- 1Department of Neuroscience, Karolinska Institutet, Stockholm, Sweden
- 2Department of Biomolecular Science, Section of Physiology, University of Urbino, Urbino, Italy
- 3Grupo Bohío-Estudio, Observatorio Cubano de Neurociencias, Yaguajay, Cuba
Based on the work in the Central Nervous System with discoveries of allosteric receptor-receptor interactions in homo- and heteroreceptor complexes representing a major integrative mechanism in synapses and extrasynaptic regions, it is proposed that a similar mechanism may exist in the immunological synapses. We discuss a putative additional molecular mechanism for the ability of the inhibitory T cell signaling proteins CTLA-4 and PD-1 and the adenosine A2AR to diminish T cell activation leading to enhancement of cancer development. We suggest that in the same immunological synapse involving T cells and antigen presenting cells multiple heteroreceptor complexes may participate and be in balance with each other. Their composition can vary between functional states and among different types of T cells. The T cell receptor (TCR) and its accelerators, strongly enhancing T cell activation, can be under inhibitory control by T cell signaling proteins CTLA4 and PD-1 and also the adenosine A2AR through inhibitory allosteric receptor-receptor interactions in different types of heteroreceptor complexes. As a result, inhibitory tumor induced immunosuppression can develop due to a dominance of the inhibitory signaling causing a brake on the TCR and/or its accelerator and the cancer immunotherapy becomes blocked.
Understanding the Mechanisms of Cancer Therapy Based on Protein Brakes on T Cells
The first phase of precision medicine in the treatment of cancer derived from the characterization of mutations that induced or inhibited intracellular signaling routes leading to the development of cancer (1). It produced a specific treatment of cancer in most patients having the mutation but with the drawback that the clinical responses were of limited duration. The second phase was produced by advancement of immunology at the molecular level which made it possible to improve our understanding of the complexity of the immune mechanisms. As a result, the impact of activation of T cells for cancer therapy was introduced.
Nobel laureates James Allison and Tasuku Honjo discovered cancer therapy by inhibition of negative immune regulation (2, 3). Their discoveries led to a new principle for immune therapy. Allison found that the T cell protein receptor CTLA-4 operates as a brake on T cell function which is activated by the T-cell receptor and the T-cell accelerator receptor, their combined activation leading to a strong immune response. Thus, there is a balance between inhibitory and activating T cell receptors regulating T cell function. Such a balance is observed also in the heteroreceptor complexes in the synapses and extrasynaptic regions in the CNS modulating synaptic function in brain circuits [see below and (4)]. Experiments performed by the Allison group suggested that altering this balance toward the activation of the T-cell function by removal of the inhibitory receptor signals may lead to a new cancer immunotherapy (5).
In parallel, the Honjo group discovered a protein (PD-1) located on the immune cells which they identified to be an immunoinhibitory receptor and acted as a brake on the stimulatory T cell immunoreceptors (3, 6).
This pioneering work by the two groups led to clinical trials based on the use of antibodies against the two immunoinhibitory receptors CTLA-4 and PD-1 which resulted in successful cancer immunotherapy, especially after PD-1 blockade, and combined blockade of the PD-1 and CTLA-4 (7, 8). It should also be noted that in our work in the brain we have proposed that cocaine addiction can be caused by cocaine induced formation of pathological A2AR-D2R-Sigma1R heteroreceptor complexes (9). They may represent long term memories involving a strong and permanent brake on D2R protomer signaling with A2AR protomer and the Sigma1R working together to produce the brake on D2R signaling and recognition (9).
Our theory on the molecular basis of learning and memory (9–12) may also be mentioned in view of its relevance for long-lived memory T-cells identified through increased expression of the interleukin 7 receptor (13). The molecular reorganization of the postsynaptic homo- and heteroreceptor complexes can produce a transient molecular engram that represents a short-term memory. The consolidation of the reorganized receptor complexes may lead to a permanent molecular engram. The consolidation can involve the transformation of parts of the receptor complexes into soluble molecules that can bind to the transcription factors and modulate their activity into forming adapter proteins that can consolidate the receptor panorama formed. It may maintain the new signaling.
In view of the above, it seems possible that integration in the T-cells not only involves changes in the signaling of the inhibitory and facilitatory immunoreceptors and integration of their intracellular pathways, but also their integration in immune synapses with the antigen presenting cells including the tumor cells. Based on the work in the CNS [see book edited by Fuxe and Borroto-Escuela (14)] it seems likely that integration in the immune system also involves the dynamic formation and operation of homo-and heteroreceptor complexes (Figure 1). The T cell receptor and the T cell accelerator receptors enhancing the immune response may form heteroreceptor complexes with the immunoinhibitory receptors CTLA4 and PD-1 inhibiting the immune responses in which the major integration of immune and transmitter/modulator signals take place (Figure 1). Multiple heteroreceptor complexes may be formed. The integration is proposed to be brought about through allosteric receptor-receptor and receptor-protein interactions which may be highly dynamic and dependent on the ligand concentrations for each receptor and the number of receptors and adapter proteins expressed in the immune synapses.
