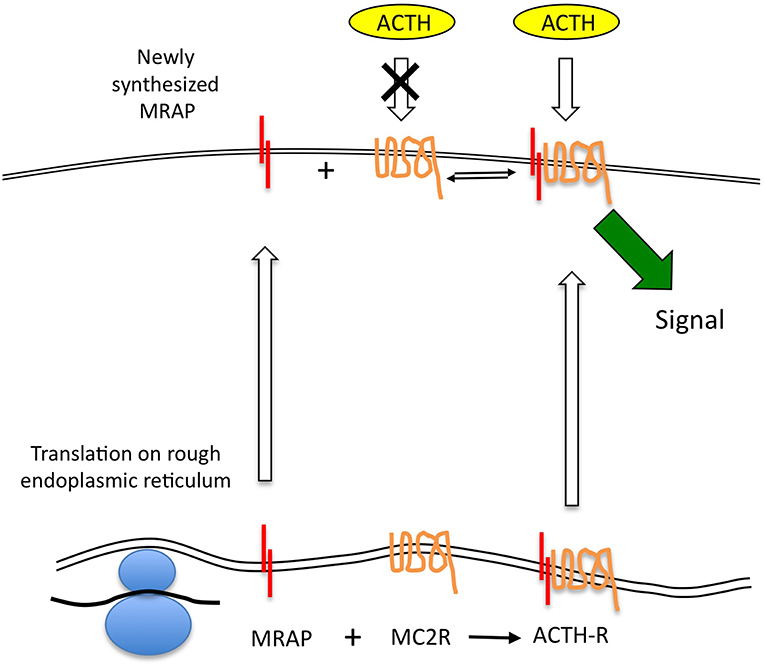
Figure 2. The MRAP-MC2R ACTH receptor complex may be relatively labile allowing MRAP protein to turnover more quickly than the MC2R, and thus leaving uncomplexed MC2R, which is believed to be unresponsive to ACTH stimulation, at the cell surface. This open the possibility that newly transcribed MRAP mRNA can be quickly translated and traffic to the cell surface to complex with “free” MC2R. Such a model permits a rapid response in the form of new signaling-competent receptor recruitment.
The ACTH receptor desensitizes and internalizes after agonist stimulation via clathrin-coated pits and re-cycles directly, or via the Golgi-recycling pathway (31, 32). Around 70% of the cell surface receptor that binds ACTH does not internalize however (31, 32) (Figure 3), and it would be interesting to know if this is the un-partnered MC2R that was unable to signal. This may be a false conclusion however as Sebag and Hinkle (11) have shown that MRAP determines ACTH ligand binding via a specific LDYI motif in the N-terminal region, so un-partnered MC2R may be unlikely to bind the ACTH tracer used in these studies. This situation may be more complex however in that MRAP2, a paralogue of MRAP, which lacks the critical LDYI ACTH binding motif of MRAP will support ACTH signaling, and hence binding, at high (micromolar) ACTH concentrations (33).
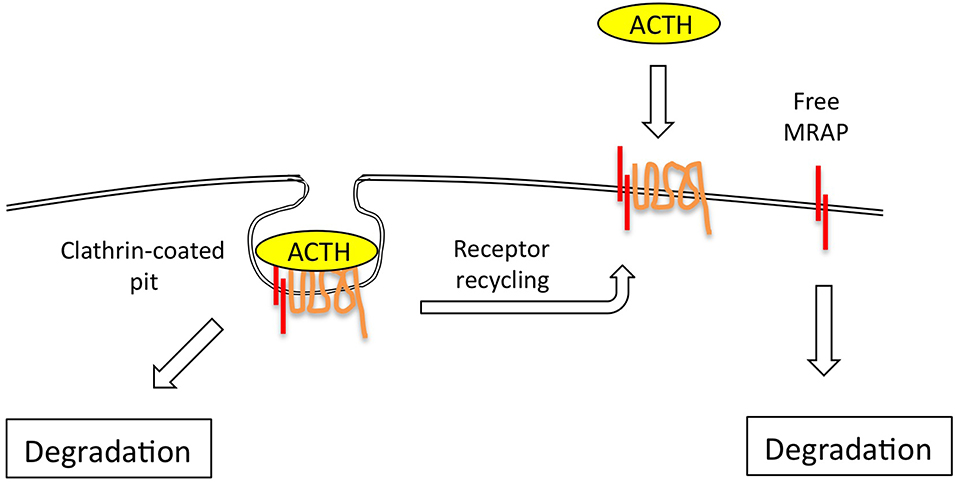
Figure 3. The ACTH receptor complex internalizes via clathrin-coated pits, following which receptor is either rapidly re-cycled to the plasma membrane, or degraded. The evidence suggests that MRAP is also independently and fairly rapidly degraded via ubiquitin-dependent and independent mechanisms.
