- 1Department of Medical Oncology, The Second Affiliated Hospital, Zhejiang University School of Medicine, Hangzhou, China
- 2Key Laboratory of Tumor Microenvironment and Immune Therapy of Zhejiang Province, The Second Affiliated Hospital, Zhejiang University School of Medicine, Hangzhou, China
- 3Cancer Institute, Key Laboratory of Cancer Prevention and Intervention, Ministry of Education, The Second Affiliated Hospital, Zhejiang University School of Medicine, Hangzhou, China
- 4Department of Orthopedics Surgery, The Second Affiliated Hospital, Zhejiang University School of Medicine, Hangzhou, China
- 5Department of Breast Surgery and Oncology, The Second Affiliated Hospital, Zhejiang University School of Medicine, Hangzhou, China
Breast cancer (BC) is one of the most common malignancies and the leading cause of cancer-related mortality in women. Androgen receptor (AR) is frequently expressed in diverse BC subtypes. Accumulating evidence has revealed that AR might be a predictive or prognostic factor and a drug target in BC. AR expression and AR pathways differ in various BC subtypes, thereby resulting in controversial inferences on the predictive and prognostic value of AR. Herein, we summarized the roles of AR in different BC subtypes and AR-targeting therapies based on preclinical and clinical studies. Moreover, we highlighted the possible efficacy of a combination therapy via exploiting the AR-related mechanisms and the research on therapeutic resistance.
Introduction
Breast cancer (BC) represents the most common malignancy in women, and is one of the leading causes of cancer-related fatality (11.6% of the total cancer deaths) (1). BC has different subtypes that are usually classified depending on the expression of the estrogen receptor (ER), progesterone receptor (PR), and human epidermal growth factor receptor 2 (HER2). ER-positive (ER+) BC is the most common subtype, comprising 70% of all BC cases (2, 3), while HER2-positive (HER2+) BC accounts for 20–25% (4). Although such patients can benefit from treatment targeting the ER or HER2, ER+ BC frequently acquires resistance to endocrine therapy (5). Triple-negative BC (TNBC), which lacks the expression of ER, PR, and HER2, constitutes 15–20% of BC cases, and has the highest probability of metastasis and the lowest overall survival (OS) among all BC subtypes (6, 7). Currently, there is no well-defined targeted therapy for TNBC (8). Given the urgency in the development of effective BC treatment strategies, it is essential to identify new markers or potential alternative therapeutic targets for this disease in order to predict prognosis, diminish drug resistance, and improve clinical outcomes.
Androgen receptor (AR), a member of the steroid receptor superfamily, is expressed in many human tissues, among which BC tissue has the third-highest expression of AR (8, 9). It has been noted that 70–90% of BC patients overexpress the AR with several studies indicating that AR might be a predictive or prognostic factor and a drug target in BC (10). Recently, the implication of AR in various stages of BC gathered significant attention. Although there have been several reviews discussing the physiology of AR, AR-related mechanisms, and AR-targeting treatments, its function appears to vary among the diverse BC subtypes, and its prognostic and predictive value in BC patients remains controversial (11, 12).
In this review, we focused on the roles and prognostic significance of AR and AR-targeting therapies in different BC subtypes based on preclinical and clinical studies. We also summarized the possibility of combination treatment and research on therapeutic resistance.
Methods
We performed a comprehensive search of the PubMed database for articles written in English and published before January 2020 (mainly after 2010), using the following search terms, alone or in combination: “androgen receptor,” “androgen,” “breast cancer,” “prognosis,” “androgen receptor agonist or antagonist,” and “resistance.” In addition, bibliographies of relevant articles were searched for additional references. The website ClinicalTrials.gov was searched for ongoing clinical trials.
Roles of AR in BC
AR is a 919-amino-acid hormone-regulated transcription factor. The gene responsible for AR measures 180 kb in length and is located on the chromosome, Xq11-12 (13). AR comprises four distinct functional domains: (i) an N-terminal domain (NTD), in charge of transcriptional activity; (ii) a DNA-binding domain (DBD) that primarily recognizes specific androgen response elements (AREs); (iii) a hinge region for nuclear localization, and (iv) a ligand-binding domain (LBD) for androgen binding (14). Androgens are steroid hormones that regulate AR transcriptional activity via AR binding (15). However, among the androgens, only testosterone (T) and dihydrotestosterone (DHT) can bind the AR (15, 16). In the breast tissue, T is converted either to DHT by 5α-reductase or to 17β-estradiol (E2) by aromatase (17). Interestingly, DHT concentrations were significantly (3-fold) higher, whereas 5α-reductase levels were elevated 4- to 8-fold in breast carcinoma tissues compared with those in non-neoplastic breast tissues (16). Of note, T is converted into estradiol only when the estrogen levels are very low (15, 17).
AR is situated in the cytoplasm, and without the ligand, it adheres to heat shock proteins (HSPs) (14). Once androgens enter the cell, they combine with the AR resulting in conformational changes that enable its dimerization. Subsequently, AR is isolated from HSPs and transferred to the nucleus. In the nucleus, the DBD attaches to AREs within the target gene and recruits additional co-stimulators, co-repressors, and transcriptional regulators that positively or negatively influence gene transcription, thus altering apoptosis, differentiation, angiogenesis, or proliferation (18, 19). AR activity can be modulated by several key proteins and signaling pathways, such as Wnt/β-catenin, PI3K/AKT pathways, phosphatase and tensin homolog deleted on chromosome 10 (PTEN), forkhead box protein A1 (FOXA1), G-protein coupled estrogen receptor (GPER), as well as long non-coding RNA (lncRNA). Moreover, the action of AR is also associated with ER and HER2 signaling pathways (20–25). The crosstalk between AR and other pathways or proteins is described in detail in the following sections and depicted in Figure 1.
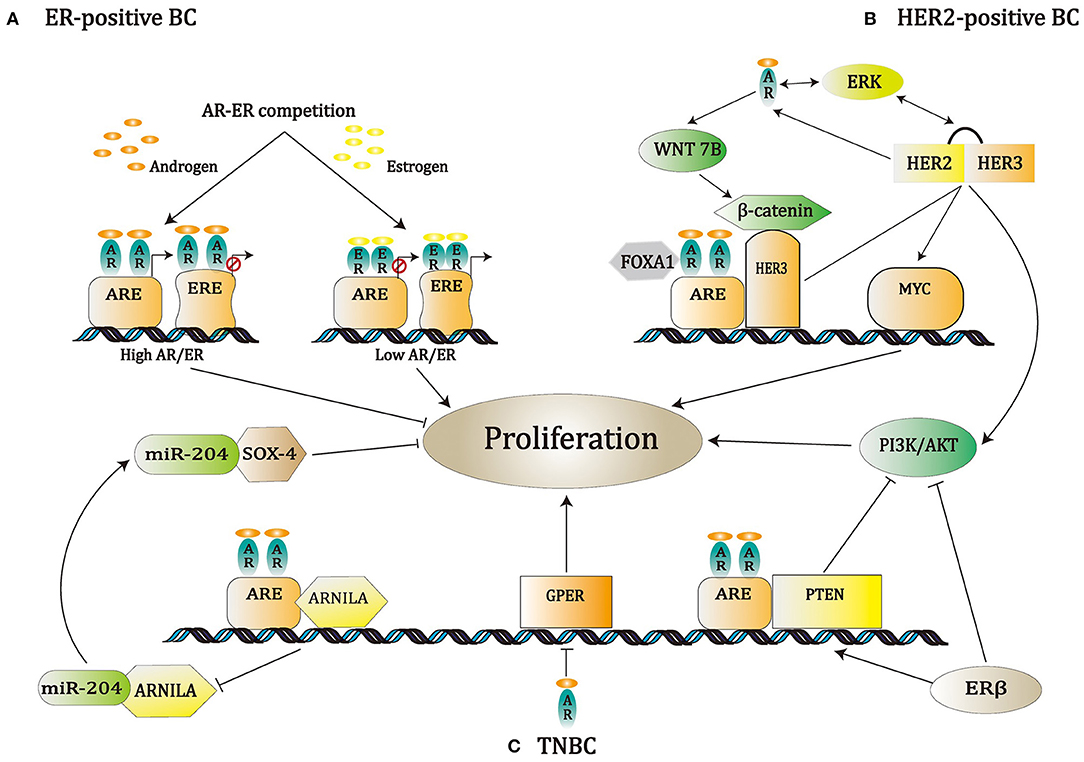
Figure 1. A schematic of known pathways regulated by AR in various breast cancer subtypes. (A) In ER-positive BC, it has been found that AR influenced the ER pathway by competition with ER for attaching to the modulatory regions of ER genes; meanwhile, the AR/ER expression ratio makes a critical difference in the regulation of BC cell proliferation. (B) In HER2-positive BC, the Wnt/β-catenin signaling pathway is up-regulated by the AR. AR/β-catenin complex, together with FOXA1, induces HER3 gene transcription. Next, HER3 and HER2 create a heterodimer, thereby stimulating the PI3K/AKT pathway and MYC gene expression. Additionally, ERK, AR, and HER2 take part in a positive feedback cycle. (C) In TNBC, the up-regulation of PTEN through AR or ERβ represses PI3K action, which in turn reduces AR activity. Suppression of GPER by AR can also modulate cell behavior positively, while suppression of ARNILA results in negative modulation.