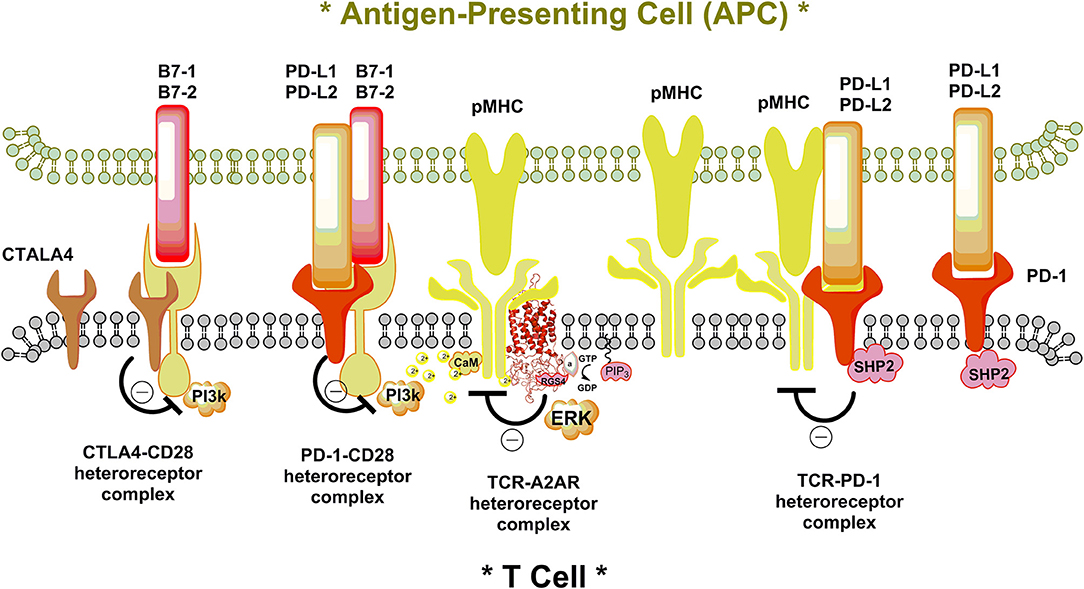
Figure 1. Illustration of a putative additional molecular mechanism for the ability of the inhibitory T cell signaling proteins CTLA-4 and PD-1 and the GPCR A2AR to diminish T cell activation leading to the enhancement of cancer development. The immune synapse between the antigen-presenting cell (APC) and T cell is shown. In the left part the accelerator costimulatory signaling protein CD28 in the plasma membrane of the T cell is shown being contacted by its activating protein B7-1linked to the plasma membrane of the APC. It is illustrated that that the inhibitory T cell proteins CTLA-4 and PD-1, activated by its ligands PD-L1 and PD-L2, may both (shown) or separately (not shown) directly contact CD28 to form a putative receptor complex. Through an inhibitory allosteric receptor-receptor interaction, the signaling of the CD28 becomes inhibited involving e.g., inhibition of phosphoinositide 3-kinase (PI3k) activation by CD28. To the right it is illustrated how the ligand bound PD1 may directly allosterically inhibit the T cell receptor (TCR) of the T cell, activated by the peptide bound major histocompatibility complex (pMHC). This may lead to reductions of TCR signaling through putative formation of a TCR-PD-1 complex. In addition, PD-1 L1 and L2 activation may also enhance the activity of protein tyrosine phosphatase 2 (SHP2) causing a reduction of phosphorylation of the TCR contributing to inhibition of TCR signaling. In the center of the immune synapse the adenosine activated adenosine receptor A2AR is proposed to directly interact with the TCR in the plasma membrane of the T cell to bring down its activation by pMHC through allosteric mechanisms. It is known that sustained activation of ERC produces strong T cell activation (15). It is therefore possible that in addition to the putative formation of TCR-A2AR heteroreceptor complexes, the A2AR induced activation of the AC-CREB pathway may also counteract the activation of the TCR by blocking sustained activation of ERK. This figure serves to indicate that the molecular mechanisms involve not only integration of the signaling pathways and their modulation of the signaling receptors involving changes in protein phosphorylation but also the allosteric integration in heteroreceptor complexes of the T cell through allosteric receptor-receptor and receptor-protein interactions. The balance of the heteroreceptor complexes with each other in the same T cell may also have a relevant role for the T cell function and help determine the state of the T cells.