Other than the adrenal cortex, MRAP is also expressed in adipocytes, and indeed was first identified as a protein of unknown function that was expressed as 3T3-L1 fibroblasts differentiated into adipocytes (34). Zhang et al. (35) have shown in differentiated 3T3-L1 adipocytes that the N-terminus of MRAP interacts with the Gαs protein and that the region between residues 14 and 17 of MRAP is critical for this role. While these findings may be compatible with each other, Sebag and Hinkle had previously demonstrated that the MRAP 14-17 deletion mutant was fully capable of signaling in their earlier studies (11).
Conclusions
MRAP is a very unusual protein that appears to have a critical role in permitting the MC2R to traffic to the cell surface and to respond to ACTH—either by influencing ACTH ligand binding or by approximating the Gαs protein to the receptor (or both). ACTH receptor expression can be stimulated by a variety of exogenous stimuli including ACTH exposure. This is mediated by the relatively rapid increase of MRAP transcription while MC2R transcription is more delayed. Newly translated MRAP will enable any MC2R still resident at the endoplasmic reticulum to traffic to the cell surface, but it seems more likely that most of the newly synthesized MRAP will traffic independently to the cell surface where it may partner with un-partnered MC2R, thus creating new functional receptor. Consistent with this is the observation that MRAP protein may turnover more rapidly than MC2R, although the mechanisms and cellular location for this degradation are not clear.
Author Contributions
This work is the result of discussion between the authors. The initial draft was prepared by AC and revised by both authors.
Funding
LC was supported by this Fellowship from the MRC.
Conflict of Interest Statement
The authors declare that the research was conducted in the absence of any commercial or financial relationships that could be construed as a potential conflict of interest.
Acknowledgments
LC was recipient of the Medical Research Council (MRC) UK/Academy of Medical Sciences Clinician Scientist Fellowship Grant G0802796 that supported work reviewed in the manuscript and is currently funded by IFCAH (International Funding for Congenital Adrenal Hyperplasia).
References
1. Haynes RC Jr. The activation of adrenal phosphorylase by the adrenocorticotropic hormone. J Biol Chem. (1958) 233:1220–2.
2. Lefkowitz RJ, Roth J, Pricer W, Pastan I. ACTH receptors in the adrenal: specific binding of ACTH-125I and its relation to adenyl cyclase. Proc Natl Acad Sci USA. (1970) 65:745–52. doi: 10.1073/pnas.65.3.745
3. Mountjoy KG, Robbins LS, Mortrud MT, Cone RD. The cloning of a family of genes that encode the melanocortin receptors. Science. (1992) 257:1248–51. doi: 10.1126/science.1325670
4. Clark AJ, McLoughlin L, Grossman A. Familial glucocorticoid deficiency associated with point mutation in the adrenocorticotropin receptor. Lancet. (1993) 341:461–2. doi: 10.1016/0140-6736(93)90208-X
5. Tsigos C, Arai K, Hung W, Chrousos GP. Hereditary isolated glucocorticoid deficiency is associated with abnormalities of the adrenocorticotropin receptor gene. J Clin Invest. (1993) 92:2458–61. doi: 10.1172/JCI116853
6. Weber A, Kapas S, Hinson J, Grant DB, Grossman A, Clark AJ. Functional characterization of the cloned human ACTH receptor: impaired responsiveness of a mutant receptor in familial glucocorticoid deficiency. Biochem Biophys Res Commun. (1993) 197:172–8. doi: 10.1006/bbrc.1993.2456
7. Yang YK, Ollmann MM, Wilson BD, Dickinson C, Yamada T, Barsh GS, et al. Effects of recombinant agouti-signaling protein on melanocortin action. Mol Endocrinol. (1997) 11:274–80. doi: 10.1210/mend.11.3.9898