AR in ER-Positive BC
In ER-positive BC, AR has been demonstrated to interfere with ER-dependent transcription through competition with the ER for binding sites at the estrogen response elements (EREs) or through competition for the transcriptional co-modulators (23). Indeed, the AR/ER expression rate determines the impact of both AR and ER in the regulation of BC cell proliferation. When the expression level of AR is higher than that of ER, the AR binds to the EREs, thereby exerting inhibitory effects on cell growth. In contrast, if the ER is more abundant than the AR, then ER binds to AREs, leading to cell proliferation (Figure 1) (15, 26–28).
AR in HER2-Positive BC
In HER2-positive BC, AR transcriptionally regulates the levels of WNT7B that leads to the transfer of β-catenin into the nucleus. In the nucleus, the AR/β-catenin complex identifies the modulatory regions of HER3 and raises its transcription under the cooperation of the transcription factor, FOXA1. HER3 and HER2 form a heterodimer that stimulates MYC gene expression and PI3K/AKT pathway, thereby resulting in cell proliferation and tumor growth (Figure 1) (29). The synergism between AR and HER2 is boosted via the mechanism in which HER2 promotes AR transcription and leads to ERK activation that, in turn, regulates both HER2 and AR, resulting in a positive feedback loop (Figure 1) (24).
AR in TNBC
It is known that the PI3K/AKT/mTOR pathway is involved in BC development (30). In TNBC, the frequency of PIK3CA mutations in AR+ tumors was higher than that in AR-negative tumors (31). Androgen up-regulates PTEN transcriptional expression when AR binds to AREs in the PTEN upstream promoter. Subsequently, PTEN restrains PI3K action, which in turn weakens AR activity. Nevertheless, in prostate cancer, AR binds to the PTEN promoter as a repressor, thereby inhibiting its transcription (32). Elevated PIK3CA protein activity in AR+ TNBC (through amplification and mutation) will bypass the AR-mediated PTEN up-regulation so that targeting AR alone may promote tumor growth by lowering PTEN expression and motivating the PI3K pathway. Therefore, dual targeting of the AR and PI3K may produce a synergistic anti-tumor effect (31).
ERβ, a second form of estrogen receptor, is found in ~30% of TNBC cases (33). ERβ overexpression can decrease AR activation by the up-regulation of PTEN and the suppression of the PI3K/AKT signaling pathway (34, 35). GPER is a member of the G-protein coupled receptor (GPCR) family, which is primarily expressed in TNBC and reportedly regulates the development of various cancer types (36). Notably, the activation of AR may promote the proliferation of TNBC by down-regulating GPER (25).
Additionally, in TNBC, it was found that AR could adjust the cell behavior via modulating the interaction between microRNAs and lncRNAs. The AR negatively induced lncRNA (ARNILA) was reported to serve as a competitive endogenous RNA of miR-204 that promotes the expression of its target gene, Sox4, which boosts epithelial–mesenchymal transition (EMT), invasion, and metastasis of TNBC. Therefore, ARNILA is associated with poor progression in TNBC (22).
The Clinical Relevance of AR in BC
AR can be detected in most BCs, and the frequency of its positivity differs between different BC subtypes and ethnicities (10, 37–42). Numerous studies exploring the prognostic value of AR in BC have been presented with contentious results (Table 1); in fact, the AR effect depends on tumor subtypes or patient populations.
ER-Positive BC
AR is expressed in 70–95% of ER+ BCs (10, 37, 38). AR has been noted to be correlated with favorable outcomes, such as smaller tumor size, lower tumor grade, less necrosis, lower Ki-67 levels, and better treatment response in ER+ BC (39, 57–59). Recently, a large study including 4,147 pre- and post-menopausal women with invasive BC from the Nurses' Health Study cohorts showed that AR expression, independent of clinicopathological characteristics, was related to the improvement of BC-specific survival in ER+ BC patients in the first 5–10 years post-diagnosis (43). A meta-analysis by Bozovic-Spasojevic et al. employing univariate and multivariate analyses, uncovered that AR expression results in significantly improved disease-free survival (DFS) and OS in ER+ BCs (44). In one study using transcriptomic data obtained from TCGA and METABRIC cohorts, high AR levels in ER+ BC correlated with fewer tumor-infiltrating lymphocytes and cytolytic activity, as well as far less sensitivity to neoadjuvant chemotherapy, yet better survival (45). However, a study of 3,021 postmenopausal women with early-stage ER+ BC showed that AR expression was not linked with the prognosis, nor could it predict the response to letrozole or tamoxifen (46).
On the contrary, the AR/ER ratio has been considered to affect the prognosis and response to anti-estrogen endocrine therapy. Cochrane et al. revealed that ER+ BC patients with an AR/ER ratio > 2, had a 4-fold increased risk of failure with tamoxifen therapy (47).
HER2-Positive BC
Approximately 30–60% of HER2+ BCs overexpress the AR (10, 40). Naturally, HER2+ BC patients show poorer prognosis compared to HER2-negative BC patients (60). However, when HER2, ER, and AR were all expressed, the tumor acted with a weak invasive phenotype, and the patient had a superior outcome (61). A study comprising of 304 HER2-enriched metastatic BC (MBC) cases, considered that AR positivity was an independent prognostic marker for progression-free survival (PFS) (HR = 0.71, P = 0.039) and OS (HR = 0.53, P = 0.013). Besides, patients treated with first-line trastuzumab, AR+ tumors had longer PFS (15.8 vs. 8.2 months, P = 0.005) and 5-year OS rate (66.2 vs. 26.2%, P = 0.009) compared with AR-negative subjects (48). In addition, a study involving 111 operated patients with BC revealed no significant correlations between AR expression and prognostic values in the HER2+ group (49). On the contrary, a notable finding of a meta-analysis, including three studies with 358 patients, revealed the worse clinical outcome conferred by AR expression in patients with HER2+ER-(Her2-enriched) BC (44).
TNBC
In TNBC cases, the expression of AR is 10–53% (39–41); however, the prognostic value of AR continues to be disputable. For instance, an analysis of the immunohistochemical results in 190 TNBC patients demonstrated markedly preferable prognosis (P = 0.019) in those with AR+ subtypes than that in those with AR-negative subtypes (50). Another similar analysis of 88 TNBC patients revealed that higher expression of AR was dramatically related to a prolonged PFS (HR = 0.12; P = 0.011) (22). Besides, a retrospective analysis showed that the AR status could be used to identify groups of ER-negative BC patients benefiting from adjuvant tamoxifen therapy. In ER-negative BC patients, AR expression predicted reduced recurrence rate with tamoxifen; even in TNBC, patients with AR+ tumors showed an improved outcome when treated with tamoxifen (51).