In parallel to the work above, the highly exciting work performed by Sitkovsky et al. (16–18) took place on the role of adenosine A2ARs in tumor-induced immunosuppression. It seems likely that also the A2AR can participate in some of these heteroreceptor complexes in the immune synapse and play an important role by favoring inhibition of the immune response (Figure 1). It will be of value to test if the A2AR antagonists may also enhance the immunotherapeutic actions of anti-CTLA4 and/or anti-PD-1 therapies.
Integration of Signaling in the CNS Support the Above Hypothesis: Existence of Allosteric Receptor-Receptor Interactions in Synaptic and Extrasynaptic Heteroreceptor Complexes Including GPCRs
The Nobel Laureates Robert Lefkowitz and Brian Kobilka discovered the structure and function of the GPCRs (19). GPCRs of the different types were shown to form heteromers which means that they can physically interact with each other in the cytoplasm and/or in the plasma membrane (20–27). When the GPCRs are of the same type they are called homomers (20, 23, 28, 29). The term heteroreceptor complexes is used to describe that the receptor assemblies are built up of different receptors with unknown stoichiometry in combination with adaptor proteins. The latter can participate in the allosteric interactions between the receptors which can involve scaffolding functions to e.g., guide the receptors toward each other (9, 30, 31). The allosteric receptor-receptor interaction is a major mechanism in the heteroreceptor complexes. It develops when the binding of a ligand to an orthosteric or an allosteric site of one receptor protomer (present in an heteromer) via direct receptor-receptor interactions produces a change in another receptor protomer (present in the same heteromer) with regard to receptor binding, pharmacology, signaling, and/or trafficking (27, 32, 33).
The allosteric receptor-receptor and receptor-protein interactions result in highly diverse and biased signaling of the heteroreceptor complexes through changes in e.g., receptor recognition, G protein coupling involving switching between different G proteins. GPCRs interact not only with each other but also with ionotropic receptors (34, 35) and tyrosine kinase receptors (36–38). Reciprocal antagonistic or enhancing allosteric interactions can develop upon single or combined activation of the receptor protomers. The receptor interface of the various heteroreceptor complexes are currently being investigated and involve conserved amino acids that form hot spots that help bind the two interfaces together (39–41). Methods are presently being developed to determine the stoichiometry of the homo- and heteromers in cellular models and in tissues to know if we deal with dimers or higher order oligomers based on super-resolution and spatial intensity distribution methods (42–45). Early on higher order homo and heteromers were postulated to exist (46) and have now been demonstrated (47–49). The affinity of the receptors for each other and their relative amounts in the membrane domain studied will have a significant role in the heteromerization process.
Many of the homo and heteroreceptor complexes studied so far are mainly found in the extrasynaptic and synaptic regions of the plasma membrane of the neuronal networks using in situ Proximity Ligation Assay (PLA) and a neuronal marker antibody (14, 50). However, they can also exist in e.g., the astroglia based on PLA and astroglial markers like antibodies against glial fibrillary acidic protein. Furthermore, A2AR-D2R interactions in astroglia can modulate the gliotransmitter glutamate release from striatal astrocyte processes (51). Furthermore, cannabinoid receptors as well as the CB1-CB2 heteroreceptor complex have been demonstrated in activated microglia (52, 53).
These mechanisms in the brain are of particular interest for understanding the ability of A2AR antagonists or A2AR gene deletion to remove tumor-induced immunosuppression from tumor-reactive CD8+ T cells (17, 18).
Possible Mechanism for the A2AR Mediated Brake on Immunosuppression of T Cells
Already in 1997 Sitkovsky et al. found that A2AR mediated signaling inhibited T cell activation (54). In 2012 the Sitkovsky group also obtained indications that the immunosuppressive functions of CD4 (+) CD25 (+) FoxP3 (+) regulated T cells involve A2AR signaling (16). Finally, in a highly significant paper in 2018 this group obtained evidence that tumor-reactive CD8(+) T cells can be liberated from tumor-induced immunosuppression by A2AR antagonists and by A2AR but not A2BR gene deletion (18). The hypoxia developed in the tumor microenvironment leads to increased expression of ectonucleotidases with enhanced formation of adenosine and activation of the A2AR involved in producing the immunosuppression. The A2AR may work together with the transcription factor HIF 1A, which is stabilized by hypoxia, to produce immunosuppression (18). The mechanism for the A2AR induced immunosuppression is proposed to be the increased formation of cAMP formed from the Gs coupled A2AR which activates intracellular pathways to inhibit the activation of the intracellular pathways of the T cell receptor (TCR). The existence of such a mechanism is supported by the ability of the A2AR antagonist to increase the extracellular levels of Interferon gamma. The release of pro-inflammatory cytokines is an important part of the signaling function of the T cell receptor in the T cells (18).