8. Metherell LA, Chapple JP, Cooray S, David A, Becker C, Ruschendorf F, et al. (2005). Mutations in MRAP, encoding a new interacting partner of the ACTH receptor, cause familial glucocorticoid deficiency type 2. Nat Genet. 37:166–70. doi: 10.1038/ng1501
9. Sebag JA, Hinkle PM. Melanocortin-2 receptor accessory protein MRAP forms antiparallel homodimers. Proc Natl Acad Sci USA. (2007) 104:20244–49. doi: 10.1073/pnas.0708916105
10. Cooray SN, Almiro Do Vale I, Leung KY, Webb TR, Chapple JP, Egertova M, et al. The melanocortin 2 receptor accessory protein exists as a homodimer and is essential for the function of the melanocortin 2 receptor in the mouse y1 cell line. Endocrinology. (2008) 149:1935–41. doi: 10.1210/en.2007-1463
11. Sebag JA, Hinkle PM. Opposite effects of the melanocortin-2 (MC2) receptor accessory protein MRAP on MC2 and MC5 receptor dimerization and trafficking. J Biol Chem. (2009) 284:22641–48. doi: 10.1074/jbc.M109.022400
12. Hinkle PM, Serasinghe MN, Jakabowski A, Sebag JA, Wilson K, et al. Use of chimeric melanocortin-2 and−4 receptors to identify regions responsible for ligand specificity and dependence on melanocortin 2 receptor accessory protein. Eur J Pharmacol. (2011) 660:94–102. doi: 10.1016/j.ejphar.2010.10.113
13. Novoselova TV, Jackson D, Campbell DC, Clark AJ, Chan LF. Melanocortin receptor accessory proteins in adrenal gland physiology and beyond. J Endocrinol. (2013) 217:R1–11. doi: 10.1530/JOE-12-0501
14. Webb TR, Clark AJ. Minireview: the melanocortin 2 receptor accessory proteins. Mol Endocrinol. (2010) 24:475–84. doi: 10.1210/me.2009-0283
15. Durand P, Locatelli A. Up regulation of corticotrophin receptors by ACTH1-24 in normal and hypophysectomized rabbits. Biochem Biophys Res Commun. (1980) 96:447–56. doi: 10.1016/0006-291X(80)91235-8
16. Durand P, Locatelli A, Cathiard AM, Dazord A, Saez JM. ACTH induction of the maturation of ACTH-sensitive adenylate cyclase system in the ovine fetal adrenal. J Steroid Biochem. (1981) 15:445–8. doi: 10.1016/0022-4731(81)90312-5
17. Penhoat A, Jaillard C, Saez JM. Corticotropin positively regulates its own receptors and cAMP response in cultured bovine adrenal cells. Proc Natl Acad Sci USA. (1989) 86:4978–4981. doi: 10.1073/pnas.86.13.4978
18. Mountjoy KG, Bird IM, Rainey WE, Cone RD. ACTH induces up-regulation of ACTH receptor mRNA in mouse and human adrenocortical cell lines. Mol Cell Endocrinol. (1994) 99:R17–20. doi: 10.1016/0303-7207(94)90160-0
19. Lebrethon MC, Naville D, Begeot M, Saez JM. Regulation of corticotropin receptor number and messenger RNA in cultured human adrenocortical cells by corticotropin and angiotensin II. J Clin Invest. (1994) 93:1828–33. doi: 10.1172/JCI117168
20. Lebrethon MC, Jaillard C, Naville D, Begeot M, Saez JM. Regulation of corticotropin and steroidogenic enzyme mRNAs in human fetal adrenal cells by corticotropin, angiotensin-II and transforming growth factor beta 1. Mol Cell Endocrinol. (1994) 106:137–43. doi: 10.1016/0303-7207(94)90195-3
21. Rehman KS, Sirianni R, Parker CR Jr, Rainey WE, Carr BR. The regulation of adrenocorticotrophic hormone receptor by corticotropin-releasing hormone in human fetal adrenal definitive/transitional zone cells. Reprod Sci. (2007) 14:578–87. doi: 10.1177/1933719107307908
22. Liu Y, Smith LI, Huang V, Poon V, Coello A, et al. Transcriptional regulation of episodic glucocorticoid secretion. Mol Cell Endocrinol. (2013) 371:62–70. doi: 10.1016/j.mce.2012.10.011
23. Gibbison B, Spiga F, Walker JJ, Russell GM, Stevenson K, Kershaw Y, et al. Dynamic pituitary-adrenal interactions in response to cardiac surgery. Crit Care Med. (2015) 43:791–800. doi: 10.1097/CCM.0000000000000773
24. Xing Y, Parker CR, Edwards M, Rainey WE. ACTH is a potent regulator of gene expression in human adrenal cells. J Mol Endocrinol. (2010) 45:59–68. doi: 10.1677/JME-10-0006