However, in a meta-analysis of 27 studies, including 4,914 TNBC patients, AR expression was not related to DFS, OS, distant DFS, or recurrence-free survival (52). Moreover, a recent study about peculiar clinical groups, including TNBC patients treated with or without radiation, showed a noticeable correlation between AR expression and locoregional recurrence only in patients who had radiation therapy, suggesting that AR expression might be a marker predicting the response to radiotherapy in TNBC (53). In addition, compared with the primary tumor, AR gene expression increased in circulating tumor cells and early lung metastases, indicating that AR may promote the spread of metastasis by supporting the survival of BC cells during metastasis (62). Several retrospective studies demonstrated that AR+ TNBC patients had an inferior response to chemotherapy and a lower opportunity of achieving a pathological complete response to neoadjuvant chemotherapy (54, 63).
A multi-institutional study of 1,407 TNBC patients from six international cohorts found that AR status presents population-specific patterns related to OS. AR positivity is a biomarker of favorable prognosis in the Nigerian and US cohorts, whereas it correlated with poor prognosis in the Indian, Norway, and Ireland cohorts, while being neutral in the UK cohort (55).
To some extent, the prognostic discrepancy mentioned above may be owing to differences in sample sizes, the methodology of detection, the antibody used to test AR, the cut-off values used to define AR positivity, the ethnic composition of cohorts, adjuvant treatments, and follow-up time of studies (55, 64, 65).
AR-Related Therapies in BC
AR-Targeted Monotherapy
Natural and synthetic androgens have been used as a treatment approach in BC with AR expression (12, 66, 67); however, they have been known to induce many side effects (68). The new selective-AR modulators (SARM), as AR agonists, can solve this problem (69). Moreover, AR antagonists have also been investigated extensively in previous studies. The first-generation non-steroidal AR antagonist, bicalutamide, blocks DBD conjugating with the AREs (70, 71). Moreover, bicalutamide possesses partial agonist effects (72). Patients resistant to bicalutamide usually can respond to enzalutamide, a second-generation AR antagonist, which has better anti-tumor efficacy than bicalutamide, because of its higher affinity for AR, ability to inhibit nuclear translocation, gene binding, and recruitment of coregulators (73, 74). Unfortunately, there have been reports of adverse events related to the central nervous system and resistance to enzalutamide (75). Darolutamide (ODM-201) is a novel AR antagonist, which enables the interruption of the tested mutant AR activity in anti-androgen treatments, especially a mutation of F876L in the LBD that is recognized as resistant to enzalutamide; it does not increase serum testosterone levels and exhibits negligible brain penetrance (76). Other novel AR antagonists are under development. For example, orteronel (TAK-700), VT-464 (Viamet), and abiraterone acetate, all CYP17A inhibitors that interrupt the synthesis of androgens through suppressing 17,20-lyase or 17α-hydroxylase activity, are currently under investigation for their therapeutic efficacy in BC (56, 77).
ER-Positive BC
So far, androgens and AR agonists have been explored in ER+ BC. A retrospective analysis of ER+ MBC patients revealed that the clinical benefit rate (CBR) of fluoxymesterone was 43% after progressing to conventional hormonal therapy, independent of the AR expression level (67). Enobosarm (GTx-024), one of the new SARM, has shown promising results in the case of an extensively pretreated woman with metastatic ER+/PR+/HER2–BC with high AR co-expression who reached partial radiographic response after four cycles of treatment with enobosarm (69). Currently, a phase II clinical trial investigating enobosarm for locally advanced or metastatic ER+ and AR+ BC patients is under way (NCT02463032) (Table 2).
ER-Negative BC
The AR-directed antagonists are mostly used in advanced BC, especially TNBC (12). Preclinical studies detected bicalutamide to inhibit proliferation and increase apoptosis in AR+/ER-negative BC cell lines, and even in the MSL TNBC BC cells, Hs578T and MDA-MB-231, which express relatively low levels of AR (9, 78). A phase II clinical trial comprising 424 ER/PR-negative BC patients, showed the CBR at 6 months of bicalutamide treatment was 19% and a median PFS duration of 12 weeks but no objective responses (79). This trial demonstrated, for the first time, the efficacy of AR-targeted therapy with advanced AR+ TNBC. Currently, there is an ongoing phase III clinical study comparing the effectiveness of bicalutamide with conventional chemotherapy as the first-line treatment of AR+ metastatic TNBC (NCT03055312) (Table 2).
Furthermore, a large preclinical study showed that enzalutamide significantly reduced proliferation, migration, and invasion, while increasing apoptosis in 11 BC cell lines (three non-TN and eight TNBC); moreover, growth inhibition was dependent on the expression of AR, which is consistent with the findings of another research that involved four different non-LAR TNBC cell lines of the MSL, BL2, and mesenchymal-like subtypes demonstrating enzalutamide-mediated cell inhibition (80, 81). A phase II clinical trial of locally advanced or metastatic AR+ TNBC patients showed that the CBR of enzalutamide was 33% at 16 weeks and 28% at 24 weeks, median PFS duration was 3.3 months, and the median OS was 17.6 months in the evaluable subgroup (NCT01889238) (Table 2) (82). Based on these encouraging results, enzalutamide is currently undergoing evaluation for its efficacy in early AR+ TNBC in a phase II clinical trial (NCT02750358) (Table 2).
Beyond that, a randomized phase II study in locally recurrent (unresectable) or metastatic AR+ TNBC treated with darolutamide or capecitabine is currently recruiting patients (NCT03383679) (Table 2) (83). Another phase II trial presented encouraging results in patients with molecular apocrine-like tumors treated with abiraterone acetate plus prednisone (NCT01842321) (Table 2) (84), and the clinical trial is still ongoing.
Combination of AR-Targeted Therapy With Other Therapies
Aside from monotherapy approaches, the combination of AR-targeted therapy with other therapies as new potential strategies has gathered considerable interest. Previous studies have revealed that in AR+ TNBC, PIK3CA kinase mutations were more common and that targeting AR alone could potentially enhance tumor growth (31). A study demonstrated that the combination of bicalutamide with GDC-0941 or GDC-0980 (pan-PI3K or dual PI3K/mTOR inhibitor) had additive effects in cell line models and xenograft tumors (31). When enzalutamide plus the mTOR inhibitor, everolimus or anti-HER2 monoclonal antibody, trastuzumab were used to treat TNBC and HER2+ cell lines, it resulted in synergistic inhibition of proliferation (85). Lehmann et al. found that the combination of enzalutamide with taselisib, a PI3K inhibitor, significantly increased the CBR of advanced TNBC patients, and was ~35.7%, compared to those treated with enzalutamide alone in a phase IB/II clinical trial (NCT02457910) (Table 2) (86). Interestingly, one patient achieved a partial response to the combination after progressing to enzalutamide alone (86), indicating that combinatorial strategies may be needed for AR+ TNBC patients to obtain a significant clinical benefit in the future.
Cyclin-dependent kinases (CDKs) are a family of protein kinases that play a crucial role in eukaryotic cell proliferation. CDK4 and CDK6, members of the CDK family, are activated by cyclin D and induce the transition from G1 phase to S phase in cell proliferation (87). Palbociclib, a highly selective CDK4/6 inhibitor, combined with enzalutamide cemented the cellular inhibition of AR+/Rb-proficient (MDA-MB-453) TNBC cells, which provided a preclinical rationale for the selection of patients who may benefit from the combined treatment of CDK4/6 inhibitors and AR antagonists (88). At present, a phase I/II trial (NCT02605486) (Table 2) is ongoing to explore the therapeutic effect of combining palbociclib and bicalutamide in metastatic AR+ TNBC.
In the studies involving BC, little research has been done on the association between AR and immune response. In a previous study on prostate cancer, increased PD-L1/2-positive dendritic cells and PD-1-positive T cells were found in the blood of patients progressing to enzalutamide, compared to those naïve or responding to enzalutamide, suggesting that the combination of enzalutamide and anti-programmed death-1 therapy may improve the prognosis in patients with enzalutamide resistance (89). Meanwhile, there is an ongoing clinical trial (NCT02971761) (Table 2) integrating AR-targeted therapy with immunotherapy. In the trial, GTx-024 (SARM) and pembrolizumab are used to treat patients with AR+ metastatic TNBC.