Based on our work in the brain as discussed above, another mechanism can also be involved, namely the formation of A2AR-TCR heteroreceptor complexes in the plasma membrane in which the A2AR can inhibit the function of the TCR through allosteric receptor-receptor interactions (Figure 1). It would be of high interest to determine if such receptor complexes exist in the immune system leading to inhibitory and/or facilitatory allosteric receptor-receptor interactions. This molecular mechanism represents a general integrative mechanism in the CNS and exist not only in nerve cells but also in glial cells (11, 55–57). Previously the focus was on integration in the intracellular signaling pathways from different receptors and is presently still the only integrative mechanism discussed in relation to communication in the immune system.
As discussed above, agonist induced activation of the A2AR protomer leads to a brake on D2R protomer signaling in A2AR-D2R complexes. Upon activation of the adaptor protein Sigma1R in this receptor complex, an enhancement of the D2R brake develops that may contribute to development of cocaine addiction (9). Higher order receptor complexes may therefore be considered to exist also in the immune system.
Finally, it may also be considered that A2AR-A2BR heteroreceptor complexes were demonstrated (58). In these complexes A2A receptor ligand recognition and signaling was blocked by A2B receptors. As a result, A2AR ligands may fail to act in T cells in which the A2AR-A2BR heteromer is the major population vs. the A2AR-TCR complex.
Based on the work of Sitkovsky and his group it can be of high relevance to test if also other GPCRs besides A2AR can be involved in tumor induced immunosuppression or enhancement. The chemokine receptor 5 (CCR5) is of interest as pointed out by one of the reviewers. It exists in the plasma membrane of T cells and can form an heterodimer with CXCR4 as shown with Fluorescence resonance energy transfer (FRET) (59). Chemokine-binding modulated this heterodimer and the CCR5 homodimer was specifically vulnerable to internalization by the protein partner Na+/H+ exchanger regulatory factor 1 (60). Thus, the type of CCR5 complex formed may determine the degree of CCR5 internalization obtained and thus its ability to mediate HIV transfection. CCR5 can also form heterodimers with CCR2b leading to negative binding cooperativity (61). These results illustrate the diversity of signaling that can develop with chemokine receptors through the formation of different types of receptor complexes. It may be proposed that distinct types of CCR complexes with a special protein composition may have the ability to form complexes that specifically can interact with TCR and/or accelerator receptors to reduce or enhance immunosuppression.
Conclusions
The blockade by antibodies of the immunosuppressive proteins appears crucial for success in cancer immunotherapy (5, 7, 18). Based on the work in the CNS with discoveries of allosteric receptor-receptor interactions in homo- and heteroreceptor complexes representing a major integrative mechanism in synapses and extrasynaptic regions, it is proposed that a similar mechanism exists in the immunological synapses. In the same immunological synapse involving T cells and antigen presenting cells multiple heteroreceptor complexes can participate and be in balance with each other. Their composition can vary between functional states and among different types of T cells. It is illustrated that the T cell receptor (TCR) and its accelerators strongly enhancing T cell activation may be under inhibitory control by T cell signaling proteins CTLA4 and PD-1 and the GPCR A2AR through inhibitory allosteric receptor-receptor in different types of heteroreceptor complexes. However, higher order receptor complexes may also be formed where e.g., the inhibitory T cell signaling proteins CTLA4 and PD-1 can both participate in further enhancing the inhibition of the TCR and/or its accelerator (Figure 1). The same can be true for the A2AR when it is part of a higher order receptor complex in which also e.g., one of the inhibitory T cell signaling proteins participates. The cancer cells have the ability e.g., to increase the secretion of signals that activate the inhibitory T cell signaling proteins or the A2AR via increasing the levels of adenosine (18). As a result, inhibitory tumor induced immunosuppression develops due to a dominance of the inhibitory signaling causing a brake on the TCR and/or its accelerator. The removal of this brake has markedly improved immune cancer therapy (7).
Data Availability
Publicly available datasets were analyzed in this study. This data can be found here: https://www.www.gpcr-hetnet.com.
Author Contributions
We confirm and declare that all authors meet the criteria for authorship according to the ICMJE, including approval of the final manuscript, and they take public responsibility for the work and have full confidence in the accuracy and integrity of the work of other group authors. They have substantially contributed to the conception or design of the current review. Also they have participated in the acquisition, analysis, and interpretation of data for the current review version. They have also helped revising it critically for important intellectual content, and final approval of the version to be published. In addition, they have contributed in this last version of the manuscript in writing assistance, technical editing, and language editing.
Funding
The work was supported by the Swedish Medical Research Council (62X-00715-50-3) to KF, by Parkinson Fonden to KF, and by Hjärnfonden (F02018-0286), Hjärnfonden (F02019-0296), and Karolinska Institutet Forskningsstiftelser to DB-E. DB-E belongs to the Academia de Biólogos Cubanos group.