25. Hofland J, Delhanty PJ, Steenbergen J, Hofland LJ, van Koetsveld PM, van Nederveen FH, et al. Melanocortin 2 receptor-associated protein (MRAP) and MRAP2 in human adrenocortical tissues: regulation of expression and association with ACTH responsiveness. J Clin Endocrinol Metab. (2012) 97:E747–54. doi: 10.1210/jc.2011-2328
26. Cooray SN, Chung TT, Mazhar K, Szidonya L, Clark AJ. Bioluminescence resonance energy transfer reveals the adrenocorticotropin (ACTH)-induced conformational change of the activated ACTH receptor complex in living cells. Endocrinology. (2011) 152:495–502. doi: 10.1210/en.2010-1053
27. Noon LA, Franklin JM, King PJ, Goulding NJ, Hunyady L, Clark AJ. Failed export of the adrenocorticotrophin receptor from the endoplasmic reticulum in non-adrenal cells: evidence in support of a requirement for a specific adrenal accessory factor. J Endocrinol. (2002) 174:17–25. doi: 10.1677/joe.0.1740017
28. Maben ZJ, Malik S, Jiang LH, Hinkle PM. Dual topology of the melanocortin-2 receptor accessory protein is stable. Front Endocrinol. (2016) 7:96. doi: 10.3389/fendo.2016.00096
29. Schimmer BP, Kwan WK, Tsao J, Qiu R. Adrenocorticotropin-resistant mutants of the Y1 adrenal cell line fail to express the adrenocorticotropin receptor. J Cell Physiol. (1995) 163:164–71. doi: 10.1002/jcp.1041630119
30. Roy S, Rached M, Gallo-Payet N. Differential regulation of the human adrenocorticotropin receptor [melanocortin-2 receptor (MC2R)] by human MC2R accessory protein isoforms alpha and beta in isogenic human embryonic kidney 293 cells. Mol Endocrinol. (2007) 21:1656–69. doi: 10.1210/me.2007-0041
31. Roy S, Roy SJ, Pinard L, Taillefer LD, Rached M, Parent JL, et al. Mechanisms of melanocortin-2 receptor (MC2R) internalization and recycling in human embryonic kidney (HEK) cells: identification of key Ser/Thr (S/T) amino acids. Mol Endocrinol. (2011) 25:1961–77. doi: 10.1210/me.2011-0018
32. Baig AH, Swords FM, Szaszak M, King PJ, Hunyady L, Clark AJ. Agonist activated adrenocorticotropin receptor internalizes via a clathrin-mediated G protein receptor kinase dependent mechanism. Endocr Res. (2002) 28:281–9. doi: 10.1081/ERC-120016798
33. Gorrigan RJ, Guasti L, King P, Clark AJ, Chan LF. Localisation of the melanocortin-2-receptor and its accessory proteins in the developing and adult adrenal gland. J Mol Endocrinol. (2011) 46:227–32. doi: 10.1530/JME-11-0011
34. Xu A, Choi KL, Wang Y, Permana PA, Xu LY, et al. Identification of novel putative membrane proteins selectively expressed during adipose conversion of 3T3-L1 cells. Biochem Biophys Res Commun. (2002) 293:1161–7. doi: 10.1016/S0006-291X(02)00354-6
Keywords: ACTH receptor, adrenal cortex, glucocorticoid, G protein-coupled receptor, adrenocorticotropin, melanocortin receptor
Citation: Clark AJL and Chan L (2019) Stability and Turnover of the ACTH Receptor Complex. Front. Endocrinol. 10:491. doi: 10.3389/fendo.2019.00491
Received: 13 February 2019; Accepted: 08 July 2019;
Published: 26 July 2019.
Edited by:
László Hunyady, Semmelweis University, HungaryReviewed by:
Rostislav Turecek, Academy of Sciences of the Czech Republic (ASCR), CzechiaPatricia M. Hinkle, Medical Center, University of Rochester, United States
Copyright © 2019 Clark and Chan. This is an open-access article distributed under the terms of the Creative Commons Attribution License (CC BY). The use, distribution or reproduction in other forums is permitted, provided the original author(s) and the copyright owner(s) are credited and that the original publication in this journal is cited, in accordance with accepted academic practice. No use, distribution or reproduction is permitted which does not comply with these terms.
*Correspondence: Adrian J. L. Clark, YS5qLmNsYXJrQHFtdWwuYWMudWs=