Furthermore, AR antagonists have been combined with conventional therapies, such as radiotherapy and chemotherapy, with agreeable results. For example, Barton et al. in a preclinical model, reported that the combination of enzalutamide with paclitaxel (simultaneous and subsequent) was more effective than paclitaxel alone in reducing tumor recurrence (90). There are several ongoing clinical trials combining chemotherapy and anti-androgen therapy, which are included as neoadjuvant therapy (NCT02689427) (Table 2). As mentioned above, AR positivity was most dramatically correlated with worse local recurrence-free survival after radiotherapy, while enzalutamide significantly radiosensitized AR+ TNBC cell lines and xenograft tumors (53). Besides, there is an ongoing phase II clinical trial (NCT02091960) (Table 2) evaluating the efficacy of enzalutamide combined with trastuzumab in HER2/AR-positive locally advanced or metastatic BC. Several clinical trials currently exploring AR-targeted therapy as mono- or multi-therapy are listed in Table 2.
Mechanisms of Resistance to AR Antagonists
While the results of AR-targeted therapies for BC are promising, some patients eventually show disease progression due to drug resistance. The underlying mechanisms of drug resistance in BC are still being explored, while those identified in prostate cancer may also apply to BC. In prostate cancer, the failure of AR-targeted therapy is related to AR gene amplification and mutation, interaction among coactivators, and overexpression of active AR splice variants, such as AR-V7 lacking the C-terminal LBD (91–96). Under the treatment of AR antagonists, overexpression, and structural mutation of AR make the receptor more sensitive to low levels of androgen and convert antagonist responses to agonistic ones (97–100). Different AR inhibitors may follow different resistance mechanisms. Recent studies found that the mutations of W741L and T877A mediate resistance to bicalutamide and hydroxyflutamide, respectively. A missense mutation of F876L in the LBD of AR was associated with the resistance to enzalutamide (76, 99–101).
Analysis of various splice variants revealed that 53.7% of primary BC samples had AR variant 7 (AR-V7) mutations (102). Interestingly, AR-V7, as AR splice variant in prostate cancer, is the most extensively researched among tumors and circulating tumor DNAs (103); it is widely accepted that monitoring and inhibiting AR-V7 is a clinical priority in prostate cancer treatment (104). Kohli et al. found that the AR variant 9 (AR-V9) is regularly co-expressed with AR-V7. Furthermore, high pre-therapy expression of AR-V9 mRNA in prostate cancer metastases was associated with the resistance to abiraterone acetate, indicating that AR-V9 might be a potential therapeutic target for drug resistance (104).
Moreover, recent studies have shown that the aberrant activation of the EMT and Wnt/β-catenin pathway also contributes to enzalutamide resistance in prostate cancer (105–107).
Conclusions and Future Directions
AR is a burgeoning and promising therapeutic target that potentially improves the survival outcome of BC patients. This review emphasizes the role of AR and AR-related mechanisms in various BC subtypes from bench to bedside. However, additional researches are needed to elucidate the significance of AR, in particular, identification of appropriate AR detection methods and novel markers of AR responsiveness to better select the patients who may benefit from AR-related treatments. Up till now, there is no clinical guideline concerning the methods to test AR positivity. Immunohistochemistry (IHC) is the cheapest method and is widely used in the definition of AR positivity in BC (12). The optimal cut-off point has not yet been identified; the discrepancy rate of AR expression between primary BC and metastatic tissue, and the influence of multiple antibodies on AR status, make it hard to explain the role of AR or select patients for AR-targeted therapy according to the expression data obtained by IHC (108, 109). The characteristics of circulating tumor cells (CTCs) in blood, which are easily accessible through a simple venipuncture, may be a valuable alternative method to identify the AR status, especially in a metastatic tumor (110, 111). Hence, the differential expression of AR in patients with BC can be assessed at any time during follow-up and treatment (112). Of note, BC CTCs can also be used to evaluate the expression of AR-V7, as in prostate cancer, which may be a potential indicator for predicting the efficacy of abiraterone and enzalutamide (12, 103). In addition to expressing the AR protein, AR gene expression signatures, and AR phosphorylation status may be a predictor to define eligible patients for AR-targeted therapies (67, 113). Given the evidences reviewed herein, the combination of AR-targeted therapies with other therapies may improve the efficacy of BC treatment and hence, need to be explored further for their clinical effectiveness.
Author Contributions
FQ and JH: conception and design. MC, YY, and KX: write, review, and revision of the manuscript. LL: supervision. All authors contributed to the article and approved the submitted version.
Funding
This work was supported by grants from the National Natural Science Foundation of China (81472640, 81672802, FQ) and the Natural Science Foundation of Zhejiang Province (LZ17H1600041, FQ).
Conflict of Interest
The authors declare that the research was conducted in the absence of any commercial or financial relationships that could be construed as a potential conflict of interest.
Abbreviations
BC, breast cancer; ER, estrogen receptor; PR, progesterone receptor; HER2, human epidermal growth factor receptor 2; AR, androgen receptor; AR+, AR-positive; HER2+, HER2-positive; TNBC, triple-negative breast cancer; NTD, N-terminal domain; DBD, DNA-binding domain; LBD, ligand-binding domain; ARE, androgen response element; HSPs, heat shock proteins; ERE, estrogen response element; AKT, serine/threonine-protein kinase; FOXA1, forkhead box protein A1; mTOR, mammalian target of rapamycin; PTEN, phosphatase and tensin homolog; PI3K, phosphoinositide 3-kinase; GPER, G-protein coupled estrogen receptor; GPCR, G-protein coupled receptor; ARNILA, AR negatively-induced lncRNA; lncRNA, long non-coding RNA; EMT, epithelial–mesenchymal transition; DFS, disease-free survival, MBC, metastatic breast cancer; CBR, clinical benefit rate; PFS, progression-free survival; OS, overall survival; LAR, luminal androgen receptor; MSL, mesenchymal stem– like; BL2, basal-like 2; RB, retinoblastoma; PCR, pathologic complete response; CR or PR, complete response or partial response; RCB-I, residual cancer burden-index; CTCs, circulating tumor cells.
References
1. Bray F, Ferlay J, Soerjomataram I, Siegel RL, Torre LA, Jemal A. Global cancer statistics 2018: globocan estimates of incidence and mortality worldwide for 36 cancers in 185 countries. CA Cancer J Clin. (2018) 68:394–424. doi: 10.3322/caac.21492
2. Jeselsohn R. Are we ready to use Esr1 mutations in clinical practice? Breast Care. (2017) 12:309–13. doi: 10.1159/000481428
3. Castellano I, Chiusa L, Vandone AM, Beatrice S, Goia M, Donadio M, et al. A simple and reproducible prognostic index in luminal ER-positive breast cancers. Ann Oncol. (2013) 24:2292–7. doi: 10.1093/annonc/mdt183
4. Chen T, Liu Z, Zeng W, Huang T. Down-regulation of long non-coding rna hotair sensitizes breast cancer to trastuzumab. Sci Rep. (2019) 9:19881. doi: 10.1038/s41598-019-53699-w
5. Centonze M, Saponaro C, Mangia A. Nherf1 between promises and hopes: overview on cancer and prospective openings. Transl Oncol. (2018) 11:374–90. doi: 10.1016/j.tranon.2018.01.006
6. Hsu KW, Huang CY, Tam KW, Lin CY, Huang LC, Lin CL, et al. The application of non-invasive apoptosis detection sensor (niads) on histone deacetylation inhibitor (hdaci)-induced breast cancer cell death. Int J Mol Sci. (2018) 19:452. doi: 10.3390/ijms19020452
7. Lin NU, Vanderplas A, Hughes ME, Theriault RL, Edge SB, Wong YN, et al. Clinicopathologic features, patterns of recurrence, and survival among women with triple-negative breast cancer in the national comprehensive cancer network. Cancer. (2012) 118:5463–72. doi: 10.1002/cncr.27581
8. Murria R, Palanca S, de Juan I, Alenda C, Egoavil C, Segui FJ, et al. Immunohistochemical, genetic and epigenetic profiles of hereditary and triple negative breast cancers relevance in personalized medicine. Am J Cancer Res. (2015) 5:2330–43.