Conflict of Interest Statement
The authors declare that the research was conducted in the absence of any commercial or financial relationships that could be construed as a potential conflict of interest.
References
1. Sharma P, Allison JP. Immune checkpoint targeting in cancer therapy: toward combination strategies with curative potential. Cell. (2015) 161:205–14. doi: 10.1016/j.cell.2015.03.030
2. Leach DR, Krummel MF, Allison JP. Enhancement of antitumor immunity by CTLA-4 blockade. Science. (1996) 271:1734–6. doi: 10.1126/science.271.5256.1734
3. Freeman GJ, Long AJ, Iwai Y, Bourque K, Chernova T, Nishimura H, et al. Engagement of the PD-1 immunoinhibitory receptor by a novel B7 family member leads to negative regulation of lymphocyte activation. J Exp Med. (2000) 192:1027–34. doi: 10.1084/jem.192.7.1027
4. Borroto-Escuela DO, Carlsson J, Ambrogini P, Narvaez M, Wydra K, Tarakanov AO, et al. Understanding the role of GPCR heteroreceptor complexes in modulating the brain networks in health and disease. Front Cell Neurosci. (2017) 11:37. doi: 10.3389/fncel.2017.00037
5. Kwon ED, Hurwitz AA, Foster BA, Madias C, Feldhaus AL, Greenberg NM, et al. Manipulation of T cell costimulatory and inhibitory signals for immunotherapy of prostate cancer. Proc Natl Acad Sci USA. (1997) 94:8099–103. doi: 10.1073/pnas.94.15.8099
6. Ishida Y, Agata Y, Shibahara K, Honjo T. Induced expression of PD-1, a novel member of the immunoglobulin gene superfamily, upon programmed cell death. EMBO J. (1992) 11:3887–95. doi: 10.1002/j.1460-2075.1992.tb05481.x
7. Chowdhury PS, Chamoto K, Honjo T. Combination therapy strategies for improving PD-1 blockade efficacy: a new era in cancer immunotherapy. J Intern Med. (2018) 283:110–20. doi: 10.1111/joim.12708
8. Wei SC, Duffy CR, Allison JP. Fundamental mechanisms of immune checkpoint blockade therapy. Cancer Discov. (2018) 8:1069–86. doi: 10.1158/2159-8290.CD-18-0367
9. Borroto-Escuela DO, Wydra K, Filip M, Fuxe K. A2AR-D2R heteroreceptor complexes in cocaine reward and addiction. Trends Pharmacol Sci. (2018) 39:1008–20. doi: 10.1016/j.tips.2018.10.007
10. Fuxe K, Borroto-Escuela DO, Ciruela F, Guidolin D, Agnati LF. Receptor-receptor interactions in heteroreceptor complexes: a new principle in biology. Focus on their role in learning and memory. Neurosci Discov. (2014) 2:6. doi: 10.7243/2052-6946-2-6
11. Borroto-Escuela DO, Agnati LF, Bechter K, Jansson A, Tarakanov AO, Fuxe K. The role of transmitter diffusion and flow versus extracellular vesicles in volume transmission in the brain neural-glial networks. Philos Trans R Soc Lond B Biol Sci. (2015) 370:20140183. doi: 10.1098/rstb.2014.0183
12. Borroto-Escuela DO, Wydra K, Pintsuk J, Narvaez M, Corrales F, Zaniewska M, et al. Understanding the functional plasticity in neural networks of the basal ganglia in cocaine use disorder: a role for allosteric receptor-receptor interactions in A2A-D2 heteroreceptor complexes. Neural Plast. (2016) 2016:4827268. doi: 10.1155/2016/4827268
13. Kaech SM, Tan JT, Wherry EJ, Konieczny BT, Surh CD, Ahmed R. Selective expression of the interleukin 7 receptor identifies effector CD8 T cells that give rise to long-lived memory cells. Nat Immunol. (2003) 4:1191–8. doi: 10.1038/ni1009
14. Fuxe K, Borroto-Escuela DO. Receptor-Receptor Interactions in the Central Nervous System. New York, NY: Humana Press (2018). doi: 10.1007/978-1-4939-8576-0
15. Deswal S, Meyer A, Fiala GJ, Eisenhardt AE, Schmitt LC, Salek M, et al. Kidins220/ARMS associates with B-Raf and the TCR, promoting sustained Erk signaling in T cells. J Immunol. (2013) 190:1927–35. doi: 10.4049/jimmunol.1200653
16. Ohta A, Kini R, Ohta A, Subramanian M, Madasu M, Sitkovsky M. The development and immunosuppressive functions of CD4(+) CD25(+) FoxP3(+) regulatory T cells are under influence of the adenosine-A2A adenosine receptor pathway. Front Immunol. (2012) 3:190. doi: 10.3389/fimmu.2012.00190
17. Yuan G, Jankins TC, Patrick CG Jr, Philbrook P, Sears O, Hatfield S, et al. Fluorinated adenosine A2A receptor antagonists inspired by preladenant as potential cancer immunotherapeutics. Int J Med Chem. (2017) 2017:4852537. doi: 10.1155/2017/4852537
18. Kjaergaard J, Hatfield S, Jones G, Ohta A, Sitkovsky M. A2A adenosine receptor gene deletion or synthetic A2A antagonist liberate tumor-reactive CD8(+) T cells from tumor-induced immunosuppression. J Immunol. (2018) 201:782–91. doi: 10.4049/jimmunol.1700850
19. Schaffhausen J. GPCRs signal a Nobel Prize. Trends Pharmacol Sci. (2013) 34:1. doi: 10.1016/j.tips.2012.11.004
20. Zoli M, Agnati LF, Hedlund PB, Li XM, Ferre S, Fuxe K. Receptor-receptor interactions as an integrative mechanism in nerve cells. Mol Neurobiol. (1993) 7:293–334. doi: 10.1007/BF02769180
21. Marshall FH, Jones KA, Kaupmann K, Bettler B. GABAB receptors - the first 7TM heterodimers. Trends Pharmacol Sci. (1999) 20:396–9. doi: 10.1016/S0165-6147(99)01383-8
22. Franco R, Ferre S, Agnati L, Torvinen M, Gines S, Hillion J, et al. Evidence for adenosine/dopamine receptor interactions: indications for heteromerization. Neuropsychopharmacology. (2000) 23:S50–9. doi: 10.1016/S0893-133X(00)00144-5
23. Bouvier M. Oligomerization of G-protein-coupled transmitter receptors. Nat Rev Neurosci. (2001) 2:274–86. doi: 10.1038/35067575
24. Milligan G, White JH. Protein-protein interactions at G-protein-coupled receptors. Trends Pharmacol Sci. (2001) 22:513–8. doi: 10.1016/S0165-6147(00)01801-0
25. Fuxe K, Marcellino D, Leo G, Agnati LF. Molecular integration via allosteric interactions in receptor heteromers. A working hypothesis. Curr Opin Pharmacol. (2010) 10:14–22. doi: 10.1016/j.coph.2009.10.010
26. Borroto-Escuela DO, Correia PA, Romero-Fernandez W, Narvaez M, Fuxe K, Ciruela F, et al. Muscarinic receptor family interacting proteins: role in receptor function. J Neurosci Methods. (2011) 195:161–9. doi: 10.1016/j.jneumeth.2010.11.025
27. Fuxe K, Borroto-Escuela DO. Heteroreceptor complexes and their allosteric receptor-receptor interactions as a novel biological principle for integration of communication in the CNS: targets for drug development. Neuropsychopharmacology. (2016) 41:380–2. doi: 10.1038/npp.2015.244
28. Canals M, Burgueno J, Marcellino D, Cabello N, Canela EI, Mallol J, et al. Homodimerization of adenosine A2A receptors: qualitative and quantitative assessment by fluorescence and bioluminescence energy transfer. J Neurochem. (2004) 88:726–34. doi: 10.1046/j.1471-4159.2003.02200.x
29. Gherbi K, May LT, Baker JG, Briddon SJ, Hill SJ. Negative cooperativity across β1-adrenoceptor homodimers provides insights into the nature of the secondary low-affinity CGP 12177 β1-adrenoceptor binding conformation. FASEB J. (2015) 29:2859–71. doi: 10.1096/fj.14-265199
30. Kourrich S, Su TP, Fujimoto M, Bonci A. The sigma-1 receptor: roles in neuronal plasticity and disease. Trends Neurosci. (2012) 35:762–71. doi: 10.1016/j.tins.2012.09.007
31. Dahoun T, Trossbach SV, Brandon NJ, Korth C, Howes OD. The impact of Disrupted-in-Schizophrenia 1 (DISC1) on the dopaminergic system: a systematic review. Transl Psychiatry. (2017) 7:e1015. doi: 10.1038/tp.2016.282
32. Borroto-Escuela DO, Fuxe K. On the G protein-coupled receptor neuromodulation of the claustrum. Neurochem Res. (2019). doi: 10.1007/s11064-019-02822-4. [Epub ahead of print].