9. Huang R, Han J, Liang X, Sun S, Jiang Y, Xia B, et al. Androgen receptor expression and bicalutamide antagonize androgen receptor inhibit beta-catenin transcription complex in estrogen receptor-negative breast cancer. Cell Physiol Biochem. (2017) 43:2212–25. doi: 10.1159/000484300
10. Collins LC, Cole KS, Marotti JD, Hu R, Schnitt SJ, Tamimi RM. Androgen receptor expression in breast cancer in relation to molecular phenotype: results from the nurses' health study. Mod Pathol. (2011) 24:924–31. doi: 10.1038/modpathol.2011.54
11. Hickey TE, Robinson JL, Carroll JS, Tilley WD. Minireview: the androgen receptor in breast tissues: growth inhibitor, tumor suppressor, oncogene? Mol Endocrinol. (2012) 26:1252–67. doi: 10.1210/me.2012-1107
12. Salvi S, Bonafe M, Bravaccini S. Androgen receptor in breast cancer: a wolf in sheep's clothing? A lesson from prostate cancer. Semin Cancer Biol. (2020) 60:132–7. doi: 10.1016/j.semcancer.2019.04.002
13. Claessens F, Denayer S, Van Tilborgh N, Kerkhofs S, Helsen C, Haelens A. Diverse roles of androgen receptor (AR) domains in ar-mediated signaling. Nucl Recept Signal. (2008) 6:e008. doi: 10.1621/nrs.06008
14. Gerratana L, Basile D, Buono G, De Placido S, Giuliano M, Minichillo S, et al. Androgen receptor in triple negative breast cancer: a potential target for the targetless subtype. Cancer Treat Rev. (2018) 68:102–10. doi: 10.1016/j.ctrv.2018.06.005
15. Vasiliou SK, Diamandis EP. Androgen receptor: a promising therapeutic target in breast cancer. Crit Rev Clin Lab Sci. (2019) 56:200–23. doi: 10.1080/10408363.2019.1575643
16. Suzuki T, Miki Y, Takagi K, Hirakawa H, Moriya T, Ohuchi N, et al. Androgens in human breast carcinoma. Med Mol Morphol. (2010) 43:75–81. doi: 10.1007/s00795-010-0494-3
17. Christopoulos PF, Vlachogiannis NI, Vogkou CT, Koutsilieris M. The role of the androgen receptor signaling in breast malignancies. Anticancer Res. (2017) 37:6533–40. doi: 10.21873/anticanres.12109
18. Venema CM, Bense RD, Steenbruggen TG, Nienhuis HH, Qiu SQ, van Kruchten M, et al. Consideration of breast cancer subtype in targeting the androgen receptor. Pharmacol Ther. (2019) 200:135–47. doi: 10.1016/j.pharmthera.2019.05.005
19. Tan MH, Li J, Xu HE, Melcher K, Yong EL. Androgen receptor: structure, role in prostate cancer and drug discovery. Acta Pharmacol Sin. (2015) 36:3–23. doi: 10.1038/aps.2014.18
20. Naderi A, Meyer M, Dowhan DH. Cross-regulation between FOXA1 and ErbB2 signaling in estrogen receptor-negative breast cancer. Neoplasia. (2012) 14:283–96. doi: 10.1593/neo.12294
21. Sarker D, Reid AH, Yap TA, de Bono JS. Targeting the PI3K/AKT pathway for the treatment of prostate cancer. Clin Cancer Res. (2009) 15:4799–805. doi: 10.1158/1078-0432.CCR-08-0125
22. Yang F, Shen Y, Zhang W, Jin J, Huang D, Fang H, et al. An androgen receptor negatively induced long non-coding rna arnila binding to mir-204 promotes the invasion and metastasis of triple-negative breast cancer. Cell Death Differ. (2018) 25:2209–20. doi: 10.1038/s41418-018-0123-6
23. Peters AA, Buchanan G, Ricciardelli C, Bianco-Miotto T, Centenera MM, Harris JM, et al. Androgen receptor inhibits estrogen receptor-alpha activity and is prognostic in breast cancer. Cancer Res. (2009) 69:6131–40. doi: 10.1158/0008-5472.CAN-09-0452
24. Chia KM, Liu J, Francis GD, Naderi A. A feedback loop between androgen receptor and ERK signaling in estrogen receptor-negative breast cancer. Neoplasia. (2011) 13:154–66. doi: 10.1593/neo.101324
25. Shen Y, Yang F, Zhang W, Song W, Liu Y, Guan X. The androgen receptor promotes cellular proliferation by suppression of g-protein coupled estrogen receptor signaling in triple-negative breast cancer. Cell Physiol Biochem. (2017). 43:2047–61. doi: 10.1159/000484187
26. Need EF, Selth LA, Harris TJ, Birrell SN, Tilley WD, Buchanan G. Research resource: interplay between the genomic and transcriptional networks of androgen receptor and estrogen receptor alpha in luminal breast cancer cells. Mol Endocrinol. (2012) 26:1941–52. doi: 10.1210/me.2011-1314
27. Rahim B, O'Regan R. AR signaling in breast cancer. Cancers. (2017) 9:21. doi: 10.3390/cancers9030021
28. McNamara KM, Moore NL, Hickey TE, Sasano H, Tilley WD. Complexities of androgen receptor signalling in breast cancer. Endocr Relat Cancer. (2014) 21:T161–81. doi: 10.1530/ERC-14-0243
29. Ni M, Chen Y, Lim E, Wimberly H, Bailey ST, Imai Y, et al. Targeting androgen receptor in estrogen receptor-negative breast cancer. Cancer Cell. (2011) 20:119–31. doi: 10.1016/j.ccr.2011.05.026
30. Costa RLB, Han HS, Gradishar WJ. Targeting the PI3K/AKT/mTOR pathway in triple-negative breast cancer: a review. Breast Cancer Res Treat. (2018) 169:397–406. doi: 10.1007/s10549-018-4697-y
31. Lehmann BD, Bauer JA, Schafer JM, Pendleton CS, Tang L, Johnson KC, et al. Pik3ca mutations in androgen receptor-positive triple negative breast cancer confer sensitivity to the combination of PI3K and androgen receptor inhibitors. Breast Cancer Res. (2014) 16:406. doi: 10.1186/s13058-014-0406-x
32. Wang Y, Romigh T, He X, Tan MH, Orloff MS, Silverman RH, et al. Differential regulation of pten expression by androgen receptor in prostate and breast cancers. Oncogene. (2011) 30:4327–38. doi: 10.1038/onc.2011.144
33. Wang J, Zhang C, Chen K, Tang H, Tang J, Song C, et al. Erbeta1 inversely correlates with PTEN/PI3K/AKT pathway and predicts a favorable prognosis in triple-negative breast cancer. Breast Cancer Res Treat. (2015) 152:255–69. doi: 10.1007/s10549-015-3467-3
34. Anestis A, Sarantis P, Theocharis S, Zoi I, Tryfonopoulos D, Korogiannos A, et al. Estrogen receptor beta increases sensitivity to enzalutamide in androgen receptor-positive triple-negative breast cancer. J Cancer Res Clin Oncol. (2019) 145:1221–33. doi: 10.1007/s00432-019-02872-9
35. Karamouzis MV, Papavassiliou KA, Adamopoulos C, Papavassiliou AG. Targeting androgen/estrogen receptors crosstalk in cancer. Trends Cancer. (2016) 2:35–48. doi: 10.1016/j.trecan.2015.12.001
36. Wang C, Lv X, He C, Hua G, Tsai MY, Davis JS. The g-protein-coupled estrogen receptor agonist g-1 suppresses proliferation of ovarian cancer cells by blocking tubulin polymerization. Cell Death Dis. (2013) 4:e869. doi: 10.1038/cddis.2013.397
37. Chia K, O'Brien M, Brown M, Lim E. Targeting the androgen receptor in breast cancer. Curr Oncol Rep. (2015) 17:4. doi: 10.1007/s11912-014-0427-8
38. Niemeier LA, Dabbs DJ, Beriwal S, Striebel JM, Bhargava R. Androgen receptor in breast cancer: expression in estrogen receptor-positive tumors and in estrogen receptor-negative tumors with apocrine differentiation. Mod Pathol. (2010) 23:205–12. doi: 10.1038/modpathol.2009.159