33. Fuxe K, Borroto-Escuela DO. Understanding receptor heteromerization and its allosteric integration of signals. Neuropharmacology. (2019) 152:1–3. doi: 10.1016/j.neuropharm.2019.05.001
34. Liu F, Wan Q, Pristupa ZB, Yu XM, Wang YT, Niznik HB. Direct protein-protein coupling enables cross-talk between dopamine D5 and gamma-aminobutyric acid A receptors. Nature. (2000) 403:274–80. doi: 10.1038/35002014
35. Liu XY, Chu XP, Mao LM, Wang M, Lan HX, Li MH, et al. Modulation of D2R-NR2B interactions in response to cocaine. Neuron. (2006) 52:897–909. doi: 10.1016/j.neuron.2006.10.011
36. Flajolet M, Wang Z, Futter M, Shen W, Nuangchamnong N, Bendor J, et al. FGF acts as a co-transmitter through adenosine A(2A) receptor to regulate synaptic plasticity. Nat Neurosci. (2008) 11:1402–9. doi: 10.1038/nn.2216
37. Borroto-Escuela DO, Romero-Fernandez W, Mudo G, Perez-Alea M, Ciruela F, Tarakanov AO, et al. Fibroblast growth factor receptor 1- 5-hydroxytryptamine 1A heteroreceptor complexes and their enhancement of hippocampal plasticity. Biol Psychiatr. (2012) 71:84–91. doi: 10.1016/j.biopsych.2011.09.012
38. Di Liberto V, Borroto-Escuela DO, Frinchi M, Verdi V, Fuxe K, Belluardo N, et al. Existence of muscarinic acetylcholine receptor (mAChR) and fibroblast growth factor receptor (FGFR) heteroreceptor complexes and their enhancement of neurite outgrowth in neural hippocampal cultures. Biochim Biophys Acta Gen Subj. (2017) 1861:235–45. doi: 10.1016/j.bbagen.2016.10.026
39. Bogan AA, Thorn KS. Anatomy of hot spots in protein interfaces. J Mol Biol. (1998) 280:1–9. doi: 10.1006/jmbi.1998.1843
40. Borroto-Escuela DO, Rodriguez D, Romero-Fernandez W, Kapla J, Jaiteh M, Ranganathan A, et al. Mapping the interface of a GPCR Dimer: a structural model of the A2A adenosine and D2 dopamine receptor heteromer. Front Pharmacol. (2018) 9:829. doi: 10.3389/fphar.2018.00829
41. Borroto-Escuela DO, Tarakanov AO, Brito I, Fuxe K. Glutamate heteroreceptor complexes in the brain. Pharmacol Rep. (2018) 70:936–50. doi: 10.1016/j.pharep.2018.04.002
42. Owen DM, Magenau A, Williamson DJ, Gaus K. Super-resolution imaging by localization microscopy. Methods Mol Biol. (2013) 950:81–93. doi: 10.1007/978-1-62703-137-0_6
43. Ward RJ, Pediani JD, Godin AG, Milligan G. Regulation of oligomeric organization of the serotonin 5-hydroxytryptamine 2C (5-HT2C) receptor observed by spatial intensity distribution analysis. J Biol Chem. (2015) 290:12844–57. doi: 10.1074/jbc.M115.644724
44. Jonas KC, Huhtaniemi I, Hanyaloglu AC. Single-molecule resolution of G protein-coupled receptor (GPCR) complexes. Methods Cell Biol. (2016) 132:55–72. doi: 10.1016/bs.mcb.2015.11.005
45. Pediani JD, Ward RJ, Marsango S, Milligan G. Spatial intensity distribution analysis: studies of G protein-coupled receptor oligomerisation. Trends Pharmacol Sci. (2018) 39:175–86. doi: 10.1016/j.tips.2017.09.001
46. Agnati LF, Fuxe K, Zoli M, Rondanini C, Ogren SO. New vistas on synaptic plasticity: the receptor mosaic hypothesis of the engram. Med Biol. (1982) 60:183–90.