39. Park S, Koo J, Park HS, Kim JH, Choi SY, Lee JH, et al. Expression of androgen receptors in primary breast cancer. Ann Oncol. (2010) 21:488–92. doi: 10.1093/annonc/mdp510
40. Micello D, Marando A, Sahnane N, Riva C, Capella C, Sessa F. Androgen receptor is frequently expressed in HER2-positive, ER/PR-negative breast cancers. Virchows Arch. (2010) 457:467–76. doi: 10.1007/s00428-010-0964-y
41. Safarpour D, Tavassoli FA. A targetable androgen receptor-positive breast cancer subtype hidden among the triple-negative cancers. Arch Pathol Lab Med. (2015) 139:612–7. doi: 10.5858/arpa.2014-0122-RA
42. Bravaccini S, Ravaioli S, Amadori D, Scarpi E, Puccetti M, Rocca A, et al. Are there differences in androgen receptor expression in invasive breast cancer in African (Tanzanian) population in comparison with the Caucasian (Italian) population? Front Endocrinol. (2018) 9:137. doi: 10.3389/fendo.2018.00137
43. Kensler KH, Poole EM, Heng YJ, Collins LC, Glass B, Beck AH, et al. Androgen receptor expression and breast cancer survival: results from the nurses' health studies. J Natl Cancer Inst. (2018) 111:700–8. doi: 10.1093/jnci/djy173
44. Bozovic-Spasojevic I, Zardavas D, Brohee S, Ameye L, Fumagalli D, Ades F, et al. The prognostic role of androgen receptor in patients with early-stage breast cancer: a meta-analysis of clinical and gene expression data. Clin Cancer Res. (2017) 23:2702–12. doi: 10.1158/1078-0432.CCR-16-0979
45. Okano M, Oshi M, Butash AL, Asaoka M, Katsuta E, Peng X, et al. Estrogen receptor positive breast cancer with high expression of androgen receptor has less cytolytic activity and worse response to neoadjuvant chemotherapy but better survival. Int J Mol Sci. (2019) 20:2655. doi: 10.3390/ijms20112655
46. Kensler KH, Regan MM, Heng YJ, Baker GM, Pyle ME, Schnitt SJ, et al. Prognostic and predictive value of androgen receptor expression in postmenopausal women with estrogen receptor-positive breast cancer: results from the breast international group trial 1-98. Breast Cancer Res. (2019) 21:30. doi: 10.1186/s13058-019-1118-z
47. Cochrane DR, Bernales S, Jacobsen BM, Cittelly DM, Howe EN, D'Amato NC, et al. Role of the androgen receptor in breast cancer and preclinical analysis of enzalutamide. Breast Cancer Res. (2014) 16:R7. doi: 10.1186/bcr3599
48. Wang X, Bi X, Huang Z, Huang J, Xia W, Shi W, et al. The prognostic value of AR in HER2-enriched metastatic breast cancer. Endocr Relat Cancer. (2020) 27:199–208. doi: 10.1530/ERC-19-0315
49. Kucukzeybek BB, Bayoglu IV, Kucukzeybek Y, Yildiz Y, Oflazoglu U, Atahan MK, et al. Prognostic significance of androgen receptor expression in HER2-positive and triple-negative breast cancer. Pol J Pathol. (2018) 69:157–68. doi: 10.5114/pjp.2018.76699
50. Asano Y, Kashiwagi S, Goto W, Tanaka S, Morisaki T, Takashima T, et al. Expression and clinical significance of androgen receptor in triple-negative breast cancer. Cancers. (2017) 9:4. doi: 10.3390/cancers9010004
51. Hilborn E, Gacic J, Fornander T, Nordenskjold B, Stal O, Jansson A. Androgen receptor expression predicts beneficial tamoxifen response in oestrogen receptor-alpha-negative breast cancer. Br J Cancer. (2016) 114:248–55. doi: 10.1038/bjc.2015.464
52. Xu M, Yuan Y, Yan P, Jiang J, Ma M, Niu X, et al. Prognostic significance of androgen receptor expression in triple negative breast cancer: a systematic review and meta-analysis. Clin Breast Cancer. (2020) 20:e385–96. doi: 10.1016/j.clbc.2020.01.002
53. Speers C, Zhao SG, Chandler B, Liu M, Wilder-Romans K, Olsen E, et al. Androgen receptor as a mediator and biomarker of radioresistance in triple-negative breast cancer. NPJ Breast Cancer. (2017) 3:29. doi: 10.1038/s41523-017-0038-2
54. Loibl S, Muller BM, von Minckwitz G, Schwabe M, Roller M, Darb-Esfahani S, et al. Androgen receptor expression in primary breast cancer and its predictive and prognostic value in patients treated with neoadjuvant chemotherapy. Breast Cancer Res Treat. (2011) 130:477–87. doi: 10.1007/s10549-011-1715-8
55. Bhattarai S, Klimov S, Mittal K, Krishnamurti U, Li XB, Oprea-Ilies G, et al. Prognostic role of androgen receptor in triple negative breast cancer: a multi-institutional study. Cancers. (2019) 11:995. doi: 10.3390/cancers11070995
56. Elebro K, Borgquist S, Simonsson M, Markkula A, Jirstrom K, Ingvar C, et al. Combined androgen and estrogen receptor status in breast cancer: treatment prediction and prognosis in a population-based prospective cohort. Clin Cancer Res. (2015) 21:3640–50. doi: 10.1158/1078-0432.CCR-14-2564
57. Hu R, Dawood S, Holmes MD, Collins LC, Schnitt SJ, Cole K, et al. Androgen receptor expression and breast cancer survival in postmenopausal women. Clin Cancer Res. (2011) 17:1867–74. doi: 10.1158/1078-0432.CCR-10-2021
58. Witzel I, Graeser M, Karn T, Schmidt M, Wirtz R, Schutze D, et al. Androgen receptor expression is a predictive marker in chemotherapy-treated patients with endocrine receptor-positive primary breast cancers. J Cancer Res Clin Oncol. (2013) 139:809–16. doi: 10.1007/s00432-013-1382-8
59. Aleskandarany MA, Abduljabbar R, Ashankyty I, Elmouna A, Jerjees D, Ali S, et al. Prognostic significance of androgen receptor expression in invasive breast cancer: transcriptomic and protein expression analysis. Breast Cancer Res Treat. (2016) 159:215–27. doi: 10.1007/s10549-016-3934-5
60. Guo W, Wang W, Zhu Y, Zhu X, Shi Z, Wang Y. HER2 status in molecular apocrine breast cancer: associations with clinical, pathological, molecular features. Int J Clin Exp Pathol. (2015) 8:8008–17.
61. Lin Fde M, Pincerato KM, Bacchi CE, Baracat EC, Carvalho FM. Coordinated expression of oestrogen and androgen receptors in HER2-positive breast carcinomas: impact on proliferative activity. J Clin Pathol. (2012) 65:64–8. doi: 10.1136/jclinpath-2011-200318
62. Christenson JL, Butterfield KT, Spoelstra NS, Norris JD, Josan JS, Pollock JA, et al. Mmtv-pymt and derived met-1 mouse mammary tumor cells as models for studying the role of the androgen receptor in triple-negative breast cancer progression. Horm Cancer. (2017) 8:69–77. doi: 10.1007/s12672-017-0285-6
63. Santonja A, Sanchez-Munoz A, Lluch A, Chica-Parrado MR, Albanell J, Chacon JI, et al. Triple negative breast cancer subtypes and pathologic complete response rate to neoadjuvant chemotherapy. Oncotarget. (2018) 9:26406–16. doi: 10.18632/oncotarget.25413
64. Masuda H, Baggerly KA, Wang Y, Zhang Y, Gonzalez-Angulo AM, Meric-Bernstam F, et al. Differential response to neoadjuvant chemotherapy among 7 triple-negative breast cancer molecular subtypes. Clin Cancer Res. (2013) 19:5533–40. doi: 10.1158/1078-0432.CCR-13-0799
65. Rampurwala M, Wisinski KB, O'Regan R. Role of the androgen receptor in triple-negative breast cancer. Clin Adv Hematol Oncol. (2016) 14:186–93.
66. Basile D, Cinausero M, Iacono D, Pelizzari G, Bonotto M, Vitale MG, et al. Androgen receptor in estrogen receptor positive breast cancer: beyond expression. Cancer Treat Rev. (2017) 61:15–22. doi: 10.1016/j.ctrv.2017.09.006
67. Kono M, Fujii T, Lyons GR, Huo L, Bassett R, Gong Y, et al. Impact of androgen receptor expression in fluoxymesterone-treated estrogen receptor-positive metastatic breast cancer refractory to contemporary hormonal therapy. Breast Cancer Res Treat. (2016) 160:101–9. doi: 10.1007/s10549-016-3986-6
68. Coss CC, Jones A, Dalton JT. Selective androgen receptor modulators as improved androgen therapy for advanced breast cancer. Steroids. (2014) 90:94–100. doi: 10.1016/j.steroids.2014.06.010
69. Vontela N, Koduri V, Schwartzberg LS, Vidal GA. Selective androgen receptor modulator in a patient with hormone-positive metastatic breast cancer. J Natl Compr Canc Netw. (2017) 15:284–7. doi: 10.6004/jnccn.2017.0029
70. Masiello D, Cheng S, Bubley GJ, Lu ML, Balk SP. Bicalutamide functions as an androgen receptor antagonist by assembly of a transcriptionally inactive receptor. J Biol Chem. (2002) 277:26321–6. doi: 10.1074/jbc.M203310200
71. Akaza H, Hinotsu S, Usami M, Arai Y, Kanetake H, Naito S, et al. Combined androgen blockade with bicalutamide for advanced prostate cancer: long-term follow-up of a phase 3, double-blind, randomized study for survival. Cancer. (2009) 115:3437–45. doi: 10.1002/cncr.24395
72. Scher HI, Fizazi K, Saad F, Taplin ME, Sternberg CN, Miller K, et al. Increased survival with enzalutamide in prostate cancer after chemotherapy. N Engl J Med. (2012) 367:1187–97. doi: 10.1056/NEJMoa1207506
73. Tran C, Ouk S, Clegg NJ, Chen Y, Watson PA, Arora V, et al. Development of a second-generation antiandrogen for treatment of advanced prostate cancer. Science. (2009) 324:787–90. doi: 10.1126/science.1168175
74. Farrow JM, Yang JC, Evans CP. Autophagy as a modulator and target in prostate cancer. Nat Rev Urol. (2014) 11:508–16. doi: 10.1038/nrurol.2014.196
75. Loriot Y, Miller K, Sternberg CN, Fizazi K, De Bono JS, Chowdhury S, et al. Effect of enzalutamide on health-related quality of life, pain, and skeletal-related events in asymptomatic and minimally symptomatic, chemotherapy-naive patients with metastatic castration-resistant prostate cancer (prevail): results from a randomised, phase 3 trial. Lancet Oncol. (2015) 16:509–21. doi: 10.1016/S1470-2045(15)70113-0
76. Moilanen AM, Riikonen R, Oksala R, Ravanti L, Aho E, Wohlfahrt G, et al. Discovery of odm-201, a new-generation androgen receptor inhibitor targeting resistance mechanisms to androgen signaling-directed prostate cancer therapies. Sci Rep. (2015) 5:12007. doi: 10.1038/srep12007
77. Toren PJ, Kim S, Pham S, Mangalji A, Adomat H, Guns ES, et al. Anticancer activity of a novel selective CYP17a1 inhibitor in preclinical models of castrate-resistant prostate cancer. Mol Cancer Ther. (2015) 14:59–69. doi: 10.1158/1535-7163.MCT-14-0521
78. Zhu A, Li Y, Song W, Xu Y, Yang F, Zhang W, et al. Antiproliferative effect of androgen receptor inhibition in mesenchymal stem-like triple-negative breast cancer. Cell Physiol Biochem. (2016) 38:1003–14. doi: 10.1159/000443052
79. Gucalp A, Tolaney S, Isakoff SJ, Ingle JN, Liu MC, Carey LA, et al. Phase ii trial of bicalutamide in patients with androgen receptor-positive, estrogen receptor-negative metastatic breast cancer. Clin Cancer Res. (2013) 19:5505–12. doi: 10.1158/1078-0432.CCR-12-3327
80. Caiazza F, Murray A, Madden SF, Synnott NC, Ryan EJ, O'Donovan N, et al. Preclinical evaluation of the ar inhibitor enzalutamide in triple-negative breast cancer cells. Endocr Relat Cancer. (2016) 23:323–34. doi: 10.1530/ERC-16-0068
81. Barton VN, D'Amato NC, Gordon MA, Lind HT, Spoelstra NS, Babbs BL, et al. Multiple molecular subtypes of triple-negative breast cancer critically rely on androgen receptor and respond to enzalutamide in vivo. Mol Cancer Ther. (2015) 14:769–78. doi: 10.1158/1535-7163.MCT-14-0926
82. Traina TA, Miller K, Yardley DA, Eakle J, Schwartzberg LS, O'Shaughnessy J, et al. Enzalutamide for the treatment of androgen receptor-expressing triple-negative breast cancer. J Clin Oncol. (2018) 36:884–90. doi: 10.1200/JCO.2016.71.3495
83. Bonnefoi H, Levy C, MacGrogan G, Grellety T, Asselain B, Pulido M, et al. Start: a randomized phase ii study in patients with triple negative, androgen receptor positive locally recurrent (unresectable) or metastatic breast cancer treated with darolutamide or capecitabine (ucbg-306). Ann Oncol. (2018) 29(Suppl. 8):viii120. doi: 10.1093/annonc/mdy272.356
84. Bonnefoi H, Grellety T, Tredan O, Saghatchian M, Dalenc F, Mailliez A, et al. A phase ii trial of abiraterone acetate plus prednisone in patients with triple-negative androgen receptor positive locally advanced or metastatic breast cancer (ucbg 12-1). Ann Oncol. (2016) 27:812–8. doi: 10.1093/annonc/mdw067
85. Gordon MA, D'Amato NC, Gu H, Babbs B, Wulfkuhle J, Petricoin EF, et al. Synergy between androgen receptor antagonism and inhibition of mtor and HER2 in breast cancer. Mol Cancer Ther. (2017) 16:1389–400. doi: 10.1158/1535-7163.MCT-17-0111
86. Lehmann BD, Abramson VG, Sanders M, Mayer EL, Haddad T, Nanda R, et al. Tbcrc 032 Ib/II multicenter study: molecular insights to AR antagonist and PI3K inhibitor efficacy in patients with ar+ metastatic triple-negative breast cancer. Clin Cancer Res. (2019) 26:2111–23. doi: 10.1158/1078-0432.CCR-19-2170
87. Asghar U, Witkiewicz AK, Turner NC, Knudsen ES. The history and future of targeting cyclin-dependent kinases in cancer therapy. Nat Rev Drug Discov. (2015) 14:130–46. doi: 10.1038/nrd4504
88. Liu CY, Lau KY, Hsu CC, Chen JL, Lee CH, Huang TT, et al. Combination of palbociclib with enzalutamide shows in vitro activity in RB proficient and androgen receptor positive triple negative breast cancer cells. PLoS ONE. (2017) 12:e0189007. doi: 10.1371/journal.pone.0189007
89. Bishop JL, Sio A, Angeles A, Roberts ME, Azad AA, Chi KN, et al. Pd-l1 is highly expressed in enzalutamide resistant prostate cancer. Oncotarget. (2015) 6:234–42. doi: 10.18632/oncotarget.2703
90. Barton VN, Christenson JL, Gordon MA, Greene LI, Rogers TJ, Butterfield K, et al. Androgen receptor supports an anchorage-independent, cancer stem cell-like population in triple-negative breast cancer. Cancer Res. (2017) 77:3455–66. doi: 10.1158/0008-5472.CAN-16-3240