47. Palczewski K, Kumasaka T, Hori T, Behnke CA, Motoshima H, Fox BA, et al. Crystal structure of rhodopsin: a G protein-coupled receptor. Science. (2000) 289:739–45. doi: 10.1126/science.289.5480.739
48. Cabello N, Gandia J, Bertarelli DC, Watanabe M, Lluis C, Franco R, et al. Metabotropic glutamate type 5, dopamine D2 and adenosine A2a receptors form higher-order oligomers in living cells. J Neurochem. (2009) 109:1497–507. doi: 10.1111/j.1471-4159.2009.06078.x
49. Borroto-Escuela DO, Narvaez M, Marcellino D, Parrado C, Narvaez JA, Tarakanov AO, et al. Galanin receptor-1 modulates 5-hydroxtryptamine-1A signaling via heterodimerization. Biochem Biophys Res Commun. (2010) 393:767–72. doi: 10.1016/j.bbrc.2010.02.078
50. Chruscicka B, Wallace Fitzsimons SE, Borroto-Escuela DO, Druelle C, Stamou P, Nally K, et al. Attenuation of oxytocin and serotonin 2A receptor signaling through novel heteroreceptor formation. ACS Chem Neurosci. (2019) 10:3225–40. doi: 10.1021/acschemneuro.8b00665
51. Cervetto C, Venturini A, Passalacqua M, Guidolin D, Genedani S, Fuxe K, et al. A2A-D2 receptor-receptor interaction modulates gliotransmitter release from striatal astrocyte processes. J Neurochem. (2017) 140:268–79. doi: 10.1111/jnc.13885
52. Navarro G, Reyes-Resina I, Rivas-Santisteban R, Sanchez De Medina V, Morales P, Casano S, et al. Cannabidiol skews biased agonism at cannabinoid CB1 and CB2 receptors with smaller effect in CB1-CB2 heteroreceptor complexes. Biochem Pharmacol. (2018) 157:148–58. doi: 10.1016/j.bcp.2018.08.046
53. Navarro G, Varani K, Reyes-Resina I, Sanchez De Medina V, Rivas-Santisteban R, Sanchez-Carnerero Callado C, et al. Cannabigerol action at cannabinoid CB1 and CB2 receptors and at CB1-CB2 heteroreceptor complexes. Front Pharmacol. (2018) 9:632. doi: 10.3389/fphar.2018.00632
54. Huang S, Apasov S, Koshiba M, Sitkovsky M. Role of A2a extracellular adenosine receptor-mediated signaling in adenosine-mediated inhibition of T-cell activation and expansion. Blood. (1997) 90:1600–10.
55. Fuxe K, Borroto-Escuela DO, Romero-Fernandez W, Diaz-Cabiale Z, Rivera A, Ferraro L, et al. Extrasynaptic neurotransmission in the modulation of brain function. Focus on the striatal neuronal-glial networks. Front Physiol. (2012) 3:136. doi: 10.3389/fphys.2012.00136
56. Fuxe K, Agnati LF, Marcoli M, Borroto-Escuela DO. Volume transmission in central dopamine and noradrenaline neurons and its astroglial targets. Neurochem Res. (2015) 40:2600–14. doi: 10.1007/s11064-015-1574-5
57. Borroto-Escuela DO, Hinz S, Navarro G, Franco R, Muller CE, Fuxe K. Understanding the role of adenosine A2AR heteroreceptor complexes in neurodegeneration and neuroinflammation. Front Neurosci. (2018) 12:43. doi: 10.3389/fnins.2018.00043
58. Hinz S, Navarro G, Borroto-Escuela D, Seibt BF, Ammon YC, De Filippo E, et al. Adenosine A2A receptor ligand recognition and signaling is blocked by A2B receptors. Oncotarget. (2018) 9:13593–611. doi: 10.18632/oncotarget.24423
59. Isik N, Hereld D, Jin T. Fluorescence resonance energy transfer imaging reveals that chemokine-binding modulates heterodimers of CXCR4 and CCR5 receptors. PLoS ONE. (2008) 3:e3424. doi: 10.1371/journal.pone.0003424
60. Hammad MM, Kuang YQ, Yan R, Allen H, Dupre DJ. Na+/H+ exchanger regulatory factor-1 is involved in chemokine receptor homodimer CCR5 internalization and signal transduction but does not affect CXCR4 homodimer or CXCR4-CCR5 heterodimer. J Biol Chem. (2010) 285:34653–64. doi: 10.1074/jbc.M110.106591
Keywords: A2AR-TCR heteroreceptor complexes, allosteric receptor-receptor interactions, immunosuppression, T cell, cancer immunotherapy
Citation: Borroto-Escuela DO and Fuxe K (2019) Can Allosteric Receptor-Protein Interactions in Receptor Complexes Be a Molecular Mechanism Involved in Cancer Immune Therapy? Front. Endocrinol. 10:574. doi: 10.3389/fendo.2019.00574
Received: 02 April 2019; Accepted: 06 August 2019;
Published: 20 August 2019.
Edited by:
Steven G. Gray, St. James's Hospital, IrelandReviewed by:
Leigh Stoddart, University of Nottingham, United KingdomPierre De Meyts, Catholic University of Louvain, Belgium
Copyright © 2019 Borroto-Escuela and Fuxe. This is an open-access article distributed under the terms of the Creative Commons Attribution License (CC BY). The use, distribution or reproduction in other forums is permitted, provided the original author(s) and the copyright owner(s) are credited and that the original publication in this journal is cited, in accordance with accepted academic practice. No use, distribution or reproduction is permitted which does not comply with these terms.
*Correspondence: Kjell Fuxe, S2plbGwuRnV4ZUBraS5zZQ==