91. Friedlander TW, Roy R, Tomlins SA, Ngo VT, Kobayashi Y, Azameera A, et al. Common structural and epigenetic changes in the genome of castration-resistant prostate cancer. Cancer Res. (2012) 72:616–25. doi: 10.1158/0008-5472.CAN-11-2079
92. Watson PA, Chen YF, Balbas MD, Wongvipat J, Socci ND, Viale A, et al. Constitutively active androgen receptor splice variants expressed in castration-resistant prostate cancer require full-length androgen receptor. Proc Natl Acad Sci USA. (2010) 107:16759–65. doi: 10.1073/pnas.1012443107
93. Scher HI, Beer TM, Higano CS, Anand A, Taplin ME, Efstathiou E, et al. Antitumour activity of mdv3100 in castration-resistant prostate cancer: a phase 1-2 study. Lancet. (2010) 375:1437–46. doi: 10.1016/S0140-6736(10)60172-9
94. Waltering KK, Urbanucci A, Visakorpi T. Androgen receptor (AR) aberrations in castration-resistant prostate cancer. Mol Cell Endocrinol. (2012) 360:38–43. doi: 10.1016/j.mce.2011.12.019
95. Grasso CS, Wu YM, Robinson DR, Cao X, Dhanasekaran SM, Khan AP, et al. The mutational landscape of lethal castration-resistant prostate cancer. Nature. (2012) 487:239–43. doi: 10.1038/nature11125
96. Li Y, Hwang TH, Oseth LA, Hauge A, Vessella RL, Schmechel SC, et al. Ar intragenic deletions linked to androgen receptor splice variant expression and activity in models of prostate cancer progression. Oncogene. (2012) 31:4759–67. doi: 10.1038/onc.2011.637
97. Chen CD, Welsbie DS, Tran C, Baek SH, Chen R, Vessella R, et al. Molecular determinants of resistance to antiandrogen therapy. Nat Med. (2004) 10:33–9. doi: 10.1038/nm972
98. Gregory CW, He B, Johnson RT, Ford OH, Mohler JL, French FS, et al. A mechanism for androgen receptor-mediated prostate cancer recurrence after androgen deprivation therapy. Cancer Res. (2001) 61:4315–9.
99. Balbas MD, Evans MJ, Hosfield DJ, Wongvipat J, Arora VK, Watson PA, et al. Overcoming mutation-based resistance to antiandrogens with rational drug design. eLife. (2013) 2:e00499. doi: 10.7554/eLife.00499
100. Korpal M, Korn JM, Gao X, Rakiec DP, Ruddy DA, Doshi S, et al. An f876l mutation in androgen receptor confers genetic and phenotypic resistance to mdv3100 (enzalutamide). Cancer Discov. (2013) 3:1030–43. doi: 10.1158/2159-8290.CD-13-0142
101. Hara T, Miyazaki J, Araki H, Yamaoka M, Kanzaki N, Kusaka M, et al. Novel mutations of androgen receptor: a possible mechanism of bicalutamide withdrawal syndrome. Cancer Res. (2003) 63:149–53.
102. Hickey TE, Irvine CM, Dvinge H, Tarulli GA, Hanson AR, Ryan NK, et al. Expression of androgen receptor splice variants in clinical breast cancers. Oncotarget. (2015) 6:44728–44. doi: 10.18632/oncotarget.6296
103. Antonarakis ES, Lu C, Wang H, Luber B, Nakazawa M, Roeser JC, et al. Ar-v7 and resistance to enzalutamide and abiraterone in prostate cancer. N Engl J Med. (2014) 371:1028–38. doi: 10.1056/NEJMoa1315815
104. Kohli M, Ho Y, Hillman DW, Van Etten JL, Henzler C, Yang R, et al. Androgen receptor variant AR-v9 is coexpressed with AR-v7 in prostate cancer metastases and predicts abiraterone resistance. Clin Cancer Res. (2017) 23:4704–15. doi: 10.1158/1078-0432.CCR-17-0017
105. Zhang Z, Cheng L, Li J, Farah E, Atallah NM, Pascuzzi PE, et al. Inhibition of the wnt/β-catenin pathway overcomes resistance to enzalutamide in castration-resistant prostate cancer. Cancer Res. (2018) 78:3147–62. doi: 10.1158/0008-5472.CAN-17-3006
106. Tucci M, Zichi C, Buttigliero C, Vignani F, Scagliotti GV, Di Maio M. Enzalutamide-resistant castration-resistant prostate cancer: challenges and solutions. OncoTargets Ther. (2018) 11:7353–68. doi: 10.2147/OTT.S153764
107. Chen X, Liu J, Cheng L, Li C, Zhang Z, Bai Y, et al. Inhibition of noncanonical wnt pathway overcomes enzalutamide resistance in castration-resistant prostate cancer. Prostate. (2020) 80:256–66. doi: 10.1002/pros.23939
108. Feng J, Li L, Zhang N, Liu J, Zhang L, Gao H, et al. Androgen and AR contribute to breast cancer development and metastasis: an insight of mechanisms. Oncogene. (2017) 36:2775–90. doi: 10.1038/onc.2016.432
109. Bronte G, Rocca A, Ravaioli S, Puccetti M, Tumedei MM, Scarpi E, et al. Androgen receptor in advanced breast cancer: is it useful to predict the efficacy of anti-estrogen therapy? BMC Cancer. (2018) 18:348. doi: 10.1186/s12885-018-4239-3
110. Baccelli I, Schneeweiss A, Riethdorf S, Stenzinger A, Schillert A, Vogel V, et al. Identification of a population of blood circulating tumor cells from breast cancer patients that initiates metastasis in a xenograft assay. Nat Biotechnol. (2013) 31:539–44. doi: 10.1038/nbt.2576
111. de Kruijff IE, Sieuwerts AM, Onstenk W, Jager A, Hamberg P, de Jongh FE, et al. Androgen receptor expression in circulating tumor cells of patients with metastatic breast cancer. Int J Cancer. (2019) 145:1083–9. doi: 10.1002/ijc.32209
112. Prekovic S, Van den Broeck T, Moris L, Smeets E, Claessens F, Joniau S, et al. Treatment-induced changes in the androgen receptor axis: liquid biopsies as diagnostic/prognostic tools for prostate cancer. Mol Cell Endocrinol. (2018) 462:56–63. doi: 10.1016/j.mce.2017.08.020
Keywords: breast cancer, androgen receptor, prognosis, targeted therapy, drug resistance
Citation: Chen M, Yang Y, Xu K, Li L, Huang J and Qiu F (2020) Androgen Receptor in Breast Cancer: From Bench to Bedside. Front. Endocrinol. 11:573. doi: 10.3389/fendo.2020.00573
Received: 03 May 2020; Accepted: 14 July 2020;
Published: 02 September 2020.
Edited by:
Anna Tesei, Romagnolo Scientific Institute for the Study and Treatment of Tumors (IRCCS), ItalyCopyright © 2020 Chen, Yang, Xu, Li, Huang and Qiu. This is an open-access article distributed under the terms of the Creative Commons Attribution License (CC BY). The use, distribution or reproduction in other forums is permitted, provided the original author(s) and the copyright owner(s) are credited and that the original publication in this journal is cited, in accordance with accepted academic practice. No use, distribution or reproduction is permitted which does not comply with these terms.
*Correspondence: Fuming Qiu, cWl1ZnVtaW5nQHpqdS5lZHUuY24=
†These authors have contributed equally to this work